- 1Berlin School of Mind and Brain, Humboldt-Universität zu Berlin, Berlin, Germany
- 2Department of Decision Neuroscience and Nutrition, German Institute of Human Nutrition (DIfE), Potsdam, Germany
- 3Charité–Universitätsmedizin Berlin, Neuroscience Research Center, Berlin Institute of Health, Corporate Member of Freie Universität Berlin, Humboldt-Universität zu Berlin, Berlin, Germany
- 4Deutsches Zentrum für Diabetesforschung, Neuherberg, Germany
Dietary choice during pregnancy is crucial not only for fetal development, but also for long-term health outcomes of both mother and child. During pregnancy, dramatic changes in endocrine, cognitive, and reward systems have been shown to take place. Interestingly, in different contexts, many of these mechanisms play a key role in guiding food intake. Here, we review how food intake may be impacted as a function of pregnancy-induced changes across species. We first summarize changes in endocrine and metabolic signaling in the course of pregnancy. Then, we show how these may be related to cognitive function and reward processing in humans. Finally, we link these to potential drivers of change in eating behavior throughout the course of pregnancy.
Introduction
Pregnancy is a time of major hormonal, physiological, and cognitive change for the mother, and of vital development for the child. Dietary intake is particularly important during this period, as it shapes both short- and long-term health outcomes of mother and child. Diet during pregnancy influences the development of gestational disease in pregnant women (1). For example, it impacts gestational diabetes mellitus (2), which is the development of glucose intolerance during pregnancy (3), and pre-eclampsia (4, 5), which is the development of hypertension and increased protein levels in the urine during pregnancy (6). Gestational diabetes increases risk for hypertensive disorders (including pre-eclampsia) as well as preterm birth and infants born large for gestational age (7). Dietary intake during pregnancy also influences health outcomes through its impact on weight gain. Overweight and obesity, as well as excessive weight gain are associated with health complications during pregnancy (8), such as thrombosis (9) and caesarian delivery (9). In offspring, maternal food intake can impact neurobiological development. For example, maternal high fat diets influence dopaminergic (10), hypothalamic (11), and hippocampal (12) development in rodents. Maternal diet also impacts other important aspects of development, such as the infant’s gut microbiome (13).
Dietary choice during pregnancy continues to impact health outcomes of mother and child even after pregnancy. In offspring, nutrient exposure during pregnancy impacts disease development later in life (14) such as obesity, diabetes (15), cancer (16), and asthma (17). Higher diet quality during pregnancy has been associated with higher neurodevelopment (18) and intelligence scores (19) in childhood. Higher intake of highly processed foods in pregnancy has been associated with worse verbal functioning in childhood (20). The aforementioned impact of maternal high fat diets on neuronal circuitry development impacts eating behaviors later in life, for example, non-human primates exposed to such diets in utero are more likely to later choose foods high in fat and sugar, and also show suppressed dopamine signaling (21). The impact of maternal diet on the infant gut microbiome has important implications for health outcomes such as asthma (22) and the functioning of the immune system (23). In pregnant women, gestational diabetes is associated with at least a sevenfold increased risk of developing type 2 diabetes later in life (24), as well as an increased risk of cardiovascular disease (25). Preeclampsia is associated with a multitude of long-term health outcomes (26), such as roughly double the risk of early cardiac disease (27) and an increased risk of renal disease (28). Further, women who gain more weight during pregnancy retain more of this weight gain both 1 and 15 years after delivery (29). Diet during pregnancy, therefore, is important to the health of mother and child both during pregnancy and post-pregnancy.
Beyond the importance of preventing undesirable health outcomes, the magnitude of an event such as pregnancy may make it a “teachable moment.” A teachable moment is a life event during which those experiencing it are especially amenable to positive lifestyle behavioral change (30). Pregnancy can be considered such an event, as it is a period in which women are more concerned about health-related behaviors, and have increased contact with healthcare providers (31). Therefore, effective nutrition interventions may be especially impactful on positive long-term health behaviors of women if they are administered during pregnancy (31).
Despite the importance of dietary intake during pregnancy, sufficient research on how to improve diet and associated health outcomes during pregnancy is lacking. According to a review by Skouteris et al. (32), diet improvement outcomes from health interventions in pregnant women have produced inconsistent results. Encouraging healthy gestational weight gain through current healthcare provider advice has also not produced consistent improvements (33). Further, interventions are still not effective at improving many critical outcomes, such as gestational diabetes (1). There is a need to move beyond simple dietary advice, and incorporate other important factors guiding food intake (32). For this, we require a better understanding of the relevant mechanisms guiding dietary choice during pregnancy (32).
Recent research on dietary choice has highlighted the importance of underlying mechanisms involving metabolic, reward, and cognitive processes (34). During pregnancy, the maternal body and brain undergo hormonally driven changes that result in alterations in these mechanisms of metabolic functioning (35), reward processing (36), and cognition (36). A better understanding of these pregnancy-related changes to important mechanisms underlying eating behavior would be helpful in understanding what shapes dietary choice during pregnancy (see Figure 1). This can foster the efficacy of healthcare provider advice and interventions to promote healthy dietary choice during pregnancy.
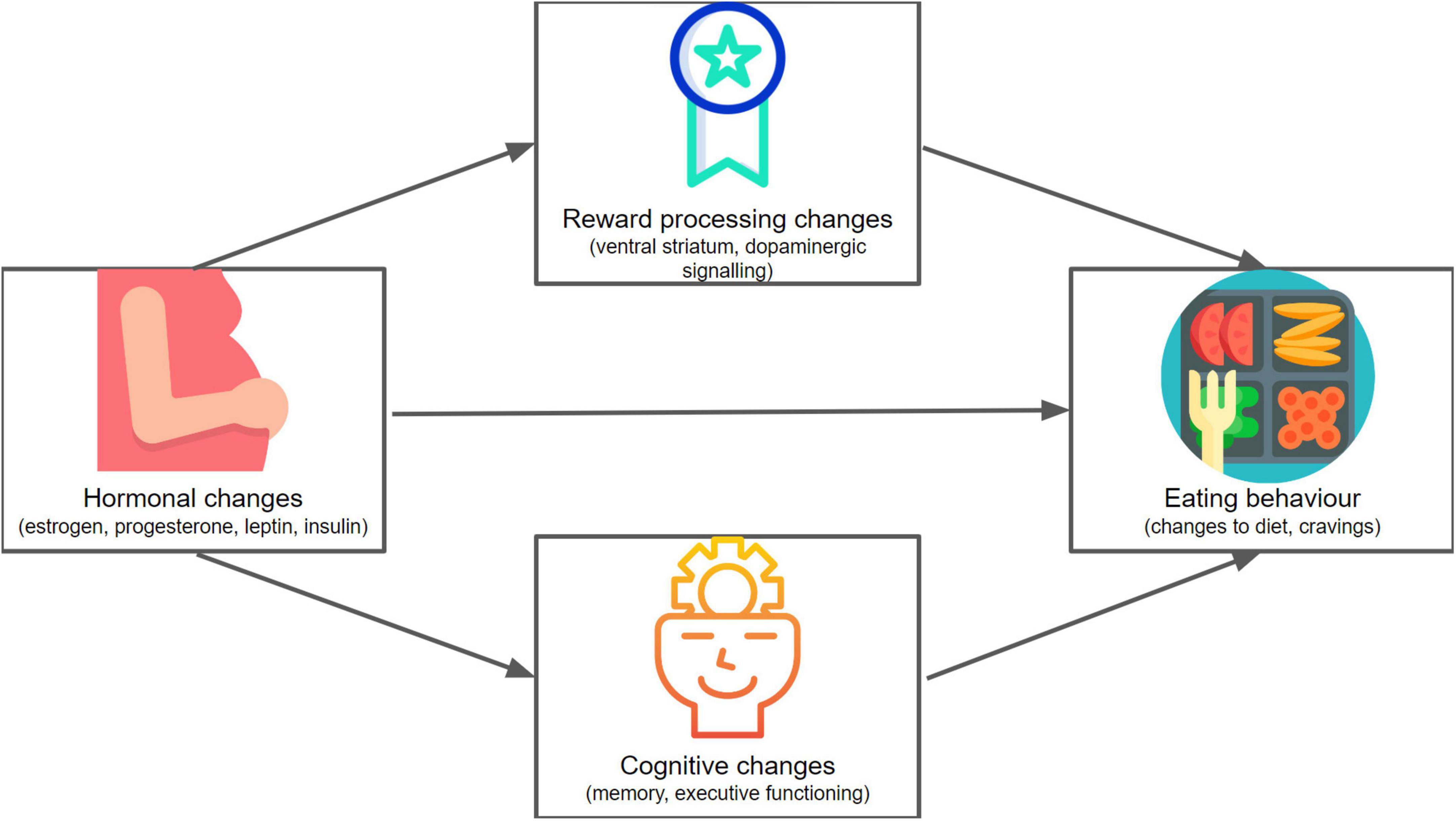
Figure 1. A conceptual mapping of the framework of this review. We explore hormonal (including metabolic), reward-related, and cognitive changes in pregnancy, and consider how these might affect eating behavior. Icons made by Freepik (136, 137), Icongeek26 (138) and catkuro (135) from www.flaticon.com.
