- 1Pediatric Oncology and Hematology “Lalla Seràgnoli,” IRCCS Azienda Ospedaliero-Universitaria di Bologna, Bologna, Italy
- 2Department of Experimental, Diagnostic and Specialty Medicine (DIMES), University of Bologna, Bologna, Italy
- 3Department of Medical and Surgical Sciences (DIMEC), University of Bologna, Bologna, Italy
Allogeneic hematopoietic stem cell transplantation (allo-HSCT) represents a potentially curative strategy for many oncological and non-oncological diseases, but it is associated with marked morbidity and mortality. The disruption of gut microbiota (GM) eubiosis has been linked to major allo-HSCT complications, including infections and acute graft vs. host disease (aGvHD), and correlates with mortality. This increasing knowledge on the role of the GM in the allo-HSCT procedure has led to fascinating ideas for modulating the intestinal ecosystem in order to improve clinical outcomes. Nutritional strategies, either by changing the route of nutritional supplementation or by administering specific molecules, are increasingly being considered as cost- and risk-effective methods of modulating the GM. Nutritional support has also emerged in the past several years as a key feature in supportive care for allo-HSCT recipients, and deterioration of nutritional status is associated with decreased overall survival and higher complication rates during treatment. Herein we provide a complete overview focused on nutritional modulation of the GM in allo-HSCT recipients. We address how pre transplant diet could affect GM composition and its ability to withstand the upsetting events occurring during transplantation. We also provide a complete overview on the influence of the route of nutritional administration on the intestinal ecosystem, with a particular focus on the comparison between enteral and parenteral nutrition (PN). Moreover, as mounting evidence are showing how specific components of post-transplant diet, such as lactose, could drastically shape the GM, we will also summarize the role of prebiotic supplementation in the modulation of the intestinal flora and in allo-HSCT outcomes.
Introduction
Allogeneic hematopoietic stem cell transplantation (allo-HSCT) represents a potentially curative strategy for many oncological, hematological, metabolic and immunological diseases (1–4). Complications, such as graft-vs.-host disease (GvHD) and infections, remain a major cause of morbidity and mortality, limiting the broader applicability of the procedure (5, 6). Patients undergoing allo-HSCT are subjected to high dose of chemotherapy, radiation, and antibiotics within a short time frame, that lead to mucosal barrier disruption and microbiota dysbiosis, characterized by reduced diversity, loss of commensals and expansions of potentially pathogenic bacteria (7–10). The degree of GM injury, and the establishment of specific GM signature are associated with major adverse outcomes. Reduced alpha diversity at engraftment is independently associated with increased mortality after transplantation (11). Overgrowth of Enterococcus is a risk factor for the development of aGvHD and for increased GvHD-related mortality and all-cause mortality (12), while Blautia is considered a protective factor from lethal GvHD (13). Intestinal domination, that occurs when a single bacterial taxon comprises 30% or more of the entire GM, is associated with the occurrence of blood stream infections (BSI) (14). Immune reconstitution, hepatic sinusoidal obstruction syndrome, febrile neutropenia, pulmonary complications and relapse of the primary disease have also been associated with intestinal microbiota composition after and/or before transplantation in single or multicenter studies (15–19). The biology underpinning the complex interplay between the GM, immune system and intestinal microenvironment has been partially addressed (15, 20–23) and needs to be fully elucidated (24). Gut microbiome-derived metabolites, among other factors, play a key role in mediating relevant biological processes during allo-HSCT. Such metabolites, particularly short chain fatty acids (SCFA), aryl hydrocarbon receptor ligands and bile acids, can protect intestinal epithelial cell from the damage occurring during transplantation and modulate the mucosal immunity reducing alloreactivity (23, 25). The increasing knowledge on the role of the GM during allo-HSCT has led to the fascinating idea of modulating the intestinal ecosystem toward a eubiotic configuration to improve clinical outcomes. Various interventions, both nutritional and non-nutritional, could be implemented with the aim of preserving GM homeostasis from the injury occurring during HSCT and/or restoring the GM diversity and composition after allo-HSCT (26). Among non-nutritional interventions, an antibiotic-sparing approach can help to preserve eubiosis (27, 28). “Direct” modulatory interventions using live microorganisms could also be applied, ranging from probiotic administration to fecal microbiota transplantation (FMT) (10). FMT directly modifies GM composition and has been used in HSCT setting, for the treatment of recurrent Clostridioides difficile infections and steroid-resistant gut GvHD or as preventive strategy in order to prevent dysbiosis (29).
Diet has also emerged in the past several years as a key candidate for the modulation of the GM, with the aim of affecting microbial composition, metabolic profile and ability to withstand the upsetting events occurring during transplantation. A profound link exists between nutrients and the GM. Diet is considered a major driver of the species and functions that reside within the GM (30). Even short-term changes in diet could rapidly affect the GM (31). For example, high-fiber and high-fermented-food consumption improves GM diversity, reduces inflammatory markers and has a distinct impact on immune response (32). Dietary interventions are now considered a key component in the management of several GM-related disease, with the main example being inflammatory bowel disease (33). Moreover, nutritional support is increasingly considered a key feature in supportive care for allo-HSCT recipients, as deterioration of nutritional status is associated with decreased overall survival and higher complication rates during treatment (34). In this review, we aim to summarize the present evidence regarding nutritional modulation of the GM in allo-HSCT recipients, ranging from changing the route of nutritional supplementation to administering specific molecules. We will also provide an in depth-focus on the potential mechanism involved in the complex relationship between diet, GM and clinical outcomes, underlining the most promising fields of research in the near future.
The relationship between nutritional status and gut microbiota
Nutritional status can influence allo-HSCT outcomes. Serum albumin deficiency prior to transplant is associated with increased non-relapse mortality (35), and malnutrition, accounting both low BMI and weight loss, is considered a risk factor for severe aGvHD (36). On the other hand, conflicting data exist regarding the relationship between obesity and clinical outcome, but the majority of studies points toward a net negative effect (37).
Nutritional status and GM have a bidirectional relationship. Disturbances in microbiome affect the risk for under nutrition and obesity through the alteration of bacterial metabolites production, and malnutrition alters GM function and composition (38–40). In particular, the reduction in microbial gene richness increases along with the severity of obesity and its metabolic complications, including type 2 diabetes mellitus (41–43). Specific taxonomic and functional markers are associated with increased BMI and glucose metabolism deterioration, such as enrichment in Bacteroides enterotype 2 and impairment of biotin metabolism (38, 44), but further studies are needed to better decipher the complex relationship between obesity and the GM.
In the HSCT setting, the impact of obesity on the GM and GvHD pathogenesis was assessed both in mouse models and humans. In the mouse model, mice with diet-induced obesity had increased incidence of severe, rapid onset gut aGvHD with high lethality. Gut aGvHD in obese mice was mediated by donor CD4 + T cells and occurred even with a minor MHC incompatibility. Obese mice presented also increased epithelial cell apoptosis, gut permeability, endotoxin translocation across the gut, and radiation- induced gastrointestinal damage after conditioning. Moreover, increased proinflammatory cytokine production and expression of the GvHD marker ST2 (IL-33R) and MHC class II molecules was observed. In the same study, the human obese cohort (BMI > 30) showed higher transplant-related mortality at 1-year, worse histological gut GvHD severity and higher serum ST2 concentrations compared with non-obese transplant recipients. GM analysis pre-transplant, both in human and mice, revealed reduced GM diversity and decreased Clostridiaceae abundance in obese patients, particularly with lower abundance of genus Clostridium. In the mouse model only, the relative abundance of Enterococcus and Akkermansia muciniphila significantly increased in obese after transplantation. Therefore, obesity-associated GM alterations may render the patient more prone to gut epithelial damage, inflammation and gut aGvHD. Interestingly, prophylactic antibiotic treatment in obese mice improved gut GvHD histological severity and mortality, as well as reduced endotoxin translocation across the intestinal epithelium and inflammatory cytokine production, but did not protect against the development of cGvHD of the skin, highlighting the possibility of modulating obesity-associated dysbiosis (37).
