- 1School of Pharmaceutical Sciences, Taizhou University, Taizhou, China
- 2Department of Pharmacological and Pharmaceutical Sciences, College of Pharmacy, University of Houston, Houston, TX, United States
- 3Department of Food Science and Engineering, Jinan University, Guangzhou, China
- 4Guangzhou Key Laboratory of Formula-Pattern Research Center, School of Traditional Chinese Medicine, Jinan University, Guangzhou, China
- 5The First Affiliated Hospital, Jinan University, Guangzhou, China
Plant-based functional foods have attracted increasing research interest to validate their use in preventing metabolic disease. Since it is increasingly recognized that inflammation, oxidative stress, and circadian rhythm play vital roles in various metabolic diseases, including diabetes, obesity and non-alcoholic liver disease, plant proteins, protein hydrolysates, and food extracts that intervene in these biological processes are promising dietary supplements to prevent metabolic diseases. Here, we reviewed the recent research on plant-based foods used for metabolic disease prevention and provided new perspectives regarding the current study gaps and future directions in this field.
1. Introduction
Plant-based functional foods have been used to prevent metabolic disorders such as type 2 diabetes, obesity, and cardiovascular diseases for years (1–5). However, the active components of many of these compounds, as well as the mechanism underlying these beneficial effects, have not yet been clearly elucidated. It is well-known that inflammation and oxidative stress promote the development of many metabolic diseases (6, 7). In particular, it has been shown that proinflammatory cytokines have profound impacts on glucose metabolism, lipogenesis and xenobiotic metabolism in the liver, which makes inhibiting inflammation a potential strategy to prevent the incidence of diabetes and non-alcoholic fatty liver disease (NAFLD) (8, 9). Markova et al. (10) recently reported that a diet high in plant protein significantly reduced liver fat and improved insulin resistance and the inflammatory response in diabetes patients. Recently, the role of the circadian clock in the development of metabolic syndromes has been revealed (11). An increasing number of studies have reported that numerous plant-based functional foods alter the regulation of circadian clock-related gene expression, but many details remain unclear (12).
In this review, we summarized the recent studies on plant-based foods for metabolic disease prevention and provide new perspectives on the current study gaps and future directions in this field. We first discussed the inflammatory reaction, oxidative damage and circadian rhythm regulation in diabetes and obesity. Next, we reviewed plant-based foods with anti-inflammatory and antioxidant functions and those that affect circadian rhythm, with a focus on plant proteins and active extracts.
2. Role of inflammation, oxidative stress, and the circadian rhythm in metabolic diseases
Accumulating evidence suggests that proinflammatory pathways, redox signaling, and the circadian clock play important roles in the initiation and development of metabolic diseases (Figure 1). Many metabolic syndromes are associated with chronic inflammation, including tissue infiltration by monocytes, macrophages, and lymphocytes (13). The two major characteristics of type-2 diabetes (T2D) are insulin resistance and pancreatic β cell dysfunction (14–16). The finding that islet inflammation is involved in pancreatic β cell dysfunction highlights the importance of inflammation in these diseases. Recent evidence has indicated that intraislet macrophages are the main source of the increased proinflammatory cytokines in diabetes. Thus, targeting islet inflammation is an effective therapeutic strategy for T2D (17). Regarding the role of inflammation in obesity, most studies have focused on adipose tissue inflammation. When excessive accumulation of fat occurs in adipocytes, the inflammatory response can be detected in these cells. Gut-derived substances, dietary components or metabolites induce adipose tissue inflammation (18, 19). Alternatively, the rapid expansion of adipose tissue increases the inflammatory response through adipocyte death, hypoxia, and mechanotransduction arising from interactions between cells and the extracellular matrix (20). NAFLD causes liver dysfunction and is typically associated with advanced diabetes and obesity (21, 22). It is increasingly recognized that mediators released from immune cells and adipocytes impair insulin signaling in the liver (23). The disruption of insulin function leads to insulin resistance, which contributes to the development of NAFLD. The NF-κB/IKKβ pathway, JNK and PPARγ are involved in inflammatory accumulation and the development of NAFLD (24).
