- 1Laboratory of Research in Food Science, Universidad Catolica de Santa Maria, Arequipa, Perú
- 2Estación Experimental Agraria Arequipa, Instituto Nacional de Innovación Agraria (INIA), Arequipa, Perú
- 3Laboratorio de Fisiologia Molecular de Plantas, PIPS de Cereales y Granos Nativos, Facultad de Agronomia, Universidad Nacional Agraria La Molina, Lima, Perú
- 4Escuela de Alimentos, Facultad de Ciencias Agronómicas y de los Alimentos, Pontificia Universidad Católica de Valparaíso, Valparaíso, Chile
- 5Department of Plant Sciences, North Dakota State University, Fargo, ND, United States
- 6Escuela Profesional de Ingeniería de Industria Alimentaria, Facultad de Ciencias e Ingenierías Biológicas y Químicas, Universidad Catolica de Santa Maria, Arequipa, Perú
The high diversity of the Peruvian Andean maize (Zea mays L.) represents a biological and genetic heritage relevant for food security, but few studies are targeted toward its characterization and consequent valorization and preservation. The objective of this study was to evaluate the potential of the Peruvian Andean maize race Cabanita with respect to its bioactive profiles (free and bound phenolic and carotenoid composition), physical characteristics, and in vitro antioxidant properties. Maize landraces with variable kernel pigmentation were collected from two provinces (Caylloma and Castilla) within the Arequipa region (among ten Andean sites) and the phytochemical profile was evaluated by Ultra High-Performance Liquid Chromatography with diode array detector (UHPLC-DAD). All maize samples were important sources of phenolic compounds mainly soluble p-coumaric and ferulic acid derivatives whereas anthocyanins were only detected in maize with partially red pigmented kernels. Major phenolic compounds in the bound phenolic fractions were ferulic acid and its derivatives along with p-coumaric acid. Carotenoid compounds including xanthophylls such as lutein, lutein isomers, and zeaxanthin were only detected in orange and white-yellow pigmented maize and are reported for the first time in Peruvian landraces. The multivariate analysis using Principal Components Analysis (PCA) revealed low variability of all data which may indicate a level of similarity among maize samples based on evaluated variables. However, maize grown in Caylloma province showed more homogeneous physical characteristics and higher yield, whereas higher phenolic contents and antioxidant capacity were observed in maize from Castilla. Samples CAY (yellow-pigmented kernel, Castilla) and COM (orange-pigmented kernel, Caylloma) had the highest total phenolic (246.7 mg/100 g dried weight basis, DW) and carotenoid (1.95 μg/g DW) contents among all samples. The variable Andean environmental conditions along with differences in farming practices may play a role and should be confirmed with further studies. Current results provide the metabolomic basis for future research using integrated omics platforms targeted toward the complete characterization of the ethnic-relevant maize race Cabanita.
Introduction
Peru is in the central western region of South America and has been considered a megadiverse country since 2002 according to the Cancun Statement recognized by the United Nations (1, 2). Around 84 of the 117 life zones in the planet are found in Peru (3). The Andes Mountains located along its territory creating a complex geography with diversity of climates and ecosystems contains unique biodiversity hotspots (3, 4). These natural features together with the targeted selection and adaptation of several plant species by ancient Peruvian inhabitants over thousands of years have given rise to the high crop biodiversity found presently in Peru (3).
Maize (Zea mays L. ssp. mays) is one of the most important staple foods in Peru and is currently cultivated from regions at sea level to different Andean highland areas (5). The maize genetic diversity is classified into races which are defined as “a group of related individuals with enough characteristics in common to permit their recognition as a group” (6). A race of maize is comprised by landraces that are highly heterogeneous and genetically diverse open-pollinated dynamic populations developed under traditional farming systems (7–9). The landraces belonging to a same race show similar morphological characteristics, geographical distribution, ecological adaptation, and cultural importance (10). Peru and Mexico have been considered the two primary centers of maize domestication and both countries have the largest number of maize races in the world (11, 12). The Andean region is likely the zone with the major diversity of maize races in the world in terms of varied phenotypic characteristics such as kernel and ear morphology and pigmentations (13, 14). Nevertheless, the scientific information about Andean maize landraces is very limited in comparison with the significant research focused on Mexican germplasm (5).
The Peruvian maize genetic diversity was initially classified by Grobman et al. (15) who described 49 races and collected around 3931 accessions that have been preserved ex situ by the Programa de Investigación y Proyección Social en Maíz (PIPS Maíz) located at UNALM (Universidad Nacional Agraria La Molina, Lima, Peru). The Peruvian Ministry of Environment has conducted a new collection of maize landraces since 2013 with the aim to update the maize race classification (14). This is an ongoing process which is also considering the relevance of the cultural background and uses associated with each maize race. As a result, 52 races have been identified including new races and removing others (14).
The maize race Cabanita has been used as staple food since pre-Hispanic times in the southern Andean region of Arequipa in Peru. Its name likely derives from “Cabanaconde” which is the district name located in the Caylloma province where it is currently grown at around 3000 m above sea level on average. This amylaceous maize has importance at nutritional and economical levels for local indigenous communities since it is used in several traditional food preparations and sometimes represents their main economic income. In fact, the production of amylaceous maize has been partially responsible for the evident overcoming of rural poverty in Peru. However, the introduction of improved hybrids by some farmers may lead to a possible genetic erosion that should be avoided for preserving its genetic heterogeneity, which is the foundation for overall food crop resilience. The race Cabanita was not considered in the first classification probably due to the few samples collected from this region at that time; however, the current classification includes this race (14). It has been reported that its geographical distribution encompasses not only Arequipa, but also Moquegua and Tacna regions which are also located in the southern area of Peru (14).
The Latin American maize diversity has been highlighted as an important biological heritage that may play a fundamental essential role on the worldwide food security, and resilience to climate change (12). Recently, the genetic diversity of maize landraces has been related to variable bioactive profiles mainly phenolic and carotenoid compounds with different health-relevant functional properties (16). In case of Peru, research has been focused mostly on purple maize (Kculli race) because of its high content of anthocyanins (16, 17). Different studies have reported the phenolic composition of Peruvian purple maize and highlighted its associated bioactivity such as the antioxidant, antimicrobial, anti-hypertensive, and anti-cancer potential (18–23). However, limited information exists about the bioactive composition of other Peruvian maize races. Ranilla et al. (24) evaluated part of the wide Peruvian maize diversity in relation to its phenolic-antioxidant bioactive compounds and in vitro potential for the management of hyperglycemia and obesity. Traditional races from Arequipa including some samples (only 6) from the race Cabanita were evaluated in this study. The Cabanita group showed the second highest total ORAC (oxygen radical absorbance antioxidant capacity) value and α-amylase inhibitory activity relevant for hyperglycemia prevention after the Kculli group (purple-pigmented maize) (24). Hence, strategies integrating critical stakeholders for sustaining conservation coupled with advancing multidisciplinary research of Peruvian maize diversity should be developed.
Based on the previous research advances, the objective of the current study was to evaluate the diversity of the Peruvian Andean maize race Cabanita with respect to its bioactive profiles (free and bound phenolic and carotenoid composition), physical characteristics, and in vitro antioxidant properties. A collection of new Cabanita germplasm from ten Andean locations in Arequipa was performed. In addition, the information about the pre-harvest agronomical practices linked to the cultivation of Cabanita race was also documented. Results from this study could be further integrated to molecular approaches aimed at the complete characterization of the race Cabanita and the future development of targeted health and climate resilience targeted breeding applications for the benefit of indigenous Andean communities.
