- 1College of Food Science and Engineering, Northwest A&F University, Xianyang, China
- 2College of Food Science and Engineering, Qingdao Agricultural University, Qingdao, China
Zein is a kind of excellent carrier materials to construct nano-sized delivery systems for hydrophobic bioactives, owing to its unique interfacial behavior, such as self-assembly and packing into nanoparticles. In this article, the chemical basis and preparation methods of zein nanoparticles are firstly reviewed, including chemical crosslinking, emulsification/solvent evaporation, antisolvent, pH-driven method, etc., as well as the pros and cons of different preparation methods. Various strategies to improve their physicochemical properties are then summarized. Lastly, the encapsulation and protection effects of zein-based nano-sized delivery systems (e.g., nanoparticles, nanofibers, nanomicelles and nanogels) are discussed, using curcumin as a model bioactive ingredient. This review will provide guidance for the in-depth development of hydrophobic bioactives formulations and improve the application value of zein in the food industry.
Introduction
Zein is the main storage protein in maize and accounts for 30%–60% of total protein content (1). It contains more than 50% of non-polar amino acids, including leucine, proline, alanine, phenylalanine, valine and isoleucine, but lacks basic and acidic amino acids (2). The unbalance of amino acid composition leads to the low nutritional value of zein. However, zein readily self-assembles into various structures depending on the solvents and the processing conditions, and it has been extensively studied in terms of encapsulating and delivering bioactive ingredients, which can be well–reflected in the yearly increase in number of publications related to the terms “zein & encapsulation” and “zein & delivery”, as shown in Figure 1. About half of the publications on zein are related to research for encapsulation and delivery over the past 3 years.
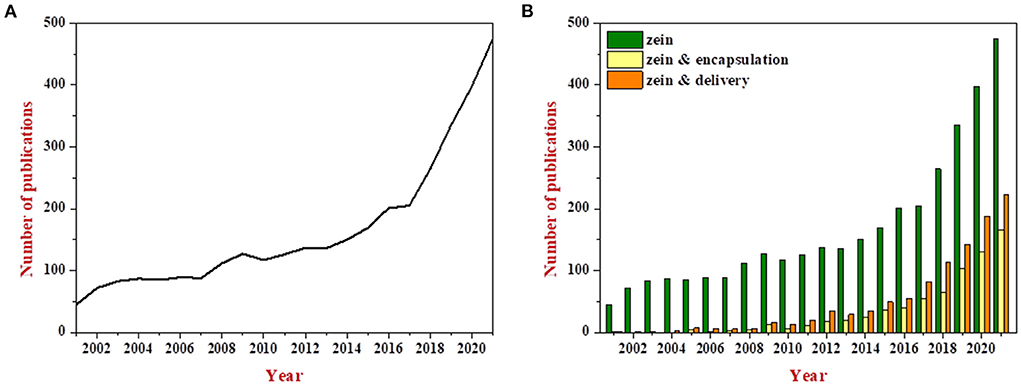
Figure 1. Evolution of number of publications related to the term “zein” during the years 2001 to 2021 (A); Comparison of the number of publications related to the terms “zein” vs. “zein & encapsulation” and “zein & delivery” (B). All the data were obtained through the Web of Science system.
In the past two decades, researchers have successively proposed that the construction of nano-sized delivery systems to encapsulate active ingredients can maximize their health effects, which is mainly attributed to (i) the high specific surface area of nanocarriers can increase the solubility and dissolution rate of actives; (ii) its nanoscale size enables the actives to circulate in the system for a longer time, improves actives distribution in the body as well as allows targeted release and increases biofilm permeability (3). Based on this, the solubility and bioavailability of hydrophobic active ingredients can be effectively enhanced with the help of nanocarriers. Common nano-sized delivery systems include nanoparticles, nanofibers, nanomicelles, solid lipid nanoparticles and nanoemulsion. Biopolymers are alternative materials for constructing nanocarriers because of their good biocompatibility, and Zein-based nano-sized delivery systems loaded with hydrophobic active ingredients have been constructed. For example, maytansine-loaded zein nanoparticles enhanced tumor targeting for non-small cell lung cancer (A549 cells) and reduced the toxic side effects of maytansine (4). Curcumin-loaded zein nanofibers showed enhanced antibacterial activity and the inhibition efficiency increased with the increase of curcumin content (5). Dispersibility of curcumin encapsulated in whey protein isolate (WPI)-zein composite nanoparticles was significantly improved (6). Zein-hyaluronic acid composite nanoparticles prepared through layer-by-layer assembly technique effectively improved the light, thermal and storage stability of curcumin and quercetagetin (7). With the help of nano-delivery system, the water solubility and chemical stability of encapsulated lutein were significantly improved, and the bioaccessibility (32.11%) was much higher than that of free lutein (16.21%) (8). In addition, many studies on hydrophobic active ingredients, such as quercetin, resveratrol, rutin, β-carotene, Vitamin D3, retinol encapsulated in zein-based nanocarriers have been reported (9–14).
In this review, we mainly introduce the chemical basis of zein and various preparation methods of zein nanoparticles, and summarize recent reports on zein-based composite nanoparticles, using curcumin as a model bioactive ingredient. In addition to zein nanoparticles, other types of zein delivery systems are also discussed, including nanofiber, nanomicelles, etc.
Chemical basis of zein
Composition and general properties
According to the difference in molecular weight and solubility, zein can be divided into four categories: α-, β-, γ-, δ-zein. Among them, α-zein accounts for 70%–85% of the total zein content, with a molecular weight of 19–22 kDa, and it is soluble in 70%–95% ethanol aqueous solution. β-zein is composed of α-zein linked by disulfide bonds, so its molecular weight is high. It is soluble in 60%–90% ethanol aqueous solution (15). Generally, Except for a certain concentration of alcohol solution, zein can also be dissolved in alkaline solutions with pH ≥ 11.5 (16, 17), and solutions with high concentration urea and other reducing agents (18). Although zein has poor water solubility, unbalanced amino acid composition and low nutritional value, its unique solubility makes it easy to self-assemble into zein micro/nanoparticles. Therefore, zein is an ideal carrier material for hydrophobic bioactive ingredients. It is also more anti-digestive than other proteins, which is one of the advantages as an oral delivery carrier.
Molecular structure
The self-assembling mechanism of zein is closely related to its structure. Several molecular models for the tertiary structure of α-zein have been proposed, including cylinder model, ribbon-like model, hairpin model and superhelical structural model (19).
The cylinder model is based on analysis of the repeat sequence units and α-helix content of zein dissolved in 70% methanol aqueous solution (20). There are 50%–60% α-helices in zein molecules, and the rest are turn and random coils. Each primary structure of a zein molecule consists of 20 amino acid residues repeated 9 times. Analysis of the physical properties (hydration potential, polarity, turn, helix formation tendency) indicates that the amino acid sequence containing repeat fragments consists of α-helixes with turn regions on both sides. The helix regions are composed of numerous polar amino acids and several hydrophobic amino acid residues, and the turn regions are rich in glutamine. In the structural model of zein, nine adjacent, topologically antiparallel homologous helices cluster form a cylindrical surface connected by polar glutamine residues (Figure 2A). The structure is stabilized by van der Waals force and intramolecular and intermolecular hydrogen bonds. Glutamine-rich turns are located between the helical structures and the cylindrical cap, facilitating the intermolecular stacking into a plane by the interaction between the side chains. The structure model can explain the dense, membrane-enveloped deposits formed by proteins in maize seeds.
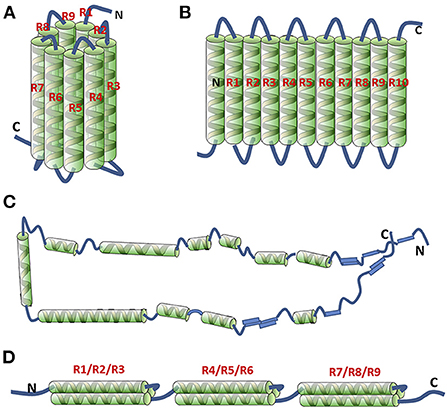
Figure 2. Proposed 3-D structural models of α-zein. Cylindrical model (A); ribbon-like model (22 kDa) (B); hairpin model (19 kDa) (C); super helical structural model (19 kDa) (D). “R” means repeat unit. Reprinted from (19) with permission from Elsevier.
To modify the cylinder model, the structure of zein dissolved in 70% ethanol aqueous solution was further analyzed by small angle X-ray scattering (SAXS) (21). In the ribbon-like model, zein is composed of 9–10 adjacent, topologically antiparallel homologous helices clustered, presenting a slender rectangular prism structure with an axial ratio of 6:1, and its three dimensions are 13, 3 and 1.2 nm (Figure 2B). The two-dimensional intermolecular hydrophobic aggregation makes the side chains in the helixes accumulate into a hydrophobic surface, forming a structure with hydrophilic ends and hydrophobic sides, which greatly improves the structural stability of α-zein. The two-dimensional ribbon-like model has been widely used to explain the different orientations of zein molecules on hydrophilic/hydrophobic surfaces and the self-assembly of zein from single molecule to nanoparticles.
In addition, the hairpin model based on nuclear magnetism resonance (NMR), SAXS and Fourier transform infrared spectroscopy (FTIR) is also composed of a series of helix structures as shown in Figure 2C (22). Different from the above two models, these helix structures are connected and extended in the form of rings, sheets or loops, so zein can fold or extend in other ways in different solvent environments. At the same time, it is thought to be related to the fibrillation ability of zein.
In 2006, a model consisting of three superhelix structures was built by using three antiparallel helix segments connected by a glutamine-rich turn, as shown in Figure 2D (23).
