- 1Guangdong Provincial Key Laboratory of Medical Molecular Diagnostics, The First Dongguan Affiliated Hospital, Guangdong Medical University, Dongguan, China
- 2Institute of Laboratory Medicine, School of Medical Technology, Guangdong Medical University, Dongguan, China
- 3Dongguan Key Laboratory of Environmental Medicine, School of Public Health, Guangdong Medical University, Dongguan, China
Autophagy, one of the major intracellular degradation systems, plays an important role in maintaining normal cellular physiological functions and protecting organisms from different diseases. Selenium (Se), an essential trace element, is involved in many metabolic regulatory signaling events and plays a key role in human health. In recent years, selenium nanoparticles (Se NPs) have attracted increasing attentions in biomedical field due to their low toxicity, high bioavailability and high bioactivity. Taking the advantage of their advanced biological activities, Se NPs can be used alone as potential therapeutic agents, or combine with other agents and served as carriers for the development of novel therapeutics. More interestingly, Se NPs have been widely reported to affect autophagy signaling, which therefor allow Se NPs to be used as potential therapeutic agents against different diseases. Here, this review suggested the relationships between Se and autophagy, followed by the trends and recent progresses of Se NPs for autophagy regulation in different diseased conditions. More importantly, this work discussed the roles and potential mechanisms of Se NPs in autophagy regulating, which might enhance our understanding about how Se NPs regulate autophagy for potential disease treatment. This work is expected to promote the potential application of Se NPs as novel autophagy regulators, which might benefit the development of novel autophagy associated therapeutics.
Introduction
Autophagy, an essential and conserved catabolic process, is one of the major intracellular degradation systems that delivers cytoplasmic or invasive materials into autolysosomes for degradation (1). Although there are three typical classes of autophagy (macroautophagy, microautophagy, and chaperone-mediated autophagy), macroautophagy is thought to be the major type of autophagy and is always referred to as “autophagy” (1). Autophagy begins with an isolation membrane, also known as a phagophore to sequester the cargos, such as proteins, organelles, ribosomes and pathogens, in a double-membrane autophagosome (2). Autophagosome matures through fusion with the lysosome to form autolysosomes, which promotes the degradation of the cargos. Lysosomal permeases and transporters could export amino acids and other by-products of degradation back out to the cytoplasm, where they can be re-used for building macromolecules and for the metabolic uses (2). Thus, autophagy is considered to be a cellular recycling factor that promotes energy efficiency and re-uses the non-functional proteins and organelles (3). Dysfunction of autophagy could induce ubiquitination inhibition, reactive oxygen species (ROS) accumulation, mitochondrial disruption and genomic instability, thus leading to lots of diseased conditions, such as cancer, neurodegeneration, infection, and aging (4, 5). And increasing evidences are suggesting that autophagy can serve an adaptive role to protect organisms against different pathologies, which therefore highlights autophagy as a critical signaling event for novel therapeutic strategy development (6, 7).
Selenium (Se), an essential trace element, has been linked to many health benefits in humans and other mammals, such as decreasing the incidence of cancer (8), protecting against cardiovascular diseases (9), treating particular muscle disorders (10), delaying the onset of AIDS in HIV-positive patients and boosting immune function in mammalian development (11, 12). The biological functions of the micronutrient selenium are mediated in large part by selenoproteins, which contain selenocysteine (Sec) in their active site. Glutathione peroxidase 1 (GPx1), the most abundant selenoprotein in mammals, has been considered as one of the major antioxidant enzymes to protect cells from oxidative damage by degrading toxic H2O2 (13). The iodothyronine deiodinase family of selenoproteins is involved in the regulation of thyroid hormone activity by reductive deiodination (14, 15). Another kind of selenoproteins-thioredoxin reductases (TRs) are involved in the control of antioxidant defense and regulation of transcription factors (15), which therefore play critical roles in cancer development and treatment (16, 17). Selenophosphate synthetase is a selenoenzyme that catalyzes the ATP-dependent synthesis of selenophosphate (12). Methionine-R-sulfoxide Reductase B1 (MsrB1), a zinc-containing selenoprotein, can reduce oxidized methionine residues to repair oxidatively damaged proteins (18). These important functions of selenoproteins, especially for their regulation effects in cellular oxidative stress, highlight the important physiological roles of Se in human health and diseased conditions.
Cell death is an irreversible cessation of life phenomena and the end of life. According to the definition of morphological criteria, cell death is always divided into apoptosis, necrosis, autophagy, ferroptosis, and cell scorching, etc. (19). Se is an essential trace element that also plays crucial role in cell death. Selenium deficiency has been widely reported to result in the dysfunction of cellular metabolism, which would lead to different modes of cell death (20, 21). Selenoproteins have also been proved to regulate autophagy in different conditions, which therefore can be served as a kind of protective agent to inhibit dysfunctioned autophagy (22, 23). Moreover, selenium compounds showed strong ability to promote cell autophagy of infected cells to kill the intracellular pathogens (24, 25), which indicated the potential of selenium compounds to serve as anti-infectious agents by promoting autophagy. Selenium compounds also possessed chemotherapeutic effects against multiple malignant cancers by regulating autophagy (26), which highlighted selenium compounds as a new generation of anticancer agents by regulating autophagy.
Nanotechnology has been developed rapidly in the past few decades, which brings novel possibilities for disease treatment. Selenium nanoparticles (Se NPs) have attracted extensive attentions for the application of selenium-based products due to their advantages, including higher safety, lower toxicity, higher bioavailability and stronger free radical scavenging ability and antioxidant activities compared to selenium element (27–30). Zhang et al. (31) reported that encapsulation of selenium in chitosan nanoparticles could improve selenium availability and protect cells from selenium-induced DNA damage response, which indicated the higher safety, lower toxicity, higher bioavailability of Se NPs compared the organic and inorganic selenium compounds. Chen et al. (30) demonstrated the higher bioavailability of Se NPs, and moreover, they also found that Se NPs showed stronger antioxidant activities compared with selenium compounds. Additionally, Se NPs have also been reported to show similar or enhanced activities against diseased cells than Se compounds, but show reduced cytotoxicity against normal cells (32).
The above advantages thus allow Se NPs to be used as a kind of novel nanosystems or selenium products for disease treatment in different conditions. Firstly, Se NPs can also be used as carriers of drugs or other biomolecules for targeted delivery. By encapsulating drugs/biomolecules into Se NPs, the obtained nanosystem could lead to the increased stability and prolonged circulation (33), which therefore provide the enhanced efficiency with better targeting effects. And Se NPs can be easily designed to suit their needs in terms of size, surface charge, genetic, drug loading and controlled release, which is significant for the construction of novel nanomedicines (34–36). Secondly, Se NPs have excellent antibacterial activity and anti-viral activity, which therefore can be used as anti-infectious agents (37, 38). Thirdly, Se NPs also have superior antitumor activities beyond the conventional inorganic and organic selenium compounds, which allows Se NPs to kill tumor cells directly or enhance the anti-cancer activity of current chemotherapeutics (33, 39). Moreover, Se NPs have also been reported to show immunological regulation effects in immune cells (40). And the regulation of autophagy was closely associated with these biological activities for Se NPs. Increasing evidences are suggesting that Se NPs play an important role in the treatment of cancer and infectious diseases through the regulation of autophagy for more effective tumor cell killing and pathogen clearance.
Due to the critical roles of Se NPs in autophagy regulation, this review summarized the effects and mechanisms of autophagy regulated by Se and Se NPs, especially the recent progresses of Se NPs in the treatment of different diseases by regulating autophagy, which is expected to promote the development of novel therapeutics.
Association between selenium and autophagy
The trace element Se can regulate cellular autophagy through different signaling pathways, which is essential for human health. By regulating autophagy under different conditions, selenium compounds and selenoproteins exhibit important effects to induce cancer cell death, reduce drug toxicity, regulate inflammatory responses and resist pathogenic bacterial infections. Considering the critical roles of Se in autophagy regulation, we summarized the mechanisms Se regulates cellular autophagy through various signaling pathways (Figure 1).
Mammalian rapamycin (mTOR) kinase is a well-known negative central regulator of the autophagic process, and its upstream regulatory signals are phosphatidylinositol 3-kinase (PI3K)/protein kinase B (AKT) pathway, which can activate mTOR signalings. The inhibition of PI3K/AKT/mTOR pathway activates autophagy, whereas AMPK downregulates mTOR expression and promotes autophagy by inhibiting the mTOR pathway (41). Qian et al. (22) observed that SeMet could attenuate OTA-induced PCV2 replication by inhibiting autophagy through activation of the PI3K/AKT/mTOR pathway. Cheng et al. (23) also found that L-selenomethionine could inhibit ammonia-induced cardiac autophagy through activation of the PI3K/AKT/mTOR signaling pathway. Yang et al. (42) also found that selenium could inhibit autophagy by regulating the PI3K/AKT/mTOR signaling pathway to prevent ischemia/reperfusion injury-induced blood-brain barrier damage in hyperglycemic patients. It has also been shown that SeMet can upregulate AMPK expression and initiate autophagy to clear tau in 3xTg-AD mice by activating the AMPK/mTOR pathway, suggesting that SeMet might be an effective drug candidate for the treatment of Alzheimer’s disease (AD) (43). Se-Allylselenocysteine (ASC) can also activate autophagy and induce rectal cancer cell death by regulating AMPK/mTOR pathway, which facilitates the development of new therapeutic strategies against colorectal cancer (44).