Materials and methods
Materials
New germplasm of the maize race Cabanita was collected from two Andean provinces (Caylloma and Castilla) within the Arequipa region in Peru according to Ranilla et al. (24). The geographical information (coordinates, altitude, localities of origin) and the codification of maize samples are shown in Table 1. Around 9–20 and 9–12 ear units per maize type (red, white, yellow, or mixed pigmentation) and at commercial maturity were collected from Caylloma and Castilla provinces, respectively. At least five locations from each province were randomly sampled (total 10 locations) during the traditional harvest periods (May 2019 and June 2019 in case of maize from Caylloma and Castilla, respectively). All samples from Caylloma were collected from the Cabanaconde district (Comision de Usuarios La Campiña) whereas maize from Castilla was collected from three districts (Andahua, Ayo, Chachas) (Table 1). Maize ears (with husks) were mostly harvested directly from the plant. However, in some cases, ears were sampled when the maize plants were already cut and piled on the land or recently stored in the farmer’s warehouses. Samples were stored in cloth bags, protected from the light, and transported at 18–20°C to the laboratory in a maximum period of 34 h. Since ear samples were partially humid, the husks were eliminated and the ears were dried at environmental conditions with light protection until constant weight trying to mimic farmers’ postharvest practices but under controlled parameters (18.5–21.5°C, 29–34% of relative humidity). After drying, samples from each location and type were divided in 3–4 groups according to the similarity of their kernel pigmentation and ear characteristics. Each group corresponded to a biological replicate. Figures 1, 2 show pictures of ears and kernel samples from Caylloma and Castilla provinces, respectively. In addition, information about the agronomic pre-harvest practices used by local farmers for the cultivation of sampled maize plants was compiled (Supplementary Tables 1, 2 for samples from Caylloma and Castilla, respectively).
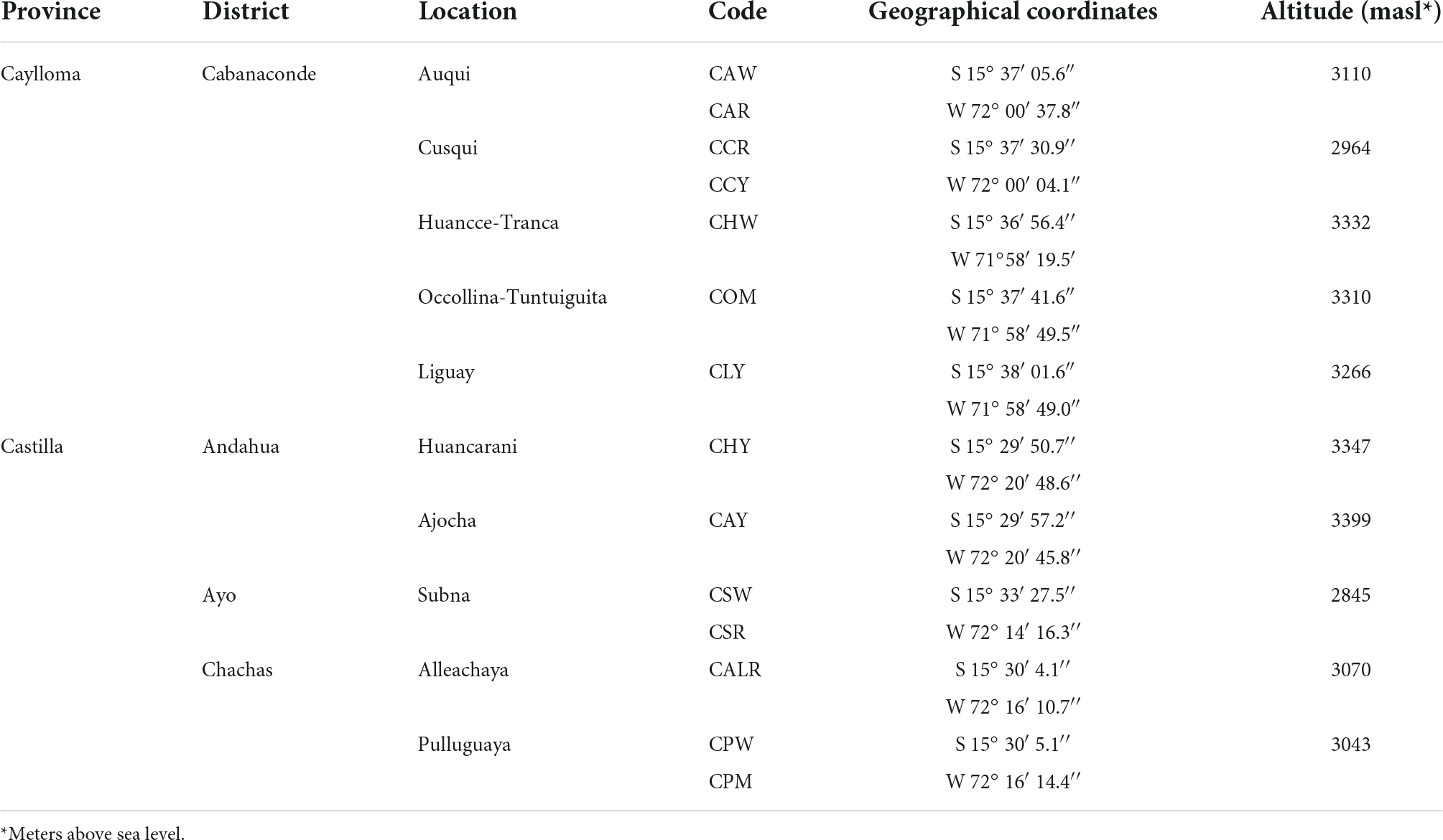
Table 1. Geographical information of Cabanita maize samples collected from Caylloma and Castilla provinces.
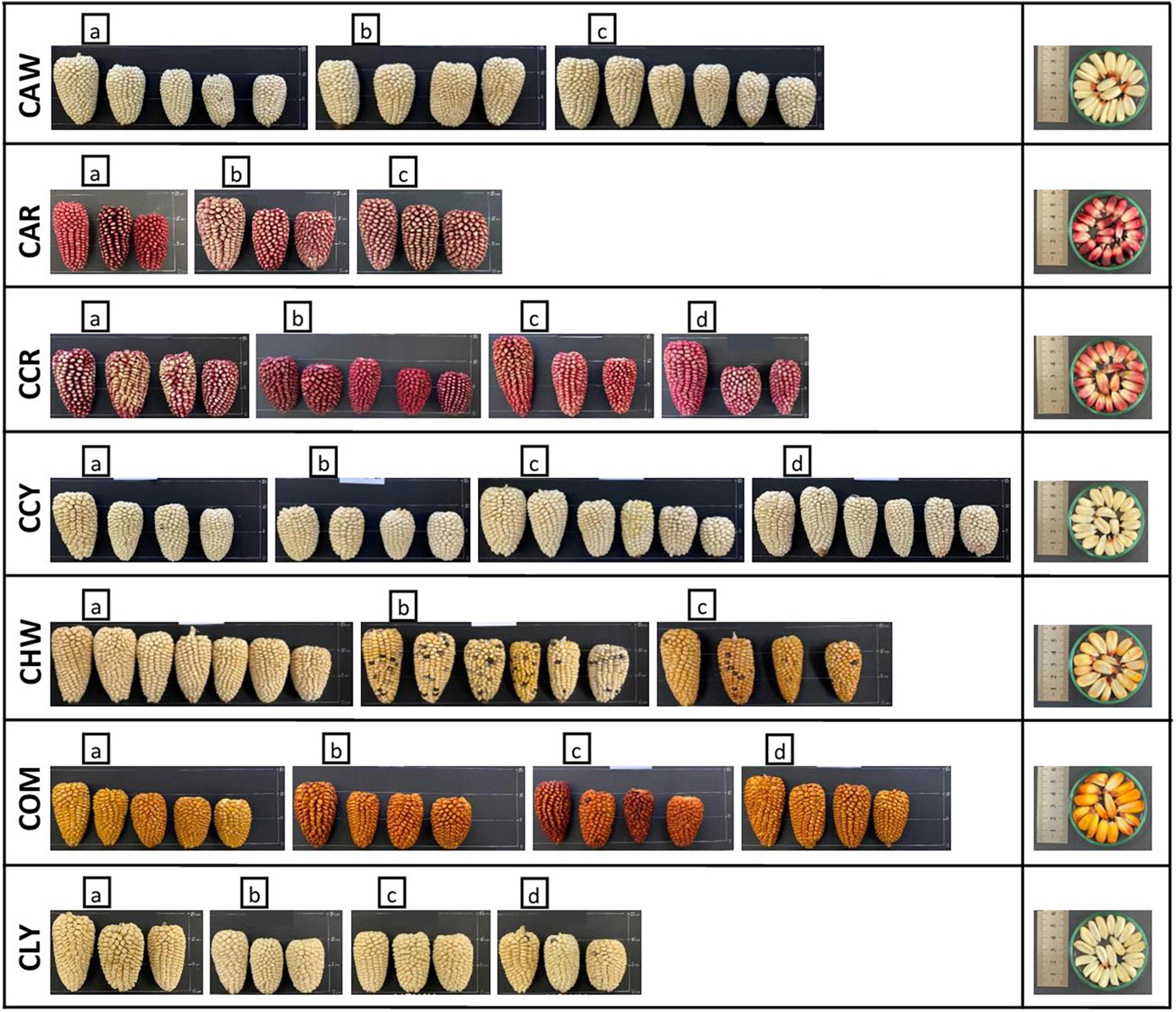
Figure 1. Ears and kernels of Cabanita maize samples collected from the Caylloma province located in the Arequipa region in Peru. Letters within each code location show the biological replicates.
