- 1School of Public Health and Health Management, Gannan Medical University, Ganzhou, China
- 2Key Laboratory of Environment and Health of Ganzhou, Gannan Medical University, Ganzhou, China
- 3Key Laboratory of Pollution Exposure and Health Intervention of Zhejiang, College of Biology and Environmental Engineering, Zhejiang Shuren University, Hangzhou, China
- 4State Key Laboratory of Food Science and Technology, Nanchang University, Nanchang, China
Natural polysaccharides (NPs) possess numerous health-promoting effects, such as liver protection, kidney protection, lung protection, neuroprotection, cardioprotection, gastrointestinal protection, anti-oxidation, anti-diabetic, and anti-aging. Nuclear factor erythroid 2-related factor 2 (Nrf2) antioxidant pathway is an important endogenous antioxidant pathway, which plays crucial roles in maintaining human health as its protection against oxidative stress. Accumulating evidence suggested that Nrf2 antioxidant pathway might be one of key regulatory targets for the health-promoting effects of NPs. However, the information concerning regulation of NPs on Nrf2 antioxidant pathway is scattered, and NPs show different regulatory behaviors in their different health-promoting processes. Therefore, in this article, structural features of NPs having regulation on Nrf2 antioxidant pathway are overviewed. Moreover, regulatory effects of NPs on this pathway for health-promoting effects are summarized. Furthermore, structure-activity relationship of NPs for health-promoting effects by regulating the pathway is preliminarily discussed. Otherwise, the prospects on future work for regulation of NPs on this pathway are proposed. This review is beneficial to well-understanding of underlying mechanisms for health-promoting effects of NPs from the view angle of Nrf2 antioxidant pathway, and provides a theoretical basis for the development and utilization of NPs in promoting human health.
Introduction
Oxidative stress, an imbalance between production of oxidants and antioxidant defenses, participates in the occurrences and progressions of many diseases (1). Nuclear factor erythroid 2-related factor 2 (Nrf2) is one of the most important endogenous anti-oxidative stress pathways, which has been demonstrated to involve in modulating oxidative stress for maintaining body health, like cardioprotection (2), neuroprotection (3), anti-aging (4), gastrointestinal protection (5), and kidney protection (6). As shown in Figure 1 (7, 8), under basal conditions, Nrf2 binds to Kelch-like epichlorohydrin-associated protein-1 (Keap1) in the cytoplasm through Cul3 ubiquitin ligase containing E3 to maintain cell homeostasis. In response to oxidative stress, Nrf2 is activated upon dissociation from Keap1. Then, Nrf2 translocates quickly into nucleus and forms a necessary region for the dimer by binds to small musculoaponeurotic fibrosarcoma oncogene homolog (sMaf) protein. Subsequently, this region binds to antioxidant response elements (ARE) and activates the expressions of target genes, thereby regulates the transcriptional activities of phase II metabolic enzymes, antioxidant enzymes or drug transporters, for restoring intracellular redox homeostasis. Recently, a variety of natural products, such as polyphenols, flavonoids and polysaccharides, have been considered as modulators of Nrf2 antioxidant pathway (9, 10).
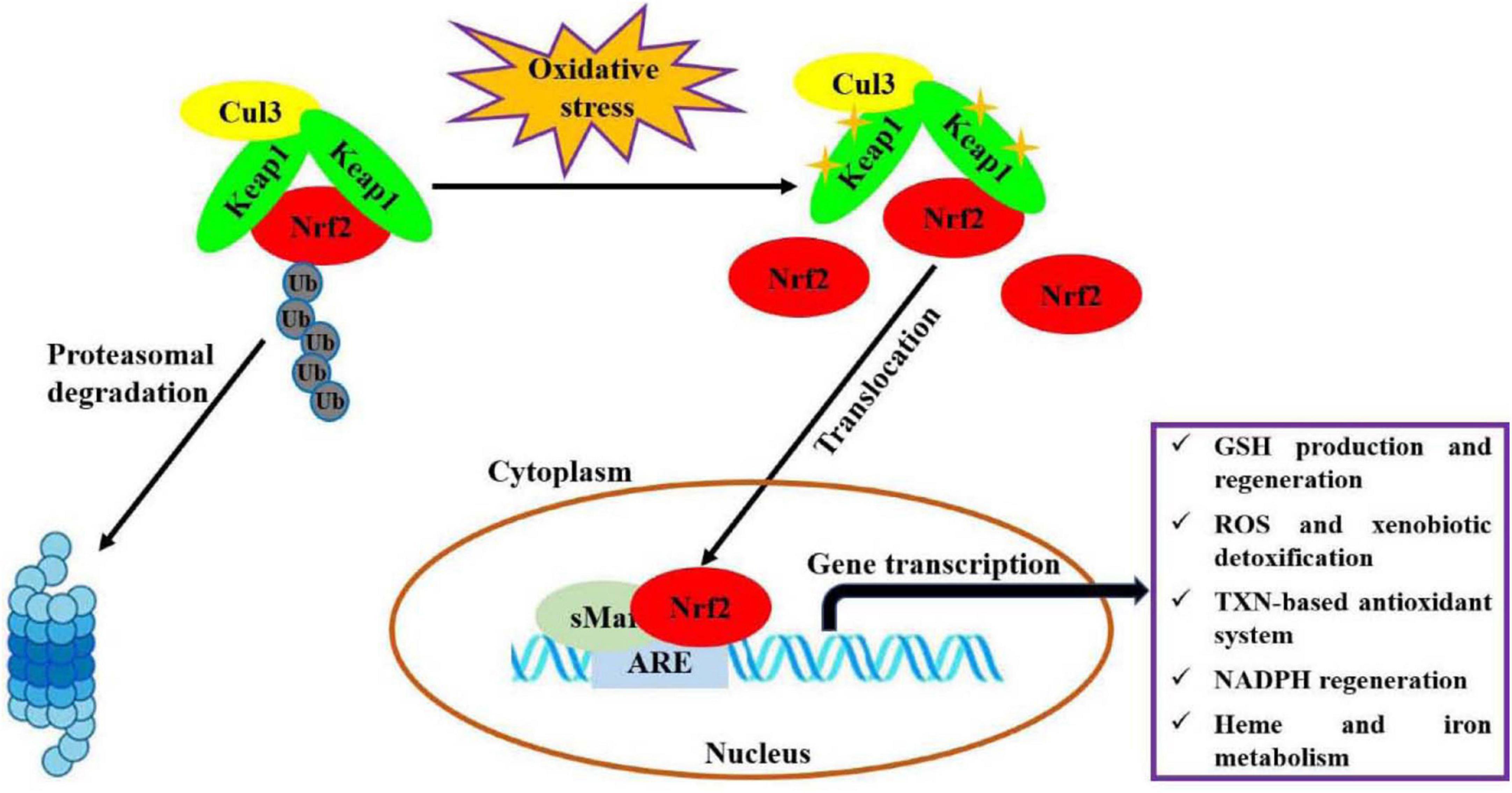
Figure 1. Molecular mechanism of Nrf2 signaling pathway regulating oxidative stress (7, 8). This figure is adapted from Transcriptional Regulation by Nrf2 by Claudia Tonelli et al., and NRF2, a Transcription Factor for Stress Response and Beyond by He et al., under CC BY 4.0.
Polysaccharides, a kind of biological macromolecules, are widely distributed in natural sources such as plants, algae and animals (11). Polysaccharides have attracted increasing attention owing to their diverse health-promoting effects, non-toxicity, extensive accessibility and renewability (12). Polysaccharides from natural resources (NPs) have been reported to play key roles in regulating excessive oxidative stress (13). In the past few decades, regulations of NPs on Nrf2 antioxidant pathway have been extensively studied in their health-promoting effects, such as liver protection (14), antioxidant (15), gastrointestinal protection (16), anti-diabetic (17), anti-aging (18), cardioprotection (19), lung protection (20), kidney protection (21), neuroprotection (22), anti-inflammation (23), immunomodulation (24), anti-depression (25), anti-cancer (26), improving reproductive function (27), anti-radiation (28), and anti-atherosclerosis (29). However, the information concerning regulation of NPs on Nrf2 antioxidant pathway is scattered, and NPs show different regulatory behaviors in their different health-promoting processes. Therefore, it is necessary to draw a summary on the recent developments on health-promoting effects of NPs from the viewing angle of regulation on Nrf2 antioxidant pathway.
In this review, structural features of NPs, having regulation on Nrf2 antioxidant pathway, from herbs, woody plants, algae, fungi, animals and bacteria, are overviewed. Moreover, regulatory effects of these NPs on the pathway for health-promoting effects in vitro and in vivo are systematically summarized. Furthermore, influences of structural characteristics like molecular weight (Mw), functional group, monosaccharide composition and side chains on the regulatory effects of NPs on Nrf2 antioxidant pathway are preliminarily discussed. Otherwise, the prospects on future work for regulation of NPs on Nrf2 antioxidant pathway are proposed.
Structural features of NPs having regulation on Nrf2 antioxidant pathway
According to Supplementary Table 1, NPs having regulation on Nrf2 antioxidant pathway can be isolated by water extraction (16, 30–33), ultrasonic-assisted extraction (34–36), ethanol precipitation (37–41) and enzymatic hydrolysis (24, 42–44). For acquiring homogeneous fractions, NPs can be further purified by stepwise ethanol precipitation (36, 45–47) and/or column chromatography (31, 42, 43, 48–53). Structural features including Mw, monosaccharide composition, glycosidic bond types, backbone, and side chains of the obtained NPs are shown in Supplementary Table 1.
Structural features of NPs from herbs
In terms of NPs having regulation on Nrf2 antioxidant pathway from herbs, Mw values of them have been determined to range from 2.273 to 2,617 kDa (41, 54–56). The polysaccharides were composed of fucose (Fuc), ribose (Rib), mannose (Man), glucose (Glc), arabinose (Ara), galactose (Gal), rhamnose (Rha), xylose (Xyl), glucuronic acid (GlcA), galacturonic acid (GalA), glucosamine (GlcN), and/or galactosamine (GalN) (18, 56–58). Moreover, Glc, Ara, Gal, and Rha were four monosaccharide types widely discovered in the polysaccharides (18, 41, 54, 55, 57–62).
Manp, Glcp, Rhap, Araf, Arap, Galp, GalAp, and Ribp sugar residues have been detected in the NPs from herbs. Manp residues exhibited as T-Manp-(1→, →3)-Manp-(1→, →4)-Manp-(1→, →6)-Manp-(1→, →3,6)-Manp-(1→ and →4,6)-Manp-(1→ (16, 47, 63). Glcp residues revealed as T-Glcp-(1→, →3)-Glcp-(1→, →4)-Glcp-(1→, →6)-Glcp-(1→, →2,4)-Glcp-(1→, →2,6)-Glcp-(1→, →3,4)-Glcp-(1→, →3,6)-Glcp-(1→ and →4,6)-Glcp-(1→ (16, 47, 57, 63). Rhap residues displayed as T-Rhap-(1→, →2)-Rhap-(1→, →3)-Rhap-(1→ and →2,4)-Rhap-(1→ (48, 54, 55, 57, 62, 64–66). Araf and Arap residues expressed as T-Araf-(1→, →2)-Araf-(1→, →4)-Araf-(1→, →5)-Araf-(1→, →3,5)-Araf-(1→ and T-Arap-(1→ (47, 48, 54, 55, 57, 62–66). Galp residues showed as T-Galp-(1→, →3)-Galp-(1→, →4)-Galp-(1→, →6)-Galp-(1→, →2,6)-Galp-(1→ and →3,6)-Galp-(1→ (47, 48, 54, 55, 57, 62, 64–66). GalAp residues manifested as T-GalAp-(1→ and →4)-GalAp-(1→ (48, 54, 55, 62, 66). Ribp residue exhibited as →4)-Ribp-(1→ (47). Moreover, T-Araf-(1→ (48, 54, 55, 57, 62–65), →5)-Araf-(1→ (48, 54, 55, 62, 64–66), →3)-Galp-(1→ (48, 55, 57, 62, 64–66), →3,6)-Galp-(1→ (48, 54, 55, 62, 64–66) and →4)-GalAp-(1→ (48, 54, 55, 62, 66) were five frequently determined residues in the polysaccharides.
Backbone of some polysaccharides with regulation on Nrf2 antioxidant pathway from herbs were composed of T-α-D-Glcp-(1→ (57), →4)-α/β-D-Glcp-(1→ (20, 63, 67–69), →6)-α-D-Glcp-(1→ (57), →3,4)-α-D-Glcp-(1→ (57), →3)-β-D-Galp-(1→ (64, 65), T-α-D-Araf-(1→ (57), →4)-β-D-Manp-(1→ (20, 40, 63, 67, 68), →4)-α-GalAp-(1→ (48, 54) and/or →2,4)-α-Rhap-(1→ (48) units as well as homogalacturonan (HG) (55, 62, 66) and/or rhamnogalacturonan I (RG-I) (62, 66) structures. While, side chains of them were made up of →3)-β-D-Glcp-(1→ (20, 63, 67, 68), →3)-β-D-Manp-(1→ (20, 63, 67, 68), →4)-α-D-Glcp-(1→ (69), →5)-β-D-Araf-(1→ (64, 65), →5)-α-L-Araf-(1→ (62), →2)-β-D-Araf-(1→ (64, 65), →6)-β-D-Galp-(1→ (64, 65), →3)-β-D-Galp-(1→ (64, 65), →2,4)-α-L-Rhap-(1→ (64, 65), T-α-L-Araf-(1→ (64, 65) and/or →3)-α-Rhap-(1→ (48) as well as RG-I (55), type I arabinogalactan (AG-I) (62, 66) and/or type II arabinogalactan (AG-II) (48, 62, 66) structures, which branched at O-6 or C-4 position of backbones.
Structural features of NPs from woody plants
To NPs having regulation on Nrf2 antioxidant pathway from woody plants, Mw of them were in the range of 4.568–3,440 kDa (70–74). The polysaccharides consisted of Fuc, Rib, Man, Glc, Ara, Gal, Rha, Xyl, GlcA, GalA, mannuronic acid (ManA), GlcN, and/or GalN (30, 75–78). Moreover, Man, Glc, Ara, Gal, and Rha were five monosaccharide types widely founded in the polysaccharides (45, 46, 76, 79–86).
Araf, Arap, Rhap, Galp, Glcp, Manp, Xylp, GalAp, and GlcAp sugar residues have been determined in the polysaccharides from woody plants. Araf residues exhibited as T-Araf-(1→, →2)-Araf-(1→, →4)-Araf-(1→, →5)-Araf-(1→, →2,5)-Araf-(1→ and →3,5)-Araf-(1→ (30, 46, 73, 74). Arap residues reflected as T-Arap-(1→, →4)-Arap-(1→, →3,4)-Arap-(1→ and →2,3,4)-Arap-(1→ (73, 74, 87–90). Rhap residues showed as T-Rhap-(1→, →2)-Rhap-(1→, →3)-Rhap-(1→ and →2,4)-Rhap-(1→ (30, 45, 87–90). Galp residues exerted as T-Galp-(1→, →2)-Galp-(1→, →3)-Galp-(1→, →4)-Galp-(1→, →6)-Galp-(1→, →2,6)-Galp-(1→, →3,4)-Galp-(1→, →3,6)-Galp-(1→ and →4,6)-Galp-(1→ (30, 45, 70, 87, 88, 91–96). Glcp residues revealed as T-Glcp-(1→, →2)-Glcp-(1→, →4)-Glcp-(1→, →6)-Glcp-(1→, →3,4)-Glcp-(1→ and →4,6)-Glcp-(1→ (49, 50, 93, 94). Manp residues behaved as T-Manp-(1→, →2)-Manp-(1→, →4)-Manp-(1→, →6)-Manp-(1→ and →3,6)-Manp-(1→ (46, 73, 74, 92). Xylp residues manifested as T-Xylp-(1→, →3)-Xylp-(1→ and →4)-Xylp-(1→ (73, 74, 87, 88). GalAp residues appeared as T-GalAp-(1→, →4)-GalAp-(1→, →2,4)-GalAp-(1→, →3,4)-GalAp-(1→ and →4,6)-GalAp-(1→ (77, 78, 91). GlcAp residue expressed as T-GlcAp-(1→ (91). Moreover, →4)-Glcp-(1→ (49, 50, 70, 73, 74, 87, 88, 91–96), T-Glcp-(1→ (49, 50, 70, 73, 74, 89–96), T-Araf-(1→ (30, 45, 72–74, 91, 93–96) and →3,4)-Galp-(1→ (30, 72, 77, 78, 91–96) were four residues commonly detected in the polysaccharides.
Backbone of some polysaccharides with regulation on Nrf2 antioxidant pathway from woody plants were comprised of →2)-α-D-Glcp-(1→ (49, 50), →4)-α-D-Glcp-(1→ (49, 50, 70, 95, 96), →6)-β-D-Glcp-(1→ (89, 90), →3)-α/β-D-Galp-(1→ (72, 77, 78, 95, 96), →4)-β-D-Galp-(1→ (70), →3,4)-α-D-Galp-(1→ (77, 78), →3)-β-D-Arap-(1→ (77, 78), →4)-α-L-Arap-(1→ (89, 90), →3,4)-α-L-Arap-(1→ (89, 90), →3,6)-Manp-(1→ (73, 74), →3)-α-L-Rhap-(1→ (89, 90), →2,4)-α-L-Rhap-(1→ (30) and/or →4)-α-D-GalAp-(1→ (30, 93, 94). While, the side chains of them were composed of α/β-D-Glcp-(1→ (49, 50, 70, 89, 90, 95, 96), →6)-α-D-Glcp-(1→ (49, 50), β-D-Galp-(1→ (72), →6)-α-D-Galp-(1→ (77, 78), →3,5,6)-β-D-Galf-(1→ (72), α-D-Manp-(1→ (70), →6)-β-D-Manp-(1→ (77, 78), α-L-Araf-(1→ (30, 72, 95, 96),→5)-α-L-Araf-(1→ (30), →3,5)-α-L-Araf-(1→ (30), and/or →4)-α-D-GalAp-6-OMe-(1→ (77, 78) residues, which branched at O-2, O-3, O-4, O-5, O-6, or C-4 position of backbones.
