- 1Institute of Animal Rearing Technologies, Faculty of Animal Sciences, Lithuanian University of Health Sciences, Kaunas, Lithuania
- 2Department of Food Safety and Quality, Faculty of Veterinary, Lithuanian University of Health Sciences, Kaunas, Lithuania
- 3Department of Anatomy and Physiology, Faculty of Veterinary, Lithuanian University of Health Sciences, Kaunas, Lithuania
- 4Institute of Microbiology and Virology, Faculty of Veterinary, Lithuanian University of Health Sciences, Kaunas, Lithuania
- 5Marine Research Institute, Klaipeda University, Klaipeda, Lithuania
- 6Lithuanian Research Centre for Agriculture and Forestry, Institute of Horticulture, Babtai, Lithuania
- 7Institute of Agricultural and Food Sciences, Vytautas Magnus University, Agriculture Academy, Kaunas, Lithuania
- 8Laboratory for Process Engineering, Environment, Biotechnology and Energy, Faculty of Engineering, University of Porto, Porto, Portugal
- 9Associate Laboratory in Chemical Engineering, Faculty of Engineering, University of Porto, Porto, Portugal
Algal biomass (AB) is prospective source of valuable compounds, however, Baltic Sea macroalgae have some challenges, because of their high microbial and chemical contamination. These problems can be solved, by using appropriate technologies for AG pre-treatment. The aim of this study was to evaluate the influence of two pre-treatments, solid-state fermentation with the Lactiplantibacillus plantarum LUHS135 and ultrasonication, on the antioxidant and antimicrobial characteristics of macro- (Cladophora rupestris, Cladophora glomerata, Furcellaria lumbricalis, Ulva intestinalis) and Spirulina (Arthrospira platensis) extracts. Also, combinations of extracts and LUHS135 were developed and their characteristics were evaluated. The total phenolic compound content was determined from the calibration curve and expressed in mg of gallic acid equivalents; antioxidant activity was measured by a Trolox equivalent antioxidant capacity assay using the DPPH• (1,1-diphenyl-2-picrylhydrazyl), ABTS•+ 2,2′-azinobis-(3-ethylbenzothiazoline-6-sulfonic acid), FRAP (Ferric Reducing Ability of Plasma) discoloration methods. Antimicrobial activity was measured by using agar well diffusion assay and in a liquid medium. The highest DPPH• and ABTS•+ was shown by C.rupestris and F.lumbricalis extract × LUHS135 combinations, the highest FRAP - by non-pretreated C.rupestris and F.lumbricalis extract × LUHS135 combinations. Ultrasonicated samples inhibited four out of seven tested pathogens. Finally, the tested pre-treatments showed good perspectives and can be recommended for AB valorization.
Introduction
Algal biomass can be converted into a wide range of functional products (1). Despite, that they are a valuable source of functional compounds, our previous studies showed that the application of Baltic Sea macroalgae have some challenges because of their high microbial and chemical contamination (2). However, algae safety parameters could be improved by applying ethanolic extraction, which is a suitable technology for pathogen decontamination and reduces the toxic metal concentration in algae extracts (3). In addition to improvements in algae products' safety parameters, it would be very beneficial to increase extraction efficiency. Therefore, in this study, two methods for algae pre-treatment were tested before extraction: (I) solid-state fermentation (SSF) with a selected lactic acid bacteria (LAB) strain and (II) ultrasonication. We hypothesized that algae biomass pre-treatment before extraction can lead to better properties of the extracts (higher antioxidant activity and total phenolic compound (TPC) content, as well as stronger antimicrobial properties against a broader spectrum of pathogenic and opportunistic strains). In addition, to increase the antimicrobial and antioxidant activity of the prepared extracts, combinations of algae extracts and a pure Lactiplantibacillus plantarum LUHS135 strain were developed. Our previous studies showed that the above-mentioned strain inhibits various pathogenic and opportunistic microorganisms and is suitable for fermentation of various substrates (4–7). The importance of algae biomass pre-treatment before extraction can be explained by algae cell composition, which is protected by complex cell walls (8, 9). It has been reported that the crucial step in obtaining bioactive compounds from micro- and macroalgal biomass is to achieve efficient cell disruption (10). Some algae pre-treatment technologies are described in the literature, and the most effective mechanical and biological techniques were mentioned (11, 12). Despite the fact that physical pre-treatment was found to be a cost-intensive process, ultrasonication was recommended as the most promising method for cell disintegration (9, 13, 14). Ultrasound breaks the cell structure and improves material transfer by enhancing the extraction from microalgae (9, 15–17). Also, biological pre-treatment with fungi, bacteria and/or their enzymes can be used to degrade lignin and hemicelluloses of algae cells (12, 18). There are numerous studies on algae pre-treatment using biological tools (19–21). In addition to the breakdown of lignin, biological pre-treatment generates other valuable compounds such as phenolic acids, benzoic acid, syringaldehyde, etc. (22). Other major advantages of biological pre-treatment are low energy consumption, simple operating conditions and equipment, no requirement for recycling the chemicals after pre-treatment, etc. (23–25). Solid state fermentation (SSF) process is based on the microorganisms grown on solid or semi-solid substrates or supports, and is more effective than the liquid phase submerged fermentation (26). We hypothesized that algae biomass SSF can lead to the deeper algae cells breakdown, which will lead to better properties of the extracts.
The aim of this study was to evaluate the influence of two pre-treatments, solid-state fermentation (SSF) with the Lactiplantibacillus plantarum LUHS135 strain and ultrasonication (for 45 min at 35 kHz), on the antioxidant and antimicrobial characteristics of macroalgae (Cladophora rupestris, Cladophora glomerata, Furcellaria lumbricalis and Ulva intestinalis) and microalgae [Spirulina (Arthrospira platensis)] extracts. In addition, combinations of algae extracts and the pure LUHS135 strain were developed and their antioxidant and antimicrobial characteristics were evaluated.
Materials and methods
Algae samples and lactic acid bacteria strain used in experiments
Samples of macroalgae (Furcellaria lumbricalis, Ulva intestinalis, Cladophora rupestris and Cladophora glomerata) were collected in May–June of 2021 on the Lithuanian coast. Ulva intestinalis and C. glomerata samples were taken from stones near the surface, while F. lumbricalis and C. rupestris samples were taken after a storm along the shore. The collected samples were cleaned three times in distilled water to remove sand and macroscopic invertebrates. Microalgae Spirulina (Arthrospira platensis) was purchased from the University of Texas Biological Labs (Austin, Texas, United States), multiplied according to instructions given by producer and used in experiments.
Before the experiments, all algal samples were lyophilized using a freeze-dryer FD8512S (ilShin® Europe, Ede, The Netherlands) and ground into a powder (particle size <0.2 mm) using a knife mill GM200 (Retsch, Düsseldorf, Germany). Freeze-dried samples were maintained at room temperature in a dark place until they were used.
The Lactiplantibacillus plantarum LUHS135 strain (LUHS135) was obtained from the Lithuanian University of Health Sciences collection (Kaunas, Lithuania). The characteristics of the LAB strain used, including the inhibition of strains of pathogenic and opportunistic bacteria, and fungi are described by Bartkiene et al. (4). In addition, our previous studies showed that fermentation of feed with LUHS135 had a positive influence in vivo on piglets' health parameters (27–29). The above-mentioned LAB strains were stored at−80°C in a Microbank system (Pro-Lab Diagnostics, United Kingdom) and propagated in de Man–Rogosa–Sharpe (MRS) broth (CM 0359, Oxoid Ltd, Hampshire, United Kingdom) at 30 ± 3°C for 48 h before their use for algae fermentation.
Fermentation and ultrasonication of algal samples
The LUHS135 strain was multiplied as described in Algae samples and lactic acid bacteria strain used in experiments and used for algae powder (AP) fermentation. A total of 3 mL of the LAB strain multiplied in MRS (cell concentration, on average, 9.0 log10 CFU mL−1) was inoculated to 100 g of AP media (for 100 g of AP, 60 mL of water was used) and fermented at 30 ± 2°C for 60 h. Control samples for pH analysis were prepared without the addition of LAB. Our previous studies showed that pure algae samples are not suitable substrates for effective LAB growth (2), thus 2% (from the algae sample amount) of yeast extract was added (ThermoFisher, Kandel, Germany), with the purpose of improving the growth of the LUHS135. Anaerobic conditions were attained by incubating the fermentable substrate in anaerobic jars (Oxoid, Basingstoke, Hampshire, United Kingdom), with GasPak Plus™ (BBL, Cockeysville MD, United States). Samples for pH analysis were taken after 12, 24, 36, 48 and 60 h of fermentation.
Algae samples were ultrasonicated before extract preparation for 45 min at 35 kHz (temperature of samples during the ultrasonication was 40 ± 2°C) using ultrasonic bath (Bandelin Sonorex, Bandelin electronic GmbH & Co. KG, Berlin, Germany).
Both fermented and ultrasonicated algae samples were lyophilised and used for extract preparation.
Extracts and extract × lactiplantibacillus plantarum LUHS135 strain combinations preparation
Five grams of the lyophilized algal samples (non-pretreated, fermented and ultrasonicated, for a total of 15 samples) were extracted with 100 mL of ethanol/water (70:30 v/v) (30) by incubation at room temperature (22 ± 2°C) overnight with stirring (Vibramax 100, Heidolph, Nuremberg, Germany). Then, extracts were centrifuged at 3,500 rpm for 10 min at 4°C and filtered through Whatman No. 4 filter paper. Ethanol was removed by rotary evaporation in the extract. The concentrate and the supernatant of the extract were lyophilized and weighted.
For the preparation of extract × LUHS135 strain combinations, it was propagated in MRS broth (CM 0359, Oxoid Ltd, Hampshire, United Kingdom) at 30 ± 3°C for 48 h, and a pure LUHS135 strain was used (LUHS135 strain/algae extract; 50/50, by volume). The principal scheme of the experiment is given in Figure 1. Three groups of samples were prepared: (I) extracts and extracts × LUHS135 combinations prepared from non-pre-treated algae, (II) extracts and extracts × LUHS135 combinations prepared from ultrasonicated algae and (III) extracts and extracts × LUHS135 combinations prepared from fermented algae. In every group pure extract as well as extract combinations with the LUHS strain were tested (ClaR = Cladophora rupestris; ClaG = Cladophora glomerata; Ul = Ulva intestinalis; Furc = Furcellaria lumbricalis; Sp = Spirulina (Arthrospira platensis); non = extracts prepared from non-pre-treated algae; ultr = extracts prepared from ultrasonicated algae; ferm = extracts prepared from fermented algae; LUHS135 = extract × LUHS135 strain combination). There were 30 samples total: Group (I): ClaRnon, ClaRnonLUHS135, ClaGnon, ClaGnonLUHS135, Furcnon, FurcnonLUHS135, Ulnon, Ul nonLUHS135, Spnon and SpnonLUHS135; Group (II): ClaRultr, ClaRultrLUHS135, ClaGultr, ClaGultrLUHS135, Furcultr, FurcultrLUHS135, Ulultr, UlultrLUHS135, Spultr and SpultrLUHS135 and Group (III): ClaRferm, ClaRfermLUHS135, ClaGferm, ClaGfermLUHS135, Furcferm, FurcfermLUHS135, Ulferm, UlfermLUHS135, Spferm and SpfermLUHS135.
Analysis of algae color characteristics and pH
The color coordinates of the algae extracts and their combinations with the LUHS135 strain were evaluated using a CIE L*a*b* system (CromaMeter CR-400, Konica Minolta, Marunouchi, Tokyo, Japan) (3). The pH of samples was evaluated with an “inoLab pH Level 3” pH meter (Hanna Instruments, Weilheim, Germany).
Determination of the total phenolic compound content
The total phenolic compound (TPC) content in the extracts was determined according to the Folin–Ciocalteu method (31) with slight modifications (32). Samples (1.0 mL) were introduced into test cuvettes followed by 5.0 mL 10% (1/10, v/v) of Folin–Ciocalteu's reagent by diluting a stock solution with ultra-pure distilled water and 4.0 mL of Na2CO3 (7.5%). The system was then placed at ambient temperature for 1 h. The absorbance was measured at 765 nm using a Genesys-10 UV/VIS spectrophotometer (Thermo Spectronic, Rochester, NY, United States). The concentration of TPC was determined from the calibration curve and expressed in mg of gallic acid equivalents (GAE) in ml of extracts.
Determination of the antioxidant capacity of algae extracts
The antioxidant activity of algae extracts was measured by DPPH•, ABTS•+ and FRAP discoloration methods. Calculation of all antioxidant activity assays was carried out using Trolox calibration curves and expressed as μmol of the Trolox equivalent (TE) per one gram of ml of extract (μmol TE/ml).