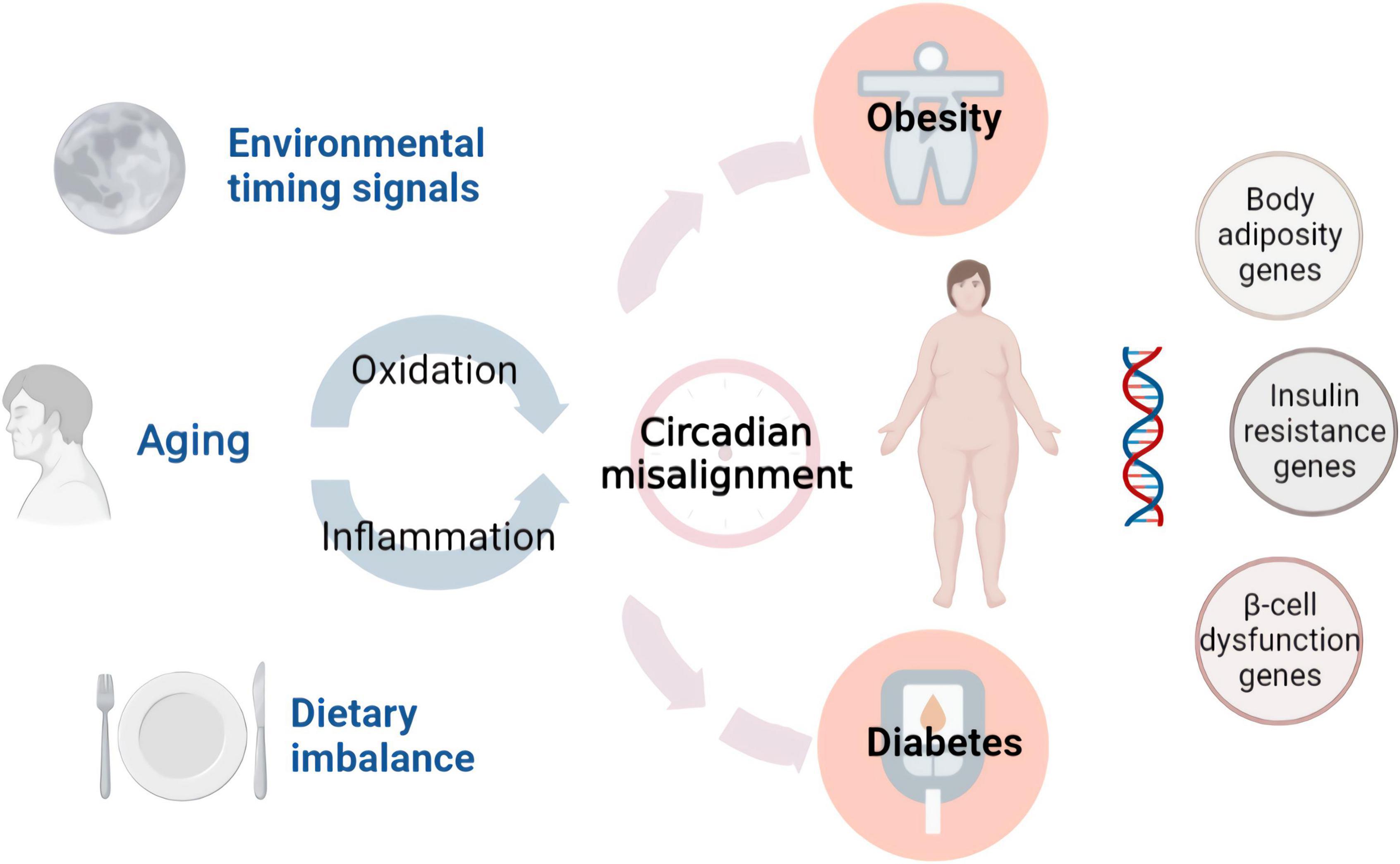
Figure 1. Environmental factors and endogenous damage in metabolic diseases. Functional foods prevent diseases by interfering with these factors.
Similar to chronic inflammation, oxidative stress occurs in diabetic and obese individuals. Oxidative stress greatly contributes to the development of metabolic disease-induced cardiovascular complications (25). High levels of metabolic activity and reactive oxygen species (ROS) are inevitable byproducts of mitochondrial respiration, and pancreatic β cells express fewer antioxidant enzymes. This imbalance may render pancreatic β cells susceptible to ROS. When glucose clearance is impaired, the increase in glycolytic flux increases ROS production in pancreatic β cells. In addition to these effects, hyperglycemia and hyperinsulinemia promote the generation of hydrogen peroxide in β cells. ROS and hydrogen peroxide induce oxidative damage to ribonucleic acids, proteins, and lipids. Consequently, the function of pancreatic β cells can be damaged by ROS through multiple mechanisms, including decreased enzyme activity, dysregulated gene expression, and apoptosis (26). The production of ROS is promoted in adipose tissue during obesity and is accompanied by increased NADPH oxidase expression and the inhibition of antioxidative enzymes. In adipocytes, elevated levels of fatty acids increase oxidative stress and dysregulate adipocytokines, including adiponectin, plasminogen activator inhibitor-1, IL-6, and monocyte chemotactic protein-1. A recent study revealed that the redox state of adipose tissue is a therapeutic target for obesity (27).
In addition to inflammation and oxidative stress, the role of the circadian rhythm in metabolic diseases has been increasingly recognized in recent years (28, 29). In the liver, the circadian clock is be associated with metabolism and energy homeostasis. This is achieved by mediating the expression of metabolic enzymes and transporters. Glucose metabolism in healthy individuals is controlled by circadian rhythm and plays a key role in pancreatic β cell function. Exogenous melatonin significantly impairs metabolic homeostasis. This finding indicates that a higher risk of T2D is associated with circadian disruptions associated with shift work, social jet lag, late chronotype, and genetic risk (30). The core clock mechanism has been shown to regulate lipogenesis pathways. Recent molecular studies have shown the involvement of brain and muscle ARNT-like 1 (BMAL1) in the regulation of adipogenesis and lipid metabolism. The loss of BMAL1 leads to a significant decrease in adipogenesis and the expression of several key lipogenic factors (31).
3. Plant-based functional foods exert anti-inflammatory effects
As an essential immune response, inflammation is involved in the development of metabolic disorders. In recent years, plant proteins have received increasing attention due to evidence showing their potential to prevent metabolic disorders through the production of proinflammatory cytokines and reactive oxygen species (Table 1).