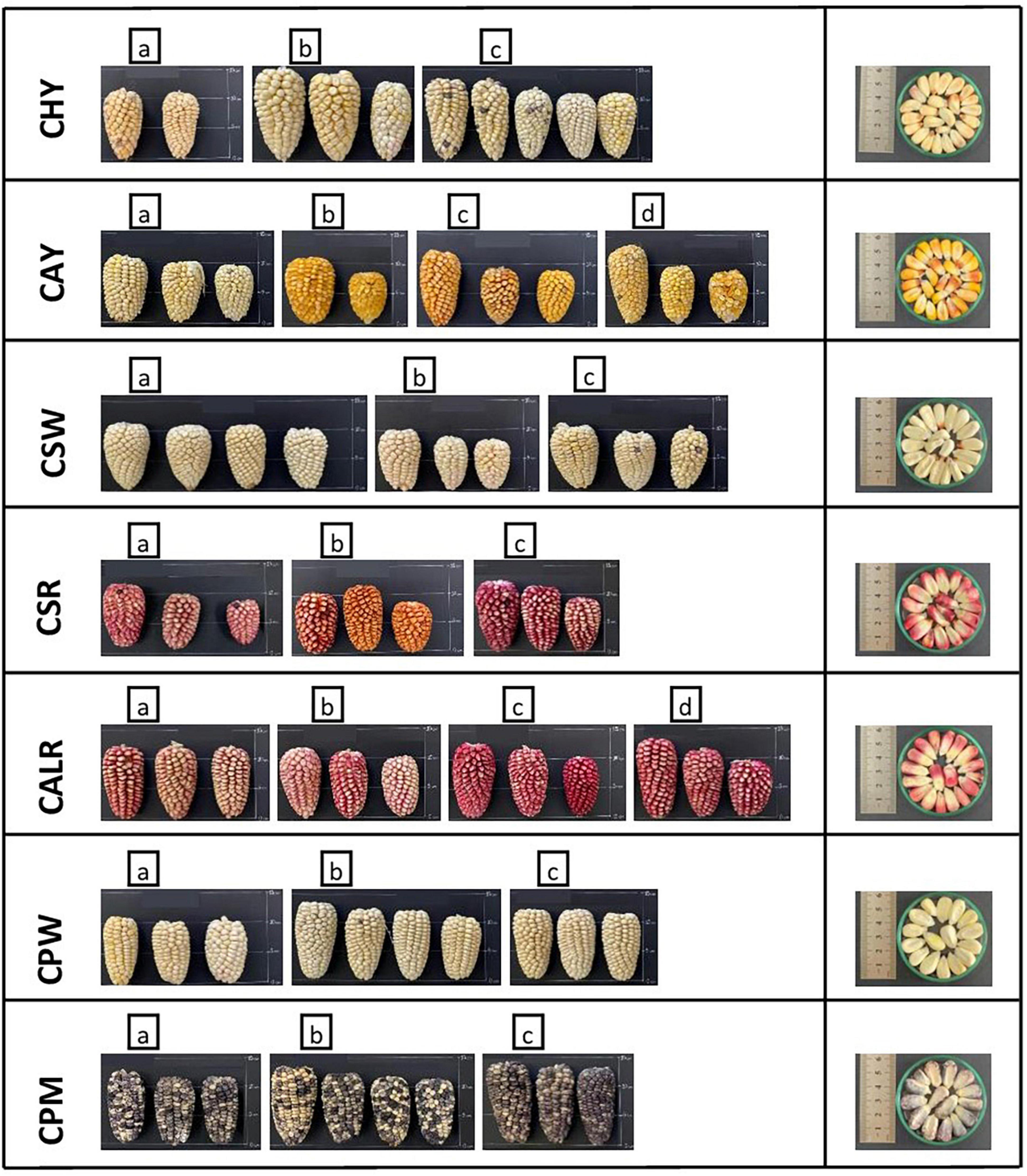
Figure 2. Ears and kernels of Cabanita maize samples collected from the Castilla province located in the Arequipa region in Peru. Letters within each code location show the biological replicates.
Dried ear maize samples and their corresponding kernels were used to evaluate the physical characteristics. Then, kernels were separated, pooled (per replicate) and stored at 5°C. A subsample of 50 g kernel was milled in a A11 Basic analytical mill (IKA, Germany) to a powdered flour (500 μm) and stored at –20°C until analysis.
Reagents
Phenolic standards (ferulic acid, p-coumaric acid, cyanidin chloride, gallic acid), carotenoid standards (lutein, zeaxanthin), and the Folin-Ciocalteu reagent were from Sigma-Aldrich (St. Louis, MO, USA). The (±)-6-hydroxy-2,5,7,8-tetramethyl-chromane-2-carboxilic acid (Trolox), and the 2,2-diphenyl-1-picrylhydrazyl (DPPH⋅), and 2-2′-azino-bis(3ethylbenothiazoline-6-sulfonic acid) (ABTS⋅+) radicals were purchased from Sigma-Aldrich, USA.
Moisture analysis and physical measurements
The moisture of kernels was determined by a gravimetric method at 105°C until constant weight (25). The physical characteristics were evaluated in both dried ears and kernels per replicate according to the descriptors defined by the Programa de Investigación y Proyección Social en Maíz (PIPS Maíz) (26) and CIMMYT (27). The weight (g), length (cm), tip, center and base diameters (cm), and the pith, rachis and cob diameters (cm) were measured in maize ear samples. Additionally, the number of rows per ear and the number of kernels per row were analyzed. In case of kernels, a row per ear with complete and sound grains was selected and 10 kernels were extracted from the central part. The kernel weight (g), length, width, and thickness (mm) were determined.
Extraction of bioactive compounds from maize samples
Free and bound phenolic fractions
The free and bound phenolic fractions were extracted from the powdered maize samples following the methodology of Ranilla et al. (18) and Ranilla et al. (24). A mix of 0.1% HCl methanol/acetone/water (45:45:10, v/v/v) was used for the extraction of the free phenolic compounds. An alkaline hydrolysis with 3 N NaOH was applied on the free phenolic extraction residue for the release of the bound phenolic fraction (18, 24). Final aqueous extracts were kept at –20°C until further analysis.