Indeed, body mass composition itself could impact HSCT outcome, and GM composition could also influence the complex metabolic pathways regulating body composition. Muscle mass is an independent predictor of survival after HSCT, with sarcopenia associated with worse disease-free and overall survival (45, 46). The GM, and particularly its metabolites, play an important role in muscle metabolism, affecting skeletal muscle mass and function (47). Administration of soy-whey blended protein for 2 months in HSCT recipients who failed to improve muscle function within half a year resulted in significantly improved muscle area and muscle strength. However, in a small number of patients, muscle status did not improve. GM Alpha diversity significantly increased in responder to treatment, whereas it did not in non-responders. Moreover, abundance of most of the butyrate- producing taxa decreased significantly in non-responders. This important SCFA is known to regulate skeletal muscle energy expenditure and metabolism (48). Ruminococcus and Veillonella abundance correlated positively with muscle status, whereas Streptococcus correlated negatively (49). Ruminococcus species can metabolize monosaccharides and degrade mucin, producing acetic acid and formic acid (50). Veillonella enhance muscle function by converting lactic acid produced by muscles to propionic acid (51). Amino acid biosynthesis and pentose phosphate pathways were also higher in the GM of responders. Bacteria can enhance protein anabolism in the muscle by increasing amino acid bioavailability (52). Moreover, the pentose phosphate pathway is important for anabolic processes in the initial phases of skeletal muscle regeneration (53). These finding underline the important role of the GM on muscle metabolism after HSCT.
The impact of nutritional route of administration on gut microbiota
Oral intake in the early post-transplantation period is severely impaired due to the side effects of the conditioning regimen, mainly vomiting, and mucositis. Parenteral nutrition (PN) has been historically used as supportive measure in order to avoid the deterioration of nutritional status in HSCT recipients (54–56). However, PN is associated with several metabolic, hepatic and intestinal complications. In recent years, enteral nutrition (EN) has been increasingly used in the HSCT setting considering the feasibility and clinical benefits of this approach (57, 58). EN is currently recommended by international guidelines as first-line nutritional support in transplant recipients if oral intake is insufficient (59). Nevertheless, PN is still largely adopted in the majority of transplantation centers, mainly due to patients and staff preference for PN and concerns regarding EN tolerance (60, 61).
The clinical positive effects of EN for nutritional support during the neutropenic phase after HSCT have been showed in several studies. In our recent meta-analysis the use of EN was associated with lower rates of aGVHD, aGVHD grade III-IV and gastrointestinal aGVHD compared to PN (62). Some studies also observed reduced infective complications, such as BSI (63). Interestingly, a survey in the Australian HSCT centers identified as the major barrier to the use of EN the perception of invasiveness and the perception of poor tolerance (58). These limitations should be directly addressed in future studies to clearly define the clinical benefit of EN.
One possible explanation for these findings is the different effects of the two nutritional strategies on GM composition, as already demonstrated in different clinical and preclinical settings. On one side, PN reduced intestinal transit with subsequent GM dysbiosis and mucosal atrophy. It also determines a proinflammatory state with resulting loss of epithelial barrier function that leads to microbial translocation and bacteremia. On the other side, EN allows the maintenance of gut transit and seems to improve mucosa integrity. EN exerts a trophic effect on enterocytes, either directly providing nutrients in the gut lumen, or indirectly via the production of SCFA from the GM (64, 65). Moreover, EN seems to be essential to maintain an healthy gut mucosal and high GM diversity, as showed in preclinical and clinical models (64–68) (Figure 1). In murine models, PN results in reduced bacterial diversity (68) and a shift of microbiome to Bacterioidetes after several days of exclusive PN administration was noted (69, 70). Furthermore, a loss of bacterial diversity was observed in a neonatal pig model feed with PN (71).
Data on humans are limited, but seems to demonstrate that PN reduces GM diversity and is associated with an increased relative abundance of Proteobacteria (particularly Enterococcaceae), that proliferate in a nutrient-deprived environment, whereas a reduction in Firmicutes is observed, that seem to dominate when a valid nutrient supply is guaranteed (68, 72). The effect of PN on the GM of HSCT recipients was investigated in two large observational studies. The first one, on 80 HSCT recipients, groups with different GM diversity showed no differences in term of PN administration, with similar percentages of patients receiving PN during the pre-engraftment period in the two groups (73). Differently, in a second study investigating the relationship between GM and GvHD on 115 patients, patients receiving PN for a shorter period (<10 days) showed a higher abundance of genus Blautia compared to those receiving PN for a longer duration. Notably the inverse association of PN and Blautia was maintained even for patients who did not receive anaerobe-active antibiotics (13) Blautia is an anaerobic commensal producer of SCFA that has been associated with reduced GvHD related mortality (13).
To date, two studies specifically investigated the impact of EN on GM composition compared to PN in HSCT setting. In a cohort of 23 adult HSCT recipients randomly allocated to receive EN or PN, a shotgun metagenomic sequencing analysis of stool samples 30 days post-transplant, revealed no difference in term of microbial diversity in the two groups. However, significant differences in GM composition were reported in patients receiving predominantly EN compared to patients receiving predominantly PN. Particularly, the former presented a higher abundance of taxa associated with increased SCFA production, including R. bromii, R. inulinivorans, A. hadrus, and several F. praunitzii species. In the PN group, a greater abundance of Enterococcus and Proteobacteria, such as Klebsiella, was noted. Furthermore, patients who maintained higher levels of oral intake during the phase of nutritional support, whether parenteral, enteral or a combination of both, present significantly different GM composition with higher microbial diversity and relative abundance of SCFA-producing taxa, including F. prausnitzii_C, R. inulinivorans, and Blautia. A greater abundance of potential pathogens, such as Klebsiella_A, Staphylococcus, and Enterococcus was observed instead in patients who maintained a longer duration of minimal oral intake. Unfortunately, the small sample size and the absence of pre-transplant sampling limit the reliability of these findings (74). We reported a positive effect of EN on GM composition after HSCT in pediatric HSCT recipients. We assessed GM composition at the baseline, close after transplantation and during the immunological recovery following the HSCT. Patients receiving EN showed lower GM injury and an almost complete recovery of the diversity after HSCT. On the other side, patients receiving PN presented a microbial shift toward a dysbiotic profile and never achieved a return to pre-transplant microbial status. Some genera, including Faecalibacterum, Dorea, Blautia, Bacteroides, Parabacteroides, and Oscillospira, were relatively more abundant in EN patients after HSCT, confirming the higher presence of SCFA-producing bacteria in EN-treated patients. As expected, the fecal levels of SCFAs were restored to baseline values only in the EN group (75). In a recent study in adult HSCT recipients receiving EN, an analysis of intestinal microbiome was performed in a small subgroup of patients who received EN before conditioning. Interestingly, in two patients who discontinued EN and oral ingestion and shifted to PN, a drastic increase of Enterococcaceae was observed (76).
The impact of specific dietary elements on gut microbiota
As mentioned, diet represents one of the most important ways to modulate the GM and has been increasingly studied (41, 77). On the other side, less is known on the effect of single dietary elements on GM. Some recent reports evaluated the impact of several dietary elements and nutritional strategies on allo-HSCT outcomes. These includes the role of nutrients, prebiotics, as well as the oral administration of probiotics, synbiotics, postbiotics, and lactoferrin (Table 1 and Figure 2).