3.1. Plant proteins that exert anti-inflammatory effects
Plant-based proteins have been the focus of research and are in extensive demand due to their bioactivity. Brewers’ spent grain (BSG) protein hydrolysates exert immunomodulatory effects in vitro, which can be beneficial in controlling inflammatory diseases (32). The regulation of the immune response includes Toll-like receptor 2 (TLR2), TLR4, NF-κB, and MAPKs. Moringa oleifera (moringa or drumstick) is a monogeneric plant. Its soluble seed fiber is a protease-resistant protein, which is a promising nutritional source to enhance the immune system. Moringa oleifera seeds significantly stimulated the proliferation of splenocytes and NO production (39). Common bean hydrolysates have been demonstrated to significantly inhibit the transactivation of NF-κB and the nuclear translocation of the NF-κB/p65 subunit, indicating that they can be used to treat inflammatory and oxidative diseases (34).
3.2. Crude extracts suppress inflammation
The methanolic extracts of leaves of Capparis spinosa showed anti-inflammatory effects in vitro. These extracts also inhibit membrane stabilization and exert anti-inflammatory effects in a mouse model (40). In addition, the extracts of Capparis spinosa leaves exhibited stronger anti-inflammatory activity than those of the fruits. An in vivo study in Swiss mice revealed that Capparis spinosa L. induced a significant decrease in immune cell infiltration, vasodilatation and dermis thickness by inhibiting the gene expression of cytokines, such as interferon gamma (IFN-γ), IL-17, and IL-4 (35). Another study reported that secondary metabolites of Capparis Spinosa, such as carotenoids and alkaloids, induced an anti-inflammatory response by suppressing IL-17 and inducing the IL-4 gene (41). This anti-inflammatory activity could be attributed to the presence of secondary metabolites in caper such as carotenoids, alkaloids (36, 42), and flavonoids or active protein hydrolysates. According to these studies, the Capparis Spinosa plant could be a valuable source of natural anti-inflammatory agents. Black raspberry has been reported to suppress superoxides, nitric oxide, and IL-6 (43). A randomized double-blind study reported that administering black raspberry for 12 weeks resulted in a significant decrease in inflammatory factors such as IL-6 and TNF-α (37). Another promising candidate for reducing the inflammatory response is extra virgin olive oil (EVOO). The anti-inflammatory activity of the SPE-Diol extract was assessed in response to LPS/IFNγ stimulation of J774.A1 macrophages (38). The results demonstrated the anti-inflammatory effects of the extract of EVOO, which decreased NO and PGE2 by inhibiting the expression of iNOS and COX-2. In addition, the anti-inflammatory effect was closely correlated with the total polyphenol content and DPPH scavenging activity. A recent study of Citri Reticulatae Pericarpium (CRP) showed that the beneficial effect of CRP extract on LPS-induced inflammation in RAW 264.7 cells involved inhibition of the MAPK and NF-κB signaling pathways. The extract acted on all three MAPK signaling pathways to reverse LPS-induced phosphorylation of JNK, ERK1/2, and p38 MAPK. Furthermore, the extract could inhibit the NF-κB signaling pathway by reducing the phosphorylation of NF-κB p65 (44).
4. Plant-based functional foods with antioxidant effects
Concerns about the long-term safety of synthetic antioxidants have led to increasing demand for natural antioxidants to lower the risk of oxidative stress-associated diseases. As the source of essential amino acids, plant proteins are considered functional ingredients that promote health. In vitro, the enzymatic hydrolysis of plant proteins to produce bioactive hydrolysates has been used to imitate protein degradation in the human gastrointestinal tract. The antioxidant properties of these hydrolysates have been reported in several studies (Table 2).
4.1. Plant proteins that exhibit antioxidant functions
The wide availability of plants belonging to the Cucurbitaceae family has prompted researchers to further explore the role and importance of the pharmacological properties of their byproducts. The functional properties of protein isolates from the byproducts of Cucurbit moschata, Citrullus lanatus, and Lagenaria siceraria have been well-investigated. A study to correlate the inhibition of free radicals with the concentrations of globulin hydrolysates prepared from the seeds of the Cucurbitaceae family showed that Cucurbitaceae seed-derived hydrolysates exhibited good antioxidant properties (51). The antioxidant activities of pumpkin (Cucurbita pepo) seed protein were reported in CCl4-induced liver injury (52). Cucurbitaceae seed protein hydrolysates showed higher antioxidant activity than unhydrolyzed seed protein, mainly due to the generation of bioactive peptides (53). The bioactivities are based on various factors, including the type of enzyme used for hydrolysis, the degree of hydrolysis, and the molecular weight distribution of the peptides. Furthermore, higher degrees of hydrolysis and smaller sizes of peptides are the key factors in the antioxidant effect. Although several in vitro studies have been performed, well-designed in vivo studies are still needed to confirm the antioxidant effects of Cucurbitaceae plant proteins on animals and humans.