Carotenoids
Preliminary trials based on the carotenoid extraction conditions reported by Egesel et al. (28) and Fuentealba et al. (29) were performed. The extraction of carotenoid compounds from powdered maize samples was applied according to Egesel et al. (28) with some modifications (30). An amount of 2–2.5 g of sample was mixed with 6 mL ethanol containing 0.01% (w/v) butylated hydroxytoluene (BHT), and then placed in a water bath at 85°C for 5 min. After this, 120 μL 80% KOH (w/v) was added, vortexed for 15 s, and further heated in the water bath for 10 min (with a 10 s mixing at minute 5). Samples were cooled in an ice bath and 3 mL of methanol:ethyl acetate (6:4, v/v) were added. The mixture was vortexed for 20 s and centrifuged at 2800 rpm for 10 min and the extract was stored under refrigeration (5°C). The resulting pellet was reextracted several times with same solvent volume until obtaining a clear final extract. Processed and stored extracts were pooled, first vacuum-evaporated, then concentrated under nitrogen atmosphere, and set to a known volume (3.5–5 mL) with methanol:ethyl acetate (6:4, v/v). All the procedure was carried out under light and oxygen protection as possible. Carotenoid extracts were analyzed by UHPLC immediately after the extraction process the same day.
Analysis of the total phenolic contents
The Folin-Ciocalteu method was applied to measure the total phenolic contents (TPC) in the free and bound phenolic fractions from maize samples (31). The absorbance was recorded at 755 nm, and results were expressed as mg of gallic acid equivalents (GAE) per 100 g sample (dry weight basis, DW).
Analysis of phenolic compounds by ultra high-performance liquid chromatography
A volume of 5 μL of the free and bound phenolic extracts previously filtered with a polyvinylidene difluoride filter (PVDF, 0.22 μm) was injected in an Ultimate 3000 RS UHPLC system (Thermo Fisher Scientific, Waltham, MA, USA) with a quaternary pump, autosampler, and coupled to a Vanquish diode array detector. Phenolic compounds were separated using a Kinetex C18 analytical column (100 × 2.1 mm i.d., 1.7 μm) with a Kinetex C18 guard column (5 × 2.1 mm i.d., 1.7 μm) (Phenomenex Inc., Torrance, CA, USA). The binary gradient elution with 0.1% formic acid and acetonitrile mobile phases, and chromatographic conditions were the same as used previously by Ranilla et al. (18) and Vargas-Yana et al. (32). The chromatograms and peaks were processed using the Chromeleon SR4 software version 7.2 (Thermo Fisher Scientific). Phenolic compounds were identified based on their retention times and spectral characteristics in comparison with external standards and the library spectra. The quantification was performed using calibration curves obtained with aglycone phenolic standards (r2 ≥ 0.9990). Peaks corresponding to phenolic acids (p-coumaric and ferulic acid) and their derivative compounds (with similar spectral features to those of pure standards, but with different retention times) were detected at 320 nm. The phenolic acid derivatives (p-coumaric and ferulic acid derivatives) were quantified using their corresponding phenolic acid calibration curves (p-coumaric and ferulic acid, respectively). Anthocyanins were detected at 525 nm and quantified as cyanidin chloride. Results were expressed as mg per 100 g sample DW.
Analysis of carotenoid compounds by ultra high-performance liquid chromatography
Carotenoid extracts were filtered with a PVDF filter (0.22 μm) and injected (50 μL) in the same UHPLC system used for the analysis of phenolic compounds. Carotenoids were separated using a YMC carotenoid C30 analytical column (150 × 4.6 mm i.d., 3 μm) protected with a YMC C30 guard column (10 × 4.0 mm, 3 μm) (YMC CO., LTD, Kyoto, Japan). The chromatographic process was carried out at a flow rate of 1.7 mL/min, sample temperature of 10°C, column temperature of 30°C, and eluates were monitored at 450 nm. The reverse phase elution was performed using a ternary gradient elution with methanol (A), dichloromethane (B), and acetonitrile (C). The initial conditions were A/B/C (76/5/19) from 0 to 3.2 min, then solvent B was increased to 34% at 23.3 min (A/B/C: 52.8/34/13.2), and changed to initial conditions until the 25.1 min. The column was equilibrated for 2.9 more min (total run time of 28 min). The carotenoid peak identification was conducted by comparison of their retention times and spectral characteristics with those of external standards. Lutein and zeaxanthin were quantified using calibration curves (r2 ≥ 0.9900) built with pure carotenoid standards. Lutein isomers were quantified as lutein. Results were presented as μg per g sample DW.
2,2-Diphenyl-1-picrylhydrazyl radical inhibition antioxidant capacity
The 2,2-diphenyl-1-picrylhydrazyl radical (DPPH) antioxidant activity was determined in the free and bound phenolic extracts. In addition, the hydrophilic and lipophilic fractions from maize samples were obtained and analyzed. The extraction procedure reported by Fuentealba et al. (29) and Campos et al. (33) was followed with some modifications. For the hydrophilic fraction, a volume of 5 mL 80% methanol was added to 0.2 g of maize sample and mixed in an orbital shaker for 30 min at 230 rpm (environmental conditions). The homogenate was centrifuged at 5000 rpm for 15 min at 4°C and the supernatant (hydrophilic fraction) was separated and kept at – 20°C until use. The residual pellet was used for the extraction of the lipophilic fraction by the addition of 2 mL of dichloromethane, followed by shaking at 230 rpm for 30 min at environmental temperature. The mixture was centrifuged at 5,000 rpm, 4°C for 15 min. The supernatant (lipophilic fraction) was separated and stored at – 20°C until further analysis.
The DPPH method of Duarte-Almeida et al. (34) with modifications was applied. Sample extracts (40 μL) were mixed with 250 μL of diluted 317 μM DPPH solution in methanol. After 25 min of reaction at 25°C, the absorbance decrease was measured at 517 nm in a Biotek Synergy HTX microplate reader (Agilent, Santa Clara, CA, USA). A control with methanol or dichloromethane was included (for the aqueous hydrophilic, or lipophilic extracts, respectively). The antioxidant capacity was expressed as μmol Trolox equivalents per 100 g DW using a standard curve of Trolox (20–160 in methanol and 10–160 μM in dichloromethane, for the aqueous and hydrophilic, and lipophilic extracts, respectively).
2,2′-Azino-bis(3-ethylbenzothiazoline-6-sulfonic acid) radical cation (ABTS⋅+) inhibition antioxidant capacity
The ABTS antioxidant assay was determined in maize phenolic extracts (free and bound fractions), and the hydrophilic and lipophilic extracts according to Fuentealba et al. (29). A volume of 250 μL of 14.3 mM ABTS⋅+ diluted in 96% ethanol was added to 40 μL of sample extract. The reaction was carried out at 25°C for 30 min. Following which, the absorbance was recorded at 734 nm in a Biotek Synergy HTX microplate reader (Agilent). A control with methanol or dichloromethane was included (for the aqueous hydrophilic, or lipophilic extracts, respectively). Results were expressed as μmol Trolox equivalents per 100 g DW using same calibration curves as previously stated for the DPPH assay.
Statistical analysis
All results (from 3 to 4 independent biological replicates) were expressed as means ± standard deviation. The data normality and variance homogeneity were evaluated with the Shapiro–Wilk and Levene tests, respectively (α = 0.05). Data were subjected to an analysis of variance (ANOVA) with the HSD Tukey test for multiple comparisons or the Kruskall–Wallis test and the Dunn’s test with Bonferoni arrangement for mean comparisons. The R software version 4.0.4 (R Foundation for Statistical Computing, Vienna, Austria) was used. The unsupervised multivariate principal component analysis (PCA) was also applied to all data using the Unscrambler® X software version 10.4 (Aspen Technology, Inc., Bedford, MA, USA). Further, Pearson correlations among all data were explored using the Statgraphics Centurion XVI software (StatPoint Inc., Rockville, MD, USA).
