- Department of Urology, Peking Union Medical College, Peking Union Medical College Hospital, Chinese Academy of Medical Sciences, Beijing, China
Background: The effect of micronutrients on urologic cancers has been explored in observational studies. We conducted the two-sample mendelian randomization (TSMR) study to investigate whether micronutrients could causally influence the risk of urologic cancers.
Methods: Summary statistics for four micronutrients and three main urologic cancers outcomes were obtained from genome-wide association studies (GWAS). MR analyses were applied to explore the potential causal association between them. Sensitivity analyses using multiple methods were also conducted.
Results: Genetically predicted one SD increase in serum copper and iron concentrations was causally associated with increased risks of renal cell carcinoma (RCC) (OR = 3.021, 95%CI = 2.204–4.687, P < 0.001, male; OR = 2.231, 95%CI = 1.524-3.953, P < 0.001, female; OR = 1.595, 95%CI = 1.310–1.758, P = 0.0238, male; OR = 1.484, 95%CI = 1.197–2.337, P = 0.0210, female, respectively) and per SD increase in serum zinc levels was related to decreased risks of RCC (OR = 0.131, 95%CI = 0.0159–0.208, P < 0.001, male; OR = 0.124, 95%CI = 0.0434–0.356, P < 0.001, female). No significant results were observed between micronutrients and the risk of bladder cancer after Bonferroni correction. Additionally, per SD increase in serum zinc level was associated with a 5.8% higher risk of prostate cancer (PCa) [OR = 1.058, 95%CI = 1.002–1.116, P = 0.0403, inverse-variance weight (IVW)].
Conclusions: Micronutrients play a vital role in the development of urological tumors. Future studies are required to replicate the findings, explore the underlying mechanisms, and examine the preventive or therapeutic role of micronutrients in clinical settings.
1. Introduction
Urologic tumor refers to tumors that affect the organs and structures of the urinary system of both men and women and the reproductive system of men. Three most prevalent types of urologic tumors are: prostate cancer (PCa), renal cell carcinoma (RCC), and bladder cancer (BCa) (1). The incidence of kidney, bladder, and prostate cancers cases increased between 1990 and 2013 and mortality increased 1.6-fold during the same time period. Urologic cancer burden has increased globally amid population growth and aging (2). Efforts to expand the global oncologic workforce and reduce preventable factors may contribute to cancer management (3). Nowadays, several risk factors have been established, such as lipid composition, obesity, and cigarette, etc (4). However, the role of nutrition in urologic cancer development is still unclear.
Dietary trace metals, including zinc, copper, iron, and selenium, etc. have been shown to influence the risk of cancer through oxidative stress, DNA injury and repair, regulating cell cycle, and angiogenesis (5). Some observational studies using food frequency questionnaires (FFQs) indicated the anti-tumor role of nutrients or dietary intake of nutrients in urologic cancer, while these results are conflicting and concerns about potential biases from confounding factors can't be dispelled (6).
Mendelian randomization (MR) that uses genetic variants as instrumental variables is widely used in epidemiological studies to examine whether a potential factor could casually influence an outcome. Different from traditional observational studies, this method could dramatically lower the effect of confounders and reverse causation (7). Two-sample Mendelian randomization (TSMR), which belongs to MR methodology and uses two samples drawn from the same underlying population with no overlap of participants between the two samples, is a method to estimate the causal effect of an exposure on an outcome using only summary statistics from genome-wide association studies (GWAS). Some large-scale GWAS on micronutrients and urologic cancers have also been published, providing high-quality genetic instruments to conduct MR study (8, 9). These GWAS have been used and validated in several previous MR studies (10, 11). To fill in the gap, we conducted the TSMR study to identify the potential effect of microelement levels on urologic cancer risk.
2. Method
2.1. Study design
The MR analysis was designed to evaluate the associations between microelement levels and risks of urologic tumors (RCC, BCa, and PCa). Single nucleotide polymorphisms (SNPs) for common microelements (Cu, Zn, Fe, and Se) were selected as instrumental variables from previously published genome-wide association study (GWAS) analyses. Three key assumptions need to be satisfied: (a) the SNPs should have strong associations with microelement levels; (b) the chosen SNPs should be independent of confounders; (3) the SNPs should affect cancer only via microelement levels. The diagram of the TSMR was shown in Figure 1 (12).
2.2. Data sources
The study utilized summarized genetic data from the Genetics of Iron Status (GIS) consortium (13), Prostate Cancer Association Group to Investigate Cancer Associated Alterations in the Genome (PRATICAL) (8), International Academic & Research Consortium (IARC) (14), and UK Biobank (UKB) (9). Details about the sources were shown in Table 1. The original GWAS had been approved by corresponding ethics committee, and the approval of current study was obtained from the Medical Research Ethics Committee of Peking Union Medical College Hospital.
2.3. Instrumental variable selection
Instrumental variable selection for Cu, Zn, and Se levels (serum), iron levels, and blood and toenail Se levels were based on a GWAS with 2,603 adults from Australia and the UK., a GWAS involving 48,972 individuals of European ancestry (GIS Consortium), and the UK Biobank study, respectively (13, 15, 16). Instrumental variables for RCC, BCa, and PCa were obtained from IARC (5,219 RCC cases and 8,011 controls of European ancestry), UKB (1,101 BCa cases and 461,832 controls of European ancestry), and PRATICAL consortium (79,148 PCa cases and 61,106 controls of European ancestry), respectively (8, 9, 14). Single nucleotide polymorphisms (SNPs) that met the locus-wide significance level (P < 10−5) and have genome-wide statistical significance (P < 5 × 10−8) were proposed as instrumental variables. Phenoscanner website was used to examine the pleiotropic effects of selected IVs and all used IVs were validated in previous studies (17, 18). All the SNPs selected in the study were shown in the Supplementary Table 1.
2.4. Study outcomes
RCC, BCa, and PCa were the outcomes. The latest GWAS involving the most complete available data on three types of cancers was selected. The sources were presented in Table 1.