4.2. Crude extracts that eliminate oxidative stress
Crude extracts that form during food processing are considered an alternative source of antioxidants for improved management and prevention of metabolic diseases associated with oxidative stress (54, 55). The major antioxidants found in plant extracts are phenolic compounds, which include phenolic acids, flavonoids, and anthocyanins (47). Numerous in vitro and in vivo studies have reported the effects of plant extract supplementation on oxidative stress in blood and peripheral tissues in humans (56). In vitro assays were performed on Canola and Brassica napus L. extracts using different extraction techniques to assess their antioxidant activities. Crude canola meal extract exhibited the highest antioxidant capacity in reducing H2O2-induced cytotoxicity, followed by sinapic acid, deodistillate extract, and accelerated canola meal solvent extract (45). Clinacanthus nutans, which belongs to the Acanthaceae family and is widely used as a traditional medicine, was examined and suppressed oxidative stress. Different phenolics were detected in these extracts, and protocatechuic acid was one of the most abundant. Aqueous methanolic leaf extract of Clinacanthus nutans was tested against hyperlipidemia-induced oxidative stress in rats. Leaf extracts attenuated oxidative stress by stimulating serum antioxidant enzyme activity and increasing hepatic antioxidant gene expression (46). Defatted Canarium odontophyllum pericarp and peel crude extracts reduced oxidative stress and lipid peroxidation in vitro. Canarium odontophyllum fruit is high in anthocyanins and possesses a stronger antioxidant capacity than most phenolic compounds. The results indicated that the crude extracts from the pericarp and peel have no toxic effects on human cell lines. However, extract derived from the peel of Canarium odontophyllum has a higher inhibitor effect against oxidative stress than the pericarp extract and could be used as a potential cardioprotective agent (47).
5. Plant-derived functional foods that regulate circadian rhythm
A number of studies have focused on circadian rhythm regulation by the crude extracts of natural source foods (Table 3). However, most studies have not examined the regulatory mechanisms of these crude extracts with respect to the circadian clock. The extract of tea could reverse daily circadian rhythm gene transcription (including Bmal1, Sirt1, Cry1/2, Per3, and Nampt) and relevant proteins in the liver and hypothalamus, leading to a series of physiological changes, including a decrease in blood pressure, the attenuation of insulin resistance and glucose/lipid metabolism regulation (57). After oral administration of proanthocyanidin obtained from grape seeds, the circadian genes Clock, Bmal1, Per2, Rorα, Rev-erbα, Nampt, and NAD were stimulated, which was accompanied by changes in melatonin and metabolite oscillation and lipid/glucose regulation in rats (58). Hericium erinaceus is an herbal medicine used in various Asian countries. The extract of Hericium erinaceus showed effects on behavioral rhythm, including the offset time of activity, sleep-wake cycle, and wakefulness around the end of the active phase (59). The extracts of Polyporus and Bupleuri radix induced phase delay and amplitude enhancement in mice and embryonic fibroblasts (MEFs) (60). Passionflower (Passiflora incarnata Linnaeus) held Generally Recognized as Safe (GRAS) status for use in foods in the USA and was listed in the European Pharmacopeia for having sedative effects. Passionflower extracts were reported to enhance dopamine and metabolic enzyme regulation by mediating Bmal1, Clock, Per1/2, and Cry1/2 (61). Treatment with momordin from bitter melon promoted PPARδ activity by upregulating PPARδ production and activation in HepG2 cells (62). The water extract of bitter melon (Momordica charantia L.) improved sleep quality and facilitated sleep by regulating the expression levels of the Clock, Per2 and Arntl genes (63).
6. Conclusion
An increasing number of plant-based functional foods have been reported to be effective in preventing metabolic diseases, some of which have been identified as plant proteins, peptides or essential amino acid residues. As circadian clock regulators, the rest of these factors have been tested as crude extracts without isolating the active components. Even for the identified plant protein, efficacy has been mostly tested in vitro. To date, no robust in vivo results have been reported, and convincing clinical studies are lacking. In addition to the lack of studies validating the mechanism of action and efficacy in humans, oral administration also limits the potential use of active plant protein-containing functional foods. The abundant microbiome in the gut may degrade these functional proteins or have undesirable effects. The relatively low absorption rate limits these active components prevent them from reaching the liver to regulate glucose and lipid mechanisms. We are sure that orally ingested plant proteins have the potential to regulate lipid and glucose metabolism and affect the expression levels of inflammation-, oxidative stress- and circadian clock-related genes. However, it is still unclear whether these signaling pathways are essential for bioactivity. In conclusion, there is still much to be explored in this area to validate plant-based functional foods as an effective approach to prevent metabolic diseases.
Author contributions
S-hY and GT wrote the original manuscript. YS and J-qH conceived and designed the study. All authors participated in drafting and revising the manuscript before its final submission and approved the submitted version.
Funding
This work was supported by the National Natural Science Foundation of China (31701594), Science and Technology Projects in Guangzhou (202102010073), Science and Technology Planning Project of Guangdong Province of China (202201020052), Natural Science Foundation of Guangdong Province (2021A1515010869), and Science and Technology Planning Project of Guangdong Province of China (KTP20190226).
Acknowledgments
We gratefully acknowledge the support of the K. C. Wong Education Foundation and Huang Zhendong Research Fund for Traditional Chinese Medicine of Jinan University (201911).
Conflict of interest
The authors declare that the research was conducted in the absence of any commercial or financial relationships that could be construed as a potential conflict of interest.
Publisher’s note
All claims expressed in this article are solely those of the authors and do not necessarily represent those of their affiliated organizations, or those of the publisher, the editors and the reviewers. Any product that may be evaluated in this article, or claim that may be made by its manufacturer, is not guaranteed or endorsed by the publisher.