Results and discussion
Environmental conditions and pre-harvest agricultural management associated with the cultivation of evaluated maize samples
The environmental conditions (maximum and minimum temperature, rainfall, and relative humidity) linked to the period of maize cultivation (August-2018 to May-2019) from each district of sample’s origin are shown in Supplementary Figures 1–4 (Cabanaconde, Andahua, Chachas, and Ayo districts, respectively). The maximum temperature was overall less variable than minimum temperature ranges in all districts. The Andahua district (Castilla province) showed the lowest minimum (2.8–6.0°C) and maximum (16–18.7°C) temperature ranges, whereas the Ayo district (Castilla province) had the highest minimum (6–13°C) and maximum (27.6–29.9°C) temperature values. The relative humidity variability was similar in the Andahua and Chachas districts (Castilla province), but their average values were lower (57.7 and 54.7%, respectively) than values shown in the Cabanaconde and Ayo districts (81.7 and 70.1%, respectively). The precipitation levels were very low in all districts, showing only some millimeters per day in January and February in all cases. The ultraviolet (UV) index was measured during the collection of maize samples in most of the localities from both provinces (data not shown). Values varied from 7.5 to 10 (at noon time approximately) and are classified as “high to very high” according to the World Health Organization scale (35). Based on these climate characteristics, Cabanita maize plants might have been exposed to variable abiotic stress factors. Garcia et al. (36) have also reported similar environmental harsh conditions in Andean highlands.
Supplementary Tables 1, 2 show information about the agricultural management practices applied by farmers during the cultivation of Cabanita maize evaluated in the current research. Maize had a vegetative stage of 8 months and is sown once a year around the same period in both provinces. Farmers from both provinces follow similar farming practices such as the exchange of selected maize kernels among their neighboring communities to be used as seeds, the use of organic fertilizers for soil preparation, and a fallow stage of 3–4 months. The traditional seed exchange systems have shown to generate a flow of genes that is crucial for the in situ agrobiodiversity conservation (37). Likewise, fallow systems have been used in traditional agriculture to regenerate and improve soil fertility through the enhancement of soil organic matter following continuous cropping specially in the smallholder farming systems (38, 39). This indicates that farmers from this southern Peruvian Andean region generally preserve ancestral agricultural techniques relevant for biodiversity conservation and overall cultivate maize under organic conditions (control of weeds and pests manually or with flood irrigation, respectively).
However, some differences have been observed in relation to the presence of previous or simultaneous crops, such as the use of organic fertilizers during maize growth, and the origin of the water for irrigation. Caylloma lands seem to be exclusively used for Cabanita maize cultivation, the soil fertilization during the maize growth is not frequent, and some Medicago species may emerge at this stage (Supplementary Tables 1, 2). In contrast, farmers from Castilla reported previous crops in same lands used for maize cultivation including tuberous species, legumes, and cereals (oat, quinoa, barley), the soil is fertilized with manure during the maize growth, and other plant species such as certain legumes (Vicia faba and Medicago sativa) are simultaneously grown with maize plants. Legumes including Vicia fava have shown high potential for nitrogen fixation, and other legume species have been already used in combination with grasses to improve the soil nutrient regeneration and its physical characteristics in the Peruvian highlands during the fallow stages (40, 41). In addition, some farmers from Caylloma stated out the use of cattle manure or domestic poultry manure, whereas other farmers from Castilla used guinea pig residues besides the cattle manure for soil preparation (information not completely provided). The type of crop rotation and organic fertilization may determine differences in the rhizosphere ecosystems influencing the soil microbiome diversity and composition, and consequently impacting its agronomic performance (42, 43). Additionally, the levels of irrigation have shown to affect the yield and nutritional quality of maize (44). No information about the soil composition and the irrigation frequency from each location were obtained in the current study. The effect of the specific agricultural management differences applied by farmers and the likely interaction with the Andean environmental conditions on soil characteristics along with the influence of these factors on maize quality and composition should be better investigated in future studies.
Physical characteristics of maize samples
Quantitative physical characteristics were measured in dried ears and kernels of Cabanita maize as shown in Table 2. In general, maize from the Castilla province showed higher variability in their physical descriptors than samples from Caylloma according to the statistical analysis. Maize ears from Caylloma were characterized for showing higher length, center diameter values, along with higher pith, rachis, and cob diameters than ears from Castilla. Samples CHW and CAW had the highest length and center diameters among all samples (12.4 and 6.0 cm, respectively). No statistical differences were observed in the tip and base diameters in maize from both provinces. However, the base diameter ranges were higher than tip values indicating that Cabanita maize ears exhibit a conic-cylindrical shape.
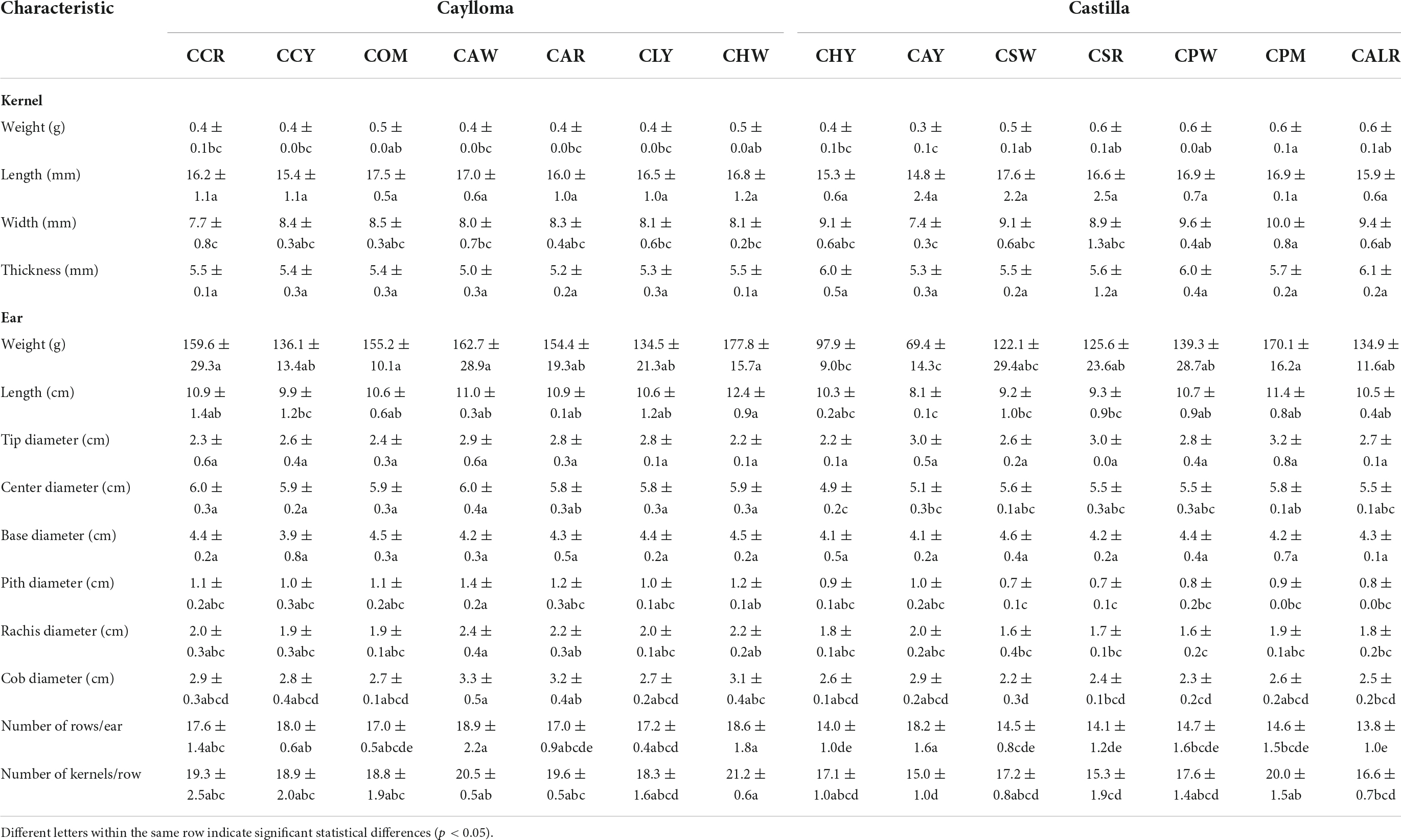
Table 2. Physical characteristics of Cabanita maize samples (ear and kernel) from Caylloma and Castilla provinces.