Structural features of NPs from algae
Regarding to NPs having regulation on Nrf2 antioxidant pathway from algae, structural features of them from Laminaria japonica (97, 98), Enteromorpha prolifera (24, 31), Sargassum fusiforme (99), Sargassum kjellmanianum (17), and Hizikia fusiforme (44) have been characterized. Their Mw values ranged from 4.929 to 250 kDa (24, 97). They were made up of Fuc, Man, Rha, Ara, Gal, Glc, Xyl, GlcA, GalA, ManA, and guluronic acid (GulA) (17, 44, 99). Comparatively, Fuc and Rha were two monosaccharide types widely detected in the polysaccharides (31, 44, 97, 99). Glycosidic bond types of above-mentioned polysaccharides have yet been ascertained. ESI-CID-MS/MS and NMR analysis have indicated that the sulfated polysaccharide from Enteromorpha prolifera possessed a backbone consisting of D-GlcUAp-α-(1→4)-3-sulfate-L-Rhap-β-(1→4)-3-sulfate-L-Rhap and D-GlcUAp-α-(1→4)-3-sulfate-L-Rhap-β-(1→4)-D-Xylp-β-(1→4)-3-sulfate-L-Rhap (100).
Structural features of NPs from fungi
For NPs having regulation on Nrf2 antioxidant pathway from fungi, Mw of them were in the range of 1.206–3,011.47 kDa (39, 51, 52, 101, 102). The polysaccharides were composed of Fuc, Man, Ara, Rha, Gal, Glc, Xyl, Rib, GalA, and GlcA (39, 103, 104). Moreover, Man, Gal, and Glc were three monosaccharide types commonly determined in the polysaccharides (32–35, 103–113).
Araf, Arap, Rhap, Galp, Glcp, Manp, Xylp, GalAp, GlcAp, and Ribp sugar residues have been characterized in the polysaccharides from fungi. Manp residues expressed as T-Manp-(1→, →2)-Manp-(1→, →3)-Manp-(1→, →4)-Manp-(1→ and →6)-Manp-(1→ (51, 52, 103). Glcp residues showed as T-Glcp-(1→, →3)-Glcp-(1→, →4)-Glcp-(1→, →6)-Glcp-(1→, →2,4)-Glcp-(1→, →3,4)-Glcp-(1→, →3,6)-Glcp-(1→ and →4,6)-Glcp-(1→ (32, 33, 51, 52, 103). Galp residues revealed as T-Galp-(1→, →2)-Galp-(1→, →3)-Galp-(1→, →4)-Galp-(1→, →6)-Galp-(1→, →2,6)-Galp-(1→, →3,6)-Galp-(1→ and →4,6)-Galp-(1→ (109, 111–113). Rhap residues exhibited as →4)-Rhap-(1→ and →6)-Rhap-(1→ (51, 52, 101). Arap, Xylp and GalAp residues displayed as →3)-Arap-(1→ (111), T-Xylp-(1→ (51, 52) and →4)-GalAp-(1→ (112, 113), successively. Moreover, T-Glcp-(1→ (32, 33, 51, 52, 103, 109, 112, 113), →3)-Glcp-(1→ (51, 52, 103, 110–113), →6)-Glcp-(1→ (101, 103, 110–113) and →6)-Galp-(1→ (32, 33, 39, 101, 103, 109–111) were four residues commonly detected in the polysaccharides.
Backbone of some polysaccharides with regulation on Nrf2 antioxidant pathway from fungi were made up of →3)-Glcp-(1→ (51, 52, 111–113), →4)-Glcp-(1→ (32, 33, 39), →6)-β-D-Glcp-(1→ (111–113), →3,4)-Glcp-(1→ (51, 52), →1,4)-Glcp-(6→ (39), →3)-α-D-Galp-(1→ (111), →4)-α-Galp-(1→ (112, 113), →6)-Galp-(1→ (32, 33, 39), →2)-α-Manp-(1→ (112, 113) and/or →4)-α-Manp-(1→ (112, 113). While, side chains of them were comprised of α/β-Glcp-(1→ (32, 33, 39, 112, 113), →3)-β-Glcp-(1→ (111–113), →6)-β-Glcp-(1→ (112, 113), T-α-D-Galp-(1→ (111),→4)-α-Galp-(1→ (112, 113), →3)-α-D-Manp-(1→ (111), →6)-β-D-Manp-(1→ (32, 33), →1)-Rhaf-(2→ (39), →3)-α-L-Arap-(1→ (111) and/or →4)-α-GalAp-(1→ (112, 113) units, which branched at O-3 and/or O-6 positions.
Structural features of NPs from animals and bacteria
In terms of NPs having regulation on Nrf2 antioxidant pathway from animals, structural features of polysaccharides from Holothuria leucospilota (114), Acaudina leucoprocta (115), and Ostrea talienwhanensis Crosse (42, 43) have been determined. Polysaccharide with a Mw of 52.80 kDa from Holothuria leucospilota was composed of GalN, Fuc, GlcA, Gal, Glc, and Xyl in a mass ratio of 39.08: 35.72: 10.72: 8.43: 4.23: 1.83 (114). Polysaccharide with a Mw of 202 kDa from Acaudina leucoprocta consisted of Man, GlcN, Rha, GlcA, GalN, Gal, and Fuc in a mass ratio of 2.04: 1.30: 3.57: 5.70: 18.73: 15.12: 65.81 (115). Polysaccharide with a Mw of 58 kDa from O. talienwhanensis Crosse was solely made up of Glc, which contained T-Glcp-(1→, →3)-Glcp-(1→, →4)-Glcp-(1→, →6)-Glcp-(1→, →2,4)-Glcp-(1→ and →4,6)-Glcp-(1→ residues (42, 43).
Regarding to NPs having regulation on Nrf2 antioxidant pathway from bacteria, structural features of high (37, 38) and low (53) Fuc polysaccharides from Bacillus megaterium have been characterized. The former was composed of Fuc, Glc, Man, Gal and GlcNAc in a relative percentage of 41.9: 26.6: 15.8: 12.2: 3.5, which possessed a backbone consisted of →4,6)-α-D-Manp-(1→, →2,4)-α-D-Manp-(1→, →4)-β-D-Glcp-(1→, →2,4)-β-D-Glcp-(1→ and →4)-β-D-GlcNAc with a branch composed of →2,4)-β-D-Galp-(1→, →4)-β-D-Galp-(1→ and →3)-α-L-Fuc4SO3p-(1→ (37, 38). The latter was composed of Gal, Ara, Man, Glc, Fuc and GlcNAc in a relative percentage of 37.6: 20.2: 19.3: 14.0: 4.9: 4.0, which had a backbone consisted of →4,6)-α-D-Manp-(1→, →4)-α-D-Manp-(1→, →4,6)-β-D-Glcp-(1→ and →2,4)-β-D-Glcp-(1→ with a branch composed of →1)-β-D-GlcNAcp, →1)-α-L-Fuc4SO3p, →4)-β-D-Galp(1→, →4,6)-β-D-Galp-(1→, →2,4)-β-D-Galp-(1→, →3,4)-β-L-Arap-(1→ and →3)-β-L-Arap-(1→ (53).
General information on structural features of NPs having regulation on Nrf2 antioxidant pathway
With above-mentioned summarizations, it could be concluded that the Mw of NPs having regulation on Nrf2 antioxidant pathway were in the range of 1.206–3,440 kDa. The NPs were mostly composed of Fuc, Rha, Ara, Gal, Glc and/or Man, and frequently consisted of T-Araf-(1→, →5)-Araf-(1→, →3)-Galp-(1→, →6)-Galp-(1→, →3,4)-Galp-(1→, →3,6)-Galp-(1→, T-Glcp-(1→, →3)-Glcp-(1→, →4)-Glcp-(1→, →6)-Glcp-(1→ and →4)-GalAp-(1→ residues. Moreover, →4)-Glcp-(1→, →6)-Glcp-(1→, →3)-Galp-(1→ and →4)-D-Manp-(1→ residues were commonly detected in their backbones, while α-L-Araf-(1→, →5)-α-L-Araf-(1→ and →6)-β-D-Galp-(1→ residues were usually found in side chains of NPs from herbs and woody plants. Some possible repeating structural units of NPs having regulation on Nrf2 antioxidant pathway, such as pectin, arabinogalactan, 2-O-acetylglucomannan, glucan and glucogalactan, have been speculated. A predicted structure of the repeating units for pectin purified from Codonopsis tangshen roots comprised HG as the backbone and RG-I structure as the side chains (55). An arabinogalactan structure from Lycium ruthenicum fruits possessed a backbone of →3)-β-Galp-(1→ residues, with branches of →5)-β-D-Araf-(1→, →2)-β-D-Araf-(1→, →6)-β-D-Galp-(1→, →3)-β-D-Galp-(1→, →2,4)-α-L-Rhap-(1→ and T-α-L-Araf-(1→ at O-6 position (64). A 2-O-acetylglucomannan from Dendrobium officinale stem had a backbone of →4)-β-D-Manp-(1→ and →4)-β-D-Glcp-(1→ residues, with branches at O-6 consisting of →3)-β-D-Glcp-(1→ and →3)-β-D-Manp-(1→, and substituted with acetyl groups at O-2 (63). A glucan units from Apios americana tubers was characterized to possess a main chain of →4)-α-D-Glcp-(1→ residues with a branched →4)-α-D-Glcp-(1→ chain (69). A glucogalactan from Anoectochilus zhejiangensis was determined to have a backbone consisted of →4)-β-D-Galp-(1→, →4,6)-α-D-Glcp-(1 → and →4)-α/β-D-Glcp-(1→, which branched with a single α-D-Glcp-(1→ at O-6 position (70).
However, the obtained purified NPs usually exhibited different structural features, owing to different methods and protocols used in above isolation and purification processes. Acidic polysaccharides (CPP-1 and CPSP-1; CTP-1 and CTSP-1) purified respectively from roots (55) and stems (66) of Codonopsis pilosula and Codonopsis tangshen had different Mw, monosaccharide composition, glycosidic bond types, backbone and side chains. Two purified fractions (TTP-1 and TVP) acquired from tubers (71) and vines (86) of Tetrastigma hemsleyanum revealed differences in Mw and monosaccharide composition. A low-fucose-content polysaccharide (LFC) (53) and a high-fucose-content one (HFC) (37, 38) were purified from the glucose mineral salts medium (GMSM) and one in GMSM-supplemented jute culture of Bacillus megaterium, and they displayed different Mw, monosaccharide composition, glycosidic bond types, backbone and side chains. Two polysaccharides (PNP80b-2 and PNP40c-1) were purified from water extracts of Pinus koraiensis pine nut by ethanol (80 and 40%, respectively) precipitation and same column chromatography procedures, and they were different in Mw, monosaccharide composition and glycosidic bond types (87–90). Two purified fractions (EPP80 and EPPS-3) from Echinacea purpurea were obtained by ultrasonic extraction and stepwise ethanol precipitation (36), and water extraction and column chromatography (116), respectively. EPP80 and EPPS-3 exhibited different Mw and monosaccharide composition. Two fractions (DRP1 and DRP2) from Dandelion root polysaccharides were obtained by column chromatography with water and 0.1 M NaCl elution, respectively, and they showed differences in Mw, monosaccharide composition, glycosidic bond types and backbone (57). Five purified fractions (PS-1, PS-2, PS-3, PS-4, and PS-5) were gained from Athyrium multidentatum subsequently eluted with 0, 0.1, 0.2, 0.3, and 0.4 M NaCl solutions, and they possessed different Mw and monosaccharide composition ratios (85). Two purified polysaccharides (CPP0.05 and CPP0.1) were obtained by eluting with 0.05 M and 0.1 M NaCl from Cyclocarya paliurus, and they behaved differences in Mw, monosaccharide composition, glycosidic bond types, backbone and side chains (72, 95, 96).
Regulation of NPs on Nrf2 antioxidant pathway for health-promoting effects
Regulation of NPs from herbs
Cell experiments have demonstrated that NPs from herbs could regulate Nrf2 antioxidant pathway for liver protection (14, 117–120), kidney protection (59, 60), lung protection (20), neuroprotection (22, 65, 121–123), cardioprotection (19, 124, 125), gastrointestinal protection (48, 55, 61, 62, 66, 126–128), anti-oxidation (85, 129–134), anti-diabetic (135–137), anti-aging (138–141), anti-inflammation (67, 69), anti-radiation (28, 142), and immunomodulation (143), as illustrated in Table 1.
Natural polysaccharides from herbs exerted liver protection against ethanol- (117, 118) or mixture of Cd + Cr + Pb + Mn + Zn + Cu-induced (14) L02 cells, isoniazid + rifampicin-induced HepG2 cells (119) and 5-fluorocrail (5-Fu)-induced MIHA cells (120), partly through modulating protein and mRNA expressions of Nrf2, HO-1, and NQO1, increasing protein expressions of GCLC and nuclear Nrf2, and decreasing protein and/or mRNA expressions of Keap1 and cytosol Nrf2. Those from Astragalus membranaceus (60) and Potentilla anserine (59) exhibited kidney protection on oxalate-induced HK-2 cells and cadmium-induced HEK293 cells, respectively, whose actions were related to regulation of Nrf2 protein expression, reduction of Keap1 and PGC-1α protein expressions and increment of SOD1 and CAT protein expressions. Polysaccharide from Dendrobium officinale showed lung protection in LPS-induced BEAS-2B cells involved with increases of HO-1 and NQO1 protein expressions as well as nuclear/cytosol Nrf2 ratio (20). NPs from herbs displayed neuroprotection against MPP+-induced N2a cells (22), H2O2- (121), UVB- (122), and OGD/R-induced (144) PC12 cells, OGD/R-induced primary cortical neurons (65), and H2O2-induced microglia BV2 cells (145) or HT22 cells (123), which were correlated with promotions of mRNA and protein expressions of Keap1, Nrf2, HO-1, NQO1, GCLC, GCLM, and GSTP1 along with nuclear Nrf2, and reduction of cytosol Nrf2 protein expression. Moreover, Salvia miltiorrhiza polysaccharides protected PC12 cells from OGD/R-induced ferroptosis and lipid peroxidation by activating Nrf2/HO-1 pathway (144). Polygonatum cyrtonema Hua polysaccharides alleviated ferroptosis in H2O2-induced microglia BV2 cells by activating Nrf2/HO-1 signaling pathway (145). NPs from herbs revealed cardioprotection on hypoxia/reoxygenation- (124), ischemia/reperfusion- (19) or H2O2-induced (125) H9c2 cells by modulating protein and mRNA expressions of Nrf2, HO-1, and NQO1 as well as nuclear and cytosol Nrf2. Those of herbs appeared gastrointestinal protection against RSL3-induced Caco-2 cells (127), H2O2- (126) or radiation-induced (128) IEC-6 cells and H2O2-induced IPEC-J2 cells (48, 55, 62, 66) or IPEC-1 cells (61), partly through modulating protein and mRNA expressions of Nrf2 and HO-1, elevating protein and mRNA expressions of NQO1, SOD, SOD1, CAT, GPX, nuclear, and cytosol Nrf2 along with cytosol HO-1, and decreasing Keap1 protein expression. Moreover, Astragalus polysaccharide exhibited inhibitory effect on ferroptosis in RSL3-induced Caco-2 cells and this effect was associated with the Nrf2/HO-1 pathway (127). NPs from herbs possessed anti-oxidation on H2O2-induced HUVECs (85), H2O2- (131) or LPS-induced (132) RAW264.7 cells, H2O2-induced MEFs cells (133), tert-butyl hydroperoxide- (130) or oleic acid-induced (129) HepG2 cells and H2O2-induced HEMs (134) via enhancing protein and mRNA expressions of Nrf2, HO-1, NQO1, GCLM, GCLC, and GST along with nuclear/cytosol Nrf2, and regulating protein and mRNA expressions of Keap1. Those from herbs exhibited anti-diabetic effect on palmitate-induced HepG2 cells (135), high glucose-induced ARPE-19 cells (136) or HRECs (146), and high-glucose- and high-insulin-induced 3T3-L1 adipocytes (137) by augmenting protein or mRNA expressions of p-Nrf2/Nrf2, Nrf2, HO-1, SOD2, CAT, and nuclear Nrf2 as well as nuclear translocation of p-Nrf2. NPs from herbs showed anti-aging activity against H2O2-induced chondrocytes (138), H2O2-induced ARPE-19 cells (139), ADM, ODM, ADM + H2O2, or ODM + H2O2-induced BMSCs cells (140), H2O2-induced MC3T3-E1 cells (141) through rising mRNA and/or protein expressions of Keap1, Nrf2, HO-1, and NQO1 as well as nuclear Nrf2. Polysaccharides from Dendrobium officinale (67) and Apios americana (69) produced anti-inflammation on LPS-induced RAW264.7 cells partly by adding protein and/or mRNA expressions of Keap1, Nrf2, HO-1 and NQO1. Polysaccharides from Lycium barbarum produced anti-radiation action on UVB-induced HSF and HaCaT cells via enlarging protein and/or mRNA expressions of Nrf2, p-Nrf2, HO-1, NQO1, GCLC, GCLM, SOD, AKR1C2, APOE, and HBEGF along with nuclear Nrf2 (28, 142). Meanwhile, Lycium barbarum polysaccharide caused immunomodulation in mycoplasma-infected splenic lymphocytes through increments of mRNA and protein expressions of Nrf2, HO-1, and NQO1 (143).
Animals experiments have demonstrated that NPs from herbs could regulate Nrf2 antioxidant pathway for liver protection (14, 36, 41, 54, 57, 67, 116, 120, 147–153), kidney protection (21, 59, 154–157), lung protection (20, 158), neuroprotection (22, 121), cardioprotection (124, 159), gastrointestinal protection (16, 40, 48, 127, 128, 160, 161), anti-oxidation (15, 47, 162–164), anti-diabetic (56, 58, 135, 146, 165, 166), anti-aging (18, 68, 84, 167), anti-inflammation (23), anti-depression (25), anti-cancer (168), and improving reproductive function (169, 170), as implied in Table 1.
