- 1Gannan Medical University, Ganzhou City, Jiangxi, China
- 2Department of Rehabilitation Medicine, First Affiliated Hospital of Gannan Medical University, Ganzhou City, Jiangxi, China
- 3Ganzhou Intelligent Rehabilitation Technology Innovation Center, Ganzhou, Jiangxi, China
- 4School of Rehabilitation, Capital Medical University, Beijing, China
- 5Beijing Bo'ai Hospital, China Rehabilitation Research Center, Beijing, China
- 6Ganzhou Key Laboratory of Rehabilitation Medicine, Ganzhou City, Jiangxi, China
Objective: We performed a systematic review and meta-analysis of existing randomized controlled trials (RCTs) to assess whether dietary supplements can prevent loss of muscle mass and strength during muscle disuse.
Methods: We searched the following databases: PubMed, Embase, Cochrane, Scopus, Web of Science, and CINAHL for RCTs assessing the effect of dietary supplements on disuse muscular atrophy without language and time restrictions. Muscle strength and leg lean mass were used as the primary outcome indicators. Muscle cross-sectional area (CSA), muscle fiber type distribution, peak aerobic capacity and muscle volume were used as secondary outcome indicators. The risk of bias was assessed using the Cochrane Collaboration's Risk of Bias tool. Heterogeneity was tested using the I2 statistic index. Mean and standard deviation of outcome indicators were extracted from the intervention and control groups to calculate effect sizes and 95% confidence intervals, with the significance level set at P < 0.05.
Results: Twenty RCTs were included with a total of 339 subjects. The results showed that dietary supplements had no effect on muscle strength, CSA, muscle fiber type distribution, peak aerobic capacity or muscle volume. But dietary supplements have a protective effect on the lean mass of the legs.
Conclusion: Dietary supplements can improve lean leg mass, but did not show a tendency to have an effect on muscle strength, CSA, muscle fiber type distribution, peak aerobic capacity or muscle volume during muscle disuse.
Systematic review registration: https://www.crd.york.ac.uk/PROSPERO/#recordDetails, identifier: CRD42022370230.
1. Introduction
Patients recovering from an illness or injury usually require a period of bed rest or limb immobilization. However even a short period of inactivity can result in a significant loss of skeletal muscle mass and strength (1, 2), which might lead to a longer recovery period and a higher risk of disease recurrence (3–6). Skeletal muscle is the most abundant tissue in the body, and when it atrophies, it affects the metabolism throughout the body, such as reduced insulin sensitivity (7, 8), decreased basal metabolic rate (9), and increased body fat mass (10). These factors will affect body functions and result in more serious health problems. Therefore, it is necessary to take measures to prevent loss of muscle mass and strength during periods of inactivity.
It is well known that exercise is the best way to maintain and increase muscle mass (11–13). However, in many cases, patients are not allowed to exercise, so other methods are needed to prevent skeletal muscle atrophy. Loss of skeletal muscle mass due to muscle disuse is attributed to a long-term imbalance between muscle protein synthesis and catabolic rates. A decrease in the basal muscle protein synthesis rate has been reported following bed rest (14, 15) and limb immobilization (16, 17). Previous studies found that supplementation with nutrients, such as dietary protein and essential amino acids, can reduce muscle loss and increase muscle growth during immobilization and aging by stimulating muscle protein synthesis (18). The growth of skeletal muscle is traditionally referred to as skeletal muscle hypertrophy, which is manifested as an increase in muscle mass, muscle thickness, muscle area, muscle volume, and muscle fiber cross-sectional area (CSA) (19). However, it remains unclear about the effect of dietary supplements on disuse muscular atrophy. Some randomized controlled trials (RCTs) showed that nutritional supplementation protected against skeletal muscle atrophy during disuse (20, 21), while some RCTs have showed the opposite results (22, 23). Therefore, a meta-analysis of existing RCTs is necessary to provide updated evidence on the effectiveness of dietary supplements in the treatment of disuse muscular atrophy.
2. Methods
This review follows the recommendations of the Preferred Reporting Items for Systematic Reviews and Meta-Analyses and the Cochrane collaboration for systematic reviews (24). It answers the following research question: Do dietary supplements prevent loss of muscle mass and strength during muscle disuse? The study is registered on PROSPERO, and the registration number is CRD42022370230.
2.1. Search strategy
We searched the PubMed, Embase, Cochrane, Scopus, Web of Science, CINAHL databases for RCTs assessing the effect of dietary supplements on disuse muscular atrophy from the year of inception to December 15, 2022 with no language restrictions. The search terms were (disused muscle atrophy OR skeletal muscle disuse atrophy OR muscle disuse OR disuse atrophy OR muscle disuse atrophy OR disuse atrophies OR immobilization OR immobilization-induced atrophy OR bed rest OR bed rests OR rest, bed OR rests, bed) AND (dietary supplements OR diet therapy OR nutrition therapy OR dietary supplement OR supplements, dietary OR dietary supplementations OR supplementations, dietary OR food supplementations OR food supplements OR food supplement OR supplement, food OR supplements, food OR therapy, nutrition OR nutrition OR diet therapies OR therapy, diet) AND (randomized controlled trial OR controlled trial OR clinical trial). The detailed search strategy was shown in Supplementary Table S1. Furthermore, we manually searched the reference lists of eligible studies to identify additional studies.
2.2. Study selection
Two authors (J-MY, YL) independently screened and selected the studies, with disagreements resolved by a third author (HY). The inclusion criteria for the studies were based on the PICOS (patients, intervention, comparison, outcomes, and study design) principle (25), as shown below:
Patients (P): Healthy subjects who were immobilized lower extremity or on prolonged bed rest according to experimental needs.
Intervention (I): Nutritional supplements were available in capsules, tablets, liquid, powder form, or supplements were added to the daily diet. Supplements can include macronutrients, such as proteins, carbohydrates, and fats; and/or micronutrients, such as vitamins.
Comparison (C): The control group was regular diet or placebo.
Outcomes (O): Muscle strength, leg lean mass, CSA, fiber type distribution (%): type I and type II, peak aerobic capacity and muscle volume.
Study design (S): Randomized controlled trial.
Studies were excluded if they met the following criteria:
(1) Individuals were excluded if they had musculoskeletal impairments, tumors, or critically ill, etc. (2) The intervention group provided dietary supplements combined with other physical therapy, or did not include dietary supplements. (3) Outcomes did not include outcome indicators any of interest. (4) The type of studies were not RCTs.
2.3. Data extraction
Relevant data were extracted from the included studies: (1) name of first author; (2) year of publication; (3) study population; (4) number of participants in the intervention and control groups; (5) type of dietary supplements; (6) dietary supplement dosage and duration; (7) age, sex and body mass index of study participants; (8) differences mean and standard deviation (SD) of muscle strength, leg lean mass, CSA, fiber type distribution, peak aerobic capacity, and muscle volume between control and intervention groups. If mean and SD of the differences were not available, we first tried to contact the authors. After no response, we extracted the mean and SD of the pre-intervention and post-intervention values for the control and intervention groups. If the data in the article was presented as a picture and no response was received after contacting the author for specific values, the WebPlot Digitizer tool (https://apps.automeris.io/wpd/index.zh_CN.html) was used to extract the mean and SD. Data were extracted independently by two authors (YL, HY), and disagreements were resolved by a third author (J-MY).