The yield-relevant physical parameters such as the ear weight, number of rows per ear and number of kernels per row were higher in maize from Caylloma (136.1–177.8 g, 17.0–18.9, 18.3–21.2; respectively) than ranges observed in samples from Castilla (69.4–170.1 g, 13.8–18.2, 15–20; respectively). Maize CHW, followed by CAW, CCR, and COM all from Caylloma exhibited the highest ear weights (177.8, 162.7, 159.6, and 155.2 g, respectively). In case of Castilla, only CPM sample had a comparable ear weight (170.0 g) as the observed ranges in maize from Caylloma. A strong positive correlation was obtained between the number of rows per ear and the ear weight (r = 0.7767, p < 0.05). Soil characteristics linked to different fallow and fertilization systems have shown to impact yield in several crops including maize (45, 46). As stated previously, some differences have been observed during the pre-harvest agricultural management of Cabanita cultivation that may determine better yields in maize grown in Caylloma than in Castilla. Other factors such as genetic, and environmental conditions might be also involved and need further research.
In relation to the physical characteristics of kernels, no differences were found in the length and thickness of samples from both provinces. Nevertheless, the weight and width values were more variable in samples from Castilla than in maize kernels from Caylloma. The kernel width and weight ranged from 7.7 to 8.5 mm and from 0.4 to 0.5 g, respectively in Caylloma maize. In case of Castilla, the width and weight results varied from 7.4 to 10.0 mm and from 0.3 to 0.6 g, respectively. Sample CPM had the highest width and weight values among all samples.
The variability of kernel pigmentations as shown in Figures 1, 2 may be related to differences in the bioactive compound contents and profiles as will be further discussed in next sections. In contrast to a previous investigation where only some Cabanita maize samples with white-yellow to mixed yellow-red-pigmented kernels were evaluated (24), a more complete in situ collection of Cabanita maize samples with variable kernel pigmentations has been performed in the current study. The wide phenotypic diversity of the Peruvian maize races has been reported by the Peruvian Ministry of Environment (14) with limited information about the race Cabanita. Results from current study contributes to the physical characterization of this ethnically important maize race for southern Peruvian Andean communities.
Phenolic bioactive composition
The phenolic profiles and contents from Cabanita maize samples are shown in Table 3. The free phenolic fractions were mostly rich in p-coumaric acid derivatives (3.5–8.2 mg/100 g DW), followed by ferulic acid derivatives (1.3–5.9 mg/100 g DW), and free forms of p-coumaric and ferulic acids (0.2–0.9 and 0.5–0.8 mg/100 g DW, respectively). Flavonoids such as anthocyanins were only detected in maize with partially red or purple-pigmented kernels (CCR, CAR, CSR, CPM, CALR) at higher concentrations (0.9–5.3 mg/100 g DW) than in white maize with some variegated-purple kernels (0.1 and 0.2 mg/100 g DW for samples CHY and CHW, respectively). The bound phenolic fraction represented on average 95% of the TPC (free + bound) similarly as in previous studies with Peruvian and Chilean maize landraces (24, 47). The major bound phenolic compounds were ferulic acid (98.6–195.1 mg/100 g DW) and ferulic acid derivatives (15.0–28.2 mg/100 g DW). Bound p-coumaric acid was found at lower concentrations (10.5–19.3 mg/100 g DW). Ranilla et al. (24) first evaluated the phenolic composition in 6 Cabanita maize accessions from the same Peruvian region reporting free p-coumaric acid and ferulic acid derivatives ranges below current results (2.6–5.5 and 0.2–0.9 mg/100 g DW, respectively).
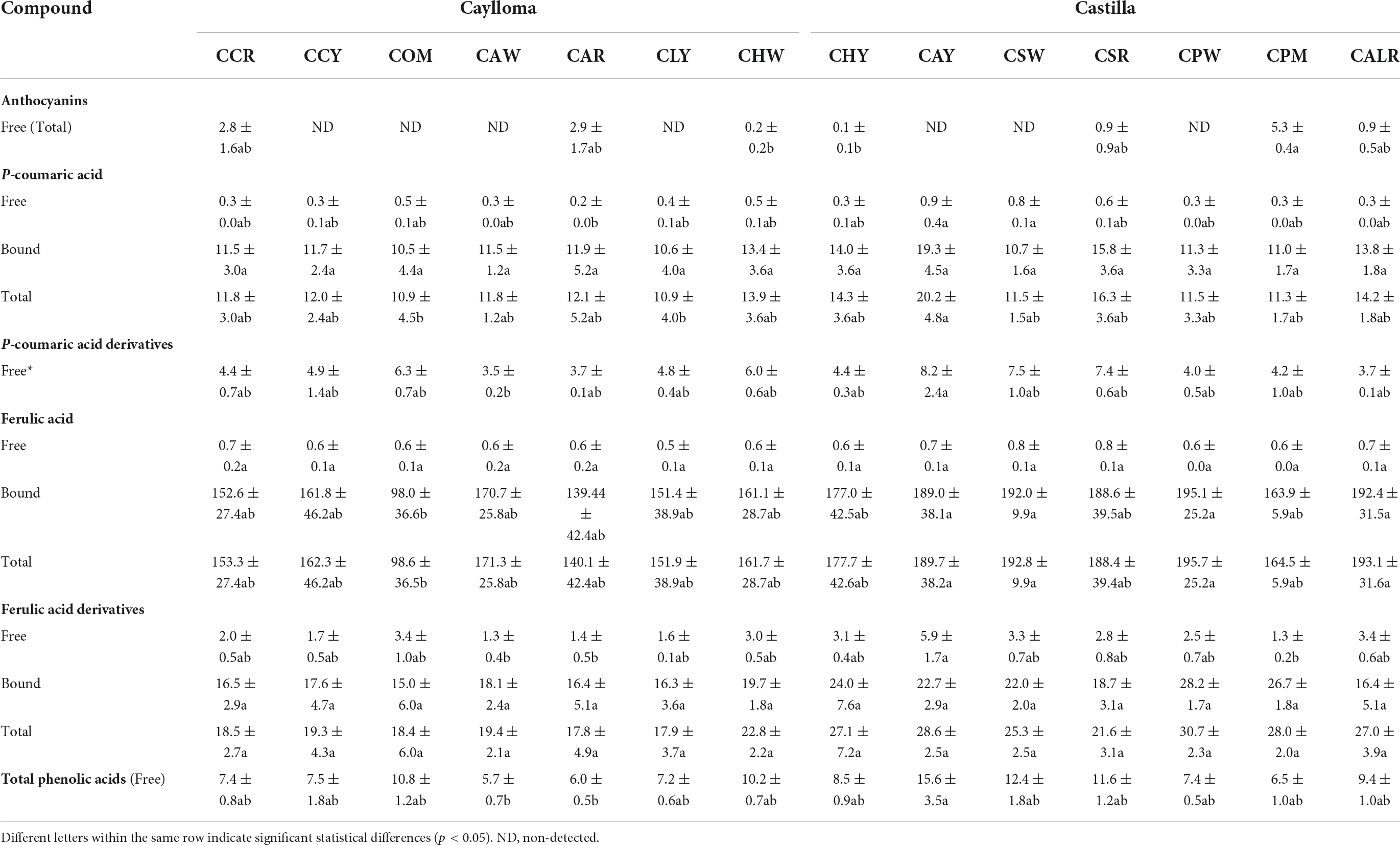
Table 3. Ultra high-performance liquid chromatography (UHPLC) phenolic profiles and contents (mg/100 g DW) of Cabanita maize samples from Caylloma and Castilla provinces.