Natural polysaccharides from herbs exerted liver protection against DSS- (67), acetaminophen- (57, 147), tilmicosin- (149), CCl4- (116, 150), ethanol- (36, 54, 148), isoniazid + rifampicin- (151), methionine and choline deficient diet- (152), mixture of Cd + Cr + Pb + Mn + Zn + Cu- (14), LPS- (153), high-fat diet- (41), and 5-Fu-induced (120) mice or rats, through increasing mRNA and protein expressions of Nrf2, nuclear Nrf2, NQO1, GCLC, GCLM, Cu/Zn-SOD, SOD1, and GPX1 in liver tissues, modulating protein and/or mRNA expressions of Keap1 and HO-1, and decreasing protein and/or mRNA expressions of cytosol Keap1, CAT, cytosol Nrf2, p67phox, p47phox, and p22phox in liver tissues. NPs from herbs exhibited kidney protection on LPS- (154, 155), lead- (156), florfenicol- (21), cadmium- (59), and STZ-induced (157) mice, rats or chicks via elevating mRNA and protein expressions of HO-1 and NQO1, regulating Nrf2 expression, and down-regulating mRNA and protein expressions of Keap1 and PGC-1α in kidney tissues. Those from herbs showed lung protection hyperoxia- (158) and DSS-induced (20) mice by enhancing activities and/or protein expressions of Nrf2, cytosol Nrf2, nuclear Nrf2, HO-1, and NQO1 in lung tissues as well as protein expressions of Nrf2 in PMVECs isolated from lung, and reducing protein expression of Keap1 in lung tissues. NPs from herbs reflected neuroprotection against ischemia-reperfusion- (171), CoCl2- (121), and MPTP-induced (22) mice or rats, which is related to increments of protein and/or mRNA expressions of nuclear Nrf2, Nrf2, HO-1 and NQO1 in retina or brain tissues. Those from herbs displayed cardioprotection on ischemia/reperfusion-induced (124) and adjuvant arthritis rats (159), involving with aggrandizement of protein expressions of nuclear and cytosol Nrf2, HO-1 and NQO1 in myocardial tissues, and declination of mRNA and/or protein expressions of Keap1, MAF, Nrf2, HO-1, and γ-GCS in heart tissues. NPs from herbs appeared gastrointestinal protection against MNNG- (161), DSS- (16, 40, 127), radiation- (128), and CTX-induced (160) mice or rats as well as aged mice (48), via up-regulating protein and/or mRNA expressions of nuclear and cytosol Nrf2, cytosol HO-1, NQO1, SOD, SOD1, SOD2, CAT, GPX, and GPX1, modulating protein and/or mRNA expressions of Nrf2 and HO-1, and down-regulating Keap1 protein expression in stomach, colon or jejunum tissues. Meanwhile, Astragalus polysaccharide inhibited ferroptosis of colonic tissue through Nrf2/HO-1 pathway in DSS-induced mice (127). NPs from herbs generated anti-oxidation effects on light exposure-induced mice (163), ethanol-induced mice (164), AAPH-induced zebrafish (47) as well as Jian carp (15) and zebrafish (162) through adding mRNA expressions of Nrf2, HO-1, Cu/Zn-SOD, GPX, GPX-3, CAT, SOD, Mn-SOD, GST, TrxR1, and p38, and modulating Keap1 mRNA expression in retinas, spleen or liver tissues. NPs from herbs produced anti-diabetic activity against high-fat diet- (135), high fat/high sucrose diet- (58) and high-fat diet + STZ-induced (56, 165, 166) mice via increasing protein and/or mRNA expressions of p-Nrf2/Nrf2, nuclear and cytosol Nrf2, Nrf2, HO-1, NQO1, SOD2, and CAT in liver or kidney tissues, and decreasing protein expressions of nuclear and cytosol Keap1 and NOX2 in liver tissues. Those from herbs caused anti-aging effects on ovariectomy or D-Gal-induced mice (18, 68, 84) and AD model APP/PS1 mice (167), through elevating mRNA and protein expressions of nuclear Nrf2, Nrf2, HO-1 and NQO1 in hippocampal, brain, and liver tissues, and reducing mRNA and/or protein expressions of Keap1 and cytosol Nrf2 in brain tissues. Lycium barbarum polysaccharide revealed anti-inflammation against cerulein-induced mice by adding nuclear Nrf2 protein expression and HO-1 activity in pancreas (23). Meanwhile, this polysaccharide implied anti-cancer action against ID-8 cells bearing-mice through up-regulation of mRNA and protein expressions of Keap1, Nrf2 and HO-1 in liver and kidney tissues (168). Polygonatum cyrtonema polysaccharide had anti-depression activity on LPS and CUMS-induced mice via increasing protein expressions of Nrf2 and HO-1 in hippocampal tissues (25). NPs from herbs possessed improving reproductive function against CTX-induced rats (169) and cisplatin-induced mice (170) by elevating protein and/or mRNA expressions of Nrf2, HO-1 and NQO1 in ovarian or testis tissues.
Regulation of NPs from woody plants
Cell experiments have indicated that NPs from woody plants could regulate Nrf2 antioxidant pathway for liver protection (30, 45, 46, 50, 70, 92, 172), kidney protection (173), gastrointestinal protection (86), neuroprotection (75), cardioprotection (78), anti-aging (74), anti-diabetic (174), anti-oxidation (72, 96, 175), and anti-inflammation (71), as showed in Table 2.
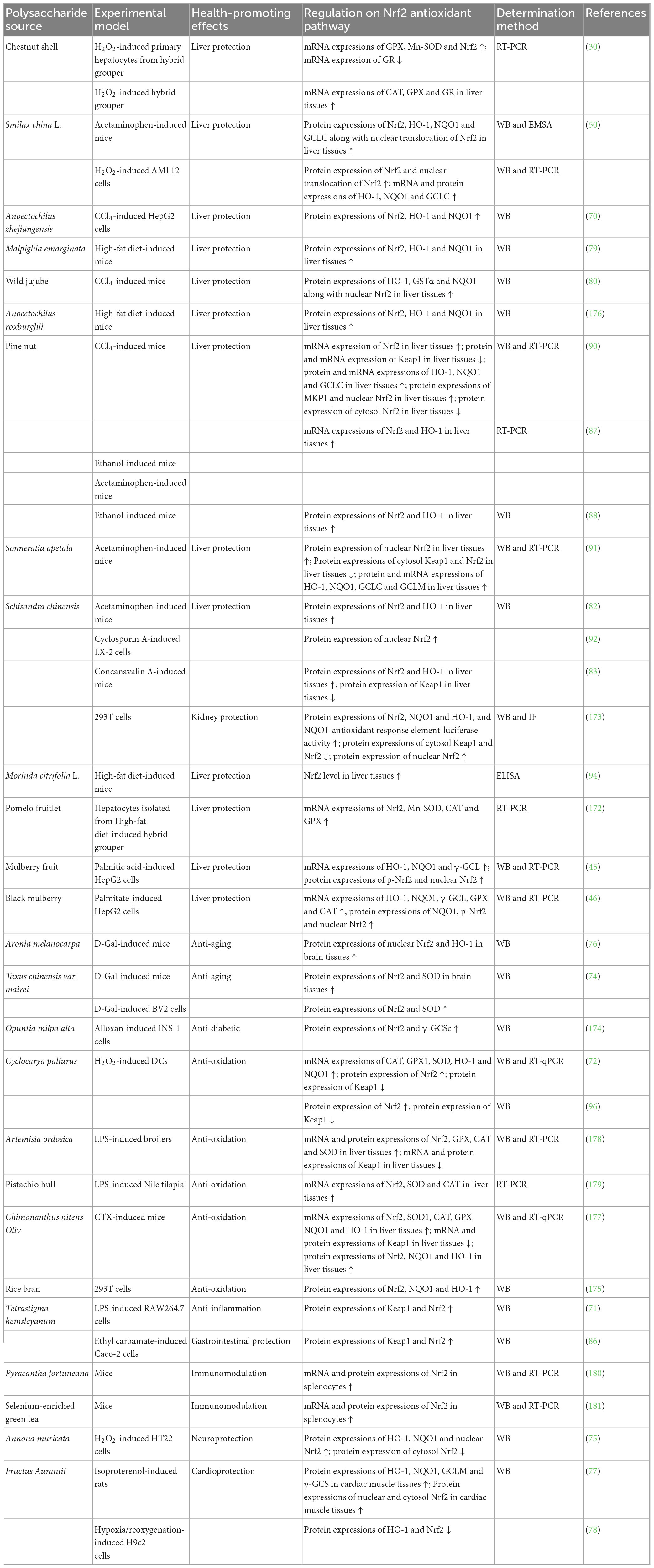
Table 2. Regulation of NPs from woody plants on Nrf2 antioxidant pathway for health-promoting effects.
Natural polysaccharides from woody plants exhibited liver protection against H2O2-induced primary hepatocytes from hybrid grouper (30), H2O2-induced AML12 cells (50), CCl4- (70) and palmitic acid-induced (45, 46) HepG2 cells, cyclosporin A-induced LX-2 cells (92), and hepatocytes isolated from high-fat diet-induced hybrid grouper (172), involving with increments of mRNA and protein expressions of p-Nrf2, nuclear Nrf2, Nrf2, HO-1, NQO1, γ-GCL, GCLC, Mn-SOD, GPX, and CAT as well as nuclear translocation of Nrf2, and reduction of GR mRNA expression. Schisandra chinensis polysaccharide generated kidney protection on 293T cells through increasing protein expressions of nuclear Nrf2, Nrf2, NQO1, and HO-1 along with NQO1-antioxidant response element-luciferase activity, and decreasing protein expressions of cytosol Keap1 and Nrf2 (173). NPs from Tetrastigma hemsleyanum showed gastrointestinal protection against ethyl carbamate-induced Caco-2 cells, by elevating protein expressions of Keap1 and Nrf2 (86). Polysaccharide from Annona muricata (75) caused neuroprotection on H2O2-induced HT22 cells via adding protein expressions of HO-1, NQO1 and nuclear Nrf2, and reducing cytosol Nrf2 protein expression. Fructus Aurantii polysaccharide produced cardioprotection against hypoxia/reoxygenation-induced H9c2 cells through lowering protein expressions of HO-1 and Nrf2 (78). Taxus chinensis var. mairei polysaccharide exerted anti-aging action on D-Gal-induced BV2 cells by promoting protein expressions of Nrf2 and SOD (74). Polysaccharide Opuntia milpa alta (174) revealed anti-diabetic activities against alloxan-induced INS-1 cells, which was related to enhancements of protein expressions of Nrf2 and γ-GCSc. NPs from woody plants displayed anti-oxidation effects on H2O2-induced DCs (72, 96) and 293T cells (175), partly by rising protein and/or mRNA expressions of Nrf2, CAT, GPX1, SOD, HO-1, and NQO1, and reducing Keap1 protein expression. Tetrastigma hemsleyanum polysaccharide reflected anti-inflammation on LPS-induced RAW264.7 cell via through improving protein expressions of Keap1 and Nrf2 (71).
Animal experiments have indicated that NPs from woody plants could regulate Nrf2 antioxidant pathway for liver protection (30, 50, 79, 80, 82, 83, 87, 88, 90, 91, 94, 172, 176), cardioprotection (77), anti-aging (74, 76), anti-oxidation (177–179), and immunomodulation (180, 181), as reflected in Table 2.
NPs from woody plants had liver protection against H2O2-induced hybrid grouper (30), acetaminophen-induced mice (50, 82, 87), high-fat diet-induced mice (79, 94, 176), CCl4-induced mice (80, 90), ethanol-induced mice (87, 88), and concanavalin A-induced mice (83), involving with increment of protein and/or mRNA expressions of nuclear Nrf2, Nrf2, HO-1, NQO1, GCLC, CAT, GSTα, GPX, GR, and MKP1 along with nuclear translocation of Nrf2, and reduction of protein and/or mRNA expressions of Keap1 and cytosol Nrf2 in liver tissues. Fructus Aurantii polysaccharide exerted cardioprotection against isoproterenol-induced rats via enhancing protein expressions of HO-1, NQO1, GCLM, γ-GCS, nuclear Nrf2, and cytosol Nrf2 in cardiac muscle tissues (77). Polysaccharides from Aronia melanocarpa (76) and Taxus chinensis var. mairei (74) revealed anti-aging activity on D-Gal-induced mice by up-regulating protein expressions of nuclear Nrf2, Nrf2, HO-1, and SOD in brain tissues. NPs from woody plants generated anti-oxidation effect against LPS-induced broilers (178) or Nile tilapia (179) and CTX-induced mice (177), which was related to enhancement of mRNA and protein expressions of Nrf2, NQO1, HO-1, GPX, CAT, SOD1, and SOD in liver tissues, intestines or larvae, and reduction of mRNA and protein expressions of Keap1 in liver tissues or larvae. Polysaccharides from Pyracantha fortuneana (180) and selenium-enriched green tea (181) reflected immunomodulation on mice through adding mRNA and protein expressions of Nrf2 in splenocytes.
Regulation of NPs from algae
The regulations of NPs on Nrf2 antioxidant pathway from algae in cell and animal experiments are revealed in Table 3.
Cell experiments have showed that NPs from algae could regulate Nrf2 antioxidant pathway for liver protection (182), lung protection (183), anti-diabetic (17), anti-oxidation (44, 184), and anti-cancer (26). Brown seaweed polysaccharide produced liver protection on acetaminophen-induced HL-7702 cells through increasing protein expressions of Nrf2 and nuclear Nrf2 (182). Laminaria digitate polysaccharide generated lung protection against H2O2-induced MRC-5 cells by up-regulating protein and/or mRNA expressions of nuclear Nrf2, Nrf2, HO-1, NQO1 and GCLC as well as nuclear translocation of Nrf2, and down-regulating Keap1 mRNA (183). Sargassum kjellmanianum polysaccharide exhibited anti-diabetic effect on H2O2-induced HUVECs via elevating protein expressions of Nrf2 and nuclear Nrf2, and declining cytosol Nrf2 protein expression (17). Polysaccharides from Padina boryana (184) and Hizikia fusiforme (44) showed anti-oxidation action against H2O2-induced Vero cells via adding protein expressions of cytosol Nrf2, Nrf2, CAT, and SOD, and reducing protein expression of cytosol Keap1. Fucus vesiculosus polysaccharide revealed anti-cancer activity on Ca9-22 and CAL27 cells through lowering mRNA expressions of Nrf2, TXN and HO-1 (26).
Animal experiments have showed that NPs from algae could regulate Nrf2 antioxidant pathway for liver protection (31, 97, 185), lung protection (186), kidney protection (97), gastrointestinal protection (187, 188), cardioprotection (189), anti-aging (98, 99), anti-oxidation (190, 191), and immunomodulation (24). NPs from algae possessed liver protection against CTX- (97), CCl4- (31), and high-fat diet-induced (185) mice, through increasing protein and/or expressions of p-Nrf2, nuclear Nrf2, cytosol Nrf2, p-Nrf2/Nrf2, HO-1, GCLM, NQO1, CAT, SOD2, Slc7a11, G6pd2, Prdx1, GPX2, and GPX4, and decreasing Keap1 protein expression in liver tissues. Ecklonia cave polysaccharide had lung protection on LPS-induced mice by enhancing protein expressions of Nrf2 and HO-1 in lung tissues (186). Laminaria japonica polysaccharide exhibited kidney protection against CTX-induced mice via up-regulating protein expressions of Nrf2, HO-1, GCLM, and NQO1 in kidney tissues (97). Meanwhile, this polysaccharide showed anti-aging effect on rotenone-induced rats through rising protein expressions of Nrf2 and PGC-1α in ventral midbrain (98). NPs from algae exerted gastrointestinal protection on heat stress-induced broilers (187) and aged mice (188) by elevating protein and/or mRNA expressions of Nrf2, NQO1, HO-1, CAT, SOD2, GPX1, and GSTT1 in intestinal tissues or duodenum. Padina tetrastromatica polysaccharide generated cardioprotection against isoproterenol-induced rats via enhancing protein and/or mRNA expressions of Nrf2 and nuclear Nrf2, and declining cytosol Nrf2 protein expression in heart tissues (189). Those from algae revealed anti-oxidation activity on heat stress-induced Gallus gallus domesticus (190) and D-gal-induced mice (191), through aggrandizing mRNA and protein expressions of Nrf2, HO-1, γ-GCS, NQO1, Cu/Zn-SOD, Mn-SOD, SOD2, GSTO1, and CAT in liver and spleen tissues. Enteromorpha prolifera polysaccharide reflected immunomodulation against aflatoxin B1-induced broilers by augmenting mRNA and/or protein expressions of SOD1, SOD2, GPX1, GPX3, CAT1, GSTT1, GSTO1, GSTA3, Nrf2, and HO-1 in bursa of fabricius (24).
Regulation of NPs from fungi
The regulations of NPs on Nrf2 antioxidant pathway from fungi in cell experiments and animal experiments are illustrated in Table 4.
Cell experiments have showed that NPs from fungi could regulate Nrf2 antioxidant pathway for liver protection (192), lung protection (33, 105), cardioprotection (193), gastrointestinal protection (113), anti-oxidation (194, 195), anti-aging (108, 196, 197), anti-diabetic (102, 198), anti-cancer (199), and anti-atherosclerosis (29). Antrodia camphorate polysaccharide exhibited liver protection on LPS-induced Kupffer cells by increasing protein expressions of Keap1, Nrf2, and γ-GCS (192). Polysaccharides from Sarcodon aspratus (105) and Morchella esculenta (33) exerted lung protection against H2O2-induced A549 cells via adding protein expressions of p-Nrf2 and HO-1, and reducing Nrf2 protein expression. Ganoderma lucidum polysaccharide showed cardioprotection on doxorubicin-induced H9c2 cells through rising protein expressions of Nrf2 and HO-1 (193). Ganoderma atrum polysaccharide reflected gastrointestinal protection in LPS-induced Caco-2/RAW264.7 co-culture inflammation model by up-regulating protein expressions of Keap1 and Nrf2 (113). NPs from fungi had anti-oxidation activity on H2O2-induced HSFs (194) and LPS-induced RAW264.7 cells (195) through augmenting protein and/or mRNA expressions of Nrf2, Gstm1, Gstt1, GCLC, GCLM, HO-1, and NQO1, and reducing Keap1 mRNA expression. Those from fungi displayed anti-aging effect against L-Glu-induced HT22 cells (108, 196) and UVA-induced HDF cells (197) via aggrandizing protein and/or mRNA expressions of nuclear Nrf2, Nrf2, SOD1, HO-1, NQO1, and GCLC, and lowering protein and/or mRNA expressions of Keap1 and cytosol Nrf2. Lentinus edodes mycelium polysaccharide had anti-diabetic action against high glucose-induced MIN6 or INS-1 cells, which was related to increment of nuclear Nrf2 protein expression (102, 198). Lachnum sp. polysaccharide possessed anti-cancer activity on HepG2 cells involved with reduction of Nrf2 protein expression, and enhancement of protein expression of Keap1, HO-1, NQO1, GST1, SOD2, GPX, and GCLM (199). Poria cocos polysaccharide caused anti-atherosclerosis effect on ox-LDL-induced VSMCs by rising protein expressions of HO-1 and nuclear Nrf2, and declining cytosol Nrf2 protein expression (29).