Abbreviations
ARNTL, aryl hydrocarbon receptor nuclear translocator-like protein 1; BMAL1, brain and muscle arnt-like 1; BSG, Brewers’ spent grain; CHS, contact hypersensitivity; CLOCK, circadian locomotor output cycles kaput; COX-2, cyclooxygenase-2; CRY, pesticidal crystal proteins; DPPH, 1,1-diphenyl-2-picrylhydrazyl; EVOO, extra virgin olive oil; GRAS, generally recognized as safe; IFN- γ, interferon gamma; IKK β, inhibitor of nf- κ b kinase- β; IL-4, interleukin-4; IL-6, interleukin-6; IL-17, interleukin-17; iNOS, inducible nitric oxide synthase; JNK, c-junn-terminal kinase; LPS, lipopolysaccharide; MAPKs, mitogen-activated protein kinases; MEFs, mice and embryonic fibroblasts; MTNR1B, melatonin receptor 1B; NAD, nicotinamide adenine dinucleotide; NAFLD, non-alcoholic fatty liver disease; NAMPT, nicotinamide phosphoribosyl transferase; NF- κ B, nuclear factor kappa B; NO, nitric oxide; PBMCs, human peripheral blood mononuclear cells; PER3, period circadian protein homolog 3 protein; PGE2, prostaglandin2; PPAR γ, peroxisome proliferator activated receptor γ; ROR α, rar-related orphan receptor alpha; ROS, reactive oxygen species; SIRT1, sirtuin 1; TLR2, toll-like receptor 2; TLR4, toll-like receptor 4; TNF- α, tumor necrosis factor- α; T2D, type-2 diabetes.
References
1. Huang J, Wang X, Tao G, Song Y, Ho C, Zheng J, et al. Feruloylated oligosaccharides from maize bran alleviate the symptoms of diabetes in streptozotocin-induced type 2 diabetic rats. Food Funct. (2018) 9:1779–89. doi: 10.1039/c7fo01825h
2. Ou J, Huang J, Zhao D, Du B, Wang M, Wu Y, et al. Protective effect of rosmarinic acid and carnosic acid against streptozotocin-induced oxidation, glycation, inflammation and microbiota imbalance in diabetic rats. Food Funct. (2018) 9:851–60. doi: 10.1039/c7fo01508a
3. Kuo T-F, Yang G, Chen T-Y, Wu Y, Tran Nguyen Minh H, Chen L, et al. Bidens pilosa: nutritional value and benefits for metabolic syndrome. Food Front. (2021) 2:32–45. doi: 10.1002/fft2.63
4. Agunloye O-M, Oboh G. Blood glucose lowering and effect of oyster (Pleurotus ostreatus)-and shiitake (Lentinus subnudus)-supplemented diet on key enzymes linked diabetes and hypertension in streptozotocin-induced diabetic in rats. Food Front. (2022) 3:161–71. doi: 10.1002/fft2.111
5. Zhang Y, Xie Q, You L, Cheung P, Zhao Z. Behavior of non-digestible polysaccharides in gastrointestinal tract: a mechanistic review of its anti-obesity effect. eFood. (2021) 2:59–72. doi: 10.2991/efood.k.210310.001
6. Song Y, Wu M-S, Tao G, Lu M, Lin J, Huang J. Feruloylated oligosaccharides and ferulic acid alter gut microbiome to alleviate diabetic syndrome. Food Res Int. (2020) 137:109410. doi: 10.1016/j.foodres.2020.109410
7. Michońska I, Łuszczki E, Zielińska M, Oleksy Ł;, Stolarczyk A, Dereń K. Nutritional programming: history, hypotheses, and the role of prenatal factors in the prevention of metabolic diseases—A narrative review. Nutrients. (2022) 14:4422. doi: 10.3390/nu14204422
8. Ou J, Huang J, Wang M, Ou S. Effect of rosmarinic acid and carnosic acid on AGEs formation in vitro. Food Chem. (2017) 2017:1057–61. doi: 10.1016/j.foodchem.2016.11.056
9. Huang J, Ren J, Tao G, Chen Y, Yao S, Han D, et al. Maize bran feruloylated oligosaccharides inhibited AGEs formation in glucose/amino acids and glucose/BSA models. Food Res Int. (2019) 122:443–9. doi: 10.1016/j.foodres.2019.04.040
10. Markova M, Pivovarova O, Hornemann S, Sucher S, Frahnow T, Wegner K, et al. Isocaloric diets high in animal or plant protein reduce liver fat and inflammation in individuals with type 2 diabetes. Gastroenterology. (2017) 152:571.e–85.e. doi: 10.1053/j.gastro.2016.10.007
11. Deota S, Panda S. New horizons: circadian control of metabolism offers novel insight into the cause and treatment of metabolic diseases. J Clin Endocrinol Metab. (2021) 106:e1488–93. doi: 10.1210/clinem/dgaa691
12. Huang J-Q, Lu M, Ho C-T. Health benefits of dietary chronobiotics: beyond resynchronizing internal clocks. Food Funct. (2021) 12:6136–56. doi: 10.1039/d1fo00661d
13. Miao L, Liu C, Cheong MS, Zhong R, Tan Y, Rengasamy K, et al. Exploration of natural flavones’ bioactivity and bioavailability in chronic inflammation induced-type-2 diabetes mellitus. Crit Rev Food Sci Nutr. (2022) 2022:1–28. doi: 10.1080/10408398.2022.2095349