DPPH• activity
The DPPH• (2,2-diphenyl-1-picrylhydrazyl hydrate free radical) scavenging capacity of the algal extracts was determined by the method of Brand-Williams et al. (33) with slight modifications (34). Twenty microliters of extract were allowed to react with 2 mL of DPPH• ethanolic solution (2 mL, 6 × 10−5 M) by mixing in a cuvette with a 1 cm path length for 30 min in the dark. The decrease in absorbance was measured at 515 nm using a Genesys-10 UV/VIS spectrophotometer (Thermo Spectronic, Rochester, NY, United States).
ABTS•+ activity
The radical scavenging activity of extracts was also measured by ABTS•+ (2,2′-azino-bis(3-ethylbenzthiazoline-6-sulphonic acid) radical cation assay (35) as described by Urbonaviciene et al. (32). ABTS•+ solution (2 mM) was prepared by dissolving 2,2′-azinobis (3-ethylbenzothiazoline-6-sulfonic acid) diammonium salt in 50 mL of phosphate-buffered saline (PBS) obtained by dissolving 8.18 g NaCl, 0.27 g KH2PO4, 1.42 g Na2HPO4 and 0.15 g KCl in 1 L of pure water. The pH of the prepared solution was adjusted to 7.4 using NaOH. Then the K2S2O8 solution (70 mM) was prepared in pure water. Briefly, 2 mL of ABTS•+ radical solution was mixed with 20 μL extract also in a 1 cm path length cuvette. The reaction mixture was kept at ambient temperature in the dark for 30 min, and the absorbance was read at 734 nm using a Genesys-10 UV/Vis spectrophotometer (Thermo Spectronic, Rochester, NY, United States). Trolox was used as a standard. A duplicate determination was made from each extract.
FRAP activity
The ferric reducing antioxidant power (FRAP) assay was carried out by the method of Benzie and Strain (36) with some modifications (37). For the FRAP assay, 0.3 M of sodium acetate buffer (pH 3.6) was prepared by dissolving 3.1 g of sodium acetate and 16 mL of acetic acid in 1,000 mL of distilled water; a 10 mM TPTZ solution was prepared by dissolving 0.031 g of TPTZ in 10 mL of 40 mM HCl; and a 20 mM ferric solution was prepared by dissolving 0.054 g of FeCl3·6H2O in 10 mL of distilled water. Working FRAP reagent was prepared by freshly mixing acetate buffer, TPTZ and ferric solutions at a ratio of 10:1:1. Two milliliters of freshly prepared FRAP working solution and 20 μL of extract were mixed and incubated for 30 min at ambient temperature. The change in absorbance due to the reduction of the ferric-tripyridyltriazine (Fe III-TPTZ) complex by the antioxidants present in the samples was measured at 593 nm using a Genesys-10 UV/VIS spectrophotometer.
Evaluation of the antimicrobial activity of algal extract samples
The algal extracts as well as algal extract × LUHS135 strain combination antimicrobial properties were evaluated by testing their abilities to inhibit the following pathogenic and opportunistic strains: Salmonella enterica, Bacillus cereus, Enterococcus faecium, Staphylococcus aureus, Escherichia coli, Streptococcus mutans and Enterococcus faecalis. Antimicrobial properties of the samples were evaluated by using the agar well diffusion method and in a liquid medium.
For the agar well diffusion assay, suspensions of 0.5 McFarland standard of each pathogenic bacterial strain were inoculated onto the surface of cooled Mueller–Hinton agar (Oxoid, Basingstoke, UK) using sterile cotton swabs. Wells 6 mm in diameter were punched in the agar and filled with 50 μL of the algal extract. The antimicrobial activities against the tested bacteria were established by measuring the inhibition zone diameters (mm). The experiments were repeated three times, and the average diameter of the inhibition zones in mm was calculated.
To evaluate the antimicrobial activity of the algal extracts and algal extracts × LUHS135 combinations in liquid medium, the algal samples were diluted 1:3 (v/v) with physiological solution. Then we added 10 μL of the pathogenic and opportunistic bacterial strains, cultured in a selective medium, to the different concentrations of samples (500 and 2,000 μL) and incubated them at 35°C for 24 h. After incubation, the viable pathogenic and opportunistic bacterial strains in algal extract and/or in algal extracts × LUHS135 combination were controlled by plating them on selective medium. The results were interpreted as (–) if the pathogens did not grow on the selective medium and (+) if the pathogens grew on the selective medium. Experiments were performed in triplicate.
Statistical analysis
Extract preparation of algal samples was performed in duplicate, while all analytical experiments were carried out in triplicate. The calculated mean values, using the statistical package SPSS for Windows (Ver.15.0, SPSS, Chicago, IL, United States), were compared using Duncan's multiple range test with significance defined at p ≤ 0.05. A linear Pearson's correlation was used to quantify the strength of the relationship between the variables. The results were recognized as statistically significant at p ≤ 0.05.
Results and Discussion
Selection of algae fermentation duration before extract preparation according to changes in their pH
The changes in pH values during algae fermentation are shown in Figure 2. In comparison to the non-fermented samples, a pH higher than 7.0 was established for Cladophora rupestris, Ulva intestinalis and Spirulina samples (7.35, 7.98 and 7.72, respectively). Non-fermented Cladophora glomerata and Furcellaria lumbricalis samples had average pH values of 5.95 and 6.74, respectively. The most intensive fermentation and reductions of pH values was found from 0–12 h and from 12–24 h of fermentation. From 0–12 h and from 12–24 h of fermentation the pH values of Cladophora rupestris, Cladophora glomerata, Ulva intestinalis, Furcellaria lumbricalis and Spirulina samples reduced by an average of 1.36 and 1.12, 1.18 and 1.04, 1.17 and 1.13, 1.26 and 1.27 and 1.28 and 1.19 times, respectively.
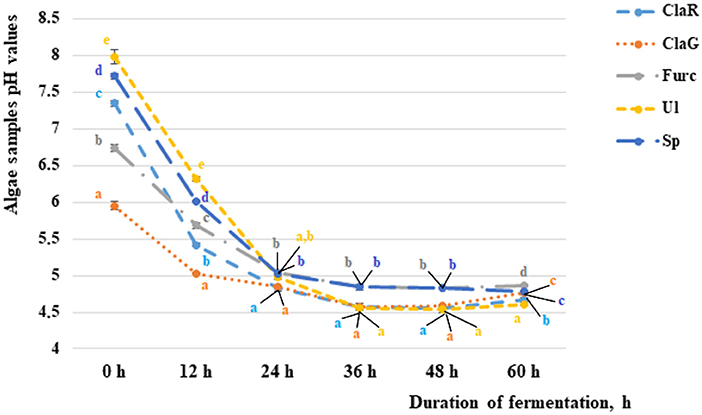
Figure 2. The pH of the non-fermented and fermented algae samples after 12, 24, 36, and 60 h of fermentation [ClaR - Cladophora rupestris; ClaG - Cladophora glomerata; Ul - Ulva intestinalis; Furc - Furcellari lumbricalis; Sp - Spirulina (Arthrospira platensis)]. Data are represented as means (n = 3) ± SE. Means with different letters (a–e) are significantly different (p ≤ 0.05). The color of the letters coincides with the color of the sample in the graph.
Although fermentation during the period from 24–36 h was not as intensive as fermentation in previous studies, after 36 h of fermentation significantly lower pH values for all of the tested algae samples were found when compared with samples fermented for 24 h. However, after 48 h of fermentation significant differences between the algae pH values were not found, and after 72 h of fermentation some of the algae sample pH values started to increase. Univariate analyses of variance showed that the variety of algae is a significant factor in sample pH (p = 0.017). However, the duration of fermentation and interaction with analyzed factors did not significantly affect the pH of the samples. According to these results, a fermentation duration of 36 h for extract preparation was selected.
Literature on algae fermentation is scarce; however, our previous studies showed that fermentation of the LUHS135 strain (duration of fermentation 12 h) significantly reduced the pH of C. rupestris. However, the pH of other tested algae samples (U. intestinalis and F. lumbricalis) remained unchanged (2). One of the main goals of the fermentation process is to drop the pH, and on average, the recommended pH for fermented food is 4.6. A decrease in pH is an indicator of an effective process; however, changes to the fermentable substrate can be caused by many factors, i.e., the technological microorganism's (used for fermentation) characteristics, nutrient source in fermentable media, duration of fermentation, humidity of the substrate, etc. It has been reported that the moisture content of the substrate has a significant influence on pH and, in most cases, lower pH values and higher total titratable acidity were obtained for peas in solid state fermentation conditions (38). The practice of LAB-based food, as well as feed fermentations, happened accidentally in the beginning, but soon spread due to its many benefits including nutrition, safety and flavor (38, 39). Overall, during the fermentation process many compounds are obtained as secondary metabolites of technological microorganisms (40, 41). Also, bound phenolic compounds are bio-converted from their conjugated forms to their free forms, and this is explained by their breakdown, activities of the fermentable substrate enzymes, as well as activity of technological microorganisms (42). Finally, this study showed that yeast extract is a suitable supplement for increasing algae samples fermentation effectiveness.
Color coordinates and pH of algae extracts and algae extracts × LUHS135 combinations
Color coordinates (L* = lightness; a* = redness; -a* = greenness; b* = yellowness; -b* = blueness) and pH of the algae extracts and algae extracts × LUHS135 combinations are shown in Table 1. When comparing all three groups of extracts (non-pre-treated, ultrasonicated and fermented before extraction), the lowest L* coordinates were from ClaGnon, ClaRultr and ClaRfermLUHS135 samples (42.5, 41.3 and 49.5 NBS, respectively). The most intensive greenness (-a*) was from Ulnon, Ulultr and Ulferm samples (-14.7,−13.7 and−6.86 NBS, respectively). The lowest yellowness (b*) was from ClaGnon, Spultr and ClaGferm samples (24.8, 23.7 and 23.1 NBS, respectively).
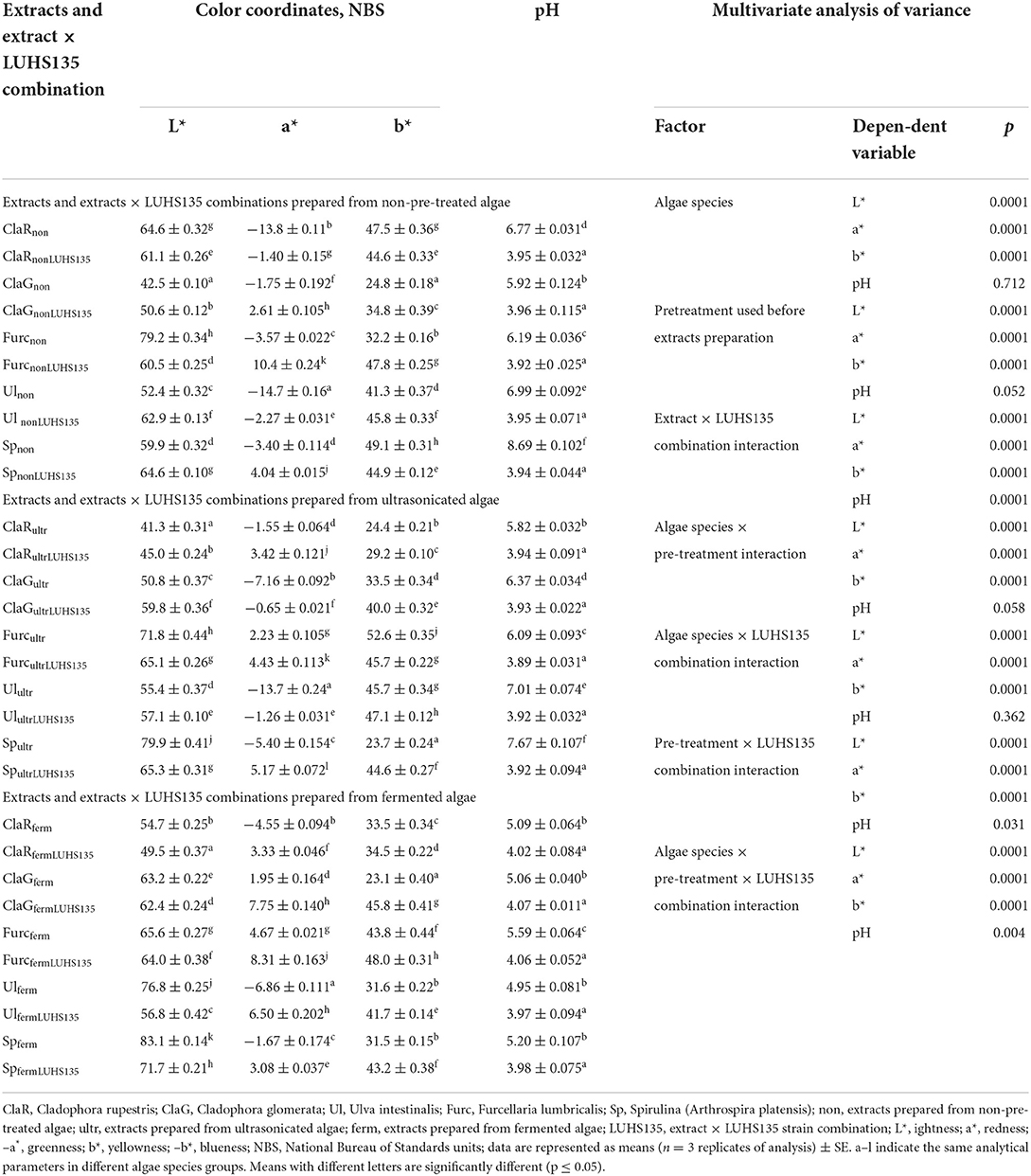
Table 1. Color coordinates (L*, lightness; a*, redness; –a*, greenness; b*, yellowness; –b*, blueness) and pH of the algae extracts and algae extracts × LUHS135 combinations.