Animal experiments have demonstrated that NPs from fungi could regulate Nrf2 antioxidant pathway for liver protection (52, 103, 104, 107, 192, 200), lung protection (35), kidney protection (39), gastrointestinal protection (106, 201), anti-aging (109, 110, 196), anti-diabetic (101, 111), improving reproductive function (27), and immunomodulation (202, 203). NPs from fungi exhibited liver protection against LPS/D-GalN- (52, 192), ethanol- (103), high-fat diet- (200), CCl4- (107), and Toxoplasma gondii-induced (104) mice, through increment of protein and/or mRNA expressions of nuclear Nrf2, Nrf2, HO-1, NQO1, and γ-GCS, and modulation of Keap1 protein expression in liver tissues. Trametes orientalis polysaccharide exerted lung protection on PM2.5-induced mice by increasing protein expressions of Nrf2 and HO-1 in lung tissues (35). Cordyceps militaris polysaccharide showed kidney protection against Pb2+-induced mice via enhancing protein expressions of Keap1, Nrf2, HO-1, and NQO1 in kidney tissues (39). NPs from fungi possessed gastrointestinal protection on water immersion and restraint stress-induced rats (106) and 5-Fu-treated CT26 tumor-bearing mice (201), through elevating protein expressions of Nrf2, HO-1, NQO1, and NOX4, and reducing Keap1 protein expression in gastric or colon tissues. Polysaccharides from Inonotus obliquus (196), Amanita caesarea (109) and Hericium erinaceus mycelium (110) revealed anti-aging activity on AD model APP/PS1 mice via elevating protein expressions of Nrf2, SOD-1, HO-1, and GCLC, and reducing Keap1 protein expression in brain tissues or hippocampus. NPs from fungi appeared anti-diabetic function against STZ-induced (111) and db/db mice (101) by promoting mRNA and protein expressions of Nrf2, HO-1 and CAT in liver or kidney tissues. Inonotus obliquus polysaccharide improved reproductive function of Toxoplasma gondii-induced mice through up-regulating protein expressions of HO-1, NQO1 and nuclear Nrf2 in testicular tissues (27). Polysaccharides from Antrodia cinnamomea (202) and Sarcodon imbricatus (203) displayed immunomodulation against CTX-induced mice by increasing protein expressions of Nrf2, HO-1, SOD1, SOD2, CAT, and NQO1, and decreasing Keap1 protein expression in spleen or thymus.
Regulation of NPs from animals and bacteria
Polysaccharides from animals (Ostrea plicatula Gmelin, Holothuria leucospilota, Acaudina leucoprocta, Sepia esculenta ink, and Ostrea rivularis) as well as chitosan could regulate Nrf2 antioxidant pathway for liver protection (114, 204), anti-oxidation (115), improving reproductive function (43, 205, 206), and gastrointestinal protection (207), as summarized in Table 5. Cell experiment indicated that Acaudina leucoprocta polysaccharide exerted anti-oxidation effect on H2O2-induced RAW264.7 cells by increasing mRNA and/or protein expressions of Nrf2, SOD1, and GPX1, and decreasing Keap1 protein expression (115). In animal experiments, polysaccharides from Ostrea plicatula Gmelin (204) and Holothuria leucospilota (114) exhibited liver protection against CTX-induced mice and type 2 diabetic rats respectively, involving with increment of protein and/or mRNA expressions of Nrf2, HO-1, and NQO1 in liver tissues. NPs from animals improved reproductive function against CTX-induced mice (43, 205, 206) through elevating protein and/or mRNA expressions of Nrf2, HO-1, and NQO1, and modulating Keap1 protein expression in ovarian or testis. Chitosan displayed gastrointestinal protection on piglets by adding protein and/or mRNA expressions of GPX1, GPX2, SOD1, SOD2, CAT, Nrf2, NQO1, and HO-1, and declining Keap1 protein expression in ileum (207).
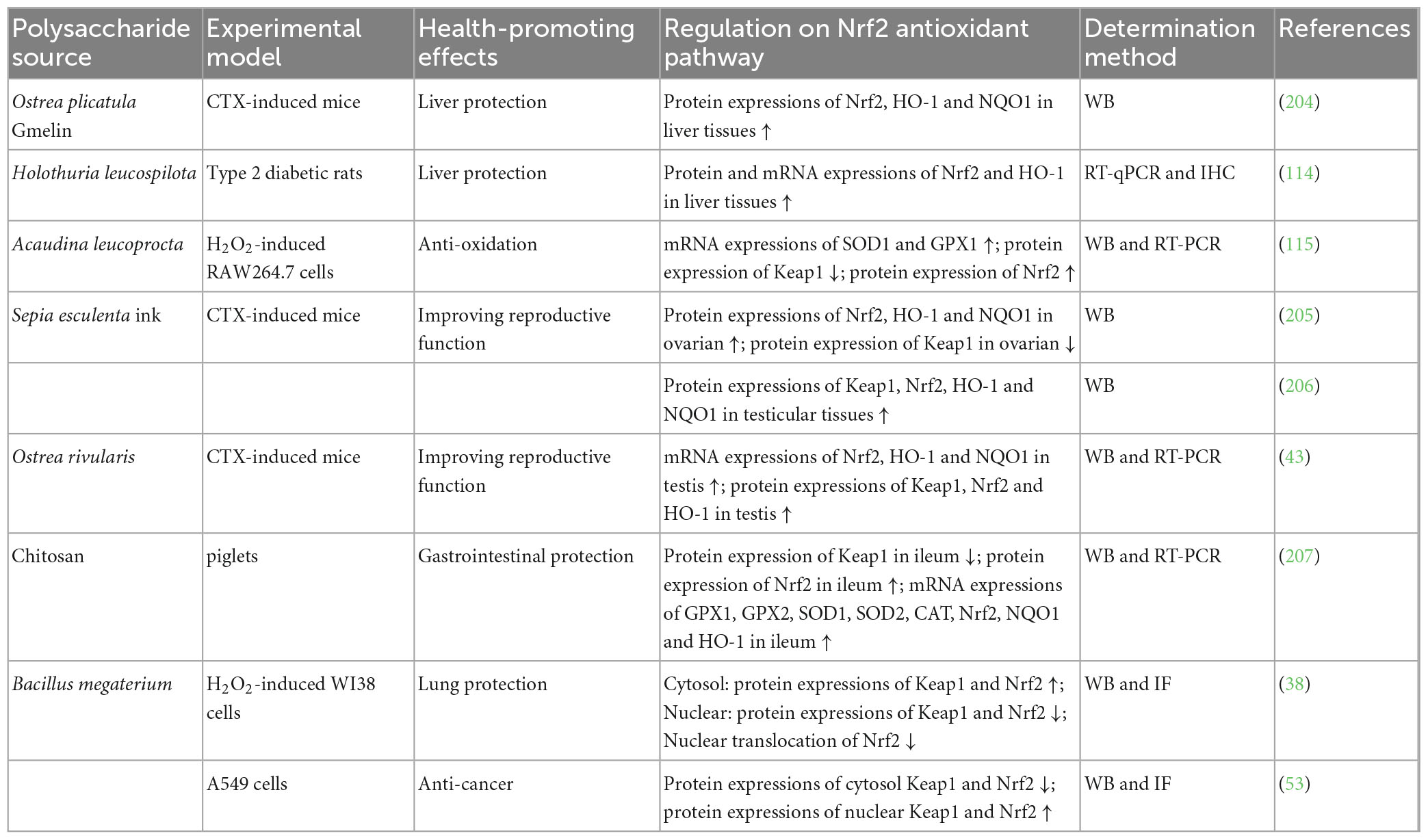
Table 5. Regulation of NPs from animals and bacteria on Nrf2 antioxidant pathway for health-promoting effects.
Polysaccharides from Bacillus megaterium could regulate Nrf2 antioxidant pathway for lung protection (38) and anti-cancer (53), as listed in Table 5. Cell experiments have demonstrated that this polysaccharide exerted lung protection on H2O2-induced WI38 cells by enhancing protein expressions of cytosol Keap1 and cytosol Nrf2, and suppressing protein expressions of nuclear Keap1 and Nrf2 as well as nuclear translocation of Nrf2 (38). Meanwhile, the polysaccharide exhibited anti-cancer effect on A549 cells through increasing protein expressions of cytosol Keap1 and Nrf2, and decreasing protein expressions of nuclear Keap1 and Nrf2 (53).
With above analyses, regulations of NPs on Nrf2 antioxidant pathway in health-promoting effects in vitro and in vivo can be summarized in Figures 2, 3, respectively.
Structure-activity relationship of NPs for health-promoting effects by regulating Nrf2 antioxidant pathway
Structure-activity relationship of NPs for health-promoting effects by regulating Nrf2 antioxidant pathway is unclear. However, the influences of Mw, functional group, monosaccharide composition and side chains on the efficacies of NPs in regulating Nrf2 antioxidant pathway could be preliminarily discussed.
Influence of Mw
There might be two different standpoints concerning the influence of Mw on the regulation of NPs to Nrf2 antioxidant pathway. One standpoint is that polysaccharide with higher Mw generated stronger regulation on Nrf2 antioxidant pathway in vitro and in vivo. Polysaccharide (AZP-1a) with higher Mw (34.1 kDa) from Anoectochilus zhejiangensis exhibited better protection on CCl4-treated HepG2 cells than that (AZP-1d) with lower Mw (4.568 kDa). And, the former enhanced more protein expressions of Nrf2, HO-1 and NQO1 in HepG2 cells (70). Jing et al. (85) have obtained five fractions (PS-1, 14.561 kDa; PS-2, 19.783 kDa; PS-3, 4.973 kDa; PS-4, 15.928 kDa; PS-5, 7.046 kDa) from Athyrium Multidentatum and evaluated theirs cytoprotective activities against H2O2-induced HUVECs. Results indicated that the two higher Mw fractions (PS-2 and PS-4) possessed relatively higher cytoprotections and caused more mRNA expressions of Nrf2 and HO-1 than other three lower Mw fractions. Polysaccharide (PNP40c-1) with higher Mw (206 kDa) from pine nut exerted stronger hepatoprotection against CCl4-induced liver damage in mice and up-regulated more mRNA expressions of Nrf2 and HO-1 in the liver than that (PNP80b-2) with lower Mw (23.0 kDa) (87, 90). Two purified polysaccharides (RGP-1-A and RGP-2-A) were obtained from Rehmannia glutinosa after decolorization using AB-8 macroporous resin and H2O2 respectively, and their Mw values were 18.964 and 3.305 kDa. RGP-1-A showed significantly higher antioxidant capacity on H2O2-induced IPEC-1 cells and caused more up-regulation on mRNA expressions of Nrf2, HO-1 and NQO1 and less Keap1 mRNA expression (208).
Another standpoint is that polysaccharide with lower Mw caused stronger regulation on Nrf2 antioxidant pathway in vitro and in vivo. Polysaccharide (TOP-2) with smaller Mw (<1 kDa) from Taraxacum officinale elevated more protein expressions of Nrf2 and HO-1 than that (TOP-1) with larger Mw (1–9.3 kDa) in LPS-induced RAW264.7 cells, although TOP-2 and TOP-1 had no significance in protecting RAW264.7 cells (132). Polysaccharide (DRP1) with lower Mw (5.695 kDa) from Dandelion root reflected better hepatoprotection on CCl4-induced liver injury in mice than that (DRP2) with higher Mw (8.882 kDa). Meanwhile, DRP1 increased relatively more mRNA expressions of Nrf2 and NQO1 while decreased more mRNA expression of Keap1 in the liver than DRP2 (57). Polysaccharide (FWBP, 21.19 kDa) from fermented wheat bran has been shown to be more effectiveness in positively regulating gut antioxidant-associated gene expression and gut microbiota in zebrafish than that (WBP, 52.03 kDa) from wheat bran. At the same time, FWBP produced more mRNA expressions of CAT, GST, and Nrf2 along with less GPX-3 mRNA expression than than WBP in zebrafish (162). Two different polysaccharides (CPSP-1, 13.1 kDa; CTSP-1, 23.0 kDa) have been obtained from stems of Codonopsis pilosula and Codonopsis tangshen, respectively (66). CPSP-1 showed higher protective effect on H2O2-induced IPEC-J2 cells and had a better promotion on GPXs and SOD1 expressions than CTSP-1. Meanwhile, a polysaccharide (CPP-1) with Mw of 21.0 kDa from Codonopsis pilosula roots showed stronger protection on H2O2-induced IPEC-J2 cells and regulation on Nrf2 antioxidant pathway than that (CTP-1) with Mw of 29.5 kDa from Codonopsis tangshen roots (55).
However, polysaccharide with moderate Mw might be more beneficial to regulate Nrf2 antioxidant pathway. For example, Han et al. (60) have investigated the repair effects of three Astragalus polysaccharides (APS0, APS1, and APS2) with different Mw (11.03, 4.72, and 2.61 KDa) against oxalate-induced HK-2 cells. The findings displayed that APS1 with the moderate Mw provided the strongest repair effect and increased the most protein expressions of Keap1, Nrf2, SOD1, and CAT.
Influence of functional group
Selenization, sulfuration, and acetylation modifications could improve the regulation of NPs on Nrf2 antioxidant pathway, owing to new functional groups have been brought in. Selenizing Codonopsis pilosula polysaccharides (sCPPS5) caused significantly stronger protective effect on H2O2-induced RAW264.7 cells and more increases in protein expressions of Nrf2, HO-1, NQO1, GCLM, and GCLC and declination in Keap1 protein expression than unmodified polysaccharide (CPPS) (131). Selenizing Astragalus polysaccharides (sAPS) exhibited markedly higher protection against CCl4-induced liver injury in rats and up-regulated more mRNA expression levels of GPX1, SOD1 and Nrf2 in the liver than the native one (APS) (150). On the other hand, sulfated Cyclocarya paliurus polysaccharide (S-CPP0.05) showed stronger antioxidant activity to H2O2-induced DCs and generated more increment in Nrf2 protein expression and reduction in Keap1 protein expression in DCs, as compared with the native one (CPP0.05) (96). At the dosages of 100 and 200 mg/kg, sulfated Codonopsis polysaccharide (SCP) produced better hepatoprotective effect on liver in ethanol-induced mice and more decreases in mRNA expressions of Nrf2 and Keap1 than the native one (CP) in the liver (164). Otherwise, acetylated Cyclocarya paliurus polysaccharide (Ac-CPP0.1) generated higher cytoprotection on H2O2-induced DCs and improved more mRNA expressions of SOD1, GPX1, CAT, HO-1, and NQO1 than the native one (CPP0.1) (72). Acetylated Stropharia rugoso-annulata polysaccharides (ASRP) exhibited better action in alleviating non-alcoholic fatty liver in HFD-induced mice and caused more HO-1 protein expression and less Keap1 protein expression in liver tissues (209).
Influence of monosaccharide composition
Natural polysaccharides with higher GalA or GlcA may cause better regulation effect on Nrf2 antioxidant pathway. Two polysaccharides (CPSP-1 and CTSP-1) gained from stems of Codonopsis pilosula and Codonopsis tangshen were determined to contain GalA of 70.1 and 61.3%, respectively. The former was proven to have better protective action on H2O2-induced IPEC-J2 cells and regulation effect on Nrf2 antioxidant pathway (66). Five fractions (PS-1, PS-2, PS-3, PS-4, and PS-4) from Athyrium multidentatum were characterized to contain GlcA content with an order as PS-1 < PS-5 < PS-4 < PS-2 < PS-3 (85). PS-1 showed the lowest cytoprotection on H2O2-induced HUVECs cells and regulation on mRNA expressions of Nrf2 and HO-1. Two purified polysaccharides (RGP-1-A and RGP-2-A) obtained from Rehmannia glutinosa were determined to have GalA contents of 19.02 and 1.1%. RGP-1-A showed significantly better cytoprotection on H2O2-induced IPEC-1 cells and caused observably more increments in mRNA expressions of Nrf2, HO-1 and NQO1 and reduction in Keap1 mRNA expression (208).
On the other hand, higher contents of Ara, Gal, and Rha may have greater regulation effect on Nrf2 antioxidant pathway. The polysaccharides (CPP-1 and CTP-1) from roots of Codonopsis pilosula and Codonopsis tangshen contained Ara+Gal+Rha contents of 41.1 and 39%, respectively. CPP-1 revealed relatively protection on H2O2-induced IPEC-J2 cells and greater regulation on Nrf2 antioxidant pathway (55). Meanwhile, the above-mentioned PS-1 with smallest Ara+Gal+Rha contents showed the lowest cytoprotection on H2O2-induced HUVECs cells and regulation on mRNA expressions of Nrf2 and HO-1, as compared with PS-2, PS-3, PS-4, and PS-5 (85).
Influence of side chains
Shorter AG side chains of NPs can be more effective in promoting Nrf2 antioxidant pathway. A polysaccharide (CPSP-1) with AG-II chains acquired from Codonopsis pilosula stems showed stronger protective effect on H2O2-induced IPEC-J2 cells and promotion on Nrf2 antioxidant pathway than that (CTSP-1) with AG-I and AG-II chains from Codonopsis tangshen stems (66). Moreover, CPP-1 with shorter AG-I chains from Codonopsis pilosula roots revealed better protection on H2O2-induced IPEC-J2 cells and regulation on Nrf2 antioxidant pathway than CTP-1 with longer AG-I chains from Codonopsis tangshen roots (55).
Conclusions and prospects
This review summarizes that NPs from natural sources can regulate Nrf2 antioxidant pathway to exert a wide spectrum of health-promoting effects in vitro and in vivo, such as liver protection, kidney protection, lung protection, neuroprotection, cardioprotection, gastrointestinal protection, anti-oxidation, anti-diabetic, anti-aging, anti-inflammation, anti-radiation, anti-depression, anti-cancer, anti-atherosclerosis, immunomodulation, and improving reproductive function. Moreover, some factors like Keap1, Nrf2, HO-1, NQO1, GCLC, GCLM, γ-GCL, γ-GCS, γ-GCSc, Mn-SOD, SODs, GPXs, CAT, GST, Gstm1, Gstt1, and PGC-1α in Nrf2 antioxidant pathway are modulated in the frequently seen in vitro health-promoting effects (liver protection, kidney protection, lung protection, cardioprotection, gastrointestinal protection, anti-oxidation, anti-diabetic and anti-aging) of NPs (Figure 2). Meanwhile, Keap1, Nrf2, HO-1, NQO1, GCLC, GCLM, γ-GCS, Cu/Zn-SOD, Mn-SOD, SODs, GPXs, GR, CAT, GSTs, NOX2, NOX4, TrxR1, Slc7a11, G6pd2, Prdx1, PGC-1α, MKP1, and p22/47/67phox are regulated in these in vivo health-promoting effects (Figure 3). On the other hand, NPs having regulation on Nrf2 antioxidant pathway can be widely acquired by water extraction and column chromatography methods. Mw of obtained NPs ranges from 1.206 to 3440 kDa, and Fuc, Rha, Ara, Gal, Glc, and/or Man are widely discovered in them. A variety of structures, like pectin, arabinogalactan, 2-O-acetylglucomannan, glucan, and glucogalactan, have been determined in NPs which having regulation on Nrf2 antioxidant pathway. NPs are frequently composed of T-Araf-(1→, →5)-Araf-(1→, →3)-Galp-(1→, →6)-Galp-(1→, →3,4)-Galp-(1→, →3,6)-Galp-(1→, T-Glcp-(1→, →3)-Glcp-(1→, →4)-Glcp-(1→, →6)-Glcp-(1→ and →4)-GalAp-(1→ residues. And →4)-Glcp-(1→, →6)-Glcp-(1→, →3)-Galp-(1→ and →4)-D-Manp-(1→ residues are commonly distributed in their backbones. Noteworthily, structural features of NPs are different owing to different methods and protocols used in extraction and purification processes, thereby structural features included Mw, functional group, monosaccharide composition and side chains have influences on the efficacies of NPs in regulating Nrf2 antioxidant pathway.
