- Key Laboratory of Tropical Crop Products Processing of Ministry of Agriculture and Rural Affairs, Agricultural Products Processing Research Institute, Chinese Academy of Tropical Agricultural Sciences, Zhanjiang, Guangdong, China
Introduction: Gamma-aminobutyric acid (GABA), one of the main active components in Moringa oleifera leaves, can be widely used to treat multiple diseases including inflammation.
Methods: In this study, the anti-inflammatory activity and the underlying anti-inflammatory mechanism of the GABA-enriched Moringa oleifera leaves fermentation broth (MLFB) were investigated on lipopolysaccharide (LPS)-induced RAW 264.7 cells model. The key active components changes like total flavonoids, total polyphenols and organic acid in the fermentation broth after fermentation was also analyzed.
Results: ELISA, RT-qPCR and Western blot results indicated that MLFB could dose-dependently inhibit the secretions and intracellular expression levels of pro-inflammatory cytokines like 1β (IL-1β), interleukin-6 (IL-6), interleukin-8 (IL-8) and tumor necrosis factor-α (TNF-α). Furthermore, MLFB also suppressed the expressions of prostaglandin E2 (PGE2) and inducible nitric oxide synthase (iNOS). Moreover, the mRNA expressions of the key molecules like Toll-like receptor 4 (TLR-4) and nuclear factor (NF)-κB in the NF-κB signaling pathway were also restrained by MLFB in a dose-dependent manner. Besides, the key active components analysis result showed that the GABA, total polyphenols, and most organic acids like pyruvic acid, lactic acid as well as acetic acid were increased obviously after fermentation. The total flavonoids content in MLFB was still remained to be 32 mg/L though a downtrend was presented after fermentation.
Discussion: Our results indicated that the MLFB could effectively alleviate LPS-induced inflammatory response by inhibiting the secretions of pro-inflammatory cytokines and its underlying mechanism might be associated with the inhibition of TLR-4/NF-κB inflammatory signaling pathway activation. The anti-inflammatory activity of MLFB might related to the relative high contents of GABA as well as other active constituents such as flavonoids, phenolics and organic acids in MLFB. Our study provides the theoretical basis for applying GABA-enriched Moringa oleifera leaves as a functional food ingredient in the precaution and treatment of chronic inflammatory diseases.
1. Introduction
Inflammation is a natural immunological response that the immune system reacted to outside stimulus like pathogen (1). However, the inordinate and uncontrolled inflammation will also lead to apoptosis of immune cells and immunologic derangement which resulting in the chronic degenerative diseases and cancer (2, 3). Although various steroids and non-steroidal anti-inflammatory drugs (NSAIDs) like azathioprine and aspirins have been used to treat these acute and chronic inflammatory diseases, long-term use of these drugs may also lead to various adverse effects to human health (1). Therefore, the development of natural anti-inflammatory drugs with greater efficacy and minimal toxicity has attracted more and more attention. Besides, with the improvement of consumption level, natural anti-inflammatory functional foods are more and more popular in humans (4).
Gamma-aminobutyric acid (GABA) is a non-protein amino acid that can be found in plants, bacteria and animals (5). It's an important inhibitory neurotransmitter that presented in mammal central nervous system with multiple physiological functions including anti-inflammation (6). For example, GABA showed obvious anti-inflammatory effect on streptozotocin-treated mice by decreasing the synthesis of inflammatory mediators like IL-1β, TNF-α, IFN-γ, IL-12, and increasing the production of anti-inflammatory mediator TGF-β1 (7). Besides, GABA restrained the expression of inflammatory cytokines (L-6, IL8, and TNF-α) and alleviated inflammatory response of MAC-T cells induced by LPS via the TLR4-MyD88-NFκB signaling pathway (8). Except for anti-inflammation, GABA has also been reported to possess hypotensive, antidiabetic, immunity enhancement, and sedative effects, and has been applied to alleviate sleeplessness, depression and improve visual cortical function (6, 9). Despite its various physiological functions, GABA content in natural animal- and plant-based food products is pretty low which cannot meet people's needs (6). Therefore, GABA enrichment has attracted increasing attention and a variety of related functional foods have been developed like GABA-enriched tea, soft sweets, beverages, dairy products and so on. At present, microbial fermentation is the most common method to enrich GABA because of its ability to produce lactic acid, flavonoids, polyphenols and other active substances beneficial to human health (5, 10). Up to now, microbial fermentation has been widely applied in the GABA enrichment in strawberry juice (6), green tea (11), water dropwort (10), etc.
Moringa oleifera, also known as “drumstick tree” or simply Moringa in English, is a perennial deciduous tropical plant with a variety of bioactive compounds (12). Because of its nutritional and medicinal value, Moringa oleifera has been regarded as one of the most economically valuable plants and is widely used in food, industry, agriculture and medicine in the developing countries (13). Moringa oleifera leaves contain multiple active constituents like protein, amino acids, polysaccharides, dietary fiber, phenols, flavonoids, phytosterols, glycosides (2), and are reported to possess multiple pharmacological activities like antioxidant, anti-inflammatory, anti-hypertensive, hypoglycemic, hypolipidemic, liver and kidney protective, as well as anti-cancer effects (14–16). Previous studies have found that GABA as well as other active constituents like flavonoids, polyphenols, most amino acids, oligosaccharides, organic acids, nucleosides of Moringa oleifera leaves are significantly enhanced after fermentation (12, 17), which suggested that Moringa oleifera leaves were ideal candidate for GABA enrichment. Therefore, GABA enrichment in Moringa oleifera leaves may provide new idea for its application in functional foods. However, to our knowledge, there are still no studies on the enrichment of GABA using Moringa oleifera leaves as raw material. As a consequence, we tried Lactobacillus fermentation to enrich GABA in Moringa oleifera leaves and the result showed that the GABA content of the fermented Moringa oleifera liquid increased to 209 mg/L under the optimal fermentation conditions, which was 1.45 times higher than that of the unfermented (18). However, the other key active components changes in the fermentation broth after fermentation has not been further studied. Besides, the anti-inflammatory activity of the fermentation broth and the underlying anti-inflammatory mechanism is still unclear, which greatly limited its application in functional foods.
Therefore, in order to exploit the application value of GABA-enriched Moringa oleifera leaves fermentation broth (MLFB) in functional foods, the key active components changes like total flavonoids, total polyphenols and organic acid in the fermentation broth after fermentation was analyzed, and the anti-inflammatory activity as well as the underlying anti-inflammatory mechanism of the fermentation broth were investigated on LPS-induced RAW 264.7 cells model. This study may provide theoretical basis for the application of MLFB on the anti-inflammatory functional foods.
2. Materials and methods
2.1. Materials
Moringa oleifera leaves powder was provided by Henan Jinlamu Bio-technology (Hebi, Henan, China). Lipopolysaccharide (LPS), 3-(4,5-dimethylthiazol-2-yl)-2,5-diphenyltetrazolium bromide (MTT), Dulbecco's modified Eagle medium (DMEM) and dimethyl sulfoxide (DMSO) were from Solarbio Science and Technology (Beijing, China). Lactobacillus plantarum LK-1 was isolated from pickles in our laboratory (19). Fetal bovine serum (FBS) and ethylene diamine tetraacetic acid (EDTA) digestive juice were from Gibco (Carlsbad, CA, USA). IL-6, IL-8, IL-1β, TNF-α detection kits (enzyme-linked immunosorbent assay) were from Nanjing Jiancheng Biotechnology (Nanjing, Jiangsu, China). Nucleic Acid Stain was from Beijing Dingguo Changsheng Biotechnology (Beijing, China). Reverse transcription kit and fluorescent dye kit were from Guangzhou Jisai Biotechnology (Guangzhou, Guangdong, China).