Despite the differences in the molecular models above, it is generally believed that α-zein is a slender conformation formed by a large number of α-helix structures. The three-dimensional structure of zein is affected by different factors, especially solvent type (24). Compared with the folded structure model, the slightly larger sizes of α-zein in acetic acid, 70% and 80% ethanol aqueous solutions indicate that α-zein structure is locally unfolded in these solutions. The CD data demonstrate the secondary structure of α-zein do not change significantly, either in acetic acid or in 70% and 80% ethanol aqueous solutions, which may be because local unfolding mostly occurs in the coil region. The size of α-zein in acetic acid is larger than that in ethanol aqueous solutions, indicating that the expansion and swelling degree of α-zein is greater in the presence of acid. With the increase of zein concentration, α-zein dissolved in acetic acid behaves like polyelectrolyte dissolved in an excellent solvent, and its particle size decreases slightly. Moreover, α-zein dissolved in ethanol aqueous solutions behaves like polymer dissolved in θ solvent (alcohol solution). The conformation and dissolution behaviors of α-zein both differ among different solvents, which may be attributed to three causes: (i) According to the similarity-intermiscibility theory, the dissolution behavior of α-zein should be related to the polarity of solvent. It is known that the hydrophilic index of water, acetic acid and ethanol is 9.0, 6.2, and 5.2 respectively referring to the solvent miscibility table, indicating the hydrophilic index is very close between acetic acid and 70% or 80% ethanol aqueous solutions. The hydrophilic index of α-zein with a molecular weight of 19 and 22 KDa is 4.70 and 4.80 respectively (25), so the similarity-intermiscibility theory should not be the main reason accounting for the difference of solvent quality. (ii) The differences can be related to the hydrophilicity/hydrophobicity of the protein surface. Partial unfolding of zein may change the solvent accessible area of zein surface and hydration. As local unfolding of α-zein is severer in acetic acid, the solvent accessible area is larger, thus promoting the dissolution of α-zein. (iii) Protein surface protonation can also inhibit protein aggregation. Protonation of zein reduces hydrophobicity and improves stability of zein solution through the long-range electrostatic repulsion induced by the positive charge on the protein surface and the existence of acetic acid solvation layer. Because -COOH in acetic acid releases free hydrogen ions more easily than -OH in ethanol, zein in acetic acid is more prone to protonation.
Fabrication of zein-based nanoparticles and its challenges
The construction of zein particles based on unique solubility behavior of zein can be traced back to the 1990s. Zein particles were used as coating materials first in pharmaceutical industry, and then in drug delivery system (26). The preparation methods of zein particles have become rich and various, and can be broadly summarized as chemical crosslinking, antisolvent precipitation, supercritical antisolvent, antisolvent dialysis, pH driving, spray drying, and electrohydrodynamic atomization. Advantages and disadvantages of these methods have been summarized in Table 1.
Chemical crosslinking
Glutaraldehyde mediated crosslinking reaction between zein and other molecules (e.g., proteins, polysaccharides, polyphenols) or zein molecules themselves is one of the early methods for zein particles formation. This method originated in the 1980s (27, 28). Glutaraldehyde is a colorless and transparent oily liquid with a pungent odor. It is a commonly homo-bifunctional crosslinking agent that combines with phenol, imidazole, hydroxyl and amino through the aldehyde groups at both ends of its molecule. The crosslinking reaction between molecules endows zein a stronger aggregation trend when exposed to water solutions owing to the distinct surface hydrophobicity of zein, and increases its molecular weight, which promotes the self-assembly of zein into particles. However, zein particles formed by chemical crosslinking are usually micro-scale. The general process for the formation of zein particles loaded with hydrophobic active ingredients (e.g., curcumin) by chemical crosslinking was shown in Figure 3A. Curcumin as a model bioactive ingredient is firstly dissolved in an ethanol aqueous solution, then mixed with a small amount of a glutaraldehyde aqueous solution, and finally zein is added to cause a cross-linking reaction, resulting in the formation of internally encapsulated curcumin particles. Noticeably, the water volume accompanying glutaraldehyde aqueous solution added must be strictly controlled because the presence of a large amount of water will lead to the aggregation and precipitation of zein, thereby inhibiting the crosslinking efficiency. After the reaction, it was highly diluted with polyvinylpyrrolidone (PVP) solution and centrifugation, followed by ultrasound and freeze-drying to obtain zein particles.
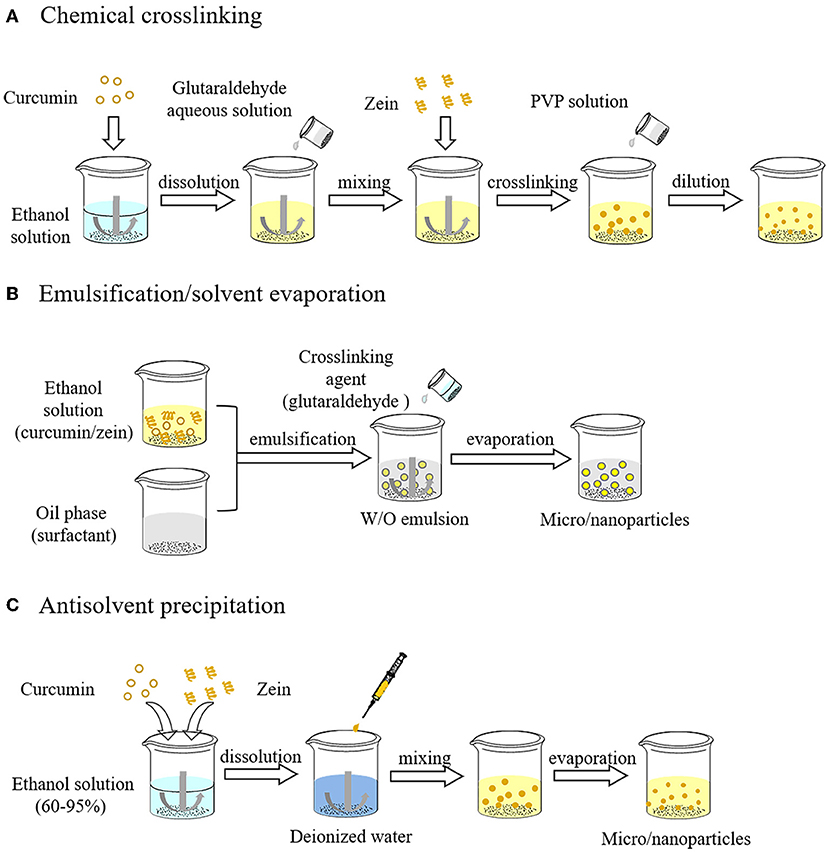
Figure 3. Preparation process of zein particles by chemical crosslinking (A), emulsification/solvent evaporation (B) and antisolvent precipitation (C).
There are some defects in the chemical crosslinking method. First, crosslinking reaction may produce adverse effects on the activity of ingredients encapsulated inside zein particles. Secondly, chemical crosslinking involves toxic agents, such as glutaraldehyde, which has a strong irritant effect on eyes, skin and mucous membrane, causing certain safety risks for the production process and the final products. Therefore, it is imperative to develop alternative green, safe and environmental-friendly chemical crosslinking agents.
Emulsification/solvent evaporation
With emulsification/solvent evaporation, the zein-ethanol aqueous solution and the oil phase containing miscible surfactants are emulsified to form the water-in-oil emulsion as a template for carrier preparation. The internal aqueous phase of the emulsion can be solidified into rigid particles by solvent evaporation. Crosslinking agents (e.g., glutaraldehyde) are added before solvent evaporation to obtain crosslinked particles, which can moderately reduce the size of particles. The operation process of emulsification/solvent evaporation is shown in Figure 3B. Briefly, curcumin as a model active ingredient and zein are co-dissolved in an ethanol aqueous solution, emulsified with oil phase, and then a crosslinking agent is added into the emulsion. With the evaporation of ethanol, zein and curcumin inside emulsion droplets are subsequently solidified into rigid crosslinked particles (29). Finally, zein particles loaded with curcumin are obtained by filtration and removal of residual oil using suitable solvents. Emulsification/solvent evaporation method has been used to prepare zein particles loaded with aceclofenac, and their particle size ranges from 30 to 300 μm, which is suitable for oral administration.
Antisolvent methods
Antisolvent precipitation method
Antisolvent precipitation, also known as liquid-liquid dispersion or phase separation, has been widely used for the preparation of zein nanoparticles by changing the polarity of solvents surrounding zein. As shown in Figure 3C, zein and curcumin are firstly dissolved in an ethanol aqueous solution (60%–95%, high solubility), which is then poured into or added dropwise into numerous water (as antisolvent) under magnetic stirring, so that the ethanol concentration decreased rapidly, resulting in supersaturation and precipitation of zein. Combined with the self-assembly characteristics, zein molecules aggregate into particles, and most of the curcumin spontaneously enters the hydrophobic core of zein particles. Subsequently, the ethanol is removed by rotary evaporation. Solvent polarity seriously affects the properties of zein particles. Ethanol, methanol and isopropanol can be used to prepare zein nanoparticles. The size of zein particles prepared from 80% alcohol solutions ranks by the alcohol type as ethanol>isopropanol>methanol, which may be due to the difference of solvent evaporation rate and zein solubility (30). In addition to the alcohol type, the initial concentration of alcohol and zein, the shear rate, the ratio of alcohol to water, pH, mixing mode and other added substances all can affect the particle properties.
Supercritical antisolvent method
In addition to using water as an antisolvent for the preparation of zein nanoparticles, supercritical fluid CO2 is also possible, due to its immiscibility with most polymers. The simple processing procedure of supercritical antisolvent method is that the co-solvent in atomized droplets is extracted by supercritical CO2 after the feedstock is continuously injected into supercritical CO2. Since most of the polymers are insoluble in CO2, the solubility of polymers decreases gradually, thus forming nuclei and gradually growing into nanoparticles or microparticles (31). The technology requires that the polymer be dissolved in one or more solvents (co-solvent), and these solvents can be miscible with supercritical CO2. Advantage of supercritical antisolvent method is that the critical temperature (31.1°C) and pressure (7.38 MPa) of CO2 can be easily achieved, and it is friendly to thermo-sensitive bioactives, so that they would not be degraded in the process of particle formation. As reported, supercritical CO2 has been used to form a micro/nano-particle delivery system for foods (32), so as to improve the physicochemical properties and health effects of active ingredients. Zein particles formed by supercritical CO2 antisolvent method were used to encapsulate lysozyme, which improved the antibacterial activity of lysozyme (33). Lutein/zein nanoparticles prepared by supercritical CO2 realized the controlled release of lutein (34).