Although many studies have disclosed the regulation of NPs on Nrf2 antioxidant pathway, there are still some problems should be explored in future: (i) compared with NPs from herbs and woody plants, less researches have been conducted to the regulative effects of NPs from algae, fungi, animals, and bacteria on Nrf2 antioxidant pathway; (ii) existing evidences are inadequate to establish structure-activity relationship for regulation of NPs on Nrf2 antioxidant pathway in their health-promoting effects; (iii) clinical research on the regulation of NPs on Nrf2 antioxidant pathway is scarce, and regulation of NPs on Nrf2 antioxidant pathway is rarely reported in some health-promoting effects; (iv) Nrf2 antioxidant pathway is activated by NPs in most cases, whilst it is inhibited by NPs in several health-promoting effects like anti-cancer. However, there is few information concerning the classification of NPs as activators and inhibitors; (v) as shown in Tables 1–5, regulation of NPs on Nrf2 antioxidant pathway has been determined by WB, RT-PCR, RT-qPCR, IHC, IF, ChIP, EMSA, and ELISA as well as assay kits. However, Nrf2 antioxidant pathway is a complex network and it has some relations with other pathways. Thus, proteomics, transcriptomics and other methods can be used to explore the regulation of NPs on Nrf2 antioxidant pathway; (vi) there are many genes like PI3K, JNK, ERK, and AKT can regulate Nrf2 antioxidant pathway (10), the effects of NPs on these genes should also be explored; (vii) which procedure is more suitable for preparing NPs with regulation on Nrf2 antioxidant pathway, and which structure has the stronger regulation, cannot be concluded.
Author contributions
J-HL and JL: investigation, writing—original draft, and visualization. Z-CS, X-FL, and Y-FW: investigation. E-SG: writing—review and editing. QZ: project administration and funding acquisition. X-YW: writing—review and editing, supervision, project administration, and funding acquisition. All authors contributed to the article and approved the submitted version.
Funding
This work was financially supported by Jiangxi Provincial Natural Science Foundation (20202BABL216081); the Open Project Program of State Key Laboratory of Food Science and Technology, Nanchang University (SKLF-KF-202212); the Open Project of Key Laboratory of Prevention and treatment of cardiovascular and cerebrovascular diseases, Ministry of Education (XN202001); and University-Level Scientific Research Projects of Gannan Medical University (QD201913, 201107, and QD202128).
Conflict of interest
The authors declare that the research was conducted in the absence of any commercial or financial relationships that could be construed as a potential conflict of interest.
Publisher’s note
All claims expressed in this article are solely those of the authors and do not necessarily represent those of their affiliated organizations, or those of the publisher, the editors and the reviewers. Any product that may be evaluated in this article, or claim that may be made by its manufacturer, is not guaranteed or endorsed by the publisher.
Supplementary material
The Supplementary Material for this article can be found online at: https://www.frontiersin.org/articles/10.3389/fnut.2023.1102146/full#supplementary-material
Abbreviations
p-Nrf2, phosphorylated nuclear factor-erythroid factor 2-related factor 2; Cd, cadmium; Cr, chromium; Pb, plumbum; Mn, manganese; Zn, zinc; Cu, cuprum; HO-1, heme oxygenase-1; NQO1, NAD(P)H quinone dehydrogenase 1; GCLC, glutamate-cysteine ligase catalytic subunit; GCLM, glutamate-cysteine ligase modifier subunit; PGC-1α, peroxisome proliferator-activated receptor-gamma coactivator 1-alpha; SOD, superoxide dismutase; Cu/Zn-SOD, copper, zinc superoxide dismutase; Mn-SOD, manganese superoxide dismutase; CAT, catalase; GST, glutathione S-transferase; GSTP1, glutathione S-transferases P1; GSTT1, glutathione S-transferase theta 1; GSTO1, glutathione S-transferase omega 1; GSTA3, glutathione S-transferase alpha 3; GPX, glutathione peroxidase; ADM, adipogenesis differentiation medium; ODM, osteogenesis differentiation medium; AKR1C2, Aldo-keto reductase family 1 member C2; APOE, Apolipoprotein E; HBEGF, heparin binding EGF like growth factor; DSS, dextran sodium sulfate; H2O2, hydrogen peroxide; CCl4, carbon tetrachloride; PMVECs, pulmonary microvascular endothelial cells; CoCl2, carbonyl chloride; MPTP, 1-methyl-4-phenyl-1,2.3,6-tetrahydropyridine; γ-GCS, γ-glutamylcysteine synthetase; MNNG, N-Methyl-N’-nitro-N-nitrosoguanidine; TrxR1, thioredoxin reductase 1; STZ, streptozotocin; NOX2, nicotinamideadenine-dinucleotide phosphate (NADPH) oxidase 2; NOX4, NADPH oxidase 4; LPS, lipopolysaccharides; CUMS, chronic unpredictable mild stress; CTX, cyclophosphamide; Maf, musculoaponeurotic fibrosarcoma oncogene homolog; γ-GCL, γ-glutamylcysteine ligase; GR, glucocorticoid receptor; MKP1, mitogen-activated protein kinase phosphatase 1; AAPH, 2, 2’-azo-bis-(2-methylpropylimid)-dihydrochloride; TXN, thioredoxin; Slc7a11, solute carrier family 7 member 11; G6pd2, glucose-6-phosphate dehydrogenase 2; Prdx1, peroxiredoxin 1; ox-LDL, oxidized low-density lipoprotein; VSMCs, vascular smooth muscle cells; DCs, dendritic cells.
References
1. Forman HJ, Zhang HQ. Targeting oxidative stress in disease: promise and limitations of antioxidant therapy. Nat Rev Drug Discov. (2021) 20:689–709. doi: 10.1038/s41573-021-00233-1
2. Zeng XL, Yu JJ, Zeng TH, Liu Y, Li B. 3’-daidzein sulfonate protects myocardial cells from hypoxic-ischemic injury via the NRF2/HO-1 signaling pathway. J Thorac Dis. (2021) 13:6897. doi: 10.21037/jtd-21-1909
3. Hannan MA, Dash R, Sohag AA, Haque MN, Moon IS. Neuroprotection against oxidative stress: phytochemicals targeting TrkB signaling and the Nrf2-ARE antioxidant system. Front Mol Neurosci. (2020) 13:116. doi: 10.3389/fnmol.2020.00116
4. Bellezza I. Oxidative stress in age-related macular degeneration: Nrf2 as therapeutic target. Front Pharmacol. (2018) 9:1280. doi: 10.3389/fphar.2018.01280
5. Yanaka A. Role of NRF2 in protection of the gastrointestinal tract against oxidative stress. J. Clin. Biochem Nutr. (2018) 63:18–25. doi: 10.3164/jcbn.17-139
6. Nezu M, Suzuki N. Roles of Nrf2 in protecting the kidney from oxidative damage. Int J Mol Sci. (2020) 21:2951. doi: 10.3390/ijms21082951
7. Tonelli C, Chio II, Tuveson DA. Transcriptional regulation by Nrf2. Antioxid Redox Sign. (2018) 29:1727–45. doi: 10.1089/ars.2017.7342
8. He F, Ru XL, Wen T. NRF2, a transcription factor for stress response and beyond. Int J Mol Sci. (2020) 21:4777. doi: 10.3390/ijms21134777
9. Gugliandolo A, Bramanti P, Mazzon E. Activation of Nrf2 by natural bioactive compounds: a promising approach for stroke? Int J Mol Sci. (2020) 21:4875. doi: 10.3390/ijms21144875
10. Zhao FJ, Ci XX, Man XX, Li JJ, Wei ZT, Zhang SL. Food-derived pharmacological modulators of the Nrf2/ARE pathway: their role in the treatment of diseases. Molecules. (2021) 26:1016. doi: 10.3390/molecules26041016
11. Lu X, Chen JH, Guo ZB, Zheng YF, Rea MC, Su H, et al. Using polysaccharides for the enhancement of functionality of foods: a review. Trends Food Sci Technol. (2019) 86:311–27.
12. Yang X, Li AQ, Li XX, Sun LJ, Guo YR. An overview of classifications, properties of food polysaccharides and their links to applications in improving food textures. Trends Food Sci Technol. (2020) 102:1–15.
13. Chen XX, Wang YK, Shen MY, Yu Q, Chen Y, Huang LX, et al. The water-soluble non-starch polysaccharides from natural resources against excessive oxidative stress: a potential health-promoting effect and its mechanisms. Int J Biol Macromol. (2021) 171:320–30. doi: 10.1016/j.ijbiomac.2021.01.022
14. Liu HS, Zhou MY, Zhang X, Li YL, Kong JW, Gao X, et al. Sagittaria sagittifolia polysaccharide protects against six-heavy-metal-induced hepatic injury associated with the activation of Nrf2 pathway to regulate oxidative stress and apoptosis. J Inorg Biochem. (2022) 232:111810. doi: 10.1016/j.jinorgbio.2022.111810
15. Yu Z, Zhao L, Zhao JL, Xu WX, Guo ZH, Zhang AZ, et al. Dietary Taraxacum mongolicum polysaccharide ameliorates the growth, immune response, and antioxidant status in association with NF-κB, Nrf2 and TOR in Jian carp (Cyprinus carpio var. Jian). Aquaculture. (2022) 547:737522.
16. Wang YJ, Li QM, Zha XQ, Luo JP. Dendrobium fimbriatum Hook polysaccharide ameliorates dextran-sodium-sulfate-induced colitis in mice via improving intestinal barrier function, modulating intestinal microbiota, and reducing oxidative stress and inflammatory responses. Food Funct. (2022) 13:143–60. doi: 10.1039/d1fo03003e
17. Lu XX, Qin L, Guo M, Geng JJ, Dong ST, Wang K, et al. A novel alginate from Sargassum seaweed promotes diabetic wound healing by regulating oxidative stress and angiogenesis. Carbohydr Polym. (2022) 289:119437. doi: 10.1016/j.carbpol.2022.119437
18. Chu WH, Wang P, Ma Z, Peng L, Wang ZM, Chen ZL. Ultrasonic treatment of Dendrobium officinale polysaccharide enhances antioxidant and anti-inflammatory activity in a mouse D-galactose-induced aging model. Food Sci Nutr. (2022) 10:2620–30. doi: 10.1002/fsn3.2867
19. Pan H, Niu L, Wu YH, Chen LY, Zhou XW, Zhao Y. Lycium barbarum polysaccharide protects rats and cardiomyocytes against ischemia/reperfusion injury via Nrf2 activation through autophagy inhibition. Mol Med Report. (2021) 24:1–14. doi: 10.3892/mmr.2021.12418
20. Wen YF, Xiao HY, Liu Y, Yang YQ, Wang YM, Xu SJ, et al. Polysaccharides from Dendrobium officinale ameliorate colitis-induced lung injury via inhibiting inflammation and oxidative stress. Chem Biol Interact. (2021) 347:109615. doi: 10.1016/j.cbi.2021.109615
21. Wang X, Liu W, Jin GZ, Wu ZJ, Zhang D, Bao YZ, et al. Salvia miltiorrhiza polysaccharides alleviates florfenicol induced kidney injury in chicks via inhibiting oxidative stress and apoptosis. Ecotoxicol Environ Saf. (2022) 233:113339. doi: 10.1016/j.ecoenv.2022.113339
22. Huang S, Yuan HY, Li WQ, Liu XY, Zhang XJ, Xiang DX, et al. Polygonatum sibiricum polysaccharides protect against MPP-induced neurotoxicity via the Akt/mTOR and Nrf2 pathways. Oxid Med Cell Longev. (2021) 2021:8843899. doi: 10.1155/2021/8843899
23. Xiong GF, Li DW, Zheng MB, Liu SC. The effects of Lycium barbarum polysaccharide (LBP) in a mouse model of cerulein-induced acute pancreatitis. Med Sci Monit. (2019) 25:3880. doi: 10.12659/MSM.913820
24. Guo Y, Balasubramanian B, Zhao ZH, Liu WC. Marine algal polysaccharides alleviate aflatoxin B1-induced bursa of Fabricius injury by regulating redox and apoptotic signaling pathway in broilers. Poult Sci. (2021) 100:844–57. doi: 10.1016/j.psj.2020.10.050
25. Shen FM, Xie P, Li CT, Bian ZJ, Wang XC, Peng DY, et al. Polysaccharides from Polygonatum cyrtonema hua reduce depression-like behavior in mice by inhibiting oxidative stress-calpain-1-NLRP3 signaling axis. Oxid Med Cell Longev. (2022) 2022:2566917. doi: 10.1155/2022/2566917
26. Shiau JP, Chuang YT, Yang KH, Chang FR, Sheu JH, Hou MF, et al. Brown algae-derived fucoidan exerts oxidative stress-dependent antiproliferation on oral cancer cells. Antioxidants. (2022) 11:841. doi: 10.3390/antiox11050841
27. Ding X, Ge BJ, Wang M, Zhou HY, Sang R, Yu YF, et al. Inonotus obliquus polysaccharide ameliorates impaired reproductive function caused by Toxoplasma gondii infection in male mice via regulating Nrf2-PI3K/AKT pathway. Int J Biol Macromol. (2020) 151:449–58. doi: 10.1016/j.ijbiomac.2020.02.178
28. Liang BH, Peng LQ, Li RX, Li HP, Mo ZY, Dai XY, et al. Lycium barbarum polysaccharide protects HSF cells against ultraviolet-induced damage through the activation of Nrf2. Cell Mol Biol Lett. (2018) 23:1–13. doi: 10.1186/s11658-018-0084-2
29. Zhao JM, Niu XY, Yu JJ, Xiao X, Li WQ, Zang LL, et al. Poria cocos polysaccharides attenuated ox-LDL-induced inflammation and oxidative stress via ERK activated Nrf2/HO-1 signaling pathway and inhibited foam cell formation in VSMCs. Int Immunopharmacol. (2020) 80:106173. doi: 10.1016/j.intimp.2019.106173
30. Liu HF, Fang YK, Li YF, Ma LK, Wang Q, Xiao GS, et al. Characterization of PCS-2A, a polysaccharide derived from chestnut shell, and its protective effects against H2O2-induced liver injury in hybrid grouper. Int J Biol Macromol. (2021) 193:814–22. doi: 10.1016/j.ijbiomac.2021.10.185
31. Guo FC, Zhuang XY, Han MY, Lin WT. Polysaccharides from Enteromorpha prolifera protect against carbon tetrachloride-induced acute liver injury in mice via activation of Nrf2/HO-1 signaling, and suppression of oxidative stress, inflammation and apoptosis. Food Funct. (2020) 11:4485–98. doi: 10.1039/d0fo00575d
32. Cai ZN, Li W, Mehmood S, Pan WJ, Wang Y, Meng FJ, et al. Structural characterization, in vitro and in vivo antioxidant activities of a heteropolysaccharide from the fruiting bodies of Morchella esculenta. Carbohydr Polym. (2018) 195:29–38. doi: 10.1016/j.carbpol.2018.04.069
33. Li W, Cai ZN, Mehmood S, Wang Y, Pan WJ, Zhang WN, et al. Polysaccharide FMP-1 from Morchella esculenta attenuates cellular oxidative damage in human alveolar epithelial A549 cells through PI3K/AKT/Nrf2/HO-1 pathway. Int J Biol Macromol. (2018) 120:865–75. doi: 10.1016/j.ijbiomac.2018.08.148
34. Zheng Y, Wang WD, Li Y. Antitumor and immunomodulatory activity of polysaccharide isolated from Trametes orientalis. Carbohydr Polym. (2015) 131:248–54. doi: 10.1016/j.carbpol.2015.05.074
35. Zheng Y, Fan J, Chen HW, Liu EQ. Trametes orientalis polysaccharide alleviates PM 2.5-induced lung injury in mice through its antioxidant and anti-inflammatory activities. Food Funct. (2019) 10:8005–15. doi: 10.1039/c9fo01777a
36. Jiang WH, Zhu HK, Xu WQ, Liu C, Hu B, Guo YH, et al. Echinacea purpurea polysaccharide prepared by fractional precipitation prevents alcoholic liver injury in mice by protecting the intestinal barrier and regulating liver-related pathways. Int J Biol Macromol. (2021) 187:143–56. doi: 10.1016/j.ijbiomac.2021.07.095
37. Chowdhury SR, Basak RK, Sen R, Adhikari B. Production of extracellular polysaccharide by Bacillus megaterium RB-05 using jute as substrate. Bioresour Technol. (2011) 102:6629–32. doi: 10.1016/j.biortech.2011.03.099
38. Roy Chowdhury S, Sengupta S, Biswas S, Sinha TK, Sen R, Basak RK, et al. Bacterial fucose-rich polysaccharide stabilizes MAPK-mediated Nrf2/Keap1 signaling by directly scavenging reactive oxygen species during hydrogen peroxide-induced apoptosis of human lung fibroblast cells. PLoS One. (2014) 9:e113663. doi: 10.1371/journal.pone.0113663
39. Song QY, Zhu ZY. Using Cordyceps militaris extracellular polysaccharides to prevent Pb 2+-induced liver and kidney toxicity by activating Nrf2 signals and modulating gut microbiota. Food Funct. (2020) 11:9226–39. doi: 10.1039/d0fo01608j
40. Liu C, Hua HY, Zhu HK, Cheng YL, Guo YH, Yao WR, et al. Aloe polysaccharides ameliorate acute colitis in mice via Nrf2/HO-1 signaling pathway and short-chain fatty acids metabolism. Int J Biol Macromol. (2021) 185:804–12. doi: 10.1016/j.ijbiomac.2021.07.007
41. Zhang KF, Xu QM, Gao Y, Cao HK, Lian YY, Li ZM, et al. Polysaccharides from Dicliptera chinensis ameliorate liver disturbance by regulating TLR-4/NF−κB and AMPK/Nrf2 signalling pathways. J Cell Mol Med. (2020) 24:6397–409. doi: 10.1111/jcmm.15286
42. Yang JF, Zhu BW, Zheng J, Sun LM, Zhou DY, Dong XP, et al. Stimulation of lymphocyte proliferation by oyster glycogen sulfated at C-6 position. Carbohydr Polym. (2013) 94:301–8. doi: 10.1016/j.carbpol.2013.01.057
43. Li SJ, Song ZY, Liu TT, Liang J, Yuan J, Xu ZC, et al. Polysaccharide from Ostrea rivularis attenuates reproductive oxidative stress damage via activating Keap1-Nrf2/ARE pathway. Carbohydr Polym. (2018) 186:321–31. doi: 10.1016/j.carbpol.2018.01.075
44. Wang L, Jayawardena TU, Yang HW, Lee HG, Kang MC, Sanjeewa KA, et al. Isolation, characterization, and antioxidant activity evaluation of a fucoidan from an enzymatic digest of the edible seaweed, Hizikia fusiforme. Antioxidants. (2020) 9:363. doi: 10.3390/antiox9050363
45. Hu DW, Bao T, Lu Y, Su HM, Ke HH, Chen W. Polysaccharide from mulberry fruit (Morus alba L.) protects against palmitic-acid-induced hepatocyte lipotoxicity by activating the Nrf2/ARE signaling pathway. J Agric Food Chem. (2019) 68:13016–24. doi: 10.1021/acs.jafc.9b03335
46. Chen W, Lu Y, Hu DW, Mo JL, Ni JD. Black mulberry (Morus nigra L.) polysaccharide ameliorates palmitate-induced lipotoxicity in hepatocytes by activating Nrf2 signaling pathway. Int J Biol Macromol. (2021) 172:394–407. doi: 10.1016/j.ijbiomac.2021.01.059
47. Li X, Wang XH, Dong Y, Song RL, Wei J, Yu AX, et al. Preparation, structural analysis, antioxidant and digestive enzymes inhibitory activities of polysaccharides from Thymus quinquecostatus Celak leaves. Ind Crop Prod. (2022) 175:114288.