The aim of this narrative review is to better understand the physiological and cognitive mechanisms shaping dietary decision-making during pregnancy, and is structured as follows: first, we review how eating behavior changes during pregnancy. Then, we review the current understanding of pregnancy-related hormonal, metabolic, reward-related, and cognitive changes. Further, we review how these mechanisms can impact eating behaviors and food intake in general. Finally, we link these mechanisms to eating behavior during pregnancy. This review, therefore, will highlight an underexplored and important research direction involving the impact of pregnancy-induced changes on the eating behavior of pregnant women. Though the focus of this review is pregnancy, we occasionally draw upon postpartum research in areas in which research in pregnancy is limited and the postpartum findings can help us to better understand the pregnancy transition. Additionally, as described above, maternal nutrition has important consequences for offspring-related outcomes. Findings from this area of research, however, are largely beyond the scope of this review.
Food intake during pregnancy
During pregnancy, total energy consumed increases (37). Specifically, resting metabolic rate can increase by about 29%, whereas energy intake can increase by about 9%, and fat mass can increase by around 4.5 kg when comparing pregnancy to pre-pregnancy (38). Self-reported food-intake of pregnant women seems to shift toward more healthy nutrition, as significant increases are observed in the consumption of fruit and vegetables, and decreases in the consumption of eggs, fried and fast foods, and coffee and tea (37).
Such a shift in nutrition seems to partly reflect the reported motivations to adjust diet in pregnancy, including the desire to optimize health outcomes for the fetus, to optimize nutrient intake, to enhance health, to lessen illness or to help pregnancy-induced nausea, as well as to satisfy craving and for enjoyment (39). Craving, in particular, is an often reported important motivator of food intake during pregnancy, but it is not yet determined what underlies reported increases in cravings during pregnancy (40) [although higher stress and worse sleep quality during pregnancy can exacerbate them (41)]. The most frequently reported changes in diet were in line with direction received by expectant mothers, such as to reduce caffeine consumption, to be careful in terms of food preparation, and to increase intake of both dairy as well as fruits and vegetables (39). Whereas advice to increase the intake of fish, meat, and alternatives are less well-followed, the motivation to reduce intake of harmful foods was more often reported than the motivation to increase intake of foods containing important nutrients, which leaves room for improvement in terms of dietary choice during pregnancy (39).
It is important to keep in mind that the research on dietary intake during pregnancy so far has primarily relied on self-reports (37). Although self-reported motivations for dietary choice can be informative, it is essential to know that these might deviate from actual food intake, and it is also important to understand how pregnancy itself might impact dietary choice. This challenge highlights the importance of understanding objectively observable factors, such as hormonal, reward, and cognitive mechanisms, since these guide eating behaviors.
Hormonal signaling
Major hormonal changes in pregnancy and food intake
Dramatic hormonal changes orchestrate the maternal adaptations necessary to meet the demands of a successful pregnancy (42). Progesterone levels, which normally decrease during the menstrual cycle, remain high and increase in response to the initial pregnancy signaling hormone human chorionic gonadotropin (hCG), and estradiol (an estrogen) levels increase during the second and third trimester (42). In the course of pregnancy, both estrogen and progesterone levels reach levels many fold higher than at any point during the menstrual cycle (43). Other important hormonal changes during pregnancy include those affecting glucocorticoids [which reach levels three times higher than usual by the third trimester (44)] as well as prolactin and growth hormone (42).
Interestingly, hormones such as estrogen, progesterone, prolactin, growth hormone, and cortisol have been reported to impact eating behavior in general. In non-pregnant populations, estrogen generally reduces food intake (45), protecting against binge eating (46), and has been a candidate hormone for treating obesity (47). In non-pregnant rodent models, estradiol administration can reduce weight gain (48, 49) and restore leptin sensitivity [a satiety-signaling hormone (48)], while estrogen depletion can lead to an increase in body fat (50). However, in pregnant populations, these estrogenic effects disappear. For example, thermogenesis of brown adipose (fat) tissue resulting from estradiol administration in non-pregnant rats is absent in pregnant rats (51).
The increased food intake and weight gain occurring alongside large increases in estrogen (35) during pregnancy suggest interactions with other pregnancy-related hormonal changes, such as an estrogen-progesterone interaction. Progesterone has been shown to influence dietary intake indirectly, for example through counteracting the effects of estrogen (35, 52). In non-pregnant populations, higher progesterone levels can increase the risk for maladaptive eating behaviors by reducing the protective effects of estrogen on, for example, binge eating behavior (46). It is the interaction of high levels of both progesterone and estrogen that has been associated with increased emotional eating across the menstrual cycle, rather than the independent effects of either (53).
In pregnant rodent models, prolactin interacts with satiety signaling by contributing to leptin insensitivity to promote food consumption (54), and growth hormone affects plasma glucose regulation and fat gain in pregnancy (35). Excessive cortisol production has been linked to increased fat accumulation in non-pregnant populations, as well as to metabolic disorders such as diabetes (55), but the role of cortisol in eating behavior during pregnancy remains unknown (35). Although many of these pregnancy-related hormones, as well as their complex interactions, point to their potential role on dietary intake and metabolic dynamics, these are largely under-investigated and present an important avenue for further research, especially since they dramatically change during pregnancy.
Metabolic modulations during pregnancy and food intake
Energy homeostasis signaling is affected by the above-described hormonal changes in a manner that ensures adequate energy for fetal development and lactation (35). Here, we focus on the ingestive hormonal changes of leptin and insulin. Leptin is a hormone released from adipose tissue that maintains fat tissue levels by signaling the body’s current energy state and suppressing further food intake (56), and insulin is a hormone involved in facilitating muscle and fat tissue glucose uptake (57).
Pregnant women display both increased serum leptin levels (58) and decreased leptin sensitivity (59). This increase in leptin levels may result from an increase in fat mass during pregnancy (60), additional leptin secretion from the placenta (61), and a slower clearing of leptin from the blood during pregnancy (62). The functional role of increased leptin levels (beyond being a by-product of increased fat stores) is not fully known (54), but it plays a role in fetal development (63). Since leptin signals a reduction in the need for food intake, a decrease in leptin sensitivity (possibly due to the effect of prolactin and growth hormone) counteracts this and allows for the important maternal adaptation of greater food intake and positive energy levels (54).
Like leptin, insulin functioning has been shown to be altered during pregnancy via higher insulin levels on the one hand and decreased insulin sensitivity on the other (64). These changes in insulin functioning are thought to play an important role in ensuring adequate energy supply to the fetus through their role in glucose regulation (42), which is to facilitate glucose uptake in fat and muscle tissue (65). Glucose is transmitted to the fetus passively; for this to be possible, the mother’s glucose levels must remain higher than that of the fetus’, and so the mother’s tissues become less insulin sensitive (66). The fetus must also, however, be protected from excessive glucose exposure following a meal, and so higher levels of insulin are released to protect the fetus from overexposure due to the mother’s insulin-insensitive tissue (54). The changes in insulin during pregnancy have been associated with pregnancy-related weight gain (67). These changes both in insulin and leptin are conducive to creating a positive energy balance, which is thought to be necessary to provide energy to the growing fetus (68). As noted by Grattan and Ladyman (42), the pregnant body is engineered for weight gain, but this increases the risk for excessive gestational weight gain in our current food environment (42).