2.5. Statistical analysis
Five different statistical methods were used to conduct the MR analyses. Firstly, the inverse-variance weight (IVW) approach was applied for the primary TSMR to quantify the causal associations between micronutrient (Cu, Fe, Se, and Zn) concentrations and the risk of three types of cancers (19). In the process, the ratio of coefficients was calculated to evaluate the causal effects. MR-Egger regression was used to examine the horizontal pleiotropy between IVs and three types of cancers, which adjusted micronutrients levels. Additionally, weighted median method (WM) only needs half of the effective SNPs was used as a supplement for the IVW approach (20). Finally, weighted mode and simple mode analyses were used to estimate the causal effect (21). https://shiny.cnsgenomics.com/mRnd/ was used for sample size test. This required four parts data: (a) Proportion of cases in the (intended) study; (b) Total sample size; (c) True odds ratio of the outcome variable per standard deviation of the exposure variable; (d) Proportion of variance in exposure variable explained by SNPs. Results indicated that with all the given sample size, analysis in each subgroup has strong statistical power (22). All traits related to screened SNPs were searched on the PhenoScanner website. Statistical analyses were performed repeatedly after removing confounder-related SNPs to improve the robustness and handle potential horizontal pleiotropy. Sensitivity analysis was also performed to assess whether some SNPs had a significantly independent influence on results via leave-one-out approach and the remaining estimate effect was shown when one SNP was excluded (23). The level of heterogeneity was estimated by using Cochran's Q statistics. All analyses were conducted in R software (version 4.1.2; http://www.rproject.org) with the “TwoSampleMR” package (version 0.5.6). Associations were considered as strong between micronutrients levels and cancer risks if they surpassed a stringent Bonferroni-corrected P-value threshold of 1.67 × 10−3 (0.05/3 cancer outcomes). The reporting of the MR study followed the existed rule (24).
3. Results
3.1. Associations between micronutrients and risk of RCC
For the four micronutrients, the primary estimate by IVW indicated that genetically predicted one SD increase in serum copper and iron concentrations was causally associated with increased risks of RCC (OR = 3.021, 95%CI = 2.204–4.687, P < 0.001, male; OR = 2.231, 95%CI = 1.524–3.953, P < 0.001, female; OR = 1.595, 95%CI = 1.310–1.758, P = 0.0238, male; OR = 1.484, 95%CI = 1.197–2.337, P = 0.0210, female, respectively) and per SD increase in serum zinc levels was related to decreased risks of RCC (OR = 0.131, 95%CI = 0.0159–0.208, P < 0.001, male; OR = 0.124, 95%CI = 0.0434–0.356, P < 0.001, female). However, no causal effect was observed in serum selenium and serum and toenail selenium (Table 2). Not all sensitivity analysis supported the causation between these micronutrients and RCC risk (Table 2 and Supplementary Figures 1–4).
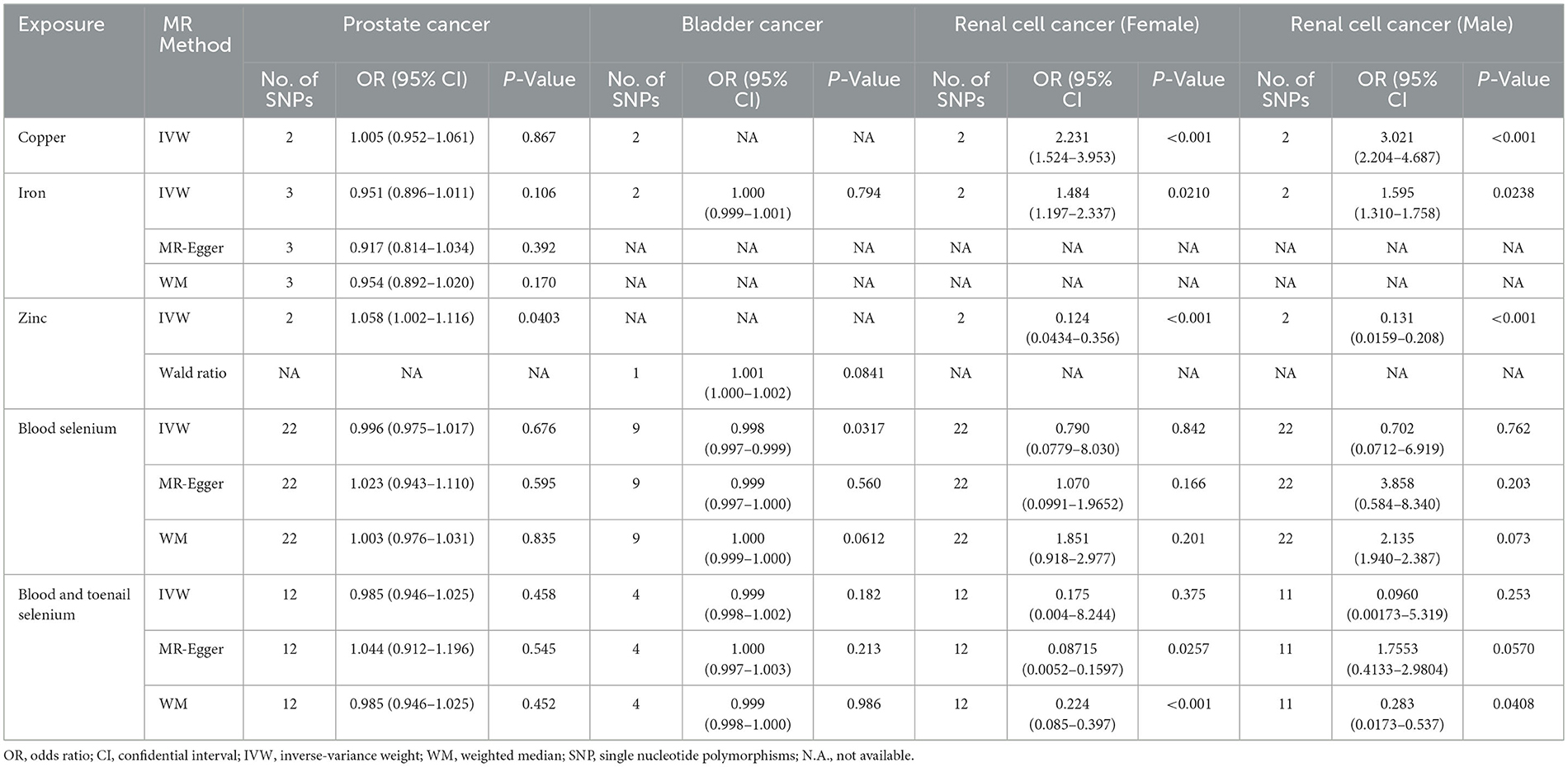
Table 2. Two-sample MR estimates of relationship between genetically predicted micronutrients and cancer.