2.2. Preparation of MLFB and its key active components analysis before and after fermentation
GABA-enriched Moringa oleifera leaves fermentation broth was obtained using Lactobacillus plantarum LK-1 as fermentation strain according to the previous study (18). In detail, the Moringa oleifera leaves powder was added to distilled water at 1:25 (w/w), 3% (w/w) of glucose was added and monosodium glutamate was then added at the final concentration of 5 g/L. After autoclaving at 105°C for 20 min, the Moringa oleifera solution was fermented with Lactobacillus plantarum LK-1 at 35°C for 72 h. After that, the fermentation mixture was centrifuged at 6,000 g for 30 min, then the supernatant was collected and named as MLFB for the following anti-inflammatory activity evaluation. The GABA content of MLFB was detected to be 209 mg/L by high performance liquid chromatography (HPLC) with pre-column o-phthaldialdehyde (OPA) derivatization (18). The result of GABA content analysis in MLFB and Moringa oleifera leaves solution (MLS) along with their HPLC spectrograms can be seen in the Appendix. The total flavonoids, total polyphenols and organic acid content in MLFB and MLS were detected according to the methods of Li et al. (17).
2.3. Cell culture
The macrophages RAW 264.7 were provided by the Procell Life Technology (Wuhan, Hubei, China). The cells were cultured in DMEM with 10% FBS at 37°C in an atmosphere of 5% CO2, as the cell provider required.
2.4. Cell viability assay
The cell viability was determined by MTT assay (2). Cells in logarithmic phase was dispersed in DMEM containing 10% FBS. Then the cell suspension was evenly transferred to 96-well plates at 5 × 103 cells/well. After cultivating for 12 h, the culture medium was removed and 100 μL fresh culture medium with different concentrations of MLFB (31.25, 62.5, 125, 250 and 500 μg/mL) was added to each well. 12 h later, 10 μL 5 mg/mL MTT was added to each well, and the supernatant was gently removed after 3 h of culture. Then 150 μL DMSO was added to each well and the mixture was oscillated for 10 min. The absorbance of the mixture was detected at 570 nm using a microplate reader (Bio-Tek, Winooski, VT, USA). The relative activity of RAW 264.7 cells was calculated considering the activity of cells treated without MLFB as 100%.
2.5. Enzyme-linked immunosorbent assay (ELISA)
The concentrations of inflammatory factors (IL-1β, IL-6, IL-8 and TNF-α) in cell supernatants were detected using ELISA kits referring to the manufacturer's descriptions. RAW 264.7 cells suspension (1 × 105 cells/mL) was inoculated into 12-well plate at 1 × 105 cells/well. After cultivating for 12 h, the supernatant in each well was removed and the cells were treated with different concentrations of MLFB (125, 250, 500 μg/mL) for 1 h. After incubating with 1 μg/mL LPS for 24 h, the mixture was collected and centrifuged for 20 min at 3,000 g. The secretion levels of IL-1β, IL-6, IL-8, and TNF-α in supernatants of each group were measured and the standard curves of these inflammatory factors were made referring to the manufacturer's descriptions in ELISA kits.
2.6. Real-time reverse transcription quantitative polymerase chain reaction
After RAW 264.7 cells were treated with LPS and different concentrations of MLFB for 24 h, the mRNA expressions of IL-1β, IL-6, TNF-α, NF-κB, PGE2, TLR-4 in RAW 264.7 cells were determined by RT-qPCR (6). Firstly, TRIzol reagent was used to extract the total RNA of cells. Then RNA was synthesized into cDNA by cDNA reverse transcription kit referring to the manufacturer's descriptions. Finally, the RT-qPCR was proceeded in 20 μL reaction system containing 2 μL cDNA, 1 μL primer pairs (10 μmol/L), 10 μL SYBR Green PCR Master Mix, 0.4 μL 50 × ROX Reference Dye 2 and 6.6 μL ultra-pure distilled water. The PCR conditions were as followings: initial denaturation at 95°C for 5 min, 40 cycles at 95°C for 10 s, 60°C for 34 s, and the melting curve was obtained at 95°C for 15 s, 60°C for 1 min and 95°C for 15 s in the ABI 7500 real-time fluorescent quantitative PCR System (Applied Biosystems, Foster, CA, USA). β-actin was used as internal control and the primers used here are shown in Table 1.
2.7. Western blot
After treated with LPS and different concentrations of MLFB for 24 h, the protein expression levels of IL-1β, IL-6, TNF-α and iNOS in RAW 264.7 cells were determined by Western blot (20). Firstly, the cells were lysed by 400 μL cell lysis buffer containing protease inhibitors. Then the lysate was stirred on ice for 30 min and transferred to a 100°C water path for 10 min. After centrifuging the lysate at 6,000 g for 6 min at 4°C, the supernatant was gathered and the protein content was determined by a BCA protein assay kit (Solarbio Science and Technology Crop., Beijing, China). Then the proteins were analyzed by 10% SDS-PAGE and transferred to a PVDF membrane. After blocking with 5% skimmed milk for 60 min, the PVDF membrane was incubated with the primary antibody at 4°C for a night. Then the PVDF membrane was washed with TBST (50 mmol/L Tris-HCl, 150 mmol/L NaCl and 0.1% v/v Tween-20) for three times before incubating with the secondary antibody for 40 min at room temperature. The bands on the membrane were quantified by an automatic gel imaging analysis system (Peiqing science and technology Crop., Shanghai, China). β-actin was used as the internal control, and the results were displayed referring to the control.
2.8. Statistical analysis
All tests were performed at least in triplicate and the experimental results were presented as mean ± standard deviation (SD). SPSS Version 17.0 (SPSS Inc., Chicago, IL) was used to analyze the significant differences between samples and P < 0.05 was regarded as statistically significant. All the figures were drawn by origin 8.0 (OriginLab Corp., Northampton, USA).
3. Results and discussion
3.1. Cytotoxicity of MLFB on RAW 264.7 cells
The cytotoxic effect of MLFB on RAW 264.7 cells was determined by MTT assay. As shown in Figure 1, after treated with different concentrations of MLFB, the viability of RAW 264.7 cells showed no significant changes (P > 0.05). After treated with 500 μg/ml MLFB for 12 h, the cell viability was measured to be 99.33%. The result indicated that the MLFB with concentration under 500 μg/mL had no obvious toxicity to cells. The result was consistent with the study result of Lin et al. (20) who stated that the brown seaweed Laminaria japonica fermented by Bacillus subtilis also showed no significant cytotoxic effects on RAW 264.7 cells. According to the result of cytotoxic effect, concentrations of 125, 250 and 500 μg/mL of MLFB were selected as the standard test concentrations in the follow-up experiments.
3.2. MLFB inhibits the productions and expressions of pro-inflammatory cytokines in LPS-induced RAW 264.7 cells
IL-1β, IL-6, IL-8, and TNF-α are typical pro-inflammatory cytokines that secreted by activated macrophages, and are important in inflammatory responses (21). Dysregulation of these pro-inflammatory cytokines is related to systemic inflammatory disorder and may lead to various inflammatory diseases like rheumatoid arthritis and inflammatory bowel disease (21). The effects of MLFB on the productions, mRNA and protein expressions of LPS-induced pro-inflammatory cytokines including IL-1β, IL-6, IL-8 and TNF-α were determined by ELISA, RT-qPCR and Western blot, respectively. As shown in Figure 2, the productions of IL-1β, IL-6, IL-8 and TNF-α in LPS-induced inflammatory model group were obviously more than those in normal group, which indicated that the cellular inflammatory model was successfully established. Compared with the inflammatory model group, the productions of pro-inflammatory factors IL-1β, IL-6, IL-8 and TNF-α in cells were dose-dependently decreased after treated with a series concentrations of MLFB. When the concentration of MLFB reached 500 μg/mL, the productions of IL-1β, IL-6, IL-8 and TNF-α in the MLFB treatment groups decreased by 63.22, 35.71, 59.89, and 60.77%, respectively. The mRNA expression levels of pro-inflammatory cytokines IL-1β, IL-6 and TNF-α in RAW 264.7 cells were shown in Figure 3. As expected, the mRNA expressions of IL-1β, IL-6 and TNF-α in LPS-induced inflammatory model group were obviously increased when compared with normal group. Despite the treatment of MLFB at concentrations of 125 μg/mL showed no significantly inhabitation effects on the mRNA expression of these pro-inflammatory cytokines induced by LPS, when the concentrations of MLFB increased to 250 and 500 μg/mL, the mRNA expression of these pro-inflammatory cytokines were obviously inhibited. Similarly, compared with LPS-induced inflammation model group, the protein expression levels of pro-inflammatory factors IL-1β, IL-6, TNF-α were decreased in different degrees after treated with different concentrations of MLFB, despite the decrease of IL-1β protein expression was not significant (Figure 4).