When comparing all of the samples, all of the analyzed factors as well as their interactions had significant effects on all color coordinates; however, algae species, pre-treatment used before extract preparation, extract × LUHS135 combination interaction, algae species × pre-treatment interaction and the algae species × LUHS135 combination interaction did not have significant effects on pH of samples (Table 1). In contrast, the pre-treatment × LUHS135 combination interaction, as well as the algae species × pre-treatment × LUHS135 combination interaction, showed a significant influence on sample acidity (p = 0.031 and p = 0.004, respectively). Also, a weak, negative correlation between the sample pH and a* coordinate was found (r = −0.289, p = 0.006) (Table 2). In all cases, the addition of the LUHS135 multiplied strain reduces the algae extracts × LUHS135 combinations until an average pH of 3.96; however, the highest pH was for Spnon, Spultr and Furcferm samples (8.69, 7.67 and 5.59, respectively).
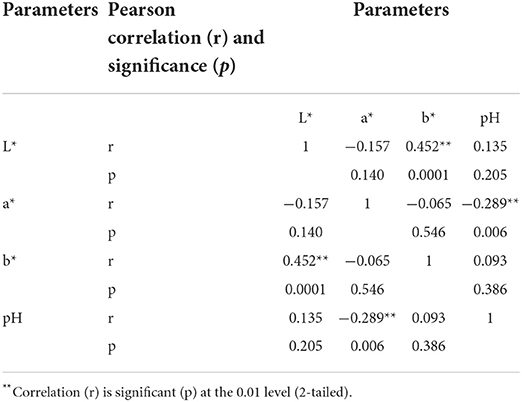
Table 2. Correlations between the color coordinates (L* = lightness; a* = redness; –a* = greenness; b* = yellowness; –b* = blueness) and pH of the algae extracts and algae extracts × LUHS135 combinations.
The color changes can be explained by the fact that during fermentation, the substrate is acidified, and organic acids have an influence on oxidation processes which can lead to color changes (38). In many cases, colored compounds lead to higher antioxidant properties of the product and/or extract; however, specific antioxidant properties are related to specific phenolic compound profile composition (3). However, oxidation of diffused phenolic compounds can also occur (43). In addition to fermentation, ultrasonication could cause color changes in compounds. Ultrasonic waves cause rapid compressions and expansions and destroy substrate cells, and the phenomenon of cavitation is responsible for a reduction of the diffusion boundary layer (44–48). It has been reported that ultrasonication increases extraction efficiency (49, 50). However, other published studies showed that the use of ultrasound as a pre-treatment for carrots contributed to significant changes in their color (51). From this point of view, it is very important to evaluate the changes of the antioxidant properties of the treated samples because reductions in colored compounds could lead to lower antioxidant activity. For this reason, during the second stage of the experiment, antioxidant activities and total phenolic compound content were analyzed.
The total phenolic compound and antioxidant activity of algae extracts and algae extracts × LUHS135 combinations
The aim of this study was to evaluate Also, combinations of extracts and LUHS135 were developed and their characteristics were evaluated. The total phenolic compounds content was determined from the calibration curve and expressed in mg of gallic acid equivalents; antioxidant activity was measured by a Trolox equivalent antioxidant capacity assay using the DPPH• (1,1-diphenyl-2-picrylhydrazyl), ABTS•+ 2,2′-azinobis-(3-ethylbenzothiazoline-6-sulfonic acid), FRAP (Ferric Reducing Ability of Plasma) discoloration methods.
The total phenolic compounds (TPC) content of the algae extracts and the influence of two pre-treatments, solid-state fermentation with the Lactiplantibacillus plantarum LUHS135 and ultrasonication is given in Table 3. In comparison, the TPC content multivariate analysis of variance showed that algae species (p ≤ 0.0001), algae × pre-treatment before extraction interaction (p ≤ 0.0001) and algae species × LUHS135 combination interaction (p ≤ 0.003) had significant effects on TPC content in samples. The lowest TPC content in the non-pre-treated samples group was found in ClaRnon, Ulnon and Spnon samples (on average 1.18 mg GAE/mL), and the highest was found in ClaRnonLUHS135 and FurcnonLUHS135 samples (on average 13.28 mg GAE/mL). In comparison, for extracts and extracts × LUHS135 combinations prepared from ultrasonicated algae, the lowest TPC content was found in Spultr samples (0.51 mg GAE/mL), and the highest TPC content was in ClaRultrLUHS135, ClaGultrLUHS135 and FurcultrLUHS135 samples (on average 12.23 mg GAE/mL). Similar tendencies were established in the fermented samples group, and the lowest TPC content was found in Spferm samples (2.77 mg GAE/mL) while the highest was in ClaRfermLUHS135 and FurcfermLUHS135 samples (on average 12.76 mg GAE/mL).
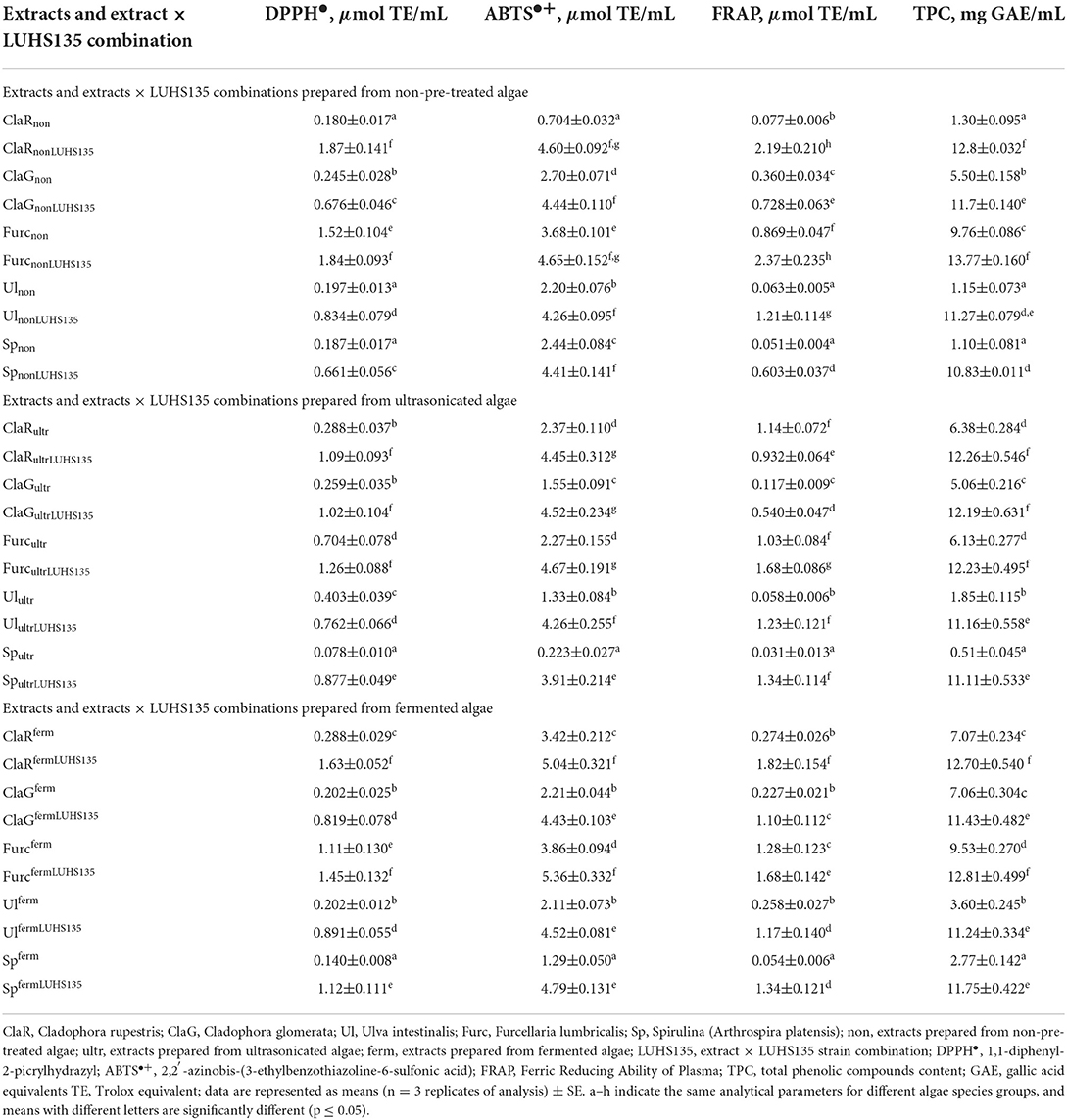
Table 3. Antioxidant activities and total phenolic compound content of algae extracts and algae extracts × LUHS135 combinations.
The antioxidant properties of two pre-treatments, solid-state fermentation with the Lactiplantibacillus plantarum LUHS135 and ultrasonication, on of macro- (Cladophora rupestris, Cladophora glomerata, Furcellaria lumbricalis, Ulva intestinalis) and Spirulina (Arthrospira platensis) extracts were estimated and compared by DPPH•, ABTS•+, and FRAP methods. In a comparison of the 2,2-diphenyl-1-picryhydrazyl (DPPH•) radical scavenging activity of all three groups of samples (non-pre-treated, ultrasonicated and fermented), multivariate analysis of variance showed that all of the analyzed factors and their interactions had significant effect on the DPPH• radical scavenging activity of the samples (factors: algae species and pre-treatment before extraction (fermentation and/or ultrasonication), LUHS135 combination, algae species × LUHS135 combination interaction, algae extract × pre-treatment before extraction interaction, pre-treatment before extraction × LUHS135 combination interaction and the algae species × LUHS135 combination × pre-treatment before extraction interaction, p ≤ 0.0001). In comparison to the non-pre-treated (before extraction) samples group, the lowest DPPH• radical scavenging activity was found in ClaRnon, Ulnon and Spnon samples (on average, 0.188 μmol TE/mL), and the highest DPPH• radical scavenging activity was shown in ClaRnonLUHS135 and FurcnonLUHS135 samples (on average 1.86 μmol TE/mL). In extracts and extracts × LUHS135 combinations prepared from ultrasonicated algae, the lowest DPPH• radical scavenging activity was found in Spultr (0.078 μmol TE/mL); however, ClaRultrLUHS135, ClaGultrLUHS135 and FurcultrLUHS135 samples showed an average of 14.4 times higher DPPH• radical scavenging activity. Similar to the ultrasonicated group samples, in fermented samples we found the lowest DPPH• radical scavenging activity in Spferm samples (0.140 μmol TE/mL) and the highest in ClaRfermLUHS135 and FurcfermLUHS135 samples (on average 1.54 μmol TE/mL). Also, DPPH• radical scavenging activity showed a weak positive correlation with samples' a* coordinates (r = 0.231, p = 0.028). The -a* and -b* coordinates are related to chlorophyll's (-a and -b) greenish lipid-soluble pigments and causes the typical coloration of green algae (52, 53). However, carotenoids with a higher number of conjugated double bonds show red color and possess antioxidant properties (54). Other colored algae compounds with antioxidant properties are astaxanthin (52, 55–59) and canthaxanthin (β,β-carotene-4,4′-dione), which belongs to xanthophylls, and is widely used as a feed additive as an antioxidant (60–64).