48. Huang C, Peng X, Pang DJ, Li J, Paulsen BS, Rise F, et al. Pectic polysaccharide from Nelumbo nucifera leaves promotes intestinal antioxidant defense in vitro and in vivo. Food Funct. (2021) 12:10828–41. doi: 10.1039/d1fo02354c
49. Zhang Y, Pan XL, Ran SQ, Wang KP. Purification, structural elucidation and anti-inflammatory activity in vitro of polysaccharides from Smilax china L. Int J Biol Macromol. (2019) 139:233–43. doi: 10.1016/j.ijbiomac.2019.07.209
50. Wang KP, Yang LL, Zhou J, Pan XL, He ZH, Liu JX, et al. Smilax china L. polysaccharide alleviates oxidative stress and protects from acetaminophen-induced hepatotoxicity via activating the Nrf2-ARE pathway. Front Pharmacol. (2022) 13:888560. doi: 10.3389/fphar.2022.888560
51. Meng M, Cheng D, Han LR, Chen YY, Wang CL. Isolation, purification, structural analysis and immunostimulatory activity of water-soluble polysaccharides from Grifola frondosa fruiting body. Carbohydr Polym. (2017) 157:1134–43. doi: 10.1016/j.carbpol.2016.10.082
52. Meng M, Zhang R, Han R, Kong Y, Wang RH, Hou LH. The polysaccharides from the Grifola frondosa fruiting body prevent lipopolysaccharide/D-galactosamine-induced acute liver injury via the miR-122-Nrf2/ARE pathways. Food Funct. (2021) 12:1973–82. doi: 10.1039/d0fo03327h
53. Chowdhury SR, Sengupta S, Biswas S, Sen R, Sinha TK, Basak RK, et al. Low fucose containing bacterial polysaccharide facilitate mitochondria-dependent ROS-induced apoptosis of human lung epithelial carcinoma via controlled regulation of MAPKs-mediated Nrf2/Keap1 homeostasis signaling. Mol Carcinog. (2015) 54:1636–55. doi: 10.1002/mc.22236
54. Wang CX, Zheng LY, Liu SN, Guo XX, Qu Y, Gao MJ, et al. A novel acidic polysaccharide from the residue of Panax notoginseng and its hepatoprotective effect on alcoholic liver damage in mice. Int J Biol Macromol. (2020) 149:1084–97. doi: 10.1016/j.ijbiomac.2020.02.034
55. Zou YF, Zhang YY, Paulsen BS, Rise F, Chen ZL, Jia RY, et al. New pectic polysaccharides from Codonopsis pilosula and Codonopsis tangshen: structural characterization and cellular antioxidant activities. J Sci Food Agric. (2021) 101:6043–52. doi: 10.1002/jsfa.11261
56. Chen X, Qian L, Wang BJ, Zhang ZJ, Liu H, Zhang YN, et al. Synergistic hypoglycemic effects of pumpkin polysaccharides and puerarin on type II diabetes mellitus mice. Molecules. (2019) 24:955. doi: 10.3390/molecules24050955
57. Cai LL, Wan DW, Yi FL, Luan LB. Purification, preliminary characterization and hepatoprotective effects of polysaccharides from dandelion root. Molecules. (2017) 22:1409. doi: 10.3390/molecules22091409
58. Zhang YD, Wang HL, Zhang L, Yuan Y, Yu DH. Codonopsis lanceolata polysaccharide CLPS alleviates high fat/high sucrose diet-induced insulin resistance via anti-oxidative stress. Int J Biol Macromol. (2020) 145:944–9. doi: 10.1016/j.ijbiomac.2019.09.185
59. Shen R, Liu DS, Hou CC, Liu D, Zhao LX, Cheng J, et al. Protective effect of Potentilla anserina polysaccharide on cadmium-induced nephrotoxicity in vitro and in vivo. Food Funct. (2017) 8:3636–46. doi: 10.1039/c7fo00495h
60. Han J, Guo D, Sun XY, Wang JM, Ouyang JM, Gui BS. Repair effects of astragalus polysaccharides with different molecular weights on oxidatively damaged HK-2 cells. Sci Rep. (2019) 9:1–15. doi: 10.1038/s41598-019-46264-y
61. Wen ZS, Xue R, Du M, Tang Z, Xiang XW, Zheng B, et al. Hemp seed polysaccharides protect intestinal epithelial cells from hydrogen peroxide-induced oxidative stress. Int J Biol Macromol. (2019) 135:203–11. doi: 10.1016/j.ijbiomac.2019.05.082
62. Zou YF, Chen MS, Fu YP, Zhu ZK, Zhang YY, Paulsen BS, et al. Characterization of an antioxidant pectic polysaccharide from Platycodon grandiflorus. Int J Biol Macromol. (2021) 175:473–80. doi: 10.1016/j.ijbiomac.2021.02.041
63. Hua YF, Zhang M, Fu CX, Chen ZH, Chan GY. Structural characterization of a 2-O-acetylglucomannan from Dendrobium officinale stem. Carbohydr Res. (2004) 339:2219–24. doi: 10.1016/j.carres.2004.05.034
64. Peng Q, Song JJ, Lv XP, Wang ZF, Huang LJ, Du YG. Structural characterization of an arabinogalactan-protein from the fruits of Lycium ruthenicum. J Agric Food Chem. (2012) 60:9424–9. doi: 10.1021/jf302619c
65. Deng KW, Li YL, Xiao M, Wang FH, Zhou P, Zhang W, et al. Lycium ruthenicum Murr polysaccharide protects cortical neurons against oxygen-glucose deprivation/reperfusion in neonatal hypoxic-ischemic encephalopathy. Int J Biol Macromol. (2020) 158:562–8. doi: 10.1016/j.ijbiomac.2020.04.122
66. Zou YF, Zhang YY, Paulsen BS, Rise F, Chen ZL, Jia RY, et al. Structural features of pectic polysaccharides from stems of two species of radix codonopsis and their antioxidant activities. Int J Biol Macromol. (2020) 159:704–13. doi: 10.1016/j.ijbiomac.2020.05.083
67. Liang J, Chen SX, Hu YD, Yang YQ, Yuan J, Wu YF, et al. Protective roles and mechanisms of Dendrobium officinal polysaccharides on secondary liver injury in acute colitis. Int J Biol Macromol. (2018) 107:2201–10. doi: 10.1016/j.ijbiomac.2017.10.085
68. Liang J, Wu YF, Yuan H, Yang YQ, Xiong QP, Liang CY, et al. Dendrobium officinale polysaccharides attenuate learning and memory disabilities via anti-oxidant and anti-inflammatory actions. Int J Biol Macromol. (2019) 126:414–26. doi: 10.1016/j.ijbiomac.2018.12.230
69. Chu Q, Zhang S, Yu LS, Li YL, Liu YY, Ye X, et al. Apios americana Medikus tuber polysaccharide exerts anti-inflammatory effects by activating autophagy. Int J Biol Macromol. (2019) 130:892–902. doi: 10.1016/j.ijbiomac.2019.03.012
70. Wu YB, Liu C, Jiang YQ, Bai BK, He XH, Wang HR, et al. Structural characterization and hepatoprotective effects of polysaccharides from Anoectochilus zhejiangensis. Int J Biol Macromol. (2022) 198:111–8. doi: 10.1016/j.ijbiomac.2021.12.128
71. Chu Q, Jia RY, Chen M, Li YL, Yu X, Wang YX, et al. Tetrastigma hemsleyanum tubers polysaccharide ameliorates LPS-induced inflammation in macrophages and Caenorhabditis elegans. Int J Biol Macromol. (2019) 141:611–21. doi: 10.1016/j.ijbiomac.2019.09.039
72. Meng Z, Yi H, En LJ, Qi A, Ye XM, Xiang L, et al. Structural characterization and antioxidant activity of an acetylated Cyclocarya paliurus polysaccharide (Ac-CPP0. 1). Int J Biol Macromol. (2021) 171:112–22. doi: 10.1016/j.ijbiomac.2020.12.201
73. Wu MB, Wu YL, Zhou J, Pan YJ. Structural characterisation of a water-soluble polysaccharide with high branches from the leaves of Taxus chinensis var. mairei. Food Chem. (2009) 113:1020–4.
74. Zhang SW, Li LL, Hu JT, Ma P, Zhu HM. Polysaccharide of Taxus chinensis var. mairei Cheng et LK Fu attenuates neurotoxicity and cognitive dysfunction in mice with Alzheimer’s disease. Pharm Biol. (2020) 58:959–68. doi: 10.1080/13880209.2020.1817102
75. Kim WS, Kim YE, Cho EJ, Byun EB, Park WY, Song HY, et al. Neuroprotective effect of Annona muricata-derived polysaccharides in neuronal HT22 cell damage induced by hydrogen peroxide. Biosci Biotechnol Biochem. (2020) 84:1001–12. doi: 10.1080/09168451.2020.1715201
76. Zhao YC, Liu XL, Zheng YN, Liu WC, Ding CB. Aronia melanocarpa polysaccharide ameliorates inflammation and aging in mice by modulating the AMPK/SIRT1/NF-κB signaling pathway and gut microbiota. Sci Rep. (2021) 11:1–15. doi: 10.1038/s41598-021-00071-6
77. Yang YN, Ding ZH, Zhong RX, Xia TY, Wang WJ, Zhao H, et al. Cardioprotective effects of a Fructus aurantii polysaccharide in isoproterenol-induced myocardial ischemic rats. Int J Biol Macromol. (2020) 155:995–1002. doi: 10.1016/j.ijbiomac.2019.11.063
78. Shu ZP, Yang YN, Ding ZH, Wang WJ, Zhong RX, Xia TY, et al. Structural characterization and cardioprotective activity of a novel polysaccharide from Fructus aurantii. Int J Biol Macromol. (2020) 144:847–56. doi: 10.1016/j.ijbiomac.2019.09.162
79. Hu YY, Yin FW, Liu ZY, Xie HK, Xu YS, Zhou DY, et al. Acerola polysaccharides ameliorate high-fat diet-induced non-alcoholic fatty liver disease through reduction of lipogenesis and improvement of mitochondrial functions in mice. Food Funct. (2020) 11:1037–48. doi: 10.1039/c9fo01611b
80. Yue Y, Wu SC, Zhang HF, Zhang XY, Niu YH, Cao XQ, et al. Characterization and hepatoprotective effect of polysaccharides from Ziziphus jujuba Mill. var. spinosa (Bunge) Hu ex HF Chou sarcocarp. Food Chem Toxicol. (2014) 74:76–84. doi: 10.1016/j.fct.2014.09.006
81. Yuan RS, Tao X, Liang S, Pan Y, He L, Sun JH, et al. Protective effect of acidic polysaccharide from Schisandra chinensis on acute ethanol-induced liver injury through reducing CYP2E1-dependent oxidative stress. Biomed Pharmacother. (2018) 99:537–42. doi: 10.1016/j.biopha.2018.01.079
82. Che JY, Yang S, Qiao ZJ, Li H, Sun JH, Zhuang WY, et al. Schisandra chinensis acidic polysaccharide partialy reverses acetaminophen-induced liver injury in mice. J Pharmacol Sci. (2019) 140:248–54. doi: 10.1016/j.jphs.2019.07.008
83. Shan YY, Jiang B, Yu JH, Wang JY, Wang XL, Li H, et al. Protective effect of Schisandra chinensis polysaccharides against the immunological liver injury in mice based on Nrf2/ARE and TLR4/NF-κ B signaling pathway. J Med Food. (2019) 22:885–95. doi: 10.1089/jmf.2018.4377
84. Jing L, Jiang JR, Liu DM, Sheng JW, Zhang WF, Li ZJ, et al. Structural characterization and antioxidant activity of polysaccharides from Athyrium multidentatum (Doll.) Ching in d-galactose-induced aging mice via PI3K/AKT pathway. Molecules. (2019) 24:3364. doi: 10.3390/molecules24183364
85. Jing L, Sheng JW, Jiang JR, Wang Y, Shen XY, Liu DM, et al. Chemical characteristics and cytoprotective activities of polysaccharide fractions from Athyrium multidentatum (Doll.) Ching. Int J Biol Macromol. (2020) 158:1227–38. doi: 10.1016/j.ijbiomac.2020.05.053
86. Chu Q, Jia RY, Chen W, Liu YY, Li YL, Ye X, et al. Purified Tetrastigma hemsleyanum vines polysaccharide attenuates EC-induced toxicity in Caco-2 cells and Caenorhabditis elegans via DAF-16/FOXO pathway. Int J Biol Macromol. (2020) 150:1192–202. doi: 10.1016/j.ijbiomac.2019.10.128
87. Qu H, Gao X, Wang ZY, Yi JJ. Comparative study on hepatoprotection of pine nut (Pinus koraiensis Sieb. et Zucc.) polysaccharide against different types of chemical-induced liver injury models in vivo. Int J Biol Macromol. (2020) 155:1050–9. doi: 10.1016/j.ijbiomac.2019.11.069
88. Qu H, Gao X, Cheng CL, Zhao HT, Wang ZY, Yi JJ. Hepatoprotection mechanism against alcohol-induced liver injury in vivo and structural characterization of Pinus koraiensis pine nut polysaccharide. Int J Biol Macromol. (2020) 154:1007–21. doi: 10.1016/j.ijbiomac.2020.03.168
89. Qu H, Gao X, Zhao HT, Wang ZY, Yi JJ. Structural characterization and in vitro hepatoprotective activity of polysaccharide from pine nut (Pinus koraiensis Sieb. et Zucc.). Carbohydr Polym. (2019) 223:115056. doi: 10.1016/j.carbpol.2019.115056
90. Qu H, Liu S, Cheng CL, Zhao HT, Gao X, Wang ZY, et al. Hepatoprotection of pine nut polysaccharide via NRF2/ARE/MKP1/JNK signaling pathways against carbon tetrachloride-induced liver injury in mice. Food Chem Toxicol. (2020) 142:111490. doi: 10.1016/j.fct.2020.111490
91. Liu JJ, Wu YL, Wang YF, Wu XL, Li YC, Gao CJ, et al. Hepatoprotective effect of polysaccharide isolated from Sonneratia apetala fruits on acetaminophen-induced liver injury mice. J Funct Foods. (2021) 86:104685.
92. Wu QW, Liu C, Zhang JN, Xiao W, Yang F, Yu Y, et al. Schisandra chinensis polysaccharide protects against cyclosporin A-induced liver injury by promoting hepatocyte proliferation. J Funct Foods. (2021) 87:104799.
93. Jin MY, Wang YX, Yang XB, Yin H, Nie SP, Wu XY. Structure characterization of a polysaccharide extracted from noni (Morinda citrifolia L.) and its protective effect against DSS-induced bowel disease in mice. Food Hydrocoll. (2019) 90:189–97.
94. Yang XB, Lin CR, Cai S, Li WZ, Tang J, Wu XY. Therapeutic effects of noni fruit water extract and polysaccharide on oxidative stress and inflammation in mice under high-fat diet. Food Funct. (2020) 11:1133–45. doi: 10.1039/c9fo01859j
95. Han Y, Ouyang KH, Li JE, Liu X, An Q, Zhao M, et al. Sulfated modification, characterization, immunomodulatory activities and mechanism of the polysaccharides from Cyclocarya paliurus on dendritic cells. Int J Biol Macromol. (2020) 159:108–16. doi: 10.1016/j.ijbiomac.2020.04.265
96. Han Y, Zhao M, Ouyang KH, Chen S, Zhang Y, Liu X, et al. Sulfated modification, structures, antioxidant activities and mechanism of Cyclocarya paliurus polysaccharides protecting dendritic cells against oxidant stress. Ind Crop Prod. (2021) 164:113353.