Zein concentration, CO2 flow rate, alcohol type and concentration all affect the formation of zein particles. It is generally believed that large size particles are easily formed at high polymer concentrations due to the high viscosity of the solution, which further complicates the solution atomization. Meanwhile, the growth rate of particles is accelerated at high polymer concentrations. The increase of CO2 flow rate can promote the particle formation. In addition, the polarity of solvent affects the extraction efficiency of supercritical CO2, thus affecting the formation of zein particles. As for higher-polarity alcohols (methanol>ethanol>isopropanol), the extraction efficiency of supercritical CO2 (non-polar solvent) is lower, and the solidification rate of zein is slower. For example, zein dissolved in 90% methanol aqueous solution did not form particles by supercritical antisolvent technology, while zein dissolved in 90% isopropanol aqueous solution produced smaller particles than that dissolved in 90% ethanol aqueous solution. Reducing the water content of zein co-solvent is also helpful to supercritical CO2 extraction (mass transfer process) and speeds up the solidification of zein, thereby forming smaller and more uniform particles (31). It can be explained as follows: as the polarity of co-solvent increases (water or more polar alcohols are added), the mass transfer rate slows down due to the poor compatibility between CO2 and co-solvent. Particles are formed when the polymer precipitation kinetics can overcome the mass transfer limitation. The particle size of zein can be adjusted from micron to nanometer by changing the type and concentration of alcohol.
Supercritical antisolvent technology with CO2 has the advantages of mild operating temperature, friendly environment, simple operation and easy control. However, it is difficult to produce uniform zein nanoparticles unless 100% methanol is used as solvent and supercritical CO2 is loaded at a high flow rate. In this case, it will increase production costs and may cause toxicity due to residual organic solvents.
Antisolvent dialysis method
Antisolvent dialysis is a variant of antisolvent precipitation. Firstly, the biopolymer is miscible with the first solvent (organic phase) to form mother liquid. Then the first solvent is replaced by another solvent (aqueous phase) which is immiscible with the polymer to form a colloidal dispersion (35). The preparation of zein particles by antisolvent dialysis is mainly based on changes in polymer concentration gradient and solvent polarity. As shown in Figure 4, the dialysis bag containing an alcohol solution dissolving zein and curcumin is firstly placed in the aqueous phase, and then the organic solvent gradually diffused into the external aqueous phase through the semipermeable membrane. With this process, the change of the polar environment of zein induces the formation of zein particles. Compared with the antisolvent precipitation method, the advantage of the antisolvent dialysis method is that it only needs one-step to realize the formation of particles and solvent replacement simultaneously. Unlike antisolvent precipitation, additional operations (rotary evaporation) are required to remove organic solvents after particle formation.
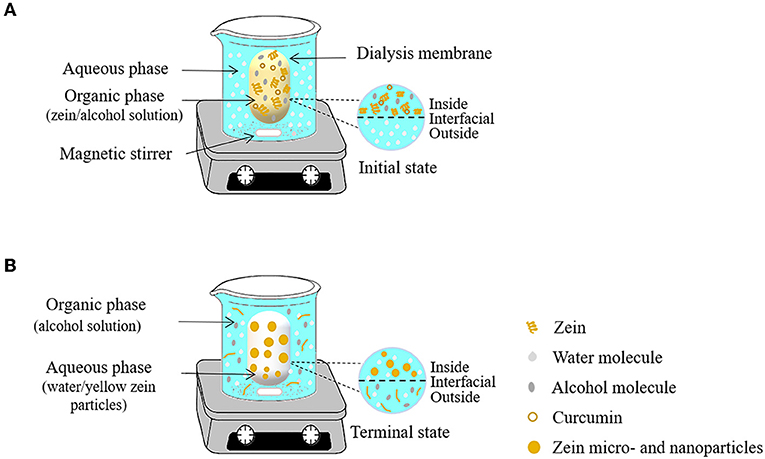
Figure 4. Schematic representation of antisolvent dialysis method for the obtaining of zein micro- and nanoparticles. At time 0 h (A) and after 12 h of the process (B).
pH-driven method
Although the preparation of zein nanoparticles by antisolvent methods is simple and efficient, the method involves a large number of organic solvents, which would bring certain safety risks (flammability) and increase the cost due to the removal of alcohols after the particle formation. To solve these problems, Pan and Zhong innovatively prepared zein nanoparticles based on the solubility of zein in alkaline environment (pH ≥ 11.5) and insolubility in neutral aqueous solution, namely pH driven method, also known as pH cycling or pH transition method (36). Briefly, zein is dissolved in deionized water at pH 7.0, then the pH is adjusted to 12 with NaOH, and then adjusted back to pH 7.0 by HCl. During the pH changing from alkalinity to neutrality, zein is protonated and its solubility decreases gradually and aggregates to form particles. In this process, hydrophobic ingredients are encapsulated inside the particles. In the past few years, the pH-driven method has been widely used to prepare zein composite nanoparticles, including zein-rhamnolipid (37), zein-NaCas (38) and zein-WPI (39) composite nanoparticles. Compounding with other hydrophilic or charged food-grade biopolymers can adjust the aggregation behavior of single zein nanoparticles, thereby improving the stability of zein nanoparticles.
Electrohydrodynamic atomization (EHDA)
EHDA, also known as electrospraying, has been used to prepare inorganic nanoparticles, drug nanoparticles and polymer drug delivery nanoparticles. The technique relies on the separation of liquids into tiny charged droplets under an electric field. The advantage of this technology is that it can produce spherical particles or fibrils with uniform morphology. The morphology and size of particles are seriously affected by processing parameters (e.g., polymer concentration, flow rate, voltage) (40). With the change of processing parameters, the particle size can change from hundreds of microns to several nanometers. The particle size is gradually enlarged with the increase of zein concentration (2.5–15%) (40).
Among the above methods, antisolvent precipitation and supercritical antisolvent method have attracted the attention of researchers owing to their simple operation and large-scale production potential.
Zein-based nanoparticles as delivery system for hydrophobic bioactives
A variety of bioactive substances derived from plants, animals and microorganisms, such as the polyphenols (curcumin, resveratrol, and quercetin), and carotenoids (lycopene, lutein, astaxanthin), and vitamins A, D, E, K, have the wide range of health effects such as antioxidant, anti-inflammatory, anti-cancer and chronic disease prevention. However, these bioactive substances are susceptible to oxidation, crosslinking, or degradation in food processing, storage and digestion, and their absorption and utilization are limited because they are mostly insoluble in water. In order to improve the compatibility of these hydrophobic bioactives with food matrices and to ensure their stability during food processing and high bioavailability after digestion, it is necessary to add them to foods with the help of nano-sized delivery systems with good biocompatibility. Zein is an extremely promising carrier material for delivering hydrophobic bioactivity due to its excellent self-assembly characteristic, which makes it easy to form nanoparticles.
General properties of zein-based nanoparticles
As an amphiphilic prolamin, zein with distinct hydrophobic and hydrophilic domains can self-assemble into nanoparticles, which have been extensively exploited in encapsulation and delivery applications for hydrophobic bioactives. Here, curcumin is used as a model hydrophobic active ingredient to discuss the effect of different zein-based nanoparticles on physicochemical and functional properties of hydrophobic bioactives. Zein nanoparticles (ZNPs) can effectively improve the stability of bioactives and facilitate their targeted delivery. Through antisolvent precipitation, the stability of curcumin encapsulated in ZNPs was improved at all physiologically relevant pH and UV irradiation (30). Moreover, the system has good mucoadhesion property in vitro, and the retention rate of curcumin was more than 60% when it was retained in the gastrointestinal tract for 150 min. The size of curcumin-loaded ZNPs prepared by electrofluid-driven atomization was within 175 to 900 nm, and increased with the increase of zein concentration. After 3 months of storage (23 °C, 43% relative humidity in the dark), no significant changes in the morphology of the nanoparticles or curcumin content were found. These nanoparticles showed good dispersion and coloring capacity in semi-skimmed milk compared to commercial curcumin (40). Furthermore, curcumin-loaded zein nanospheres formulated by antisolvent precipitation method improved liver targeting efficiency and antifibrotic activity of curcumin in a mouse model of carbon tetrachloride-induced liver fibrosis, compared with free curcumin (41).
Zein peptides nanoparticles
Due to the high surface hydrophobicity of zein and its isoelectric point of 6.2, ZNPs without any stabilizer tend to aggregate at neutral pH (42). Therefore, their dispersion stability should be optimized for the delivery application of ZNPs. Proline-rich zein contains a large number of amphiphilic peptides (43). Amphiphilic zein peptide is also a potential delivery system due to its small size and self-assembly property in water solution (43–45). Under chemical or enzymatic treatments, glutamine and asparagine residues of zein undergo a deamidation reaction, which can increase the hydrophilicity of zein (46). Enzymatic method is desirable to modify zein structure. Transglutaminase (TGase), protease, peptidoglutaminase (PGase), glutaminase, and protein glutaminase (PG) can be used for protein deamidation (46). Alcalase-degraded zein peptides tend to self-assemble into micelles, leading to strong hydrophobic complexation with curcumin (44). Without any stabilizer, curcumin-loaded zein peptide nanoparticles exhibited a monodisperse size distribution (<50 nm) and enhanced water solubility (above 8,200-fold vs. free curcumin in water). In addition, this system showed improved storage stability, and more than 60% of curcumin was retained after 72 h of storage at ambient conditions (46). The deamidated zein peptide, produced by alkaline hydrolysis for 36 h, was dissolved together with curcumin in an alkaline aqueous solution and then acidified to induce the co-assembly of the peptide and curcumin to form complex nanoparticles (47). The nanoparticles displayed a curcumin loading capacity of 31.9%, in vitro bioaccessibility of 75%, and a significant antioxidant effect after oral administration in mice.