Effects of hormonal modulations on reward processing and cognition
Receptors for hormones such as estradiol (69), progesterone (70), glucocorticoids (71), leptin (72), and insulin (73) are present throughout the brain, and have been shown to impact both reward processing and cognition (42, 74, 75). For example, the rewarding value of pup stimuli in rodents is mediated by progesterone and estradiol (76, 77). Across species, leptin and insulin have been shown to modulate reward processing via the dopamine system (34). Specifically, both leptin (78) and insulin (79) dampen dopaminergic signaling in (non-pregnant) rodents. Estradiol, progesterone, and glucocorticoid levels have been linked to cognitive functioning in both pregnant (80) and non-pregnant (for example, menopausal) (81) women. For instance, higher estradiol and lower cortisol levels have been associated with worse verbal recall ability in pregnant populations (82). Insulin resistance (83) and leptin resistance (84) have been associated with cognitive impairment (such as mild cognitive impairment) in non-pregnant populations, and insulin resistance in gestational diabetes mellitus may contribute to worse cognitive performance during pregnancy (85). For instance, pregnant women with gestational diabetes mellitus performed worse than non-diabetic pregnant women on the Montreal Cognitive Assessment test, which measures a range of cognitive functions (86). Although more work on these relationships in pregnancy is needed (80), initial evidence points to how these systems are interrelated. In the following sections, we will more closely explore how pregnancy-induced changes in reward processing and cognition may impact eating behavior.
Reward processing
Pregnancy and reward processing
Pregnancy alters reward processing in humans. Hoekzema et al. (87) found a reduction in gray matter volume in the right ventral striatum (a region important to reward processing) from pre- to post-pregnancy. Further, the striatum was more strongly activated when viewing images of one’s own vs. other baby, and the degree of this striatal activation was also associated with the degree of gray matter reduction (87). This has been interpreted as synaptic pruning representing an adaptive specialization, such that mothers’ reward processing areas are altered during pregnancy and show strong responsivity to infant cues (87). Such reward processing adaptations during pregnancy may predict responsiveness in domains other than infant-reward. Indeed, a neural reward signal in response to monetary reward during pregnancy predicts later self-reported bonding between mother and child (88). Research on reward processing in pregnancy is currently quite limited, but presents an exciting avenue for future research on dietary choice.
More research exists regarding the postpartum period, and these findings support our current understanding of the reward processing changes that occur in the transition to parenthood beginning in pregnancy. Gray matter volume change from early to later postpartum is associated with a positive perception of one’s baby (89), and reward processing of infant cues in postpartum women show strong activation in response to own-infant stimuli in reward-related areas including the nucleus accumbens (74). The role of reward processing in parenthood is also supported by the finding that dopamine receptor genes in human mothers have been associated with maternal behavior, for example, orienting to one’s infant (90). Pregnant and postpartum rodent models further highlight this important transition in reward processing, since rodents transition from finding pup cueing aversive to rewarding (75). Postpartum rodent mothers have greater activation in dopamine reward pathways in reaction to the suckling of their offspring than they do in reaction to cocaine exposure (91). Further, they will bar press for contact with pups (92). Additionally, agonists of D1 receptors [which are important in reward-related learning (93)] can facilitate maternal behavior in pregnancy-terminated rats (94). Much of this reward-related research has focused on the postpartum period and reward responses toward offspring, likely because this is most relevant to maternal behavior. However, it is important to remember that a substantial transition in reward-related brain areas occurs already during pregnancy in humans (87), and this carries important implications for eating behavior during pregnancy, as we will see in the following sections.
Reward processing and eating behavior
Reward processing is one of the primary drivers of food consumption (34), and motivates consumption through the rewarding properties of the food rather than due to metabolic demand (95). Reward-related eating has been associated with excess food intake and higher body mass index (96). Reward motivations for eating consist of both liking and wanting motivations (97). Liking can be described as a positive, affective reaction to a food’s palatability, whereas wanting can be described as an incentive motivation to eat (97). Dopamine functioning, such as dopaminergic projections to the striatum, is especially important in wanting (98). For example, using lesions to eliminate dopamine in rats resulted in a lack of motivation to seek out or consume food, even though taste reactions remained the same (99). Further evidence for the role of dopaminergic functioning in eating behavior is that altered dopaminergic functioning has been implicated in obesity (100). Therefore, an event like pregnancy which alters such reward pathways may impact eating behavior through affecting reward-related eating.
Pregnancy, reward processing, and eating behavior
Reward processing is a key mechanism of food intake and seems to be modulated during pregnancy. One of the rare studies investigating this question in humans is the Pregnancy Eating Attributes Study (101). Here, greater reward-related eating during pregnancy was associated with lower scores on the Healthy Eating Index (102). Further analyses showed that reward-related eating was associated with higher calorie consumption after satiety (103). Interestingly, questionnaire-based food reward measures (self-reports), however, did not correlate with excessive gestational weight gain (96). Changes in the processing of food reward in pregnant rodents have been reported, with a recent association being found between changes in dopaminergic signaling and craving-like eating episodes (104). Postpartum rats, alongside an increased preference for pup cueing, show an attenuation in preference for food cueing in a conditioned-place paradigm compared to virgin rats (105), suggesting a reprioritization of reward types.
Since reward processing plays a large role in guiding dietary decision-making, these changes to reward processing during pregnancy should be considered when attempting to promote healthy eating behavior. Future research could employ neuroimaging methods to better understand how changes to the structure of the striatum and to dopaminergic signaling during pregnancy impact reward-related eating.
Cognition
Changes to cognition during pregnancy
Pregnant women experience changes in cognitive functioning. A majority of pregnant women report cognitive impairment, often termed “pregnancy brain” (80). It has often been suggested that this may demonstrate a trade-off with gestation, parturition, and maternal behaviors (82, 106). Women may undergo some “cognitive reorganization” during pregnancy, with functions such as social cognition given precedence, and others, such as memory, given a lower priority (107). It could also be that the energy demands of the fetus may impact upon cognitive function (108). Structurally, there are overall decreases in gray matter volume in pregnancy (109, 110) in areas associated with cognitive functions like memory that may be altered during pregnancy, such as the hippocampus (109).
One domain of cognition impacted by pregnancy is executive functioning. This is a collection of higher order cognitive processes that are utilized when we act in a flexible, goal-oriented manner, and include inhibition, working memory, and flexibility (111). A recent meta-analysis by Davies et al. (112) found that executive functioning (including attention, planning, cognitive flexibility, and inhibition) significantly decreases in pregnant women in the third trimester. This supports a previous review that found that working memory seemed to be particularly impaired in pregnant women (113). Conversely, Fiterman and Raz (114) found that pregnant women have better inhibition in a behavioral task, and these findings were supported by event-related potential (ERP) neural signaling (114). Pregnant women’s response times were also slower (114), and these results suggest that pregnant women may be more cautious in their decision making. This finding is supported by a recent study by Chen et al. (115), in which pregnant women show higher risk aversion in the Columbia Card Sorting Task. Research so far suggests pregnancy may alter executive functioning, but work on this is limited (112). The direction of change remains unclear, but pregnancy may be associated with better inhibition.
A prevalent cognitive impairment reported during pregnancy is worsened memory (116). Overall, pregnancy appears to be associated with a decrease in memory function in both subjective reports and objective measurements. A meta-analysis by Davies et al. (112) found that memory (including working memory, long-term memory retrieval, and recognition) was broadly impacted by pregnancy, with a decrease in overall memory performance. This occurred during the third trimester in correlational studies, and the largest reduction in memory performance in longitudinal studies occurred between the first and second trimester (112). A previous meta-analysis by Henry and Rendell (113) found some measures of memory to be impacted by pregnancy, specifically free recall and delayed free recall, and the executive component of working memory. It is important to note that, while these findings are robust (112), the effects that have been found are small (113) and within normal ranges of cognitive functioning (112).
Despite small effect sizes, these memory impairments might impact the daily lives of pregnant women (117). Pregnant women report subjective memory impairment (118). Further, “naturalistic” measures of memory function, such as remembering to make a phone call or complete a time-logging task in the upcoming week found that pregnant women performed significantly worse than non-pregnant controls, even though they performed equally well on lab-based measures of memory function (113, 119), and this correlated with subjective impressions of memory function (119). Such studies shed important light on the ways in which cognitive impairment may affect the everyday lives of pregnant women, and an important domain that may be affected is eating behavior.
Cognition and eating behavior
Executive functioning has been linked with dietary choice. This relationship depends both on the facet of executive functioning as well as the facet of eating behavior being considered. For example, initiation of healthy eating behaviors, vs. inhibition of unhealthy eating behaviors, may be affected by different processes within executive functioning (120). A study by Allom and Mullan (121) found better inhibition to predict lower unhealthy food intake (saturated fat) while updating in working memory was associated with initiation of healthy food intake (fruits and vegetables). Overall, lower inhibition and greater impulsivity have been associated with a greater risk of becoming overweight or obese (101, 120).