2, 2′-azino-bis ethylbenzthiazoline-6-sulfonic acid (ABTS•+) radical cation scavenging of the samples showed similar tendencies to DPPH• and FRAP, and a multivariate analysis of variance showed that all of the analyzed factors and their interactions had significant effects on sample ABTS•+ (algae species p ≤ 0.0001, pre-treatment before extraction p ≤ 0.0001, LUHS135 combination p ≤ 0.0001, algae species × LUHS135 combination interaction p = 0.015, algae extract × pre-treatment before extraction interaction p ≤ 0.0001, pre-treatment before extraction × LUHS135 combination interaction p ≤ 0.0001, algae species × LUHS135 combination × pre-treatment before extraction interaction p ≤ 0.0001). In comparison, in the non-pre-treated before extraction sample group, the lowest ABTS•+ was in ClaRnon samples (0.704 μmol TE/mL) and the highest was in ClaRnonLUHS135 and FurcnonLUHS135 samples (on average 4.63 μmol TE/mL). The highest ABTS•+ in the ultrasonicated group was from ClaRultrLUHS135, ClaGultrLUHS135 and FurcultrLUHS135 samples (on average 4.55 μmol TE/mL) and the lowest was from Spultr samples (0.223 μmol TE/mL). Similar tendencies were found in the fermented samples group: the lowest ABTS•+ was from Spferm samples (1.29 μmol TE/mL) and the highest was from ClaRfermLUHS135 and FurcfermLUHS135 (on average 5.20 μmol TE/mL). ABTS•+ showed a weak, positive correlation with samples' a* coordinates (r = 0.303, p = 0.004).
The ferric reducing antioxidant power (FRAP), which shows the ability of an antioxidant in reducing Fe(III) into Fe(II), demonstrated that all of the analyzed factors and their interactions had significant effects on the FRAP of the samples (p ≤ 0.0001). In comparison to the group that was not pre-treated before extraction, the lowest FRAP was established in Ulnon and Spnon samples (on average 0.057 μmol TE/mL) and the highest FRAP was found in ClaRnonLUHS135 and FurcnonLUHS135 samples (on average 2.28 μmol TE/mL). In comparison to the ultrasonicated sample group, the lowest FRAP was found in Spultr samples (0.031 μmol TE/mL) and the highest in FurcultrLUHS135 samples (1.68 μmol TE/mL). In the fermented samples group, the lowest FRAP was in Spferm samples (0.054 μmol TE/mL) and the highest was in ClaRfermLUHS135 (1.82 μmol TE/mL). FRAP showed a moderate negative correlation with the b* coordinates of samples (r = 0.509, p = 0.0001). Phycobilin pigments are found in cyanobacteria and in the chloroplasts of red algae (52, 65). Liutein has a strong antioxidant effect (66). The main colored compounds in microalgae are fucoxanthin, lutein and β-carotene, and they also are described as good antioxidants (58, 59, 67–69). Zeaxanthin is a xanthophyll family carotenoid (70) and possesses antioxidant properties as well (71–75).
In essence, the radical scavenging activities of DPPH• and ABTS•+ are based on the ability of antioxidants to donate a hydrogen atom or an electron to stabilize radicals by converting them to the non-radical species (76, 77). Our results reflected the ability of all prepared ethanolic extracts to donate a hydrogen atom or electron to both radicals. In general, algal extracts rich in natural polyphenolics can function as antioxidants (76, 78).
In this study, several methods based on different principles were used to determine the in vitro antioxidant activity of algae extracts. Other studies have reported that the FRAP method should be used in combination with other methods because it cannot measure all antioxidants of complex compounds (79, 80). Antioxidant properties of food and/or feed are desirable characteristics because antioxidants reduce oxidation processes (81). Also, it has been reported that both scavenging and antioxidant activities are related to TPC content (82). We found that TPC content in samples showed a moderate positive correlation with samples' a* coordinates (r = 0.592, p = 0.0001), a negative weak correlation with samples' pH (r = −0.294, p = 0.005) and a moderate positive correlation with samples' ABTS•+ (r = 0.300, p = 0.004) and FRAP (r = 0.247, p = 0.019). However, a correlation between the DPPH• and TPC content was not found. It was previously reported that in ethanolic extracts the correlation between TPC content and total antioxidant capacity is high, but the correlation with FRAP assay is minimal, and the correlation between the total antioxidant capacity and TPC content is positive and very significant in ethanolic extracts, whereas it is negative in methanolic ones (83). However, in the free form, phenolic compounds have a better bio-accessibility because of released free aglycones and increased antioxidative activity (84, 85), and fermentation could decrease free phenolic compound content in samples because they may bind with other molecules present in the fermentable matrix, i.e., might be hydrolysed and/or be degraded by microbial enzymes (42, 84). According to Li et al. (86), LAB fermentation has a significant impact on the phenolic profile, as well as on antioxidant activity, because during the process, various phenolic acids could be excreted to the fermentable matrix (86). It was reported that Furcellaria extracts, in comparison with Cladophora and Ulva sp., had the highest antioxidant activity of all the macroalgae alcoholic extracts tested (87). It has also been shown that the ethanolic extract of green and red seaweeds exhibit a high scavenging activity and a higher DPPH• of brown and green seaweeds in comparison with red (83, 88–90). The lower correlation between FRAP values and TPC content in extracts shows that the phenolic compounds are not involved in the antioxidant activity through this pathway, but there might be some effects involving other active compounds (83). The current study showed that the combinations of extracts and LUHS135 could improve antioxidant properties of the substrate.
Antimicrobial characteristics of the algal extracts
Antimicrobial activity of the algae extracts and algae extracts × LUHS135 combinations were evaluated using the agar well–diffusion method. The results are shown in Table 4 and Figure 3. In a comparison of all three groups (non-pre-treated, ultrasonicated and fermented), the highest number of samples (of all tested samples) that showed antimicrobial properties against at least one pathogen was found in the non-pre-treated samples group. All of the tested samples in this group showed inhibition properties against Bacillus cereus (the highest diameter of inhibition zones (DIZ), on average 16.0 mm, was found by ClaRnon, ClaGnon, ClaGnonLUHS135, Ul nonLUHS135 and SpnonLUHS135samples). Also, 3 out of 10 samples of this group showed inhibition properties against Enterococcus faecium (ClaRnon, ClaRnonLUHS135 and ClaGnonLUHS135, with DIZ of 15.3, 11.5 and 8.0 mm, respectively) and 4 out of 10 samples of this group showed inhibition properties against Staphylococcus aureus (ClaRnonLUHS135, ClaGnonLUHS135, FurcnonLUHS135and Ul nonLUHS135, with DIZ of 12.4, 11.5, 12.3 and 8.0 mm, respectively). Despite the fact that the highest number of samples (of all tested samples) showed antimicrobial properties against at least one pathogen in the non-pre-treated samples group, a broader spectrum of pathogen inhibition was found in the ultrasonicated sample group (inhibition properties against Bacillus cereus showed in ClaRultr, ClaRultrLUHS135 and SpultrLUHS135 samples, inhibition properties against Enterococcus faecium showed in ClaGultrLUHS135, inhibition properties against Staphylococcus aureus showed in ClaRultrLUHS135, ClaGultrLUHS135 and SpultrLUHS135 and inhibition properties against Stretococcus mutans showed in FurcultrLUHS135 and UlultrLUHS135 samples). In the comparison of extract samples prepared from fermented algae, ClaRferm, ClaRfermLUHS135 and FurcfermLUHS135 showed inhibition properties against one out of seven tested pathogens [ClaRferm and FurcfermLUHS135 inhibited Bacillus cereus (DIZ 16.3 and 13.1 mm, respectively) and FurcfermLUHS135 inhibited Staphylococcus aureus (DIZ 16.3 mm)]. Also, ClaGfermLUHS135 and Furcferm samples showed inhibition properties against both Bacillus cereus and Staphylococcus aureus strains (DIZ against Bacillus cereus 14.2 mm and 13.4 mm, respectively, and DIZ against Staphylococcus aureus 12.1 mm and 13.3 mm, respectively).
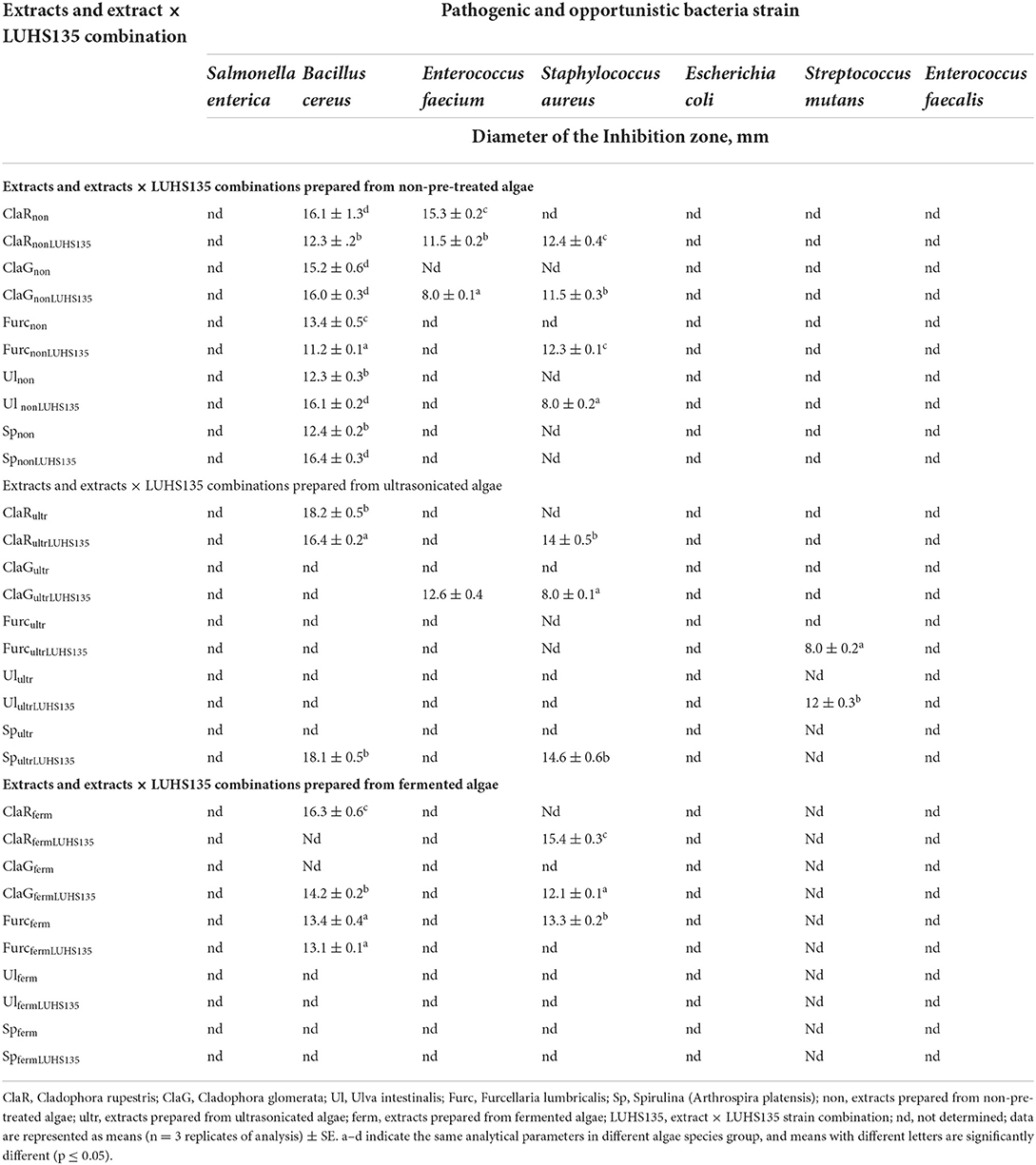
Table 4. Antimicrobial activity of the algae extracts and algae extracts × LUHS135 combinations evaluated using the agar well–diffusion method.