2.4. Quality assessment of the study
The methodological quality of the included studies was assessed independently by two raters (Y-BZ, YL) using the Physiotherapy Evidence Database (PEDro) scale, with discrepancies resolved via discussion with a third rater (FG). The PEDro scale consists of 11 items encompassing external validity (Item 1), internal validity (Items 2 to 9), and statistical reporting (Items 10 to 11). The items are as follows: (1) eligibility criteria and source, (2) random allocation, (3) concealed allocation, (4) baseline comparability, (5) blinding of participants, (6) blinding of therapists, (7) blinding of assessors, (8) adequate follow-up (>85%), (9) intention-to-treat analysis, (10) between-group statistical comparisons, and (11) reporting of point measures and measures of variability. Items are rated yes or no (1 or 0) according to whether the criterion is clearly satisfied in the study. A total PEDro score is achieved by adding the ratings of Items 2 to 11 for a combined total score between 0 and 10. Studies with scores < 4 are considered poor, 4 to 5 are considered fair, 6 to 8 are considered good and 9 to 10 are considered excellent (26).
In addition, we assessed the quality of each outcome according to the Grading of Recommendations Assessment Development and Evaluation (GRADE) criteria (27), assigning ratings of very low, low, moderate, and high, which involved assessing five domains, including risk of bias, directness of evidence, consistency, precision of results, publication bias.
2.5. Risk of bias assessment
The risk of bias for the included RCTs was assessed independently by two authors (J-HZ, YL) using the Cochrane Collaboration Network's Risk of Bias tool (28). The following items were assessed for each study: (1) random sequence generation, (2) allocation concealment, (3) blinding of participants and personnel, (4) blinding of outcome assessment, (5) incomplete outcome data, (6) selective reporting, and (7) other bias. According to the Cochrane Handbook recommendations, each item was classified as low risk, high risk (not fulfilling the criteria) or unclear risk (specific details or descriptions were not reported), and disagreements were discussed with and resolved by a third author (FG).
2.6. Data synthesis and statistical analysis
Statistical analysis was performed using Review Manager 5.3 statistical software. The mean and SD of differences, sample size were input into the statistical software. If the difference values were not directly available and after contacting the authors without a response, they were calculated using Equation ➀, where SD (b), SD (f), and SD (d) represent the SD of the baseline, final, and difference values, respectively, with a correlation coefficient R value estimated at 0.8. If the standard error (SE) was provided in the article, after contacting the author to obtain the SD without getting a response, the SD was calculated according to Equation ➁, where n represents the sample size. If the median was provided in the article, the median was considered as the mean value. Heterogeneity was tested using the I2 statistical indicator, and I2 > 50% was considered as high heterogeneity (29). If I2 > 50%, a random-effects model was used; otherwise, a fixed-effects model was used (29, 30). We reported the effect sizes using the weighted mean differences (WMD) or standardized mean differences (SMD) and 95% confidence interval (95% CI). According to the Cochrane Handbook, the choice of WMD and SMD depends on whether the outcome index evaluation criteria are the same, and WMD is chosen when they are the same, while SMD is chosen when they are not. In addition, subgroup analyses were performed to assess whether the results differed across conditions, and sensitivity analyses were performed to assess the robustness of the results.
3. Results
3.1. Search results
The flow chart of study selection was shown in Figure 1. After systematic search from the six databases and other sources, 901 studies were identified. Of which 590 remained after removing duplicates literature. Then, after screening the titles and abstracts of the articles, 533 irrelevant articles were excluded, and 57 were left. Finally, a total of 20 studies (20, 21, 23, 31–47)were included for meta-analysis, and 37 studies were excluded after reading the full article for the following reasons: (1) non-healthy subjects (n = 6), (2) no outcome of interest (n = 24), (3) conference article (n = 2), (4) upper extremity immobilization (n = 4), (5) data can't be extracted (n = 1). The list of literatures exclusion and reasons for exclusion are shown in Supplementary Table S2.
3.2. Characteristics of the included studies
The characteristics of the 20 included RCTs were shown in Table 1. A total of 339 subjects were enrolled, of which about 22% were women, the number of subjects per study ranged from 10 to 30, and their ages ranged from 20 to 70 years. Of the included 20 RCTs, the dietary supplement was leucine in four studies (20, 32, 35, 36), essential amino acids/carbohydrates in the five (41, 43–46), protein in seven (21, 23, 33, 34, 38, 40, 47), creatine in two (31, 37), omega-3 fatty acids in one (39), and β-hydroxy-β-methylbutyrate in one (42). Details of the composition, dose and duration of dietary supplementation performed daily in each study were shown in Table 1. Outcome indicators for only two of the twenty RCTs were expressed as mean and SD (39, 45). The outcome indicators of the other eighteen RCTs were expressed as mean and SE (20, 21, 23, 31–38, 40–44, 46, 47). There are a total of seven studies for the outcome indicator of muscle strength gave the mean and SD of differences (20, 21, 35, 36, 40–42), the eight RCTs did not give, and we calculated SD of differences by the Equation (1) (31–34, 37–39, 46). For the outcome indicator of leg lean mass, there are six RCTs gave the mean and SD of differences (20, 36, 41, 42, 44, 46), and three RCTs did not give (39, 43, 47), we calculate the SD of difference according to Equation (1). For the outcome indicator of CSA, two RCTs had mean and SD of differences (40, 45), and five RCTs were calculated by Equation (1) to obtain the SD of difference (31, 32, 34, 37, 39). For the outcome indicator of muscle fiber distribution, the SD of the difference of all four RCTs was calculated (31–34). For the outcome indicator of peak aerobic capacity, three RCTs gave the mean and SD of differences (20, 21, 36), the SD of difference was calculated for the two RCTs (33, 38). For muscle volume, two RCTs gave the mean and SD of differences (39, 45), the SD of difference was calculated for the two RCTs (23, 38). The data for the six RCTs were presented as pictures in the article, and we extracted the mean and SD by using the WebPlot Digitizer tool (33, 34, 38, 40, 42, 45).
3.3. Measurement of outcome indicators
In total, fifteen RCTs measured muscle strength as an outcome indicator. Muscle strength was measured in two ways. Ten RCTs measured knee extensor muscles isometric torque by isokinetic dynamometry (20, 21, 33, 35–40, 42), while the other five RCTs measured knee extension strength by performing one repetition of the maximum strength test on the leg extension machine (31, 32, 34, 41, 46). For the measurement of the lean mass of the legs were all used dual-energy x-ray absorptiometry (20, 36, 39, 41–44, 46, 47). Seven studies reported CSA as an outcome indicator, three of which used CT for detection (31, 32, 34), three studies used MRI for detection (37, 39, 45), and one was detected using Stratec XCT 3000 pQCT with software version 6.20C (40). Outcome indicators of fiber type distribution were taken from the vastus lateralis muscle and then muscle biopsy was performed (31–34). Peak muscle aerobic capacity for the four RCTs was measured by a graded exercise test on a cycle ergometer and metabolic cart (20, 21, 33, 36). Another RCT measure of peak aerobic capacity was the single-leg ramp exercise test (38). The muscle volume was all measured by MRI (23, 38, 39, 45).