Memory plays an important role in eating behavior [see Higgs and Spetter (122) and Seitz et al. (123) for recent reviews]. Episodic recall is important in food consumption, and this has been demonstrated by the “meal recall” effect. Cueing participants to recall a recent meal is related to less food consumption compared to being cued to recall something else, such as a meal from longer ago (124). This finding has been replicated several times, although it can also be modulated by contextual factors, such as mood (125) or dietary disinhibition (126). Initial memory encoding also guides later food consumption, and an oft-replicated finding is that distracting participants while they eat leads to greater food consumption later (122). For example, watching television while snacking has been associated with more food consumed at a later meal, along with worse recall of the amount that they had snacked (127, 128). Further, better episodic recall is associated with less uncontrolled and emotional eating, and more strategic dieting, as well as a higher likelihood of avoiding fatty food consumption (129). Interestingly, hippocampal volume (a brain area important in memory functioning) has been repeatedly associated with diet-related outcomes, such as being overweight or obese (123). Different cognitive functions play an important role in eating behavior, therefore changes in these cognitive functions during pregnancy will impact eating behavior.
Pregnancy-induced cognitive function changes and links to eating behavior
From the above-described findings, we can conclude that pregnancy-induced changes in cognitive functioning, such as in memory and executive functioning, are very likely to impact dietary choices. Though there is very limited research on executive functioning during pregnancy, some of the available evidence suggests that pregnant women may have slower response times and greater inhibition. Future research could determine if this may positively impact dietary choice, since better inhibition may limit unhealthy food intake. Conversely, worse memory in pregnancy may exacerbate excessive food intake, since memory function has been linked to the regulation of eating behavior. It would also be interesting to better understand the possible relationship between a reduction of hippocampal volume during pregnancy, and the association between reduced hippocampal volume and a higher likelihood of being overweight or obese in non-pregnant populations. A better understanding of these relationships would allow us to work toward optimizing dietary advice and interventions during this critical period, by tailoring such approaches to both counteract cognitive impairment, and harness cognitive changes that could promote healthy eating behavior.
Conclusion and future perspectives
This review has considered pregnancy-induced changes to hormonal, metabolic, reward-related, and cognitive processes guiding dietary choice. As outlined above, some changes, such as metabolic and memory changes, may make it difficult to make healthy dietary decisions during pregnancy, since the pregnant body is adaptively geared for weight gain, and impaired memory may negatively impact eating behaviors. Other changes, such as those related to reward processing and inhibition, may encourage beneficial eating behavior, since pregnant women may be more attuned to offspring-related reward (including the health of the baby), and greater inhibition could encourage healthy food choice. Future research is needed to further investigate the influence of these changing mechanisms on dietary intake.
Despite limitations in terms of research available in this area, we are already able to integrate these findings from different fields to produce tangible suggestions for the improvement of dietary behavior in pregnant women. For example, we have seen that memory impairment negatively impacts healthy food choices, and that memory is impaired in pregnancy. Therefore, practices aimed at improving memory of food consumption, such as food journaling (122), may be especially helpful during this time period. Further, since reward responsiveness to offspring develops during pregnancy, it may be helpful to increase education regarding the impact of nutrition on offspring outcomes. Currently, nutrition education for pregnant women is inadequate (130). If it were improved, pregnant women would be more aware of health information which may increase the reward value of healthy food once it has been explicitly connected to offspring wellbeing.
Effective promotion of healthy nutrition during pregnancy could be implemented both through healthcare providers as well as via digital devices. Healthcare providers such as physicians, midwives, and counselors could educate pregnant women on the reward-related and cognitive processes guiding dietary choice, and implement practices that target the pregnancy-induced changes in such processes. A more cost-efficient strategy could involve current technology such as mHealth (the use of mobile devices in healthcare) that have wide availability (131). Current mobile health interventions for pregnancy have shown only limited success (132), suggesting room for improvement. Mobile interventions for pregnancy could be improved by integrating practices targeted at the pregnancy-induced changes discussed in this review. For example, smartphone interventions can effectively improve memory function in the context of food consumption in non-pregnant populations, through, for example, recording meals, and this can lead to increased reported awareness of food consumption and weight loss (133). Another mobile intervention altered reward value of food through increasing awareness with mindfulness practices in a non-pregnant population (134). Such a practice could be tailored to the changes women experience in reward processing in pregnancy by promoting awareness of the health associations of their dietary choices for their offspring, as this could alter food reward value for pregnant women in a manner encouraging healthy food choices. Understanding pregnancy-induced changes to hormonal, metabolic, reward-related, and cognitive processes would provide evidence for which tasks, training, and educational materials would be most effective in promoting healthy eating behavior in pregnant women, both from healthcare providers and from digital sources.
A limitation of this review is the limited research in this domain, and therefore our use of postpartum studies. However, from what we know of the dramatic hormonal and anatomical changes occurring during pregnancy, we can assume that postpartum findings have something valuable to offer in helping us better understand changes in pregnancy. Another limitation is that much of the research currently available employs rodent models. While these are helpful in gaining an understanding of how we might expect pregnancy to affect women, it is important to verify such findings in humans.
In conclusion, a better understanding of pregnancy-induced changes to hormonal, metabolic, reward-related, and cognitive changes would provide actionable suggestions for improving important health outcomes for pregnant women. More research in this domain is essential because dietary choice during pregnancy affects both short- and long-term outcomes for mother and child, such as cardiovascular and metabolic health. The health consequences of dietary choice during this period extend throughout the lifetime, carrying significant personal and financial implications, making this an important research area to pursue.
Author contributions
TW and SP: conceptualization, investigation, methodology, formal analysis, writing, and project administration. TW: visualization. SP: supervision and funding acquisition. Both authors have read and agreed to the published version of the manuscript.
Funding
This research was funded by the German Ministry of Education and Research (BMBF), the State of Brandenburg, and the German Center for Diabetes Research (DZD, 82DZD03D03).
Acknowledgments
We thank Annabel Losecaat Vermeer and Eva Froehlich for their feedback on the manuscript.
Conflict of interest
The authors declare that the research was conducted in the absence of any commercial or financial relationships that could be construed as a potential conflict of interest.
Publisher’s note
All claims expressed in this article are solely those of the authors and do not necessarily represent those of their affiliated organizations, or those of the publisher, the editors and the reviewers. Any product that may be evaluated in this article, or claim that may be made by its manufacturer, is not guaranteed or endorsed by the publisher.