3.2. Associations between micronutrients and risk of BCa
No causal associations were observed between risks of BCa and serum iron level (OR = 1.000, 95%CI = 0.999–1.001, P = 0.794, IVW), zinc level (OR = 1.001, 95%CI = 1.000–1.002, P = 0.0841, Wald ratio), serum selenium (OR = 0.998, 95%CI = 0.997–0.999, P = 0.0317, IVW), and blood and toenail selenium (OR = 0.999, 95%CI = 0.998–1.002, P = 0.182, IVW) (Figures 2, 3). Sensitivity analyses revealed consistent results (Table 2 and Supplementary Figures 5–8).
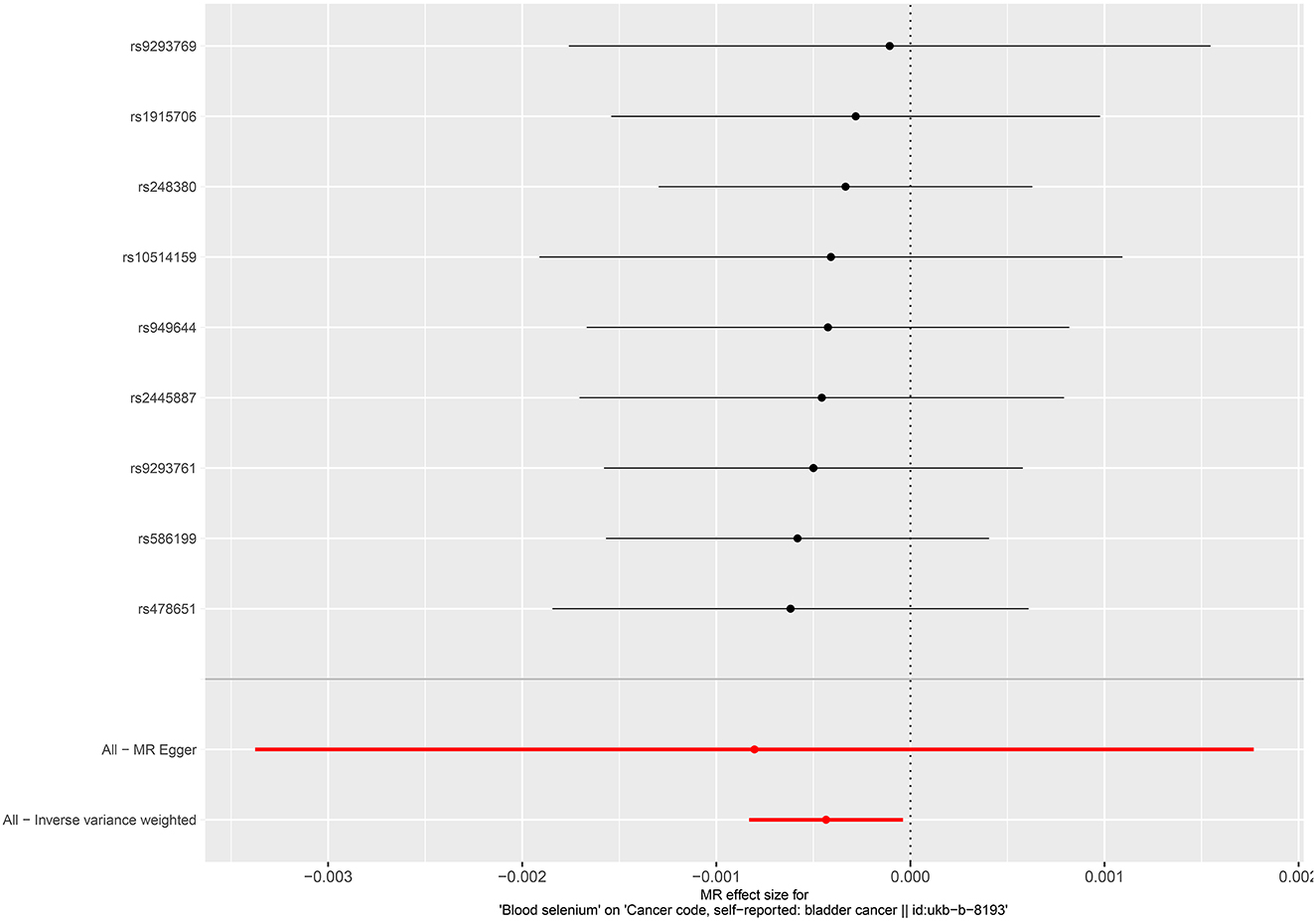
Figure 2. Forest plot for the association between circulating selenium and the risk of bladder cancer.