Zein-based binary composite nanoparticles
The introduction of a biopolymer into the preparation process of ZNPs has been a prominent way to improve the stability and endue new functional properties. The resultant zein composite nanoparticles provide greater encapsulation efficiency (EE), better protection of the encapsulated bioactives from degradation and better controlled release during digestion than ZNPs alone. Zein-based binary composite nanoparticles fabricated with sodium caseinate (48, 49), WPI (6), chitosan (50, 51) and its derivative (52), pectin (53, 54), hyaluronan (55), gum Arabic (56), and carrageenan (57) have been constructed to improve their physicochemical and functional properties.
The addition of amphiphilic protein can reduce the surface hydrophobicity of ZNPs, and enhance their electrostatic and steric repulsion. Zein-caseinate composite nanoparticles were fabricated by a low-energy liquid-liquid dispersion method (48). It was found that the solubility, thermal and UV irradiation stability of encapsulated curcumin were improved, which may be ascribed to the electrostatic and steric repulsion between the zein particles provided by anionic caseinate. Moreover, the cellular studies showed that zein-caseinate nanoparticles with the same level of curcumin exhibited enhanced bioavailability, compared with free curcumin. In another study, WPI-zein composite nanoparticles were fabricated using the pH-driven method (6). Compared with individual WPI nanoparticles or ZNPs, the WPI-zein composite nanoparticles showed improved physicochemical properties. ZNPs aggregated at pH 7.0 and WPI nanoparticles showed relatively poor resistance against high temperature (80 °C).
Many researches also focus on the effects of polysaccharide on the formation, properties and stability of ZNPs. For instance, water-soluble chitosan derivatives (N-(2-hydroxyl)propyl-3-trimethyl ammonium chitosan chloride, HTCC) with different molecular weight were synthesized and used to prepare zein-HTCC nanoparticles (52). HTCC coating was helpful to obtain high EE of curcumin, and EE was positively correlated with the molecular weight of HTCC due to the longer chain of the HTCC molecule could entrap more curcumin. In addition, zein composite nanoparticles with a unique structure were fabricated with chitosan in step-by-step to enhance the loading space of bioactives (51). Curcumin-zein-resveratrol-chitosan composite nanoparticles appeared as the three-dimensional network structure, which was proved to be an effective carrier for greatly improving the physical-, light-, thermal-, and storage stability of both curcumin and resveratrol. Shellac, a resin secreted by the female lac beetle, is insoluble in water but highly soluble in anhydrous ethanol. Zein-shellac binary nanoparticles provided a stronger encapsulation ability of curcumin. After combining shellac, the EE of curcumin increased from 82.7% to 93.2%. Moreover, zein-shellac nanoparticles exhibited improved photochemical, thermal stability and strong curcumin sustained-release ability (in both PBS and simulated gastrointestinal tract), which were ascribed to compact structure induced by binary complexation between zein and shellac (58).
Furthermore, some surfactants can stabilize ZNPs against aggregation by reducing hydrophobic attraction and increasing electrostatic and steric repulsion. Dai et al. (37) successfully prepared zein-rhamnolipid composite nanoparticles using a pH-driven method, which exhibited good stability from pH 3 to 8 and at low salt concentrations (0–100 mM NaCl, pH 7). Nevertheless, they were unstable under extremely acidic conditions or at high ionic strengths due to the suppression of these electrostatic forces. Interestingly, ZNPs stabilized by different levels of surfactants may have different structures, which affect EE of curcumin and related physicochemical properties of nanoparticles. At a relatively low level of lecithin, the nanoparticles tended to aggregate due to embedded alkyl chains of lecithin in the hydrophobic region of zein. With the further increase in lecithin concentration, the strong hydrophobic interaction between the alkyl chain of lecithin with zein and curcumin led to compact structure and smaller size of zein-lecithin composite nanoparticles (59). There are two distinct binding behaviors of curcumin in ZNPs depending on the surfactant concentration (60). At Tween 20 concentration below 0.2 g/L, curcumin was bound in the hydrophobic cavities of the ZNPs; at Tween 20 concentration above 0.2 g/L, some curcumin was also bound in the micellar-like Tween 20 aggregates of the particles.
Cross-linking is another option that may improve the stability and delivery potentials of ZNPs. It is known that zein has some resistance to acid environment and pepsin digestion, but is susceptible to pancreatic enzymes. In terms of the stability under simulated gastric and intestinal conditions, tannic acid cross-linked ZNPs were more resistant against digestion by pancreatin (which was able to degrade α-zein dimers) than non-crosslinked ones (61).
Zein-based ternary composite nanoparticles
Although zein-based binary composite nanoparticles show desirable stability, many reports indicate that the development of ternary composite nanoparticles may be a more promising means of encapsulating, protecting and delivering bioactives. Zein/polysaccharide/surfactant ternary composite nanoparticles were prepared by antisolvent co-precipitation recently. The encapsulation efficiency of curcumin in zein-propylene glycol alginate-rhamnolipid (or lecithin) ternary composite nanoparticles was higher than that in pure zein or binary zein-propylene glycol alginate nanoparticles. Moreover, the presence of the surfactants significantly improved bioaccessibility of curcumin (62). In another study, ternary composite nanoparticles consisting of zein, chondroitin sulfate (CS) and sophorolipid enhanced EE, chemical stability, aqueous solubility and anticancer activity in vitro against HepG2, MCF-7 and HeLa cells of curcumin, compared with ZNPs and zein-CS nanoparticles (63), which were attributed to amphiphilic Spl. Its hydrophilic disaccharide sophorose could produce hydrogen bonding with –OH of CS, and its lipophilic long monounsaturated fatty acid chain could absorb on the hydrophobic region of zein by hydrophobic interaction. Zein/polysaccharide/protein ternary composite nanoparticles have also been extensively explored. The electrostatic biopolymer coatings consisting of a mixture of sodium alginate (70%) and fish gelatin (30%) improved the pH, salt, and thermal stability of ZNPs, and produced a high bioaccessibility of curcumin in vitro and antioxidant capacity (64). The re-dispersibility of the freeze-dried nanoparticles was enhanced due to hydrophilic sodium caseinate and sodium alginate adsorbed sufficiently on the surface of the ZNPs, resulting in a decrease in zein surface hydrophobicity (65).
In the ternary composite nanoparticles, the type of polysaccharide critically influences the stability of the system. Partial replacement of the pectin (low charge density) with alginate (high charge density) to form the shell in the ternary composite nanoparticles improved aggregation stability at pH 5 to 7 and at high ionic strengths (2,000 mM NaCl), and the encapsulated curcumin exhibited better antioxidant activity (66). In another study, ternary composite nanoparticles composed of zein, hyaluronic acid and chitosan were prepared using the layer-by-layer technique to co-deliver curcumin and piperine, the nanoparticles were able to control the release of the bioactive ingredients in simulated gastrointestinal conditions and protected them from chemical degradation when exposed to UV light, high temperature, or long-term storage by manipulating the number of layers (67). Chitosan with lower molecular weight is a suitable outer coating to protect and control the release rate of both bioactive ingredients during simulated digestion than either medium- or high-molecular weight chitosan. In addition, crosslinking can significantly influence the release sustainability of active ingredients by modifying carrier microstructures (19). Caseinate-zein-pectin composite nanoparticles were prepared via a pH- and heating-induced electrostatic deposition, and 1-ethyl-3-(3-dimethyl aminopropyl) carbodiimide and N-hydroxysuccinimide (EDC/NHS) as chemical cross-linkers were used to covalently bridge the protein and polysaccharide layers. The obtained nanoparticles showed improved stability under simulated gastrointestinal conditions (68). Oxidized dextran as a macromolecular crosslinker and coating can also successfully apply to stabilize the zein-caseinate composite nanoparticles, which not only enhanced the colloidal stability of composite nanoparticles but also improved the controlled release rate of curcumin under simulated gastrointestinal conditions (69). We summarize the physicochemical properties of different zein nanoparticles loaded with hydrophobic bioactives consisting of zein with proteins, polysaccharides, polyphenols, and surfactants reported in recent years (Supplementary Table S1, Supporting Information).
Other Zein-based nanocarriers as delivery systems for hydrophobic bioactives
In addition to zein-based nanoparticles detailed above, nanofibers, nanomicelles, nanogels based on zein are excellent candidates as delivery systems for bioactives (Figure 5).

Figure 5. Schematic presentation of common zein-based nanosized delivery systems including nanoparticles (A), nanofibers (B), nanomicelles (C), nanogels (D), nanoemulsions (E).
Zein-based nanofibers
Electrospinning is a unique and cost-effective route to prepare nanofibers, and large surface area-to-volume ratio nanofibers can be provided by adjusting the preparation parameters (electrostatic field strength, flow rate, solution concentration, etc.,). Ultrafine zein fluorescence nanofibers loaded with curcumin were developed by electrospinning (70). It was found the diameter of zein nanofibers increased slightly from 250 nm to 300 nm with the increase of the concentration of embedded curcumin (0–10%), the morphology and distribution of nanofibers did not change significantly, and curcumin aggregates were also not found in fibers surfaces, indicating curcumin was well-embedded within the fibers. It was also confirmed in the study of Deng et al. (71) that the embedded bioactive ingredient did not affect the appearance and morphology of nanofibers. However, pure zein nanofibers have poor mechanical properties and solvent resistance (72). Hybrid electrospinning of zein and other biopolymers has been considered as an effective mean to remedy these deficiencies. It has been reported that hybrid electrospinning of zein and gelatin enhanced the mechanical properties of pure zein nanofibers, elongation at break and elastic modulus were up to 87%, 72.1 MPa respectively (73). Additionally, both the glucose cross-linked gelatin/zein nanofibers and zein/tragacanth nanofibers with core-shell structure showed good sustained release properties (71, 74). Therefore, zein-based nanofibers prepared by electrospinning technology could be a potential candidate for delivering hydrophobic bioactives.