References
1. Thangaratinam S, Rogozinska E, Jolly K, Glinkowski S, Roseboom T, Tomlinson JW, et al. Effects of interventions in pregnancy on maternal weight and obstetric outcomes: meta-analysis of randomised evidence. BMJ. (2012) 344:e2088. doi: 10.1136/bmj.e2088
2. Mijatovic-Vukas J, Capling L, Cheng S, Stamatakis E, Louie J, Cheung N, et al. Associations of diet and physical activity with risk for gestational diabetes mellitus: a systematic review and meta-analysis. Nutrients. (2018) 10:698. doi: 10.3390/nu10060698
3. Buchanan TA, Xiang A, Kjos SL, Watanabe R. What is gestational diabetes? Diab Care. (2007) 30(Supplement. 2):S105–11. doi: 10.2337/dc07-s201
4. Xu H, Shatenstein B, Luo Z-C, Wei S, Fraser W. Role of nutrition in the risk of preeclampsia. Nutr Rev. (2009) 67:639–57. doi: 10.1111/j.1753-4887.2009.00249.x
5. Ikem E, Halldorsson TI, Birgisdóttir BE, Rasmussen MA, Olsen SF, Maslova E. Dietary patterns and the risk of pregnancy-associated hypertension in the danish national birth cohort: a prospective longitudinal study. BJOG. (2019) 126:663–73. doi: 10.1111/1471-0528.15593
6. Roberts JM, Gammill HS. Preeclampsia: recent insights. Hypertension. (2005) 46:1243–9. doi: 10.1161/01.HYP.0000188408.49896.c5
7. Xiong X, Saunders LD, Wang FL, Demianczuk NN. Gestational diabetes mellitus: prevalence, risk factors, maternal and infant outcomes. Int J Gynecol Obstetr. (2001) 75:221–8. doi: 10.1016/S0020-7292(01)00496-9
8. Ramachenderan J, Bradford J, Mclean M. Maternal obesity and pregnancy complications: a review. Aus New Zealand J Obstetr Gynaecol. (2008) 48:228–35. doi: 10.1111/j.1479-828X.2008.00860.x
9. Edwards L. Pregnancy complications and birth outcomes in obese and normal-weight women: effects of gestational weight change. Obstetr Gynecol. (1996) 87:389–94. doi: 10.1016/0029-7844(95)00446-7
10. Lippert RN, Hess S, Klemm P, Burgeno LM, Jahans-Price T, Walton ME, et al. Maternal high-fat diet during lactation reprograms the dopaminergic circuitry in mice. J Clin Invest. (2020) 130:3761–76. doi: 10.1172/JCI134412
11. Vogt MC, Paeger L, Hess S, Steculorum SM, Awazawa M, Hampel B, et al. Neonatal insulin action impairs hypothalamic neurocircuit formation in response to maternal high-fat feeding. Cell. (2014) 156:495–509. doi: 10.1016/j.cell.2014.01.008
12. Cortés-Álvarez NY, Vuelvas-Olmos CR, Pinto-González MF, Guzmán-Muñiz J, Gonzalez-Perez O, Moy-López NA. A high-fat diet during pregnancy impairs memory acquisition and increases leptin receptor expression in the hippocampus of rat offspring. Nutr Neurosci. (2022) 25:146–58. doi: 10.1080/1028415X.2020.1728473
13. Mirpuri J. Evidence for maternal diet-mediated effects on the offspring microbiome and immunity: implications for public health initiatives. Pediatr Res. (2021) 89:301–6. doi: 10.1038/s41390-020-01121-x
14. Hsu C, Tain Y. The good, the bad, and the ugly of pregnancy nutrients and developmental programming of adult disease. Nutrients. (2019) 11:894. doi: 10.3390/nu11040894
15. Vafa M, Mahmoodianfard S. Long-Term effects of maternal nutrition and childhood growth on later health. J Womens Health Issues Care. (2016) 5:3. doi: 10.4172/2325-9795.1000230
16. Musselman JRB, Jurek AM, Johnson KJ, Linabery AM, Robison LL, Shu X-O, et al. Maternal dietary patterns during early pregnancy and the odds of childhood germ cell tumors: a children’s oncology group study. Am J Epidemiol. (2011) 173:282–91. doi: 10.1093/aje/kwq365
17. Parr CL, Magnus MC, Karlstad Ø, Holvik K, Lund-Blix NA, Haugen M, et al. Vitamin A and D intake in pregnancy, infant supplementation, and asthma development: the norwegian mother and child cohort. Am J Clin Nutr. (2018) 107:789–98. doi: 10.1093/ajcn/nqy016
18. de Lauzon-Guillain CM, Marques C, Kadawathagedara M, Bernard JY, Lioret S, Charles MA. Maternal diet during pregnancy and child neurodevelopment up to age 3.5 years: the nationwide étude longitudinale française depuis l’enfance (ELFE) birth cohort. Am J Clin Nutr. (2022) 116:1101–11. doi: 10.1093/ajcn/nqac206
19. Mahmassani HA, Switkowski KM, Scott TM, Johnson EJ, Rifas-Shiman SL, Oken E, et al. Maternal diet quality during pregnancy and child cognition and behavior in a US cohort. Am J Clin Nutr. (2022) 115:128–41. doi: 10.1093/ajcn/nqab325
20. Puig-Vallverdú J, Romaguera D, Fernández-Barrés S, Gignac F, Ibarluzea J, Santa-Maria L, et al. The association between maternal ultra-processed food consumption during pregnancy and child neuropsychological development: a population-based birth cohort study. Clin Nutr. (2022) 41:2275–83. doi: 10.1016/j.clnu.2022.08.005
21. Rivera HM, Kievit P, Kirigiti MA, Bauman LA, Baquero K, Blundell P, et al. Maternal high-fat diet and obesity impact palatable food intake and dopamine signaling in nonhuman primate offspring. Obesity (Silver Spring, Md). (2015) 23:2157–64. doi: 10.1002/oby.21306
22. Thorburn AN. Evidence that asthma is a developmental origin disease influenced by maternal diet and bacterial metabolites. Nat Commun. (2015) 6:7320. doi: 10.1038/ncomms8320
23. Sanidad KZ, Zeng MY. Neonatal gut microbiome and immunity. Curr Opin Microbiol. (2020) 56:30–7. doi: 10.1016/j.mib.2020.05.011
24. Bellamy L, Casas J-P, Hingorani AD, Williams D. Type 2 diabetes mellitus after gestational diabetes: a systematic review and meta-analysis. Lancet. (2009) 373:1773–9.
25. Vrachnis N, Augoulea A, Iliodromiti Z, Lambrinoudaki I, Sifakis S, Creatsas G. Previous gestational diabetes mellitus and markers of cardiovascular risk. Int J Endocrinol. (2012) 2012:458610. doi: 10.1155/2012/458610
26. Williams D. Long-Term complications of preeclampsia. Sem Nephrol. (2011) 31:111–22. doi: 10.1016/j.semnephrol.2010.10.010
27. McDonald SD, Malinowski A, Zhou Q, Yusuf S, Devereaux PJ. Cardiovascular sequelae of preeclampsia/eclampsia: a systematic review and meta-analyses. Am Heart J. (2008) 156:918–30. doi: 10.1016/j.ahj.2008.06.042
28. Vikse BE, Leivestad T, Iversen BM. Preeclampsia and the risk of end-stage renal disease. N Engl J Med. (2008) 359:800–9.
29. Linné Y, Dye L, Barkeling B, Rössner S. Long-term weight development in women: a 15- year follow-up of the effects of pregnancy. Obesity Res. (2012) 12:1166–78. doi: 10.1038/oby.2004.146
30. Rockliffe L, Peters S, Heazell AEP, Smith DM. Understanding pregnancy as a teachable moment for behaviour change: a comparison of the COM-B and teachable moments models. Health Psychol Behav Med. (2022) 10:41–59. doi: 10.1080/21642850.2021.2014851
31. Phelan S. Pregnancy: a “teachable moment” for weight control and obesity prevention. Am J Obstetr Gynecol. (2010) 202:135.e1–8. doi: 10.1016/j.ajog.2009.06.008
32. Skouteris H, Hartley-Clark L, McCabe M, Milgrom J, Kent B, Herring SJ, et al. Preventing excessive gestational weight gain: a systematic review of interventions: preventing excessive gestational weight gain. Obesity Rev. (2010) 11:757–68. doi: 10.1111/j.1467-789X.2010.00806.x
33. Whitaker KM, Becker C, Healy H, Wilcox S, Liu J. Women’s report of health care provider advice and gestational weight gain: a systematic review. J Women’s Health. (2021) 30:73–89. doi: 10.1089/jwh.2019.8223
34. Higgs S, Spetter MS, Thomas JM, Rotshtein P, Lee M, Hallschmid M, et al. Interactions between metabolic, reward and cognitive processes in appetite control: implications for novel weight management therapies. J Psychopharmacol. (2017) 31:1460–74. doi: 10.1177/0269881117736917
35. Clarke GS, Gatford KL, Young RL, Grattan DR, Ladyman SR, Page AJ. Maternal adaptations to food intake across pregnancy: central and peripheral mechanisms. Obesity. (2021) 29:1813–24. doi: 10.1002/oby.23224
36. Cárdenas EF, Kujawa A, Humphreys KL. Neurobiological changes during the peripartum period: implications for health and behavior. Soc Cogn Affect Neurosci. (2020) 15:1097–110. doi: 10.1093/scan/nsz091
