- 1Academy of Chinese Medical Science, Henan University of Chinese Medicine, Zhengzhou, China
- 2Henan Polysaccharide Research Center, Zhengzhou, China
- 3Henan Key Laboratory of Chinese Medicine for Polysaccharides and Drugs Research, Zhengzhou, China
- 4College of Pharmacy, Henan University of Chinese Medicine, Zhengzhou, China
Non-alcoholic fatty liver disease (NAFLD) has become the most common chronic liver disease and is a leading cause of cirrhosis and hepatocellular carcinoma. Due to its complex pathophysiology, there is currently no approved therapy. Polysaccharide, a kind of natural product, possesses a wide range of pharmacological activities. Numerous preclinical studies have confirmed that polysaccharides could interfere with the occurrence and development of NAFLD at multiple interrelated levels, such as improvement of glucose and lipid metabolism, antioxidation, anti-inflammation, and regulation of gut-liver axis, thus showing great potential as novel anti-NAFLD drugs. In this paper, we reviewed the polysaccharides with anti-NAFLD effect in recent years, and also systematically analyzed their possible pharmacological mechanisms.
Introduction
Non-alcohol fatty liver disease (NAFLD) has become the most prevalent chronic liver disease worldwide, with a global morbidity of more than 32.4%, thus representing an enormous health care burden (1). NAFLD encompasses a wide spectrum of conditions, ranging from simple hepatic steatosis to non-alcoholic steatohepatitis (NASH) with or without fibrosis, which may eventually evolve to cirrhosis or hepatocellular carcinoma (2). The NAFLD pathophysiology is complex, and is not yet fully understood. The current, and most accepted, theory explaining the pathogenesis of NAFLD is the “multiple-hit” hypothesis, which suggests that the progression of NAFLD is a result of a multitude of ‘hits,’ involving hyperlipidemia, insulin resistance (IR), mitochondrial dysfunction, inflammatory cytokines/adipokines, endoplasmic reticulum (ER) stress, dysregulation of intestinal microflora, oxidative stress and environmental and dietary factors (3). Fortunately, Dietary and lifestyle adjustments can effectively control NAFLD, but when these approaches do not work, pharmaceutical intervention is necessary. However, there are still no approved drugs for NAFLD (4, 5).
Natural products contribute greatly to drug discovery and development, and today up to 100 active ingredients derived from plants are used as medicines in clinic. As a major part of natural resources, polysaccharides are captivating growing interest due to their unique advantages, such as low toxicity, high safety, multi-target and multi-pathway. Importantly, polysaccharides have a wide range of pharmacological activities such as anti-inflammatory, antioxidant, anti-fibrotic, immunomodulatory, anti-tumor effects, and so on (6–9). In terms of NAFLD, some progress has been made recently in the study of anti-NAFLD polysaccharides, and numerous preclinical studies have confirmed that polysaccharides could interfere with the occurrence and development of NAFLD by targeting multiple mechanisms. Accordingly, in present review, we systematically summarized the potential anti-NAFLD polysaccharides and their possible pharmacological mechanisms, with the hope to provide a reference for the development of novel carbohydrate drugs against NAFLD.
Therapeutic effects of polysaccharides on NAFLD
Targeting glucose and lipid metabolism
The defining characteristic of NAFLD is an excessive buildup of triglycerides (TG) in hepatocytes. Accumulation of TG arises from abnormal lipid metabolism. More specifically, either enhanced fat synthesis and import, or reduced fat consumption and exportation, or a combination of both processes contribute to the lipid accumulation (10). Inhibition of free fatty acids (FFA) influx or de novo lipogenesis is conductive to the reduction of TG deposition, while enhancing fatty acid oxidation or export is favorable to lipid removal. Therefore, targeting these metabolic pathways may ameliorate NAFLD. Moreover, IR is always implicated in pathogenesis of NAFLD (11). Considering the potent action of insulin to suppress adipose tissue lipolysis, therefore, mitigating insulin resistance also represents a promising therapeutic strategy.
A polysaccharide from jackfruit pulp (JFP-Ps) was found to improve liver function in high-fat diets (HFD) -induced mice by modulating the expression of genes involved in lipid metabolism, such as peroxisome proliferator activated receptor alpha (PPARα), hormonesensitive lipase (HSL), carnitine palmitoyltransferase 1A (CPT1), lipoprotein lipase (LPL), acetyl-CoA carboxylase alpha (ACC), fatty acid synthase (FAS) and sterol regulatory element binding transcription factor (SREBP-1c) (12). The activation of PPAR and AMP-activated protein kinase (AMPK) signaling pathways may be responsible for its therapeutic effect. Wang et al. (13) isolated an acidic heteropolysaccharide from walnut green husk (WGHP), and found that WGHP was able to alleviate NAFLD by improving glucose and lipid metabolism. Cichorium intybus L. polysaccharide (CP) was reported to be beneficial for the treatment of metabolic dysfunctions. In NAFLD mice model, Wu et al. (14) found that CP reduced lipid contents through activating AMPK cascade. Another study showed that CP reduced the degree of steatosis by increasing the levels of L-palmitoylcarnitine and hexadecanoyl-CoA (15), which played an essential role in the fatty acid β-oxidation. Additionally, CP can also inhibit de novo lipogenesis by improving the expression of X-box binding protein 1 (Xbp1), insulin-induced gene 2 (Insig2), and cystathionine-γ-lyase (Cth). Interestingly, a similar therapeutic effect was observed in NAFLD zebrafish model. Li et al. (16) found CP could also exert lipid-lowering effect through enhancing fatty acid β-oxidation and inhibiting lipogenesis.