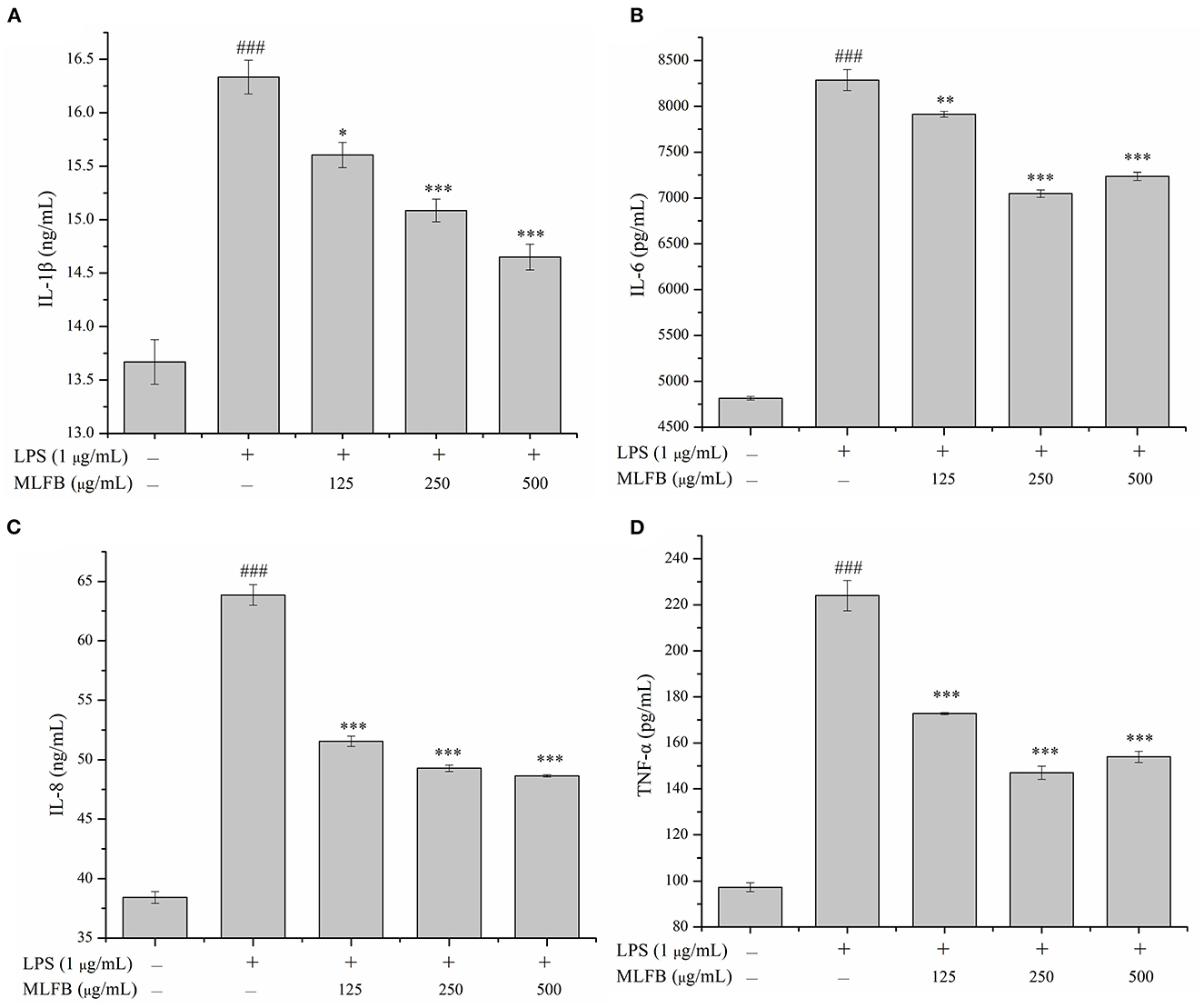
Figure 2. Effects of MLFB on the secretions of IL-1β (A), IL-6 (B), IL-8 (C), and TNF-α (D) in LPS-induced RAW 264.7 cells. #Means statistical difference compared with blank control group (###P < 0.001). *Means statistical difference compared with LPS-induced inflammatory model group (*P < 0.05, **P < 0.01, ***P < 0.001).
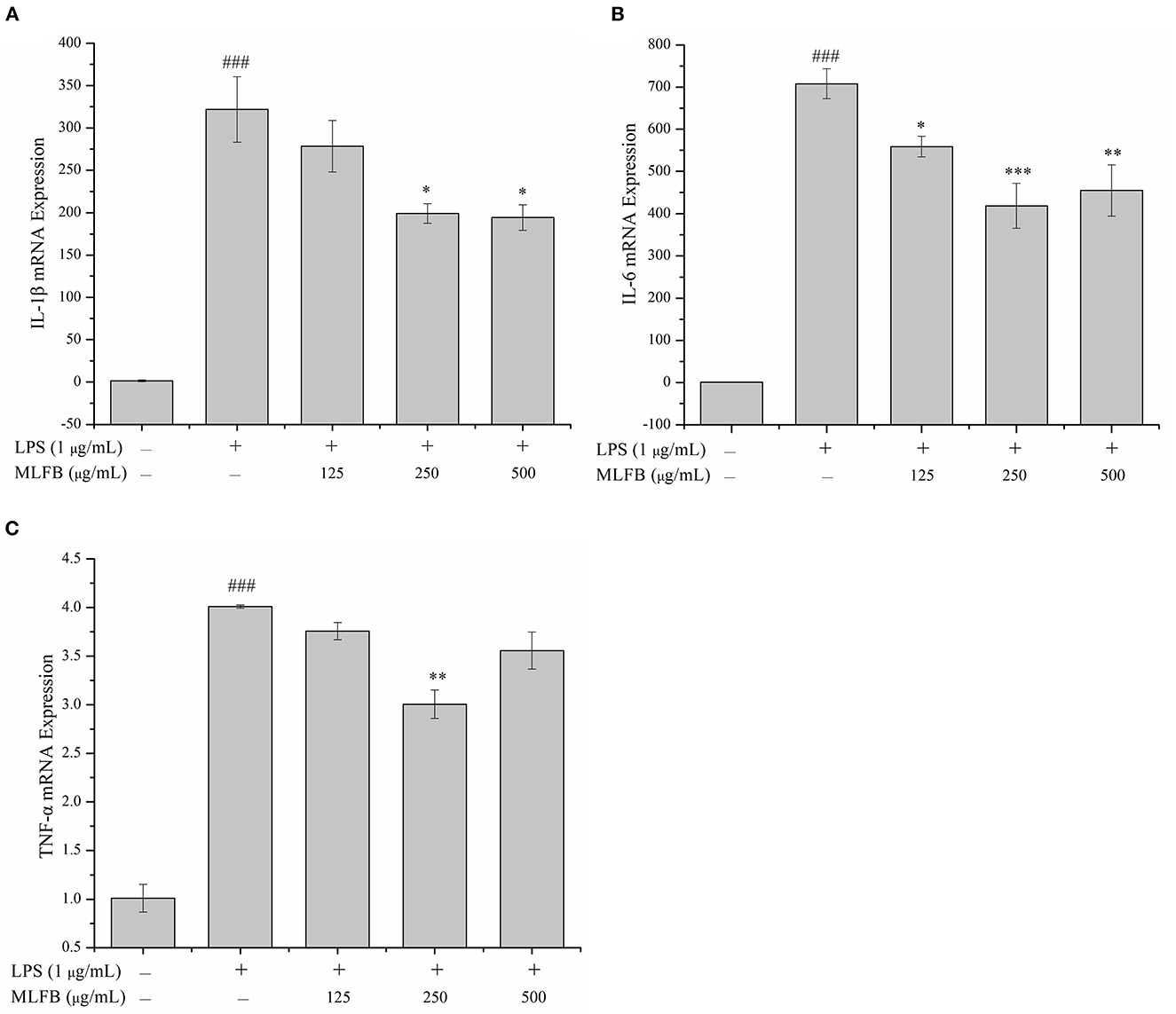
Figure 3. Effects of MLFB on the mRNA expressions of IL-1β (A), IL-6 (B), TNF-α (C) in LPS-induced RAW 264.7 cells. #Means statistical difference compared with blank control group (###P < 0.001). *Means statistical difference compared with LPS-induced inflammatory model group (*P < 0.05, **P < 0.01, ***P < 0.001).