97. Tian SS, Jiang XX, Tang YP, Han T. Laminaria japonica fucoidan ameliorates cyclophosphamide-induced liver and kidney injury possibly by regulating Nrf2/HO-1 and TLR4/NF−κB signaling pathways. J Sci Food Agric. (2022) 102:2604–12. doi: 10.1002/jsfa.11602
98. Zhang L, Hao JW, Zheng Y, Su RJ, Liao YJ, Gong XL, et al. Fucoidan protects dopaminergic neurons by enhancing the mitochondrial function in a rotenone-induced rat model of Parkinson’s disease. Aging Dis. (2018) 9:590. doi: 10.14336/AD.2017.0831
99. Zhang Y, Xu M, Hu CX, Liu AM, Chen JJ, Gu CF, et al. Sargassum fusiforme fucoidan SP2 extends the lifespan of Drosophila melanogaster by upregulating the Nrf2-mediated antioxidant signaling pathway. Oxid Med Cell Longev. (2019) 2019:8918914. doi: 10.1155/2019/8918914
100. Yu Y, Li YP, Du CY, Mou HJ, Wang P. Compositional and structural characteristics of sulfated polysaccharide from Enteromorpha prolifera. Carbohydr Polym. (2017) 165:221–8. doi: 10.1016/j.carbpol.2017.02.011
101. Hu WJ, Wang J, Guo WY, Liu YG, Guo ZA, Miao YG, et al. Studies on characteristics and anti-diabetic and-nephritic effects of polysaccharides isolated from Paecilomyces hepiali fermentation mycelium in db/db mice. Carbohydr Polym. (2020) 232:115766. doi: 10.1016/j.carbpol.2019.115766
102. Cao XY, Liu D, Bi RC, He YL, He Y, Liu JL. The protective effects of a novel polysaccharide from Lentinus edodes mycelia on islet β (INS-1) cells damaged by glucose and its transportation mechanism with human serum albumin. Int J Biol Macromol. (2019) 134:344–53. doi: 10.1016/j.ijbiomac.2019.05.033
103. Song XL, Sun WX, Cui WJ, Jia L, Zhang JJ. A polysaccharide of PFP-1 from Pleurotus geesteranus attenuates alcoholic liver diseases via Nrf2 and NF-κB signaling pathways. Food Funct. (2021) 12:4591–605. doi: 10.1039/d1fo00310k
104. Xu L, Sang R, Yu YF, Li JX, Ge BJ, Zhang XM. The polysaccharide from Inonotus obliquus protects mice from Toxoplasma gondii-induced liver injury. Int J Biol Macromol. (2019) 125:1–8. doi: 10.1016/j.ijbiomac.2018.11.114
105. Dong HB, Yang JL, Wang Y, Jiang Y, Chen J, Zhang WN, et al. Polysaccharide SAFP from Sarcodon aspratus attenuates oxidative stress-induced cell damage and bleomycin-induced pulmonary fibrosis. Int J Biol Macromol. (2020) 164:1215–36. doi: 10.1016/j.ijbiomac.2020.07.120
106. Zhang DJ, Xiang M, Jiang Y, Wu F, Chen HQ, Sun M, et al. The protective effect of polysaccharide SAFP from Sarcodon aspratus on water immersion and restraint stress-induced gastric ulcer and modulatory effects on gut microbiota dysbiosis. Foods. (2022) 11:1567. doi: 10.3390/foods11111567
107. Zhao HJ, Li HP, Feng YB, Zhang YW, Yuan FF, Zhang JJ, et al. Mycelium polysaccharides from Termitomyces albuminosus attenuate CCl4-induced chronic liver injury via inhibiting TGFβ1/Smad3 and NF-κB signal pathways. Int J Mol Sci. (2019) 20:4872. doi: 10.3390/ijms20194872
108. Zeng P, Li J, Chen Y, Zhang L. The structures and biological functions of polysaccharides from traditional Chinese herbs. Prog Mol Biol Transl Sci. (2019) 163:423–44. doi: 10.1016/bs.pmbts.2019.03.003
109. Hu WJ, Li ZP, Wang WQ, Song MK, Dong RT, Zhou YL, et al. Structural characterization of polysaccharide purified from Amanita caesarea and its pharmacological basis for application in Alzheimer’s disease: endoplasmic reticulum stress. Food Funct. (2021) 12:11009–23. doi: 10.1039/d1fo01963e
110. Hu WJ, Song MK, Wang CY, Guo ZA, Li Y, Wang D. Structural characterization of polysaccharide purified from Hericium erinaceus fermented mycelium and its pharmacological basis for application in Alzheimer’s disease: oxidative stress related calcium homeostasis. Int J Biol Macromol. (2021) 193:358–69. doi: 10.1016/j.ijbiomac.2021.10.117
111. Liu YT, Liu YX, Zhang MY, Li C, Zhang ZQ, Liu AP, et al. Structural characterization of a polysaccharide from Suillellus luridus and its antidiabetic activity via Nrf2/HO-1 and NF-κB pathways. Int J Biol Macromol. (2020) 162:935–45. doi: 10.1016/j.ijbiomac.2020.06.212
112. Zhang H, Li WJ, Nie SP, Chen Y, Wang YX, Xie MY. Structural characterisation of a novel bioactive polysaccharide from Ganoderma atrum. Carbohydr Polym. (2012) 88:1047–54.
113. Hu XY, Yu Q, Hou KY, Ding XM, Chen Y, Xie JH, et al. Regulatory effects of Ganoderma atrum polysaccharides on LPS-induced inflammatory macrophages model and intestinal-like Caco-2/macrophages co-culture inflammation model. Food Chem Toxicol. (2020) 140:111321. doi: 10.1016/j.fct.2020.111321
114. Zhao FQ, Zhu KX, Zhao QC, Liu QB, Cao J, Xia GH, et al. Holothuria leucospilota polysaccharides alleviate liver injury via AMPK and NF-κB signaling pathways in type 2 diabetic rats. J Funct Foods. (2021) 85:104657.
115. Zhou TY, Xiang XW, Du M, Zhang LF, Cheng NX, Liu XL, et al. Protective effect of polysaccharides of sea cucumber Acaudina leucoprocta on hydrogen peroxide-induced oxidative injury in RAW264. 7 cells. Int J Biol Macromol. (2019) 139:1133–40. doi: 10.1016/j.ijbiomac.2019.08.092
116. Hou RR, Xu TL, Li Q, Yang FF, Wang CY, Huang TT, et al. Polysaccharide from Echinacea purpurea reduce the oxidant stress in vitro and in vivo. Int J Biol Macromol. (2020) 149:41–50. doi: 10.1016/j.ijbiomac.2020.01.129
117. Wang H, Li YS, Liu JF, Di DL, Liu YW, Wei JT. Hepatoprotective effect of crude polysaccharide isolated from Lycium barbarum L. against alcohol-induced oxidative damage involves Nrf2 signaling. Food Sci Nutr. (2020) 8:6528–38. doi: 10.1002/fsn3.1942
118. Wei JT, Zhang LH, Liu JF, Pei D, Wang NL, Wang H, et al. Protective effect of Lycium barbarum polysaccharide on ethanol-induced injury in human hepatocyte and its mechanism. J Food Biochem. (2020) 44:e13412. doi: 10.1111/jfbc.13412
119. Tang YB, Lv JP, Wang J, Li B, Zhang QH, Liu ZQ, et al. Sagittaria sagittifolia polysaccharide, a Chinese herbal extract, protects against isoniazid-and rifampicin-induced hepatotoxicity in in-vitro model. Pharmacogn Mag. (2020) 16:637.
120. Zeng D, Wang YP, Chen Y, Li DY, Li GL, Xiao HX, et al. Angelica polysaccharide antagonizes 5-FU-induced oxidative stress injury to reduce apoptosis in the liver through Nrf2 pathway. Front Oncol. (2021) 11:720620. doi: 10.3389/fonc.2021.720620
121. Cao SM, Du JL, Hei QH. Lycium barbarum polysaccharide protects against neurotoxicity via the Nrf2-HO-1 pathway. Exp Ther Med. (2017) 14:4919–27. doi: 10.3892/etm.2017.5127
122. Yuan LL, Duan XW, Zhang RT, Zhang YB, Qu MW. Aloe polysaccharide protects skin cells from UVB irradiation through Keap1/Nrf2/ARE signal pathway. J Dermatol Treat. (2020) 31:300–8. doi: 10.1080/09546634.2019.1591579
123. Byun EB, Cho EJ, Kim YE, Kim WS, Byun EH. Neuroprotective effect of polysaccharide separated from Perilla frutescens Britton var. acuta Kudo against H2O2-induced oxidative stress in HT22 hippocampus cells. Biosci Biotechnol Biochem. (2018) 82:1344–58. doi: 10.1080/09168451.2018.1460572
124. Liu JJ, Zhao GX, He LL, Wang Z, Zibrila AI, Niu BC, et al. Lycium barbarum polysaccharides inhibit ischemia/reperfusion-induced myocardial injury via the Nrf2 antioxidant pathway. Toxcol Rep. (2021) 8:657–67. doi: 10.1016/j.toxrep.2021.03.019
125. Jiang Y, Zhou W, Zhang X, Wang Y, Yang DY, Li SJ. Protective effect of blood cora polysaccharides on H9c2 rat heart cells injury induced by oxidative stress by activating Nrf2/HO-1 signal pathway. Front Nutr. (2021) 8:73. doi: 10.3389/fnut.2021.632161
126. Jia YN, Wang YJ, Li RL, Li SQ, Zhang M, He CW, et al. The structural characteristic of acidic-hydrolyzed corn silk polysaccharides and its protection on the H2O2-injured intestinal epithelial cells. Food Chem. (2021) 356:129691. doi: 10.1016/j.foodchem.2021.129691
127. Chen YJ, Wang JY, Li JT, Zhu JH, Wang RQ, Xi QH, et al. Astragalus polysaccharide prevents ferroptosis in a murine model of experimental colitis and human Caco-2 cells via inhibiting NRF2/HO-1 pathway. Eur J Pharmacol. (2021) 911:174518. doi: 10.1016/j.ejphar.2021.174518
128. Zhang T, Shi L, Li Y, Mu W, Zhang HM, Li Y, et al. Polysaccharides extracted from Rheum tanguticum ameliorate radiation-induced enteritis via activation of Nrf2/HO-1. J Radiat Res. (2021) 62:46–57. doi: 10.1093/jrr/rraa093
129. Yun LY, Wu T, Mao ZT, Li W, Zhang M, Sun XT. A novel wheat germ polysaccharide: structural characterization, potential antioxidant activities and mechanism. Int J Biol Macromol. (2020) 165:1978–87. doi: 10.1016/j.ijbiomac.2020.10.112
130. Wu JS, Huang R, Jiao DX, Liu SY, Liu HM, Liu HZ. Protection by Hosta ventricosa polysaccharides against oxidative damage induced by t-BHP in HepG2 cells via the JNK/Nrf2 pathway. Int J Biol Macromol. (2022) 208:453–62. doi: 10.1016/j.ijbiomac.2022.03.134
131. Qin T, Ren Z, Liu XP, Luo Y, Long Y, Peng S, et al. Study of the selenizing Codonopsis pilosula polysaccharides protects RAW264. 7 cells from hydrogen peroxide-induced injury. Int J Biol Macromol. (2019) 125:534–43. doi: 10.1016/j.ijbiomac.2018.12.025
132. Park CM, Cho CW, Song YS. TOP 1 and 2, polysaccharides from Taraxacum officinale, inhibit NFκB-mediated inflammation and accelerate Nrf2-induced antioxidative potential through the modulation of PI3K-Akt signaling pathway in RAW 264.7 cells. Food Chem Toxicol. (2014) 66:56–64. doi: 10.1016/j.fct.2014.01.019
133. Wang LX, Xie YH, Yang WR, Yang ZB, Jiang SZ, Zhang CY, et al. Alfalfa polysaccharide prevents H2O2-induced oxidative damage in MEFs by activating MAPK/Nrf2 signaling pathways and suppressing NF-κB signaling pathways. Sci. Rep. (2019) 9:1–11. doi: 10.1038/s41598-018-38466-7
134. Hu YB, Huang JH, Li YX, Jiang L, Ouyang YJ, Li YM, et al. Cistanche deserticola polysaccharide induces melanogenesis in melanocytes and reduces oxidative stress via activating NRF2/HO-1 pathway. J Cell Mol Med. (2020) 24:4023–35. doi: 10.1111/jcmm.15038
135. Yang Y, Li W, Li Y, Wang Q, Gao L, Zhao JJ. Dietary Lycium barbarum polysaccharide induces Nrf2/ARE pathway and ameliorates insulin resistance induced by high-fat via activation of PI3K/AKT signaling. Oxid Med Cell Longev. (2014) 2014:145641. doi: 10.1155/2014/145641
136. Wang WJ, Li S, Song MX. Polygonatum sibiricum polysaccharide inhibits high glucose-induced oxidative stress, inflammatory response, and apoptosis in RPE cells. J Recept Signal Transduct. (2022) 42:189–96. doi: 10.1080/10799893.2021.1883061
137. Cai JL, Zhu YL, Zuo YJ, Tong QZ, Zhang ZG, Yang L, et al. Polygonatum sibiricum polysaccharide alleviates inflammatory cytokines and promotes glucose uptake in high-glucose-and high-insulin-induced 3T3-L1 adipocytes by promoting Nrf2 expression. Mol Med Rep. (2019) 20:3951–8. doi: 10.3892/mmr.2019.10626
138. Chen Y, Bi Q, Zhu ZG, Zhang SJ, Xu JF, Dou XF, et al. Lycium barbarum polysaccharides exert an antioxidative effect on rat chondrocytes by activating the nuclear factor (erythroid-derived 2)-like 2 signaling pathway. Arch Med Sci. (2020) 16:964–73. doi: 10.5114/aoms.2018.77036
139. Liang R, Zhao Q, Zhu Q, He X, Gao MJ, Wang YR. Lycium barbarum polysaccharide protects ARPE-19 cells against H 2 O 2-induced oxidative stress via the Nrf2/HO-1 pathway. Mol Med Rep. (2021) 24:1–8. doi: 10.3892/mmr.2021.12409
140. Peng H, Yang M, Guo Q, Su T, Xiao Y, Xia ZY. Dendrobium officinale polysaccharides regulate age-related lineage commitment between osteogenic and adipogenic differentiation. Cell Prolif. (2019) 52:e12624. doi: 10.1111/cpr.12624
141. Fu YH, Hu X, Zhou DY, Li X, Tao XY, Yang D, et al. Anti-osteoporotic effect of viscozyme-assisted polysaccharide extracts from Portulaca oleracea L. on H2O2-treated MC3T3-E1 cells and zebrafish. Separations. (2022) 9:128.
142. Li HP, Li ZJ, Peng LQ, Jiang N, Liu Q, Zhang ET, et al. Lycium barbarum polysaccharide protects human keratinocytes against UVB-induced photo-damage. Free Radical Res. (2017) 51:200–10. doi: 10.1080/10715762.2017.1294755
143. Huang B, Zheng WK, Xu ZW, Chen YP. Impact of Lycium barbarum polysaccharide on apoptosis in Mycoplasma-infected splenic lymphocytes. Trop J Pharm Res. (2017) 16:2127–33.
144. Meng HH, Wu JJ, Shen L, Chen GW, Jin L, Yan MX, et al. Microwave assisted extraction, characterization of a polysaccharide from Salvia miltiorrhiza Bunge and its antioxidant effects via ferroptosis-mediated activation of the Nrf2/HO-1 pathway. Int J Biol Macromol. (2022) 215:398–412. doi: 10.1016/j.ijbiomac.2022.06.064
145. Li J, Wang X, Zhou R, Cheng F, Tang X, Lao J, et al. Polygonatum cyrtonema Hua polysaccharides protect BV2 microglia relief oxidative stress and ferroptosis by regulating NRF2/HO-1 PATHWAY. Molecules. (2022) 27:7088. doi: 10.3390/molecules27207088
146. Sun MM, He N, Lv ZG. Polysaccharides extracted from Cassia seeds protect against high glucose-induced retinal endothelial cell injury. Int Ophthalmol. (2021) 41:2465–72. doi: 10.1007/s10792-021-01801-5
147. Lin GS, Luo DD, Liu JJ, Wu XL, Chen JF, Huang QH, et al. Hepatoprotective effect of polysaccharides isolated from Dendrobium officinale against acetaminophen-induced liver injury in mice via regulation of the Nrf2-Keap1 signaling pathway. Oxid Med Cell Longev. (2018) 2018:6962439. doi: 10.1155/2018/6962439
148. Nepali S, Ki HH, Lee JH, Cha JY, Lee YM, Kim DK. Triticum aestivum sprout-derived polysaccharide exerts hepatoprotective effects against ethanol-induced liver damage by enhancing the antioxidant system in mice. Int J Mol Med. (2017) 40:1243–52. doi: 10.3892/ijmm.2017.3095
149. Farag MR, Elhady WM, Ahmed SY, Taha HS, Alagawany M. Astragalus polysaccharides alleviate tilmicosin-induced toxicity in rats by inhibiting oxidative damage and modulating the expressions of HSP70, NF-kB and Nrf2/HO-1 pathway. Res Vet Sci. (2019) 124:137–48. doi: 10.1016/j.rvsc.2019.03.010
150. Hamid M, Liu DD, Abdulrahim Y, Liu YH, Qian G, Khan A, et al. Amelioration of CCl4-induced liver injury in rats by selenizing Astragalus polysaccharides: role of proinflammatory cytokines, oxidative stress and hepatic stellate cells. Res Vet Sci. (2017) 114:202–11. doi: 10.1016/j.rvsc.2017.05.002
151. Wang J, Luo WZ, Li B, Lv JP, Ke XH, Ge DY, et al. Sagittaria sagittifolia polysaccharide protects against isoniazid-and rifampicin-induced hepatic injury via activation of nuclear factor E2-related factor 2 signaling in mice. J Ethnopharmacol. (2018) 227:237–45. doi: 10.1016/j.jep.2018.09.002
152. Deng XQ, Ke XH, Tang YB, Luo WZ, Dong RJ, Ge DY, et al. Sagittaria sagittifolia polysaccharide interferes with arachidonic acid metabolism in non-alcoholic fatty liver disease mice via Nrf2/HO-1 signaling pathway. Biomed Pharmacother. (2020) 132:110806. doi: 10.1016/j.biopha.2020.110806
153. Han C, Wei YY, Wang X, Cui YQ, Bao YZ, Shi WY. Salvia miltiorrhiza polysaccharides protect against lipopolysaccharide-induced liver injury by regulating NF-κb and Nrf2 pathway in mice. Food Agric Immunol. (2019) 30:979–94.
154. Huang YY, Zhou F, Shen C, Wang HX, Xiao YD. LBP reduces theinflammatory injuryof kidney in septic rat and regulates the Keap1-Nrf2/ ARE signaling pathway. Acta Cir Bras. (2019) 34:e20190010000003. doi: 10.1590/s0102-865020190010000003
155. Wu Q, Liu LT, Wang XY, Lang ZF, Meng XH, Guo SF, et al. Lycium barbarum polysaccharides attenuate kidney injury in septic rats by regulating Keap1-Nrf2/ARE pathway. Life Sci. (2020) 242:117240. doi: 10.1016/j.lfs.2019.117240
156. Xie W, Huang YY, Chen HG, Zhou X. Study on the efficacy and mechanism of Lycium barbarum polysaccharide against lead-induced renal injury in mice. Nutrients. (2021) 13:2945. doi: 10.3390/nu13092945
157. Raish M, Ahmad A, Jan BL, Alkharfy KM, Ansari MA, Mohsin K, et al. Momordica charantia polysaccharides mitigate the progression of STZ induced diabetic nephropathy in rats. Int J Biol Macromol. (2016) 91:394–9. doi: 10.1016/j.ijbiomac.2016.05.090
158. Zheng GZ, Ren HJ, Li HQ, Li XH, Dong TC, Xu SM, et al. Lycium barbarum polysaccharide reduces hyperoxic acute lung injury in mice through Nrf2 pathway. Biomed Pharmacother. (2019) 111:733–9. doi: 10.1016/j.biopha.2018.12.073
159. Sun Y, Liu J, Wan L, Wang F, Zhang XJ, Qi YJ. Improving effects of Astragalus polysaccharides on cardiac function via Keap1/Nrf2-ARE signal pathway in adjuvant arthritis rats. Chin Herb Med. (2016) 8:143–53.