A polysaccharide from Stropharia rugoso-annulata (ASRP) was an acetylated polysaccharide (17). Studies showed that ASRP could reduce fat synthesis and increase fatty acids β-oxidation by activating AMPK/SREBP-1c signaling pathway. In another study, Gracilaria lemaneiformis polysaccharide (GLP), a sulfated polysaccharide, was found to dramatically decrease serum total cholesterol (TC), TG and FFA levels in high-fat diet mice and promote the conversion of cholesterol to bile acids, thus improving lipid metabolism (18). Sargassum fusiforme polysaccharide (SFPS) was also a sulfated polysaccharide. He et al. (19) found that the lipid-lowering effect of SFPS was achieved by decreasing lipogenic genes (Srebp, Fas) expression and increasing the expression of lipolytic genes (PPARɑ, CPT1). Interestingly,another sulfated polysaccharide from Enteromorpha prolifera (EP) was able to regulate cholesterol metabolism. Ren et al. (20) found EP clearly decreased the expression of SREBP-2 and 3-hydroxy-3-methylglutaryl-CoA reductase (HMGCR), which were closely associated with the decreased hepatic cholesterol biosynthesis. Additionally, hydrogen sulfide (H2S) has been found to possess many physiological effects. In another study, Ren et al. (21) reported that EP could increase H2S production in HFD-induced mice, which may account for the reduced serum TG. Lycium barbarum polysaccharide (LBP) was reported to have hepatoprotective efficacy. One study in NASH rat model showed that LBP treatment ameliorated obesity, IR and lipid accumulation, thus counteracting HFD-induced NASH (22). Another study in HFD-induced NAFLD model showed that LBP effectively decreased hepatic TG accumulation, and its regulatory effect was related to the activation of sirtuin 1 (SIRT1)/liver kinase B1 (LKB1)/AMPK pathway (23).
Yan et al. (24) found that Ginkgo biloba leaf polysaccharide (GBLP) could play a certain protective role against NAFLD by decreasing hepatic fat accumulation, improving liver function, and ameliorating insulin resistance. Another study showed that Radix hedysari polysaccharides (RHP) was able to improve lipid metabolism disorders, decrease hepatic lipid content by regulating the genes involved in lipid metabolism including PPARα and SREBP-1c (25). A polysaccharide from Poria cocos (PCP) was found to improve lipid metabolism in NAFLD mice model (26). Not only did PCP increase liver lipid transportation to the blood by enhancing the expression of genes involved in lipid transportation, such as solute carrier family 27 member 4 (Scl27a4), apolipoprotein C2 (Apoc2), and CD36 (a fatty acid translocase), but it could also promote glucose metabolism through enhancing glucose oxidative utilization.
Taken together, polysaccharide exhibited remarkable benefits on glucose and lipid metabolism in NAFLD (Figure 1). Polysaccharide could exert therapeutic effect via stimulating hepatic lipolysis, increasing fatty acid oxidation, or inhibiting lipogenesis, which are closely associated with the activation of PPAR and AMPK-related signaling pathways.
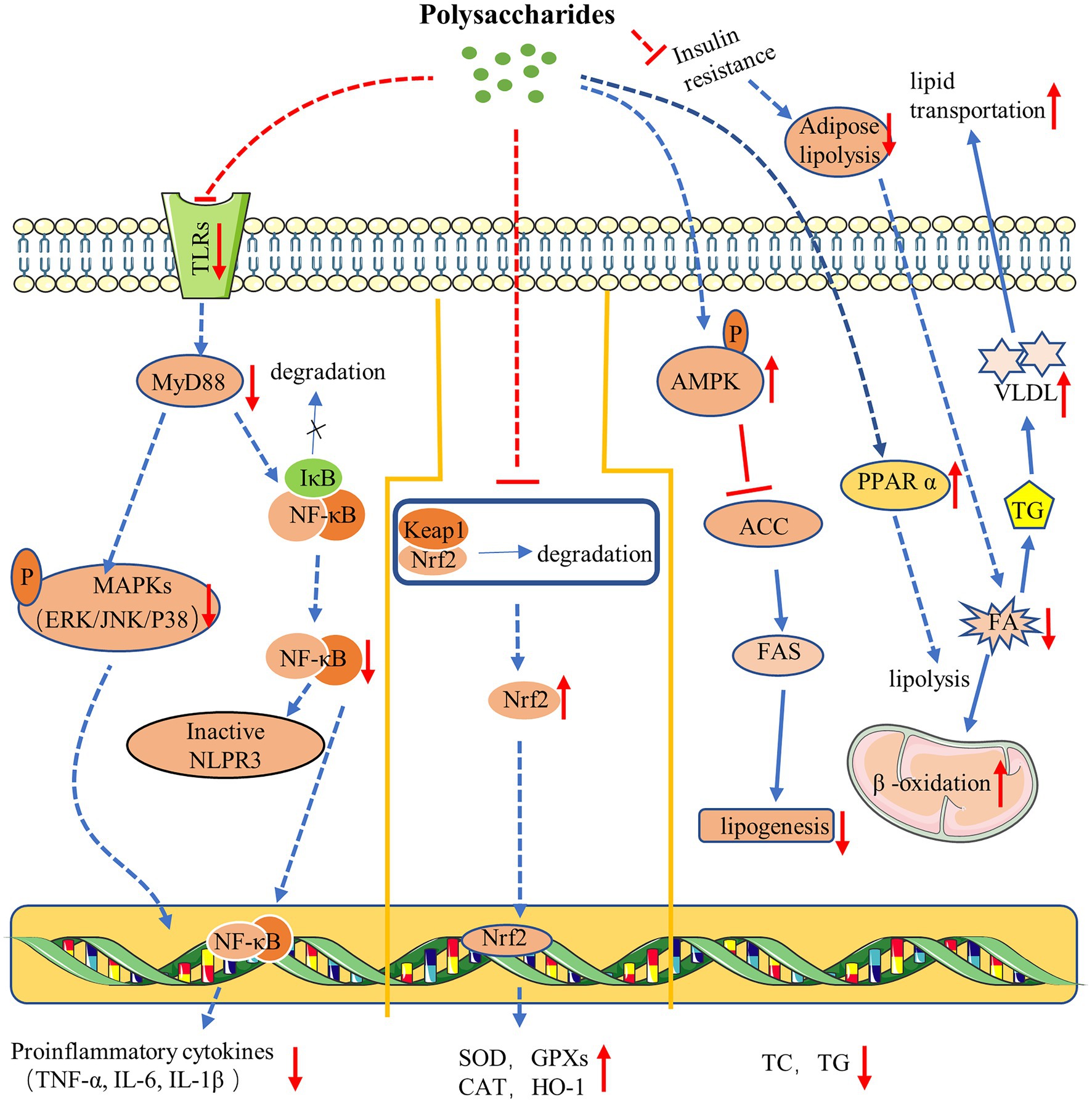
Figure 1. Mechanism of polysaccharides action on NAFLD. Polysaccharides ameliorate NAFLD via anti-inflammation (left). Polysaccharides improve NAFLD via antioxidation (middle). Polysaccharides exert therapy effect on NAFLD via regulating glucose and lipid metabolism (right).