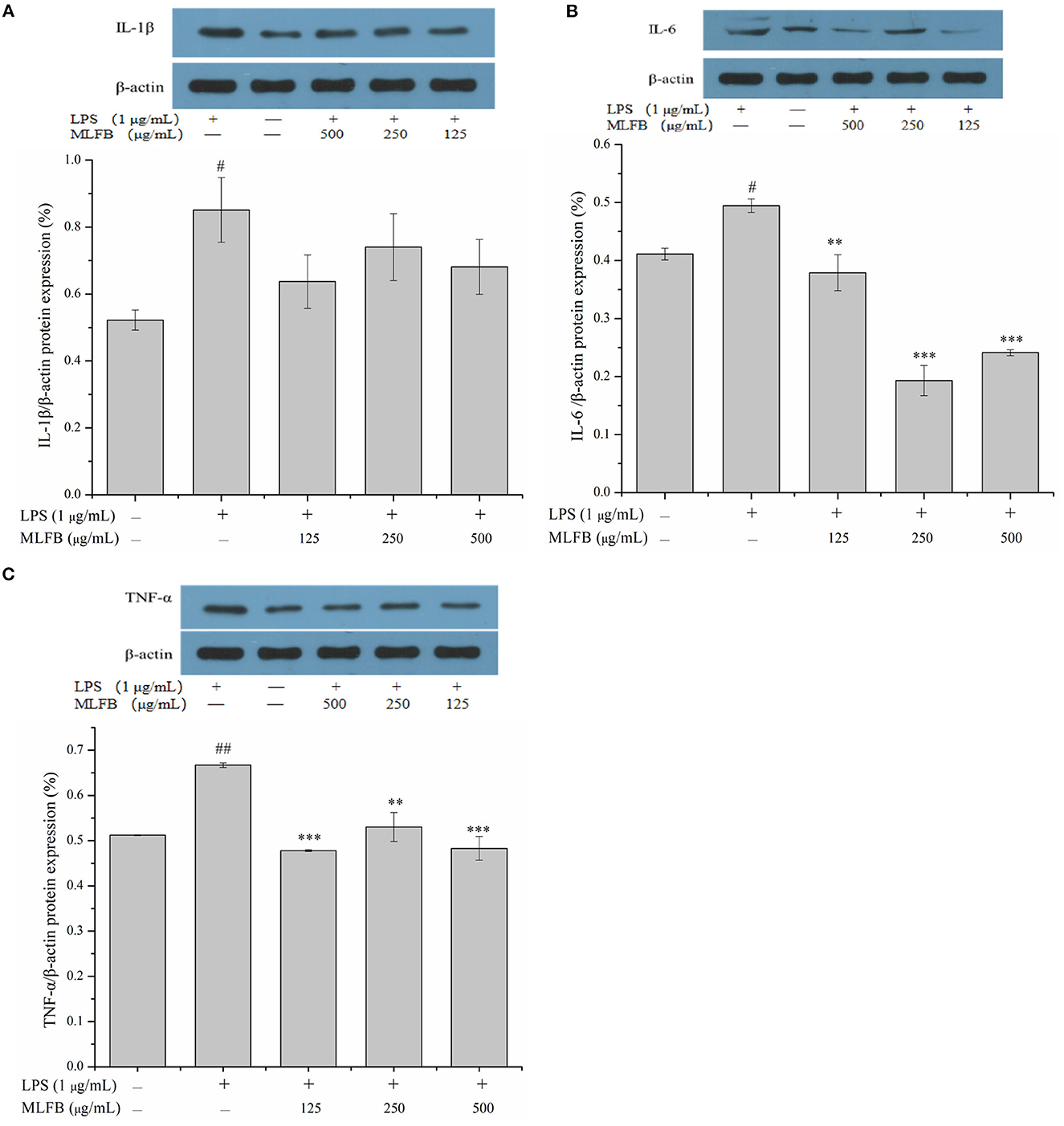
Figure 4. Effects of MLFB on the protein expressions of IL-1β (A), IL-6 (B), TNF-α (C) in LPS-induced RAW 264.7 cells. #Means statistical difference compared with blank control group (#P < 0.05, ##P < 0.01). *Means statistical difference compared with LPS-induced inflammatory model group (**P < 0.01, ***P < 0.001).
The above results indicated that the MLFB could down-regulate the expressions of pro-inflammatory cytokines in macrophages activated by LPS, thus further inhibit the secretions of these pro-inflammatory cytokines and exerts anti-inflammatory effect. Previous studies have showed that GABA could promote proliferation of T-cells, inhibit apoptosis in β-cells, decrease the synthesis of inflammatory mediators like IL-1β, TNF-α, IFN-γ, IL-12, increase the production of anti-inflammatory mediator TGF-β1, and thus played important roles in anti-inflammatory responses (7, 8). Besides, the ability of GABA to restrain the secretions of TNF-α, COX-2 and iNOS, IL-6 and IL-12 by LPS-induced macrophages was also reported (22, 23). Therefore, the anti-inflammatory activity of MLFB may be attributed to its high content of GABA. Our study result is similar to the study result of Chang et al. (24) who reported that GABA-enriched pepino extract obtained after fermentation can inhibit the expression of TNF-α in LPS-induced RAW 264.7 macrophages.
3.3. MLFB inhibits the expressions of PGE2 and iNOS in LPS-induced RAW 264.7 cells
Prostaglandin E2 (PGE2), an important prostanoid metabolite mainly produced by cyclooxygenase-2 (COX-2), is involved in multiple inflammatory processes including activating immune cell and promoting the secretion of pro-inflammatory cytokines (25). A variety of stimulations of inflammation or tissue injury will lead to overproduction of PGE2, thus inhibiting the expression of PGE2 is also an effective measure to treat inflammation (20). The effect of MLFB on the mRNA expression of PGE2 was shown in Figure 5A. The result showed that the mRNA expression of PGE2 in cells were significantly increased after treated with LPS, and 125 μg/mL of MLFB displayed no significant effect on the mRNA expression of PGE2 compared with LPS-treated group. Notably, the mRNA expression of PGE2 in cells treated with 250 μg/mL of MLFB was significantly decreased. The result indicated that MLFB at a certain dose could inhibit the expressions of PGE2 and therefore control the overproduction of PGE2 induced by LPS.