Nutrients and prebiotics
Stein-Thoeringer et al. evaluated the role of lactose in the setting of allo-HSCT (12). 16S ribosomal RNA gene sequencing was used to study the fecal microbiota of 1,325 adult allo-HSCT recipients. Fecal Enterococcus spp. domination was found in up to 65% of patients after allo-HSCT and was associated with a significant reduction of overall survival and an increased risk of moderate-to-severe acute GVHD. Given that the growth of enterococci depends on lactose availability in vitro, the authors found that luminal lactose modulates the post-transplant Enterococcus overgrowth and GM recovery in both mouse models and in vivo (12). In particular, dietary lactose depletion in mice mitigated enterococcal expansion and reduced the severity of GvHD, with consequent improved survival. In humans, administration of broad-spectrum antibiotics generated Enterococcus overgrowth both in lactose absorbers and in patients carrying lactose-non-absorber genotypes, but Enterococcus domination was significantly prolonged in malabsorbers after antibiotics exposure (12). It is possible to speculate that enterocytes damage due to chemo-radiotherapy toxicity and alloreactivity induces loss of lactase and consequent increased luminal concentrations of lactose, which in turn is critical as a growth substrate for Enterococcus expansion. These findings might suggest that non-antibiotic-based interventions, such as lactose-free diet, could be a useful strategy to mitigate fecal pathobiont domination, possibly improving clinical outcome by reducing aGvHD and BSI in allo-HSCT recipients (Figure 3).
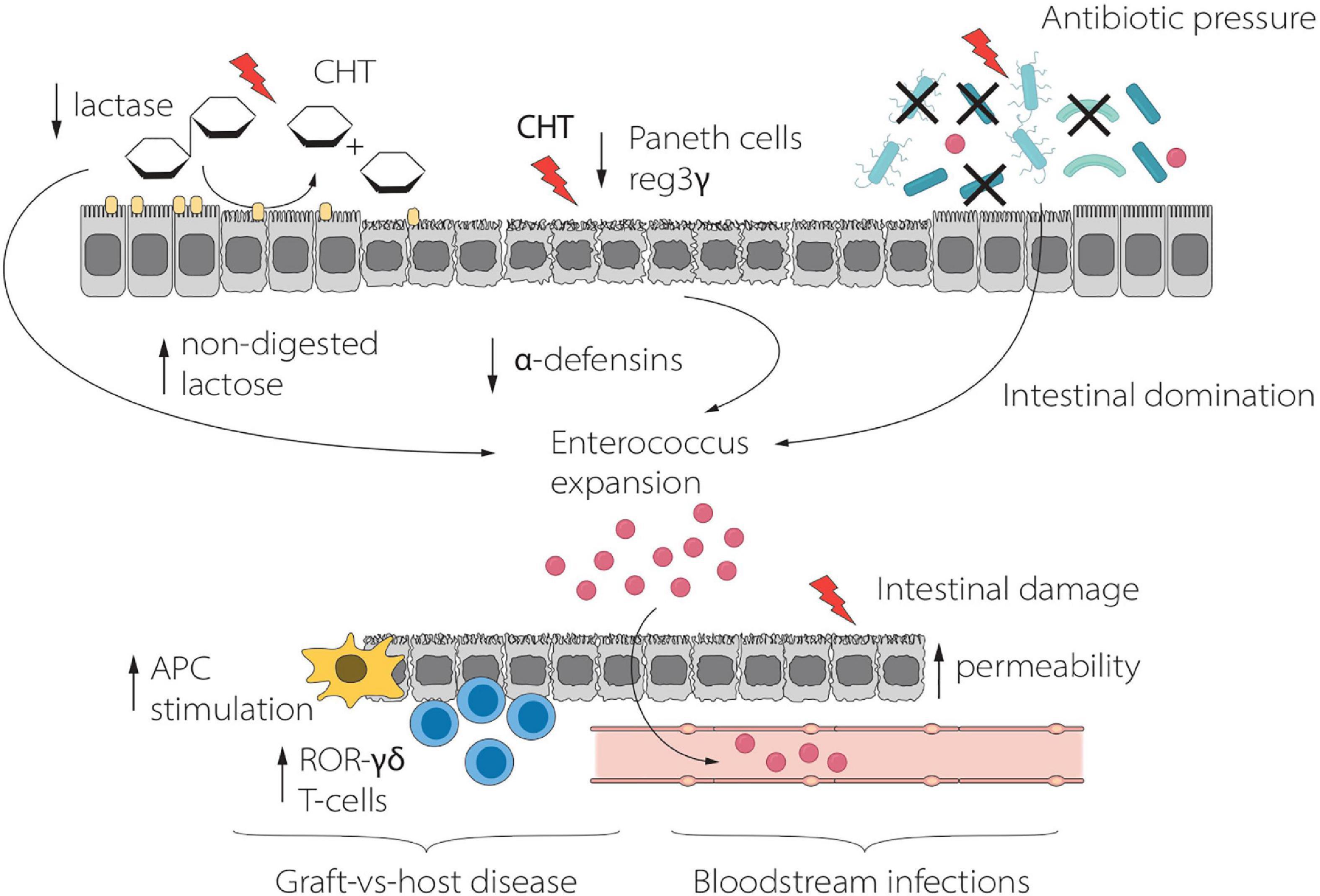
Figure 3. Schematic representation of the relationship between Lactose, Enterococcus and clinical outcome during HSCT. Enterococcus expansion, driven by lactose, promotes inflammation and GvHD, whereas translocation through the damaged intestinal mucosa results in BSI. APC, Antigen Presenting Cell; CHT, Chemotherapy.
Prebiotics are defined as “a substrate which is selectively utilized by host microorganism conferring a health benefit” (78). They are selectively fermentable, non-digestible carbohydrates such as resistant starch, and in particular potato starch, fructo-oligosaccharides, galacto-oligosaccharides, and trans-galacto-oligosaccharides. The fermentation of prebiotics by the GM results mainly in the production of SCFAs, which directly modulate acidity in the colon (79), intestinal epithelium development (80) and immune system regulation (81). Iyama et al. reported that including a combination of glutamine, fiber and oligosaccharides in the diet during allo-HSCT decreased the severity of the intestinal mucositis and was associated with higher overall survival at day + 100 compared to the control. Furthermore, this diet was associated with a significant reduction in translocation of Enterococcus species from the GM to the bloodstream (82). Yoshifuji et al. administered resistant starch and a prebiotics mixture containing glutamine, polydextrose, and lactosucrose from prior to conditioning to day 28 after allo-HSCT. They observed shortened duration of oral mucositis and diarrhea and reduced cumulative incidence of aGvHD grades 2–4, with a particular decrease of skin aGvHD in the prebiotic group compared to an historical cohort. GM diversity, relative abundance of butyrate-producing taxa and fecal butyrate concentration were preserved by prebiotics intake (83).
Another interesting dietary compound that could be administered is Lactoferrin. Lactoferrin is an iron-binding protein of bovine and human milk involved in iron homeostasis, with pleiotropic functions such as antimicrobial and immunoregulatory activity. Preclinical models showed that Lactoferrin seems to reduce bacterial translocation, improving GM eubiosis (84). In preterm neonates, bovine Lactoferrin administration reduced the incidence of late-onset sepsis and necrotizing enterocolitis improving eubiosis, strengthening the intestinal epithelial barrier and promoting antagonism toward enteropathogens (85–87). However, clinical evidence in other settings remain scarce. Rota et al. reported that symptoms of gut GvHD disappeared soon after lactoferrin therapy was started in an HSCT patient (88) highlighting the potential role of lactoferrin administration in transplant recipients. Interestingly, in a randomized controlled trial of pediatric patients receiving induction chemotherapy for hematologic malignancies, oral lactoferrin supplementation promoted the maintenance of diversity during chemotherapy and was associated with reduced outgrowth of pathobionts, like Enterococcus (89).