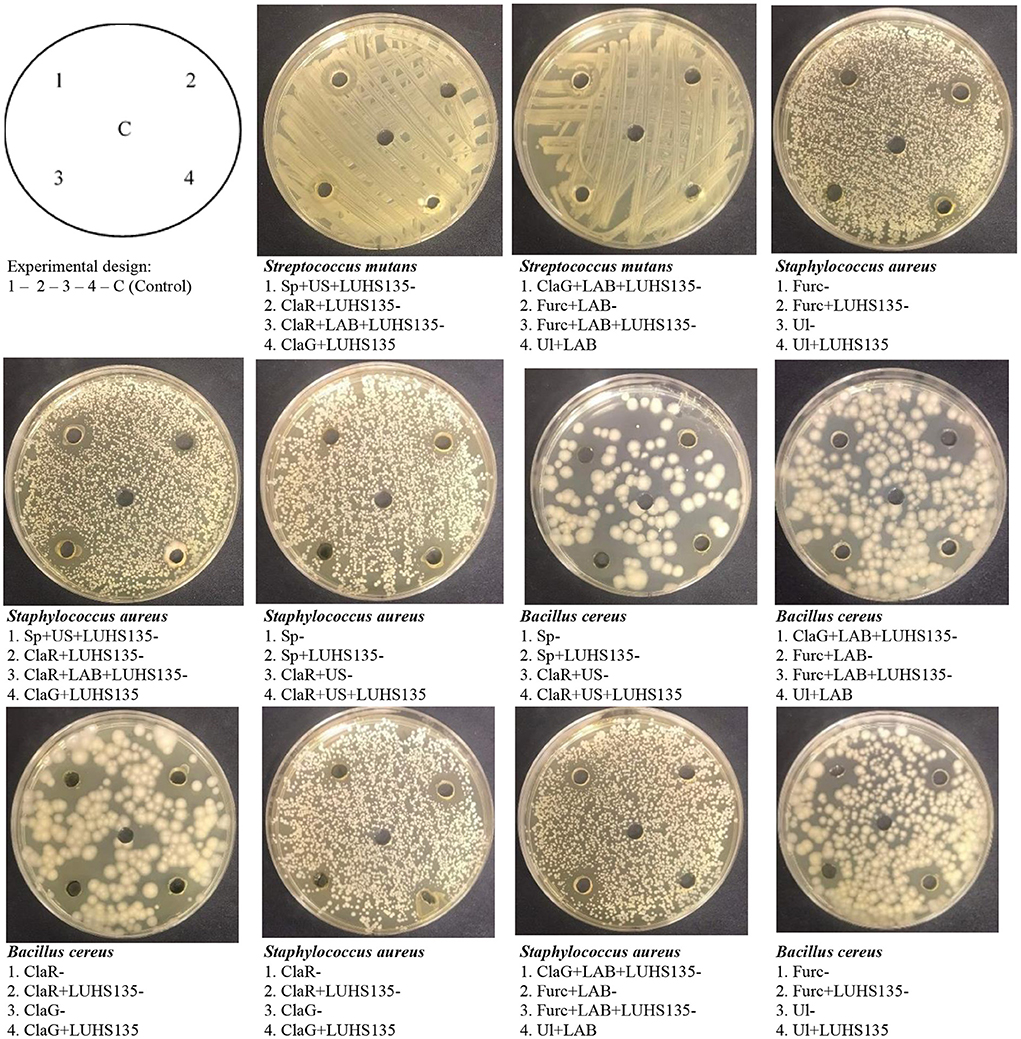
Figure 3. Images of the inhibition zones of the algae extracts and algae extracts × LUHS135 combinations evaluated using the agar well diffusion method [ClaR, Cladophora rupestris; ClaG, Cladophora glomerata; Ul, Ulva intestinalis; Furc, Furcellaria lumbricalis; Sp, Spirulina (Arthrospira platensis)]; US, algae biomass pre-treated with ultrasound; LAB, algae biomas fermented with LUHS135 strain before extraction; LUHS135, extract composition with LUHS135 strain; C,control (physiological solution)].
The results of antimicrobial activity of the algae extracts and algae extracts × LUHS135 combinations evaluated in liquid medium by testing concentrations of algae extract and/or the algae extract × LUHS135 combination in 500 and 2000 μL concentrations and pathogen concentration of 10 μL are shown in Tables 5, 6, respectively. We found that at a concentration of 500 μL in liquid medium, Spnon samples inhibited Bacillus cereus growth, ClaRferm samples inhibited Enterococcus faecium growth, SpultrLUHS135, ClaRferm and SpfermLUHS135 samples inhibited Staphylococcus aureus growth and Spnon samples inhibited Streptococcus mutans growth. By increasing algae extract and algae extracts × LUHS135 combinations concentrations to 2000 μL, in addition to the mentioned antimicrobial properties, Enterococcus faecium was also inhibited by ClaRnon, Spnon, SpnonLUHS135 and Ulultr samples, Streptococcus mutans was inhibited by ClaRultr, Ulultr and Spferm samples and Enterococcus faecalis was inhibited by ClaRnon, ClaGnon, Spnon and ClaGferm samples.
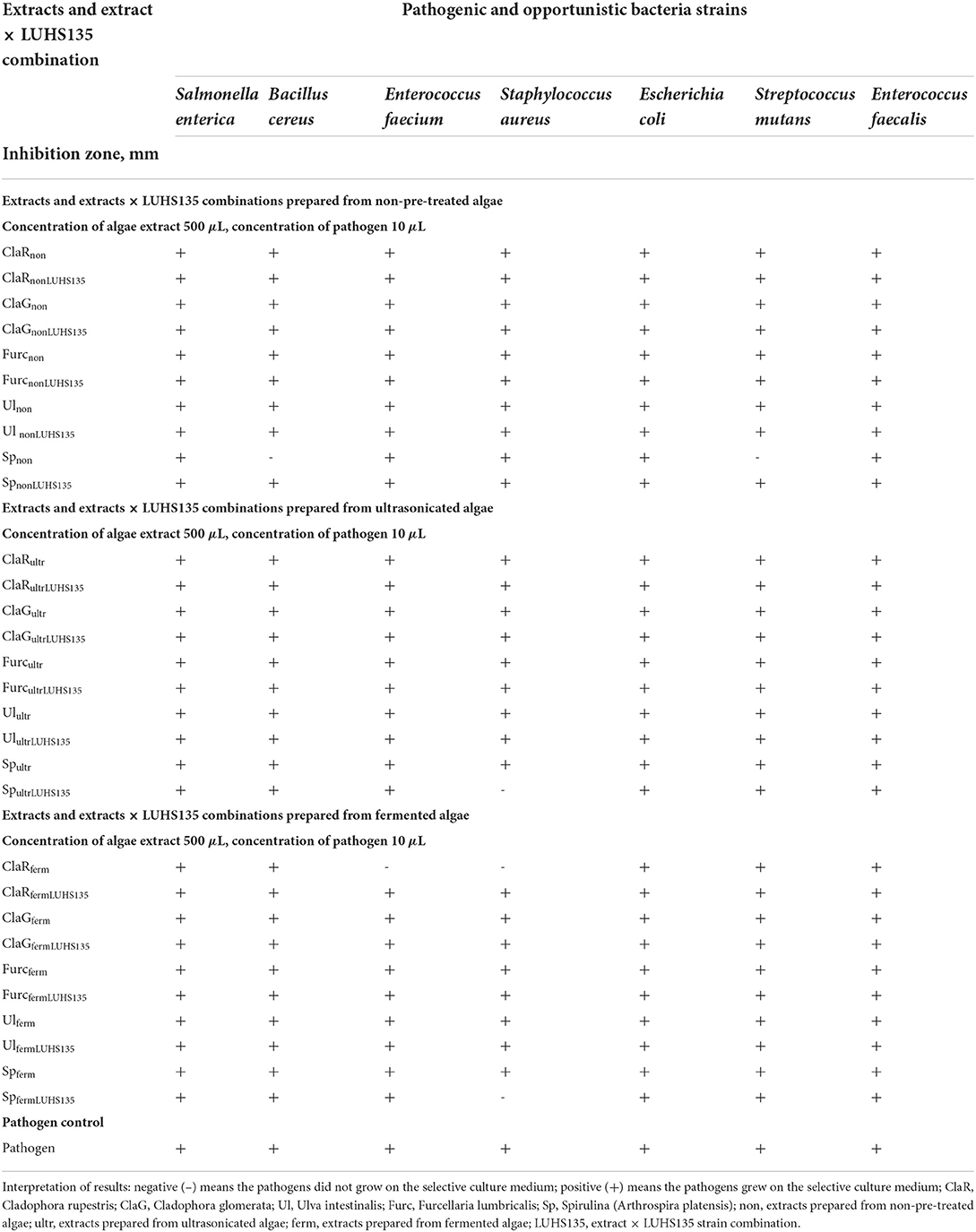
Table 5. Antimicrobial activity of the algae extracts and algae extracts × LUHS135 combinations evaluated in liquid medium by testing concentration of algae extract and/or algae extract × LUHS135 combination at a concentration of 500 μL and pathogen concentration at 10 μL.
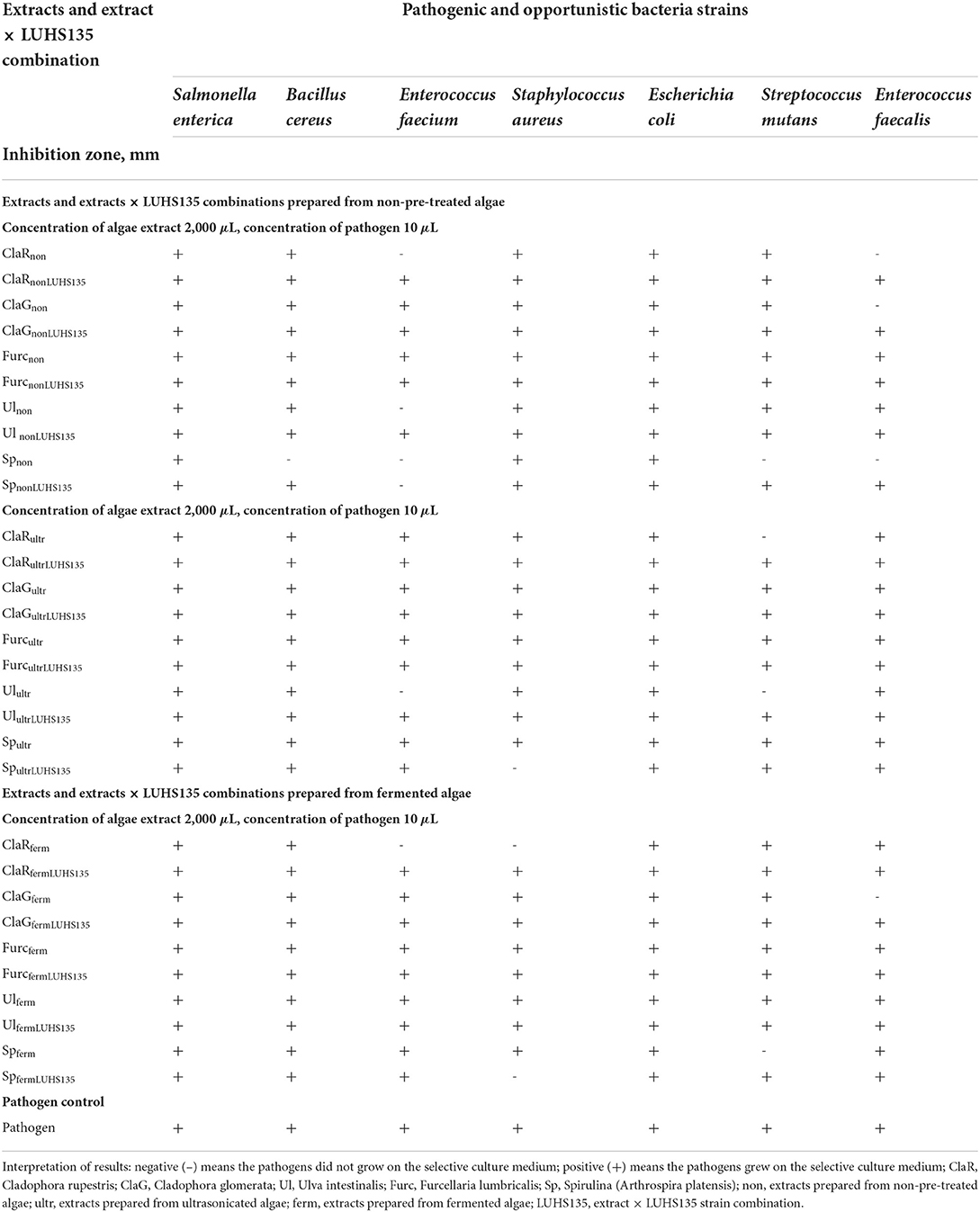
Table 6. Antimicrobial activity of the algae extracts and algae extracts × LUHS135 combinations evaluated in liquid medium by testing the concentration of algae extract and/or algae extract × LUHS135 combination at a concentration of 2,000 μL and pathogen concentration at 10 μL.