In addition, curcumin-loaded zein nanofibers exhibited excellent antibacterial activity, and the inhibition efficiency against food-borne pathogens increased with the increase of curcumin content (75–78). In addition, curcumin-loaded nanofibril films prepared by dispersing electrospun zein into konjac and glucomannan solution exhibited better hydrophobicity, water resistance property and excellent antibacterial activity, which can be used as food packaging materials (38).
Zein-based nanomicelles
Polymer micelles are also important nano-sized delivery systems, formed through spontaneous self-assembly of amphiphilic block copolymers into specific supramolecularly ordered aggregates for improving stability and bioavailability of active ingredients (79). In aqueous solution, polymer self-assembles into a core-shell architecture, in which the hydrophobic segment forms the core and the hydrophilic segment forms the external corona, when the copolymer concentration approaches the critical micelle concentration (80). The hydrophobic active ingredients can be located in the micelle core regardless of inclusion or bonding, and the hydrophilic shell of the micelles increases the water solubility of the hydrophobic active ingredients. Many amphiphilic materials have been used to synthesize micelles, including synthetic polymers (81–83) and natural polymers (84–86).
Naturally accessible proteins and polysaccharides are attractive alternatives to synthetic polymers due to their good biocompatibility and low cost. Among them, zein with inherent hydrophobic regions have been widely discussed. In a previous study, methoxy polyethylene glycol grafted α-zein micelles (mPEG-g-α-zein) exhibited a sustained release effect of curcumin and low cytotoxicity (87). Although some PEG-modified products have been approved by FDA and other regulatory agencies and successfully used in the clinic, some researchers are still worried about whether it will cause subsequent toxicity and other side effects after long-term accumulation in the body (88). For this reason, zein-lactoferrin micelles were prepared via carbodiimide coupling reaction between the primary amine of zein and the carboxyl group of lactoferrin, which greatly enhanced the tumor targeting of the loaded drug (89). The effect could be attributed to: (i) the hydrophobic zein core in micelles had high drug loading; (ii) the hydrophilic lactoferrin shell enhanced the stability of micelles; (iii) lactoferrin provided the tumor targeting (90, 91). Similarly, the hydrophilic polysaccharide chondroitin sulfate (ChS) with the CD44-mediated tumor targeting effect was also used to prepared zein-ChS micelles (92). In addition, superhydrophilic zwitterionic polymer, poly(sulfobetaine methacrylate) (PSBMA) was also used to synthesize zein-PSBMA micelles. The micelles significantly improved the water solubility of curcumin, and curcumin loaded in micelles exhibited better stability, cellular uptake, cytotoxicity against cancer cells compared with native curcumin (93). Therefore, the amphiphilic zein-based micelles have great potential for delivering bioactives due to their outstanding stability and targeting capability.
Others
Nanogel is a nanosized hydrogel, and it possess the features of hydrogels and nanoparticles, which is an excellent active ingredient delivery system with high loading and controlled release performance (94, 95). Zein-hyaluronic acid nanogels loaded with curcumin exhibited high anticancer activity against the CT26 colorectal cancer cells due to the enhanced targetability to CD44 receptor by the presence of hyaluronic acid (96). On the other hand, all these nano-sized zein materials could be used as emulsifiers to construct stable nanoemulsions for delivering curcumin, either oil-in-water single or multi-layer nanoemulsions (70, 97, 98).
Zein-based hybrid nano-delivery systems
Nano-sized delivery systems such as nanoparticles, nanofibers, nanomicelles, nanogels and nanoemulsions have been proved to possess several protective functional advantages for bioactives. Comparatively, zein-based nanoparticles have higher barrier properties than oil-in-water nanoemulsion in limiting peroxyl radical-induced oxidation processes of encapsulated curcumin (99). However, these two encapsulation systems were ineffectiveness in limiting oxygen permeation. In a comparative study of improving curcumin bioavailability by three potential delivery systems (ZNPs, nanoemulsions, and phospholipid nanoparticles), ZNPs were able to incorporate the highest level of curcumin per unit mass of particles. Nevertheless, nanoemulsions appeared to be the most effective at promoting both the chemical stability and solubilization of curcumin at an equal initial curcumin level, under simulated gastrointestinal conditions (100). Chuacharoen and Sabliov (101) also synthesized nanoemulsions and nanoparticles with entrapped curcumin at the same level of surfactant concentration. Compared with nanoemulsions, zein-based nanoparticles were found to be the better carriers based on their storage stability and sustained release profile.
To fully utilize the advantages of different systems, is it possible to achieve better encapsulation and delivery of hydrophobic bioactives if two or more systems are combined? The advantages and disadvantages of different nano-sized delivery systems are summarized in Table 2. Reportedly, mixing curcumin-loaded ZNPs with lipid nanoparticles can increase the bioaccessibility and chemical stability of lipophilic bioactive agents (100). ZNPs can be designed to protect the curcumin from chemical degradation, whereas another type of nanoparticles (like lipid nanoparticles) can be designed to rapidly digest within the gastrointestinal tract and form mixed micelles that can solubilize and transport the hydrophobic curcumin. In a another study (102), zein-EGCG conjugate nanoparticles (Figure 6A) aggregated in water solution due to hydrophobic interaction of protein in the absence of surfactant (Figure 6B). However, mixing the nanoparticles with rhamnolipids vesicles formed uniform and stable bioactive-loaded colloids nanoparticles due to rhamnolipids adsorption and hydrophobic effect around the protein nanoparticles (Figure 6C). Furthermore, the mixed colloidal delivery system improved the digestibility of the nanoparticles and bioaccessibility of curcumin and resveratrol.
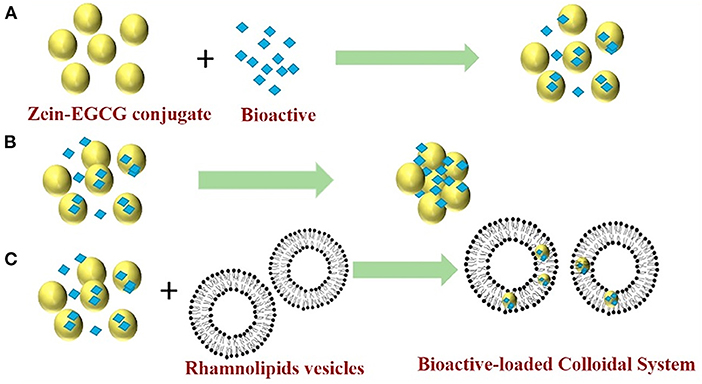
Figure 6. Proposed mechanisms for a mixed colloidal delivery system consisting of zein-EGCG conjugate nanoparticles and rhamnolipids vesicles. Zein-EGCG conjugate interacts with bioactive and self-assembles to form nanoparticles that embedded bioactive inside by antisolvent (A); In the absence of surfactant, the nanoparticles aggregate due to hydrophobic interactions of zein in water solution (B); In the presence of rhamnolipids vesicles, the nanoparticles do not aggregate because the coating of surfactant reduces hydrophobic interactions and increases the electrostatic and steric effects between nanoparticles (C). Reprinted from (102) with permission from Elsevier.
Conclusions and future trends for zein-based nano-sized carriers
Zein is considered as an ideal biopolymer for constructing nanoscale carriers owing to its intrinsic hydrophobic and self-assembly properties. Developing zein nanocarriers can provide controlled release possibilities and enhance the oral bioavailability of hydrophobic bioactives. The colloid stability of zein-based delivery system can be improved using chemical crosslinking, coating and complexation by introducing other biopolymers (e.g., proteins, polysaccharide, phenols, surfactant). Most of these modification strategies can further enhance the encapsulation and delivery efficiency of bioactives.
Nevertheless, some issues remain to be considered for future study: (i) The appropriate loading level of nutrient and the actual sensory properties of these carriers for commercial foods should be determined; (ii) Various factors introduced in the process of carrier construction should be carefully considered, such as the involvement of toxic organic solvents; (iii) Whether the effects of the observations would also occur under more realistic physiological gastrointestinal conditions should be determined via using animal or human feeding models. In the future, zein based delivery systems will be designed to be functional and even more complex based on specific needs. More studies will focus on elucidating the efficacy and toxicity of these carriers as well as the biological fate of oral administration under physiological conditions. The preparation process requires more work based on a comprehensive analysis not only of their reproducibility, biocompatibility and immunogenicity, but also their scalability, storage stability and cost.
Author contributions
XY: writing—original draft preparation and revision. ML: writing—original draft preparation. XX and XL: project administration. FL: writing—review and editing, project administration, and funding acquisition. All authors contributed to the article and approved the submitted version.
Funding
This research was supported by Innovation Talents Promotion Plan of Shaanxi Province [No. 2020KJXX-034], the Fundamental Research Funds for the Central Universities [No. 2452020008], and the Breeding Plan of Shandong Provincial Qingchuang Research Team (2021-Innovation Team of Functional Plant Protein-Based Food).
Conflict of interest
The authors declare that the research was conducted in the absence of any commercial or financial relationships that could be construed as a potential conflict of interest.
Publisher's note
All claims expressed in this article are solely those of the authors and do not necessarily represent those of their affiliated organizations, or those of the publisher, the editors and the reviewers. Any product that may be evaluated in this article, or claim that may be made by its manufacturer, is not guaranteed or endorsed by the publisher.
Supplementary material
The Supplementary Material for this article can be found online at: https://www.frontiersin.org/articles/10.3389/fnut.2022.999373/full#supplementary-material
References
1. Hamaker B, Mohamed A, Habben J, Huang C, Larkins B. Efficient procedure for extracting maize and sorghum kernel proteins reveals higher prolamin contents than the conventional method. Cereal Chem. (1995) 72:583–8.