Probiotics
Probiotics are defined as “live microorganisms which, when administered in adequate amounts, confer a health benefit on the host” according to the Food and Agriculture Organization of the United Nations and World Health Organization (90), and could be also found in traditional and commonly eaten foods. Probiotic use among cancer patients undergoing treatment is increasing (91), with Lactobacillus spp. and Bifidobacterium spp. being the most utilized (92). The administration of living organisms in patients with hematologic malignancies and altered gut permeability is still controversial due to safety concerns. Various authors reported cases of BSI or sepsis caused by probiotic bacterial strains (93–95). However, Ladas et al. reported that administration of Lactobacillus plantarum in a cohort of 30 pediatric patients is safe and feasible, with no bacteremia or adverse events reported (96). A Phase I clinical trial to evaluate the safety and tolerability of Clostridium butyricum CBM 588 Probiotic Strain administration during HSCT (from day + 1 to + 28) is ongoing (NCT03922035). Moreover, the use of fungal probiotics such as Saccharomyces boulardii CNCM I-745 has been explored, but its use remains controversial, since several cases of fungemia were reported (97, 98).
A diet richer in fiber and probiotics prior to transplant was associated with earlier neutrophil engraftment and a shorter duration of febrile neutropenia in a small observational study (99). In a randomized, non-placebo-controlled trial 20 HSCT recipients were supplemented with Lactobacillus rhamnosus GG. No appreciable probiotic-related change in the GM or difference in the incidence of GvHD were observed (100). To date, given the uncertain safety profile and the lack of clinical evidence, the benefit of probiotic supplementation during allo-HSCT still needs to be determined. Moreover, in patient receiving immune checkpoint inhibitors for melanoma, a diet richer in fiber without probiotic administration was associated with improved outcome compared to probiotic use (101), suggesting that also in the HSCT setting other strategies rather than single probiotics could be prioritized for nutritional modulation of the GM.
Synbiotics and post-biotics
Synbiotic are defined as a combination of probiotic bacteria and growth-promoting prebiotic that achieve “synergism” (102). The clinical application of synbiotic in the onco-hematological settings is limited. In an animal model of leukemic mice with cachexia, Bindels et al. reported that a synbiotic containing inulin-type fructan and L. reuteri 100–23 promoted the restoration of Lactobacillus species and the reduction of Enterobacteriaceae, with a positive effect on intestinal homeostasis and a prolonged survival (103).
Postbiotics are a heterogeneous group of functional bioactive compounds, defined as a preparation of inanimate microorganisms and/or their components that confers a health benefit on the host. They therefore contain inactivated microbial cells or cell components, with or without metabolites (104). Since they do not contain any living organism, they could be an attractive option for immunocompromised patients, where safety of live-bacterial products is still matter of concerns. In mouse models, the administration of exopolysaccharide derived from Bacillus subtilis ameliorated aGvHD improving GvHD related mortality. This effect was mediated by the induction of inhibitory dentritic cells in a TLR4-dependent manner (105).
SCFAs are the most investigated, and they represent a effector route in common with prebiotic compounds. Given the strong evidence associating increased GM-production of SCFA with protection from chronic GvHD (106), and acute GvHD (107), their administration could be explored in the near future, even if the challenge of delivering sufficient amounts of SCFA to the human colon is still present (108).
Conclusion
We summarized the present evidence regarding the role of nutrition in the modulation of the GM in allo-HSCT recipients. Detailed investigations on the biological mechanism of nutritional intervention have been provided for the role of lactose, but still lacks for other dietary compound and for the use of EN vs. PN.
We believe that the following three areas of research should receive the most attention in the near future: the exact impact of which enteral formula is used for providing EN still needs to be evaluated, and lessons should be learned from dietary modulation applied in the management of IBD (109).
Several other prebiotics and postbiotics are currently under investigation in preclinical or clinical models, such as Lactoferrin, Inulin and Exopolysaccharide, and could be supplemented together with oral or enteral feeding. To date, several clinical trials evaluating the safety and efficacy of specific dietary elements for GM modulation in allo-HSCT recipients are ongoing, as summarized in Table 2. Other compounds that should receive attention are bile acids. Prophylactic administration of ursodeoxycholic acid is associated with reduced incidence of hepatic complications and severe aGvHD, and lower non-relapse mortality (110), and this clinical benefit could be partially explained by its effect on the GM (21, 25, 111). Therefore, studies analyzing the impact of bile acids administration on the metabolomic and metagenomic configuration of the GM are awaited.
Moreover, food consumption after the neutropenic phase should be strictly monitored, in order to unravel the potential relationship between consumed foods and GM modifications. Therefore, since evidences of the influence of single nutrients on HSCT outcomes are gathering, it would be interesting to analyze the impact of specific foods as well. All these interventions could provide effective, safe and cost effective ways to modulate the GM in order to improve clinical outcomes. A change in nutritional support during HSCT is awaited in the next years considering the growing evidence regarding its positive microbiological and clinical impact.
Author contributions
EM designed the work. DL, FB, and FV wrote the manuscript. AP and RM critically revised the manuscript. All authors contributed to the article and approved the submitted version.
Conflict of interest
The authors declare that the research was conducted in the absence of any commercial or financial relationships that could be construed as a potential conflict of interest.
Publisher’s note
All claims expressed in this article are solely those of the authors and do not necessarily represent those of their affiliated organizations, or those of the publisher, the editors and the reviewers. Any product that may be evaluated in this article, or claim that may be made by its manufacturer, is not guaranteed or endorsed by the publisher.
References
1. Carreras E, Dufour C, Mohty M, Kröger N. The EBMT Handbook?: Hematopoietic Stem Cell Transplantation and Cellular Therapies. Cham: Springer International Publishing (2019).
2. Merli P, Algeri M, Del Bufalo F, Locatelli F. Hematopoietic stem cell transplantation in pediatric acute lymphoblastic leukemia. Curr Hematol Malig Rep. (2019) 14:94–105. doi: 10.1007/s11899-019-00502-2
3. Masetti R, Vendemini F, Zama D, Biagi C, Pession A, Locatelli F. Acute myeloid leukemia in infants: biology and treatment. Front Pediatr. (2015) 3:37. doi: 10.3389/fped.2015.00037
4. Dalle JH, Peffault de Latour R. Allogeneic hematopoietic stem cell transplantation for inherited bone marrow failure syndromes. Int J Hematol. (2016) 103:373–9. doi: 10.1007/s12185-016-1951-0
5. Jenq RR, van den Brink MRM. Allogeneic haematopoietic stem cell transplantation: individualized stem cell and immune therapy of cancer. Nat Rev Cancer. (2010) 10:213–21. doi: 10.1038/nrc2804
6. Copelan EA. Hematopoietic stem-cell transplantation. N Engl J Med. (2006) 354:1813–26. doi: 10.1056/NEJMra052638
7. Masetti R, Zama D, Leardini D, Muratore E, Turroni S, Prete A, et al. The gut microbiome in pediatric patients undergoing allogeneic hematopoietic stem cell transplantation. Pediatr Blood Cancer. (2020) 67:e28711. doi: 10.1002/pbc.28711
8. Cullin N, Azevedo Antunes C, Straussman R, Stein-Thoeringer CK, Elinav E. Microbiome and cancer. Cancer Cell. (2021) 39:1317–41.