14. Wondmkun YT. Obesity, insulin resistance, and type 2 diabetes: associations and therapeutic implications. Diabetes Metab Syndr Obes. (2020) 13:3611–6. doi: 10.2147/dmso.s275898
15. Marselli L, Piron A, Suleiman M, Colli M, Yi X, Khamis A, et al. Persistent or transient human beta cell dysfunction induced by metabolic stress: specific signatures and shared gene expression with type 2 diabetes. Cell Rep. (2020) 33:108466. doi: 10.1016/j.celrep.2020.108466
16. Eizirik DL, Pasquali L, Cnop M. Pancreatic β-cells in type 1 and type 2 diabetes mellitus: different pathways to failure. Nat Rev Endocrinol. (2020) 16:349–62. doi: 10.1038/s41574-020-0355-7
17. Eguchi K, Nagai R. Islet inflammation in type 2 diabetes and physiology. J Clin Invest. (2017) 127:14–23. doi: 10.1172/jci88877
18. Ou J-Y, Huang J-Q, Song Y, Yao S, Peng X, Wang M, et al. Feruloylated oligosaccharides from maize bran modulated the gut microbiota in rats. Plant Foods Hum Nutr. (2016) 71:123–8. doi: 10.1007/s11130-016-0547-4
19. Xia X, Zhu L, Lei Z, Song Y, Tang F, Yin Z, et al. Feruloylated oligosaccharides alleviate dextran sulfate sodium-induced colitis in vivo. J Agric Food Chem. (2019) 67:9522–31. doi: 10.1021/acs.jafc.9b03647
20. Reilly SM, Saltiel AR. Adapting to obesity with adipose tissue inflammation. Nat Rev Endocrinol. (2017) 13:633–43. doi: 10.1038/nrendo.2017.90
21. Targher G, Corey KE, Byrne CD, Roden M. The complex link between NAFLD and type 2 diabetes mellitus–mechanisms and treatments. Nat Rev Gastroenterol Hepatol. (2021) 18:599–612. doi: 10.1038/s41575-021-00448-y
22. Godoy-Matos AF, Júnior WSS, Valerio CM. NAFLD as a continuum: from obesity to metabolic syndrome and diabetes. Diabetol Metab Syndr. (2020) 12:1–20. doi: 10.1186/s13098-020-00570-y
23. Zhang H, Gao X, Li K, Liu Y, Hettiarachichi D, Sunderland B, et al. Sandalwood seed oil ameliorates hepatic insulin resistance by regulating the JNK/NF-κB inflammatory and PI3K/AKT insulin signaling pathways. Food Funct. (2021) 12:2312–22. doi: 10.1039/d0fo03051a
24. Tilg H, Moschen AR. Insulin resistance, inflammation, and non-alcoholic fatty liver disease. Trends Endocrinol Metab. (2008) 19:371–9. doi: 10.1016/j.tem.2008.08.005
25. Monserrat-Mesquida M, Quetglas-Llabrés M, Capó X, Bouzas C, Mateos D, Pons A, et al. Metabolic syndrome is associated with oxidative stress and proinflammatory state. Antioxidants. (2020) 9:236. doi: 10.3390/antiox9030236
26. Gerber PA, Rutter GA. The role of oxidative stress and hypoxia in pancreatic beta-cell dysfunction in diabetes mellitus. Antioxid Redox Signal. (2017) 26:501–18. doi: 10.1089/ars.2016.6755
27. Fukunaka A, Fujitani Y. Role of zinc homeostasis in the pathogenesis of diabetes and obesity. Int J Mol Sci. (2018) 19:476. doi: 10.3390/ijms19020476
28. Song D, Yang CS, Zhang X, Wang Y. The relationship between host circadian rhythms and intestinal microbiota: a new cue to improve health by tea polyphenols. Crit Rev Food Sci Nutr. (2021) 61:–148. doi: 10.1080/10408398.2020.1719473
29. Lopez DEG, Lashinger LM, Weinstock GM, Bray M. Circadian rhythms and the gut microbiome synchronize the host’s metabolic response to diet. Cell Metab. (2021) 33:873–87. doi: 10.1016/j.cmet.2021.03.015
30. Mason IC, Qian J, Adler GK, Scheer F. Impact of circadian disruption on glucose metabolism: implications for type 2 diabetes. Diabetologia. (2020) 63:462–72. doi: 10.1007/s00125-019-05059-6
31. Froy O. Circadian rhythms and obesity in mammals. Int Sch Res Notices. (2012) 2012:437198. doi: 10.5402/2012/437198
32. Cian RE, Hernández-Chirlaque C, Gámez-Belmonte R, Drago S, Sánchez de Medina F, Martínez-Augustin O. Molecular action mechanism of anti-inflammatory hydrolysates obtained from brewers’ spent grain. J Sci Food Agric. (2020) 100:2880–8. doi: 10.1002/jsfa.10313
33. Anudeep S, Prasanna VK, Adya SM, Radha C. Characterization of soluble dietary fiber from Moringa oleifera seeds and its immunomodulatory effects. Int J Biol Macromol. (2016) 91:656–62. doi: 10.1016/j.ijbiomac.2016.06.013
34. Oseguera-Toledo ME, De Mejia EG, Dia VP, Amaya-Llano S. Common bean (Phaseolus vulgaris L.) hydrolysates inhibit inflammation in LPS-induced macrophages through suppression of NF-κB pathways. Food Chem. (2011) 127:1175–85. doi: 10.1016/j.foodchem.2011.01.121