Algae are a good source of bioactive compounds, and some of them possess broad spectrum activities, including antimicrobial activities (3, 91, 92). Bacillus cereus is a facultative aerobic spore-forming bacterium (93, 94), and is a well-known foodborne pathogen that is able to grow in the intestinal tracts of insects and mammals (94). Ulva species inhibit the growth of some Gram-positive pathogens (Bacillus cereus and Staphylococcus aureus) at ≤ 500 μg/mL concentration (95). Gram-positive bacteria are more susceptible to algae extracts, in comparison with Gram-negative bacteria, which is explained by extracts' compositions (high concentration of phenolic compounds, terpenoids, alkaloids, etc.), which damage the cellular wall. In contrast, the external membrane of Gram-negative bacteria acts as a barrier, preventing any substance from passing through (96). Among the predominant human pathogens, Staphylococcus aureus is the foremost cause of gastroenteritis (94, 97). Cladophora rupestris inhibits S. aureus growth (with DIZ 16.3 mm) (98, 99). Also, ethanolic extracts of Cladophora sp. possess stronger antibacterial activity against S. aureus in comparison with Ulva sp. extracts (96). However, different compositions of extrahent can lead to different properties of the extracts (96). In red seaweeds, including F. lumbricalis, strong inhibition properties against S. aureus were also reported (99–102), and it is thought that red types of seaweed are very promising agents against S. aureus (99). Also, Gram-positive bacteria (B. cereus and S. aureus) showed higher sensitivity to Spirulina extracts in comparison with Gram-negative ones (103). Elshouny et al. (104) reported, that Spirulina possesses antimicrobial activity against not only S. aureus, but also inhibits E. coli and Salmonella spp. growth. Mohammed et al. (105) reported, that Gram-positive strains are more sensitive to Cladophora, Spirulina platensis and S. glomerata extracts than Gram-negative ones, and the highest inhibitory efficacy was found to be against S. aureus (105). Another pathogenic and opportunistic strain, E. faecium, is a significant opportunistic human pathogen with a broad host range (106). Enterococcus faecium causes big problems because of its broad resistance to antimicrobials (106). From this point of view, natural antimicrobials, which could be used for opportunistic pathogenic strain inhibition, become very important. Streptococcus mutans can cause dental decay (107, 108), and some S. mutans proteins contribute to the pathogenesis of S. mutans by promoting adherence to dental plaque (107, 109–112). Also, Sirbu et al. (113) reported that TPC in algae extracts is related with their antibacterial activity. In this study we established that there are moderate correlations between ABTS•+ and E. faecalis DIZ and between the TPC content in extracts and S. aureus DIZ (r = 0.388, p = 0.0001; r = 0.340, p = 0.001, respectively). However, further research is needed to evaluate which compounds are responsible for the inhibition of these pathogens.
Conclusions
This study confirmed, that the species of algae is significant factor on samples pH (p = 0.017) and 2% of yeast extract leads to more effective fermentation of algal biomass, as after 36 h of SSF, significant lower algae pH values were obtained. The highest DPPH•, ABTS•+, and FRAP antioxidant properties were shown by non-pretreated Cladophora rupestris and Furcellaria lumbricalis extract combinations with LUHS135, in comparison with extracts without LUHS135. A moderate positive correlation of TPC with samples ABTS•+ (r = 0.300, p = 0.004) and FRAP (r = 0.247, p = 0.019) was established, however, between samples DPPH• and TPC content correlations were not found. Despite, that in the non-pre-treated samples group the highest number of samples showed antimicrobial properties at least against one pathogen, a broader spectrum of pathogens inhibition showed ultrasonicated samples group (inhibited 4 out of 7 tested pathogens). Finally, despite, that the extract combinations with LUHS135 strain showed prospective results, further research is needed to evaluate, which compounds are responsible for antioxidant properties of the extracts, as well as pathogens inhibition.
Data availability statement
The raw data supporting the conclusions of this article will be made available by the authors, without undue reservation.
Author contributions
Conceptualization: EB, PV, and MR. Methodology: EB, PV, DU, and MR. Software, validation, writing—original draft, and preparation: ET and EB. Formal analysis: VS, EZ, DU, and RR. Investigation: EB, ET, and MR. Resources, supervision, and project administration: EB. Data curation: ET. Writing—review and editing: EB, PV, MR, RP, and JR. Visualization: ET, VS, and EZ. All authors have read and agreed to the published version of the manuscript.
Acknowledgments
The authors gratefully acknowledge the LSMU Science Foundation, support No. 12001120101/01030202. Also, this work is based upon the work from COST Action 18101 SOURDOMICS — Sourdough biotechnology network toward novel, healthier and sustainable food and bioprocesses (https://sourdomics.com/; https://www.cost.eu/actions/CA18101/, accessed in 2022-07-15), where the author JR is the Chair and Grant Holder Scientific Representative, and the author EB is the Vice-Chair, and is supported by COST (European Cooperation in Science and Technology) (https://www.cost.eu/, accessed in 2022-07-15). COST is a funding agency for research and innovation networks. Regarding to the author JR this work was also financially supported by LA/P/0045/2020 (ALiCE) and UIDB/00511/2020 - UIDP/00511/2020 (LEPABE) funded by national funds through FCT/MCTES (PIDDAC).
Conflict of interest
The authors declare that the research was conducted in the absence of any commercial or financial relationships that could be construed as a potential conflict of interest.
Publisher's note
All claims expressed in this article are solely those of the authors and do not necessarily represent those of their affiliated organizations, or those of the publisher, the editors and the reviewers. Any product that may be evaluated in this article, or claim that may be made by its manufacturer, is not guaranteed or endorsed by the publisher.
References
1. Oginni O, Wahlen B, Wendt L, Walton M, Dempster T, Gerken H. Effects of inoculation with lactic acid bacteria on the preservation of nannochloropsis gaditana biomass in wet anaerobic storage and its impact on biomass quality. Fermentation. (2022) 8:159. doi: 10.3390/fermentation8040159
2. Tolpeznikaite E, Ruzauskas M, Pilkaityte R, Bartkevics V, Zavistanaviciute P, Starkute V, et al. Influence of fermentation on the characteristics of baltic sea macroalgae, including microbial profile and trace element content. Food Control. (2021) 129:108235. doi: 10.1016/j.foodcont.2021.108235
3. Tolpeznikaite E, Bartkevics V, Ruzauskas M, Pilkaityte R, Viskelis P, Urbonaviciene D, et al. Characterization of Macro- and microalgae extracts bioactive compounds and micro- and macroelements transition from algae to extract. Foods. (2021) 10:2226. doi: 10.3390/foods10092226
4. Bartkiene E, Lele V, Ruzauskas M, Domig KJ, Starkute V, Zavistanaviciute P, et al. Lactic acid bacteria isolation from spontaneous sourdough and their characterization including antimicrobial and antifungal properties evaluation. Microorganisms. (2020) 8:64. doi: 10.3390/microorganisms8010064
5. Bartkiene E, Lele V, Sakiene V, Zavistanaviciute P, Ruzauskas M, Bernatoniene J, et al. Improvement of the antimicrobial activity of lactic acid bacteria in combination with berries/fruits and dairy industry by-products. J Sci Food Agri. (2019) 99:3992–4002. doi: 10.1002/jsfa.9625
6. Bartkiene E, Bartkevics V, Lele V, Pugajeva I, Zavistanaviciute P, Mickiene R, et al. A concept of mould spoilage prevention and acrylamide reduction in wheat bread: application of lactobacilli in combination with a cranberry coating. Food Control. (2018) 91:284–93. doi: 10.1016/j.foodcont.2018.04.019
7. Bartkiene E, Bartkevics V, Krungleviciute V, Pugajeva I, Zadeike D, Juodeikiene G. Lactic acid bacteria combinations for wheat sourdough preparation and their influence on wheat bread quality and acrylamide formation. J Food Sci. (2017) 82:2371–8. doi: 10.1111/1750-3841.13858
8. Yap BH, Crawford SA, Dagastine RR, Scales PJ, Martin GJ. Nitrogen deprivation of microalgae: effect on cell size, cell wall thickness, cell strength, and resistance to mechanical disruption. J Ind Microbiol Biotechnol. (2016) 43:1671–80. doi: 10.1007/s10295-016-1848-1
9. Rokicka M, Zieliński M, Dudek M, et al. Effects of ultrasonic and microwave pretreatment on lipid extraction of microalgae and methane production from the residual extracted biomass. Bioenerg Res. (2021) 14:752–60. doi: 10.1007/s12155-020-10202-y
10. Tavanandi HA, Chandralekha Devi AA, Raghavarao KSMS. Sustainable pre-treatment methods for downstream processing of harvested microalgae. In: Gayen K, Bhowmick TK, Maity SK (eds) Sustainable Downstream Processing of Microalgae for Industrial Application. 1st edn. Florida: Taylor & Francis Group. (2019). p. 1–28.
11. Montingelli ME, Tedesco S. Olabi, AG. Biogas production from algal biomass: a review. Renew Sust Energ Rev. (2015) 43:961–72. doi: 10.1016/j.rser.2014.11.052
12. Terrell M. Thompson, Brent R, Young, Saeid Baroutian. Advances in the pretreatment of brown macroalgae for biogas production. Fuel Process Technol. (2019) 195:106151. doi: 10.1016/j.fuproc.2019.106151
13. Borowitzka MA, Moheimani NR. Sustainable biofuels from algae. Mitig Adapt Strateg Glob Change. (2013) 18:13–25. doi: 10.1007/s11027-010-9271-9
14. Rodriguez C, Alaswad A, Benyounis K, Olabi A. Pretreatment techniques used in biogas production from grass, Renew. Sust Energ Rev. (2017) 68:1193–204. doi: 10.1016/j.rser.2016.02.022
15. Martinez-Guerra E, Gude VG, Mondala A, Holmes W, Hernandez R. Microwave and ultrasound enhanced extractive transesterification of algal lipids. Appl Energy. (2014) 129:354–63. doi: 10.1016/j.apenergy.2014.04.112
16. Ma YA, Cheng YM, Huang JW, Jen JF, Huang YS Yu CC. Effects of ultrasonic and microwave pretreatments on lipid extraction of microalgae. Bioprocess Biosyst Eng. (2014) 37:1543–9. doi: 10.1007/s00449-014-1126-4
17. Adam F, Abert-Vian M, Peltier G, Chemat F. “Solvent-free” ultrasound-assisted extraction of lipids from fresh microalgae cells: a green, clean and scalable process. Bioresour Technol. (2012) 114:457–65. doi: 10.1016/j.biortech.2012.02.096
18. Hossain M. Zabed, Suely Akter, Junhua Yun, Guoyan Zhang, Faisal N. Awad, Xianghui Qi, JN Sahu Recent advances in biological pretreatment of microalgae and lignocellulosic biomass for biofuel production Renew. Sustain Energy Rev. (2019) 105:105–28. doi: 10.1016/j.rser.2019.01.048
19. da Silva Machado A, Ferraz A. Biological pretreatment of sugarcane bagasse with basidiomycetes producing varied patterns of biodegradation. Bioresour Technol. (2017) 225:17–22. doi: 10.1016/j.biortech.2016.11.053
20. Carrillo-Reyes J, Barragán-Trinidad M, Buitrón G. Biological pretreatments of microalgal biomass for gaseous biofuel production and the potential use of rumen microorganisms: a review. Algal Res. (2016) 18:341–51. doi: 10.1016/j.algal.2016.07.004
21. Zhao X, Luo K, Zhang Y, Zheng Z, Cai Y, Wen B, et al. Improving the methane yield of maize straw: focus on the effects of pretreatment with fungi and their secreted enzymes combined with sodium hydroxide. Bioresour Technol. (2018) 250:204–13. doi: 10.1016/j.biortech.2017.09.160
22. Arora R, Sharma NK, Kumar S. Valorization of by-products following the biorefinery concept: commercial aspects of by-products of lignocellulosic biomass. In: Chandel AK, Luciano Silveira MH, editors. Advances in Sugarcane Biorefinery. Amsterdam: Elsevier. (2018) 163–78. doi: 10.1016/B978-0-12-804534-3.00008-2
23. Sindhu R, Binod P, Pandey A. Biological pretreatment of lignocellulosic biomass–an overview. Bioresour Technol. (2016) 199:76–82. doi: 10.1016/j.biortech.2015.08.030
24. Millati R, Syamsiah S, Niklasson C, Cahyanto MN, Ludquist K, Taherzadeh MJ. Biological pretreatment of lignocelluloses with white-rot fungi and its applications: a review. BioResources. (2011) 6:5224–59. doi: 10.15376/biores.6.4.Isroi
25. Wahlen BD, Wendt LM, Murphy JA, Seibel F. Mitigation of variable seasonal productivity in algae biomass through blending and ensiling: an assessment of compositional changes in storage. Algal Res. (2019) 42:101584. doi: 10.1016/j.algal.2019.101584
26. Solis-Pereira S, Favela-Torres E, Viniegra-Gonzfilez G, Gutirrez-Rojas M. Effects of different carbon sources on the synthesis of pectinase by Aspergillus niger in submerged and solid state fermentations. Microbiol Biotechnol. (1993) 39:36–41. doi: 10.1007/BF00166845
27. Bartkiene E, Zokaityte E, Lele V, Starkute V, Zavistanaviciute P, Klupsaite D, et al. Combination of extrusion and fermentation with lactobacillus plantarum and L. uvarum strains for improving the safety characteristics of wheat bran. Toxins. (2021) 13:163. doi: 10.3390/toxins13020163
28. Vadopalas L, Ruzauskas M, Lele V, Starkute V, Zavistanaviciute P, Zokaityte E, et al. Pigs' feed fermentation model with antimicrobial lactic acid bacteria strains combination by changing extruded soya to biomodified local feed stock. Animals. (2020) 10:783. doi: 10.3390/ani10050783
29. Vadopalas L, Badaras S, Ruzauskas M, Lele V, Starkute V, Zavistanaviciute P, et al. Influence of the fermented feed and vaccination and their interaction on parameters of large white/norwegian landrace piglets. Animals. (2020) 10:1201. doi: 10.3390/ani10071201
30. Cagalj M, Skroza D, Tabanelli G, Özogul F, Šimat V. Maximizing the antioxidant capacity of padina pavonica by choosing the right drying and extraction methods. Processes. (2021) 9:587. doi: 10.3390/pr9040587
31. Slinkard K, Singleton VL. Total phenol analysis: automation and comparison with manual methods. Am J Enol Viticul. (1977) 1977:49–55.