9. Masetti R, Muratore E, Leardini D, Zama D, Turroni S, Brigidi P, et al. Gut microbiome in pediatric acute leukemia: from predisposition to cure. Blood Adv. (2021) 5:4619–29. doi: 10.1182/bloodadvances.2021005129
10. Zama D, Bossù G, Leardini D, Muratore E, Biagi E, Prete A, et al. Insights into the role of intestinal microbiota in hematopoietic stem-cell transplantation. Ther Adv Hematol. (2020) 11:204062071989696. doi: 10.1177/2040620719896961
11. Peled JU, Gomes ALC, Devlin SM, Littmann ER, Taur Y, Sung AD, et al. Microbiota as predictor of mortality in allogeneic hematopoietic-cell transplantation. N Engl J Med. (2020) 382:822–34. doi: 10.1056/NEJMoa1900623
12. Stein-Thoeringer CK, Nichols KB, Lazrak A, Docampo MD, Slingerland AE, Slingerland JB, et al. Lactose drives Enterococcus expansion to promote graft-versus-host disease. Science. (2019) 366:1143–9. doi: 10.1126/science.aax3760
13. Jenq RR, Taur Y, Devlin SM, Ponce DM, Goldberg JD, Ahr KF, et al. Intestinal blautia is associated with reduced death from graft-versus-host disease. Biol Blood Marrow Transplant. (2015) 21:1373–83. doi: 10.1016/j.bbmt.2015.04.016
14. Taur Y, Xavier JB, Lipuma L, Ubeda C, Goldberg J, Gobourne A, et al. Intestinal domination and the risk of bacteremia in patients undergoing allogeneic hematopoietic stem cell transplantation. Clin Infect Dis. (2012) 55:905–14. doi: 10.1093/cid/cis580
15. Schluter J, Peled JU, Taylor BP, Markey KA, Smith M, Taur Y, et al. The gut microbiota is associated with immune cell dynamics in humans. Nature. (2020) 588:303–7. doi: 10.1038/s41586-020-2971-8
16. Masetti R, Biagi E, Zama D, Muratore E, Amico FD, Leardini D, et al. Early modifications of the gut microbiome in children with hepatic sinusoidal obstruction syndrome after hematopoietic stem cell transplantation. Sci Rep. (2021) 11:14307. doi: 10.1038/s41598-021-93571-4
17. Masetti R, D’Amico F, Zama D, Leardini D, Muratore E, Ussowicz M, et al. Febrile neutropenia duration is associated with the severity of gut microbiota dysbiosis in pediatric allogeneic hematopoietic stem cell transplantation recipients. Cancers. (2022) 14:1932. doi: 10.3390/cancers14081932
18. Harris B, Morjaria SM, Littmann ER, Geyer AI, Stover DE, Barker JN, et al. Gut microbiota predict pulmonary infiltrates after allogeneic hematopoietic cell transplantation. Am J Respir Crit Care Med. (2016) 194:450–63. doi: 10.1164/rccm.201507-1491OC
19. Peled JU, Devlin SM, Staffas A, Lumish M, Khanin R, Littmann ER, et al. Intestinal microbiota and Relapse after hematopoietic-Cell transplantation. J Clin Oncol. (2017) 35:1650–9. doi: 10.1200/JCO.2016.70.3348
20. Ingham AC, Kielsen K, Cilieborg MS, Lund O, Holmes S, Aarestrup FM, et al. Specific gut microbiome members are associated with distinct immune markers in pediatric allogeneic hematopoietic stem cell transplantation. Microbiome. (2019) 7:131. doi: 10.1186/s40168-019-0745-z
21. Haring E, Uhl FM, Andrieux G, Proietti M, Bulashevska A, Sauer B, et al. Bile acids regulate intestinal antigen presentation and reduce graft-versus-host disease without impairing the graft-versus-leukemia effect. Haematologica. (2021) 106:2131–46. doi: 10.3324/haematol.2019.242990
22. Koyama M, Mukhopadhyay P, Schuster IS, Henden AS, Hülsdünker J, Varelias A, et al. MHC Class II antigen presentation by the intestinal epithelium initiates graft-versus-host disease and is influenced by the microbiota. Immunity. (2019) 51:885–98.e7. doi: 10.1016/j.immuni.2019.08.011
23. Mathewson ND, Jenq R, Mathew AV, Koenigsknecht M, Hanash A, Toubai T, et al. Gut microbiome–derived metabolites modulate intestinal epithelial cell damage and mitigate graft-versus-host disease. Nat Immunol. (2016) 17:505–13. doi: 10.1038/ni.3400
24. Masetti R, Zama D, Leardini D, Muratore E, Turroni S, Brigidi P, et al. Microbiome-derived metabolites in allogeneic hematopoietic stem cell transplantation. Int J Mol Sci. (2021) 22:1197. doi: 10.3390/ijms22031197
25. Michonneau D, Latis E, Curis E, Dubouchet L, Ramamoorthy S, Ingram B, et al. Metabolomics analysis of human acute graft-versus-host disease reveals changes in host and microbiota-derived metabolites. Nat Commun. (2019) 10:5695. doi: 10.1038/s41467-019-13498-3
26. Henig I, Yehudai-Ofir D, Zuckerman T. The clinical role of the gut microbiome and fecal microbiota transplantation in allogeneic stem cell transplantation. Haematologica. (2021) 106:933–46. doi: 10.3324/haematol.2020.247395
27. Weber D, Hiergeist A, Weber M, Dettmer K, Wolff D, Hahn J, et al. Detrimental effect of broad-spectrum antibiotics on intestinal microbiome diversity in patients after allogeneic stem cell transplantation: lack of commensal sparing antibiotics. Clin Infect Dis. (2019) 68:1303–10. doi: 10.1093/cid/ciy711
28. Muratore E, Baccelli F, Leardini D, Campoli C, Belotti T, Viale P, et al. Antimicrobial stewardship interventions in pediatric oncology: a systematic review. J Clin Med. (2022) 11:4545. doi: 10.3390/jcm11154545
29. Pession A, Zama D, Muratore E, Leardini D, Gori D, Guaraldi F, et al. Fecal microbiota transplantation in allogeneic hematopoietic stem cell transplantation recipients: a systematic review. J Pers Med. (2021) 11:100. doi: 10.3390/jpm11020100
30. Flint HJ, Duncan SH, Louis P. The impact of nutrition on intestinal bacterial communities. Curr Opin Microbiol. (2017) 38:59–65. doi: 10.1016/j.mib.2017.04.005
31. David LA, Maurice CF, Carmody RN, Gootenberg DB, Button JE, Wolfe BE, et al. Diet rapidly and reproducibly alters the human gut microbiome. Nature. (2014) 505:559–63. doi: 10.1038/nature12820
32. Wastyk HC, Fragiadakis GK, Perelman D, Dahan D, Merrill BD, Yu FB, et al. Gut-microbiota-targeted diets modulate human immune status. Cell. (2021) 184:4137–53.e14. doi: 10.1016/j.cell.2021.06.019
33. Fitzpatrick JA, Melton SL, Yao CK, Gibson PR, Halmos EP. Dietary management of adults with IBD - the emerging role of dietary therapy. Nat Rev Gastroenterol Hepatol. (2022) 19:652–69. doi: 10.1038/s41575-022-00619-5
34. Fabozzi F, Trovato CM, Diamanti A, Mastronuzzi A, Zecca M, Tripodi SI, et al. Management of nutritional needs in pediatric oncology?: a consensus statement. Cancers. (2022) 14:3378.