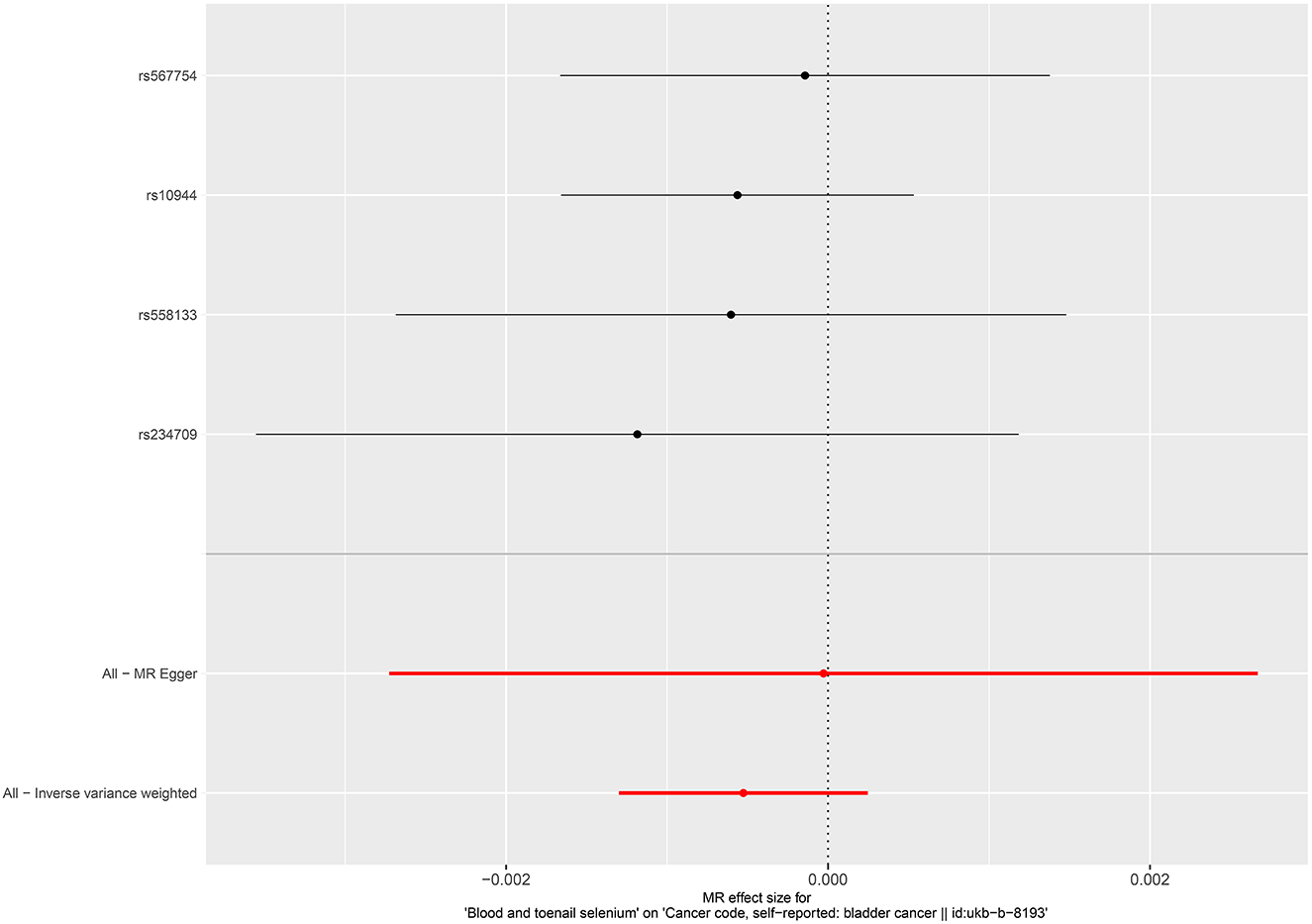
Figure 3. Forest plot for the association between blood and toenail selenium and the risk of bladder cancer.
3.3. Associations between micronutrients and risk of PCa
Per SD increase in serum zinc level was associated with a 5.8% higher risk of PCa (OR = 1.058, 95%CI = 1.002–1.116, P = 0.0403, IVW). No causal associations were observed between risks of PCa and serum copper level (OR = 1.005, 95%CI = 0.952–1.061, P = 0.867, IVW), iron level (OR = 0.951, 95%CI = 0.896–1.011, P = 0.106, IVW), serum selenium (OR = 0.996, 95%CI = 0.975–1.017, P = 0.676), and blood and toenail selenium (OR = 0.985, 95%CI = 0.946–1.025, P = 0.458, IVW) (Figures 4–6). Consistent results were also achieved in sensitivity analysis (Table 2 and Supplementary Figures 9–14).
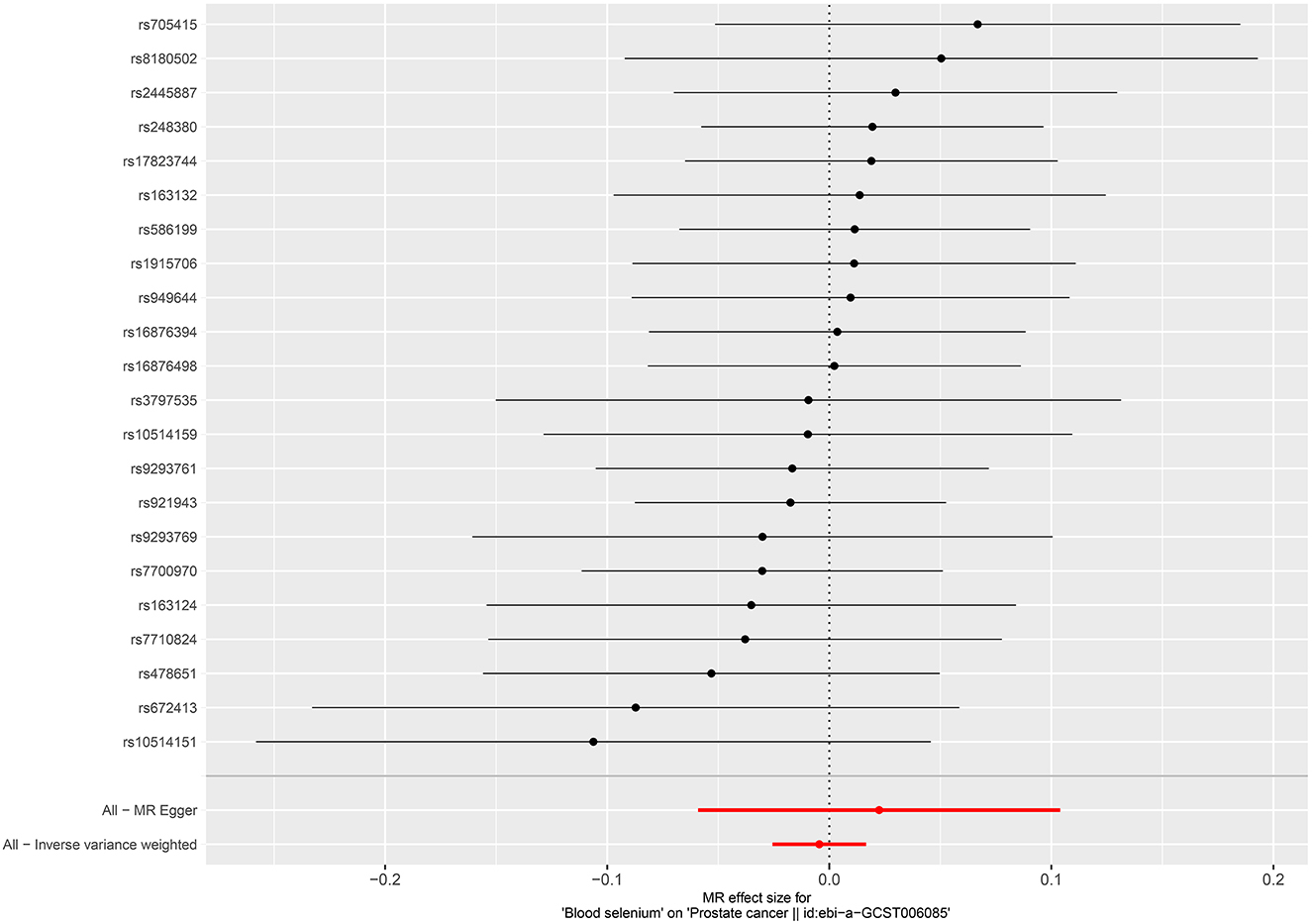
Figure 4. Forest plot for the association between circulating selenium and the risk of prostate cancer.