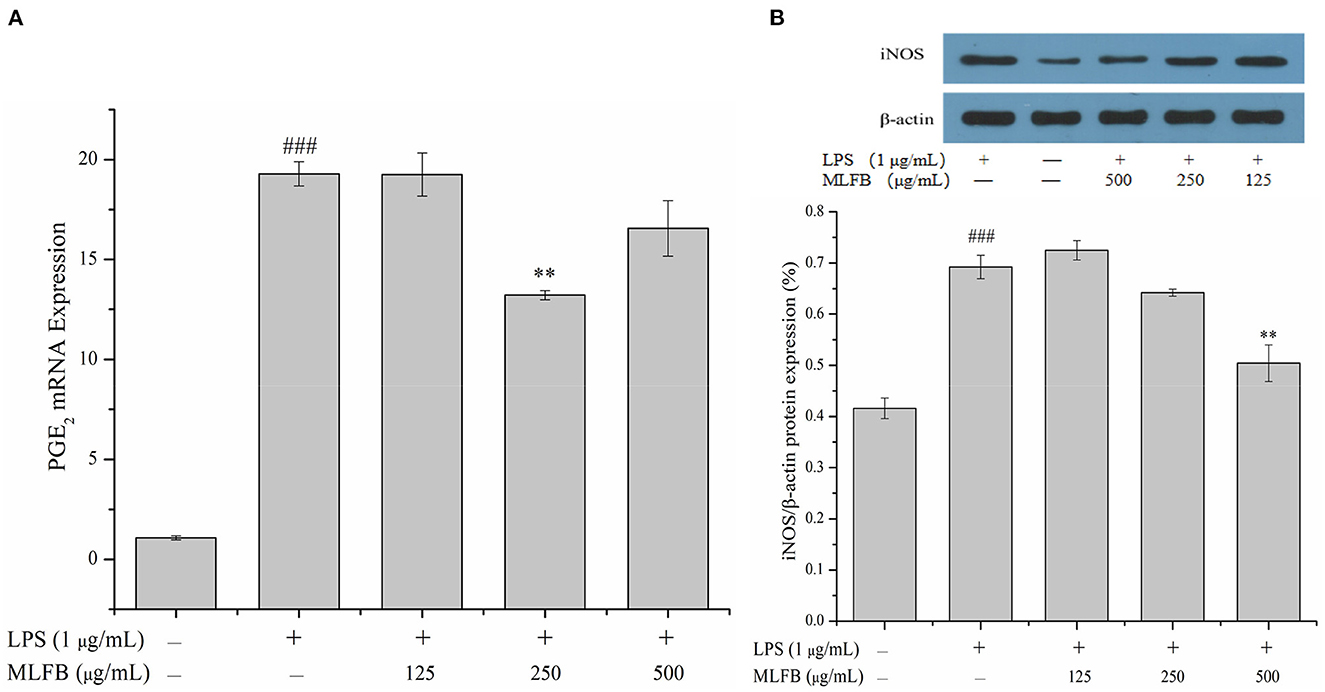
Figure 5. Effects of MLFB on the expressions of PGE2 (A) and iNOS (B) in LPS-induced RAW 264.7 cells. #Means statistical difference compared with blank control group (###P < 0.001). *Means statistical difference compared with LPS-induced inflammatory model group (**P < 0.01).
Inducible NOS (iNOS), an isoform of nitric oxide synthase (NOS), is especially expressed during inflammatory response and involved in the synthesis of pro-inflammatory mediator nitric oxide (NO) (25). The over expression of iNOS will produce excess amount of NO, thus leading to tissue damage, septic shock, and further resulting in other complication during persistent chronic inflammatory response (21). The effect of MLFB on the protein expression of iNOS in RAW 264.7 macrophages was exhibited in Figure 5B. The intracellular protein expression of iNOS in LPS-induced inflammatory model group was obviously higher than that in normal group. Compared with the inflammatory model group, the protein expression of iNOS in cells was dose-dependently decreased after treated with different concentrations of MLFB. The result indicated that MLFB could inhibit the expression of iNOS, which may further suppress the production of NO, thus alleviate LPS-induced inflammatory response.
COX-2 and iNOS are primary inflammatory mediators expressed in macrophages which are involved in the synthesis of PGE2 and NO, and the production of PGE2 and NO are closely related with initiation of the early stage of inflammatory pathways (1, 26). In the present study, pretreatment with MLFB effectively inhibited the mRNA expression of PGE2 and protein expression level of iNOS in LPS-stimulated RAW 264.7 macrophages at different degree, which indicated that MLFB involved in the suppression in the early stage of inflammatory pathways. Previous studies illuminated that ethyl acetate fraction, concentrate and isothiocyanates of Moringa oleifera could effectively down-regulate the expression of COX-2 and iNOS, and concomitantly inhibited the production of NO and PGE2 in LPS-stimulated RAW 264.7 macrophages (1, 13). In addition, GABA is also reported to have the ability of inhibiting immune cells activation by diminishing the production of COX-2 and iNOS (6). Therefore, the GABA as well as other active constituents contained in Moringa oleifera leaves may play roles in the inhibition of PGE2 and iNOS expressions.
3.4. MLFB suppresses inflammation mediated by the TLR-4/NF-κB signaling pathway
Toll-like receptors (TLRs) are important transmembrane signaling receptors mediating signal transductions, and TLR-4 is one of the most studied receptors involved in inflammatory response, immune response and other processes (27, 28). It has been reported that LPS could interact with TLR-4 and following activate several intracellular signaling pathways including NF-κB signaling pathway (29, 30). NF-κB, mainly composed of p50 and p65 subunits, is a transcription factor that plays an important role in regulating innate and adaptive immunity, including inflammation signal transduction of macrophages (31, 32). In the normal state, NF-κB exists in cytosol and is combined with inhibitory protein IκB. When the cells were stimulated by LPS, IκB is phosphorylated and rapidly degraded by proteasomes in the cytoplasm, thus NF-κB is released (28). The free NF-κB transfers into the nucleus and turn on gene expression of pro-inflammatory mediators (33, 34). In a word, TLR-4/NF-κB signaling pathway participates in the regulations of various inflammatory cytokines like IL-1β, IL-6, IL-8, and TNF-α, and plays an important role in both immune and inflammatory response (8, 35). Interdicting the TLR/NF-κB signaling pathway will be an effective method for treatment of chronic inflammatory diseases. Therefore, the key molecules (TLR-4 and NF-κB p65) in the TLR-4/NF-κB signaling pathway were detected to explore the anti-inflammatory mechanism of MLFB on LPS-induced RAW 264.7 cells. As shown in Figure 6, comparing to the normal group, the mRNA expression level of NF-κB p65 and TLR-4 in RAW 264.7 cells were significantly increased after stimulated by LPS, indicating that the TLR-4/NF-κB signaling pathway was activated by LPS. When the cells were pretreated with different concentrations of MLFB, the mRNA expression levels of NF-κB p65 and TLR-4 in cells were obviously decreased in a dose-dependently manner. The result demonstrated that MLFB suppressed the mRNA expression levels of the key molecules in the NF-κB signaling pathway induced by LPS in RAW 264.7 cells. Therefore, MLFB may exert anti-inflammatory activity by inhibiting the TLR-4/NF-κB signaling pathway.
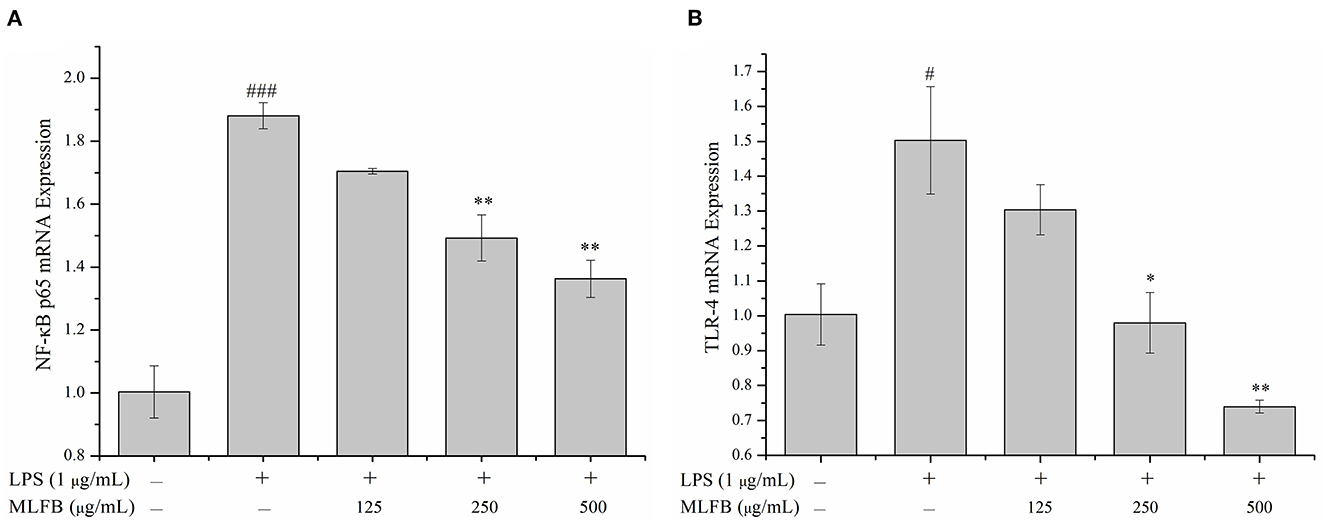
Figure 6. Effects of MLFB on the mRNA expressions of NF-κB p65 (A) and TLR-4 (B) in LPS-induced RAW 264.7 cells. #Means statistical difference compared with blank control group (#P < 0.05, ###P < 0.001). *Means statistical difference compared with LPS-induced inflammatory model group (*P < 0.05, **P < 0.01).