35. El Azhary K, Tahiri Jouti N, El Khachibi M, Moutia M, Tabyaoui I, El Hou A, et al. Anti-inflammatory potential of Capparis spinosa L. in vivo in mice through inhibition of cell infiltration and cytokine gene expression. BMC Complement Altern Med. (2017) 17:81. doi: 10.1186/s12906-017-1569-7
36. Anwar F, Muhammad G, Hussain MA, Zengin G, Alkharfy KM, Ashraf M, et al. Capparis spinosa L.: a plant with high potential for development of functional foods and nutraceuticals/pharmaceuticals. Int J Pharmacol. (2016) 12:201–19. doi: 10.3923/ijp.2016.201.219
37. Jeong J-H, Jung H, Lee S-R, Leea H, Hwanga KT, Kimb T. Anti-oxidant, anti-proliferative and anti-inflammatory activities of the extracts from black raspberry fruits and wine. Food Chem. (2010) 123:338–44. doi: 10.1016/j.foodchem.2010.04.040
38. Urpi-Sarda M, Casas R, Chiva-Blanch G, Romero-Mamani E, Valderas-Martínez P, Arranz S, et al. Virgin olive oil and nuts as key foods of the Mediterranean diet effects on inflammatory biomarkers related to atherosclerosis. Pharmacol Res. (2012) 65:577–83. doi: 10.1016/j.phrs.2012.03.006
39. Sandanamudi A, Bharadwaj KR, Cheruppanpullil R. Data for chitin binding activity of Moringa seed resistant protein (MSRP). Data Brief. (2016) 9:335–7. doi: 10.1016/j.dib.2016.08.070
40. Zhou H, Jian R, Kang J, Huang X, Li Y, Zhuang C, et al. Anti-inflammatory effects of caper (Capparis spinosa L.) fruit aqueous extract and the isolation of main phytochemicals. J Agric Food Chem. (2010) 58:12717–21. doi: 10.1021/jf1034114
41. Moutia M, El Azhary K, Elouaddari A, Al Jahid A, Jamal Eddine J, Seghrouchni F, et al. Capparis spinosa L. promotes anti-inflammatory response in vitro through the control of cytokine gene expression in human peripheral blood mononuclear cells. BMC Immunol. (2016) 17:26. doi: 10.1186/s12865-016-0164-x
42. Serafini M, Peluso I, Raguzzini A. Flavonoids as anti-inflammatory agents. Proc Nutr Soc. (2010) 69:273–8. doi: 10.1017/s002966511000162x
43. Azofeifa G, Quesada S, Boudard F, Morena M, Cristol J, Pérez A, et al. Antioxidant and anti-inflammatory in vitro activities of phenolic compounds from tropical highland blackberry (Rubus adenotrichos). J Agric Food Chem. (2013) 61:5798–804. doi: 10.1021/jf400781m
44. Zhang X, Zhou Y, Cheong M-S, Khan H, Ruan C-C, Fu M, et al. Citri reticulatae Pericarpium extract and flavonoids reduce inflammation in RAW 264.7 macrophages by inactivation of MAPK and NF−κB pathways. Food Front. (2022) 14:785–95. doi: 10.1002/fft2.169
45. Li Y-F, Tang L-P, He R-R, Xu Z, He Q-Q, Xiang F-J, et al. Anthocyanins extract from bilberry enhances the therapeutic effect of pollen of Brassica napus L. on stress-provoked benign prostatic hyperplasia in restrained mice. J Funct Foods. (2013) 5:1357–65. doi: 10.1016/j.jff.2013.05.003
46. Sarega N, Imam MU, Ooi D-J, Chan K, Md EN, Zawawi N, et al. Phenolic rich extract from Clinacanthus nutans attenuates hyperlipidemia-associated oxidative stress in rats. Oxid Med Cell Longev. (2016) 2016:4137908. doi: 10.1155/2016/4137908
47. Khoo HE, Azlan A, Ismail A, Abas F, Hamid M. Inhibition of oxidative stress and lipid peroxidation by anthocyanins from defatted Canarium odontophyllum pericarp and peel using in vitro bioassays. PLoS One. (2014) 9:e81447. doi: 10.1371/journal.pone.0081447
48. Dal Forno AH, Câmara D, Parise B, Rodriguesa CF, Soares JJ, Wagner R, et al. Antioxidant and lipid lowering effects of dried fruits oil extract of Pterodon emarginatusin Caenorhabditis elegans. Arab J Chem. (2019) 12:4131–41. doi: 10.1016/j.arabjc.2016.04.001
49. Kuraya E, Nakada S, Touyama A, Itoh S. Improving the antioxidant functionality of Citrus junos Tanaka (Yuzu) fruit juice by underwater shockwave pretreatment. Food Chem. (2017) 216:123–9. doi: 10.1016/j.foodchem.2016.08.026
50. Wang F, Bao Y, Shen X, Zengin G, Lyu Y, Xiao J, et al. Niazirin from Moringa oleifera Lam. attenuates high glucose-induced oxidative stress through PKCζ/Nox4 pathway. Phytomedicine. (2021) 86:153066. doi: 10.1016/j.phymed.2019.153066
51. Ozuna C, León-Galván MF. Cucurbitaceae seed protein hydrolysates as a potential source of bioactive peptides with functional properties. Biomed Res Int. (2017) 2017:2121878. doi: 10.1155/2017/2121878