Another downstream signaling pathway of AMPK is FoxO3a/GABARAPL1, and the activation of AMPK/Fo xO3a/GABARAPL1 pathway can promote cellular autophagy. Yu et al. (26) found that sodium selenite could promote ROS/AMPK/FoxO3a/GABARAPL-1-mediated autophagy to downregulate apoptosis in colon cancer cells and colon xenograft models. In addition, Pant et al. (45) also found that probiotics enriched with selenium could improve hepatic steatosis by regulating AMPK/SIRT-1 mediated autophagy to alleviate NAFLD. The transcription factor NF-κB (nuclear factor kappa B) is not only a regulator of inflammatory response, but also plays an important role in the regulation of autophagy (46). It has been shown that selenium deficiency can induce chicken French bursa autophagy through the ChTLR4/MyD88/NF-κB pathway (47), and sodium selenite can also block TLR4/NF-κB and mitochondrial signaling pathways to inhibit mitochondrial autophagy in bovine support cells exposed to microcystins leucine arginine (MC-LR) (48). Selenite can also regulate the NF-κB signaling pathway by activating the ATM/IKK alpha axis to promote autophagy in leukemic Jurkat cells (49). Moreover, selenium donors can also induce macrophage autophagy through c-Jun-mediated pathway, thereby upregulating c-Jun expression and limiting the intracellular growth of Mycobacterium tuberculosis (25).
Autophagy is an important physiological process that delivers cytoplasmic or invasive materials into autolysosomes for degradation, which is critical to maintain the normal metabolism and thus beneficial to human health. However, in some pathological conditions, abnormal regulations of autophagy may also be harmful to human health. Se has exhibited powerful protective effects on the organism through its dual-role regulation effects on autophagy. It has been shown that maternal Se supplementation during gestation increases the levels of antioxidant selenoproteins and reduces autophagy and inflammation levels in the offspring, which helps to improve the immune function of the offspring (50), whereas maternal Se deficiency induces the dysfunction of autophagy and the damages of placenta (51). Se can also mitigate the damage caused by toxic substances such as cadmium (52–54), lead (55, 56), mercury (57), and ammonia (23, 58) through the inhibition of these toxins-induced and oxidative stress-mediated autophagy. In addition, Se may also attenuate oxidative stress, and ochratoxin A-induced PCV2 replication promotion by inhibiting autophagy (22, 59), which may be beneficial in developing new ideas for the prevention of PCV2 infection. These results indicated that Se can block unhealthy autophagic pathways to protect human health. It has also been shown that Se can exert antitumor effects by promoting autophagy in different tumor cells for more effective tumor inhibition. Sodium selenite can induce autophagy not only in A549 human lung cancer cells by promoting ROS production (60), but also in colorectal cancer cells (26, 61), and these findings could help explore sodium selenite as a potential anti-cancer agent in clinical practice. In addition, Se not only limits the proliferation of Staphylococcus aureus and Mycobacterium tuberculosis by promoting macrophage autophagy (24, 25) but also alleviates the E. coli-induced inflammatory response by enhancing bovine mammary epithelial cell autophagy (62), which exhibits potent anti-infective activity. These results suggest that Se can not only block unhealthy autophagic pathways to protect human health, but can also promote healthy autophagic pathways to protect human health.
Mechanisms for Se NPs regulated autophagy in tumor
Similar with the conventional inorganic or organic selenium compounds, Se NPs can also modulate cellular autophagy through various signaling pathways. Despite being a promising strategy to inhibit cancer progression, current chemotherapy strategies for cancer are still limited due to adverse side effects and poor survival rates. Thus, the development of novel strategies, such as drug-delivery platforms with good biocompatibility that could enhance chemotherapy efficiency and reduce the side effects against cancer, remain a challenge. Wang et al. (63) developed a novel selenium-nanoparticle based drug-delivery agent for cancer treatment from Kaempferia parviflora (black ginger) root extract and selenium salts. The obtained KP-Se NPs showed significant cytotoxicity in human gastric adenocarcinoma cells (AGS cells), but showed no significant cytotoxicity in normal cells. These specific anticancer effects of KP-Se NPs were closely related to apoptosis, which was associated with the upregulation of intrinsic apoptotic signaling markers, such as Bcl-2, Bax and caspase 3 in AGS cells. Moreover, KP-Se NPs also caused autophagy of AGS by increasing the autophagic flux-marker protein, LC3B-II, whilst inhibiting autophagic cargo protein, p62. Additionally, phosphorylation of PI3K/Akt/mTOR pathway markers and downstream targets were decreased in KP-SeNP-treated AGS cells. Therefore, this work strongly suggested that KP-Se NPs could act as a novel potential therapeutic agent for GC by regulating autophagy, and also indicated that plant-based synthesis of Se NPs could be considered as one of the best strategies for cancer treatment.
Silymarin is well-known as a traditional hepatoprotective agent, but increasing evidence and clinical results have established the chemopreventive roles of silymarin as a therapeutic option for gastric cancer treatment (64, 65). However, silymarin has major limitations in clinical cancer therapy due to its poor water and lipid solubility (66). Therefore, Mi et al. (67) developed silymarin selenium nanoparticles (Si-Se NPs) by silymarin-mediated green synthesis and investigated their possibility as an anticancer agent. Compared with silymarin, the Si-Se NPs exhibited significantly increased cytotoxic effect of AGS cells without exhibiting toxicity on normal cells. Here, Se NPs could act as a carrier of silymarin to enhance the biocompatibility of silymarin, and silymarin could also further increase the anticancer effects of Se NPs. These Si-Se NPs were also proved to induce autophagy in AGS cells through the inhibition of PI3K/Akt/mTOR pathway. Their results demonstrated that Si-Se NPs could induce stronger inhibition effects on the phosphorylation of PI3Kand Akt than that of silymarin in gastric adenocarcinoma cells, which indicated that the loading of silymarin onto Se NPs could further enhance the anticancer effects of silymarin by inhibiting PI3K/Akt/mTOR pathway. These results demonstrated that the anti-cancer activities of Se NPs are closely associated with their ability to promote cancer cell autophagy by inhibiting PI3K/Akt/mTOR pathway, which could be combined with some anticancer agents, such as silymarin, for more effective PI3K/Akt/mTOR pathway inhibition and autophagy induction in cancer cells.
It is worth noting that the reports of organic molecules composing diselenide-containing nanoparticles can also be applied as autophagy regulators. diselenide-containing fluorescent molecules (SeBDP) and antitumor drug paclitaxel (SePTX) were synthesized and used for constructing SeBDP nanoparticles (SeBDP NPs) and SePTX NPs in aqueous solution through nanoprecipitation method (68). The cellular proliferation inhibition toward tumor cells (including HeLa and MCF-7 cells) was obviously higher than that toward normal cells (BEAS-2B and L929 cells), which might be attributed to the increasing reactive oxygen species in cancer cells treated by Selenium containing nanoparticles. Moreover, they further found that SePTX NPs can successfully induce oxidative stress, cause mitochondrial dysfunction, resulting in mitochondrial pathway-mediated apoptosis, which is related to the upregulation of autophagy-related protein LC3-II (69). These data suggested that SePTX NPs exhibited high inhibiting efficiency against the growth of tumors and were able to reduce the side effects by enhancing cancer cell autophagy.
Se and curcumin have both showed excellent antitumor effects individually or in combination with other therapeutic agents. Based on these two aspects, Kumari et al. (70) synthesized curcumin-supported selenium nanoparticles (SeCurNPs) to achieve an enhanced therapeutic effect. They found that the therapeutic effect of SeCurNPs on colorectal cancer cells (HCT116) was mainly attributable to increased levels of autophagy and apoptosis as autophagy-associated protein (LC3B-II) and proapoptotic protein (Bax) were significantly upregulated and anti-apoptotic protein (Bcl-2) and cytochrome C (Cyt C) were downregulated (70). These results indicated the ability of SeCurNPs for tumor treatment by increasing cancer cells autophagy. In the following work, they further synthesized CD44-targeted DOX loaded nanoparticles (PSHA-DOXNPs) and evaluated their anticancer efficacy in combination with curcumin loaded selenium nanoparticles (Se-Cur NPs) (71). Combination of these nanoparticles (NPs) increased ROS level, decreased mitochondrial membrane potential, induced cell cycle arrest and apoptosis in HCT116 cells, and also enhanced the autophagy of these cancer cells. These results also demonstrated the potential of Se NPs to work with other nanoparticles for synergetic anticancer treatment by regulating autophagy.
Huang et al. (72) synthesized Pleurotus tuber-regium (PTR)-conjugated Se NPs (PTR-Se NPs) and investigated its application in colorectal cancer. They found that PTR-Se NPs can trigger intracellular G2/M phase arrest and promote HCT 116 cell death by activating autophagy through upregulation of intracellular Beclin 1. In addition, they also found that autophagy plays an important role in the apoptotic promotion and induction of cell death by PTR-Se NPs, which can promote the release of pro-apoptotic proteins Bax and Bak and initiate mitochondria-mediated apoptosis (72). This study confirmed the high efficacy of PTR-Se NPs in the treatment of colorectal cancer and found that autophagy plays an important role in promoting apoptosis to induce cancer cell death. In same specific situation, Se NPs could both be autophagy activators and inhibitors. Cui et al. (39) synthesized laminarin polysaccharides (LP) decorated selenium nanoparticles (LP-Se NPs) and found that these nanoparticles could induce mitochondria-mediated apoptosis by upregulating Bax expression, cutting caspase-9, and downregulating Bcl-2 expression. More interestingly, LP-Se NPs can induce early autophagy activation but block late autophagy in these cancer cells. The inhibition of autophagy in the late stage could lead to the failure of damaged organelles to be cleared, which could further aggravate cell apoptosis. The results suggest that LP-Se NPs play a cytotoxic role by inhibiting autophagy and promoting apoptosis.
Biomimetic materials are often capable of subtly affecting tissue development, and regeneration, and are also expected to mediate tumor suppression due to their high similarity to natural tissues. Li et al. (73) prepared hierarchically constructed bone-mimetic selenium-doped hydroxyapatite nanoparticles (B-SeHANs), which recapitulated the uniaxially oriented hierarchical structure of bone HA and could potentially play a dual role in the postoperative treatment of bone tumors via the chemotherapy from selenium and the promotion of bone repair by hydroxyapatite. In this work, they found that B-SeHANs could induce excess ROS production and promote autophagy in human MNNG/HOS osteosarcoma cells by activating ROS-mediated JNK pathway and inhibiting Akt/mTOR pathway. This work introduces a viable strategy for the development, evaluation and fundamental study of biomimetic selenium nanoparticles to inhibit tumor growth. Huang et al. (74) proposed dual-targeted modified selenium nanoparticles (u/A-Se NPs) as a biocompatible tumor chemotherapeutic drug, which was proved to promote ROS accumulation and autophagosome formation for synergistic HepG2 cell death. These results collectively suggested that Se NPs might be served as a novel anti-tumor agent due to their ability to promote cancer cell autophagy, which is closely related to their ability to induce high ROS levels.