2. Patel AR. Functional and engineered colloids from edible materials for emerging applications in designing the food of the future. Adv Funct Mater. (2020) 30:1806809. doi: 10.1002/adfm.201806809
3. Wang T, Zhang D, Sun D, Gu J. Current status of in vivo bioanalysis of nano drug delivery systems. J Pharm Sci. (2020) 10:221–32. doi: 10.1016/j.jpha.2020.05.002
4. Yu X, Wu H, Hu H, Dong Z, Dang Y, Qi Q, et al. Zein nanoparticles as nontoxic delivery system for maytansine in the treatment of non-small cell lung cancer. Drug Deliv. (2020) 27:100–9. doi: 10.1080/10717544.2019.1704942
5. Wang H, Hao L, Wang P, Chen M, Jiang S, Jiang S. Release kinetics and antibacterial activity of curcumin loaded zein fibers. Food Hydrocoll. (2017) 63:437–46. doi: 10.1016/j.foodhyd.2016.09.028
6. Zhan X, Dai L, Zhang L, Gao Y. Entrapment of curcumin in whey protein isolate and zein composite nanoparticles using pH-driven method. Food Hydrocoll. (2020) 106:105839. doi: 10.1016/j.foodhyd.2020.105839
7. Chen S, Han Y, Huang J, Dai L, Du J, McClements DJ, et al. Fabrication and characterization of layer-by-layer composite nanoparticles based on zein and hyaluronic acid for codelivery of curcumin and quercetagetin. Appl Mater Inter. (2019) 11:16922–33. doi: 10.1021/acsami.9b02529
8. Li H, Yuan Y, Zhu J, Wang T, Wang D, Xu Y. Zein/soluble soybean polysaccharide composite nanoparticles for encapsulation and oral delivery of lutein. Food Hydrocoll. (2020) 103:105715. doi: 10.1016/j.foodhyd.2020.105715
9. Rodríguez-Félix F, Del-Toro-Sánchez CL, Javier Cinco-Moroyoqui F, Juárez J, Ruiz-Cruz S, López-Ahumada GA, et al. Preparation and characterization of quercetin-loaded zein nanoparticles by electrospraying and study of in vitro bioavailability. J Food Sci. (2019) 84:2883–97. doi: 10.1111/1750-3841.14803
10. Ren X, Hou T, Liang Q, Zhang X, Hu D, Xu B, et al. Effects of frequency ultrasound on the properties of zein-chitosan complex coacervation for resveratrol encapsulation. Food Chem. (2019) 279:223–30. doi: 10.1016/j.foodchem.2018.11.025
11. Gali L, Bedjo F, Ferrari G, Donsì F. Formulation and characterization of zein/gum arabic nanoparticles for the encapsulation of a rutin-rich extract from Ruta chalepensis L. Food Chem. (2022) 367:129982. doi: 10.1016/j.foodchem.2021.129982
12. Wang M, Fu Y, Chen G, Shi Y, Li X, Zhang H, et al. Fabrication and characterization of carboxymethyl chitosan and tea polyphenols coating on zein nanoparticles to encapsulate β-carotene by anti-solvent precipitation method. Food Hydrocoll. (2018) 77:577–87. doi: 10.1016/j.foodhyd.2017.10.036
13. Luo Y, Teng Z, Wang Q. Development of zein nanoparticles coated with carboxymethyl chitosan for encapsulation and controlled release of vitamin D3. J Agri Food Chem. (2012) 60:836–43. doi: 10.1021/jf204194z
14. Park CE, Park DJ, Kim BK. Effects of a chitosan coating on properties of retinol-encapsulated zein nanoparticles. Food Sci Biotechnol. (2015) 24:1725–33. doi: 10.1007/s10068-015-0224-7
15. Shukla R, Cheryan M. Zein: the industrial protein from corn. Ind Crops Prod. (2001) 13:171–92. doi: 10.1016/S0926-6690(00)00064-9
16. Sun C, Gao Y, Zhong Q. Effects of acidification by glucono-delta-lactone or hydrochloric acid on structures of zein-caseinate nanocomplexes self-assembled during a pH cycle. Food Hydrocoll. (2018) 82:173–85. doi: 10.1016/j.foodhyd.2018.04.007
17. Zhang H, Fu Y, Xu Y, Niu F, Li Z, Ba C, et al. One-step assembly of zein/caseinate/alginate nanoparticles for encapsulation and improved bioaccessibility of propolis. Food Funct. (2019) 10:635–45. doi: 10.1039/C8FO01614C
18. Kasaai MR. Zein and zein-based nano-materials for food and nutrition applications: a review. Trends Food Sci Technol. (2018) 79:184–97. doi: 10.1016/j.tifs.2018.07.015
19. Zhang Y, Cui L, Li F, Shi N, Li C, Chen Y, et al. Design, fabrication and biomedical applications of zein-based nano/micro-carrier systems. Int J Pharm. (2016) 513:191–210. doi: 10.1016/j.ijpharm.2016.09.023
20. Argos P, Pedersen K, Marks MD, Larkins BA. A structural model for maize zein proteins. J Biol Chem. (1982) 257:9984–90. doi: 10.1016/S0021-9258(18)33974-7
21. Matsushima N, Danno GI, Takezawa H, Izumi Y. Three-dimensional structure of maize α-zein proteins studied by small-angle X-ray scattering. BBA-Protein Struct M. (1997) 1339:14–22. doi: 10.1016/S0167-4838(96)00212-9
22. Forato LA, Doriguetto AC, Fischer H, Mascarenhas YP, Craievich AF, Colnago LA. Conformation of the Z19 prolamin by FTIR, NMR, and SAXS. J Agri Food Chem. (2004) 52:2382–5. doi: 10.1021/jf035020+
23. Momany FA, Sessa DJ, Lawton JW, Selling GW, Hamaker SAH, Willett JL. Structural characterization of alpha-zein. J Agri Food Chem. (2006) 54:543–7. doi: 10.1021/jf058135h
24. Li Y, Li J, Xia Q, Zhang B, Wang Q, Huang Q. Understanding the dissolution of alpha-zein in aqueous ethanol and acetic acid solutions. J Phys Chem B. (2012) 116:12057–64. doi: 10.1021/jp305709y
25. Kyte J, Doolittle RF. A simple method for displaying the hydropathic character of a protein. J Mol Biol. (1982) 157:105–32. doi: 10.1016/0022-2836(82)90515-0
26. Katayama H, Kanke M. Drug release from directly compressed tablets containing zein. Drug Dev Ind Pharm. (1992) 18:2173–84. doi: 10.3109/03639049209038755
27. Matsuda Y, Suzuki T, Sato E, Sato M, Koizumi S, Unno K, et al. Novel preparation of zein microspheres conjugated with PS-K available for cancer immunotherapy. Chem Pharm Bull. (1989) 37:757–9. doi: 10.1248/cpb.37.757
28. Suzuki T, Sato E, Matsuda Y, Tada H, Unno K, Kato T. Preparation of zein microspheres conjugated with antitumor drugs available for selective cancer chemotherapy and development of a simple colorimetric determination of drugs in microspheres. Chem Pharm Bull. (1989) 37:1051–4. doi: 10.1248/cpb.37.1051
29. Karthikeyan K, Lakra R, Rajaram R, Korrapati PS. Development and characterization of zein-based micro carrier system for sustained delivery of aceclofenac sodium. AAPS PharmSciTech. (2012) 13:143–9. doi: 10.1208/s12249-011-9731-x
30. Patel AR, Hu Y, Tiwari JK, Velikov KP. Synthesis and characterisation of zein-curcumin colloidal particles. Soft Matter. (2010) 6:6192–9. doi: 10.1039/c0sm00800a
31. Zhong Q, Jin M, Xiao D, Tian H, Zhang W. Application of supercritical anti-solvent technologies for the synthesis of delivery systems of bioactive food components. Food Biophys. (2008) 3:186–90. doi: 10.1007/s11483-008-9059-6
32. Jung J, Perrut M. Particle design using supercritical fluids: Literature and patent survey. J Supercrit Fluid. (2001) 20:179–219. doi: 10.1016/S0896-8446(01)00064-X
33. Zhong Q, Jin M, Davidson PM, Zivanovic S. Sustained release of lysozyme from zein microcapsules produced by a supercritical anti-solvent process. Food Chem. (2009) 115:697–700. doi: 10.1016/j.foodchem.2008.12.063
34. Hu D, Lin C, Liu L, Li S, Zhao Y. Preparation, characterization, and in vitro release investigation of lutein/zein nanoparticles via solution enhanced dispersion by supercritical fluids. J Food Eng. (2012) 109:545–52. doi: 10.1016/j.jfoodeng.2011.10.025
35. Rodríguez-Félix F, Del-Toro-Sanchez CL, Tapia-Hernandez JA A new design for obtaining of white zein micro- and nanoparticles powder: antisolvent-dialysis method. Food Sci Biotechnol. (2019) 29:619–29. doi: 10.1007/s10068-019-00702-9
36. Pan K, Zhong Q. Low energy, organic solvent-free co-assembly of zein and caseinate to prepare stable dispersions. Food Hydrocoll. (2016) 52:600–6. doi: 10.1016/j.foodhyd.2015.08.014
37. Dai L, Zhou H, Wei Y, Gao Y, McClements DJ. Curcumin encapsulation in zein-rhamnolipid composite nanoparticles using a pH-driven method. Food Hydrocoll. (2019) 93:342–50. doi: 10.1016/j.foodhyd.2019.02.041
38. Wang L, Xue J, Zhang Y. Preparation and characterization of curcumin loaded caseinate/zein nanocomposite film using pH-driven method. Ind Crops Prod. (2019) 130:71–80. doi: 10.1016/j.indcrop.2018.12.072
39. Zhou D, Li S, Pei M, Yang H, Gu S, Tao Y, et al. Dopamine-modified hyaluronic acid hydrogel adhesives with fast-forming and high tissue adhesion. ACS Appl Mater Inter. (2020) 12:18225–34. doi: 10.1021/acsami.9b22120