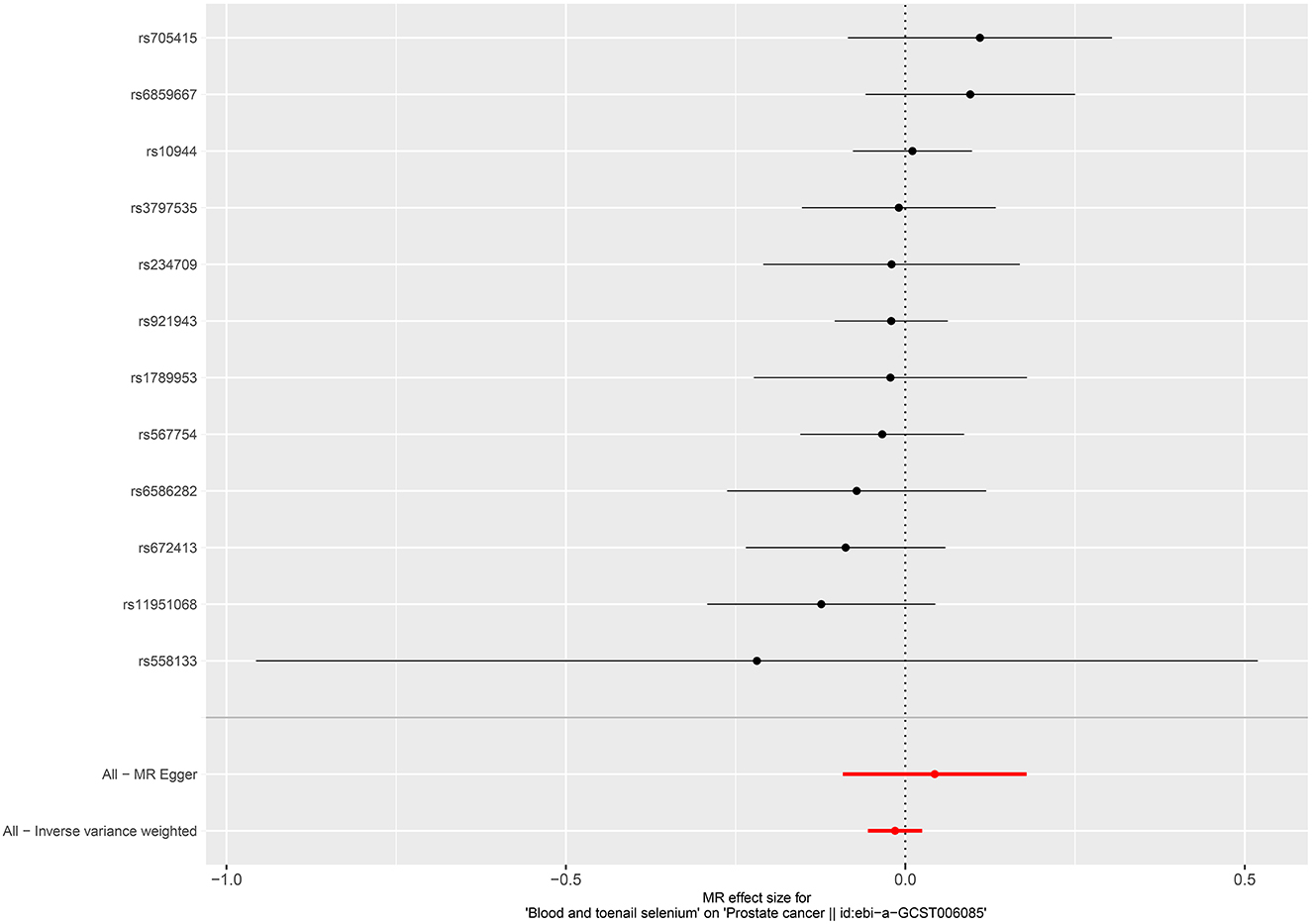
Figure 6. Forest plot for the association between blood and toenail selenium and the risk of prostate cancer.
4. Discussion
In the current study, we investigated the causal effects of four micronutrients (Copper, Iron, Selenium, and Zinc) on risks of 3 urological tumors. The findings indicated that genetically increased Zinc levels were related to increased risk of PCa, while reduced risk of RCC. Furthermore, increased Copper and iron level were associated with increased risk of RCC. In terms of BCa, no causal effects were observed.