3.4. Quality of the studies
The methodological quality of the included studies was shown in Table 1 and Supplementary Table S3. Eight studies (20, 21, 31, 33, 34, 38, 41, 45) were rated as good quality. 7 studies (32, 35–37, 39, 40, 42) were rated as excellent quality, and remaining 5 articles were rated fair quality (23, 43, 44, 46, 47). The results indicated insufficient level of concealed allocation, and the sample sizes included in the study were small. The GRADE evidence for the outcome indicators was rated as low, as detailed in Table 2.
3.5. Risk of bias of the studies
The risk of bias of the 20 included studies was shown in Figure 2. Twelve studies were rated as high risk of bias for selection bias because they only mentioned the randomization but did not specify which method was used for random assignment (20, 31–37, 41, 42, 44, 46). Information on allocation concealment was not available in sixteen studies and was therefore rated as unclear risk of bias (20, 21, 23, 31–37, 41, 43–47). Most of the studies on performance bias and detection bias were described as double-blinded and therefore rated as low risk, while those not described were rated as unclear risk of bias. All studies had follow-up rates above 85%, so attrition bias was rated as low risk of bias. Nine studies' reporting bias were rated as low risk because the study protocols were consistent with the outcome indicators in the studies (20, 21, 23, 31, 32, 41–43, 45). And ten studies were rated as unclear risk of bias when there is no enough information about selective reporting (33–40, 44, 46). Other risks of bias that were not mentioned or could not be judged according to the study and were rated as unclear risks. The results of the sensitivity analysis were shown in Supplementary Figure S3.
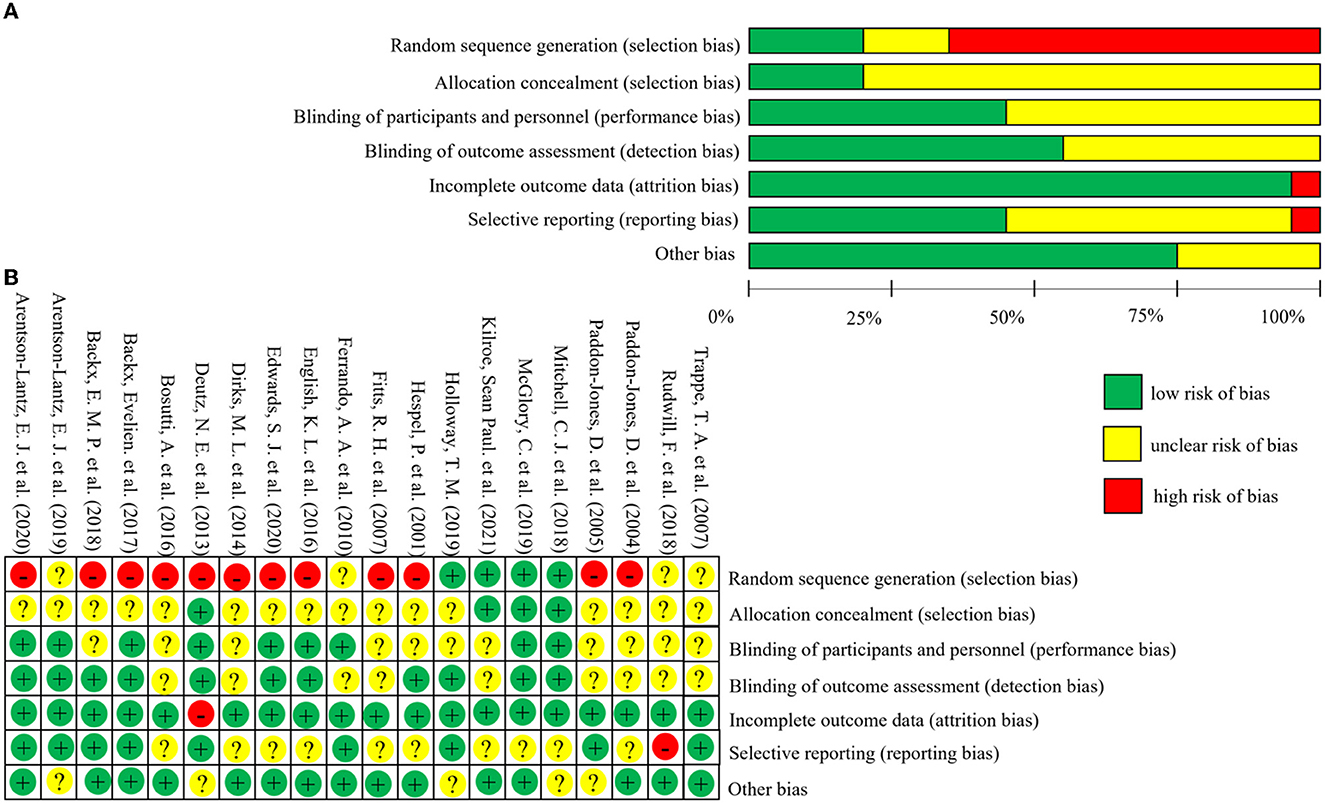
Figure 2. Risk of bias graph and summary of included studies. The figure (A) (risk of bias graph) shows the overall risk of bias in each domain. The figure (B) (risk of bias summary) indicates the risk of bias in each domain for each study.
3.6. Effect of dietary supplements on muscle strength in disuse muscular atrophy
A total of 15 studies measured muscle strength (20, 31–41). Overall meta-analysis showed that dietary supplements had no protective effect on muscle strength during muscle disuse (SMD: 0.19; 95% CI:−0.04, 0.42; p: 0.11). As the types of dietary supplements in both intervention group and control group were heterogenous among the included studies, we performed subgroup analysis to test the effect of different types of dietary supplements on muscle strength. Firstly, we divided the dietary supplements in the intervention group into protein, amino acid, and other nutrients. Other nutrients include β-hydroxy-β-methylbutyric acid, creatine and Omega-3 fatty acids. The results of subgroup analysis (Figure 3) showed that neither protein (SMD: 0.03; 95% CI: −0.36, 0.41; p: 0.89), amino acids (SMD: 0.24; 95% CI: −0.16, 0.63; p: 0.24) nor other nutrients (SMD: 0.34; 95% CI: −0.10, 0.77; p: 0.13) had a protective effect on muscle strength. In addition, we performed a subgroup analysis based on the use of placebo and non-placebo in the control group. Supplementary Figure S1 showed that the use of different supplement types in the control group had no effect on the results.