37. Hillier SE, Olander EK. Women’s dietary changes before and during pregnancy: a systematic review. Midwifery. (2017) 49:19–31. doi: 10.1016/j.midw.2017.01.014
38. Kopp-Hoolihan LE, van Loan MD, Wong WW, King JC. Longitudinal assessment of energy balance in well-nourished, pregnant women. Am J Clin Nutr. (1999) 69:697–704. doi: 10.1093/ajcn/69.4.697
39. Forbes L, Graham J, Berglund C, Bell R. Dietary change during pregnancy and women’s reasons for change. Nutrients. (2018) 10:1032. doi: 10.3390/nu10081032
40. Orloff NC, Hormes JM. Pickles and ice cream! Food cravings in pregnancy: hypotheses, preliminary evidence, and directions for future research. Front Psychol. (2014) 5:1076. doi: 10.3389/fpsyg.2014.01076
41. Betts GM, Lipsky LM, Temmen CD, Siega-Riz AM, Faith MS, Nansel TR. Poorer mental health and sleep quality are associated with greater self-reported reward-related eating during pregnancy and postpartum: an observational cohort study. Int J Behav Nutr Phys Act. (2021) 18:58. doi: 10.1186/s12966-021-01124-9
42. Grattan DR, Ladyman SR. Neurophysiological and cognitive changes in pregnancy. Handb Clin Neurol. (2020) 171:25–55. doi: 10.1016/B978-0-444-64239-4.00002-3
43. Altemus M. Neuroendocrine networks and functionality. Med Clin North Am. (2019) 103:601–12. doi: 10.1016/j.mcna.2019.03.003
44. Duthie L, Reynolds RM. Changes in the maternal hypothalamic-pituitary-adrenal axis in pregnancy and postpartum: influences on maternal and fetal outcomes. Neuroendocrinology. (2013) 98:106–15. doi: 10.1159/000354702
45. Hirschberg AL. Sex hormones, appetite and eating behaviour in women. Maturitas. (2012) 71:248–56. doi: 10.1016/j.maturitas.2011.12.016
46. Mikhail ME, Culbert KM, Sisk CL, Klump KL. Gonadal hormone contributions to individual differences in eating disorder risk. Curr Opin Psychiatry. (2019) 32:484–90. doi: 10.1097/YCO.0000000000000543
47. Huang K-P, Raybould HE. Estrogen and gut satiety hormones in vagus-hindbrain axis. Peptides. (2020) 133:170389. doi: 10.1016/j.peptides.2020.170389
48. Litwak SA, Wilson JL, Chen W, Garcia-Rudaz C, Khaksari M, Cowley MA, et al. Estradiol prevents fat accumulation and overcomes leptin resistance in female high-fat diet mice. Endocrinology. (2014) 155:4447–60. doi: 10.1210/en.2014-1342
49. Roesch D. Effects of selective estrogen receptor agonists on food intake and body weight gain in rats. Physiol Behav. (2006) 87:39–44. doi: 10.1016/j.physbeh.2005.08.035
50. Rogers NH, Perfield JW, Strissel KK, Obin MS, Greenberg AS. Reduced energy expenditure and increased inflammation are early events in the development of ovariectomy induced obesity. Endocrinology. (2009) 150:2161–8. doi: 10.1210/en.2008-1405
51. Martínez de Morentin PB, Lage R, González-García I, Ruíz-Pino F, Martins L, et al. Pregnancy induces resistance to the anorectic effect of hypothalamic Malonyl-CoA and the thermogenic effect of hypothalamic AMPK inhibition in female rats. Endocrinology. (2015) 156:947–60. doi: 10.1210/en.2014-1611
52. Wade GN. Some effects of ovarian hormones on food intake and body weight in female rats. J Comp Physiol Psychol. (1975) 88:183–93. doi: 10.1037/h0076186
53. Klump KL, Keel PK, Racine SE, Burt SA, Neale M, Sisk CL, et al. The interactive effects of estrogen and progesterone on changes in emotional eating across the menstrual cycle. J Abnormal Psychol. (2013) 122:131–7. doi: 10.1037/a0029524
54. Khant Aung Z, Grattan DR, Ladyman SR. Pregnancy-induced adaptation of central sensitivity to leptin and insulin. Mol Cell Endocrinol. (2020) 516:110933. doi: 10.1016/j.mce.2020.110933
55. Geiker NRW, Astrup A, Hjorth MF, Sjödin A, Pijls L, Markus CR. Does stress influence sleep patterns, food intake, weight gain, abdominal obesity and weight loss interventions and vice versa?: effect of stress on food intake. Obes Rev. (2018) 19:81–97. doi: 10.1111/obr.12603
56. Myers MG, Cowley MA, Münzberg H. Mechanisms of leptin action and leptin resistance. Ann Rev Physiol. (2008) 70:537–56.
58. Yamada M, Matsuzaki T, Iwasa T, Shimizu F, Tanaka N, Ogata R, et al. Serum leptin levels in women throughout life: relationship to body mass index and serum estradiol levels. Jpn J Reprod Endocrinol. (2003) 8:55–60.
59. Tessier DR, Ferraro ZM, Gruslin A. Role of leptin in pregnancy: consequences of maternal obesity. Placenta. (2013) 34:205–11. doi: 10.1016/j.placenta.2012.11.035
60. García MD, Casanueva FF, Diéguez C, Señarís RM. Gestational profile of leptin messenger ribonucleic acid (mRNA) content in the placenta and adipose tissue in the rat, and regulation of the mRNA levels of the leptin receptor subtypes in the hypothalamus during pregnancy and lactation1. Biol Reproduct. (2000) 62:698–703. doi: 10.1095/biolreprod62.3.698
61. Sagawa N, Yura S, Itoh H, Kakui K, Takemura M, Nuamah MA, et al. Possible role of placental leptin in pregnancy. Endocrine. (2002) 19:65–72.
62. Gavrilova O, Barr V, Marcus-Samuels B, Reitman M. Hyperleptinemia of pregnancy associated with the appearance of a circulating form of the leptin receptor. J Biol Chem. (1997) 272:30546–51. doi: 10.1074/jbc.272.48.30546
63. Briffa JF, McAinch AJ, Romano T, Wlodek ME, Hryciw DH. Leptin in pregnancy and development: a contributor to adulthood disease? Am J Physiology-Endocrinol Metab. (2015) 308:E335–50. doi: 10.1152/ajpendo.00312.2014
64. Vejrazkova D, Vcelak J, Vankova M, Lukasova P, Bradnova O, Halkova T, et al. Steroids and insulin resistance in pregnancy. J Steroid Biochem Mol Biol. (2014) 139:122–9. doi: 10.1016/j.jsbmb.2012.11.007
65. Saltiel AR, Kahn CR. Insulin signalling and the regulation of glucose and lipid metabolism. Nature. (2001) 414:799–806. doi: 10.1038/414799a
66. Baeyens L, Hindi S, Sorenson RL, German MS. β-Cell adaptation in pregnancy. Diab Obes Metab. (2016) 18:63–70. doi: 10.1111/dom.12716
67. Scholl TO, Chen X. Insulin and the “Thrifty” woman: the influence of insulin during pregnancy on gestational weight gain and postpartum weight retention. Maternal Child Health J. (2002) 6:255–61. doi: 10.1023/a:1021162117177
68. Xu Y, López M. Central regulation of energy metabolism by estrogens. Mol Metab. (2018) 15:104–15. doi: 10.1016/j.molmet.2018.05.012
69. Merchenthaler I, Lane MV, Numan S, Dellovade TL. Distribution of estrogen receptor ? and ? in the mouse central nervous system: in vivo autoradiographic and immunocytochemical analyses. J Comp Neurol. (2004) 473:270–91. doi: 10.1002/cne.20128
70. Brinton RD, Thompson RF, Foy MR, Baudry M, Wang J, Finch CE, et al. Progesterone receptors: form and function in brain. Front Neuroendocrinol. (2008) 29:313–39. doi: 10.1016/j.yfrne.2008.02.001
71. Joëls M. Corticosteroids and the brain. J Endocrinol. (2018) 238:R121–30. doi: 10.1530/JOE-18-0226
72. Morrison CD. Leptin signaling in brain: a link between nutrition and cognition? Biochim Biophys Acta (BBA) - Mol Basis Dis. (2009) 1792:401–8. doi: 10.1016/j.bbadis.2008.12.004
73. Schulingkamp RJ, Pagano TC, Hung D, Raffa RB. Insulin receptors and insulin action in the brain: review and clinical implications. Neurosci Biobehav Rev. (2000) 24:855–72. doi: 10.1016/S0149-7634(00)00040-3
74. Barba-Müller E, Craddock S, Carmona S, Hoekzema E. Brain plasticity in pregnancy and the postpartum period: links to maternal caregiving and mental health. Arch Women’s Mental Health. (2019) 22:289–99. doi: 10.1007/s00737-018-0889-z
75. Rincón-Cortés M, Grace AA. Adaptations in reward-related behaviors and mesolimbic dopamine function during motherhood and the postpartum period. Front Neuroendocrinol. (2020) 57:100839. doi: 10.1016/j.yfrne.2020.100839
76. Bridges RS. A quantitative analysis of the roles of dosage, sequence, and duration of estradiol and progesterone exposure in the regulation of maternal behavior in the rat. Endocrinology. (1984) 114:930–40. doi: 10.1210/endo-114-3-930