52. Nkosi CZ, Opoku AR, Terblanche SE. Antioxidative effects of pumpkin seed (Cucurbita pepo) protein isolate in CCl4-Induced liver injury in low-protein fed rats. Phytother Res. (2006) 20:935–40. doi: 10.1002/ptr.1977
53. Venuste M, Zhang X, Shoemaker CF, Karangwa E, Abbasa S, Kamdem PE. Influence of enzymatic hydrolysis and enzyme type on the nutritional and antioxidant properties of pumpkin meal hydrolysates. Food Funct. (2013) 4:811–20. doi: 10.1039/c3fo30347k
54. Oluwajuyitan T-D, Ijarotimi O-S, Fagbemi T-N. Plantain-based dough meal: nutritional property, antioxidant activity and dyslipidemia ameliorating potential in high-fat-induced rats. Food Front. (2022) 3:489–504. doi: 10.1002/fft2.133
55. Liu Y, Mei S, Xiao A, Liu L. Xanthine oxidase inhibitors screening, antioxidation, and DNA protection properties of geranium wilfordii maxim. eFood. (2022) 1:147–55. doi: 10.2991/efood.k.190802.004
56. Michiels JA, Kevers C, Pincemail J, Defraigne JO, Dommes J. Extraction conditions can greatly influence antioxidant capacity assays in plant food matrices. Food Chem. (2012) 130:986–93. doi: 10.1016/j.foodchem.2011.07.117
57. Qi G, Mi Y, Liu Z, Fan R, Qiao Q, Sun Y, et al. Dietary tea polyphenols ameliorate metabolic syndrome and memory impairment via circadian clock related mechanisms. J Funct Foods. (2017) 34:168–80. doi: 10.1016/j.jff.2017.04.031
58. Ribas-Latre A, Del Bas JM, Baselga-Escudero L, Casanova E, Arola-Arnal A, Salvadó M, et al. Dietary proanthocyanidins modulate the rhythm of BMAL1 expression and induce RORα transactivation in HepG2 cells. J Funct Foods. (2015) 13:336–44. doi: 10.1016/j.jff.2015.01.017
59. Furuta S, Kuwahara R, Hiraki E, Ohnuki K, Yasuo S, Shimizu K. Hericium erinaceus extracts alter behavioral rhythm in mice. Biomed Res. (2016) 37:227–32. doi: 10.2220/biomedres.37.227
60. Motohashi H, Sukigara H, Tahara Y, Saito K, Yamazaki M, Shiraishi T, et al. Polyporus and Bupleuri radix effectively alter peripheral circadian clock phase acutely in male mice. Nutr Res. (2017) 43:16–24. doi: 10.1016/j.nutres.2017.05.001
61. Man AWC, Xia N, Daiber A, Huige L. The roles of gut microbiota and circadian rhythm in the cardiovascular protective effects of polyphenols. Br J Pharmacol. (2020) 177:1278–93. doi: 10.1111/bph.14850
62. Sasa M, Inoue I, Shinoda Y, Takahashi S, Seo M, Komoda T, et al. Activating effect of momordin, extract of bitter melon (Momordica charantia L.), on the promoter of human PPARδ. J Atheroscler Thromb. (2009) 16:888–92. doi: 10.5551/jat.2790
63. Lin Y-H, Chen I-H. Method for Increasing Expressions of Clock Gene, Arntl Gene, and/Or Per2 Gene by Using Momordica Charantia Extract. Alexandria, VA: U.S. Patent Application (2017).
64. Mi Y, Qi G, Fan R, Ji X, Liu Z, Liu X. EGCG ameliorates diet-induced metabolic syndrome associating with the circadian clock. Biochim Biophys Acta Mol Basis Dis. (2017) 1863:1575–89. doi: 10.1016/j.bbadis.2017.04.009
65. Qi G, Mi Y, Fan R, Zhao B, Ren B, Liu X. Tea polyphenols ameliorates neural redox imbalance and mitochondrial dysfunction via mechanisms linking the key circadian regular Bmal1. Food Chem Toxicol. (2017) 110:189–99. doi: 10.1016/j.fct.2017.10.031
Keywords: food waste, inflammation, oxidative stress, circadian rhythm, metabolic disease
Citation: Yang S-h, Tao G, Yang L, Wu X, Liu J-w, Dagher F, Ou S-y, Song Y and Huang J-q (2023) Dietary phytochemical and metabolic disease prevention: Focus on plant proteins. Front. Nutr. 10:1089487. doi: 10.3389/fnut.2023.1089487
Received: 04 November 2022; Accepted: 06 January 2023;
Published: 25 January 2023.
Edited by:
Luca Rastrelli, University of Salerno, ItalyReviewed by:
Xin Zhang, Ningbo University, ChinaMuhammad Sohail Sarwar, Forman Christian College, Pakistan
Copyright © 2023 Yang, Tao, Yang, Wu, Liu, Dagher, Ou, Song and Huang. This is an open-access article distributed under the terms of the Creative Commons Attribution License (CC BY). The use, distribution or reproduction in other forums is permitted, provided the original author(s) and the copyright owner(s) are credited and that the original publication in this journal is cited, in accordance with accepted academic practice. No use, distribution or reproduction is permitted which does not comply with these terms.
*Correspondence: Jun-qing Huang, jqhuang@jnu.edu.cn; Yuan Song,
sybil9876@126.com
†These authors have contributed equally to this work