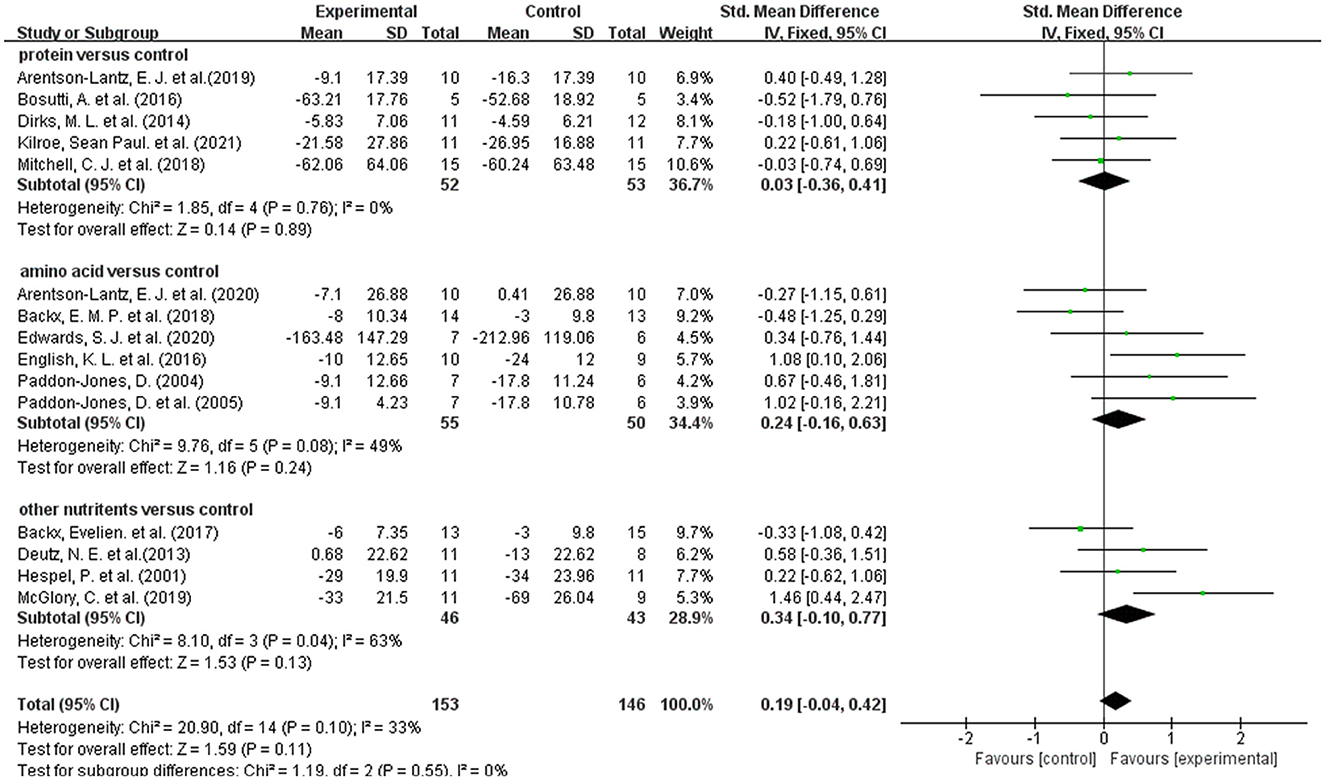
Figure 3. Forest plot of subgroup analysis of SMD difference and 95% confidence intervals for the effect of dietary supplements on muscle strength according to the type of experimental group.
3.7. Effect of dietary supplements on leg lean mass in disuse muscular atrophy
A total of 9 studies reported on leg lean mass as an outcome indicator (20, 36, 39, 41–44, 46, 47). Overall meta-analysis showed that dietary supplements had protective effect on leg lean mass during muscle disuse (WMD: 0.20; 95% CI: 0.09, 0.31; p: = 0.0003). As the types of dietary supplements in both intervention group and control group were heterogenous among the included studies, we performed subgroup analysis to test the effect of different types of dietary supplements on leg lean mass. We divided the dietary supplements in the intervention group into protein, amino acid, and other nutrients. But the protein group had only one article and meta-analysis was not possible (47). The results of subgroup analysis (Figure 4) showed that the amino acid group significantly improved the lean mass of the legs (WMD: 0.20; 95% CI: 0.08, 0.31; p: = 0.0007), but the other group did not show an effect (WMD: 0.32; 95% CI: −0.19, 0.82; p: 0.22). In addition, we performed a subgroup analysis based on the use of placebo and non-placebo in the control group. Supplementary Figure S2 showed that the use of different supplement types in the control group had no effect on the results.
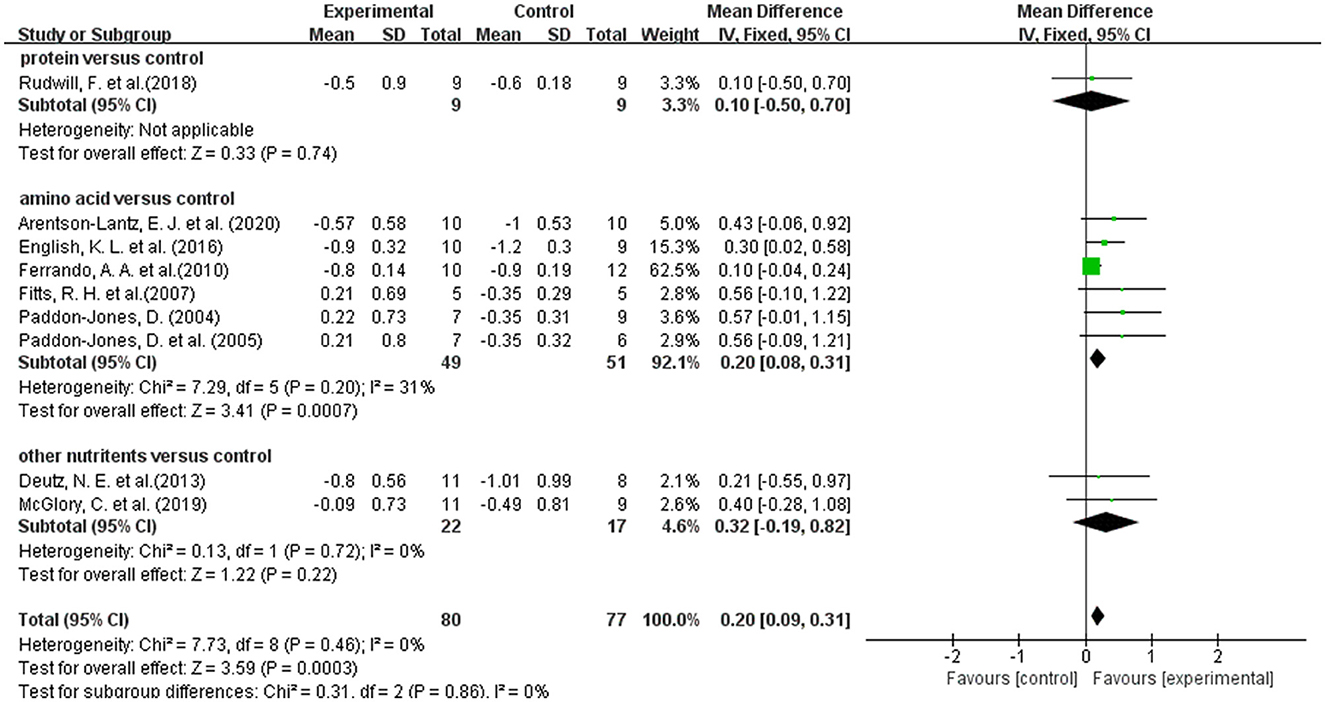
Figure 4. Forest plot of subgroup analysis of WMD difference and 95% confidence intervals for the effect of dietary supplements on leg lean mass according to the type of experimental group.