Targeting oxidative stress in NAFLD
Oxidative stress can be defined as overproduction of reactive oxygen species (ROS) and/or a decrease or deficiency in antioxidants. Increasing evidence indicates that oxidative stress plays a central role in NAFLD development (27). Mitochondria are the primary source of ROS and excessive accumulation of ROS may lead to oxidative stress, mitochondria damage, and even cell death. In addition, ROS can also oxidize plasma membranes leading to destruction of biological membranes and production of lipid peroxidation products. Malondialdehyde (MDA) is one of the lipid peroxidation products, which is always regarded as a marker of oxidative damage. Nuclear factor E2-related factor 2 (Nrf2) is a key redox balance regulator and when oxidative stress occurs (28), it will initiate antioxidant defense response by elevating the expression of key antioxidant enzymes including superoxide dismutase (SOD), glutathione peroxidase (GSH-Px) and catalase (CAT), to maintain the redox equilibrium. Therefore, agents capable of improving mitochondrial function and ameliorating oxidative stress may prove useful for NAFLD. Indeed, anti-oxidative therapy in animal studies have already shown a promising therapeutic effect. Hence, targeting oxidative stress may represent a valuable therapeutic strategy.
In a NASH mouse model, LBP significantly reduced MDA and nitrotyrosine while increasing CAT and GSH-Px (29). Furthermore, LBP was also shown to downregulate the expression of cytochrome P450 family 2 subfamily E member 1 (CYP2E1), which is a key enzyme responsible for the production of ROS in the liver. Another study also confirmed its beneficial effect. Zhang et al. (30) found that in a NAFLD cell model, LBP clearly decreased lipid accumulation and oxidative stress, as evidenced by the reduction of TG, ALT and AST and by the increase of SOD, CAT and GSH-Px. Interestingly, LBP could also promote mitochondrial biogenesis through activating peroxisome proliferator-activated receptor γ coactivator 1 (PGC-1α)/nuclear factor erythroid 2-like 1 (NRF1) signaling pathway (31).
A polysaccharide from Sagittaria sagittifolia (SSP) was observed to improve oxidative stress in the HFD-induced NAFLD mouse by enhancing Nrf2 cascade to reduce MDA content, and increase SOD and GSH activity (32). Another polysaccharide ACP isolated from Malpighia emarginata DC. was also found to promote the Nrf2 activation, as reflected by significantly reduced MDA, and elevation of SOD, and CAT contents. Moreover, ACP could mitigate mitochondrial dysfunction by decreasing uncoupling protein 2 (UCP2) expression, and increasing mitochondrial complex I, IV, and V activity (33). Codonopsis lanceolate polysaccharide (CLPS) was able to decrease MDA level, increase the GSH content, and enhance the expression of antioxidant enzymes such as SOD and CAT, showing a significant antioxidant effect. This protective effect was closely associated with the activation of Nrf2 signaling pathway (34). Ganoderma lucidum polysaccharide (FYGL) was a hyperbranched proteoglycan consisting of polysaccharides (77%) and protein (17%). Studies indicated that FYGL could ameliorate ROS and MDA, inhibit lactate dehydrogenase release, elevate SOD content, and enhance total antioxidant capacity (35). Polysaccharide CPP-2 was obtained from Cyclocarya paliurus, exhibiting remarkable antioxidant capacity. It was reported that CPP-2 could promote the activities of enzymic and non-enzymic antioxidants involving SOD, CAT and GSH-PX while decreasing the contents of MDA and lipid peroxide (36).
In all, a series of studies have tested the effectiveness of polysaccharides as antioxidants in the treatment of NAFLD (Figure 1). Polysaccharides exert antioxidant effect through promoting the nuclear translocation of Nrf2 protein, which subsequently trigger the expression of numerous downstream antioxidant enzymes, leading to an enhanced cellular antioxidant capacity. What is more, polysaccharide can also improve mitochondrial function through diverse pathways to protect cell from oxidative damage.