32. Urbonavičiene D, Bobinas C, Bobinaite R, Raudone L, Trumbeckaite S, Viškelis J, et al. Composition and antioxidant activity, supercritical carbon dioxide extraction extracts, and residue after extraction of biologically active compounds from freeze-dried tomato matrix. Processes. (2021) 9:467. doi: 10.3390/pr9030467
33. Brand-Williams W, Cuvelier ME, Berset C. Use of a free radical method to evaluate antioxidant activity. LWT- Food Sci Technol. (1995) 28:25–30. doi: 10.1016/S0023-6438(95)80008-5
34. Urbonaviciene D, Bobinaite R, Viskelis P, Bobinas C, Petruskevicius A, Klavins L. Viskelis J. Geographic variability of biologically active compounds, antioxidant activity and physico-chemical properties in wild bilberries (Vaccinium myrtillus L). Antioxidants. (2022) 11:588. doi: 10.3390/antiox11030588
35. Re R, Pellegrini N, Proteggente A, Pannala A, Yang M, Rice-Evans C. Antioxidant activity applying an improved ABTS radical cation decolorization assay. Free Radic Biol Med. (1999) 26:1231–7. doi: 10.1016/S0891-5849(98)00315-3
36. Benzie IF, Strain JJ. The ferric reducing ability of plasma (FRAP) as a measure of “antioxidant power”: the FRAP Assay. Anal Biochem. (1996) 239:70–6. doi: 10.1006/abio.1996.0292
37. Bobinaite R, Pataro G, Lamanauskas N, Šatkauskas S, Viškelis P, Ferrari G. Application of pulsed electric field in the production of juice and extraction of bioactive compounds from blueberry fruits and their by-products. J Food Sci Technol. (2015) 52:5898–905. doi: 10.1007/s13197-014-1668-0
38. Gunasekaran YK, Lele V, Sakiene V, Zavistanaviciute P, Zokaityte E, Klupsaite D, et al. Plant-based proteinaceous snacks: Effect of fermentation and ultrasonication on end-product characteristics. Food Sci Nutr. (2020) 8:4746–56. doi: 10.1002/fsn3.1705
39. Liu X, Narbad A. Lactic acid bacteria-based food fermentations. In: W. Chen, & A. Narbad (Eds.), Lactic Acid Bacteria in Foodborne Hazards Reduction. Singapore City Singapore: Springer (2018). p. 141–181.
40. Rodríguez LGR, Gasga VMZ, Pescuma M, Van Nieuwenhove C, Mozzi F, Burgos JAS. Fruits and fruit by-products as sources of bioactive compounds. Benefits and trends of lactic acid fermentation in the development of novel fruit-based functional beverages. Food Res Inter. (2021) 140:109854. doi: 10.1016/j.foodres.2020.109854
41. Dey TB, Chakraborty S, Jain KK, Sharma A, Kuhad RC. Antioxidant phenolics and their microbial production by submerged and solid state fermentation process: a review. Trends Food Sci Technol. (2016) 53:60–74. doi: 10.1016/j.tifs.2016.04.007
42. Adebo OA, Medina-Meza IG. Impact of fermentation on the phenolic compounds and antioxidant activity of whole cereal grains: a mini review. Molecules. (2020) 25:927. doi: 10.3390/molecules25040927
43. Othman NB, Roblain D, Chammen N, Thonart P, Hamdi M. Antioxidant phenolic compounds loss during the fermentation of Ch'etoui olives. Food Chem. (2009) 116:662–9. doi: 10.1016/j.foodchem.2009.02.084
44. Nowacka M, Wiktor A, Sledz M, Jurek N, Witrowa-Rajchert D. Drying of ultrasound pretreated apple and its selected physical properties. J Food Eng. (2012) 113:427–33. doi: 10.1016/j.jfoodeng.2012.06.013
45. Nowacka M, Wedzik M. Effect of ultrasound treatment on microstructure, colour and carotenoid content in fresh and dried carrot tissue. Applied Acoustics. (2016) 103:163–71. doi: 10.1016/j.apacoust.2015.06.011
46. Witrowa-Rajchert D, Wiktor A, Sledz M, Nowacka M. Selected emerging technologies to enhance the drying process: a review. Drying Technol. (2014) 32:1386–96. doi: 10.1080/07373937.2014.903412
47. Bermudez-Aguirre D, Mobbs T, Barbosa-Canovas GV. Ultrasound applications in food processing. In: Feng H, Barbosa-Canovas GV, Weiss J, editors. Ultrasound Technologies for Food and Bioprocessing Food Engineering Series. New York: Springer (2011). p. 65–105. doi: 10.1007/978-1-4419-7472-3_3
48. Kutyla-Olesiuk A, Nowacka M, Wesoły M, Ciosek P. Evaluation of organoleptic and texture properties of dried apples by hybrid electronic tongue. Sens Actuators B: Chem. (2013) 187:234–40. doi: 10.1016/j.snb.2012.10.133
49. Liu J, Li J, Tang J. Ultrasonically assisted extraction of total carbohydrates from Stevia rebaudiana Bertoni and identification of extracts. Food Bioprod Proc. (2010) 88:215–21. doi: 10.1016/j.fbp.2009.12.005
50. Zhao G, Chen X, Wang L, Zhou S, Feng H, Chen WN, et al. Ultrasound assisted extraction of carbohydrates from microalgae as feedstock for yeast fermentation. Biores Technol. (2013) 128:337–44. doi: 10.1016/j.biortech.2012.10.038
51. Abid M, Jabbar S, Wu T, Hashim MM, Hu B, Lei S, et al. Sonication enhances polyphenolic compounds, sugars, carotenoids and mineral elements of apple juice. Ultrason Sonochem. (2014) 21:93–7. doi: 10.1016/j.ultsonch.2013.06.002
52. Demarco M, de Moraes JO, Matos ÂP, Derner RB, de Farias Neves F, Tribuzi G. Digestibility, bioaccessibility and bioactivity of compounds from algae. Trends Food Sci Technol. (2022) 7. doi: 10.1016/j.tifs.2022.02.004
53. Takaichi S. Carotenoids in algae: distributions, biosyntheses and functions. Mar Drugs. (2011) 9:1101–18. doi: 10.3390/md9061101
54. Gupta AK, Seth K, Maheshwari K, Baroliya PK, Meena M, Kumar A, et al. Biosynthesis and extraction of high-value carotenoid from algae. Front Biosci. (2021) 26:171–90. doi: 10.52586/4932
55. Rao AR, Baskaran V, Sarada R, Ravishankar GA. In vivo bioavailability and antioxidant activity of carotenoids from microalgal biomass—a repeated dose study. Food Res Inter. (2013) 54:711–7. doi: 10.1016/j.foodres.2013.07.067
56. Gonzalez-Vega RI, Cardenas-Lopez JL, Lopez-Elias JA, Ruiz-Cruz S, Reyes-Diaz A, et al. Optimization of growing conditions for pigments production from microalga Navicula incerta using response surface methodology and its antioxidant capacity. Saudi J Biol Sci. (2021) 28:1401–16. doi: 10.1016/j.sjbs.2020.11.076
57. Ana J, Gao F, Ma Q, Xiang Y, Ren D, Lu J. Screening for enhanced astaxanthin accumulation among Spirulina platensis mutants generated by atmospheric and room temperature plasmas. Algal Res. (2017) 25:464–72. doi: 10.1016/j.algal.2017.06.006
58. Mularczyk M, Michalak I, Marycz K. Astaxanthin and other nutrients from haematococcus pluvialis-multifunctional applications. Mar Drugs. (2020) 18:459. doi: 10.3390/md18090459
59. Patel AK, Albarico FP, Perumal PK, Vadrale AP, Ntan CT, Chau HT, et al. Algae as an emerging source of bioactive pigments. Biores Technol. (2022) 26:126910. doi: 10.1016/j.biortech.2022.126910
60. Rebelo BA, Farrona S, Ventura MR, Abranches R. Canthaxanthin, a red-hot carotenoid: applications, synthesis, and biosynthetic evolution. Plants. (2020) 9:1039. doi: 10.3390/plants9081039
61. Fawzy S, Wang W, Wu M, Yi G, Huang X. Effects of dietary different canthaxanthin levels on growth performance, antioxidant capacity, biochemical and immune-physiological parameters of white shrimp (Litopenaeus Vannamei). Aquaculture. (2022) 21:738276. doi: 10.1016/j.aquaculture.2022.738276
62. Elia AC, Prearo M, Dorr AJM, Pacini N, Magara G, Brizio P, et al. Effects of astaxanthin and canthaxanthin on oxidative stress biomarkers in rainbow trout. J Toxicol Environ Health Part A. (2019) 82:760–8. doi: 10.1080/15287394.2019.1648346
63. Shahidi F, Brown JA. Carotenoid pigments in seafoods and aquaculture. Crit Rev Food Sci Nutr. (1998) 38:1–67. doi: 10.1080/10408699891274165
64. Venugopalan V, Tripathi SK, Nahar P, Saradhi PP, Das RH, Gautam HK. Characterization of canthaxanthin isomers isolated from a new soil Dietzia sp. and their antioxidant activities. J Microbiol Biotechnol. (2013) 23:237–45. doi: 10.4014/jmb.1203.03032
65. Eriksen NT. Production of phycocyanin—a pigment with applications in biology, biotechnology, foods and medicine. Appl Microbiol Biotechnol. (2008) 80:1–14. doi: 10.1007/s00253-008-1542-y
66. Lou S, Lin X, Liu C, Anwar M, Li H, Hu Z. Molecular cloning and functional characterization of CvLCYE, a key enzyme in lutein synthesis pathway in Chlorella vulgaris. Algal Res. (2021) 55:102246. doi: 10.1016/j.algal.2021.102246
67. Wang F, Huang L, Gao B, Zhang C. Optimum production conditions, purification, identification, and antioxidant activity of violaxanthin from microalga Eustigmatos cf. polyphem (Eustigmatophyceae) Mar Drugs. (2018) 16:190. doi: 10.3390/md16060190
68. Wang N, Manabe Y, Sugawara T, Paul NA, Zhao J. Identification and biological activities of carotenoids from the freshwater alga Oedogonium intermedium. Food Chem. (2018) 242:247–55. doi: 10.1016/j.foodchem.2017.09.075
69. Li Y, Li S, Lin SJ, Zhang JJ, Zhao CN Li HB. Microwave-assisted extraction of natural antioxidants from the exotic Gordonia axillaris fruit: optimization and identification of phenolic compounds. Molecule. (2017) 22:1481. doi: 10.3390/molecules22091481
70. Gao Y-Y, Ji J, Jin L, Sun B-L, Xu L-H, Wang C-K, et al. Xanthophyll supplementation regulates carotenoid and retinoid metabolism in hens and chicks. Poul Sci. (2016) 95:541–9. doi: 10.3382/ps/pev335
71. Sompong D, Trakanrungroj P. The flower of Radermacheraignea (kurz) steenis, a new source of zeaxanthin. Suranaree J Sci Technol. (2010) 17:303–8. doi.org/10.1159/000504364
72. Barker FM. Snodderly DM, Johnson EJ, Schalch W, Koepcke W, Gerss J, et al. Nutritional manipulation of primate retinas, V: effects of lutein, zeaxanthin, and n −3 fatty acids on retinal sensitivity to blue-light–induced damage. Investig Opthalmol Vis Sci. (2011) 52:3934. doi: 10.1167/iovs.10-5898
73. Bian Q, Gao S, Zhou J, Qin J, Taylor A, Johnson EJ, et al. Lutein and zeaxanthin supplementation reduces photooxidative damage and modulates the expression of inflammation-related genes in retinal pigment epithelial cells. Free Radical Biol Med. (2012) 53:1298–307. doi: 10.1016/j.freeradbiomed.2012.06.024
74. Bouyahya A, El Omari N, Hakkur M, El Hachlafi N, Charfi S, Balahbib A, et al. Sources, health benefits, and biological properties of zeaxanthin. Trends Food Sci Technol. (2021) 118:519–38. doi: 10.1016/j.tifs.2021.10.017
75. Sahin K, Akdemir F, Orhan C, Tuzcu M, Gencoglu H, Sahin N, et al. (3R, 3'R)-zeaxanthin protects the retina from photo-oxidative damage via modulating the inflammation and visual health molecular markers. Cutan Ocular Toxicol. (2019) 38:161–8. doi: 10.1080/15569527.2018.1554667
76. Yarnpakdee S, Senphan T, Wangtueai S, Jaisan C, Nalinanon S. Characteristic and antioxidant activity of Cladophora glomerata ethanolic extract as affected by prior chlorophyll removal and drying methods. J Food Process Preserv. (2021) 24:e15534. doi: 10.1111/jfpp.15534
77. Chandrasekara A, Shahidi F. Inhibitory activities of soluble and bound millet seed phenolics on free radicals and reactive oxygen species. J Agricul Food Chemistry. (2011) 59:428–36. doi: 10.1021/jf103896z
78. Yarnpakdee S, Benjakul S, Senphan T. Antioxidant activity of the extracts from freshwater macroalgae (Cladophora glomerata) grown in Northern Thailand and its preventive effect against lipid oxidation of refrigerated eastern little tuna slice. Turkish J Fisher Aqua Sci. (2019) 19:209–19. doi: 10.4194/1303-2712-v19_3_04
79. Gaber NB, El-Dahy SI, Shalaby EA. Comparison of ABTS, DPPH, permanganate, and methylene blue assays for determining antioxidant potential of successive extracts from pomegranate and guava residues. Biomass Conv Bioref . (2021) 1–10. doi: 10.1007/s13399-021-01386-0
80. Shalaby EA, Shanab SM. Antioxidant compounds, assays of determination and mode of action. Af J Pharm Pharmacol. (2013) 7:528–39. doi: 10.5897/AJPP2013.3474
81. Shahidi F, Zhong Y. Novel antioxidants in food quality preservation and health promotion. Eur J Lipid Sci Technol. (2010) 112:930–40. doi: 10.1002/ejlt.201000044
82. Shahidi F, Ambigaipalan P. Phenolics and polyphenolics in foods, beverages and spices: antioxidant activity and health effects—a review. J Funct Foods. (2015) 18:820–97. doi: 10.1016/j.jff.2015.06.018
83. Mole Megha N, Anjali SB. Antioxidant potential of seaweeds from Kunakeshwar along the West coast Maharashtra. Asian J Biomed Pharm Sci. (2013) 3:45–50.