3.8. Effect of dietary supplements on secondary outcome indicators in disuse muscular atrophy
3.8.1. CSA and muscle fiber type distribution
The results of the meta-analysis of CSA and muscle fiber type distribution are shown in Figure 5. When skeletal muscle atrophy occurs, the CSA becomes smaller. Dietary supplement group did not exhibit greater CSA compared to the control group (SMD: 0.10; 95% CI: −0.20, 0.41; p: 0.55). When skeletal muscle atrophy occurs, type I muscle fibers are converted to type II muscle fibers (48, 49). And then the distribution of type I and type II muscle fibers was altered. As shown in Figure 5, dietary supplementation had no effect on the distribution of type I (WMD: 1.00; 95% CI: −4.58, 6.58; p: 0.73) and type II (WMD: −1.27; 95% CI: −4.58, 6.58; p: 0.49) muscle fibers.
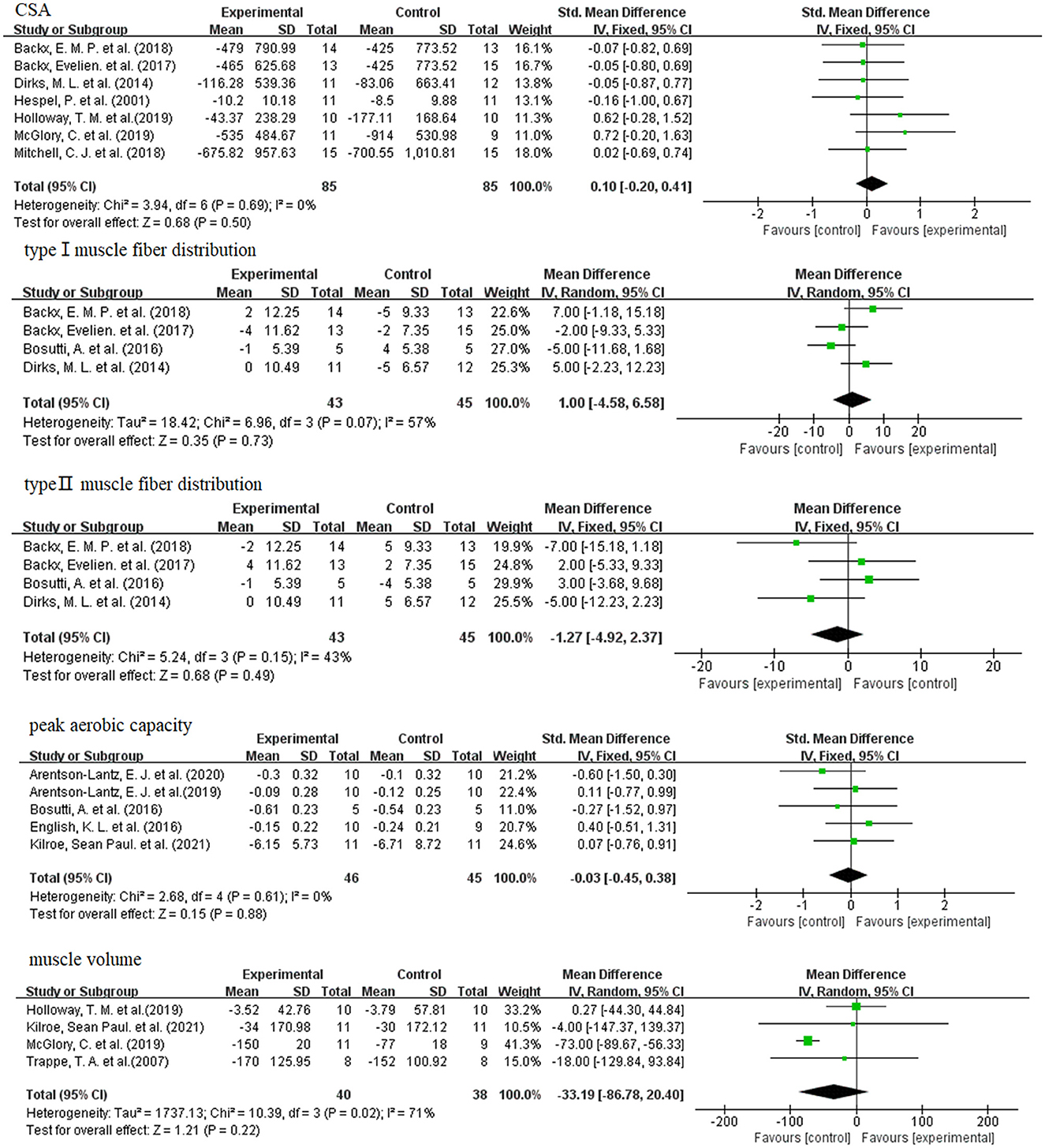
Figure 5. Forest plots of SMD, WMD and 95% confidence intervals for the effect of dietary supplements on CSA, type I muscle fiber distribution (%), and type II muscle fiber distribution (%), peak aerobic capacity, and muscle volume.
3.8.2. Peak aerobic capacity
When skeletal muscle atrophy occurs, the peak aerobic capacity of the muscle becomes poor. However, as shown in Figure 5, meta-analysis showed that dietary supplementation did not improve the peak aerobic capacity of the disuse muscular atrophy process (SMD: −0.03; 95% CI: −0.45, 0.38; p: 0.88).
3.8.3. Muscle volume
As shown in Figure 5, meta-analysis showed that dietary supplements had no effect on muscle volume during muscle disuse (WMD: −33.19; 95% CI: −86.78, 20.40; p: 0.22).
4. Discussion
In this systematic review and meta-analysis, we included 20 RCTs with a total of 339 subjects to analyze whether dietary supplements prevent loss of skeletal muscle mass and strength during muscle disuse. The results of the meta-analysis showed that dietary supplements had no effect on muscle strength, but could improve the lean mass of the legs. For the two primary outcome indicators, muscle strength and lean mass, we performed subgroup analysis based on different types of supplements in the control and intervention groups. The results of all subgroup analyses of muscle strength showed that protein, amino acids and other dietary supplements did not improve muscle strength during muscle disuse. Subgroup analyses of leg lean mass showed that the amino acid group significantly improved lean leg mass during muscle disuse, but the other group did not show an effect. However, their total result was that dietary supplements could improve leg lean mass during muscle disuse. This may be related to the relatively small number of studies in the other group. Our results did not support dietary supplementation had effect on secondary outcome indicators including CSA, muscle fiber type distribution, peak aerobic capacity and muscle volume.
Transient muscle disuse after injury or during recovery from disease results in a loss of muscle mass and function, including a progressive decrease in muscle strength, lean muscle mass, muscle volume, aerobic capacity, CSA, atrophy of type I muscle fibers and a shift from type I to type II muscle fibers (50). It is accompanied by many other negative health consequences, such as reduced insulin sensitivity (7, 8), decreased basal metabolic rate (9), and increased body fat mass (10). Therefore, it is important to find a safe and effective measure to alleviate skeletal muscle atrophy during muscle disuse. Our findings suggest that dietary supplementation can play a role in maintaining lean body mass during muscle disuse. Dietary supplements may be considered to maintain lean muscle mass when patients are unable to exercise during muscle disuse. Overall, the risk of bias in our included RCTs was low, and the heterogeneity of the outcome indicators was small. Therefore, our conclusions may provide some guidance for clinical practice.