Targeting inflammation in NAFLD
The transition from steatosis to NASH is mainly characterized by the occurrence of inflammation (37). Cytokines and inflammatory cells play a major role in the inflammatory processes. Kupffer cells (KCs) are the tissue-resident macrophages, and serve as the first line of protection for the liver against potential damage (38). Upon abnormal activation, however, KCs will secrete more cytokines including tumor necrosis factor-alpha (TNF-α), interleukin-6 (IL-6) and interleukin-1β (IL-1β), and further aggravate inflammatory infiltration leading to tissue injury. Among these cytokines, TNF-α and IL-6 are critically involved in the pathophysiology of NAFLD. Therefore, reducing the levels of these proinflammatory factors by regulating inflammation process appears as a possible therapeutic approach against NAFLD.
A polysaccharide from Gynostemma pentaphyllum (GP) could exert anti-inflammatory effects by regulating the Toll-like receptor 2 (TLR2)/NOD-like receptor protein 3 (NLRP3) signaling pathway (39). In a NASH mice model, GP treatment significantly inhibited the expression of TLRs genes (TLR1, TLR2, and TLR4), reduced the levels of NLRP3, and decreased the expression of pro-inflammatory genes (IL-1β, IL-18rap, and TNF-α). Zhong et al. (40) found that the polysaccharide mAPS from Astragalus mongholicus could reduce HFD-induced high expression of TNF-α, TLR4, NLRP3 and phosphorylated- nuclear factor-κB (NF-κB) by downregulating the TLR4/NF-κB signaling pathway. Fucoidan, a sulfated polysaccharide extracted from marine brown algae, was observed to ameliorate hepatic inflammatory status by reducing the hepatic mRNA expressions of TNF-α and IL-1β (41). TFCP was a kind of crude polysaccharides derived from Tremella fuciformis. A recent study by Zhou et al. (42) found that TFCP could ameliorate inflammation in NAFLD mice via reducing the expressions of inflammation-associated genes (IL-1β, TLR4, TNF-α, and IL-6) and enhancing the HNF4α expression. Li et at (15) found that Chicory polysaccharide (CP) could improve inflammation in NAFLD rats by downregulating the expression of interferon regulatory factor 1 (Ifr1), which played a key role in hepatitis.
Lycium barbarum polysaccharide (LBP) has been shown to attenuate inflammation. One study showed that LBP treatment significantly decreased pro-inflammatory mediators (IL-6, and TNF-α) expressions, reduced NF-κB activity, and lowered the levels of NLRP3/6, indicating that the action of LBP was implicated in the regulation of NF-κB and NLRP3/6 pathways (43). Another study also confirmed its anti-inflammatory effect. Xiao et at (22) reported that LBP was able to reduce the levels of proinflammatory markers (TNF-α, IL-1β, and COX-2) by decreasing NF-κB activity. An acetylated Stropharia rugoso-annulata polysaccharide (ASRP) was found to exert anti-inflammatory effect on NAFLD mice model via regulating JNK1/AP-1 signaling pathway. Li et al. (17) reported that ASRP treatment significantly decreased the levels of pro-inflammatory mediators (TNF-α, IL-1β, and IL-6) while increasing the content of anti-inflammatory mediators (IL-10). Additionally, ASRP also decreased p-JNK1, p-c-Jun and p-c-Fos expression.
Collectively, TLRs play an essential role in liver inflammation, and activated TLRs activate the cells and promote the release of proinflammatory cytokines that facilitate the progression of NAFLD, while the polysaccharides could interact with TLRs, and further regulate TLRs-mediated NF-κB and MAPK cascades, thus inhibiting the expression of proinflammatory cytokines and ameliorating inflammation (Figure 1).
Targeting gut-liver axis
The gut–liver axis represents a complex interplay between gut microbiota, intestinal barrier and liver, and its homeostasis plays a critical role in human health (44–46). The disruption of gut-liver axis, however, often contributes to the pathogenesis of liver diseases. For example, NALFD is the most common chronic liver disease, and diet, drug or other environmental factors may lead to flora disequilibrium or intestinal barrier damage, favoring the occurrence of lipid metabolic dysfunction, oxidative stress, low-grade inflammation, thereby contributing to the onset and progression of this disease. Therefore, prebiotics and probiotics capable of modulating gut microbiota and maintaining intestinal barrier integrity may be promising therapeutic agents for NAFLD.
Polysaccharides could improve NAFLD through modulating the ecological balance of gut microbiota. Hong et al. (47) evaluated the therapeutic effect of Astragalus polysaccharides (APS) on HFD-induced NAFLD mice. Studies showed that APS significantly increased the abundance and uniformity of intestinal bacteria. Of note, the richness of D. vulgaris from Desulfovibrio genus was also improved, which showed a potent anti-NAFLD effect by regulating lipid metabolism genes. Similarly, Wang et al. (48) used HFD-induced NAFLD mice model to investigate the effect and mechanism of Ophiopogon polysaccharide (MDG-1). Results indicated that MDG-1 could restore gut microbiota balance and improve relative abundance of beneficial bacteria, thus ameliorating hepatic steatosis.
Polysaccharides could improve NAFLD through protecting the intestinal barrier. Ye et al. (49) evaluated the therapeutic effect of Poria cocos polysaccharides (PCP) on HFD-induced NASH mice. It was found that PCP reduced pyroptosis-driven gut vascular barrier disruption and entry of endotoxin into the circulation caused by a high-fat diet, thereby ameliorating the inflammation. In addition, another study by Zhang et al. (50) found that Artemisia sphaerocephala Krasch polysaccharide (ASKP) could exert beneficial effect on high fructose-induced NAFLD mice by increasing the relative abundance of Akkermansia, which was responsible for the reduced intestinal permeability and LPS leakage.