160. Xue R, Du M, Zhou TY, Ai WZ, Zhang ZS, Xiang XW, et al. Polysaccharides from hemp seed protect against cyclophosphamide-induced intestinal oxidative damage via Nrf2-Keap1 signaling pathway in mice. Oxid Med Cell Longev. (2020) 2020:1813798. doi: 10.1155/2020/1813798
161. Zhao Y, Sun YZ, Wang GY, Ge SC, Liu HN. Dendrobium officinale polysaccharides protect against MNNG-induced PLGC in rats via activating the NRF2 and antioxidant enzymes HO-1 and NQO-1. Oxid Med Cell Longev. (2019) 2019:9310245. doi: 10.1155/2019/9310245
162. Chen QY, Wang Y, Yin N, Wang RF, Zheng Y, Yang YP, et al. Polysaccharides from fermented wheat bran enhanced the growth performance of zebrafish (Danio rerio) through improving gut microflora and antioxidant status. Aquacult Rep. (2022) 25:101188.
163. Tang LJ, Bao SY, Du Y, Jiang ZY, Wuliji A, Ren X, et al. Antioxidant effects of Lycium barbarum polysaccharides on photoreceptor degeneration in the light-exposed mouse retina. Biomed Pharmacother. (2018) 103:829–37. doi: 10.1016/j.biopha.2018.04.104
164. Yang SJ, Sun JQ, Gu DX, Li P, Yao LL, Shi DY, et al. Antioxidant activities of sulfated Codonopsis polysaccharides in acute oxidative stress. J Food Biochem. (2021) 45:e13974. doi: 10.1111/jfbc.13974
165. Liao ZZ, Zhang JY, Wang JY, Yan TX, Xu FX, Wu B, et al. The anti-nephritic activity of a polysaccharide from okra (Abelmoschus esculentus (L.) Moench) via modulation of AMPK-Sirt1-PGC-1α signaling axis mediated anti-oxidative in type 2 diabetes model mice. Int J Biol Macromol. (2019) 140:568–76. doi: 10.1016/j.ijbiomac.2019.08.149
166. Liao ZZ, Zhang JY, Liu B, Yan TX, Xu FX, Xiao F, et al. Polysaccharide from okra (Abelmoschus esculentus (L.) Moench) improves antioxidant capacity via PI3K/AKT pathways and Nrf2 translocation in a type 2 diabetes model. Molecules. (2019) 24:1906. doi: 10.3390/molecules24101906
167. Qin XD, Hua J, Lin SJ, Zheng HJ, Wang JJ, Li W, et al. Astragalus polysaccharide alleviates cognitive impairment and β-amyloid accumulation in APP/PS1 mice via Nrf2 pathway. Biochem Biophys Res Commun. (2020) 531:431–7. doi: 10.1016/j.bbrc.2020.07.122
168. Zhu L, Han YG, Wang HM. Efficacy of Lycium barbarum polysaccharide and synergism with paclitaxel/cisplatin in ovarian cancer in mice. Trop J Pharm Res. (2017) 16:1645–53.
169. Yang DM, Zhang JM, Fei YF. Lycium barbarum polysaccharide attenuates chemotherapy-induced ovarian injury by reducing oxidative stress. J Obstet Gynaecol Res. (2017) 43:1621–8. doi: 10.1111/jog.13416
170. Yao WW, Hong ZP, Chen XZ, Liu B, Lu CB. DOP1 attenuates cisplatin-induced reproductive injury in male mice via the Nrf2/ARE signaling pathway. Int J Sci. (2021) 8:101–11.
171. He MH, Pan H, Chang RC, So KF, Brecha NC, Pu M. Activation of the Nrf2/HO-1 antioxidant pathway contributes to the protective effects of Lycium barbarum polysaccharides in the rodent retina after ischemia-reperfusion-induced damage. PLoS One. (2014) 9:e84800. doi: 10.1371/journal.pone.0084800
172. Zou CY, Fang YK, Lin NY, Liu HF. Polysaccharide extract from pomelo fruitlet ameliorates diet-induced nonalcoholic fatty liver disease in hybrid grouper (Epinephelus lanceolatus♂ × Epinephelus fuscoguttatus♀). Fish Shellfish Immunol. (2021) 119:114–27. doi: 10.1016/j.fsi.2021.09.034
173. Li Q, Qin XK, Yu Y, Quan SJ, Xiao P. Schisandra chinensis polysaccharides exerts anti-oxidative effect in vitro through Keap1-Nrf2-ARE pathway. Food Sci Tech Brazil. (2022) 42:e44621.
174. Li WL, Lin K, Zhou M, Xiong Q, Li CY, Ru Q. Polysaccharides from Opuntia milpa alta alleviate alloxan-induced INS-1 cells apoptosis via reducing oxidative stress and upregulating Nrf2 expression. Nutr Res. (2020) 77:108–18. doi: 10.1016/j.nutres.2020.02.004
175. Zhong J, Fang L, Chen R, Xu J, Guo D, Guo C, et al. Polysaccharides from sporoderm-removed spores of Ganoderma lucidum induce apoptosis in human gastric cancer cells via disruption of autophagic flux. Oncol Lett. (2021) 21:425. doi: 10.3892/ol.2021.12686
176. Chen C, Kang MS, Wang QW, Liu WL, Yang MG, Liang SX, et al. Combination of Anoectochilus roxburghii polysaccharide and exercise ameliorates diet-induced metabolic disorders in obese mice. Front Nutr. (2021) 658:735501. doi: 10.3389/fnut.2021.735501
177. Zhang Y, Han Y, He J, Ouyang KH, Zhao M, Cai L, et al. Digestive properties and effects of Chimonanthus nitens Oliv polysaccharides on antioxidant effects in vitro and in immunocompromised mice. Int J Biol Macromol. (2021) 185:306–16. doi: 10.1016/j.ijbiomac.2021.06.114
178. Xing YY, Zheng YK, Yang S, Zhang LH, Guo SW, Shi LL, et al. Artemisia ordosica polysaccharide alleviated lipopolysaccharide-induced oxidative stress of broilers via Nrf2/Keap1 and TLR4/NF-κB pathway. Ecotoxicol Environ Saf. (2021) 223:112566. doi: 10.1016/j.ecoenv.2021.112566
179. Mohammadi G, Karimi AA, Hafezieh M, Dawood MA, Abo-Al-Ela HG. Pistachio hull polysaccharide protects Nile tilapia against LPS-induced excessive inflammatory responses and oxidative stress, possibly via TLR2 and Nrf2 signaling pathways. Fish Shellfish Immunol. (2022) 121:276–84. doi: 10.1016/j.fsi.2021.12.042
180. Yuan CF, Wang CD, Bu YQ, Xiang TX, Huang XN, Wang ZW, et al. Antioxidative and immunoprotective effects of Pyracantha fortuneana (Maxim.) Li polysaccharides in mice. Immunol Lett. (2010) 133:14–8. doi: 10.1016/j.imlet.2010.04.004
181. Yuan C, Li Z, Peng F, Xiao F, Ren D, Xue H, et al. Combination of selenium-enriched green tea polysaccharides and Huo-ji polysaccharides synergistically enhances antioxidant and immune activity in mice. J Sci Food Agric. (2015) 95:3211–7. doi: 10.1002/jsfa.7287
182. Wang YQ, Wei JG, Tu MJ, Gu JG, Zhang W. Fucoidan alleviates acetaminophen-induced hepatotoxicity via oxidative stress inhibition and Nrf2 translocation. Int J Mol Sci. (2018) 19:4050. doi: 10.3390/ijms19124050
183. Liu X, Liu HM, Zhai Y, Li Y, Zhu X, Zhang W. Laminarin protects against hydrogen peroxide-induced oxidative damage in MRC-5 cells possibly via regulating NRF2. PeerJ. (2017) 5:e3642. doi: 10.7717/peerj.3642
184. Jayawardena TU, Wang L, Sanjeewa KA, Kang SI, Lee J, Jeon Y. Antioxidant potential of sulfated polysaccharides from Padina boryana; protective effect against oxidative stress in in vitro and in vivo zebrafish model. Mar Drugs. (2020) 18:212. doi: 10.3390/md18040212
185. Zhang Y, Zuo JH, Yan LP, Cheng Y, Li QJ, Wu SY, et al. Sargassum fusiforme fucoidan alleviates high-fat diet-induced obesity and insulin resistance associated with the improvement of hepatic oxidative stress and gut microbiota profile. J Agric Food Chem. (2020) 68:10626–38. doi: 10.1021/acs.jafc.0c02555
186. Zhu DZ, Wang YT, Zhuo YL, Zhu KJ, Wang XZ, Liu AJ. Fucoidan inhibits LPS-induced acute lung injury in mice through regulating GSK-3β-Nrf2 signaling pathway. Arch Pharmacal Res. (2020) 43:646–54. doi: 10.1007/s12272-020-01234-1
187. Liu WC, Zhu YR, Zhao ZH, Jiang P, Yin FQ. Effects of dietary supplementation of algae-derived polysaccharides on morphology, tight junctions, antioxidant capacity and immune response of duodenum in broilers under heat stress. Animals. (2021) 11:2279. doi: 10.3390/ani11082279
188. Chen P, Yang S, Hu C, Zhao Z, Liu J, Cheng Y, et al. Sargassum fusiforme polysaccharide rejuvenat es the small intestine in mice through altering its physiol ogy and gut microbiota composition. Curr Mol Med. (2017) 17:350–8. doi: 10.2174/1566524018666171205115516
189. Lekshmi V, Rauf AA, Kurup GM. Sulfated polysaccharides from the edible marine algae Padina tetrastromatica attenuates isoproterenol-induced oxidative damage via activation of PI3K/Akt/Nrf2 signaling pathway-an in vitro and in vivo approach. Chem Biol Interact. (2019) 308:258–68. doi: 10.1016/j.cbi.2019.05.044
190. Liu WC, Zhuang DP, Zhao Y, Balasubramanian B, Zhao ZH. Seaweed-derived polysaccharides attenuate heat stress-induced splenic oxidative stress and inflammatory response via regulating Nrf2 and NF-κB signaling pathways. Mar Drugs. (2022) 20:358. doi: 10.3390/md20060358
191. Yi RK, Deng L, Mu JF, Li C, Tan F, Zhao X. The impact of antarctic ice microalgae polysaccharides on d-galactose-induced oxidative damage in mice. Front Nutr. (2021) 8:651088. doi: 10.3389/fnut.2021.651088
192. Yang Y, Han CY, Sheng YJ, Wang J, Li WY, Zhou XH, et al. Antrodia camphorata polysaccharide improves inflammatory response in liver injury via the ROS/TLR4/NF−κB signal. J Cell Mol Med. (2022) 26:2706–16. doi: 10.1111/jcmm.17283
193. Xu F, Li X, Xiao X, Liu LF, Zhang L, Lin PP, et al. Effects of Ganoderma lucidum polysaccharides against doxorubicin-induced cardiotoxicity. Biomed Pharmacother. (2017) 95:504–12. doi: 10.1016/j.biopha.2017.08.118
194. Shi XQ, Cheng WJ, Wang Q, Zhang JC, Wang CT, Li M, et al. Exploring the protective and reparative mechanisms of G. lucidum polysaccharides against H2O2-induced oxidative stress in human skin fibroblasts. Clin Cosmet Investig Dermatol. (2021) 14:1481. doi: 10.2147/CCID.S334527
195. Yu CW, Chen H, Du DH, Lv WT, Li SJ, Li DF, et al. β-Glucan from Saccharomyces cerevisiae alleviates oxidative stress in LPS-stimulated RAW264. 7 cells via Dectin-1/Nrf2/HO-1 signaling pathway. Cell Stress Chaperon. (2021) 26:629–37. doi: 10.1007/s12192-021-01205-5
196. Han YQ, Nan SJ, Fan J, Chen QH, Zhang YZ. Inonotus obliquus polysaccharides protect against Alzheimer’s disease by regulating Nrf2 signaling and exerting antioxidative and antiapoptotic effects. Int J Biol Macromol. (2019) 131:769–78. doi: 10.1016/j.ijbiomac.2019.03.033
197. Fu H, You SQ, Zhao D, An Q, Zhang JC, Wang CT, et al. Tremella fuciformis polysaccharides inhibit UVA-induced photodamage of human dermal fibroblast cells by activating up-regulating Nrf2/Keap1 pathways. J Cosmet Dermatol. (2021) 20:4052–9. doi: 10.1111/jocd.14051
198. Cao XY, Liu D, Xia Y, Cai TG, Liu JL. A novel polysaccharide from Lentinus edodes mycelia protects MIN6 cells against high glucose-induced damage via the MAPKs and Nrf2 pathways. Food Nutr Res. (2019) 63:1598. doi: 10.29219/fnr.v63.1598
199. Zong S, Li JL, Yang L, Huang QL, Hou GH, Ye ZY, et al. Mechanism of bioactive polysaccharide from Lachnum sp. acts synergistically with 5-fluorouracil against human hepatocellular carcinoma. J Cell Physiol. (2019) 234:15548–62. doi: 10.1002/jcp.28202
200. Li HN, Zhao LL, Zhou DY, Chen DQ. Ganoderma lucidum polysaccharides ameliorates hepatic steatosis and oxidative stress in db/db mice via targeting nuclear factor E2 (erythroid-derived 2)-related factor-2/heme oxygenase-1 (HO-1) pathway. Med Sci Monit. (2020) 26:e921905. doi: 10.12659/MSM.921905
201. Wang CH, Yang SX, Gao L, Wang LL, Cao L. Carboxymethyl pachyman (CMP) reduces intestinal mucositis and regulates the intestinal microflora in 5-fluorouracil-treated CT26 tumour-bearing mice. Food Funct. (2018) 9:2695–704. doi: 10.1039/c7fo01886j
202. Liu YG, Yang AH, Qu YD, Wang ZQ, Zhang YQ, Liu Y, et al. Ameliorative effects of Antrodia cinnamomea polysaccharides against cyclophosphamide-induced immunosuppression related to Nrf2/HO-1 signaling in BALB/c mice. Int J Biol Macromol. (2018) 116:8–15. doi: 10.1016/j.ijbiomac.2018.04.178
203. Wang X, Wang ZQ, Wu HH, Jia W, Teng LS, Song J, et al. Sarcodon imbricatus polysaccharides protect against cyclophosphamide-induced immunosuppression via regulating Nrf2-mediated oxidative stress. Int J Biol Macromol. (2018) 120:736–44. doi: 10.1016/j.ijbiomac.2018.08.157
204. Lin ST, Hao GX, Long M, Lai F, Li QQ, Xiong YM, et al. Oyster (Ostrea plicatula Gmelin) polysaccharides intervention ameliorates cyclophosphamide–induced genotoxicity and hepatotoxicity in mice via the Nrf2–are pathway. Biomed Pharmacother. (2017) 95:1067–71. doi: 10.1016/j.biopha.2017.08.058
205. Liu HZ, Zhang YB, Li MW, Luo P. Beneficial effect of Sepia esculenta ink polysaccharide on cyclophosphamide-induced immunosuppression and ovarian failure in mice. Int J Biol Macromol. (2019) 140:1098–105. doi: 10.1016/j.ijbiomac.2019.08.200
206. Le XY, Luo P, Gu YP, Tao YX, Liu HZ. Squid ink polysaccharide reduces cyclophosphamide-induced testicular damage via Nrf2/ARE activation pathway in mice. Iran J Basic Med Sci. (2015) 18:827.
207. Wen ZS, Tang Z, Ma L, Zhu TL, Wang YM, Xiang XW, et al. Protective effect of low molecular weight seleno-aminopolysaccharide on the intestinal mucosal oxidative damage. Mar Drugs. (2019) 17:64. doi: 10.3390/md17010064
208. Ren H, Li ZY, Gao R, Zhao TX, Luo D, Yu ZH, et al. Structural characteristics of Rehmannia glutinosa polysaccharides treated using different decolorization processes and their antioxidant effects in intestinal epithelial cells. Foods. (2022) 11:3449. doi: 10.3390/foods11213449
209. Li XP, Cui WJ, Cui YF, Song XL, Jia L, Zhang JJ. Stropharia rugoso-annulata acetylated polysaccharides alleviate NAFLD via Nrf2/JNK1/AMPK signaling pathways. Int J Biol Macromol. (2022) 215:560–70. doi: 10.1016/j.ijbiomac.2022.06.156
210. Chen QY, Wang RF, Wang Y, An XP, Liu N, Song M, et al. Characterization and antioxidant activity of wheat bran polysaccharides modified by Saccharomyces cerevisiae and Bacillus subtilis fermentation. J Cereal Sci. (2021) 97:103157.
211. Chen PC, He D, Zhang Y, Yang SS, Chen LJ, Wang SQ, et al. Sargassum fusiforme polysaccharides activate antioxidant defense by promoting Nrf2-dependent cytoprotection and ameliorate stress insult during aging. Food Funct. (2016) 7:4576–88. doi: 10.1039/c6fo00628k
Keywords: natural polysaccharides, Nrf2 antioxidant pathway, structural features, regulatory effects, structure-activity relationship, health-promoting
Citation: Luo J-H, Li J, Shen Z-C, Lin X-F, Chen A-Q, Wang Y-F, Gong E-S, Liu D, Zou Q and Wang X-Y (2023) Advances in health-promoting effects of natural polysaccharides: Regulation on Nrf2 antioxidant pathway. Front. Nutr. 10:1102146. doi: 10.3389/fnut.2023.1102146
Received: 18 November 2022; Accepted: 30 January 2023;
Published: 16 February 2023.
Edited by:
Vignesh Muthusamy, Indian Agricultural Research Institute (ICAR), IndiaReviewed by:
Veda Krishnan, Indian Agricultural Research Institute (ICAR), IndiaAmirtham Dhamodarasamy, Tamil Nadu Agricultural University, India
Copyright © 2023 Luo, Li, Shen, Lin, Chen, Wang, Gong, Liu, Zou and Wang. This is an open-access article distributed under the terms of the Creative Commons Attribution License (CC BY). The use, distribution or reproduction in other forums is permitted, provided the original author(s) and the copyright owner(s) are credited and that the original publication in this journal is cited, in accordance with accepted academic practice. No use, distribution or reproduction is permitted which does not comply with these terms.
*Correspondence: Dan Liu, danliu@zjsru.edu.cn; Xiao-Yin Wang,
xywang@gmu.edu.cn
†These authors share first authorship