To our knowledge, this is the first systematic review and meta-analysis of the role of nutritional interventions on disuse muscular atrophy. Our results are consistent with previously published systematic reviews of nutritional interventions for elderly or sarcopenic patients, where nutritional supplementation alone had no effect on muscle strength (51–53). This may be related to the fact that muscle strength is determined by many factors, such as neuromuscular control and muscle mass. However, studies have found that nutritional interventions combined with exercise training can improve muscle strength and physical function (53–56). Therefore, we recommend that nutritional supplementation combined with exercise training interventions should be performed to improve muscle strength after the release of exercise contraindications. For lean mass, the results of our meta-analysis are inconsistent with previously published systematic reviews of nutrition interventions in older adults (52, 57), which may be related to the different populations we included. Our systematic review included healthy subjects, whereas their systematic review included older adults, whose body composition would have changed.
However, this study has some limitations. Firstly, the sample sizes of the included studies in the systematic review were relatively small. Secondly, the number of studies on outcome indicators such as CSA, muscle fiber type distribution, peak aerobic capacity and muscle volume was relatively small. Third, the number of studies for subgroup analysis was also small. Fourth, some of the RCTs we included do not directly give the SD of the difference, which we calculated by the formula and may differ from the actual value. In addition, some of the outcome indicators are presented in the form of pictures, and the results we obtained by using the WebPlot Digitizer tool to extract the mean and SD may also be different from the actual values. Fifth, the types of dietary supplements in both intervention group and control group were heterogenous among the included studies. Therefore, more RCTs with larger sample sizes are needed in the future to validate the effects of dietary supplements on these outcome indicators.
5. Conclusion
Dietary supplements can improve the lean mass of the legs during muscle disuse. However, our results do not support the role for dietary supplements on muscle strength, CSA, muscle fiber type distribution, peak aerobic capacity or muscle volume.
Data availability statement
The original contributions presented in the study are included in the article/Supplementary material, further inquiries can be directed to the corresponding authors.
Author contributions
Conception and design and drafted the manuscript: HY, J-MY, and Y-BZ. Collection and analysis of the data: YLu, YLo, and J-HZ. Final approval of the article: FG and M-YW. All authors contributed to the article and approved the submitted version.
Funding
This study was supported by the National Science Foundation of Jiangxi Province, China (grant number 20212BAB206004) and the Postgraduate Innovation Special Fund Project of Jiangxi Province, China (grant number YC2022-S966).
Conflict of interest
The authors declare that the research was conducted in the absence of any commercial or financial relationships that could be construed as a potential conflict of interest.
Publisher's note
All claims expressed in this article are solely those of the authors and do not necessarily represent those of their affiliated organizations, or those of the publisher, the editors and the reviewers. Any product that may be evaluated in this article, or claim that may be made by its manufacturer, is not guaranteed or endorsed by the publisher.
Supplementary material
The Supplementary Material for this article can be found online at: https://www.frontiersin.org/articles/10.3389/fnut.2023.1093988/full#supplementary-material
Supplementary Figure S1. Forest plot of subgroup analysis of SMD difference and 95% confidence intervals for the effect of dietary supplements on muscle strength according to the type of control group.
Supplementary Figure S2. Forest plot of subgroup analysis of SMD difference and 95% confidence intervals for the effect of dietary supplements on leg lean mass according to the type of control group.
Supplementary Figure S3. Results of sensitivity analysis on muscle strength, leg lean mass, CSA, type I muscle fiber distribution (%), and type II muscle fiber distribution (%), peak aerobic capacity, and muscle volume.
References
1. Suetta C, Hvid LG, Justesen L, Christensen U, Neergaard K, Simonsen L, et al. Effects of aging on human skeletal muscle after immobilization and retraining. J Appl Physiol. (1985) 107:1172–80. doi: 10.1152/japplphysiol.00290.2009
2. Wall BT, Dirks ML, Snijders T, Senden JM, Dolmans J, Van Loon LJ. Substantial skeletal muscle loss occurs during only 5 days of disuse. Acta physiologica. (2014) 210:600–11. doi: 10.1111/apha.12190
3. Stevens JE, Walter GA, Okereke E, Scarborough MT, Esterhai JL, George SZ, et al. Muscle adaptations with immobilization and rehabilitation after ankle fracture. Med Sci Sports Exerc. (2004) 36:1695–701. doi: 10.1249/01.MSS.0000142407.25188.05
4. Vandenborne K, Elliott MA, Walter GA, Abdus S, Okereke E, Shaffer M, et al. Longitudinal study of skeletal muscle adaptations during immobilization and rehabilitation. Muscle Nerve. (1998) 21: 1006–12. doi: 10.1002/(SICI)1097-4598(199808)21:8<1006::AID-MUS4>3.0.CO;2-C
5. Yu A, Xie Y, Zhong M, Wang F, Huang H, Nie L, et al. Comparison of the initiation time of enteral nutrition for critically Ill patients: at admission vs. 24 to 48 hours after admission. Emerg Med Int. (2021) 2021: 3047732. doi: 10.1155/2021/3047732
6. Huang S, Wang S, Xie Y, He X, Yi X, Zhang J, et al. Application of NRS2002 in preoperative nutritional screening for patients with liver cancer. J Oncol. (2021) 2021: 8943353. doi: 10.1155/2021/8943353
7. Stuart CA, Shangraw RE, Prince MJ, Peters EJ, Wolfe RR, et al. Bed-rest-induced insulin resistance occurs primarily in muscle. Metabolism. (1988) 37: 802–6. doi: 10.1016/0026-0495(88)90018-2
8. Günther O, Frenzel P. Effect of prolonged physical inactivity on carbohydrate tolerance. Z Gesamte Inn Med. (1969) 24:814–7.
9. Haruna Y, Suzuki Y, Kawakubo K, Yanagibori R, Gunji A. Decremental reset in basal metabolism during 20-days bed rest. Acta Physiol Scand Suppl. (1994) 616:43–9.