35. Schaffrath J, Diederichs T, Unverzagt S, Wass M, Gläser U, Weber T, et al. Correlation of nutrition-associated parameters with non-relapse mortality in allogeneic hematopoietic stem cell transplantation. Ann Hematol. (2022) 101:681–91. doi: 10.1007/s00277-021-04736-0
36. Kerby EH, Li Y, Getz KD, Smith EC, Smith LT, Bunin NJ, et al. Nutritional risk factors predict severe acute graft-versus-host disease and early mortality in pediatric allogeneic hematopoietic stem cell transplantation. Pediatr Blood Cancer. (2018) 65:e26853. doi: 10.1002/pbc.26853
37. Khuat LT, Le CT, Pai C-CS, Shields-Cutler RR, Holtan SG, Rashidi A, et al. Obesity induces gut microbiota alterations and augments acute graft-versus-host disease after allogeneic stem cell transplantation. Sci Transl Med. (2020) 12:eaay7713. doi: 10.1126/scitranslmed.aay7713
38. Belda E, Voland L, Tremaroli V, Falony G, Adriouch S, Assmann KE, et al. Impairment of gut microbial biotin metabolism and host biotin status in severe obesity: effect of biotin and prebiotic supplementation on improved metabolism. Gut. (2022) doi: 10.1136/gutjnl-2021-325753 [Epub ahead of print].
39. Smith MI, Yatsunenko T, Manary MJ, Trehan I, Mkakosya R, Cheng J, et al. Gut microbiomes of malawian twin pairs discordant for kwashiorkor. Science. (2013) 339:548–54. doi: 10.1126/science.1229000
40. Subramanian S, Huq S, Yatsunenko T, Haque R, Mahfuz M, Alam MA, et al. Persistent gut microbiota immaturity in malnourished Bangladeshi children. Nature. (2014) 510:417–21. doi: 10.1038/nature13421
41. Cotillard A, Kennedy SP, Kong LC, Prifti E, Pons N, Le Chatelier E, et al. Dietary intervention impact on gut microbial gene richness. Nature. (2013) 500:585–8. doi: 10.1038/nature12480
42. Aron-Wisnewsky J, Prifti E, Belda E, Ichou F, Kayser BD, Dao MC, et al. Major microbiota dysbiosis in severe obesity: fate after bariatric surgery. Gut. (2019) 68:70–82. doi: 10.1136/gutjnl-2018-316103
43. Kawano Y, Edwards M, Huang Y, Bilate AM, Araujo LP, Tanoue T, et al. Microbiota imbalance induced by dietary sugar disrupts immune-mediated protection from metabolic syndrome. Cell. (2022) 185:3501–19.e20. doi: 10.1016/j.cell.2022.08.005
44. Vieira-Silva S, Falony G, Belda E, Nielsen T, Aron-Wisnewsky J, Chakaroun R, et al. Statin therapy is associated with lower prevalence of gut microbiota dysbiosis. Nature. (2020) 581:310–5. doi: 10.1038/s41586-020-2269-x
45. Armenian SH, Xiao M, Berano Teh J, Lee B, Chang HA, Mascarenhas K, et al. Impact of sarcopenia on adverse outcomes after allogeneic hematopoietic cell transplantation. J Natl Cancer Inst. (2019) 111:837–44. doi: 10.1093/jnci/djy231
46. Nagayama T, Fujiwara S, Kikuchi T, Onda K, Murahashi R, Nakashima H, et al. Impact of muscle mass loss assessed by computed tomography on the outcome of allogeneic stem cell transplantation. Leuk Lymphoma. (2022) 63:1694–700. doi: 10.1080/10428194.2022.2034159
47. Lahiri S, Kim H, Garcia-Perez I, Reza MM, Martin KA, Kundu P, et al. The gut microbiota influences skeletal muscle mass and function in mice. Sci Transl Med. (2019) 11:eaan5662. doi: 10.1126/scitranslmed.aan5662
48. Henagan TM, Stefanska B, Fang Z, Navard AM, Ye J, Lenard NR, et al. Sodium butyrate epigenetically modulates high-fat diet-induced skeletal muscle mitochondrial adaptation, obesity and insulin resistance through nucleosome positioning. Br J Pharmacol. (2015) 172:2782–98. doi: 10.1111/bph.13058
49. Ren G, Zhang J, Li M, Tang Z, Yang Z, Cheng G, et al. Gut microbiota composition influences outcomes of skeletal muscle nutritional intervention via blended protein supplementation in posttransplant patients with hematological malignancies. Clin Nutr. (2020) 40:94–102. doi: 10.1016/j.clnu.2020.04.030
50. Arumugam M, Raes J, Pelletier E, Le Paslier D, Yamada T, Mende DR, et al. Enterotypes of the human gut microbiome. Nature. (2011) 473:174–80. doi: 10.1038/nature09944
51. Scheiman J, Luber JM, Chavkin TA, MacDonald T, Tung A, Pham L-D, et al. Meta-omics analysis of elite athletes identifies a performance-enhancing microbe that functions via lactate metabolism. Nat Med. (2019) 25:1104–9. doi: 10.1038/s41591-019-0485-4
52. Ticinesi A, Nouvenne A, Cerundolo N, Catania P, Prati B, Tana C, et al. Gut Microbiota, muscle mass and function in aging: a focus on physical frailty and sarcopenia. Nutrients. (2019) 11:1633. doi: 10.3390/nu11071633
53. Wagner KR, Kauffman FC, Max SR. The pentose phosphate pathway in regenerating skeletal muscle. Biochem J. (1978) 170:17–22. doi: 10.1042/bj1700017
54. Yokoyama S, Fujimoto T, Mitomi T, Yabe M, Yabe H, Kato S. Use of total parenteral nutrition in pediatric bone marrow transplantation. Nutrition. (1989) 5:27–30.