Prostate cancer is a common malignancy that affects millions of men globally (1). Prior observational and mendelian studies have indicated that serum calcium and selenium levels were not associated with the risk of PCa (10, 11), which was consistent with our results. A former study indicated that decreased zinc or increased copper level might play important role in the initiation of PCa, while no mendelian studies ever investigated the genetic causation between them (25). We found that increased serum zinc level was causatively related to increased risk of PCa, which was in accordance with a population-based study (26). A possible explanation for the phenomenon is the redistribution of zinc, leading to increased serum zinc and reduced intracellular zinc in prostate (27). However, no GWAS about intracellular zinc were available, the underlying mechanisms require further investigation.
RCC is another common urologic malignancy (1). No mendelian study ever investigated the effect of micronutrients on the risk of RCC. Previous studies focus on exploring the role of zinc-finger protein in RCC (28, 29), demonstrating that these zinc-finger proteins could suppress the proliferation, invasion and improve long-term prognosis. Only one study indicated that Zn in the medulla was significantly lower in RCC cases than in controls (30). The result is consistent with what we found. Greene et al. demonstrated that RCC development is commonly represented by accumulated iron and Wu et al. conducted an in vivo study that indicated that STEAP3 played a crucial role in the iron dysfunction in ccRCC (31, 32). Few clinical studies had showed the association between iron level and ccRCC risk in humans to date. Sridhar et al. indicated that a significantly higher copper concentration is noted in the blood and urine in RCC patients as compared to healthy controls (33). In accordance with this, we found that increased serum copper levels could genetically increase the risk of RCC. It might be attributed to oxidative stress responses to accumulation of heavy metals, while the exact underlying mechanism requires further studies.
For bladder cancer, former studies indicated that increased copper or zinc levels in the blood of patients were associated with angiogenesis in BCa and the risk of BCa (34, 35). However, we found no genetic associations between copper or zinc levels and BCa risk. Two reasons might explain the difference. Firstly, the small number of available SNPs may cause statistical biases. Secondly, the results of former observational studies were influenced by confounders. More GWAS and experimental studies are warranted.
Two uncommon diseases featured by the pathological accumulation of micronutrients should be mentioned. Wilson disease (WD) is an inherited disorder of copper metabolism, which is caused by homozygous or compound heterozygous mutations (the presence of two different mutant alleles) in ATP7B (36). Copper absorbed from the diet and copper released from hepatocytes with exhausted endogenous copper storage capacity progressively accumulate in other organs, most notably in the brain, eyes, kidneys, bones, and heart, exerting extrahepatic toxicity. Almost 90% of patients with WD has reduced level of serum copper and copper is mainly accumulated in organs. The most frequently reported cancer in WD patients is hepatocellular carcinoma (37). Few studies have reported WD patients complicated with urologic cancers, while the anti-copper therapy has been used and verified as a validated treatment in several cancers (38, 39). Haemochromatosis is a systemic iron overload of genetic origin, caused by a reduction in the concentration of the iron regulatory hormone hepcidin, or a reduction in hepcidin-ferroportin binding. Similar to WD, haemochromatosis featured by the accumulation of iron in the liver, is associated with elevated serum ferritin and increased serum transferrin saturation rather than serum iron level and it is mostly reported to have an association with hepatocellular carcinoma (40). The association between the two diseases and urologic tumors still requires further investigation.
Our findings have some clinical and research implications. Firstly, we firstly indicated the genetic associations between micronutrients and three main urologic tumors by using mendelian randomization. Some of the micronutrients we identified in this study can be used as cancer biomarkers for risk prediction. While the prerequisite to achieving this is the clear association between the serum micronutrient level and cancer risks (linear or U-shape or …). Based on this, we can further make a classification strategy, for example, using the median level as the cut-off. Given current evidence, there is still a long way to go. Secondly, appropriate therapy that could adjust micronutrient levels in the blood will contribute to the prevention of urologic tumors and, eventually, of the cancer-associated disease burden and mortality. However, it should be noted that no clear evidence (the number of RCTs is <20) about the micronutrient intervention and cancer risk or cancer progression can be found. While more research is needed to assess whether micronutrients may modify the risk of cancer in individuals with a specific genetic background or nutritional status, and to investigate possible differential effects of various forms of micronutrients. Thirdly, the bias caused by limited numbers of SNPs should be validated in experimental studies. Fourthly, the conflicting findings on the effect of Zinc on PCa and RCC should be examined in experimental studies. According to currently available literature, we supposed that different zinc-related protein expressions in the kidney and the prostate might play a role in the development of the two cancers. Moreover, the balance between the zinc influx protein family and zinc efflux transporters on different organs might make a difference (27).
Our study has some strengths. Firstly, the study was the first MR study to investigate the casual association between micronutrient levels and the risk of urologic tumors. Effects of confounders in observational studies are avoided. Secondly, all the included individuals were of European-descent, which could minimize the potential bias from population stratification. Additionally, four common micronutrients and three main urologic tumors were analyzed, which is comprehensive and informative. Finally, limitations should be pointed out. Firstly, findings achieved from the MR study consisted of European-descent population limited the generativity to other races. Secondly, serum micronutrients might have associations with nutrition status, intelligence, income, and education level, etc (41). All these factors might play as a confounder between micronutrients and urologic tumors, while a concrete role of these factors was not the aim of the study and it requires further research.
In conclusion, we found that genetically increased Zinc levels were related to increased risk of PCa, while reduced risk of RCC. Furthermore, increased Copper and iron level were associated with increased risk of RCC, no causal effects were observed in BCa. The results indicate that micronutrients play a vital role in urological tumors. Future studies are therefore warranted to validate our findings and examine whether micronutrient concentration surveillance or supplements could be potential interventions for urologic cancer prevention and treatment.
Data availability statement
The original contributions presented in the study are included in the article/Supplementary material, further inquiries can be directed to the corresponding author.
Ethics statement
Written informed consent was obtained from the individual(s) for the publication of any potentially identifiable images or data included in this article.
Author contributions
YL, YW, and HL: conception and design. HL: administrative support. YL, HS, and YW: collection and assembly of data. YW and HS: data analysis and interpretation. All author manuscript writing and final approval of manuscript.