Selenite, a touted cancer chemopreventive agent, has multiple mechanisms of cytotoxicity in cancer cells that are thought to be induced by selenite metabolites. Bao et al. (75) found that the intracellular metabolism of selenite can produce endogenous Se NPs in cancer cells, and its chelation with heat shock protein 90 can reduce the expression of LC3-II, showing the ability of these endogenous Se NPs for the inhibition of autophagy. In addition, Se NPs can also induce glycolytic inhibition and glycolytic-dependent mitochondrial dysfunction, suggesting that endogenous Se NPs may be the main cause of selenite-induced cytotoxicity. Although the exact mechanisms for how these endogenous Se NPs inhibit cancer cell autophagy remain to be further investigated, this work also introduces the potential for cancer cell inhibition by manipulating the endogenous Se NPs sysnthesis.
We have summarized the current knowledge of how Se NPs inhibit cancer cell growth or induce cancer cell death by promoting cancer cell autophagy (Figure 2). Se NPs are capable of activating ROS-mediated JNK pathway and inhibiting PI3K/Akt/mTOR pathway. These autophagy induction properties of Se NPs could further promote cancer cell apoptosis, which finally results in cancer cell death. Moreover, the ability of Se NPs to promote cancer cell autophagy by activating ROS-mediated JNK pathway and inhibiting PI3K/Akt/mTOR pathway might also be combined with current chemotherapy method for synergetic anticancer treatment.
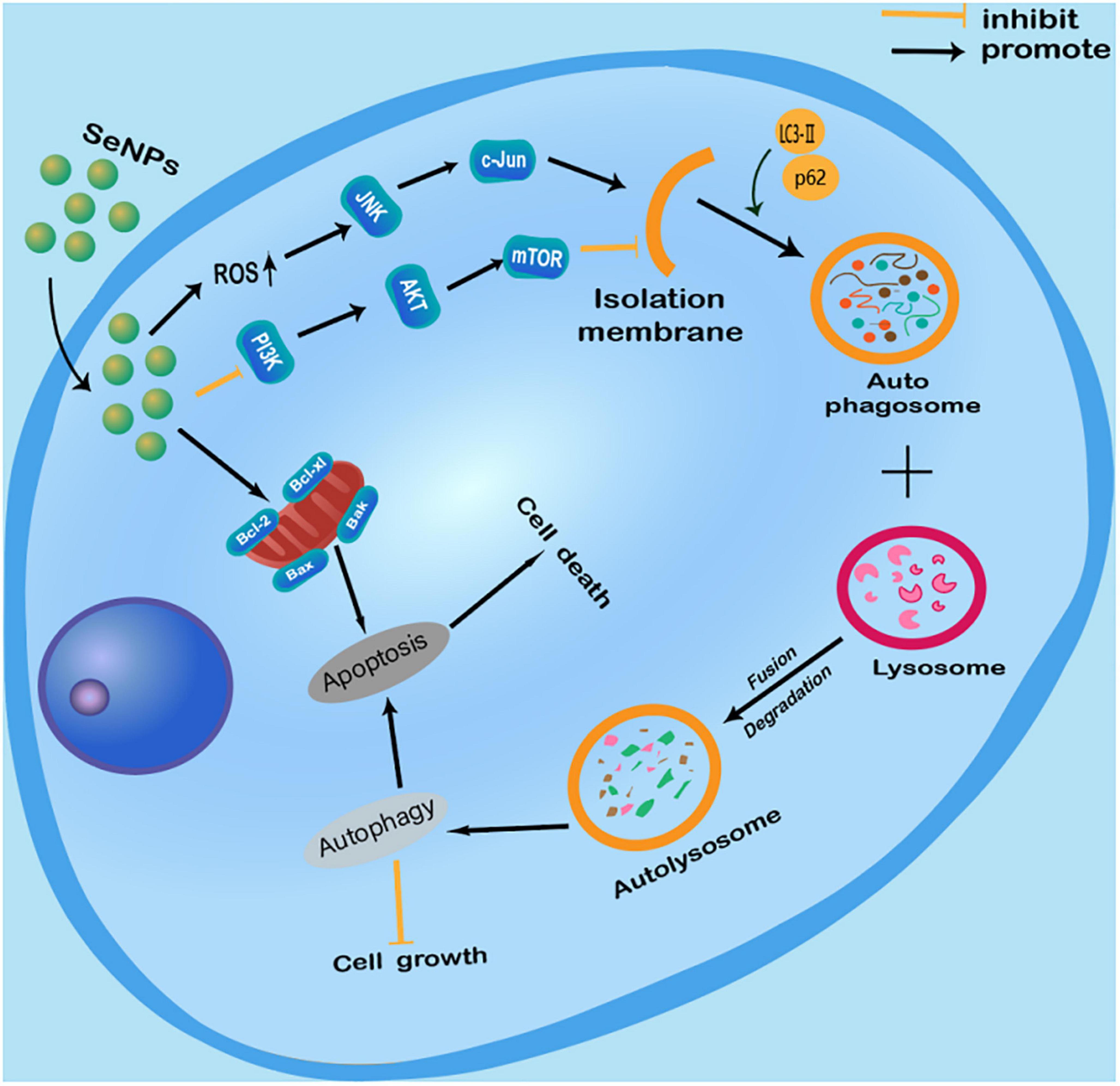
Figure 2. Anticancer effects of selenium nanoparticles (Se NPs) by promoting cancer cell autophagy through the regulation of reactive oxygen species (ROS)-mediated c-Jun N-terminal kinase pathway and PI3K/Akt/mTOR pathway.
Mechanisms for Se NPs regulated autophagy in infectious diseases
Except for the ability of Se NPs to inhibit cancer cells by regulating autophagy, our previous work also indicated the potentials of Se NPs to inhibit intracellular pathogens. Using some immune escaping mechanisms, such as their ability to inhibit autophagy and apoptosis of the host cells, Mycobacterium tuberculosis (Mtb) can escape from the immunological killings of host cells. We previously prepared a kind of Se NPs modified with mannose and encapsulated with isoniazide (Ison@Man-Se NPs) for synergistic drug-induced and phagolysosomal destruction of Mtb. Except for the ability of Ison@Man-Se NPs to promote Mtb-lysosome fusion, apoptosis and M1 polarization in Mtb infected macrophages, we also found that Ison@Man-Se NPs could promote autophagy of Mtb infected macrophages (76). The obtained results also indicated that Se NPs promoted autophagy might be associated with PI3K/Akt/mTOR pathway. And the formation of autophagosomes in the Mtb infected macrophages could finally promote the destruction of Mtb for synergistic intracellular Mtb killings with the anti-tuberculosis drugs. These results indicated that Se NPs, with the ability to promote autophagy of Mtb infected macrophages, could also serve as a kind of novel anti-tuberculosis agents for anti-tuberculosis strategy development. Moreover, these results also indicate the ability of Se NPs for intracellular pathogen clearance by regulating autophagy, which therefore can be served as potential anti-infectious agents.
Mechanisms for Se NPs regulated autophagy in other diseases
Rheumatoid arthritis (RA), a chronic autoimmune disease, is still lack of effective treatments. Liu et al. (77) prepared Se NPs-PEG-RGD@Ru by modifying selenium nanoparticles with PEG, RGD, and Ru, to target the abundant neovascular network of inflammatory sites, which increased NO production to activate autophagy by modulating signaling AMPK and mTOR pathways and inhibiting the activity of NF-κB-p65 to modulate the levels of inflammatory cytokines in Human Umbilical Vein Endothelial Cells (HUVECs). These unexpected results provided an effective strategy for the target treatment of RA based on the ability of Se NPs to promote autophagy of HUVECs.
Adolescence, a period of intense development, is accompanied by important physiological endocrine, and neurodevelopmental changes. Obesity, insulin resistance, and anorexia nervosa are particularly rising during this period. Ojeda et al. (78) firstly demonstrated in vivo that low selenite supplementation could promote adipogenesis through the insulin signaling pathway and lipocalin2 regulation, while low Se NPs administration could prevent fat storage in white adipose tissue and alleviate inflammation by reducing insulin signaling pathway and FOXO3A autophagy. These results provided data for dietary approaches to prevent obesity and/or anorexia during adolescence using Se NPs as autophagy regulators and also highlighted the potential roles of Se NPs for the prevention of adipocyte differentiation.
Se NPs may have a potential role in treating dermal disorders due to their wide therapeutic properties, but there is a need to evaluate their toxicity in keratinocytes. Thus, Kirwale et al. (79) synthesized Se NPs and tested their effects on keratinocytes, which indicated that Se NPs could promote autophagy by inducing AMPK phosphorylation and acidic lysosome formation, which finally enhanced the apoptosis of keratinocytes. These results indicated that Se NPs could induce the oxidative stress and autophagy mediated apoptotic cell death in human keratinocytes cells, which reminds the toxicity issues of Se NPs for further therapeutic application against dermal disorders.