3.5. The key active components analysis of MLFB and its anti-inflammatory mechanism elaboration
The changes of key active components like total flavonoids, total polyphenols and organic acid content in MLFB after fermentation was shown in Table 2. After fermentation, the total flavonoids content decreased from 48.66 mg/L to 31.81 mg/L, while total polyphenols content increased from 21.43 mg/L to 26.02 mg/L, which was increased by 21.42% compared with the unfermented MLS. The content changes of flavonoids and polyphenols during fermentation may be due to their continuous degradation, convertation, and synthesis during the growth and metabolic processes of microorganisms (36). For instance, it is reported that microbial degradation may cause the cleavage and hydroxylation of the aromatic ring in flavonoids and polyphenols (37). Their precipitation or oxidation during the fermentation process may also lead to decrease of these compounds (38). Conversely, some lactobacillus like L. rhamnosus are reported to have the ability of releasing esterases which can hydrolyze ester bonds between phenolic acids and cell wall substances, thus resulting in the release of phenols (39). Previous studies also showed that lactobacillus can produce glycosyl hydrolases which can convert the bound phenolics linked with glycosides into free phenols during fermentation (40). As shown in Table 2, the contents of citric acid and succinic acid in MLFB decreased to < 1 mg/L after fermentation, but the content of pyruvic acid, lactic acid and acetic acid were increased obviously (P < 0.05). The content of lactic acid greatly increased to 303.84 mg/L and became the main organic acid in MLFB. However, the contents of oxalic acid showed no significantly changes after fermentation. The content changes of organic acids in MLFB may be associated with glycolytic pathway and tricarboxylic acid (TCA) cycle during the growth and metabolic processes of lactobacillus. During fermentation process, lactobacillus can catabolize sugars into lactic acid and acetic acid through the glycolytic pathway (38, 40). Besides, citric acid, pyruvic acid and succinic acid are important intermediate metabolites of the TCA cycle and the contents of these organic acid change dynamically with the involved biochemical reactions (40, 41). For example, citric acid is used as the second carbon source by lactobacillus and can be converted into acetic acid and oxaloacetic acid by a citrate lyase, while the oxaloacetic acid can be further translated into pyruvic acid which is eventually reduced to lactic acid by the lactate dehydrogenase (42). In addition, in the oxidative branch pathway of the TCA cycle, oxaloacetate can also be converted into succinic acid through a serious of enzymes like aconitase, isocitrate dehydrogenase, and succinic acid can be further converted to fumarate by succinate dehydrogenase (43). The changes of total polyphenols as well as citric acid, succinic acid, lactic acid and oxalic acid contents were similar to our previous study (17), in which the contents of polyphenols and lactic acid increased, citric acid and succinic acid decreased, and oxalic acid showed no obvious changes after the Moringa oleifera leaves were fermented with Lactobacillus plantarum S35. However, the changes of total flavonoids, pyruvic acid and acetic acid content in this study showed opposite trend with our previous study (17), which might due to the difference of fermentation condition and the used fermentation strain. Furthermore, in the study of Jin et al. (11), except for GABA content, the lactic acid and acetic acid contents in GABA-enriched green tea were also significantly increased after fermentation with Levilactobacillus strain GTL 79, which was similar with present study result.
It has been reported that GABA suppressed the mRNA expression levels of key mediators in the NF-κB signaling pathway induced by LPS in MAC-T cells, such as TLR-4, myeloid differentiation 88 (MyD88), NF-κB p65 and TNF receptor-associated factor 6 (TRAF6), which indicated that GABA might suppresses inflammation by inhibiting the activity of the NF-κB signaling pathway (8). Furthermore, previous studies also showed that Moringa oleifera extract pretreatment could block LPS-induced activation of NF-κB p65 subunit in MAC-T cells and thus protect bovine mammary epithelial cells against inflammation (35). Several reports indicated that the high content of flavonoids and phenolic compounds contained in Moringa oleifera leaves contributed to its anti-inflammatory activity (15, 44, 45). In addition, it has been reported that the enhanced effect of fermentation on the anti-inflammatory activity of Laminaria japonica was possibly due to the productions of more bioactive constituents after fermentation, such as fucoidan, alginate and phenols (20). Zhang et al. (3) also verified that the enhancement of antioxidant and anti-inflammatory activities of rape bee pollen after fermentation were mainly due to the increase of phenolics, flavonoids, fatty acids, and amino acids. Previous studies showed that except for the increase of GABA content, other active constituents like flavonoids, polyphenols, oligosaccharides, organic acids of Moringa oleifera leaves were also significantly increased after fermentation (12, 17). In our study, the GABA (18), total polyphenols, and most organic acids like pyruvic acid, lactic acid as well as acetic acid were increased obviously after fermentation. Though the total flavonoids content in MLFB decreased after fermentation, there were still 32 mg/L of flavonoids remained. Therefore, the anti-inflammatory activity that MLFB showed on LPS-induced cell inflammatory model might be associated with GABA and other active constituents such as flavonoids, phenolics and organic acids contained in the fermentation broth.
The possible anti-inflammatory mechanism of MLFB was summarized in Figure 7. In detail, the high content of GABA and other active constituents like flavonoids, polyphenols contained in MLFB inhibited the expression of the main transmembrane signaling receptor TLR-4, and therefore disturbed its interaction with LPS. As a result, the stimulation of LPS to the macrophages was reduced and the NF-κB signaling pathway was inhibited, which further led to the secretion decrease of pro-inflammatory mediators and cytokines like iNOS, PGE2, IL-1β, IL-6, IL-8 and TNF-α, eventually the inflammation was alleviated.
4. Conclusion
In this study, the anti-inflammatory activity of MLFB was investigated and its underlying anti-inflammatory mechanism was clarified on LPS-induced RAW 264.7 cells model combining with its key active components analysis before and after fermentation. The results showed that MLFB suppressed the secretions and expressions of pro-inflammatory cytokines such as IL-1β, IL-6, IL-8 and TNF-α in LPS-induced RAW 264.7 cells. In addition, the expressions of PGE2 and iNOS were inhibited by MLFB, which might contribute to the decrease of pro-inflammatory cytokines and NO productions. Moreover, the mRNA expressions of the key molecules (TLR-4 and NF-κB p65) in the NF-κB signaling pathway were also restrained by MLFB in a dose-dependent manner, which indicated that the activity of the NF-κB signaling pathway was inhibited. In conclusion, MLFB can effectively ameliorate LPS-induced inflammation by inhibiting the secretions of pro-inflammatory cytokines and its underlying mechanism may be associated with the inhibition of TLR-4/NF-κB inflammatory signaling pathway activation. Besides, the anti-inflammatory activity of MLFB might related to the relative high contents of GABA as well as other active constituents such as flavonoids, phenolics and organic acids in MLFB. Our research provides a theoretical basis for the follow-up development of GABA-enriched Moringa oleifera functional foods with anti-inflammatory functions. However, the present study about the anti-inflammatory mechanism of MLFB was preliminary. Its in-depth mechanisms against inflammation should be further clarified and the active ingredients contained in MLFB also need to be further clarified.