Polysaccharides could improve NAFLD through regulating the production of short-chain fatty acids (SCFAs). An water insoluble polysaccharide from Poria cocos (WIP) was found to significantly increase the relative abundance of butyrate-producing bacteria in NAFLD mice (51), while butyrate could enhance the expression of mucosal integrity proteins (Muc-5) and tight junction proteins in the ileum, to maintain the integrity of the intestinal barrier and prevent harmful bacteria from entering the liver. In another study, Li et al. (52) found that in NAFLD mice pectin could dose-dependently increase the contents of SCFAs including acetic acid and propionic acid, which may account for the beneficial effect of pectin on preventing NAFLD.
Polysaccharides could ameliorate NAFLD through modulating bile acids metabolism. Zhong et al. (53) investigated the therapeutic effect of Ganoderma lucidum polysaccharide peptide (GLPP) (polysaccharide-peptide ratio of 95%: 5%) on NAFLD. Results showed that GLPP increased the synthesis of bile acids through enhancing cholesterol 7-alpha-monooxygenase (CYP7A1) and 25-hydroxycholesterol 7-alpha-hydroxylase (CYP8B1) expression, while the increased bile acids promoted the farnesoid X receptor- small heterodimer partner (FXR-SHP)/fibroblast growth factor (FGF) pathway, which finally inhibited fatty acid synthesis and thus improved the steatosis in NAFLD.
From all these studies it becomes evident that polysaccharides can ameliorate NAFLD through regulating gut–liver axis at multiple interrelated levels (Figure 2). Polysaccharides could exert therapeutic effect via restoring intestinal microecological homeostasis. In addition, polysaccharides could reduce LPS translocation through improving intestinal barrier function, to mitigate hepatic inflammation. SCFAs, produced as by-products of polysaccharides metabolism by gut microbiota, are not only an important fuel for the body but also serve as signaling molecules involved in lipid metabolism and inflammation. Polysaccharide could regulate the relative abundance of certain SCFA-producing bacteria to affect the development of NAFLD. The metabolism of bile acid is closely associated with the homeostasis of liver, while polysaccharides could affect the metabolic homeostasis of bile acids and thereby show beneficial therapeutic effect. Therefore, polysaccharides may function as prebiotics for NAFLD treatment.
Conclusion and prospects
Considering that the burden of end-stage liver disease will increase two- to three-fold worldwide by 2030 and NAFLD is a primary cause of end-stage liver disease and liver transplantation, slowing NAFLD progression or reversing this disease is becoming considerably imperative. Fortunately, natural polysaccharides provide a promising therapeutic alternative. Numerous preclinical trials have demonstrated that polysaccharides possess a clear and significant anti-NAFLD effect by targeting multiple mechanisms involving improvement of glucose and lipid metabolism, antioxidation, anti-inflammation, and regulation of liver-gut axis. More importantly, some polysaccharides could ameliorate NAFLD by simultaneously targeting different mechanisms with almost no side effects, thus showing intriguing potential as novel anti-NAFLD drugs or agents.
However, there are still some big challenges to face. On the one hand, polysaccharides are high-molecular weight compounds with a very complex structure, which makes quality control a big obstacle, thus overshadowing their health benefits; on the other hand, most of the polysaccharides in Table 1 are crude polysaccharides, most of which exert anti-NAFLD effect in a dose-dependent way. Apart from the actually effective components, glycoproteins, glycolipids or other structurally different polysaccharides may also be present in the mixture, which explains in part why a high-dose polysaccharides administration is usually required in preclinical studies. Of note, these impurities may also increase the potential risks or toxicity, such as hepatotoxicity or nephrotoxicity. And these adverse effects cannot be neglected in toxicological studies. Additionally, the anti-NAFLD effect of polysaccharide is closely associated with its structure. To better understand the effects of polysaccharides on NAFLD, obtaining homogeneous polysaccharides with detailed structural characteristics is particularly warranted, which is favorable to the exploration of structure–activity relationship of anti-NAFLD polysaccharides and unveil the target of anti-NAFLD action. Last but not least, the effectiveness of polysaccharide in current studies is mostly acknowledged in a preclinical stage. Therefore, systematic evaluation involving their actual efficacy and underlying mechanisms in clinic is critically essential. In the end, a myriad of effort is still needed before polysaccharides could become commercialized anti-NAFLD drugs or agents.
Author contributions
All authors listed have made a substantial, direct, and intellectual contribution to the work and approved it for publication.
Funding
This work was financially supported by China Postdoctoral Science Fund Project (2021M690936), and Miao Pu Research Funding of Henan University of Chinese Medicine (MP2021-27 and MP2021-15).
Conflict of interest
The authors declare that the research was conducted in the absence of any commercial or financial relationships that could be construed as a potential conflict of interest.
Publisher’s note
All claims expressed in this article are solely those of the authors and do not necessarily represent those of their affiliated organizations, or those of the publisher, the editors and the reviewers. Any product that may be evaluated in this article, or claim that may be made by its manufacturer, is not guaranteed or endorsed by the publisher.