Moreover, Se NPs can also show different protection effects by regulating autophagy. Yan et al. (80) found that Se NPs synthesized by Lactobacillus casei ATCC 393 could attenuate the H2O2-induced intestinal epithelial barrier dysfunction and ROS overproduction, as well as alleviate the adenosine triphosphate (ATP) level and the mitochondrial membrane potential (MMP) decrease. In addition, these Se NPs inhibited H2O2-induced phosphorylation of the mammalian target of rapamycin (m-TOR), and the increased expression levels of UNC-51-like kinase 1 (ULK1), light chain 3 (LC3)-II/LC3-I, PTEN-induced kinase 1 (PINK1), and Parkin proteins (80). However, the reasons for the simultaneous increased expression of m-TOR and LC3-II are not well-investigated. It has been widely known that m-TOR can also promote necrosis (81), so we speculate that H2O2 may induce autophagy and cell necrosis simultaneously, and some unknown signaling events involved in necrosis may promote the expression of m-TOR. In conclusion, these results suggested that these Se NPs can effectively alleviate H2O2-induced intestinal epithelial barrier dysfunction by regulating mTOR/PINK1-mediated autophagy (80). The same group also found that Se NPs can inhibit protein kinase R-like endoplasmic reticulum kinase (PERK), eukaryotic initiation factor 2 (eIF2α), and the expression levels of CHOP and p-PERK activating transcription factor 4 (ATF4) to inhibit endoplasmic reticulum stress (ERS) in intestinal epithelial cells exposed to H2O2 (82). And Se NPs were found to regulate endoplasmic reticulum stress-mediated mitophagy by inhibiting the AMPK/mTOR/PINK1 signaling pathway, thereby reducing intestinal epithelial barrier damage (82).
These results suggested the protective effects of Se NPs regulated autophagy against H2O2-induced cell dysfunction by regulating mTOR/PINK1-mediated pathway, PERK/eIF2α/ATF4 and AMPK/mTOR/PINK1 signaling pathway (Figure 3). Although more works are still needed to further elucidate the exact mechanisms involved in their protective effects, the protective roles of Se NPs in different disease models by regulating autophagy have strongly suggested the potential uses of Se NPs as novel protective agents.
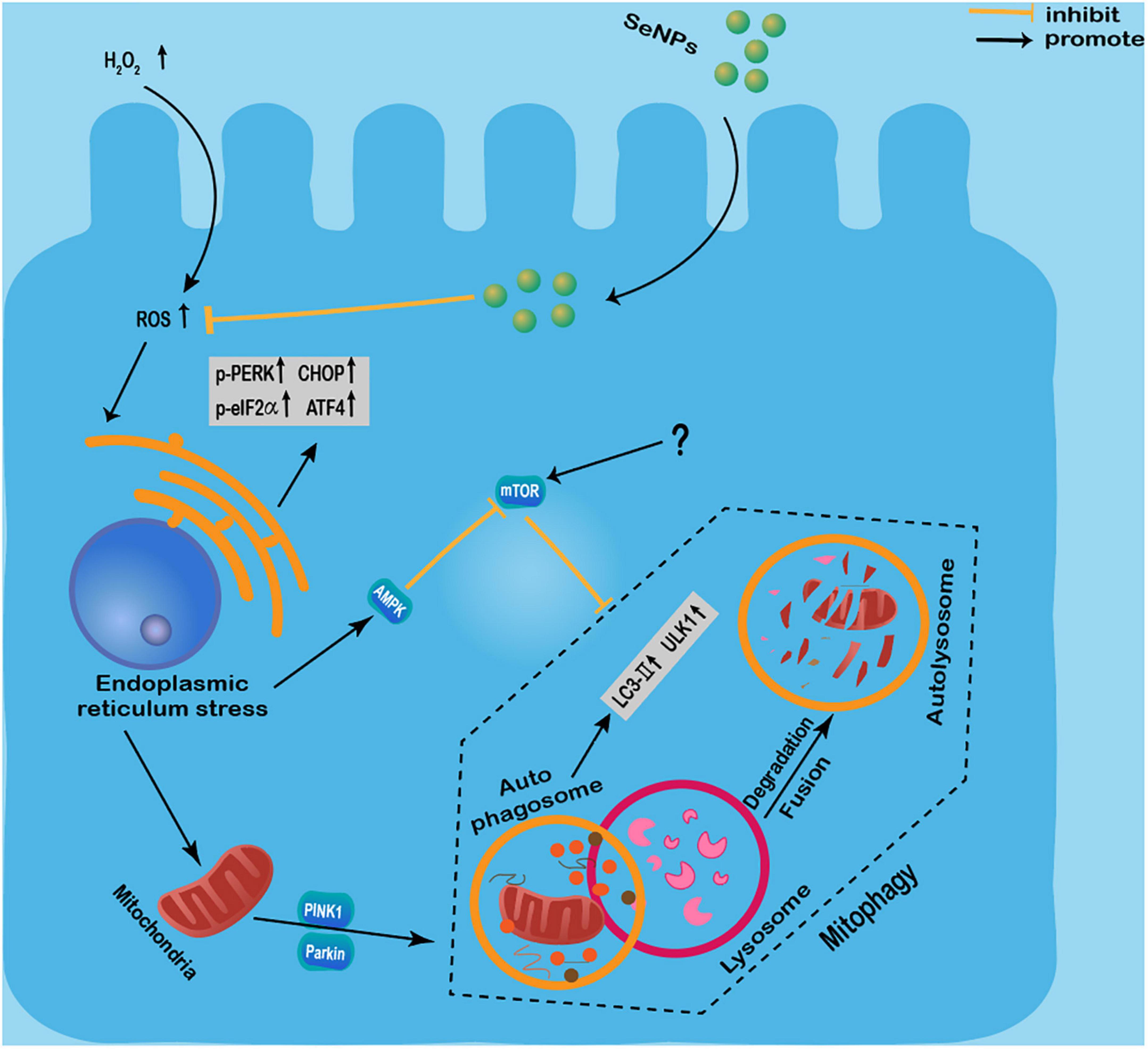
Figure 3. Protective effects of selenium nanoparticles (Se NPs) regulated autophagy against H2O2-induced cell dysfunction by regulating mTOR/PINK1-mediated pathway, PERK/eIF2α/ATF4 and AMPK/mTOR/PINK1 signaling pathway.
Potentials of Se NPs for disease treatment through autophagy modulation
Se NPs have attracted widespread attentions in biomedical field due to their unique biological, physical and chemical properties. By the modulation of autophagy, Se NPs exhibit excellent biological activities that can directly contribute to the treatment of cancer, infection and other diseases, which are expected to facilitate the development of novel therapeutics.
Cancer, one of the greatest threats to human health, is causing very high mortality rate worldwide that dramatically threatens human lives (83–85). Currently, the main treatment for cancer is chemotherapy, however, while chemotherapy kills cancer cells, it can also damage normal cells and cause serious side effects that also injure the human health (86–88). Se NPs, a potential anti-cancer agent, can not only directly kill cancer cells, but can also enhance the targeting effects of current chemotherapeutics and achieve synergetic anti-cancer therapy. For instance, adriamycin could be loaded onto Se NPs to achieve enhanced cellular uptake of adriamycin, which therefore resulted in greater anti-cervical cancer activity (89). By promoting the autophagy and apoptosis, Se NPs loaded with curcumin could kill the cancer cells more effectively and inhibit the tumor growth in tumor-bearing mice with prolonged survival time (70). In addition, the combination of curcumin loaded Se NPs and doxorubicin loaded Se NPs can target multiple molecular targets, which is beneficial to enhance anticancer effect (71).
Drug resistance of cancer is a widely-known issue that makes cancer cells to be tolerant to pharmaceutical treatment, which results in the more and more difficult therapy of cancer. Se NPs can also reduce the resistance of tumor cells to chemotherapy drugs by regulating autophagy. It has been shown that Se NPs modified with kelp polysaccharides can inhibit autophagy in HepG-2 cells by reducing the fusion of autophagosomes with lysosomes or decreasing the enzymatic activity of lysosomes, which is beneficial in reducing the resistance of tumor cells to chemotherapeutic agents in vitro (39).
Se NPs can also be combined with radiation therapy to increase the autophagy of breast cancer cells as a kind of novel radiosensitizer. For example, Chen et al. (90) reported that Se NPs could reinforce the toxic effects of irradiation, lead to a higher mortality rate than either treatment used alone, induce cell cycle arrest and the activation of autophagy, and increase both endogenous and irradiation-induced reactive oxygen species formation for enhanced radiation therapy efficiency. PEG-modified Se NPs can synergistically inhibit tumor cell growth by DNA fragmentation and caspase-3 activation, which similarly shows radiosensitization to X-rays (91).
Infectious diseases, caused by pathogens infections such as bacteria, fungi and viruses, remain a serious diseases that threaten public health (92, 93). Plenty of works have indicated the ability of selenium compounds to kill intracellular pathogens by regulating the autophagy of pathogen infected host cells (22, 24). The antibacterial and antiviral activities of selenium compounds through modulation of autophagy indicate the potential ability of Se NPs for anti-infection treatments. It has been shown that macrophage targeted Man-Se NPs can kill Mycobacterium tuberculosis (Mtb) by promoting macrophage autophagy (76). This well-designed Se NPs can increase the expression of LC3B-II protein in BCG-infected THP-1 cells by regulating of PI3K/mTOR/AKT pathway, inducing autophagosome formation and promoting the fusion of Mtb into autolysosomes for more effective Mtb killing (76).
Diabetes is a chronic (long-lasting) health condition that affects how your body turns food into energy (94). It has been shown that Se NPs can alleviate the symptoms of weight loss, lower blood glucose levels and improve antioxidant status in diabetic mice (95). Diabetic nephropathy during pregnancy in diabetic female rats can also be alleviated by supplementation with Se NPs (96). These results suggest the promising application of Se NPs for anti-diabetic therapy. Due to critical roles of autophagy in diabetes development (97), we believe that more attentions would be paid into the effects of Se NPs on autophagy regulation for diabetes treatment.
Renal injury is a non-negligible issue for chemotherapy as lots of chemotherapeutic drugs would lead to inevitable renal toxicity (98). The intriguing relationships between Se physiology and several derangements and comorbidities associated with acute and chronic kidney disease have been well-demonstrated in recent years (99). Se NPs have also been proved to efficiently reduce renal tissue injury and regulate the expression pattern of aldose reductase in the diabetic-nephropathy rat model (100), indicating the potentials of Se NPs to relieve renal injury. In addition, Se NPs can also improve gentamicin-induced kidney damage by inhibiting oxidative damage, inflammation and apoptosis-induced by autophagy (101), which introduced a good option of Se NPs as an adjunctive treatment to reduce its side effects for kidney damage.