Data availability statement
The original contributions presented in the study are included in the article/Supplementary material, further inquiries can be directed to the corresponding authors.
Author contributions
LZ: conceptualization, methodology, and validation. XL: data curation, writing—original draft preparation, and visualization. SY: methodology and data curation. FZ: funding acquisition and validation. YZ: resources and writing—review and editing. SX: formal analysis and validation. YC: conceptualization and funding acquisition. WZ: writing—review and editing, funding acquisition, and supervision. All authors contributed to the article and approved the submitted version.
Funding
This research was funded by the Central Public-interest Scientific Institution Basal Research Fund (1630122022004), the Scientific and Technological Achievements Transformation Project of Zhanjiang City (2022A05027), the Innovative and Entrepreneurship Team Project for Pilot Program of Zhanjiang City (211207157080998), the Innovative Research Team Construction Project for Modern Agricultural Industry Common Key Technologies of Guangdong Province (2021KJ117), and Guangdong Special Support Program Science and Technology Innovation Youth Top Talent Project (2019TQ05N133).
Conflict of interest
The authors declare that the research was conducted in the absence of any commercial or financial relationships that could be construed as a potential conflict of interest.
Publisher's note
All claims expressed in this article are solely those of the authors and do not necessarily represent those of their affiliated organizations, or those of the publisher, the editors and the reviewers. Any product that may be evaluated in this article, or claim that may be made by its manufacturer, is not guaranteed or endorsed by the publisher.
Supplementary material
The Supplementary Material for this article can be found online at: https://www.frontiersin.org/articles/10.3389/fnut.2023.1093036/full#supplementary-material
References
1. Arulselvan P, Tan WS, Gothai S, Muniandy K, Fakurazi S, Esa NM, et al. Anti-inflammatory potential of ethyl acetate fraction of Moringa oleifera in downregulating the NF-κB signaling pathway in lipopolysaccharide-stimulated macrophages. Molecules. (2016) 21:1452. doi: 10.3390/molecules21111452
2. Cui C, Chen S, Wang X, Yuan G, Jiang F, Chen X, et al. Characterization of Moringa oleifera roots polysaccharide MRP-1 with anti-inflammatory effect. Int J Biol Macromol. (2019) 132:844–51. doi: 10.1016/j.ijbiomac.2019.03.210
3. Zhang H, Zhu X, Huang Q, Zhang L, Liu X, Liu R, et al. Antioxidant and anti-inflammatory activities of rape bee pollen after fermentation and their correlation with chemical components by ultra-performance liquid chromatography-quadrupole time of flight mass spectrometry-based untargeted metabolomics. Food Chem. (2023) 409:135342. doi: 10.1016/j.foodchem.2022.135342
4. Lu CC, Yen GC. Antioxidative and anti-inflammatory activity of functional foods. Curr Opin Food Sci. (2015) 2:1–8. doi: 10.1016/j.cofs.2014.11.002
5. Wang Y, Liu C, Ma T, Zhao J. Physicochemical and functional properties of gamma-aminobutyric acid-treated soy proteins. Food Chem. (2019) 295:267–73. doi: 10.1016/j.foodchem.2019.05.128
6. Cataldo PG, Villena J, Elean M, Savoy de Giori G, Saavedra L, Hebert EM. Immunomodulatory properties of a gamma-aminobutyric acid-enriched strawberry juice produced by Levilactobacillus brevis CRL 2013. Front Microbiol. (2020) 11:610016. doi: 10.3389/fmicb.2020.610016
7. Soltani N, Qiu H, Aleksic M, Glinka Y, Zhao F, Liu R, et al. GABA exerts protective and regenerative effects on islet β cells and reverses diabetes. P Natl A Sci. (2011) 108:11692–7. doi: 10.1073/pnas.1102715108
8. Wang YY, Sun SP, Zhu HS, Jiao XQ, Zhong K, Guo YJ, et al. GABA regulates the proliferation and apoptosis of MAC-T cells through the LPS-induced TLR4 signaling pathway. Res Vet Sci. (2018) 118:395–402. doi: 10.1016/j.rvsc.2018.04.004
9. Cui Y, Miao K, Niyaphorn S, Qu X. Production of gamma-aminobutyric acid from Lactic acid bacteria: a systematic review. Int J Mol Sci. (2020) 21:995. doi: 10.3390/ijms21030995
10. Kwon SY, Garcia CV, Song YC, Lee SP. GABA-enriched water dropwort produced by co-fermentation with Leuconostoc mesenteroides SM and Lactobacillus plantarum K154. LWT Food Sci Technol. (2016) 73:233–8. doi: 10.1016/j.lwt.2016.06.002
11. Jin YH, Hong JH, Lee JH, Yoon H, Pawluk AM, Yun SJ, et al. Lactic acid fermented green tea with Levilactobacillus brevis capable of producing γ-aminobutyric acid. Fermentation. (2021) 7:110. doi: 10.3390/fermentation7030110
12. Shi H, Yang E, Yang H, Huang X, Zheng M, Chen X, et al. Dynamic changes in the chemical composition and metabolite profiles of drumstick (Moringa oleifera Lam.) leaf flour during fermentation. LWT Food Sci Technol. (2022) 155:112973. doi: 10.1016/j.lwt.2021.112973
13. Ma ZF, Ahmad J, Zhang H, Khan I, Muhammad S. Evaluation of phytochemical and medicinal properties of Moringa (Moringa oleifera) as a potential functional food. S Afr J Bot. (2020) 129:40–6. doi: 10.1016/j.sajb.2018.12.002
14. Leone A, Spada A, Battezzati A, Schiraldi A, Aristil J, Bertoli S. Cultivation, genetic, ethnopharmacology, phytochemistry and pharmacology of Moringa oleifera leaves: an overview. Int J Mol Sci. (2015) 16:12791–835. doi: 10.3390/ijms160612791
15. Xu YB, Chen GL, Guo MQ. Antioxidant and anti-inflammatory activities of the crude extracts of Moringa oleifera from Kenya and their correlations with flavonoids. Antioxidants. (2019) 8:296. doi: 10.3390/antiox8080296
16. Ahmad S, Pandey AR, Rai AK, Singh SP, Kumar P, Singh S, et al. Moringa oleifera impedes protein glycation and exerts reno-protective effects in streptozotocin-induced diabetic rats. J Ethnopharmacol. (2023) 305:116–7. doi: 10.1016/j.jep.2022.116117
17. Li Q, Xia X, Zhou W, Liao L, Li J, Peng S, et al. Changes of nutritional components and antioxidant activities of Moringa oleifera leaf extract during fermentation. Sci Technol Food Ind. (2019) 40:110–5. doi: 10.13386/j.issn1002-0306.2019.01.021
18. Li Q, Zhou W, Xia X, Liao L, Peng S, Li J. Enrichment of γ-aminobutyric acid in Moringa oleifera leaves by Lactobacillus fermentation. J Guangdong Ocean Univ. (2018) 38:50–6. doi: 10.3969/j.issn.1673-9159.2018.05.008
19. Liao LK, Wei XY, Gong X, Li JH, Huang T, Xiong T. Microencapsulation of Lactobacillus casei LK-1 by spray drying related to its stability and in vitro digestion. LWT Food Sci Technol. (2017) 82:82–9. doi: 10.1016/j.lwt.2017.03.065
20. Lin HTV, Lu WJ, Tsai GJ, Chou CT, Hsiao HI, Hwang PA. Enhanced anti-inflammatory activity of brown seaweed Laminaria japonica by fermentation using Bacillus subtilis. Process Biochem. (2016) 51:1945–53. doi: 10.1016/j.procbio.2016.08.024