References
1.Riazi, K, Azhari, H, Charette, JH, Underwood, FE, King, JA, Afshar, EE, et al. The prevalence and incidence of NAFLD worldwide: a systematic review and meta-analysis. Lancet Gastroenterol. (2022) 7:851–61. doi: 10.1016/s2468-1253(22)00165-0
2.Noureddin, M, Zhang, A, and Loomba, R. Promising therapies for treatment of nonalcoholic steatohepatitis. Expert Opin Emerg Drugs. (2016) 21:343–57. doi: 10.1080/14728214.2016.1220533
3.Ji, L, Li, Q, He, Y, Zhang, X, Zhou, Z, Gao, Y, et al. Therapeutic potential of traditional Chinese medicine for the treatment of NAFLD: a promising drug Potentilla discolor Bunge. Acta Pharm Sin B. (2022) 12:3529–47. doi: 10.1016/j.apsb.2022.05.001
4.Sumida, Y, and Yoneda, M. Current and future pharmacological therapies for NAFLD/NASH. J Gastroenterol. (2018) 53:362–76. doi: 10.1007/s00535-017-1415-1
5.Shen, K, Singh, AD, Modaresi Esfeh, J, and Wakim-Fleming, J. Therapies for non-alcoholic fatty liver disease: a 2022 update. World J Hepatol. (2022) 14:1718–29. doi: 10.4254/wjh.v14.i9.1718
6.Wang, K, Cai, M, Sun, S, Cheng, W, Zhai, D, Ni, Z, et al. Therapeutic prospects of polysaccharides for ovarian cancer. Front Nutr. (2022) 9:879111. doi: 10.3389/fnut.2022.879111
7.Kong, Y, Hu, Y, Li, J, Cai, J, Qiu, Y, and Dong, C. Anti-inflammatory effect of a novel pectin polysaccharide from Rubus chingii Hu on colitis mice. Front Nutr. (2022) 9:868657. doi: 10.3389/fnut.2022.868657
8.Hou, C, Chen, L, Yang, L, and Ji, X. An insight into anti-inflammatory effects of natural polysaccharides. Int J Biol Macromol. (2020) 153:248–55. doi: 10.1016/j.ijbiomac.2020.02.315
9.Wang, M, Lu, S, Zhao, H, Liu, Z, Sheng, K, and Fang, J. Natural polysaccharides as potential anti-fibrotic agents: a review of their progress. Life Sci. (2022) 308:120953. doi: 10.1016/j.lfs.2022.120953
10.Tessari, P, Coracina, A, Cosma, A, and Tiengo, A. Hepatic lipid metabolism and non-alcoholic fatty liver disease. Nutr Metab Cardiovasc Dis. (2009) 19:291–302. doi: 10.1016/j.numecd.2008.12.015
11.Li, M, Chi, X, Wang, Y, Setrerrahmane, S, Xie, W, and Xu, H. Trends in insulin resistance: insights into mechanisms and therapeutic strategy. Signal Transduct Target Ther. (2022) 7:216. doi: 10.1038/s41392-022-01073-0
12.Zeng, S, Chen, Y, Wei, C, Tan, L, Li, C, Zhang, Y, et al. Protective effects of polysaccharide from Artocarpus heterophyllus lam. (jackfruit) pulp on non-alcoholic fatty liver disease in high-fat diet rats via PPAR and AMPK signaling pathways. J Funct Foods. (2022) 95:105195. doi: 10.1016/j.jff.2022.105195
13.Wang, G, Zhang, Y, Zhang, R, Pan, J, Qi, D, Wang, J, et al. The protective effects of walnut green husk polysaccharide on liver injury, vascular endothelial dysfunction and disorder of gut microbiota in high fructose-induced mice. Int J Biol Macromol. (2020) 162:92–106. doi: 10.1016/j.ijbiomac.2020.06.055
14.Wu, Y, Zhou, F, Jiang, H, Wang, Z, Hua, C, and Zhang, Y. Chicory (Cichorium intybus L.) polysaccharides attenuate high-fat diet induced non-alcoholic fatty liver disease via AMPK activation. Int J Biol Macromol. (2018) 118:886–95. doi: 10.1016/j.ijbiomac.2018.06.140
15.Li, S, Wu, Y, Jiang, H, Zhou, F, Ben, A, Wang, R, et al. Chicory polysaccharides alleviate high-fat diet-induced non-alcoholic fatty liver disease via alteration of lipid metabolism- and inflammation-related gene expression. Food Sci Hum Well. (2022) 11:954–64. doi: 10.1016/j.fshw.2022.03.025
16.Li, M, Ma, J, Ahmad, O, Cao, Y, Wang, B, He, Q, et al. Lipid-modulate activity of Cichorium glandulosum Boiss. Et Huet polysaccharide in nonalcoholic fatty liver disease larval zebrafish model. J Pharm Sci. (2018) 138:257–62. doi: 10.1016/j.jphs.2018.09.012
17.Li, X, Cui, W, Cui, Y, Song, X, Jia, L, and Zhang, J. Stropharia rugoso-annulata acetylated polysaccharides alleviate NAFLD via Nrf2/JNK1/AMPK signaling pathways. Int J Biol Macromol. (2022) 215:560–70. doi: 10.1016/j.ijbiomac.2022.06.156
18.Huang, S, Pang, D, Li, X, You, L, Zhao, Z, Cheung, PCK, et al. A sulfated polysaccharide from Gracilaria lemaneiformis regulates cholesterol and bile acid metabolism in high-fat diet mice. Food Funct. (2019) 10:3224–36. doi: 10.1039/c9fo00263d
19.He, D, Yan, L, Zhang, J, Li, F, Wu, Y, Su, L, et al. Sargassum fusiforme polysaccharide attenuates high-sugar-induced lipid accumulation in HepG2 cells and Drosophila melanogaster larvae. Food Sci Nutr. (2021) 9:5590–9. doi: 10.1002/fsn3.2521
20.Ren, R, Gong, J, Zhao, Y, Zhuang, X, Ye, Y, and Lin, W. Sulfated polysaccharides from Enteromorpha prolifera suppress SREBP-2 and HMG-CoA reductase expression and attenuate non-alcoholic fatty liver disease induced by a high-fat diet. Food Funct. (2017) 8:1899–904. doi: 10.1039/C7FO00103G