Conclusion and perspectives
Autophagy is an evolutionarily conserved process that mediates the degradation of long-lived proteins and damaged organelles in response to a variety of stressful stimulus, including starvation, oxidative stress, and viral infection (1, 102, 103). During autophagy, the cytoplasmic fraction is isolated in autophagosomes, which eventually fuse with lysosomal compartments for overall degradation (104, 105). Se is an essential trace element in animals, and has been proved to show important biological function in anti-oxidative stress, anti-tumor and improving the immunity of the body. Different forms Se (including sodium selenite, selenoprotein, and selenium-rich yeast) act in different ways in the human body, and Se NPs have attracted increasing of attention in recent years due to their attractive biological activities (26, 106, 107). Here, we summarized the current research progress on the ability and how could Se NPs induce tumor cell death, reduce drug toxicity, modulate inflammatory responses, resist pathogenic bacterial infections, treat Alzheimer’s disease as well as other diseased conditions by regulating autophagy.
Although Se has showed attractive biological activities, selenium compounds are widely restricted by their high toxicity and poor targeting. Selenium compounds, such as sodium selenite, have strong anticancer effects only at high doses, but the toxicity at high doses just provided a weak gap between beneficial and toxic effects. Therefore, toxicity has been one of the key issues in the development of selenium-based anticancer drugs. Se NPs, a new type of monomeric selenium, have indicated strong biological activities, such as low toxicity, high bioavailability, regulation of selenoprotein functions, scavenging of free radicals, protection of oxidative DNA damage, and strong anti-cancer effects compared to inorganic or organic selenium compound (29, 108–110). In addition, taking the advantages of good biocompatibility, high loading rate, low toxicity, easy synthesis and easy storage, Se NPs can also serve as drug carriers to improve the solubility and stability with prolonged cycle time, thus increasing drug efficiency (33, 111, 112).
More importantly, increasing studies are suggesting that Se NPs can serve as a kind of novel autophagy regulators, which might provide the potential use of Se NPs against different diseased conditions. In recent years, the ability of Se NPs for autophagy regulation has been proved to show strong potentials in some impotent diseases, including cancer, tuberculosis, rheumatoid arthritis, adolescent obesity or anorexia, skin diseases, diabetes and kidney injury. The attractive bioactivity of Se NPs for autophagy modulation not only suggests Se NPs for disease treatment alone (78, 79), but also introduces the possibilities to combine Se NPs and current chemotherapeutics to achieve enhanced efficiency (70, 71), which would be beneficial in facilitating the development of new therapies.
However, there are still lots of critical issues for the further clinical application of Se NPs by regulating autophagy. Firstly, although some works have indicated the potential mechanisms of Se NPs induced autophagy, most of these works just provided some phenotype results without depth investigations (68, 75, 78). To further confirm the detailed mechanisms of Se NPs induced autophagy, knockout/knockdown experiments in cell and mice models are needed. These works would further explain whether these potential pathways are critical for the control of Se NPs induced autophagy. Secondly, the relations between autophagy and apoptosis are also needed to be further investigated in Se NPs induced autophagy, as most of the results indicated that Se NPs could both induce autophagy and apoptosis (63, 67, 73).
Thirdly, the toxicity issue of Se NPs should be considered as one of the most important issues for the further application of Se NPs. Although some works have indicated that Se NPs could inhibit cancer cells selectively without significant inhibition effects on normal cells (63, 89), the detailed mechanisms for these selectivity are still unknown. And there are still few studies that specifically focus on the systemic toxicity of long-term Se NPs treatment in vivo. Although Se NPs have been claimed to show relatively lower toxicity and higher degradability, the degradation and metabolism processes of Se NPs in vivo have not been well-explored. More concerns should be paid to the safety issues of Se NPs to investigate how they uptake, metabolize, degrade and eliminate in animal models, as well as how they interact with normal tissues and cells in vivo and in vitro, on account of excessive Se causing toxic symptoms.
Overall, taking the advantages of their advanced ability to regulate autophagy, Se NPs have demonstrated attractive potentials for the treatment of different diseases. And these autophagy regulation effects of Se NPs can be served as an adjuvant treatment to boost the efficiency of current therapeutics, such as chemotherapy, radiotherapy and immunotherapy. With the increased understanding of their functions and mechanisms, especially their metabolism, degradation and long-term safety in vivo, we believe that Se NPs could be applied as kind of novel autophagy regulators, which might provide new possibilities to benefit the clinical therapeutics against some important diseases.
Author contributions
DC and HL drafted this manuscript. YM, SF, YuH, TZ, WL, and YiH helped to revise the manuscript. YR, J-FX, and JP helped to revise the manuscript and were responsible for leading this work. All authors contributed to the article and approved the submitted version.
Funding
This work was supported by National Natural Science Foundation of China (82272348, 82270013, and 81870016), High Talent Project of Guangdong Province (2021QN02Y720), Natural Science Foundation of Guangdong Province (2022A1515010525 and 2022A1515011223), Characteristic Innovation Project of Universities in Guangdong Province (2021KTSCX038), Key Project of Universities in Guangdong Province (2022ZDZX2021), Innovation Team Project of Universities in Guangdong Province (2022KCXTD010), Key Project of Science and Technology of Dongguan (20211800905072), Funds for Ph.D. Researchers of Guangdong Medical University in 2021 (4SG22209G), Discipline Construction Project of Guangdong Medical University (4SG21229GDGFY01), Open Research Fund for Key Laboratory of Tropical Disease Control Sun Yat-sen University, Ministry of Education (2022kfkt01), and Open Research Fund of Songshan Lake Materials Laboratory (2021SLABFN10).
Conflict of interest
The authors declare that the research was conducted in the absence of any commercial or financial relationships that could be construed as a potential conflict of interest.
Publisher’s note
All claims expressed in this article are solely those of the authors and do not necessarily represent those of their affiliated organizations, or those of the publisher, the editors and the reviewers. Any product that may be evaluated in this article, or claim that may be made by its manufacturer, is not guaranteed or endorsed by the publisher.
References
1. Mizushima N, Komatsu M. Autophagy: renovation of cells and tissues. Cell. (2011) 147:728–41. doi: 10.1016/j.cell.2011.10.026
2. Mizushima N. Autophagy: process and function. Genes Dev. (2007) 21:2861–73. doi: 10.1101/gad.1599207
3. Glick D, Barth S, Macleod KF. Autophagy: cellular and molecular mechanisms. J Pathol. (2010) 221:3–12. doi: 10.1002/path.2697
4. Levine B, Kroemer G. Autophagy in the pathogenesis of disease. Cell. (2008) 132:27–42. doi: 10.1016/j.cell.2007.12.018
5. Ichimiya T, Yamakawa T, Hirano T, Yokoyama Y, Hayashi Y, Hirayama D, et al. Autophagy and autophagy-related diseases: a review. Int J Mol Sci. (2020) 21:8974. doi: 10.3390/ijms21238974
6. Cuervo AM, Bergamini E, Brunk UT, Dröge W, Ffrench M, Terman A. Autophagy and aging: the importance of maintaining “clean” cells. Autophagy. (2005) 1:131–40. doi: 10.4161/auto.1.3.2017
7. Levine B, Deretic V. Unveiling the roles of autophagy in innate and adaptive immunity. Nat Rev Immunol. (2007) 7:767–77. doi: 10.1038/nri2161
8. Chen YC, Prabhu KS, Mastro AM. Is selenium a potential treatment for cancer metastasis? Nutrients. (2013) 5:1149–68. doi: 10.3390/nu5041149
9. Joseph J, Loscalzo J. Selenistasis: epistatic effects of selenium on cardiovascular phenotype. Nutrients. (2013) 5:340–58. doi: 10.3390/nu5020340