77. Fleming AS, Cheung U, Myhal N, Kessler Z. Effects of maternal hormones on ‘timidity’ and attraction to pup-related odors in female rats. Physiol Behav. (1989) 46:449–53. doi: 10.1016/0031-9384(89)90019-X
78. Domingos AI, Vaynshteyn J, Voss HU, Ren X, Gradinaru V, Zang F, et al. Leptin regulates the reward value of nutrient. Nat Neurosci. (2011) 14:1562–8. doi: 10.1038/nn.2977
79. Mebel DM, Wong JC, Dong YJ, Borgland SL. Insulin in the ventral tegmental area reduces hedonic feeding and suppresses dopamine concentration via increased reuptake: insulin attenuates somatodendritic dopamine. Eur J Neurosci. (2012) 36:2336–46. doi: 10.1111/j.1460-9568.2012.08168.x
80. Brown E, Schaffir J. “Pregnancy brain”: a review of cognitive changes in pregnancy and postpartum. Obstetr Gynecol Survey. (2019) 74:178–85. doi: 10.1097/OGX.0000000000000655
81. Beltz AM, Moser JS. Ovarian hormones: a long overlooked but critical contributor to cognitive brain structures and function. Ann N Y Acad Sci. (2020) 1464:156–80. doi: 10.1111/nyas.14255
82. Glynn LM. Giving birth to a new brain: hormone exposures of pregnancy influence human memory. Psychoneuroendocrinology. (2010) 35:1148–55. doi: 10.1016/j.psyneuen.2010.01.015
83. Ma L, Wang J, Li Y. Insulin resistance and cognitive dysfunction. Clin Chim Acta. (2015) 444:18–23. doi: 10.1016/j.cca.2015.01.027
84. Witte AV, Köbe T, Graunke A, Schuchardt JP, Hahn A, Tesky VA, et al. Impact of leptin on memory function and hippocampal structure in mild cognitive impairment: leptin, memory, hippocampal structure in MCI. Hum Brain Mapp. (2016) 37:4539–49. doi: 10.1002/hbm.23327
85. John C, Mohamed Yusof N, Abdul Aziz S, Mohd Fauzi F. Maternal cognitive impairment associated with gestational diabetes mellitus—a review of potential contributing mechanisms. Int J Mol Sci. (2018) 19:3894. doi: 10.3390/ijms19123894
86. Keskin F, Ozyazar M, Pala A, Elmali A, Yilmaz B, Uygunoglu U, et al. Evaluation of cognitive functions in gestational diabetes mellitus. Exp Clin Endocrinol Diab. (2015) 123:246–51. doi: 10.1055/s-0034-1395634
87. Hoekzema E, Tamnes CK, Berns P, Barba-Müller E, Pozzobon C, Picado M, et al. Becoming a mother entails anatomical changes in the ventral striatum of the human brain that facilitate its responsiveness to offspring cues. Psychoneuroendocrinology. (2020) 112:104507. doi: 10.1016/j.psyneuen.2019.104507
88. Mulligan EM, Lowe M, Flynn H, Hajcak G. The rewards of motherhood: neural response to reward in pregnancy prospectively predicts maternal bonding with the infant in the postpartum period. Biol Psychol. (2021) 163:108148. doi: 10.1016/j.biopsycho.2021.108148
89. Kim P, Leckman JF, Mayes LC, Feldman R, Wang X, Swain JE. The plasticity of human maternal brain: longitudinal changes in brain anatomy during the early postpartum period. Behav Neurosci. (2010) 124:695–700. doi: 10.1037/a0020884
90. Mileva-Seitz V, Fleming AS, Meaney MJ, Mastroianni A, Sinnwell JP, Steiner M, et al. Dopamine receptors D1 and D2 are related to observed maternal behavior. Genes Brain Behav. (2012) 11:684–94. doi: 10.1111/j.1601-183X.2012.00804.x
91. Ferris CF. Pup suckling is more rewarding than cocaine: evidence from functional magnetic resonance imaging and three-dimensional computational analysis. J Neurosci. (2005) 25:149–56. doi: 10.1523/JNEUROSCI.3156-04.2005
92. Lee A, Clancy S, Fleming AS. Mother rats bar-press for pups: effects of lesions of the mpoa and limbic sites on maternal behavior and operant responding for pup-reinforcement. Behav Brain Res. (1999) 100:15–31. doi: 10.1016/S0166-4328(98)00109-0
93. Beninger R. Dopamine D1-like receptors and reward-related incentive learning. Neurosci Biobehav Rev. (1998) 22:335–45. doi: 10.1016/S0149-7634(97)00019-5
94. Stolzenberg DS, McKenna JB, Keough S, Hancock R, Numan MJ, Numan M. Dopamine D1 receptor stimulation of the nucleus accumbens or the medial preoptic area promotes the onset of maternal behavior in pregnancy-terminated rats. Behav Neurosci. (2007) 121:907–19. doi: 10.1037/0735-7044.121.5.907
95. Ziauddeen H, Alonso-Alonso M, Hill JO, Kelley M, Khan NA. Obesity and the neurocognitive basis of food reward and the control of intake. Adv Nutr. (2015) 6:474–86. doi: 10.3945/an.115.008268
96. Nansel TR, Lipsky LM, Burger K, Faith M, Nicholson W, Stuebe A, et al. Reward-related eating, self-regulation, and weight change in pregnancy and postpartum: the pregnancy eating attributes study (PEAS). Int J Obes. (2020) 44:2444–54. doi: 10.1038/s41366-020-00685-2
97. Castro DC, Berridge KC. Advances in the neurobiological bases for food ‘liking’ versus ‘wanting.’. Physiol Behav. (2014) 136:22–30. doi: 10.1016/j.physbeh.2014.05.022
98. Berridge KC, Robinson TE. Liking, wanting, and the incentive-sensitization theory of addiction. Am Psychol. (2016) 71:670–9. doi: 10.1037/amp0000059
99. Berridge KC, Venier IL, Robinson TE. Taste reactivity analysis of 6-hydroxydopamine-induced aphagia: implications for arousal and anhedonia hypotheses of dopamine function. Behav Neurosci. (1989) 103:36–45. doi: 10.1037//0735-7044.103.1.36
100. Stice E, Spoor S, Ng J, Zald DH. Relation of obesity to consummatory and anticipatory food reward. Physiol Behav. (2009) 97:551–60. doi: 10.1016/j.physbeh.2009.03.020
101. Nansel TR, Lipsky LM, Siega-Riz AM, Burger K, Faith M, Liu A. Pregnancy eating attributes study (PEAS): a cohort study examining behavioral and environmental influences on diet and weight change in pregnancy and postpartum. BMC Nutr. (2016) 2:45. doi: 10.1186/s40795-016-0083-5
102. Nansel TR, Lipsky LM, Faith M, Liu A, Siega-Riz AM. The accelerator, the brake, and the terrain: associations of reward-related eating, self-control, and the home food environment with diet quality assessed by 24-hour recalls during pregnancy and postpartum in the pregnancy eating attributes study (PEAS) cohort. Int J Behav Nutr Phys Act. (2020) 17:149. doi: 10.21203/rs.3.rs-22914/v2
103. Lipsky LM, Burger KS, Faith MS, Siega-Riz AM, Liu A, Shearrer GE, et al. Pregnant women consume a similar proportion of highly vs minimally processed foods in the absence of hunger, leading to large differences in energy intake. J Acad Nutr Dietet. (2021) 121:446–57. doi: 10.1016/j.jand.2020.09.036
104. Haddad-Tóvolli R, Ramírez S, Muñoz-Moreno E, Milà-Guasch M, Miquel-Rio L, Pozo M, et al. Food craving-like episodes during pregnancy are mediated by accumbal dopaminergic circuits. Nat Metab. (2022) 4:424–34. doi: 10.1038/s42255-022-00557-1
105. Fleming AS, Korsmit M, Deller M. Rat pups are potent reinforcers to the maternal animal: effects of experience, parity, hormones, and dopamine function. Psychobiology. (1994) 22:44–53. doi: 10.3758/BF03327079
106. Pawluski JL, Lambert KG, Kinsley CH. Neuroplasticity in the maternal hippocampus: relation to cognition and effects of repeated stress. Hormones Behav. (2016) 77:86–97. doi: 10.1016/j.yhbeh.2015.06.004
107. Barda G, Mizrachi Y, Borokchovich I, Yair L, Kertesz DP, Dabby R. The effect of pregnancy on maternal cognition. Sci Rep. (2021) 11:12187. doi: 10.1038/s41598-021-91504-9
108. Ziomkiewicz A, Wichary S, Jasienska G. Cognitive costs of reproduction: life-history trade-offs explain cognitive decline during pregnancy in women. Biol Rev. (2019) 94:1105–15. doi: 10.1111/brv.12494
109. Hoekzema E, Barba-Müller E, Pozzobon C, Picado M, Lucco F, García-García D, et al. Pregnancy leads to long-lasting changes in human brain structure. Nat Neurosci. (2017) 20:287–96. doi: 10.1038/nn.4458
110. Oatridge A, Holdcroft A, Saeed N, Hajnal JV, Puri BK, Fusi L, et al. Change in brain size during and after pregnancy: study in healthy women and women with preeclampsia. AJNR Am J Neuroradiol. (2002) 23:19–26.