Different soluble conjugated phenolic compounds have been found in cereals including maize (48, 49). Hydroxycinnamic acid amides (HCAAs) mainly derived from p-coumaric and ferulic acids such as N,N′-di-p-coumaroylspermine, N-p-coumaroyl-N′-feruloylputrescine and N,N′-diferuloylputrescine (DFP) have been detected in the soluble fraction of the yellow maize variety Amagrano from Germany (50). These phenolic amides were found at higher concentrations (∼14.8–18.03 mg/100 g DW) than the free forms of p-coumaric and ferulic acids (∼3.5–3.9 mg/100 g DW) whereas only traces of mono- and dihydroxycinnamoyl glycerides have been detected (50). Likewise, DFP, feruloylputrescine, cinnamoylputrescine and caffeoylputrescine (0.1–2.4 mg/100 g DW in total in whole grain) have been found in a wide diversity of Mexican maize landraces and have been proposed as taxonomic markers (51). The concentrations of hydroxycinnamic acid derivatives found in this investigation are consistent with ranges reported in above studies. Additional analyses with better analytical detection are needed to confirm the identification of soluble phenolic compounds in Cabanita maize. HCAAs are more concentrated in the outer layers (pericarp and aleurone) of maize kernel and have been shown to be part of the natural plant defense against pests and other biotic and abiotic stress factors (51–53). The anthocyanin concentrations found in Cabanita maize agree with the amounts reported by Paulsmayer et al. (54) (0.3–12.8 mg/100 g DW of total anthocyanins with a HPLC method) in diverse pink aleurone maize germplasm from Mexico and the United States with similar kernel pigmentations as those observed in Cabanita maize samples (Figures 1, 2). Partially red-pigmented kernels in some evaluated samples indicate lower anthocyanin contents in comparison to purple pericarp-pigmented maize (18).
In case of the bound phenolic fraction, overall higher concentrations of ferulic acid, and ferulic acid derivatives have been detected in current Cabanita maize than in a previous study with Peruvian germplasm (107–139 and 17–21 mg/100 DW) (24). In addition, comparable bound p-coumaric acid values (19.6–22.5 mg/100 g DW) has been obtained in the same research as those found in this current research. Discrepancy of current results from previously reported phenolic values in same maize race may be related to differences in the pre-harvest practices and sampling conditions and is explained at the end of this section. Ferulic acid and p-coumaric acid have also been highlighted as the major bound or insoluble phenolic compounds in other maize landraces and different mature cereal grains such as wheat, rice, and barley (47, 55–57). Other minor bound phenolic compounds in maize comprise of diferulic acids such as 8-O-4′-diferulic acid, 5,5′-diferulic acid, and 8,5′-diferulic acid along with triferulic acids (50). It is most likely that ferulic acid derivatives observed in Cabanita maize are diferulic or triferulic acids, but this should be further confirmed with mass spectrometry analyses. Total diferulic acid contents found by Zavala-López et al. (58) in several modern and traditional maize hybrids were lower (4.3–13.9 mg/100 g DW) than ferulic acid derivatives quantified in this research (15.0–28.2 mg/100 g DW).
Table 4 shows the TPC determined in the free and bound phenolic fractions using the UHPLC and the Folin-Ciocalteu methods. Bound phenolic contents were overall similar with both methods; however, higher free TPC values were detected with the spectrophotometric method than by UHPLC. This may be explained by the presence of other non-phenolic soluble reducing compounds in evaluated maize extracts. It has been reported that the Folin-Ciocalteu method lacks specificity because of several interfering compounds including proteins, amino acids, aromatic amines, sugars, organic acids, among other organic compounds (59). Overestimated free phenolic contents have been found in yellow corn flour which was related to the presence of soluble interfering compounds such as proteins and reducing sugars (60).
More statistical variability was observed in the UHPLC results than in those obtained by the spectrophotometric method (TPC). In general, phenolic levels in the free fraction were not much affected by the province of origin. UHPLC free phenolic ranges were 8.7–15.6 mg/100 g DW and 5.7–10.8 mg/100 g DW, for Castilla and Caylloma samples, respectively. However, Cabanita maize from Castilla had higher bound phenolic contents than Caylloma samples. The total bound phenolic values were 201.6–234.6 and 123.4–200.3 mg/100 g DW for Castilla and Caylloma samples, respectively. A similar trend was observed in the TPCs (free + bound) with both methods. Sample CAY (Castilla) showed the highest UHPLC TPCs followed by CPW sample (Castilla) (246.7 and 242.0 mg/100 g DW, respectively) whereas COM (Caylloma) maize had the lowest concentrations (134.3 mg/100 g DW). CAY maize also exhibited the highest total p-coumaric acid and p-coumaric acid derivatives along with high ferulic acid contents similarly as in other Castilla samples (CPW, CALR, CSW).
The TPC (free + bound) range of this investigation (201.5–291.1 mg GAE/100 g DW) was almost twofold higher than results obtained previously in kernels from the same Peruvian race (133.5–158.4 mg GAE/100 g DW) (24). This may be explained by differences in the sampling procedure. In current study, the ears collection was generally performed directly from the plant or recently harvested plants. Ranilla et al. (24) evaluated stored kernels from germplasm bank or farmers’ warehouses who generally stored dried kernels under indeterminate time and after a sun-drying process for several weeks on the land. The storage time have shown to decrease the phenolic concentrations in sorghum grain and flour (61). Other factors related to the cultivation and harvesting practices also influence the phenolic contents in cereal grains and might also explain observed differences (62). The TPC range obtained in this study was comparable to that reported by De la Parra et al. (63) (243.8–320.1 mg GAE/100 g DW, samples from the United States) and higher than results found in maize landraces with variable pigmentations from Chile (132.2–262.5 mg GAE/100 g DW) (47), Mexico (77.3–123.6 mg GAE/100 g DW) (64) and India (102.3–206.4 mg GAE/100 g DW) (65).
Carotenoid composition
The total carotenoid contents in evaluated samples were lower (0.09–1.95 μg/g DW) than levels of phenolic compounds (Table 5). Xanthophylls such as lutein, lutein isomers and zeaxanthin were found in orange-pigmented (COM) and white-yellow samples (CCY, CLY, CHW, CAY, CPW). No carotenoids have been detected in most partially red-pigmented kernels (CCR, CAR, CALR) and some white maize (CHY, CSW). Lutein and lutein isomers were the major carotenoids in all Cabanita maize except in CHW sample where higher zeaxanthin (0.39 μg/g DW) than lutein levels (∼0.20 μg/g DW) were found. Sample COM (Caylloma) had the highest total carotenoid values (1.95 μg/g DW), followed by CAY (0.85 μg/g DW, Castilla) and CHW (0.57 μg/g DW, Caylloma). Samples CSR and CPM (with red-orange and purple variegated-pigmented kernels, respectively) which showed anthocyanin compounds (Table 3), also had carotenoids but at lower concentrations (0.09–0.31 μg/g DW).

Table 5. Carotenoid profiles and contents (μg/g DW) of Cabanita maize samples from Caylloma and Castilla provinces.