40. Gomez-Estaca J, Balaguer MP, Gavara R, Hernandezmunoz P. Formation of zein nanoparticles by electrohydrodynamic atomization: effect of the main processing variables and suitability for encapsulating the food coloring and active ingredient curcumin. Food Hydrocoll. (2012) 28:82–91. doi: 10.1016/j.foodhyd.2011.11.013
41. Algandaby MM, Al-Sawahli MM, Ahmed OAA, Fahmy UA, Abdallah HM, Hattori M, et al. Curcumin-zein nanospheres improve liver targeting and antifibrotic activity of curcumin in carbon tetrachloride-induced mice liver fibrosis. J Biomed Nanotechnol. (2016) 12:1746–57. doi: 10.1166/jbn.2016.2270
42. Cheng CJ, Ferruzzi M, Jones OG. Fate of lutein-containing zein nanoparticles following simulated gastric and intestinal digestion. Food Hydrocoll. (2019) 87:229–36. doi: 10.1016/j.foodhyd.2018.08.013
43. Fernández-Carneado J, Kogan MJ, Castel S, Giralt E. Potential peptide carriers: Amphipathic proline-rich peptides derived from the N-terminal domain of γ-zein. Angew Chem Int Ed. (2004) 43:1811–4. doi: 10.1002/anie.200352540
44. Wang Y, Wang J, Yang X, Guo J, Lin Y. Amphiphilic zein hydrolysate as a novel nano-delivery vehicle for curcumin. Food Funct. (2015) 6:2636–45. doi: 10.1039/C5FO00422E
45. Pan R, Zou Y, Wang J, Wan Z, Guo J, Yang J, et al. Gamma/alpha-zein hydrolysates as oral delivery vehicles: enhanced physicochemical stability and in vitro bioaccessibility of curcumin. Int J Food Sci Tech. (2018) 53:1622–30. doi: 10.1111/ijfs.13744
46. Cabra V, Arreguin R, Vazquez-Duhalt R, Farres A. Effect of alkaline deamidation on the structure, surface hydrophobicity, and emulsifying properties of the Z19 α-zein. J Agri Food Chem. (2007) 55:439–45. doi: 10.1021/jf061002r
47. Li L, Yao P. High dispersity, stability and bioaccessibility of curcumin by assembling with deamidated zein peptide. Food Chem. (2020) 319:126577. doi: 10.1016/j.foodchem.2020.126577
48. Xue J, Zhang Y, Huang G, Liu J, Slavin M, Yu L. Zein-caseinate composite nanoparticles for bioactive delivery using curcumin as a probe compound. Food Hydrocoll. (2018) 83:25–35. doi: 10.1016/j.foodhyd.2018.04.037
49. Yan X, Zhang X, McClements DJ, Zou L, Liu X, Liu F. Co-encapsulation of epigallocatechin gallate (EGCG) and curcumin by two proteins-based nanoparticles: Role of EGCG. J Agri Food Chem. (2019) 67:13228–36. doi: 10.1021/acs.jafc.9b04415
50. Li M, Chen L, Xu M, Zhang J, Wang Q, Zeng Q, et al. The formation of zein-chitosan complex coacervated particles: Relationship to encapsulation and controlled release properties. Int J Biol Macromol. (2018) 116:1232–9. doi: 10.1016/j.ijbiomac.2018.05.107
51. Chen S, Han Y, Jian L, Liao W, Zhang Y, Gao Y. Fabrication, characterization, physicochemical stability of zein-chitosan nanocomplex for co-encapsulating curcumin and resveratrol. Carbohyd Polym. (2020) 236:116090. doi: 10.1016/j.carbpol.2020.116090
52. Liang H, Zhou B, He L, An Y, Lin L, Li Y, et al. Fabrication of zein/quaternized chitosan nanoparticles for the encapsulation and protection of curcumin. RSC Adv. (2015) 5:13891–900. doi: 10.1039/C4RA14270E
53. Hu K, Huang X, Gao Y, Huang X, Xiao H, McClements DJ. Core-shell biopolymer nanoparticle delivery systems: Synthesis and characterization of curcumin fortified zein-pectin nanoparticles. Food Chem. (2015) 182:275–81. doi: 10.1016/j.foodchem.2015.03.009
54. Cai T, Xiao P, Yu N, Zhou Y, Mao J, Peng H, et al. A novel pectin from Akebia trifoliata var. australis fruit peel and its use as a wall-material to coat curcumin-loaded zein nanoparticle. Int J Biol Macromol. (2020) 152:40–9. doi: 10.1016/j.ijbiomac.2020.02.234
55. Chen S, Han Y, Sun C, Dai L, Yang S, Wei Y, et al. Effect of molecular weight of hyaluronan on zein-based nanoparticles: Fabrication, structural characterization and delivery of curcumin. Carbohyd Polym. (2018) 201:599–607. doi: 10.1016/j.carbpol.2018.08.116
56. Chen G, Fu Y, Niu F, Zhang H, Li X, Li X. Evaluation of the colloidal/chemical performance of core-shell nanoparticle formed by zein and gum Arabic. Colloid Surface A. (2019) 560:130–5. doi: 10.1016/j.colsurfa.2018.10.006
57. Chen S, Li Q, Julian D, Han Y, Dai L, Mao L, et al. Co-delivery of curcumin and piperine in zein-carrageenan core-shell nanoparticles: Formation, structure, stability and in vitro gastrointestinal digestion. Food Hydrocoll. (2020) 99:105334. doi: 10.1016/j.foodhyd.2019.105334
58. Sun C, Xu C, Mao L, Wang D, Yang J, Gao Y. Preparation, characterization and stability of curcumin-loaded zein-shellac composite colloidal particles. Food Chem. (2017) 228:656–67. doi: 10.1016/j.foodchem.2017.02.001
59. Dai L, Sun C, Li R, Mao L, Liu F, Gao Y. Structural characterization, formation mechanism and stability of curcumin in zein-lecithin composite nanoparticles fabricated by antisolvent co-precipitation. Food Chem. (2017) 237:1163–71. doi: 10.1016/j.foodchem.2017.05.134
60. Wang X, Huang H, Chu X, Han Y, Li M, Li G, et al. Encapsulation and binding properties of curcumin in zein particles stabilized by Tween 20. Colloid Surface A. (2019) 577:274–80. doi: 10.1016/j.colsurfa.2019.05.094
61. Hu S, Wang T, Fernandez ML, Luo Y. Development of tannic acid cross-linked hollow zein nanoparticles as potential oral delivery vehicles for curcumin. Food Hydrocoll. (2016) 61:821–31. doi: 10.1016/j.foodhyd.2016.07.006
62. Dai L, Wei Y, Sun C, Mao L, McClements DJ, Gao Y. Development of protein-polysaccharide-surfactant ternary complex particles as delivery vehicles for curcumin. Food Hydrocoll. (2018) 85:75–85. doi: 10.1016/j.foodhyd.2018.06.052
63. Yuan Y, Ma M, Zhang S, Liu C, Chen P, Li H, et al. Effect of sophorolipid on the curcumin-loaded ternary composite nanoparticles self-assembled from zein and chondroitin sulfate. Food Hydrocoll. (2021) 113:106493. doi: 10.1016/j.foodhyd.2020.106493
64. Liu Q, Jing Y, Han C, Zhang H, Tian Y. Encapsulation of curcumin in zein/caseinate/sodium alginate nanoparticles with improved physicochemical and controlled release properties. Food Hydrocoll. (2019) 93:432–42. doi: 10.1016/j.foodhyd.2019.02.003
65. Yao K, Chen W, Song F, McClements DJ, Hu K. Tailoring zein nanoparticle functionality using biopolymer coatings: Impact on curcumin bioaccessibility and antioxidant capacity under simulated gastrointestinal conditions. Food Hydrocoll. (2018) 79:262–72. doi: 10.1016/j.foodhyd.2017.12.029
66. Huang X, Huang X, Gong Y, Xiao H, McClements DJ. Hu, K. Enhancement of curcumin water dispersibility and antioxidant activity using core-shell protein-polysaccharide nanoparticles. Food Res Int. (2016) 87:1–9. doi: 10.1016/j.foodres.2016.06.009
67. Chen S, McClements DJ, Jian L, Han Y, Dai L, Mao L, et al. Core-shell biopolymer nanoparticles for co-delivery of curcumin and piperine: sequential electrostatic deposition of hyaluronic acid and chitosan shells on the zein core. ACS Appl Mater Inter. (2019) 11:38103–15. doi: 10.1021/acsami.9b11782
68. Chang C, Wang T, Hu Q, Luo Y. Caseinate-zein-polysaccharide complex nanoparticles as potential oral delivery vehicles for curcumin: effect of polysaccharide type and chemical cross-linking. Food Hydrocoll. (2017) 72:254–62. doi: 10.1016/j.foodhyd.2017.05.039
69. Rodriguez NJ, Hu Q, Luo Y. Oxidized dextran as a macromolecular crosslinker stabilizes the zein/caseinate nanocomplex for the potential oral delivery of curcumin. Molecules. (2019) 24:4061. doi: 10.3390/molecules24224061
70. Brahatheeswaran D, Mathew A, Aswathy RG, Nagaoka Y, Venugopal K, Yoshida Y, et al. Hybrid fluorescent curcumin loaded zein electrospun nanofibrous scaffold for biomedical applications. Biomed Mater. (2012) 7:045001. doi: 10.1088/1748-6041/7/4/045001
71. Deng L, Li Y, Feng F, Wu D, Zhang H. Encapsulation of allopurinol by glucose cross-linked gelatin/zein nanofibers: Characterization and release behavior. Food Hydrocoll. (2019) 94:574–84. doi: 10.1016/j.foodhyd.2019.04.004
72. Zhang Y, Cui L, Chen Y, Zhang H, Zhong J, Sun Y, et al. Zein-based nanofibres for drug delivery: classes and current applications. Curr Pharm Design. (2015) 21:3199–207. doi: 10.2174/1381612821666150531170448