112. Davies SJ, Lum JA, Skouteris H, Byrne LK, Hayden MJ. Cognitive impairment during pregnancy: a meta-analysis. Med J Australia. (2018) 208:35–40. doi: 10.5694/mja17.00131
113. Henry JD, Rendell PG. A review of the impact of pregnancy on memory function. J Clin Exp Neuropsychol. (2007) 29:793–803. doi: 10.1080/13803390701612209
114. Fiterman O, Raz S. Cognitive, neural and endocrine functioning during late pregnancy: an event-related potentials study. Hormones Behav. (2019) 116:104575. doi: 10.1016/j.yhbeh.2019.104575
115. Chen J, Guo Y, Liao Z, Xia W, She S. Does pregnancy make women more cautious and calm? the impact of pregnancy on risk decision-making. Judgment Decision Making. (2020) 15:807–22.
116. Brindle PM, Brown MW, Brown J, Griffith HB, Turner GM. Objective and subjective memory impairment in pregnancy. Psychol Med. (1991) 21:647–53. doi: 10.1017/S0033291700022285
117. Brett M. Motherhood and memory: a review. Psychoneuroendocrinology. (2001) 26:339–62. doi: 10.1016/S0306-4530(01)00003-8
118. Pownall M, Hutter RRC, Rockliffe L, Conner M. Memory and mood changes in pregnancy: a qualitative content analysis of women’s first-hand accounts. J Reproduct Infant Psychol. (2022). [Epub ahead of print]. doi: 10.1080/02646838.2022.2052827
119. Cuttler C, Graf P, Pawluski JL, Galea LAM. Everyday life memory deficits in pregnant women. Can J Exp Psychology/Revue Canad Psychol Exp. (2011) 65:27–37. doi: 10.1037/a0022844
120. Dohle S, Diel K, Hofmann W. Executive functions and the self-regulation of eating behavior: a review. Appetite. (2018) 124:4–9. doi: 10.1016/j.appet.2017.05.041
121. Allom V, Mullan B. Individual differences in executive function predict distinct eating behaviours. Appetite. (2014) 80:123–30. doi: 10.1016/j.appet.2014.05.007
122. Higgs S, Spetter MS. Cognitive control of eating: the role of memory in appetite and weight gain. Curr Obes Rep. (2018) 7:50–9. doi: 10.1007/s13679-018-0296-9
123. Seitz BM, Tomiyama AJ, Blaisdell AP. Eating behavior as a new frontier in memory research. Neurosci Biobehav Rev. (2021) 127:795–807. doi: 10.1016/j.neubiorev.2021.05.024
124. Szypula J, Ahern A, Cheke L. The role of memory ability, depth and mode of recall in the impact of memory on later consumption. Appetite. (2020) 149:104628. doi: 10.1016/j.appet.2020.104628
125. Collins R, Stafford LD. Feeling happy and thinking about food. counteractive effects of mood and memory on food consumption. Appetite. (2015) 84:107–12. doi: 10.1016/j.appet.2014.09.021
126. Higgs S, Williamson AC, Attwood AS. Recall of recent lunch and its effect on subsequent snack intake. Physiol Behav. (2008) 94:454–62. doi: 10.1016/j.physbeh.2008.02.011
127. Higgs S, Woodward M. Television watching during lunch increases afternoon snack intake of young women. Appetite. (2009) 52:39–43. doi: 10.1016/j.appet.2008.07.007
128. Mittal D, Stevenson RJ, Oaten MJ, Miller LA. Snacking while watching TV impairs food recall and promotes food intake on a later TV free test meal. Appl Cogn Psychol. (2011) 25:871–7. doi: 10.1002/acp.1760
129. Martin AA, Davidson TL, McCrory MA. Deficits in episodic memory are related to uncontrolled eating in a sample of healthy adults. Appetite. (2018) 124:33–42. doi: 10.1016/j.appet.2017.05.011
130. Lucas C, Charlton KE, Yeatman H. Nutrition advice during pregnancy: do women receive it and can health professionals provide it? Maternal Child Health J. (2014) 18:2465–78. doi: 10.1007/s10995-014-1485-0
131. Rowland SP, Fitzgerald JE, Holme T, Powell J, McGregor A. What is the clinical value of mHealth for patients? NPJ Digital Med. (2020) 3:4. doi: 10.1038/s41746-019-0206-x
132. Sandborg J, Söderström E, Henriksson P, Bendtsen M, Henström M, Leppänen MH, et al. Effectiveness of a smartphone app to promote healthy weight gain, diet, and physical activity during pregnancy (HealthyMoms): randomized controlled trial. JMIR Mhealth Uhealth. (2021) 9:e26091. doi: 10.2196/26091
133. Robinson E, Higgs S, Daley AJ, Jolly K, Lycett D, Lewis A, et al. Development and feasibility testing of a smart phone based attentive eating intervention. BMC Public Health. (2013) 13:639. doi: 10.1186/1471-2458-13-639
134. Taylor VA, Moseley I, Sun S, Smith R, Roy A, Ludwig VU, et al. Awareness drives changes in reward value which predict eating behavior change: probing reinforcement learning using experience sampling from mobile mindfulness training for maladaptive eating. J Behav Addict. (2021) 10:482–97. doi: 10.1556/2006.2021.00020
135. catkuro. Thinking. (n.d.). Available online at: https://www.flaticon.com/free-icon/thinking_1241581?term=thinking&page=1&position=35&origin=style&related_id=1241581 (accessed July 17, 2022).
136. Freepik. Lunch Box. (n.d.). Available online at: https://www.flaticon.com/free-icon/lunchbox_1205755?term=lunch&page=1&position=20&page=1&position=20&related_id=1205755&origin=tag (accessed July 17, 2022).
137. Freepik. Pregnant. (n.d.). Available online at: https://www.flaticon.com/free-icon/pregnant_1012567?term=pregnant&page=3&position=80&origin=tag&related_id=1012567 (accessed July 17, 2022).
138. Icongeek26. Badge. (n.d.). Available online at: https://www.flaticon.com/free-icon/badge_1250161?term=badge&page=1&position=5&origin=style&related_id=1250161 (accessed July 17, 2022).
Keywords: nutrition, pregnancy, reward processing, cognition, hormones, metabolism, diet
Citation: Waclawek T and Park SQ (2023) Potential mechanisms and modulators of food intake during pregnancy. Front. Nutr. 10:1032430. doi: 10.3389/fnut.2023.1032430
Received: 30 August 2022; Accepted: 03 January 2023;
Published: 20 January 2023.
Edited by:
Juan Juan, Peking University First Hospital, ChinaReviewed by:
Morten Arendt Rasmussen, University of Copenhagen, DenmarkMarc Schneeberger Pane, Yale University, United States
Luisa Torri, University of Gastronomic Sciences, Italy
Copyright © 2023 Waclawek and Park. This is an open-access article distributed under the terms of the Creative Commons Attribution License (CC BY). The use, distribution or reproduction in other forums is permitted, provided the original author(s) and the copyright owner(s) are credited and that the original publication in this journal is cited, in accordance with accepted academic practice. No use, distribution or reproduction is permitted which does not comply with these terms.
*Correspondence: Soyoung Q. Park, c295b3VuZy5xLnBhcmtAZ21haWwuY29t