Funding
This work was supported by the grant from National Population Health Science Data Sharing Service Platform Clinical Medical Science Data Center (NCMI-ABD02-201906) to HL.
Conflict of interest
The authors declare that the research was conducted in the absence of any commercial or financial relationships that could be construed as a potential conflict of interest.
Publisher's note
All claims expressed in this article are solely those of the authors and do not necessarily represent those of their affiliated organizations, or those of the publisher, the editors and the reviewers. Any product that may be evaluated in this article, or claim that may be made by its manufacturer, is not guaranteed or endorsed by the publisher.
Supplementary material
The Supplementary Material for this article can be found online at: https://www.frontiersin.org/articles/10.3389/fnut.2023.1016243/full#supplementary-material
References
1. Siegel RL, Miller KD, Fuchs HE, Jemal A. Cancer statistics, 2021. CA Cancer J Clin. (2021) 71:7–33. doi: 10.3322/caac.21654
2. Siegel RL, Miller KD, Jemal A. Cancer statistics, 2016. CA Cancer J Clin. (2016) 66:7–30. doi: 10.3322/caac.21332
3. Dy GW, Gore JL, Forouzanfar MH, Naghavi M, Fitzmaurice C. Global burden of urologic cancers, 1990-2013. Eur Urol. (2017) 71:437–46. doi: 10.1016/j.eururo.2016.10.008
5. Valko M, Rhodes CJ, Moncol J, Izakovic M, Mazur M. Free radicals, metals and antioxidants in oxidative stress-induced cancer. Chem Biol Interact. (2006) 160:1–40. doi: 10.1016/j.cbi.2005.12.009
6. Masko EM, Allott EH, Freedland SJ. The relationship between nutrition and prostate cancer: is more always better? Eur Urol. (2013) 63:810–20. doi: 10.1016/j.eururo.2012.11.012
7. Minică CC, Boomsma DI, Dolan CV, de Geus E, Neale MC. Empirical comparisons of multiple Mendelian randomization approaches in the presence of assortative mating. Int J Epidemiol. (2020) 49:1185–93. doi: 10.1093/ije/dyaa013
8. Schumacher FR, Al Olama AA, Berndt SI, Benlloch S, Ahmed M, Saunders EJ, et al. Association analyses of more than 140,000 men identify 63 new prostate cancer susceptibility loci. Nat Genet. (2018) 50:928–36. doi: 10.1038/s41588-018-0142-8
9. Sudlow C, Gallacher J, Allen N, Beral V, Burton P, Danesh J, et al. UK biobank: an open access resource for identifying the causes of a wide range of complex diseases of middle and old age. PLoS Med. (2015) 12:e1001779. doi: 10.1371/journal.pmed.1001779
10. Yarmolinsky J, Berryman K, Langdon R, Bonilla C, Davey Smith G, Martin RM, et al. Mendelian randomization does not support serum calcium in prostate cancer risk. Cancer Causes Control. (2018) 29:1073–80. doi: 10.1007/s10552-018-1081-5
11. Yarmolinsky J, Bonilla C, Haycock PC, Langdon RJQ, Lotta LA, Langenberg C, et al. Circulating selenium and prostate cancer risk: a mendelian randomization analysis. J Natl Cancer Inst. (2018) 110:1035–8. doi: 10.1093/jnci/djy081
12. Little M. Mendelian randomization: methods for using genetic variants in causal estimation. J R Stat Soc Ser A Stat Soc. (2018) 181:549–50. doi: 10.1111/rssa.12343
13. Benyamin B, Esko T, Ried JS, Radhakrishnan A, Vermeulen SH, Traglia M, et al. Novel loci affecting iron homeostasis and their effects in individuals at risk for hemochromatosis. Nat Commun. (2014) 5:4926. doi: 10.1038/ncomms5926
14. Laskar RS, Muller DC Li P, Machiela MJ, Ye Y, Gaborieau V, et al. Sex specific associations in genome wide association analysis of renal cell carcinoma. Eur J Hum Genet. (2019) 27:1589–98. doi: 10.1038/s41431-019-0455-9
15. Cornelis MC, Fornage M, Foy M, Xun P, Gladyshev VN, Morris S, et al. Genome-wide association study of selenium concentrations. Hum Mol Genet. (2015) 24:1469–77. doi: 10.1093/hmg/ddu546
16. Evans DM, Zhu G, Dy V, Heath AC, Madden PA, Kemp JP, et al. Genome-wide association study identifies loci affecting blood copper, selenium and zinc. Hum Mol Genet. (2013) 22:3998–4006. doi: 10.1093/hmg/ddt239
17. Papadimitriou N, Dimou N, Gill D, Tzoulaki I, Murphy N, Riboli E, et al. Genetically predicted circulating concentrations of micronutrients and risk of breast cancer: a mendelian randomization study. Int J Cancer. (2021) 148:646–53. doi: 10.1002/ijc.33246
18. Ruth KS, Day FR, Tyrrell J, Thompson DJ, Wood AR, Mahajan A, et al. Using human genetics to understand the disease impacts of testosterone in men and women. Nat Med. (2020) 26:252–8. doi: 10.1038/s41591-020-0751-5
19. Burgess S, Butterworth A, Thompson SG. Mendelian randomization analysis with multiple genetic variants using summarized data. Genet Epidemiol. (2013) 37:658–65. doi: 10.1002/gepi.21758
20. Bowden J, Davey Smith G, Haycock PC, Burgess S. Consistent estimation in mendelian randomization with some invalid instruments using a weighted median estimator. Genet Epidemiol. (2016) 40:304–14. doi: 10.1002/gepi.21965
21. Hemani G, Bowden J, Davey Smith G. Evaluating the potential role of pleiotropy in Mendelian randomization studies. Hum Mol Genet. (2018) 27:R195–r208. doi: 10.1093/hmg/ddy163
22. Brion MJ, Shakhbazov K, Visscher PM. Calculating statistical power in mendelian randomization studies. Int J Epidemiol. (2013) 42:1497–501. doi: 10.1093/ije/dyt179