55. Beckerson J, Szydlo RM, Hickson M, Mactier CE, Innes AJ, Gabriel IH, et al. Impact of route and adequacy of nutritional intake on outcomes of allogeneic haematopoietic cell transplantation for haematologic malignancies. Clin Nutr. (2019) 38:738–44. doi: 10.1016/j.clnu.2018.03.008
56. Walrath M, Bacon C, Foley S, Fung HC. Gastrointestinal side effects and adequacy of enteral intake in hematopoietic stem cell transplant patients. Nutr Clin Pract. (2015) 30:305–10. doi: 10.1177/0884533614547084
57. Baumgartner A, Bargetzi A, Zueger N, Bargetzi M, Medinger M, Bounoure L, et al. Revisiting nutritional support for allogeneic hematologic stem cell transplantation – a systematic review. Bone Marrow Transplant. (2017) 52:506–13. doi: 10.1038/bmt.2016.310
58. Andersen S, Weber N, Kennedy G, Brown T, Banks M, Bauer J. Tolerability of proactive enteral nutrition post allogeneic haematopoietic progenitor cell transplant: a randomised comparison to standard care. Clin Nutr. (2020) 39:1364–70. doi: 10.1016/j.clnu.2019.06.012
59. Arends J, Bachmann P, Baracos V, Barthelemy N, Bertz H, Bozzetti F, et al. ESPEN guidelines on nutrition in cancer patients. Clin Nutr. (2017) 36:11–48. doi: 10.1016/j.clnu.2016.07.015
60. Peric Z, Botti S, Stringer J, Krawczyk J, van der Werf S, van Biezen A, et al. Variability of nutritional practices in peritransplant period after allogeneic hematopoietic stem cell transplantation: a survey by the complications and quality of life working party of the EBMT. Bone Marrow Transplant. (2018) 53:1030–7. doi: 10.1038/s41409-018-0137-1
61. Andersen S, Banks M, Brown T, Weber N, Kennedy G, Bauer J. Nutrition support during allogeneic stem cell transplantation: evidence versus practice. Support Care Cancer. (2020) 11:5441–7. doi: 10.1007/s00520-020-05397-x
62. Zama D, Gori D, Muratore E, Leardini D, Rallo F, Turroni S, et al. Enteral versus parenteral nutrition as nutritional support after allogeneic hematopoietic stem cell transplantation: a systematic review and meta-analysis. Transplant Cell Ther. (2021) 27:180.e1–e8. doi: 10.1016/j.jtct.2020.11.006
63. Zama D, Muratore E, Biagi E, Forchielli ML, Rondelli R, Candela M, et al. Enteral nutrition protects children undergoing allogeneic hematopoietic stem cell transplantation from blood stream infections. Nutr J. (2020) 19:29. doi: 10.1186/s12937-020-00537-9
64. Shen T-Y, Qin H-L, Gao Z-G, Fan X-B, Hang X-M, Jiang Y-Q. Influences of enteral nutrition combined with probiotics on gut microflora and barrier function of rats with abdominal infection. World J Gastroenterol. (2006) 12:4352. doi: 10.3748/wjg.v12.i27.4352
65. Ríos-Covián D, Ruas-Madiedo P, Margolles A, Gueimonde M, de Los Reyes-Gavilán CG, Salazar N. Intestinal short chain fatty acids and their link with diet and human health. Front Microbiol. (2016) 7:185. doi: 10.3389/fmicb.2016.00185
66. Costello EK, Gordon JI, Secor SM, Knight R. Postprandial remodeling of the gut microbiota in Burmese pythons. ISME J. (2010) 4:1375–85. doi: 10.1038/ismej.2010.71
67. Hills RDJ, Pontefract BA, Mishcon HR, Black CA, Sutton SC, Theberge CR. Gut microbiome: profound implications for diet and disease. Nutrients. (2019) 11:1613. doi: 10.3390/nu11071613
68. Demehri FR, Barrett M, Teitelbaum DH. Changes to the intestinal microbiome with parenteral nutrition: Review of a murine model and potential clinical implications. Nutr Clin Pract. (2015) 30:798–806. doi: 10.1177/0884533615609904
69. Miyasaka EA, Feng Y, Poroyko V, Falkowski NR, Erb-Downward J, Gillilland MG III, et al. Total parenteral nutrition-associated lamina propria inflammation in mice is mediated by a MyD88-dependent mechanism. J Immunol. (2013) 190:6607–15. doi: 10.4049/jimmunol.1201746
70. Hodin CM, Visschers RGJ, Rensen SS, Boonen B, Olde Damink SWM, Lenaerts K, et al. Total parenteral nutrition induces a shift in the Firmicutes to Bacteroidetes ratio in association with Paneth cell activation in rats. J Nutr. (2012) 142:2141–7. doi: 10.3945/jn.112.162388
71. Harvey RB, Andrews K, Droleskey RE, Kansagra KV, Stoll B, Burrin DG, et al. Qualitative and quantitative comparison of gut bacterial colonization in enterally and parenterally fed neonatal pigs. Curr Issues Intest Microbiol. (2006) 7:61–4.
72. Buchman AL, Moukarzel AA, Bhuta S, Belle M, Ament ME, Eckhert CD, et al. Parenteral nutrition is associated with intestinal morphologic and functional changes in humans. JPEN J Parenter Enteral Nutr. (1995) 19:453–60. doi: 10.1177/0148607195019006453
73. Taur Y, Jenq RR, Perales M-A, Littmann ER, Morjaria S, Ling L, et al. The effects of intestinal tract bacterial diversity on mortality following allogeneic hematopoietic stem cell transplantation. Blood. (2014) 124:1174–82. doi: 10.1182/blood-2014-02-554725
74. Andersen S, Staudacher H, Weber N, Kennedy G, Varelias A, Banks M, et al. Pilot study investigating the effect of enteral and parenteral nutrition on the gastrointestinal microbiome post-allogeneic transplantation. Br J Haematol. (2019) 2:188. doi: 10.1111/bjh.16218
75. D’Amico F, Biagi E, Rampelli S, Fiori J, Zama D, Soverini M, et al. Enteral nutrition in pediatric patients undergoing hematopoietic SCT promotes the recovery of gut microbiome homeostasis. Nutrients. (2019) 11:2958. doi: 10.3390/nu11122958
76. Iyama S, Tatsumi H, Shiraishi T, Yoshida M, Tatekoshi A, Endo A, et al. Possible clinical outcomes using early enteral nutrition in individuals with allogeneic hematopoietic stem cell transplantation: a single-center retrospective study. Nutrition. (2021) 83:111093. doi: 10.1016/j.nut.2020.111093
77. Krezalek MA, Yeh A, Alverdy JC, Morowitz M. Influence of nutrition therapy on the intestinal microbiome. Curr Opin Clin Nutr Metab Care. (2016) 20:1. doi: 10.1097/MCO.0000000000000348
78. Gibson GR, Hutkins R, Sanders ME, Prescott SL, Reimer RA, Salminen SJ, et al. Expert consensus document: the International Scientific Association for Probiotics and Prebiotics (ISAPP) consensus statement on the definition and scope of prebiotics. Nat Rev Gastroenterol Hepatol. (2017) 14:491–502. doi: 10.1038/nrgastro.2017.75
79. Zhou Z, Zhang Y, Zheng P, Chen X, Yang Y. Starch structure modulates metabolic activity and gut microbiota profile. Anaerobe. (2013) 24:71–8. doi: 10.1016/J.ANAEROBE.2013.09.012
80. Hamer HM, Jonkers D, Venema K, Vanhoutvin S, Troost FJ, Brummer RJ. Review article: the role of butyrate on colonic function. Aliment Pharmacol Ther. (2008) 27:104–19. doi: 10.1111/J.1365-2036.2007.03562.X
81. Arpaia N, Campbell C, Fan X, Dikiy S, Van Der Veeken J, Deroos P, et al. Metabolites produced by commensal bacteria promote peripheral regulatory T-cell generation. Nature. (2013) 504:451–5. doi: 10.1038/nature12726
82. Iyama S, Sato T, Tatsumi H, Hashimoto A, Tatekoshi A, Kamihara Y, et al. Efficacy of enteral supplementation enriched with glutamine, fiber, and oligosaccharide on mucosal injury following hematopoietic stem cell transplantation. Case Rep Oncol. (2014) 7:692–9. doi: 10.1159/000368714
83. Yoshifuji K, Inamoto K, Kiridoshi Y, Takeshita K, Sasajima S, Shiraishi Y, et al. Prebiotics protect against acute graft-versus-host disease and preserve the gut microbiota in stem cell transplantation. Blood Adv. (2020) 4:4607–17. doi: 10.1182/bloodadvances.2020002604
84. Drago-Serrano ME, Campos-Rodriguez R, Carrero JC, de la Garza M. Lactoferrin and Peptide-derivatives: Antimicrobial Agents with Potential Use in Nonspecific Immunity Modulation. Curr Pharm Des. (2018) 24:1067–78. doi: 10.2174/1381612824666180327155929