21.Ren, R, Yang, Z, Zhao, A, Huang, Y, Lin, S, Gong, J, et al. Sulfated polysaccharide from Enteromorpha prolifera increases hydrogen sulfide production and attenuates non-alcoholic fatty liver disease in high-fat diet rats. Food Funct. (2018) 9:4376–83. doi: 10.1039/C8FO00518D
22.Xiao, J, Xing, F, Huo, J, Fung, ML, Liong, EC, Ching, YP, et al. Lycium barbarum polysaccharides therapeutically improve hepatic functions in non-alcoholic steatohepatitis rats and cellular steatosis model. Sci Rep. (2014) 4:5587. doi: 10.1038/srep05587
23.Jia, L, Li, W, Li, J, Li, Y, Song, H, Luan, Y, et al. Lycium barbarum polysaccharide attenuates high-fat diet-induced hepatic steatosis by up-regulating SIRT1 expression and deacetylase activity. Sci Rep. (2016) 6:36209. doi: 10.1038/srep36209
24.Yan, Z, Fan, R, Yin, S, Zhao, X, Liu, J, Li, L, et al. Protective effects of Ginkgo biloba leaf polysaccharide on nonalcoholic fatty liver disease and its mechanisms. Int J Biol Macromol. (2015) 80:573–80. doi: 10.1016/j.ijbiomac.2015.05.054
25.Sun, WM, Wang, YP, Duan, YQ, Shang, H-X, and Cheng, W-D. Radix hedysari polysaccharide suppresses lipid metabolism dysfunction in a rat model of non-alcoholic fatty liver disease via adenosine monophosphate-activated protein kinase pathway activation. Mol Med Rep. (2014) 10:1237–44. doi: 10.3892/mmr.2014.2327
26.Wang, J, Zheng, D, Huang, F, Zhao, A, Kuang, J, Ren, Z, et al. Theabrownin and Poria cocos polysaccharide improve lipid metabolism via modulation of bile acid and fatty acid metabolism. Front Pharmacol. (2022) 13:875549. doi: 10.3389/fphar.2022.875549
27.Delli Bovi, AP, Marciano, F, Mandato, C, Siano, MA, Savoia, M, and Vajro, P. Oxidative stress in non-alcoholic fatty liver disease. An updated mini review. Front Med. (2021) 8:595371. doi: 10.3389/fmed.2021.595371
28.Mu, S, Yang, W, and Huang, G. Antioxidant activities and mechanisms of polysaccharides. Chem Biol Drug Des. (2021) 97:628–32. doi: 10.1111/cbdd.13798
29.Xiao, J, Liong, EC, Ching, YP, Chang, RC, Fung, ML, Xu, AM, et al. Lycium barbarum polysaccharides protect rat liver from non-alcoholic steatohepatitis-induced injury. Nutr Diabetes. (2013) 3:e81. doi: 10.1038/nutd.2013.22
30.Zhang, F, Zhang, X, Gu, Y, Wang, M, Guo, S, Liu, J, et al. Hepatoprotection of Lycii Fructus polysaccharide against oxidative stress in hepatocytes and larval Zebrafish. Oxidative Med Cell Longev. (2021) 2021:3923625–14. doi: 10.1155/2021/3923625
31.Zhang, YN, Guo, YQ, Fan, YN, Tao, XJ, Gao, QH, and Yang, JJ. Lycium barbarum polysaccharides promotes mitochondrial biogenesis and energy balance in a NAFLD cell model. Chin J Integr Med. (2022) 28:975–82. doi: 10.1007/s11655-021-3309-6
32.Deng, X, Ke, X, Tang, Y, Luo, W, Dong, R, Ge, D, et al. Sagittaria sagittifolia polysaccharide interferes with arachidonic acid metabolism in non-alcoholic fatty liver disease mice via Nrf2/HO-1 signaling pathway. Biomed Pharmacother. (2020) 132:110806. doi: 10.1016/j.biopha.2020.110806
33.Hu, Y, Yin, F, Liu, Z, Xie, H, Xu, Y, Zhou, D, et al. Acerola polysaccharides ameliorate high-fat diet-induced non-alcoholic fatty liver disease through reduction of lipogenesis and improvement of mitochondrial functions in mice. Food Funct. (2020) 11:1037–48. doi: 10.1039/c9fo01611b
34.Zhang, Y, Wang, H, Zhang, L, and Yuan, YYD. Codonopsis lanceolata polysaccharide CLPS alleviates high fat/high sucrose diet-induced insulin resistance via anti-oxidative stress. Int J Biol Macromol. (2020) 145:944–9. doi: 10.1016/j.ijbiomac.2019.09.185
35.Yuan, S, Pan, Y, Zhang, Z, He, Y, Teng, Y, Liang, H, et al. Amelioration of the lipogenesis, oxidative stress and apoptosis of hepatocytes by a novel proteoglycan from Ganoderma lucidum. Biol Pharm Bull. (2020) 43:1542–50. doi: 10.1248/bpb.b20-00358
36.Yang, Z, Wang, J, Li, J, Xiong, L, Chen, H, Liu, X, et al. Antihyperlipidemic and hepatoprotective activities of polysaccharide fraction from Cyclocarya paliurus in high-fat emulsion-induced hyperlipidaemic mice. Carbohydr Polym. (2018) 183:11–20. doi: 10.1016/j.carbpol.2017.11.033
37.Albhaisi, S, and Noureddin, M. Current and potential therapies targeting inflammation in NASH. Front Endocrinol (Lausanne). (2021) 12:767314. doi: 10.3389/fendo.2021.767314
38.Huby, T, and Gautier, EL. Immune cell-mediated features of non-alcoholic steatohepatitis. Nat Rev Immunol. (2022) 22:429–43. doi: 10.1038/s41577-021-00639-3