10. Ferrando AA, Lane HW, Stuart CA, Davis-Street JA, Wolfe RR. Prolonged bed rest decreases skeletal muscle and whole body protein synthesis. Am J Physiol. (1996) 270:E627–33. doi: 10.1152/ajpendo.1996.270.4.E627
12. Short KR, Vittone JL, Bigelow ML, Proctor DN, Coenen-Schimke JM, Rys P, et al. Changes in myosin heavy chain mRNA and protein expression in human skeletal muscle with age and endurance exercise training. J Appl Physiol. (1985) 99: 95–102. doi: 10.1152/japplphysiol.00129.2005
13. Yarasheski KE, Pak-Loduca J, Hasten DL, Obert KA, Brown MB, Sinacore DR. Resistance exercise training increases mixed muscle protein synthesis rate in frail women and men >/=76 yr old. Am J Physiol. (1999) 277:E118–25. doi: 10.1152/ajpendo.1999.277.1.E118
14. Ferrando AA, Tipton KD, Bamman MM, Wolfe RR. Resistance exercise maintains skeletal muscle protein synthesis during bed rest. J Appl Physiol. (1985) 82:807–10. doi: 10.1152/jappl.1997.82.3.807
15. Kortebein P, Ferrando A, Lombeida J, Wolfe R, Evans WJ. Effect of 10 days of bed rest on skeletal muscle in healthy older adults. JAMA. (2007) 297:1772–4. doi: 10.1001/jama.297.16.1772-b
16. Glover EI, Phillips SM, Oates BR, Tang JE, Tarnopolsky MA, Selby A, et al. Immobilization induces anabolic resistance in human myofibrillar protein synthesis with low and high dose amino acid infusion. J Physiol. (2008) 586:6049–61. doi: 10.1113/jphysiol.2008.160333
17. De Boer MD, Selby A, Atherton P, Smith K, Seynnes OR, Maganaris CN, et al. The temporal responses of protein synthesis, gene expression and cell signalling in human quadriceps muscle and patellar tendon to disuse. J Physiol. (2007) 585:241–51. doi: 10.1113/jphysiol.2007.142828
18. Cholewa JM, Dardevet D, Lima-Soares F, de Araújo Pessôa K, Oliveira PH, dos Santos Pinho JR, et al. Dietary proteins and amino acids in the control of the muscle mass during immobilization and aging: role of the MPS response. Amino Acids. (2017) 49:811–20. doi: 10.1007/s00726-017-2390-9
19. Haun CT, Vann CG, Roberts BM, Vigotsky AD, Schoenfeld BJ, Roberts MD, et al. Critical Evaluation of the Biological Construct Skeletal Muscle Hypertrophy: Size Matters but So Does the Measurement. Front Physiol. (2019) 10:247. doi: 10.3389/fphys.2019.00247
20. Arentson-Lantz EJ, Fiebig KN, Anderson-Catania KJ, Deer RR, Wacher A, Fry CS, et al. Countering disuse atrophy in older adults with low-volume leucine supplementation. J. Appl. Physiol. (2020) 128: 967–977. doi: 10.1152/japplphysiol.00847.2019
21. Arentson-Lantz EJ, Galvan E, Ellison J, Wacher A, Paddon-Jones D, et al. Improving dietary protein quality reduces the negative effects of physical inactivity on body composition and muscle function. J Gerontol A Biol Sci Med Sci. (2019) 74: 1605–1611. doi: 10.1093/gerona/glz003
22. Blottner D, Bosutti A, Degens H, Schiffl G, Gutsmann M, Buehlmeier J, et al. Whey protein plus bicarbonate supplement has little effects on structural atrophy and proteolysis marker immunopatterns in skeletal muscle disuse during 21 days of bed rest. Musculoskeletal Neuronal Int. (2014) 14:432–44.
23. Trappe TA, Burd NA, Louis ES, Lee GA, Trappe SW. Influence of concurrent exercise or nutrition countermeasures on thigh and calf muscle size and function during 60 days of bed rest in women. Acta Physiol. (2007) 191:147–59. doi: 10.1111/j.1748-1716.2007.01728.x
24. Liberati A, Altman DG, Tetzlaff J, Mulrow C, Gøtzsche PC, Ioannidis JP, et al. The PRISMA statement for reporting systematic reviews and meta-analyses of studies that evaluate health care interventions: explanation and elaboration. PLoS Med. (2009) 6:e1000100. doi: 10.1371/journal.pmed.1000100
25. Schardt C, Adams MB, Owens T, Keitz S, Fontelo P. Utilization of the PICO framework to improve searching PubMed for clinical questions. BMC Med Inform Decis Mak. (2007) 7:16. doi: 10.1186/1472-6947-7-16
26. Cashin AG, McAuley JH. Clinimetrics: Physiotherapy Evidence Database (PEDro) Scale. J Physiother. (2020) 66:59. doi: 10.1016/j.jphys.2019.08.005
27. Atkins D. Grading quality of evidence and strength of recommendations. BMJ. (2004) 328:1490. doi: 10.1136/bmj.328.7454.1490
28. Higgins JP, Altman DG, Gøtzsche PC, Jüni P, Moher D, Oxman AD, et al. The Cochrane Collaboration's tool for assessing risk of bias in randomised trials. BMJ. (2011) 343: d5928. doi: 10.1136/bmj.d5928
29. Liu Q, Qin C, Liu M, Liu J. Effectiveness and safety of SARS-CoV-2 vaccine in real-world studies: a systematic review and meta-analysis. Infect Dis Poverty. (2021) 10:132. doi: 10.1186/s40249-021-00915-3
30. Farsad-Naeimi A, Asjodi F, Omidian M, Askari M, Nouri M, Pizarro AB. Sugar consumption, sugar sweetened beverages and attention deficit hyperactivity disorder: a systematic review and meta-analysis. Complement Ther Med. (2020) 53:102512. doi: 10.1016/j.ctim.2020.102512
31. Backx EM, Hangelbroek R, Snijders T, Verscheijden ML, Verdijk LB, de Groot LC. Creatine loading does not preserve muscle mass or strength during leg immobilization in healthy, young males: a randomized controlled trial. Sports Med. (2017) 47:1661–71. doi: 10.1007/s40279-016-0670-2
32. Backx EM, Horstman AM, Marzuca-Nassr GN, Van Kranenburg J, Smeets JS, Fuchs CJ. Leucine supplementation does not attenuate skeletal muscle loss during leg immobilization in healthy, young men. Nutrients. (2018) 10:635. doi: 10.3390/nu10050635
33. Bosutti A, Salanova M, Blottner D, Buehlmeier J, Mulder E, Rittweger J. Whey protein with potassium bicarbonate supplement attenuates the reduction in muscle oxidative capacity during 19 days of bed rest. J Appl Physiol. (2016) 121:838–48. doi: 10.1152/japplphysiol.00936.2015
34. Dirks ML, Wall BT, Nilwik R, Weerts DH, Verdijk LB, van Loon LJ, et al. Skeletal muscle disuse atrophy is not attenuated by dietary protein supplementation in healthy older men. J Nutr. (2014) 144:1196–203. doi: 10.3945/jn.114.194217
35. Edwards SJ, Smeuninx B, Mckendry J, Nishimura Y, Luo D, Marshall RN. High-dose leucine supplementation does not prevent muscle atrophy or strength loss over 7 days of immobilization in healthy young males. Am J Cli Nutr. (2020) 112:1368–81. doi: 10.1093/ajcn/nqaa229
36. English KL, Mettler JA, Ellison JB, Mamerow MM, Arentson-Lantz E, Pattarini JM, et al. Leucine partially protects muscle mass and function during bed rest in middle-aged adults. Am J Clin Nutrition. (2016) 103:465–73. doi: 10.3945/ajcn.115.112359
37. Hespel P, Op't Eijnde B, Leemputte MV, Ursø B, Greenhaff PL, Labarque V. Oral creatine supplementation facilitates the rehabilitation of disuse atrophy and alters the expression of muscle myogenic factors in human. J Physiol. (2001) 536: 625–33. doi: 10.1111/j.1469-7793.2001.0625c.xd