10. Yüksel E, Nazıroğlu M, şahin M, Çiğ B. Involvement of TRPM2 and TRPV1 channels on hyperalgesia, apoptosis and oxidative stress in rat fibromyalgia model: protective role of selenium. Sci Rep. (2017) 7:17543. doi: 10.1038/s41598-017-17715-1
11. Rayman MP. Selenium and human health. Lancet. (2012) 379:1256–68. doi: 10.1016/S0140-6736(11)61452-9
12. Hatfield DL, Tsuji PA, Carlson BA, Gladyshev VN. Selenium and selenocysteine: roles in cancer, health, and development. Trends Biochem Sci. (2014) 39:112–20. doi: 10.1016/j.tibs.2013.12.007
13. Lubos E, Loscalzo J, Handy DE. Glutathione peroxidase-1 in health and disease: from molecular mechanisms to therapeutic opportunities. Antioxid Redox Signal. (2011) 15:1957–97. doi: 10.1089/ars.2010.3586
14. Bianco AC, Salvatore D, Gereben B, Berry MJ, Larsen PR. Biochemistry, cellular and molecular biology, and physiological roles of the iodothyronine selenodeiodinases. Endocr Rev. (2002) 23:38–89. doi: 10.1210/edrv.23.1.0455
15. Labunskyy VM, Hatfield DL, Gladyshev VN. Selenoproteins: molecular pathways and physiological roles. Physiol Rev. (2014) 94:739–77. doi: 10.1152/physrev.00039.2013
16. Fath MA, Ahmad IM, Smith CJ, Spence J, Spitz DR. Enhancement of carboplatin-mediated lung cancer cell killing by simultaneous disruption of glutathione and thioredoxin metabolism. Clin Cancer Res. (2011) 17:6206–17. doi: 10.1158/1078-0432.CCR-11-0736
17. Scarbrough PM, Mapuskar KA, Mattson DM, Gius D, Watson WH, Spitz DR. Simultaneous inhibition of glutathione- and thioredoxin-dependent metabolism is necessary to potentiate 17AAG-induced cancer cell killing via oxidative stress. Free Radic Biol Med. (2012) 52:436–43. doi: 10.1016/j.freeradbiomed.2011.10.493
18. Kryukov GV, Kumar RA, Koc A, Sun Z, Gladyshev VN. Selenoprotein R is a zinc-containing stereo-specific methionine sulfoxide reductase. Proc Natl Acad Sci U.S.A. (2002) 99:4245–50. doi: 10.1073/pnas.072603099
19. Kroemer G, Galluzzi L, Vandenabeele P, Abrams J, Alnemri ES, Baehrecke EH, et al. Classification of cell death: recommendations of the Nomenclature Committee on Cell Death 2009. Cell Death Differ. (2009) 16:3–11. doi: 10.1038/cdd.2008.150
20. Chi Q, Luan Y, Zhang Y, Hu X, Li S. The regulatory effects of miR-138-5p on selenium deficiency-induced chondrocyte apoptosis are mediated by targeting SelM. Metallomics. (2019) 11:845–57. doi: 10.1039/c9mt00006b
21. Cui J, Liu H, Xu S. Selenium-deficient diet induces necroptosis in the pig brain by activating TNFR1 via mir-29a-3p. Metallomics. (2020) 12:1290–301. doi: 10.1039/d0mt00032a
22. Qian G, Liu D, Hu J, Gan F, Hou L, Zhai N, et al. SeMet attenuates OTA-induced PCV2 replication promotion by inhibiting autophagy by activating the AKT/mTOR signaling pathway. Vet Res. (2018) 49:15. doi: 10.1186/s13567-018-0508-z
23. Cheng Z, Shu Y, Li X, Li Y, Zhou S, Liu H. Evaluation of potential cardiotoxicity of ammonia: l-selenomethionine inhibits ammonia-induced cardiac autophagy by activating the PI3K/AKT/mTOR signaling pathway. Ecotoxicol Environ Saf. (2022) 233:113304. doi: 10.1016/j.ecoenv.2022.113304
24. Zang H, Qian S, Li J, Zhou Y, Zhu Q, Cui L, et al. The effect of selenium on the autophagy of macrophage infected by Staphylococcus aureus. Int Immunopharmacol. (2020) 83:106406. doi: 10.1016/j.intimp.2020.106406
25. Chen W, Liu Z, Zheng Y, Wei B, Shi J, Shao B, et al. Selenium donor restricts the intracellular growth of Mycobacterium tuberculosis through the induction of c-Jun-mediated both canonical autophagy and LC3-associated phagocytosis of alveolar macrophages. Microb Pathog. (2021) 161:105269. doi: 10.1016/j.micpath.2021.105269
26. Yu H, Huang Y, Ge Y, Hong X, Lin X, Tang K, et al. Selenite-induced ROS/AMPK/FoxO3a/GABARAPL-1 signaling pathway modulates autophagy that antagonize apoptosis in colorectal cancer cells. Discov Oncol. (2021) 12:35. doi: 10.1007/s12672-021-00427-4
27. Skalickova S, Milosavljevic V, Cihalova K, Horky P, Richtera L, Adam V. Selenium nanoparticles as a nutritional supplement. Nutrition. (2017) 33:83–90. doi: 10.1016/j.nut.2016.05.001
28. Hosnedlova B, Kepinska M, Skalickova S, Fernandez C, Ruttkay-Nedecky B, Peng Q, et al. Nano-selenium and its nanomedicine applications: a critical review. Int J Nanomedicine. (2018) 13:2107–28. doi: 10.2147/IJN.S157541
29. Menon S, Ks SD, Santhiya R, Rajeshkumar S, Venkat Kumar S. Selenium nanoparticles: a potent chemotherapeutic agent and an elucidation of its mechanism. Colloids Surf B Biointerfaces. (2018) 170:280–92. doi: 10.1016/j.colsurfb.2018.06.006
30. Chen J, Feng T, Wang B, He R, Xu Y, Gao P, et al. Enhancing organic selenium content and antioxidant activities of soy sauce using nano-selenium during soybean soaking. Front Nutr. (2022) 9:970206. doi: 10.3389/fnut.2022.970206
31. Zhang S, Luo Y, Zeng H, Wang Q, Tian F, Song J, et al. Encapsulation of selenium in chitosan nanoparticles improves selenium availability and protects cells from selenium-induced DNA damage response. J Nutr Biochem. (2011) 22:1137–42. doi: 10.1016/j.jnutbio.2010.09.014
32. Chen T, Wong YS, Zheng W, Bai Y, Huang L. Selenium nanoparticles fabricated in Undaria pinnatifida polysaccharide solutions induce mitochondria-mediated apoptosis in A375 human melanoma cells. Colloids Surf B Biointerfaces. (2008) 67:26–31. doi: 10.1016/j.colsurfb.2008.07.010
33. Ferro C, Florindo HF, Santos HA. Selenium nanoparticles for biomedical applications: from development and characterization to therapeutics. Adv Healthc Mater. (2021) 10:e2100598. doi: 10.1002/adhm.202100598
34. Li Y, Lin Z, Zhao M, Xu T, Wang C, Xia H, et al. Multifunctional selenium nanoparticles as carriers of HSP70 siRNA to induce apoptosis of HepG2 cells. Int J Nanomedicine. (2016) 11:3065–76. doi: 10.2147/IJN.S109822
35. Zheng W, Yin T, Chen Q, Qin X, Huang X, Zhao S, et al. Co-delivery of Se nanoparticles and pooled SiRNAs for overcoming drug resistance mediated by P-glycoprotein and class III β-tubulin in drug-resistant breast cancers. Acta Biomater. (2016) 31:197–210. doi: 10.1016/j.actbio.2015.11.041
36. Xia Y, Lin Z, Li Y, Zhao M, Wang C, Guo M, et al. Targeted delivery of siRNA using RGDfC-conjugated functionalized selenium nanoparticles for anticancer therapy. J Mater Chem B. (2017) 5:6941–52. doi: 10.1039/C7TB01315A
37. Wang C, Chen H, Chen D, Zhao M, Lin Z, Guo M, et al. The inhibition of H1N1 influenza virus-induced apoptosis by surface decoration of selenium nanoparticles with β-thujaplicin through reactive oxygen species-mediated AKT and p53 signaling pathways. ACS Omega. (2020) 5:30633–42. doi: 10.1021/acsomega.0c04624
38. Zhang H, Li Z, Dai C, Wang P, Fan S, Yu B, et al. Antibacterial properties and mechanism of selenium nanoparticles synthesized by Providencia sp. DCX. Environ Res. (2021) 194:110630. doi: 10.1016/j.envres.2020.110630
39. Cui D, Ma J, Liang T, Sun L, Meng L, Liang T, et al. Selenium nanoparticles fabricated in laminarin polysaccharides solutions exert their cytotoxicities in HepG2 cells by inhibiting autophagy and promoting apoptosis. Int J Biol Macromol. (2019) 137:829–35. doi: 10.1016/j.ijbiomac.2019.07.031
40. Hu Y, Liu T, Li J, Mai F, Li J, Chen Y, et al. Selenium nanoparticles as new strategy to potentiate γδ T cell anti-tumor cytotoxicity through upregulation of tubulin-α acetylation. Biomaterials. (2019) 222:119397. doi: 10.1016/j.biomaterials.2019.119397
41. Wang Y, Zhang H. Regulation of autophagy by mTOR signaling pathway. Adv Exp Med Biol. (2019) 1206:67–83. doi: 10.1007/978-981-15-0602-4_3
42. Yang B, Li Y, Ma Y, Zhang X, Yang L, Shen X, et al. Selenium attenuates ischemia/reperfusion injury-induced damage to the blood-brain barrier in hyperglycemia through PI3K/AKT/mTOR pathway-mediated autophagy inhibition. Int J Mol Med. (2021) 48:178. doi: 10.3892/ijmm.2021.5011
43. Zhang ZH, Wu QY, Zheng R, Chen C, Chen Y, Liu Q, et al. Selenomethionine mitigates cognitive decline by targeting both tau hyperphosphorylation and autophagic clearance in an Alzheimer’s disease mouse model. J Neurosci. (2017) 37:2449–62. doi: 10.1523/JNEUROSCI.3229-16.2017
44. Wu JC, Wang FZ, Tsai ML, Lo CY, Badmaev V, Ho CT, et al. Se-Allylselenocysteine induces autophagy by modulating the AMPK/mTOR signaling pathway and epigenetic regulation of PCDH17 in human colorectal adenocarcinoma cells. Mol Nutr Food Res. (2015) 59:2511–22. doi: 10.1002/mnfr.201500373
45. Pant R, Sharma N, Kabeer SW, Sharma S, Tikoo K. Selenium-enriched probiotic alleviates western diet-induced non-alcoholic fatty liver disease in rats via modulation of autophagy through AMPK/SIRT-1 Pathway. Biol Trace Elem Res. (2022). doi: 10.1007/s12011-022-03247-x [Epub ahead of print].