39.Yue, S-R, Tan, Y-Y, Zhang, L, Zhang, B-J, Jiang, F-Y, Ji, G, et al. Gynostemma pentaphyllum polysaccharides ameliorate non-alcoholic steatohepatitis in mice associated with gut microbiota and the TLR2/NLRP3 pathway. Front Endocrinol. (2022) 13:13. doi: 10.3389/fendo.2022.885039
40.Zhong, M, Yan, Y, Yuan, HAR, Xu, G, Cai, F, Yang, Y, et al. Astragalus mongholicus polysaccharides ameliorate hepatic lipid accumulation and inflammation as well as modulate gut microbiota in NAFLD rats. Food Funct. (2022) 13:7287–301. doi: 10.1039/d2fo01009g
41.Heeba, GH, and Morsy, MA. Fucoidan ameliorates steatohepatitis and insulin resistance by suppressing oxidative stress and inflammatory cytokines in experimental non-alcoholic fatty liver disease. Environ Toxicol Pharmacol. (2015) 40:907–14. doi: 10.1016/j.etap.2015.10.003
42.Khan, TJ, Xu, X, Xie, X, Dai, X, Sun, P, Xie, Q, et al. Tremella fuciformis crude polysaccharides attenuates steatosis and suppresses inflammation in diet-induced NAFLD mice. Curr Issues Mol Biol. (2022) 44:1224–34. doi: 10.3390/cimb44030081
43.Xiao, J, Wang, F, Liong, EC, So, KF, and Tipoe, GL. Lycium barbarum polysaccharides improve hepatic injury through NFkappa-B and NLRP3/6 pathways in a methionine choline deficient diet steatohepatitis mouse model. Int J Biol Macromol. (2018) 120:1480–9. doi: 10.1016/j.ijbiomac.2018.09.151
44.Chen, X, Liu, M, Tang, J, Wang, N, Feng, Y, and Ma, H. Research Progress on the therapeutic effect of polysaccharides on non-alcoholic fatty liver disease through the regulation of the gut-liver Axis. Int J Mol Sci. (2022) 23:23. doi: 10.3390/ijms231911710
45.Gan, L, Wang, J, and Guo, Y. Polysaccharides influence human health via microbiota-dependent and -independent pathways. Front Nutr. (2022) 9:9. doi: 10.3389/fnut.2022.1030063
46.Grabherr, F, Grander, C, Effenberger, M, Adolph, TE, and Tilg, H. Gut dysfunction and non-alcoholic fatty liver disease. Front Endocrinol (Lausanne). (2019) 10:611. doi: 10.3389/fendo.2019.00611
47.Hong, Y, Sheng, L, Zhong, J, Tao, X, Zhu, W, Ma, J, et al. Desulfovibrio vulgaris, a potent acetic acid-producing bacterium, attenuates nonalcoholic fatty liver disease in mice. Gut Microbes. (2021) 13:1–20. doi: 10.1080/19490976.2021.1930874
48.Wang, X, Shi, L, Wang, X, Feng, Y, and Wang, Y. MDG-1, an Ophiopogon polysaccharide, restrains process of non-alcoholic fatty liver disease via modulating the gut-liver axis. Int J Biol Macromol. (2019) 141:1013–21. doi: 10.1016/j.ijbiomac.2019.09.007
49.Ye, H, Ma, S, Qiu, Z, Huang, S, Deng, G, Li, Y, et al. Poria cocos polysaccharides rescue pyroptosis-driven gut vascular barrier disruption in order to alleviates non-alcoholic steatohepatitis. J Ethnopharmacol. (2022) 296:115457. doi: 10.1016/j.jep.2022.115457
50.Zhang, B, Ren, D, Zhao, Y, Liu, Y, Zhai, X, and Yang, X. Artemisia sphaerocephala Krasch polysaccharide prevents hepatic steatosis in high fructose-fed mice associated with changes in the gut microbiota. Food Funct. (2019) 10:8137–48. doi: 10.1039/c9fo01890e
51.SUN, SS, WANG, K, MA, K, BAO, L, and LIU, H-W. An insoluble polysaccharide from the sclerotium of Poria cocos improves hyperglycemia, hyperlipidemia and hepatic steatosis in Ob/Ob mice via modulation of gut microbiota. Chin J Nat Med. (2019) 17:3–14. doi: 10.1016/S1875-5364(19)30003-2
52.Li, W, Zhang, K, and Yang, H. Pectin alleviates high fat (lard) diet-induced nonalcoholic fatty liver disease in mice: possible role of short-chain fatty acids and gut microbiota regulated by pectin. J Agric Food Chem. (2018) 66:8015–25. doi: 10.1021/acs.jafc.8b02979
Keywords: polysaccharides, NAFLD, NASH, therapy, liver diseases
Citation: Hu Y-l, Ma Q, Dong X, Kong Y, Cai J, Li J and Dong C (2023) Research progress on the therapeutic effects of polysaccharides on non-alcoholic fatty liver diseases. Front. Nutr. 10:1107551. doi: 10.3389/fnut.2023.1107551
Edited by:
Warintorn Ruksiriwanich, Chiang Mai University, ThailandReviewed by:
Feng Zhou, China Agricultural University, ChinaChuda Chittasupho, Chiang Mai University, Thailand
Thanongsak Chaiyaso, Chiang Mai University, Thailand
Copyright © 2023 Hu, Ma, Dong, Kong, Cai, Li and Dong. This is an open-access article distributed under the terms of the Creative Commons Attribution License (CC BY). The use, distribution or reproduction in other forums is permitted, provided the original author(s) and the copyright owner(s) are credited and that the original publication in this journal is cited, in accordance with accepted academic practice. No use, distribution or reproduction is permitted which does not comply with these terms.
*Correspondence: Yu-long Hu, aHV5dWxvbmcwMDFAMTYzLmNvbQ==; Chunhong Dong, Y2h1bmhvbmdfZG9uZ0BoYWN0Y20uZWR1LmNu