73. Deng L, Zhang X, Li Y, Que F, Kang X, Liu Y, et al. Characterization of gelatin/zein nanofibers by hybrid electrospinning. Food Hydrocoll. (2018) 75:72–80. doi: 10.1016/j.foodhyd.2017.09.011
74. Dehcheshmeh MA, Fathi M. Production of core-shell nanofibers from zein and tragacanth for encapsulation of saffron extract. Int J Biol Macromol. (2019) 122:272–9. doi: 10.1016/j.ijbiomac.2018.10.176
75. Bui HT, Chung OH, Park JS. Fabrication of electrospun antibacterial curcumin-loaded zein nanofibers. Polym Korea. (2014) 38:744–51. doi: 10.7317/pk.2014.38.6.744
76. Yilmaz A, Bozkurt F, Cicek PK, Dertli E, Durak MZ, Yilmaz MT, et al. novel antifungal surface-coating application to limit postharvest decay on coated apples: Molecular, thermal and morphological properties of electrospun zein-nanofiber mats loaded with curcumin. Innov Food Sci Emerg. (2016) 37:74–83. doi: 10.1016/j.ifset.2016.08.008
77. Wang L, Zhang Y. Eugenol nanoemulsion stabilized with zein and sodium caseinate by self-assembly. J Agri Food Chem. (2017) 65:2990–8. doi: 10.1021/acs.jafc.7b00194
78. Akrami-Hasan-Kohal M, Tayebi L, Ghorbani M. Curcumin-loaded naturally-based nanofibers as active wound dressing mats: Morphology, drug release, cell proliferation, and cell adhesion studies. New J Chem. (2020) 44:10343–51. doi: 10.1039/D0NJ01594F
79. Bu X, Ji N, Dai L, Dong X, Chen M, et al. Self-assembled micelles based on amphiphilic biopolymers for delivery of functional ingredients. Trends Food Sci Technol. (2021) 114:386–98. doi: 10.1016/j.tifs.2021.06.001
80. Sabra S, Abdelmoneem M, Abdelwakil M, Mabrouk MT, Anwar D, Mohamed R, et al. Self-assembled nanocarriers based on amphiphilic natural polymers for anti-cancer drug delivery applications. Curr Pharm Design. (2017) 23:5213–29. doi: 10.2174/1381612823666170526111029
81. Guan J, Zhou Z, Chen M, Li H, Tong D, Yang J, et al. Folate-conjugated and pH-responsive polymeric micelles for target-cell-specific anticancer drug delivery. Acta Biomater. (2017) 60:244–55. doi: 10.1016/j.actbio.2017.07.018
82. Li Q, Yao W, Yu X, Zhang B, Dong J, Jin Y. Drug-loaded pH-responsive polymeric micelles: Simulations and experiments of micelle formation, drug loading and drug release. Colloid Surface B. (2017) 158:709–16. doi: 10.1016/j.colsurfb.2017.07.063
83. Yotsumoto K, Ishii K, Kokubo M, Yasuoka S. Improvement of the skin penetration of hydrophobic drugs by polymeric micelles. Int J Pharm. (2018) 553:132–40. doi: 10.1016/j.ijpharm.2018.10.039
84. Di Prima G, Licciardi M, Bongiovì F, Pitarresi G, Giammona G. Inulin-based polymeric micelles functionalized with ocular permeation enhancers: improvement of dexamethasone permeation/penetration through bovine corneas. Pharmaceutics. (2021) 13:1431. doi: 10.3390/pharmaceutics13091431
85. Ranadheera CS, Liyanaarachchi WS, Dissanayake M, Chandrapala J, Huppertz T, Vasiljevic T. Impact of shear and pH on properties of casein micelles in milk protein concentrate. LWT-Food Sci Technol. (2019) 108:370–6. doi: 10.1016/j.lwt.2019.03.090
86. Šmejkalová D, Muthný T, Nešporová K, Hermannová M, Achbergerová E, Huerta-Angeles G, et al. Hyaluronan polymeric micelles for topical drug delivery. Carbohyd Polym. (2017) 156:86–96. doi: 10.1016/j.carbpol.2016.09.013
87. Song R, Zhou Y, Li Y, Yang Z, Li F, Huang Q, et al. Preparation and characterization of mPEG-g-α-zein biohybrid micelles as a nano-carrier. J Appl Polym Sci. (2015) 132:42555. doi: 10.1002/app.42555
88. Schellekens H, Hennink WE, Brinks V. The immunogenicity of polyethylene glycol: Facts and fiction. Pharmacol Res. (2013) 30:1729–34. doi: 10.1007/s11095-013-1067-7
89. Sabra SA, Elzoghby AO, Sheweita SA, Haroun M, Helmy MW, Eldemellawy MA, et al. Self-assembled amphiphilic zein-lactoferrin micelles for tumor targeted co-delivery of rapamycin and wogonin to breast cancer. Eur J Pharm Biopharm. (2018) 128:156–69. doi: 10.1016/j.ejpb.2018.04.023
90. Abdelmoneem MA, Elnaggar MA, Hammady RS, Kamel SM, Helmy MW, Abdulkader MA, et al. Dual-targeted lactoferrin shell-oily core nanocapsules for synergistic targeted/herbal therapy of hepatocellular carcinoma. ACS Appl Mater Inter. (2019) 11:26731–44. doi: 10.1021/acsami.9b10164
91. Lin C, Lin I, Sung S, Su Y, Huang Y, Chiang CS, et al. Dual-targeted photopenetrative delivery of multiple micelles/hydrophobic drugs by a nanopea for enhanced tumor therapy. Adv Funct Mater. (2016) 26:4169–79. doi: 10.1002/adfm.201600498
92. Elhasany KA, Khattab SN, Bekhit AA, Ragab DM, Abdulkader MA, Zaky A, et al. Combination of magnetic targeting with synergistic inhibition of NF-κB and glutathione via micellar drug nanomedicine enhances its anti-tumor efficacy. Eur J Pharm Biopharm. (2020) 155:162–76. doi: 10.1016/j.ejpb.2020.08.004
93. Chen S, Li Q, Li H, Yang L, Yi J, Xie M, et al. Long-circulating zein-polysulfobetaine conjugate-based nanocarriers for enhancing the stability and pharmacokinetics of curcumin. Mater Sci Eng C. (2020) 109:110636. doi: 10.1016/j.msec.2020.110636
94. Ahmed S, Alhareth K, Mignet N. Advancement in nanogel formulations provides controlled drug release. Int J Pharm. (2020) 584:119435. doi: 10.1016/j.ijpharm.2020.119435
95. Cao Z, Zhou X, Wang G. Selective release of hydrophobic and hydrophilic cargos from multi-stimuli-responsive nanogels. ACS Appl Mater Inter. (2016) 8:28888–96. doi: 10.1021/acsami.6b10360
96. Seok HY, Rejinold NS, Lekshmi KM, Cherukula K, Park IK, Kim YC. CD44 targeting biocompatible and biodegradable hyaluronic acid cross-linked zein nanogels for curcumin delivery to cancer cells: In vitro and in vivo evaluation. J Control Release. (2018) 280:20–30. doi: 10.1016/j.jconrel.2018.04.050
97. Cui F, McClements DJ, Liu X, Liu F, Ngai T. Development of pH-responsive emulsions stabilized by whey protein fibrils. Food Hydrocoll. (2022) 122:107067. doi: 10.1016/j.foodhyd.2021.107067
98. Razavi R, Kenari RE, Farmani J, Jahanshahi M. Preparation of double-layer nanoemulsions with controlled release of glucose as prevention of hypoglycemia in diabetic patients. Biomed Pharmacother. (2021) 138:111464. doi: 10.1016/j.biopha.2021.111464
99. Pan Y, Tikekar RV, Wang MS, Avena-Bustillos RJ, Nitin N. Effect of barrier properties of zein colloidal particles and oil-in-water emulsions on oxidative stability of encapsulated bioactive compounds. Food Hydrocoll. (2015) 43:82–90. doi: 10.1016/j.foodhyd.2014.05.002
100. Zou L, Zheng B, Zhang R, Zhang Z, Liu W, Liu C, et al. Enhancing the bioaccessibility of hydrophobic bioactive agents using mixed colloidal dispersions: curcumin-loaded zein nanoparticles plus digestible lipid nanoparticles. Food Res Int. (2016) 81:74–82. doi: 10.1016/j.foodres.2015.12.035
101. Chuacharoen T, Sabliov CM. Comparative effects of curcumin when delivered in a nanoemulsion or nanoparticle form for food applications: study on stability and lipid oxidation inhibition. LWT-Food Sci Technol. (2019) 113:108319. doi: 10.1016/j.lwt.2019.108319
Keywords: zein, self-assembly, nanoparticle, delivery, curcumin
Citation: Yan X, Li M, Xu X, Liu X and Liu F (2022) Zein-based nano-delivery systems for encapsulation and protection of hydrophobic bioactives: A review. Front. Nutr. 9:999373. doi: 10.3389/fnut.2022.999373
Received: 21 July 2022; Accepted: 25 August 2022;
Published: 28 September 2022.
Edited by:
Xiao Feng, Nanjing University of Finance and Economics, ChinaReviewed by:
Liqiang Zou, Nanchang University, ChinaXiangwei Zhu, Hubei University of Technology, China
Copyright © 2022 Yan, Li, Xu, Liu and Liu. This is an open-access article distributed under the terms of the Creative Commons Attribution License (CC BY). The use, distribution or reproduction in other forums is permitted, provided the original author(s) and the copyright owner(s) are credited and that the original publication in this journal is cited, in accordance with accepted academic practice. No use, distribution or reproduction is permitted which does not comply with these terms.
*Correspondence: Fuguo Liu, ZnVndW8mI3gwMDA0MDtud2FmdS5lZHUuY24=