46. Zhang N, Zhao Y. Other molecular mechanisms regulating autophagy. Adv Exp Med Biol. (2019) 1206:261–71. doi: 10.1007/978-981-15-0602-4_13
47. Zhang R, Liu Q, Guo R, Zhang D, Chen Y, Li G, et al. Selenium deficiency induces autophagy in chicken bursa of fabricius through ChTLR4/MyD88/NF-κB pathway. Biol Trace Elem Res. (2022) 200:3303–14. doi: 10.1007/s12011-021-02904-x
48. Adegoke EO, Xue W, Machebe NS, Adeniran SO, Hao W, Chen W, et al. Sodium Selenite inhibits mitophagy, downregulation and mislocalization of blood-testis barrier proteins of bovine sertoli cell exposed to microcystin-leucine arginine (MC-LR) via TLR4/NF-kB and mitochondrial signaling pathways blockage. Ecotoxicol Environ Saf. (2018) 166:165–75. doi: 10.1016/j.ecoenv.2018.09.073
49. Shi K, Meng D, Wang Y, Tian W, Zhang Y, An J. ATM/IKK alpha axis regulates the crosstalk between autophagy and apoptosis in selenite-treated Jurkat cells. Chem Biol Interact. (2022) 367:110178. doi: 10.1016/j.cbi.2022.110178
50. Ding D, Mou D, Zhao L, Jiang X, Che L, Fang Z, et al. Maternal organic selenium supplementation alleviates LPS induced inflammation, autophagy and ER stress in the thymus and spleen of offspring piglets by improving the expression of selenoproteins. Food Funct. (2021) 12:11214–28. doi: 10.1039/D1FO01653A
51. Li M, Cheng W, Zhang L. Maternal selenium deficiency suppresses proliferation, induces autophagy dysfunction and apoptosis in the placenta of mice. Metallomics. (2021) 13:mfab058. doi: 10.1093/mtomcs/mfab058
52. Liu R, Jia T, Cui Y, Lin H, Li S. The protective effect of selenium on the chicken pancreas against cadmium toxicity via alleviating oxidative stress and autophagy. Biol Trace Elem Res. (2018) 184:240–6. doi: 10.1007/s12011-017-1186-9
53. Zhang C, Wang LL, Cao CY, Li N, Talukder M, Li JL. Selenium mitigates cadmium-induced crosstalk between autophagy and endoplasmic reticulum stress via regulating calcium homeostasis in avian leghorn male hepatoma (LMH) cells. Environ Pollut. (2020) 265:114613. doi: 10.1016/j.envpol.2020.114613
54. Zhang L, Yang F, Li Y, Cao H, Huang A, Zhuang Y, et al. The protection of selenium against cadmium-induced mitophagy via modulating nuclear xenobiotic receptors response and oxidative stress in the liver of rabbits. Environ Pollut. (2021) 285:117301. doi: 10.1016/j.envpol.2021.117301
55. Huang H, Wang Y, An Y, Jiao W, Xu Y, Han Q, et al. Selenium alleviates oxidative stress and autophagy in lead-treated chicken testes. Theriogenology. (2019) 131:146–52. doi: 10.1016/j.theriogenology.2019.03.015
56. Wang S, Hou L, Wang M, Feng R, Lin X, Pan S, et al. Selenium-alleviated testicular toxicity by modulating inflammation, heat shock response, and autophagy under oxidative stress in lead-treated chickens. Biol Trace Elem Res. (2021) 199:4700–12. doi: 10.1007/s12011-021-02588-3
57. El Asar HM, Mohammed EA, Aboulhoda BE, Emam HY, Imam AA. Selenium protection against mercury neurotoxicity: modulation of apoptosis and autophagy in the anterior pituitary. Life Sci. (2019) 231:116578. doi: 10.1016/j.lfs.2019.116578
58. Zhou S, Zhang X, Fu Q, Cheng Z, Ji W, Liu H. The use of selenomethionine to reduce ammonia toxicity in porcine spleen by inhibiting endoplasmic reticulum stress and autophagy mediated by oxidative stress. Ecotoxicol Environ Saf. (2022) 242:113887. doi: 10.1016/j.ecoenv.2022.113887
59. Liu D, Xu J, Qian G, Hamid M, Gan F, Chen X, et al. Selenizing astragalus polysaccharide attenuates PCV2 replication promotion caused by oxidative stress through autophagy inhibition via PI3K/AKT activation. Int J Biol Macromol. (2018) 108:350–9. doi: 10.1016/j.ijbiomac.2017.12.010
60. Park SH, Kim JH, Chi GY, Kim GY, Chang YC, Moon SK, et al. Induction of apoptosis and autophagy by sodium selenite in A549 human lung carcinoma cells through generation of reactive oxygen species. Toxicol Lett. (2012) 212:252–61. doi: 10.1016/j.toxlet.2012.06.007
61. Yang Y, Luo H, Hui K, Ci Y, Shi K, Chen G, et al. Selenite-induced autophagy antagonizes apoptosis in colorectal cancer cells in vitro and in vivo. Oncol Rep. (2016) 35:1255–64. doi: 10.3892/or.2015.4484
62. Tao L, Liu K, Li J, Zhang Y, Cui L, Dong J, et al. Selenomethionine alleviates NF-κB-mediated inflammation in bovine mammary epithelial cells induced by Escherichia coli by enhancing autophagy. Int Immunopharmacol. (2022) 110:108989. doi: 10.1016/j.intimp.2022.108989
63. Wang R, Ha KY, Dhandapani S, Kim YJ. Biologically synthesized black ginger-selenium nanoparticle induces apoptosis and autophagy of AGS gastric cancer cells by suppressing the PI3K/Akt/mTOR signaling pathway. J Nanobiotechnology. (2022) 20:441. doi: 10.1186/s12951-022-01576-6
64. Hosseinabadi T, Lorigooini Z, Tabarzad M, Salehi B, Rodrigues CF, Martins N, et al. Silymarin antiproliferative and apoptotic effects: insights into its clinical impact in various types of cancer. Phytother Res. (2019) 33:2849–61. doi: 10.1002/ptr.6470
65. Kim SH, Choo GS, Yoo ES, Woo JS, Han SH, Lee JH, et al. Silymarin induces inhibition of growth and apoptosis through modulation of the MAPK signaling pathway in AGS human gastric cancer cells. Oncol Rep. (2019) 42:1904–14. doi: 10.3892/or.2019.7295
66. Javed S, Kohli K, Ali M. Reassessing bioavailability of silymarin. Altern Med Rev. (2011) 16:239–49.
67. Mi XJ, Choi HS, Perumalsamy H, Shanmugam R, Thangavelu L, Balusamy SR, et al. Biosynthesis and cytotoxic effect of silymarin-functionalized selenium nanoparticles induced autophagy mediated cellular apoptosis via downregulation of PI3K/Akt/mTOR pathway in gastric cancer. Phytomedicine. (2022) 99:154014. doi: 10.1016/j.phymed.2022.154014
68. Zhang W, Lin W, Pei Q, Hu X, Xie Z, Jing X. Redox-hypersensitive organic nanoparticles for selective treatment of cancer cells. Chem Mater. (2016) 12:4440–6. doi: 10.1021/acs.chemmater.6b01641
69. Li J, Gu Y, Zhang W, Bao CY, Li CR, Zhang JY, et al. Molecular mechanism for selective cytotoxicity towards cancer cells of diselenide-containing paclitaxel nanoparticles. Int J Biol Sci. (2019) 15:1755–70. doi: 10.7150/ijbs.34878
70. Kumari M, Ray L, Purohit MP, Patnaik S, Pant AB, Shukla Y, et al. Curcumin loading potentiates the chemotherapeutic efficacy of selenium nanoparticles in HCT116 cells and Ehrlich’s ascites carcinoma bearing mice. Eur J Pharm Biopharm. (2017) 117:346–62. doi: 10.1016/j.ejpb.2017.05.003
71. Kumari M, Purohit MP, Patnaik S, Shukla Y, Kumar P, Gupta KC. Curcumin loaded selenium nanoparticles synergize the anticancer potential of doxorubicin contained in self-assembled, cell receptor targeted nanoparticles. Eur J Pharm Biopharm. (2018) 130:185–99. doi: 10.1016/j.ejpb.2018.06.030
72. Huang G, Liu Z, He L, Luk KH, Cheung ST, Wong KH, et al. Autophagy is an important action mode for functionalized selenium nanoparticles to exhibit anti-colorectal cancer activity. Biomater Sci. (2018) 6:2508–17. doi: 10.1039/C8BM00670A
73. Li X, Wang Y, Chen Y, Zhou P, Wei K, Wang H, et al. Hierarchically constructed selenium-doped bone-mimetic nanoparticles promote ROS-mediated autophagy and apoptosis for bone tumor inhibition. Biomaterials. (2020) 257:120253. doi: 10.1016/j.biomaterials.2020.120253
74. Huang J, Liu Y, Liu T, Chang Y, Chen T, Li X. Dual-targeting nanotherapeutics antagonize hyperinsulinemia-promoted tumor growth via activating cell autophagy. J Mater Chem B. (2019) 7:6751–8. doi: 10.1039/C9TB01197H
75. Bao P, Chen Z, Tai RZ, Shen HM, Martin FL, Zhu YG. Selenite-induced toxicity in cancer cells is mediated by metabolic generation of endogenous selenium nanoparticles. J Proteome Res. (2015) 14:1127–36. doi: 10.1021/pr501086e
76. Pi J, Shen L, Yang E, Shen H, Huang D, Wang R, et al. Macrophage-targeted isoniazid-selenium nanoparticles promote antimicrobial immunity and synergize bactericidal destruction of Tuberculosis Bacilli. Angew Chem Int Ed Engl. (2020) 59:3226–34. doi: 10.1002/anie.201912122
77. Liu Y, Ma L, Zhou H, Zhu X, Yu Q, Chen X, et al. Polypeptide nano-Se targeting inflammation and theranostic rheumatoid arthritis by anti-angiogenic and NO activating AMPKα signaling pathway. J Mater Chem B. (2018) 6:3497–514. doi: 10.1039/C8TB00080H
78. Ojeda ML, Nogales F, Carreras O, Pajuelo E, Gallego-López MDC, Romero-Herrera I, et al. Different effects of low selenite and selenium-nanoparticle supplementation on adipose tissue function and insulin secretion in adolescent male rats. Nutrients. (2022) 14:3571. doi: 10.3390/nu14173571
79. Kirwale S, Pooladanda V, Thatikonda S, Murugappan S, Khurana A, Godugu C. Selenium nanoparticles induce autophagy mediated cell death in human keratinocytes. Nanomedicine. (2019) 14:1991–2010. doi: 10.2217/nnm-2018-0397
80. Yan S, Qiao L, Dou X, Song X, Chen Y, Zhang B, et al. Biogenic selenium nanoparticles by Lactobacillus casei ATCC 393 alleviate the intestinal permeability, mitochondrial dysfunction and mitophagy induced by oxidative stress. Food Funct. (2021) 12:7068–80. doi: 10.1039/D0FO03141K
81. Xie Y, Zhao Y, Shi L, Li W, Chen K, Li M, et al. Gut epithelial TSC1/mTOR controls RIPK3-dependent necroptosis in intestinal inflammation and cancer. J Clin Invest. (2020) 130:2111–28. doi: 10.1172/JCI133264
82. Qiao L, Yan S, Dou X, Song X, Chang J, Pi S, et al. Biogenic selenium nanoparticles alleviate intestinal epithelial barrier damage through regulating endoplasmic reticulum stress-mediated mitophagy. Oxid Med Cell Longev. (2022) 2022:3982613. doi: 10.1155/2022/3982613
83. Torre LA, Siegel RL, Ward EM, Jemal A. Global cancer incidence and mortality rates and trends–an update. Cancer Epidemiol Biomarkers Prev. (2016) 25:16–27. doi: 10.1158/1055-9965.EPI-15-0578
84. Siegel RL, Miller KD, Jemal A. Cancer statistics, 2019. CA Cancer J Clin. (2019) 69:7–34. doi: 10.3322/caac.21551
85. Dyba T, Randi G, Bray F, Martos C, Giusti F, Nicholson N, et al. The European cancer burden in 2020: incidence and mortality estimates for 40 countries and 25 major cancers. Eur J Cancer. (2021) 157:308–47. doi: 10.1016/j.ejca.2021.07.039
86. Peer D, Karp JM, Hong S, Farokhzad OC, Margalit R, Langer R. Nanocarriers as an emerging platform for cancer therapy. Nat Nanotechnol. (2007) 2:751–60. doi: 10.1038/nnano.2007.387
87. Pérez-Herrero E, Fernández-Medarde A. Advanced targeted therapies in cancer: drug nanocarriers, the future of chemotherapy. Eur J Pharm Biopharm. (2015) 93:52–79. doi: 10.1016/j.ejpb.2015.03.018
88. Schirrmacher V. From chemotherapy to biological therapy: a review of novel concepts to reduce the side effects of systemic cancer treatment (Review). Int J Oncol. (2019) 54:407–19.