38. Kilroe SP, Fulford J, Jackman S, Holwerda A, Gijsen A, van Loon L. Dietary protein intake does not modulate daily myofibrillar protein synthesis rates or loss of muscle mass and function during short-term immobilization in young men: a randomized controlled trial. Am J Clin Nutr. (2021) 113:548–61. doi: 10.1093/ajcn/nqaa136
39. McGlory C, Gorissen SH, Kamal M, Bahniwal R, Hector AJ, Baker SK, et al. Omega-3 fatty acid supplementation attenuates skeletal muscle disuse atrophy during two weeks of unilateral leg immobilization in healthy young women. FASEB J. (2019) 33:4586–97. doi: 10.1096/fj.201801857RRR
40. Mitchell CJ, D'Souza RF, Mitchell SM, Figueiredo VC, Miller BF, Hamilton KL, et al. Impact of dairy protein during limb immobilization and recovery on muscle size and protein synthesis; a randomized controlled trial. J Appl Physiol. (2018) 124:717–28. doi: 10.1152/japplphysiol.00803.2017
41. Paddon-Jones D, Sheffield-Moore M, Urban RJ, Aarsland A, Wolfe RR, Ferrando AA. The catabolic effects of prolonged inactivity and acute hypercortisolemia are offset by dietary supplementation. J Clin Endocrinol Metab. (2005) 90:1453–9. doi: 10.1210/jc.2004-1702
42. Deutz NE, Pereira SL, Hays NP, Oliver JS, Edens NK, Evans CM. Effect of β-hydroxy-β-methylbutyrate (HMB) on lean body mass during 10 days of bed rest in older adults. Clin Nutr. (2013) 32:704–12. doi: 10.1016/j.clnu.2013.02.011
43. Ferrando AA, Paddon-Jones D, Hays NP, Kortebein P, Ronsen O, Williams RH, et al. EAA supplementation to increase nitrogen intake improves muscle function during bed rest in the elderly. Clin Nutr. (2010) 29:18–23. doi: 10.1016/j.clnu.2009.03.009
44. Fitts RH, Romatowski JG, Peters JR, Paddon-Jones D, Wolfe RR, Ferrando AA. The deleterious effects of bed rest on human skeletal muscle fibers are exacerbated by hypercortisolemia and ameliorated by dietary supplementation. Am J Physiol Cell Physiol. (2007) 293:C313–20. doi: 10.1152/ajpcell.00573.2006
45. Holloway TM. A novel amino acid composition ameliorates short-term muscle disuse atrophy in healthy young men. Front Nutr. (2019) 6:105. doi: 10.3389/fnut.2019.00105
46. Paddon-Jones D, Sheffield-Moore M, Urban RJ, Sanford AP, Aarsland A, Wolfe RR, et al. Essential amino acid and carbohydrate supplementation ameliorates muscle protein loss in humans during 28 days bedrest. J Clin Endocrinol Metab. (2004) 89: 4351–8. doi: 10.1210/jc.2003-032159
47. Rudwill F, O'gorman D, Lefai E, Chery I, Zahariev A, Normand S, et al. Metabolic inflexibility is an early marker of bed-rest-induced glucose intolerance even when fat mass is stable. J Clin Endocrinol Metab. (2018) 103: 1910–20. doi: 10.1210/jc.2017-02267
48. Lu JJ, Wang Q, Xie LH, Zhang Q, Sun SH, et al. Tumor necrosis factor-like weak inducer of apoptosis regulates quadriceps muscle atrophy and fiber-type alteration in a rat model of chronic obstructive pulmonary disease. Tob Induc Dis. (2017) 15:43. doi: 10.1186/s12971-017-0148-5
49. De Paepe B, Brusselle GG, Maes T, Creus KK, D'hose S, D'Haese N, et al. TNF alpha receptor genotype influences smoking-induced muscle-fibre-type shift and atrophy in mice. Acta Neuropathol. (2008) 115: 675–81. doi: 10.1007/s00401-008-0348-4
50. Ciciliot S, Rossi AC, Dyar KA, Blaauw B, Schiaffino S. Muscle type and fiber type specificity in muscle wasting. Int J Biochem Cell Biol. (2013) 45:2191–9. doi: 10.1016/j.biocel.2013.05.016
51. Hsu KJ, Liao CD, Tsai MW, Chen CN. Effects of exercise and nutritional intervention on body composition, metabolic health, and physical performance in adults with sarcopenic obesity: a meta-analysis. Nutrients. (2019) 11:9. doi: 10.3390/nu11092163
52. Moraes MB, Avgerinou C, Fukushima FB, Vidal EI, et al. Nutritional interventions for the management of frailty in older adults: systematic review and meta-analysis of randomized clinical trials. Nutr Rev. (2021) 79:889–913. doi: 10.1093/nutrit/nuaa101
53. Yoshimura Y, Wakabayashi H, Yamada M, Kim H, Harada A, Arai H. Interventions for treating sarcopenia: a systematic review and meta-analysis of randomized controlled studies. J Am Med Dir Assoc. (2017) 18:553. doi: 10.1016/j.jamda.2017.03.019
54. Liao CD, Tsauo JY, Wu YT, Cheng CP, Chen HC, Huang YC, et al. Effects of protein supplementation combined with resistance exercise on body composition and physical function in older adults: a systematic review and meta-analysis. Am J Clin Nutr. (2017) 106:1078–91. doi: 10.3945/ajcn.116.143594
55. Finger D, Goltz FR, Umpierre D, Meyer E, Rosa LH. Effects of protein supplementation in older adults undergoing resistance training: a systematic review and meta-analysis. Sports Med. (2015) 45:245–55. doi: 10.1007/s40279-014-0269-4
56. Liao CD, Lee PH, Hsiao DJ, Huang SW, Tsauo JY, Chen HC. Effects of protein supplementation combined with exercise intervention on frailty indices, body composition, and physical function in frail older adults. Nutrients. (2018) 10:1916. doi: 10.3390/nu10121916
57. van Wijngaarden JP, Wojzischke J, van den Berg C, Cetinyurek-Yavuz A, Diekmann R. Effects of nutritional interventions on nutritional and functional outcomes in geriatric rehabilitation patients: a systematic review and meta-analysis. J Am Med Dir Assoc. (2020) 21:1207–15. doi: 10.1016/j.jamda.2020.04.012
Keywords: disuse muscular atrophy, dietary supplements, muscle strength, leg lean mass, meta-analysis
Citation: Ye H, Yang J-M, Luo Y, Long Y, Zhang J-H, Zhong Y-B, Gao F and Wang M-Y (2023) Do dietary supplements prevent loss of muscle mass and strength during muscle disuse? A systematic review and meta-analysis of randomized controlled trials. Front. Nutr. 10:1093988. doi: 10.3389/fnut.2023.1093988
Received: 09 November 2022; Accepted: 21 April 2023;
Published: 11 May 2023.
Edited by:
Ming Yang, West China Hospital, Sichuan University, ChinaReviewed by:
Sarvin Sanaie, Tabriz University of Medical Sciences, IranSousana Konstantinos Papadopoulou, International Hellenic University, Greece
Copyright © 2023 Ye, Yang, Luo, Long, Zhang, Zhong, Gao and Wang. This is an open-access article distributed under the terms of the Creative Commons Attribution License (CC BY). The use, distribution or reproduction in other forums is permitted, provided the original author(s) and the copyright owner(s) are credited and that the original publication in this journal is cited, in accordance with accepted academic practice. No use, distribution or reproduction is permitted which does not comply with these terms.
*Correspondence: Mao-Yuan Wang, wmy.gmu.kf@gmail.com; Feng Gao, gaofeng5960@126.com
†These authors share first authorship
‡ORCID: Hua Ye orcid.org/0000-0002-3347-6895