21. Van Thanh N, Jang HJ, Vinh LB, Linh KTP, Huong PTT, Cuong NX, et al. Chemical constituents from Vietnamese mangrove Calophyllum inophyllum and their anti-inflammatory effects. Bioorg Chem. (2019) 88:102921. doi: 10.1016/j.bioorg.2019.102921
22. Prudhomme GJ, Glinka Y, Wang Q. Immunological GABAergic interactions and therapeutic applications in autoimmune diseases. Autoimmun Rev. (2015) 14:1048–56. doi: 10.1016/j.autrev.2015.07.011
23. Reyes-Garcia MG, Hernandez-Hernandez F, Hernandez-Tellez B, Garcia-Tamayo F, GABA. (A) receptor subunits RNA expression in mice peritoneal macrophages modulate their IL-6/IL-12 production. J Neuroimmunol. (2007) 188:64–8. doi: 10.1016/j.jneuroim.2007.05.013
24. Vincent Hung-Shu C, Fu S-C. In vitro anti-inflammatory properties of fermented pepino (Solanum muricatum) milk by gammaaminobutyric acid-producing Lactobacillus brevis and an in vivo animal model for evaluating its effects on hypertension. J Sci Food Agric. (2016) 96:192–8. doi: 10.1002/jsfa.7081
25. Han BH, Lee YJ, Yoon JJ, Choi ES, Namgung S, Jin XJ, et al. Hwangryunhaedoktang exerts anti-inflammation on LPS-induced NO production by suppressing MAPK and NF-κB activation in RAW2647 macrophages. J Integr Med. (2017) 15:326–36. doi: 10.1016/S2095-4964(17)60350-9
26. Shi J, Li H, Liang S, Evivie SE, Huo G, Li B, et al. Selected lactobacilli strains inhibit inflammation in LPS-induced RAW2647 macrophages by suppressing the TLR4-mediated NF-κB and MAPKs activation. Food Sci Technol. (2022) 42:e107621. doi: 10.1590/fst.107621
27. Dange RB, Agarwal D, Masson GS, Vila J, Wilson B, Nair A, et al. Central blockade of TLR4 improves cardiac function and attenuates myocardial inflammation in angiotensin II-induced hypertension. Cardiovasc Res. (2014) 103:17–27. doi: 10.1093/cvr/cvu067
28. Hwangbo H, Ji SY, Kim MY, Kim SY, Lee H, Kim GY, et al. Anti-inflammatory effect of auranofin on palmitic acid and LPS-induced inflammatory response by modulating TLR4 and NOX4-mediated NF-κB signaling pathway in RAW2647 macrophages. Int J Mol Sci. (2021) 22:5920. doi: 10.3390/ijms22115920
29. Guha M, Mackman N. LPS induction of gene expression in human monocytes. Cell Signal. (2001) 13:85–94. doi: 10.1016/S0898-6568(00)00149-2
30. Chang J, Wang L, Zhang M, Lai Z. Glabridin attenuates atopic dermatitis progression through downregulating the TLR4/MyD88/NF-κB signaling pathway. Genes Genom. (2021) 43:847–55. doi: 10.1007/s13258-021-01081-4
31. Chalmers SA, Garcia SJ, Reynolds JA, Herlitz L, Putterman C. NF-κB signaling in myeloid cells mediates the pathogenesis of immune-mediated nephritis. J Autoimmun. (2019) 98:33–43. doi: 10.1016/j.jaut.2018.11.004
32. Niu X, Zang L, Li W, Xiao X, Yu J, Yao Q, et al. Anti-inflammatory effect of Yam Glycoprotein on lipopolysaccharide-induced acute lung injury via the NLRP3 and NF-κB/TLR4 signaling pathway. Int Immunopharmacol. (2020) 81:106024. doi: 10.1016/j.intimp.2019.106024
33. Tsai YC, Chen SH, Lin LC, Fu SL. Anti-inflammatory principles from Sarcandra glabra. J Agric Food Chem. (2017) 65:6497–505. doi: 10.1021/acs.jafc.6b05125
34. Cheng WN, Jeong CH, Seo HG, Han SG. Moringa extract attenuates inflammatory responses and increases gene expression of casein in bovine mammary epithelial cells. Animals. (2019) 9:391. doi: 10.3390/ani9070391
35. Luo J, Li XJ, Lee GH, Huang JJ, Whang WK, Zhang XD, et al. Anti-inflammatory effects of two lupane-type triterpenes from leaves of Acanthopanax gracilistylus on LPS-induced RAW2647 macrophages. Food Sci Technol. (2022) 42:e89721. doi: 10.1590/fst.89721
36. Ru YR, Wang ZX Li YJ, Kan H, Kong KW, Zhang XC. The influence of probiotic fermentation on the active compounds and bioactivities of walnut flowers. J Food Biochem. (2022) 46:e13887. doi: 10.1111/jfbc.13887
37. Chang YT, Chen HC, Chou HL Li H, Boyd SA, A. coupled UV photolysis- biodegradation process for the treatment of decabrominated diphenyl ethers in an aerobic novel bioslurry reactor. Environ Sci Pollut R. (2021) 28:6078–89. doi: 10.1007/s11356-020-10753-9
38. Chen R, Chen W, Chen H, Zhang G, Chen W. Comparative evaluation of the antioxidant capacities, organic acids, and volatiles of papaya juices fermented by Lactobacillus acidophilus and Lactobacillus plantarum. J Food Quality. (2018) 2018:9490435. doi: 10.1155/2018/9490435
39. Shahidi F, Yeo JD. Insoluble-bound phenolics in food. Molecules. (2016) 21:1216. doi: 10.3390/molecules21091216
40. Ji G, Liu G, Li B, Tan H, Zheng R, Sun X, et al. Influence on the aroma substances and functional ingredients of apple juice by lactic acid bacteria fermentation. Food Biosci. (2023) 51:102337. doi: 10.1016/j.fbio.2022.102337
41. Chidi BS, Bauer FF, Rossouw D. Organic acid metabolism and the impact of fermentation practices on wine acidity: a review. South African J Enol Vitic. (2018) 39:315–29. doi: 10.21548/39-2-3172
42. Cirlini M, Ricci A, Galaverna G, Lazzi C. Application of lactic acid fermentation to elderberry juice: Changes in acidic and glucidic fractions. LWT Food Sci Technol. (2020) 118:108779. doi: 10.1016/j.lwt.2019.108779
43. Liu X, Zhao G, Sun S, Fan C, Feng X, Xiong P. Biosynthetic pathway and metabolic engineering of succinic acid. Front Bioeng Biotechnol. (2022) 10:843887. doi: 10.3389/fbioe.2022.843887
44. Guo Y, Sun L, Zhuang Y. UPLC-Q-Orbitrap-MS2 analysis of Moringa oleifera leaf extract and its antioxidant, antibacterial and anti-inflammatory activities. Nat Prod Res. (2020) 34:2090–4. doi: 10.1080/14786419.2019.1573237
Keywords: Moringa oleifera leaves, fermentation, gamma-aminobutyric acid, anti-inflammatory activity, RAW 264.7 cells
Citation: Zheng L, Lu X, Yang S, Zou Y, Zeng F, Xiong S, Cao Y and Zhou W (2023) The anti-inflammatory activity of GABA-enriched Moringa oleifera leaves produced by fermentation with Lactobacillus plantarum LK-1. Front. Nutr. 10:1093036. doi: 10.3389/fnut.2023.1093036
Received: 08 November 2022; Accepted: 20 February 2023;
Published: 09 March 2023.
Edited by:
Lin Zhou, Guangdong Pharmaceutical University, ChinaReviewed by:
Kashif Ameer, Chonnam National University, Republic of KoreaYongai Xiong, Zunyi Medical University, China
Copyright © 2023 Zheng, Lu, Yang, Zou, Zeng, Xiong, Cao and Zhou. This is an open-access article distributed under the terms of the Creative Commons Attribution License (CC BY). The use, distribution or reproduction in other forums is permitted, provided the original author(s) and the copyright owner(s) are credited and that the original publication in this journal is cited, in accordance with accepted academic practice. No use, distribution or reproduction is permitted which does not comply with these terms.
*Correspondence: Xuli Lu, bHV4dWxpMDUwMiYjeDAwMDQwOzE2My5jb20=; Ying Zou, bTE4OTk4MzY4NjQxJiN4MDAwNDA7MTYzLmNvbQ==