23. Hemani G, Zheng J, Elsworth B, Wade KH, Haberland V, Baird D, et al. The MR-Base platform supports systematic causal inference across the human phenome. Elife. (2018) 7:e34408. doi: 10.7554/eLife.34408
24. Skrivankova VW, Richmond RC, Woolf BAR, Yarmolinsky J, Davies NM, Swanson SA, et al. Strengthening the reporting of observational studies in epidemiology using mendelian randomization: the STROBE-MR statement. JAMA. (2021) 326:1614–21. doi: 10.1001/jama.2021.18236
25. Saleh SAK, Adly HM, Abdelkhaliq AA, Nassir AM. Serum levels of selenium, zinc, copper, manganese, and iron in prostate cancer patients. Curr Urol. (2020) 14:44–9. doi: 10.1159/000499261
26. Gutiérrez-González E, Castelló A, Fernández-Navarro P, Castaño-Vinyals G, Llorca J, Salas D, et al. Dietary zinc and risk of prostate cancer in Spain: MCC-Spain study. Nutrients. (2018) 11:18. doi: 10.3390/nu11010018
27. To PK, Do MH, Cho J-H, Jung C. Growth modulatory role of zinc in prostate cancer and application to cancer therapeutics. Int J Mol Sci. (2020) 21:2991. doi: 10.3390/ijms21082991
28. Liang W, Chen S, Yang G, Feng J, Ling Q, Wu B, et al. Overexpression of zinc-finger protein 677 inhibits proliferation and invasion by and induces apoptosis in clear cell renal cell carcinoma. Bioengineered. (2022) 13:5292–304. doi: 10.1080/21655979.2022.2038891
29. Ren LX, Qi JC, Zhao AN, Shi B, Zhang H, Wang DD, et al. Myc-associated zinc-finger protein promotes clear cell renal cell carcinoma progression through transcriptional activation of the MAP2K2-dependent ERK pathway. Cancer Cell Int. (2021) 21:323. doi: 10.1186/s12935-021-02020-9
30. Hardell L, Wing AM, Ljungberg B, Dreifaldt AC, Degerman A, Halmans G. Levels of cadmium, zinc and copper in renal cell carcinoma and normal kidney. Eur J Cancer Prev. (1994) 3:45–8. doi: 10.1097/00008469-199401000-00006
31. Greene CJ, Attwood K, Sharma NJ, Balderman B, Deng R, Muhitch JB, et al. Iron accumulation typifies renal cell carcinoma tumorigenesis but abates with pathological progression, sarcomatoid dedifferentiation, and metastasis. Front Oncol. (2022) 12:923043. doi: 10.3389/fonc.2022.923043
32. Wu J, Bi Q, Zheng X, Cao H, Hao C, Sun Z, et al. STEAP3 can predict the prognosis and shape the tumor microenvironment of clear cell renal cell carcinoma. BMC Cancer. (2022) 22:1204. doi: 10.1186/s12885-022-10313-z
33. Panaiyadiyan S, Quadri JA, Nayak B, Pandit S, Singh P, Seth A, et al. Association of heavy metals and trace elements in renal cell carcinoma: a case-controlled study. Urol Oncol. (2022) 40:111.e11–8. doi: 10.1016/j.urolonc.2021.11.017
34. Mao S, Huang S. Zinc and copper levels in bladder cancer: a systematic review and meta-analysis. Biol Trace Elem Res. (2013) 153:5–10. doi: 10.1007/s12011-013-9682-z
35. Mortada WI, Awadalla A, Khater S, Ahmed A, Hamam ET, El-Zayat M, et al. Copper and zinc levels in plasma and cancerous tissues and their relation with expression of VEGF and HIF-1 in the pathogenesis of muscle invasive urothelial bladder cancer: a case-controlled clinical study. Environ Sci Pollut Res Int. (2020) 27:15835–41. doi: 10.1007/s11356-020-08113-8
36. Bandmann O, Weiss KH, Kaler SG. Wilson's disease and other neurological copper disorders. Lancet Neurol. (2015) 14:103–13. doi: 10.1016/S1474-4422(14)70190-5
37. Członkowska A, Litwin T, Dusek P, Ferenci P, Lutsenko S, Medici V, et al. Wilson disease. Nat Rev Dis Primers. (2018) 4:21. doi: 10.1038/s41572-018-0018-3
38. Brewer GJ. Copper control as an antiangiogenic anticancer therapy: lessons from treating Wilson's disease. Exp Biol Med. (2001) 226:665–73. doi: 10.1177/153537020222600712
39. Brewer GJ, Dick RD, Grover DK, LeClaire V, Tseng M, Wicha M, et al. Treatment of metastatic cancer with tetrathiomolybdate, an anticopper, antiangiogenic agent: phase I study. Clin Cancer Res. (2000) 6:1–10.
40. Brissot P, Pietrangelo A, Adams PC, De Graaff B, McLaren CE, Loréal O. Haemochromatosis. Nat Rev Dis Primers. (2018) 4:18016. doi: 10.1038/nrdp.2018.16
Keywords: micronutrients, prostate cancer, renal cell carcinoma, bladder cancer, Mendelian randomization
Citation: Lu Y, Su H, Wang Y and Li H (2023) Micronutrients and risks of three main urologic cancers: A mendelian randomization study. Front. Nutr. 10:1016243. doi: 10.3389/fnut.2023.1016243
Received: 11 August 2022; Accepted: 13 February 2023;
Published: 27 February 2023.
Edited by:
Surasak Saokaew, University of Phayao, ThailandReviewed by:
Paul Hegarty, Mater Misericordiae University Hospital, IrelandMahdieh Khodarahmi, Isfahan University of Medical Sciences, Iran
Ralph Mücke, Self-Employed, Bad Kreuznach, Germany
Copyright © 2023 Lu, Su, Wang and Li. This is an open-access article distributed under the terms of the Creative Commons Attribution License (CC BY). The use, distribution or reproduction in other forums is permitted, provided the original author(s) and the copyright owner(s) are credited and that the original publication in this journal is cited, in accordance with accepted academic practice. No use, distribution or reproduction is permitted which does not comply with these terms.
*Correspondence: Hongjun Li, bGlob25nanVuQHB1bWNoLmNu