85. Pammi M, Suresh G. Enteral lactoferrin supplementation for prevention of sepsis and necrotizing enterocolitis in preterm infants (Review). Cochrane Database Syst Rev. (2017) 6:CD007137. doi: 10.1002/14651858.CD007137.pub5
86. Manzoni P. Clinical benefits of lactoferrin for infants and children. J Pediatr. (2016) 173:S43–52. doi: 10.1016/j.jpeds.2016.02.075
87. Sherman MP, Sherman J, Arcinue R, Niklas V. Randomized control trial of human recombinant lactoferrin: a substudy reveals effects on the fecal microbiome of very low birth weight infants. J Pediatr. (2016) 173:S37–42. doi: 10.1016/j.jpeds.2016.02.074
88. Rota H, One A, Cmv D, The D. Lactoferrin for gut GVHD. Bone Marrow Transplant. (2001) 28:1091–2. doi: 10.1038/sj.bmt.1703283
89. D’Amico F, Decembrino N, Muratore E, Turroni S, Muggeo P, Mura R, et al. Oral lactoferrin supplementation during induction chemotherapy promotes gut microbiome eubiosis in pediatric patients with hematologic malignancies. Pharmaceutics. (2022) 14:1705. doi: 10.3390/pharmaceutics14081705
90. Hill C, Guarner F, Reid G, Gibson GR, Merenstein DJ, Pot B, et al. The international scientific association for probiotics and prebiotics consensus statement on the scope and appropriate use of the term probiotic. Nat Rev Gastroenterol Hepatol. (2014) 11:506–14. doi: 10.1038/nrgastro.2014.66
91. Ciernikova S, Kasperova B, Drgona L, Smolkova B, Stevurkova V, Mego M. Targeting the gut microbiome: an emerging trend in hematopoietic stem cell transplantation. Blood Rev. (2021) 48:100790. doi: 10.1016/j.blre.2020.100790
92. Andermann TM, Rezvani A, Bhatt AS. Microbiota manipulation with prebiotics and probiotics in patients undergoing stem cell transplantation. Curr Hematol Malig Rep. (2016) 11:19–28. doi: 10.1007/s11899-016-0302-9
93. Ambesh P, Stroud S, Franzova E, Gotesman J, Sharma K, Wolf L, et al. Recurrent Lactobacillus bacteremia in a patient with leukemia. J Investig Med High Impact Case Rep. (2017) 5:232470961774423. doi: 10.1177/2324709617744233
94. Vahabnezhad E, Mochon AB, Wozniak LJ, Ziring DA. Lactobacillus bacteremia associated with probiotic use in a pediatric patient with ulcerative colitis. J Clin Gastroenterol. (2013) 47:437–9. doi: 10.1097/MCG.0b013e318279abf0
95. Salminen MK, Rautelin H, Tynkkynen S, Poussa T, Saxelin M, Valtonen V, et al. Lactobacillus bacteremia, clinical significance, and patient outcome, with special focus on probiotic L. Rhamnosus GG. Clin Infect Dis. (2004) 38:62–9. doi: 10.1086/380455
96. Ladas EJ, Bhatia M, Chen L, Sandler E, Petrovic A, Berman DM, et al. The safety and feasibility of probiotics in children and adolescents undergoing hematopoietic cell transplantation. Bone Marrow Transplant. (2016) 51:262–6. doi: 10.1038/bmt.2015.275
97. Cesaro S, Chinello P, Rossi L, Zanesco L. Saccharomyces cerevisiae fungemia in a neutropenic patient treated with Saccharomyces boulardii. Support Care Cancer. (2014) 8:504–5. doi: 10.1007/S005200000123
98. Lolis N, Veldekis D, Moraitou H, Kanavaki S, Velegraki A, Triandafyllidis C, et al. Saccharomyces boulardiifungaemia in an intensive care unit patient treated with caspofungin. Crit Care. (2008) 12:414. doi: 10.1186/CC6843
99. Tavil B, Koksal E, Songul Yalcin S, Uckan D. Pretransplant nutritional habits and clinical outcome in children undergoing hematopoietic stem cell transplant. Exp Clin Transplant. (2012) 10:55–61. doi: 10.6002/ect.2011.0082
100. Gorshein E, Wei C, Ambrosy S, Budney S, Vivas J, Shenkerman A, et al. Lactobacillus rhamnosus GG probiotic enteric regimen does not appreciably alter the gut microbiome or provide protection against GVHD after allogeneic hematopoietic stem cell transplantation. Clin Transplant. (2017) 31:e12947. doi: 10.1111/ctr.12947
101. Spencer CN, McQuade JL, Gopalakrishnan V, McCulloch JA, Vetizou M, Cogdill AP, et al. Dietary fiber and probiotics influence the gut microbiome and melanoma immunotherapy response. Science. (2021) 374:1632–40. doi: 10.1126/science.aaz7015
102. Raman M, Ambalam P, Kondepudi KK, Pithva S, Kothari C, Patel AT, et al. Potential of probiotics, prebiotics and synbiotics for management of colorectal cancer. Gut Microbes. (2013) 4:181–92. doi: 10.4161/GMIC.23919
103. Bindels LB, Neyrinck AM, Claus SP, Le Roy CI, Grangette C, Pot B, et al. Synbiotic approach restores intestinal homeostasis and prolongs survival in leukaemic mice with cachexia. ISME J. (2015) 10:1456–70. doi: 10.1038/ismej.2015.209
104. Salminen S, Collado MC, Endo A, Hill C, Lebeer S, Quigley EMM, et al. The International Scientific Association of Probiotics and Prebiotics (ISAPP) consensus statement on the definition and scope of postbiotics. Nat Rev Gastroenterol Hepatol. (2021) 18:649–67. doi: 10.1038/s41575-021-00440-6
105. Kalinina O, Talley S, Zamora-Pineda J, Paik W, Campbell EM, Knight KL. Amelioration of graft-versus-host disease by exopolysaccharide from a commensal bacterium. J Immunol. (2021) 206:2101–8. doi: 10.4049/jimmunol.2000780
106. Markey KA, Schluter J, Gomes ALC, Littmann ER, Pickard AJ, Taylor BP, et al. The microbe-derived short-chain fatty acids butyrate and propionate are associated with protection from chronic GVHD. Blood. (2020) 136:130–6. doi: 10.1182/blood.2019003369
107. Riwes M, Reddy P. Short chain fatty acids: postbiotics/metabolites and graft versus host disease colitis. Semin Hematol. (2020) 57:1–6. doi: 10.1053/j.seminhematol.2020.06.001
108. Gill PA, van Zelm MC, Muir JG, Gibson PR. Review article: short chain fatty acids as potential therapeutic agents in human gastrointestinal and inflammatory disorders. Aliment Pharmacol Ther. (2018) 48:15–34. doi: 10.1111/apt.14689
109. Ghiboub M, Penny S, Verburgt CM, Sigall Boneh R, Wine E, Cohen A, et al. Metabolome changes with diet-induced remission in pediatric Crohn’s disease. Gastroenterology. (2022) 163:922–36.e15. doi: 10.1053/j.gastro.2022.05.050
110. Ruutu T, Eriksson B, Remes K, Juvonen E, Volin L, Remberger M, et al. Ursodeoxycholic acid for the prevention of hepatic complications in allogeneic stem cell transplantation. Blood. (2002) 100:1977–83. doi: 10.1182/blood-2001-12-0159
Keywords: gut microbiota, hematopoietic stem cell transplantation, nutrition, diet, enteral nutrition, prebiotics, lactose
Citation: Muratore E, Leardini D, Baccelli F, Venturelli F, Prete A and Masetti R (2022) Nutritional modulation of the gut microbiome in allogeneic hematopoietic stem cell transplantation recipients. Front. Nutr. 9:993668. doi: 10.3389/fnut.2022.993668
Received: 13 July 2022; Accepted: 04 October 2022;
Published: 20 October 2022.
Edited by:
Sonia González, University of Oviedo, SpainReviewed by:
Shigeo Fuji, Osaka International Cancer Institute, JapanShoubao Ma, Soochow University, China
Copyright © 2022 Muratore, Leardini, Baccelli, Venturelli, Prete and Masetti. This is an open-access article distributed under the terms of the Creative Commons Attribution License (CC BY). The use, distribution or reproduction in other forums is permitted, provided the original author(s) and the copyright owner(s) are credited and that the original publication in this journal is cited, in accordance with accepted academic practice. No use, distribution or reproduction is permitted which does not comply with these terms.
*Correspondence: Francesco Baccelli, francesco.baccelli2@studio.unibo.it
†These authors have contributed equally to this work and share first authorship