89. Xia Y, Xiao M, Zhao M, Xu T, Guo M, Wang C, et al. Doxorubicin-loaded functionalized selenium nanoparticles for enhanced antitumor efficacy in cervical carcinoma therapy. Mater Sci Eng C Mater Biol Appl. (2020) 106:110100. doi: 10.1016/j.msec.2019.110100
90. Chen F, Zhang XH, Hu XD, Liu PD, Zhang HQ. The effects of combined selenium nanoparticles and radiation therapy on breast cancer cells in vitro. Artif Cells Nanomed Biotechnol. (2018) 46:937–48. doi: 10.1080/21691401.2017.1347941
91. Yu B, Liu T, Du Y, Luo Z, Zheng W, Chen T. X-ray-responsive selenium nanoparticles for enhanced cancer chemo-radiotherapy. Colloids Surf B Biointerfaces. (2016) 139:180–9. doi: 10.1016/j.colsurfb.2015.11.063
92. Riley LW, Blanton RE. Advances in molecular epidemiology of infectious diseases: definitions, approaches, and scope of the field. Microbiol Spectr. (2018) 6:6. doi: 10.1128/microbiolspec.AME-0001-2018
93. Levitt A, Mermin J, Jones CM, See I, Butler JC. Infectious diseases and injection drug use: public health burden and response. J Infect Dis. (2020) 222:S213–7. doi: 10.1093/infdis/jiaa432
94. Petersmann A, Müller-Wieland D, Müller UA, Landgraf R, Nauck M, Freckmann G, et al. Definition, classification and diagnosis of diabetes mellitus. Exp Clin Endocrinol Diabetes. (2019) 127:S1–7. doi: 10.1055/a-1018-9078
95. Zeng S, Ke Y, Liu Y, Shen Y, Zhang L, Li C, et al. Synthesis and antidiabetic properties of chitosan-stabilized selenium nanoparticles. Colloids Surf B Biointerfaces. (2018) 170:115–21. doi: 10.1016/j.colsurfb.2018.06.003
96. Alhazza IM, Ebaid H, Omar MS, Hassan I, Habila MA, Al-Tamimi J, et al. Supplementation with selenium nanoparticles alleviates diabetic nephropathy during pregnancy in the diabetic female rats. Environ Sci Pollut Res Int. (2022) 29:5517–25. doi: 10.1007/s11356-021-15905-z
97. Kitada M, Koya D. Autophagy in metabolic disease and ageing. Nat Rev Endocrinol. (2021) 17:647–61. doi: 10.1038/s41574-021-00551-9
98. Volarevic V, Djokovic B, Jankovic MG, Harrell CR, Fellabaum C, Djonov V, et al. Molecular mechanisms of cisplatin-induced nephrotoxicity: a balance on the knife edge between renoprotection and tumor toxicity. J Biomed Sci. (2019) 26:25. doi: 10.1186/s12929-019-0518-9
99. Iglesias P, Selgas R, Romero S, Díez JJ. Selenium and kidney disease. J Nephrol. (2013) 26:266–72. doi: 10.5301/jn.5000213
100. Khater SI, Mohamed AA, Arisha AH, Ebraheim LLM, El-Mandrawy SAM, Nassan MA, et al. Stabilized-chitosan selenium nanoparticles efficiently reduce renal tissue injury and regulate the expression pattern of aldose reductase in the diabetic-nephropathy rat model. Life Sci. (2021) 279:119674. doi: 10.1016/j.lfs.2021.119674
101. Zheng S, Hameed Sultan A, Kurtas PT, Kareem LA, Akbari A. Comparison of the effect of vitamin C and selenium nanoparticles on gentamicin-induced renal impairment in male rats: a biochemical, molecular and histological study. Toxicol Mech Methods. (2022) 18:1–11. doi: 10.1080/15376516.2022.2124136
102. Kroemer G, Mariño G, Levine B. Autophagy and the integrated stress response. Mol Cell. (2010) 40:280–93. doi: 10.1016/j.molcel.2010.09.023
103. Sir D, Ou JH. Autophagy in viral replication and pathogenesis. Mol Cells. (2010) 29:1–7. doi: 10.1007/s10059-010-0014-2
104. Maiuri MC, Zalckvar E, Kimchi A, Kroemer G. Self-eating and self-killing: crosstalk between autophagy and apoptosis. Nat Rev Mol Cell Biol. (2007) 8:741–52. doi: 10.1038/nrm2239
105. Kundu M, Thompson CB. Autophagy: basic principles and relevance to disease. Annu Rev Pathol. (2008) 3:427–55. doi: 10.1146/annurev.pathmechdis.2.010506.091842
106. Selenius M, Rundlöf AK, Olm E, Fernandes AP, Björnstedt M. Selenium and the selenoprotein thioredoxin reductase in the prevention, treatment and diagnostics of cancer. Antioxid Redox Signal. (2010) 12:867–80. doi: 10.1089/ars.2009.2884
107. Cao C, Zhang H, Wang K, Li X. Selenium-Rich yeast mitigates aluminum-mediated testicular toxicity by blocking oxidative stress. Inhibiting no production, and disturbing ionic homeostasis. Biol Trace Elem Res. (2020) 195:170–7. doi: 10.1007/s12011-019-01820-5
108. Tan L, Jia X, Jiang X, Zhang Y, Tang H, Yao S, et al. In vitro study on the individual and synergistic cytotoxicity of adriamycin and selenium nanoparticles against Bel7402 cells with a quartz crystal microbalance. Biosens Bioelectron. (2009) 24:2268–72. doi: 10.1016/j.bios.2008.10.030
109. Wang Y, Chen P, Zhao G, Sun K, Li D, Wan X, et al. Inverse relationship between elemental selenium nanoparticle size and inhibition of cancer cell growth in vitro and in vivo. Food Chem Toxicol. (2015) 85:71–7. doi: 10.1016/j.fct.2015.08.006
110. Wadhwani SA, Shedbalkar UU, Singh R, Chopade BA. Biogenic selenium nanoparticles: current status and future prospects. Appl Microbiol Biotechnol. (2016) 100:2555–66. doi: 10.1007/s00253-016-7300-7
111. Zheng L, Li C, Huang X, Lin X, Lin W, Yang F, et al. Thermosensitive hydrogels for sustained-release of sorafenib and selenium nanoparticles for localized synergistic chemoradiotherapy. Biomaterials. (2019) 216:119220. doi: 10.1016/j.biomaterials.2019.05.031
Keywords: selenium, selenium nanoparticles, autophagy, regulators, therapeutics
Citation: Chen D, Lu H, Ma Y, Huang Y, Zhang T, Fan S, Lin W, Huang Y, Jin H, Ruan Y, Xu J-F and Pi J (2023) Trends and recent progresses of selenium nanoparticles as novel autophagy regulators for therapeutic development. Front. Nutr. 10:1116051. doi: 10.3389/fnut.2023.1116051
Received: 05 December 2022; Accepted: 09 January 2023;
Published: 02 February 2023.
Edited by:
Tao Hou, Huazhong Agricultural University, ChinaReviewed by:
Weiwei Liu, Beijing Technology and Business University, ChinaXiao Xu, Wuhan Polytechnic University, China
Copyright © 2023 Chen, Lu, Ma, Huang, Zhang, Fan, Lin, Huang, Jin, Ruan, Xu and Pi. This is an open-access article distributed under the terms of the Creative Commons Attribution License (CC BY). The use, distribution or reproduction in other forums is permitted, provided the original author(s) and the copyright owner(s) are credited and that the original publication in this journal is cited, in accordance with accepted academic practice. No use, distribution or reproduction is permitted which does not comply with these terms.
*Correspondence: Yongdui Ruan, ruanyongduitangxia@163.com; Jun-Fa Xu,
xujunfa@gdmu.edu.cn; Jiang Pi,
jiangpi@gdmu.edu.cn
†These authors have contributed equally to this work and share first authorship