84. Melini F, Melini V. Impact of Fermentation on Phenolic Compounds and Antioxidant Capacity of Quinoa. Fermentation. (2021) 7:20. doi: 10.3390/fermentation7010020
85. Hur SJ, Lee SY, Kim YC, Choi I, Kim GB. Effect of fermentation on the antioxidant activity in plant-based foods. Food Chem. (2014) 160:346–56. doi: 10.1016/j.foodchem.2014.03.112
86. Li T, Jiang T, Liu N, Wu C, Xu H, Lei H. Biotransformation of phenolic profiles and improvement of antioxidant capacities in jujube juice by select lactic acid bacteria. Food Chem. (2021) 339:127859. doi: 10.1016/j.foodchem.2020.127859
87. Kulikova Y, Sukhikh S, Kalashnikova O, Chupakhin E, Ivanova S, Chubarenko B, et al. Assessment of the resource potential of baltic sea macroalgae. Appl Sci. (2022) 12:3599. doi: 10.3390/app12073599
88. Devi KP, Suganthy N, Kesika P, Pandian SK. Bioprotective properties of seaweeds: in vitro evaluation of antioxidant activity and antimicrobial activity against food borne bacteria in relation to polyphenolic content. BMC Complement Altern Med. (2008) 8:38–49. doi: 10.1186/1472-6882-8-38
89. Wang T, Jonsdottir R, Olafsdottir G. Total phenolic compounds, radical scavenging and metal chelation of extracts from Icelandic seaweeds. Food Chem. (2009) 116:240–8. doi: 10.1016/j.foodchem.2009.02.041
90. Zubia M, Fabre MS, Kerjean V, Lann KL, Stigerpoureau V, Fauchon M, et al. Antioxidant and antitumoural activities of some Phaeophyta from Brittany coasts. Food Chem. (2009) 116:693–701. doi: 10.1016/j.foodchem.2009.03.025
91. Saim S, Sahnouni F, Bouhadi D, Kharbouche S. Antimicrobial activity of two marine red algae collected from Algerian West Coast. Trends Pharmacet Sci. (2021) 7:233–42. doi: 10.30476/tips.2021.89827.1078
92. Domettila C, Brintha TSS, Sukumaran S, Jeeva S. Diversity and distribution of seaweeds in the Muttom coastal waters, south-west coast of India. Biodivers J. (2013) 4:105–10.
93. Chorin E, Thuault D, Cléret J-J. Bourgeois, C.-M. Modelling Bacillus cereus growth. Int J Food Microbiol. (1997) 38:229–34. doi: 10.1016/S0168-1605(97)00110-4
94. Silva A, Rodrigues C, Garcia-Oliveira P, Lourenço-Lopes C, Silva SA, Garcia-Perez P, et al. J. Screening of bioactive properties in brown algae from the Northwest Iberian Peninsula. Foods. (2021) 10:1915. doi: 10.3390/foods10081915
95. Dussault D, Vu KD, Vansach T, Horgen FD, Lacroix M. Antimicrobial effects of marine algal extracts and cyanobacterial pure compounds against five foodborne pathogens. Food Chem. (2016) 199:114–8. doi: 10.1016/j.foodchem.2015.11.119
96. Muñoz RA, Santome S, León JQ. Antibacterial activity of hexane and ethanolic extracts of marine macroalgae of the Bay of Ancón, Lima – Peru. Rev Investig Vet del Peru. (2020) 31, 1–14. doi: 10.15381/rivep.v31i2.17829
97. Loir YL, Baron F, Gautier M. Staphylococcus aureus and food poisoning. Genet Mol Res. (2003) 7–28. doi.org/10.1007/978-3-540-29676-8
98. Krish S, Das A. In-vitro bioactivity of marine seaweed, Cladophora rupestris. Inter J Pharmacy Biol Sci. (2014) 5:898–908.
99. Zamimi NN, Halim NA, Mustafa MM, Darnis DS, Musa MF, Yusof F. Antimicrobial effect of seaweeds against oral-borne pathogens: a review. Intern J All Health Sci. (2020) 4:1049–56.
100. Bouhlal R, Riadi H, Martínez J. The antibacterial potential of the seaweeds (Rhodophyceae) of the strait of gibraltar and the mediterranean coast of Morocco. Af J Biotechnol. (2010) 9:6365–72. doi: 10.5897/AJB09.2023
101. Muñoz-Ochoa M, Murillo-Álvarez JI, ZermeñoCervantes LA, Martínez-Díaz S. Rodríguez-Riosmena, R. Screening of extracts of algae from Baja California Sur, Mexico as reversers of the antibiotic resistance of some pathogenic bacteria. Eur Rev Med Pharmacoll Sci. (2010) 14:739–74.
102. Ravikumar S, Anburajan L, Ramanathan G. Screening of seaweed extracts against antibiotic resistant post operative infectious pathogens. Seaweed Res Util. (2002) 24:95–9.
103. Abdel-Moneim AM, El-Saadony MT, Shehata AM, Saad AM, Aldhumri SA, Ouda SM, et al. Antioxidant and antimicrobial activities of Spirulina platensis extracts and biogenic selenium nanoparticles against selected pathogenic bacteria and fungi. Saudi J Biol Sci. (2022) 29:1197–209. doi: 10.1016/j.sjbs.2021.09.046
104. Elshouny WAE.-F, El-Sheekh MM, Sabae SZ, Khalil MA, Badr HM. Antimicrobial activity of Spirulina platensis against aquatic bacterial isolates. J Microbiol Biotechnol Food Sci. (2021) 1203–8. doi.org/10.15414/jmbfs.2017.6.5.1203-1208
105. Mohammed ZA, Jawad HM, Reda NM. The effect of algal extract of spirulina platensis, cladophora glomerata on some positive and negative bacterial isolates of gram stain. NeuroQuantology. (2021) 19:67. doi: 10.14704/nq.2021.19.6.NQ21070
106. Freitas AR, Pereira AP, Novais C. Peixe L. Multidrug-resistant high-risk Enterococcus faecium clones: can we really define them? Intern J Antimicrob Agents. (2021) 57:106227. doi: 10.1016/j.ijantimicag.2020.106227
107. Elyassi M, Babaeekhou L, Ghane M. Streptococcus mutans and Streptococcus sobrinus contributions in dental caries in Iranian and Afghan children: a report from serotype distribution and novel STs. Arch Oral Biol. (2022) 139:105431. doi: 10.1016/j.archoralbio.2022.105431
108. Krzyściak W, Jurczak A. Ko'scielniak D, Bystrowska B, Skalniak A. The virulence of Streptococcus mutans and the ability to form biofilms. Eur J Clin Microbiol Infect Dis. (2014) 33:499–515. doi: 10.1007/s10096-013-1993-7
109. Sato Y, Okamoto K, Kagami A, Yamamoto Y, Igarashi T, Kizaki H. Streptococcus mutans strains harboring collagen-binding adhesin. J Dent Res. (2004) 83:534–9. doi: 10.1177/154405910408300705
110. Matsumoto-Nakano M. Role of Streptococcus mutans surface proteins for biofilm formation. Jap Dent Sci Rev. (2018) 54:22–9. doi: 10.1016/j.jdsr.2017.08.002
111. Babaei Hatkehlouei M, Tari H, Goudarzian AH, Hali H. Decayed, missing, and filled teeth (DMFT) index among first-grade elementary students in Mazandaran Province, Northern Iran. Intern J Pediatr. (2017) 5:5069–77. doi.org/10.22038/ijp.2017.22650.1891
112. Nakano K, Nomura R, Taniguchi N, Lapirattanakul J, Kojima A, Naka S, et al. Molecular characterization of Streptococcus mutans strains containing the cnm gene encoding a collagen-binding adhesin. Arch Oral Biol. (2010) 55:34–9. doi: 10.1016/j.archoralbio.2009.11.008
Keywords: solid-state fermentation, ultrasonication, algae, extracts, antimicrobial properties, antioxidant properties, lactic acid bacteria
Citation: Tolpeznikaite E, Starkute V, Zokaityte E, Ruzauskas M, Pilkaityte R, Viskelis P, Urbonaviciene D, Ruibys R, Rocha JM and Bartkiene E (2022) Effect of solid-state fermentation and ultrasonication processes on antimicrobial and antioxidant properties of algae extracts. Front. Nutr. 9:990274. doi: 10.3389/fnut.2022.990274
Received: 09 July 2022; Accepted: 12 August 2022;
Published: 26 August 2022.
Edited by:
Oana Lelia Pop, University of Agricultural Sciences and Veterinary Medicine of Cluj-Napoca, RomaniaReviewed by:
Pavan Kumar, Guru Angad Dev Veterinary and Animal Sciences University, IndiaAhmed A. Zaky, National Research Centre, Egypt
Copyright © 2022 Tolpeznikaite, Starkute, Zokaityte, Ruzauskas, Pilkaityte, Viskelis, Urbonaviciene, Ruibys, Rocha and Bartkiene. This is an open-access article distributed under the terms of the Creative Commons Attribution License (CC BY). The use, distribution or reproduction in other forums is permitted, provided the original author(s) and the copyright owner(s) are credited and that the original publication in this journal is cited, in accordance with accepted academic practice. No use, distribution or reproduction is permitted which does not comply with these terms.
*Correspondence: Elena Bartkiene, elena.bartkiene@lsmuni.lt