Lutein and zeaxanthin have also been shown as the major carotenoid compounds specially in white, yellow, and red-pigmented maize kernels. Kuhnen et al. (66) reported lower zeaxanthin and lutein ranges (0.08–0.18 μg/g DW and 0.03–0.07 μg/g DW, respectively) in white Brazilian maize landraces compared with yellow landraces (0.07–7.05 μg/g DW and 0.48–3.69 μg/g DW, for zeaxanthin and lutein, respectively). White maize landraces from Malawi have shown ranges of 0.14–0.18 and 0.05–0.11 μg/g DW for lutein and zeaxanthin, respectively (67). In contrast, higher contents of both xanthophylls (4.7–34.97 and 2.36–21.18 for lutein and zeaxanthin, respectively) have been obtained by Uarrota et al. (68) in yellow Brazilian landraces along with moderate ranges of different pro-vitamin A carotenoids. Further, zeaxanthin and lutein have been found at higher concentrations in a red-pigmented Italian landrace (6.6 and 2.2 μg/g DW, respectively) following by lower contents of β-cryptoxanthin and β-carotene (1.2 and 0.2 μg/g DW, respectively) (69).
Carotenoid concentrations observed in the current research are more comparable to those found in white maize, but lower than values reported in yellow and red-pigmented maize. Ryu et al. (70) pointed out that hard maize types such as pop, dent, and flint have significantly higher carotenoid contents than floury types. In addition, the endosperm fraction has been shown to contain the highest carotenoid concentrations in maize and in other cereal kernels, therefore yellow and orange endosperms have higher carotenoid concentrations than white endosperm (71, 72). Cabanita maize is an amylaceous floury type with white endosperms which likely explains its lower carotenoid levels than other maize landraces. Only sample COM showed a creamy-white endosperm among all samples and had the highest carotenoid values. The orange pigmentation of COM kernel pericarp could be partly linked to the presence of other not detected phenolic compounds in this study such as phlobaphenes. These flavan-4-ols polymers have been identified in Italian maize landraces with brick red pigmentation which is similar as that observed in COM sample (69). Phlobaphenes have been associated with resistance to mycotoxin-producing fungal infection in maize (73). Domínguez-Hernández et al. (16) have recently stated that the carotenoid characterization of maize landrace diversity is very limited and germplasm from few regions of the world including only Mexico and Brazil from the American continent have been investigated. Results from the present study contribute for the first time with information about the carotenoid composition of part of the maize diversity from Peru which is another important primary center of maize domestication in the world.
In vitro antioxidant capacity
Only one sample from each location was selected based on its higher TPCs for the following in vitro antioxidant capacity assays. Table 6 shows the health-relevant antioxidant potential of the free and bound phenolic fractions from Cabanita maize samples using the DPPH and ABTS methods. In addition, the hydrophilic and lipophilic fractions were evaluated. The bound phenolic fractions from all Cabanita samples showed the highest DPPH and ABTS free radical scavenging capacity and represented 93.5–98.2% of the total antioxidant capacity (free + bound). A significant correlation was found between the UHPLC total bound phenolic contents and the antioxidant capacity (r = 0.6111 and r = 0.5675, p < 0.05 with the DPPH and ABTS methods, respectively). Bound hydroxycinnamic acids such as p-coumaric and ferulic acids were correlated with this property (r = 0.5168, r = 0.6058 and r = 0.5444, r = 0.5454, p < 0.05, with the DPPH and ABTS methods, respectively) suggesting a high contribution of these bound phenolic acids to the antioxidant potential of Cabanita maize. The free antioxidant capacity was highly correlated with the anthocyanin contents using both methods (r = 0.8367 and r = 0.8197, p < 0.05, DPPH and ABTS assays, respectively) indicating an important contribution of anthocyanins to the free antioxidant property in partially red and purple-pigmented kernels.
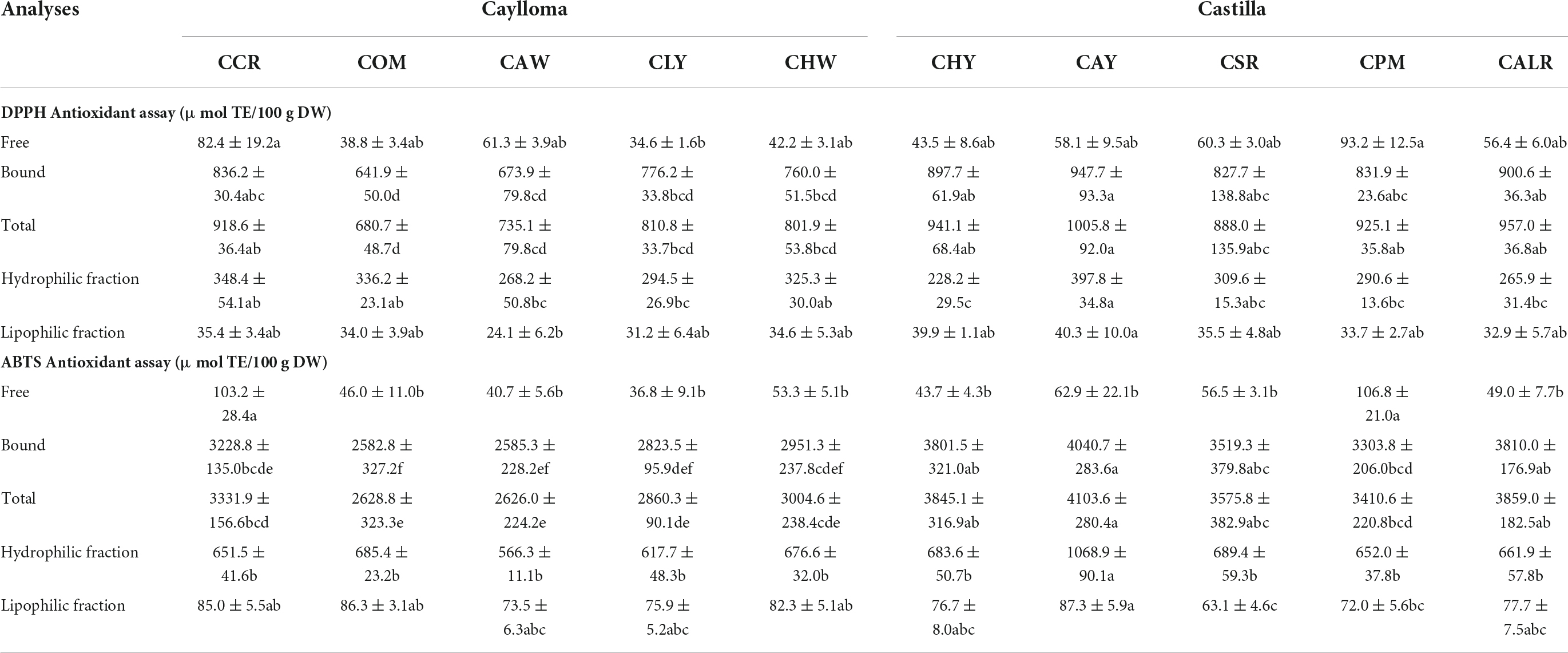
Table 6. In vitro antioxidant capacity of Cabanita maize samples from Caylloma and Castilla provinces.
The total ABTS antioxidant capacity (free + bound) from this research (2626.0–4103.6 μmol TE/100 g DW) is almost 1.4 times higher than levels reported by Ranilla et al. (24) in some Cabanita maize samples (1879.3–2942.1 μmol TE/100 g DW). This may be related to the higher phenolic contents found in this study as was previously discussed. Lower total antioxidant values than those from current investigation were determined in Chilean (1307–1850 μmol TE/100 g DW, ABTS method) and Southern Mexican maize landraces with different kernel pigmentations (377–484 μmol TE/100 g DW, DPPH method) (47, 64). In addition, comparable total antioxidant capacity has been observed in landraces from the Northeast of Mexico (2827–4264 μmol TE/100 g DW, ABTS method) and in diverse waxy maize genotypes grown in Thailand (average of 1096 and 3791 μmol TE/100 g DW with the DPPH and ABTS methods, respectively) (74, 75).
The hydrophilic antioxidant capacity (228.2–397.8 and 566.3–1068 μmol TE/100 g DW, with DPPH and ABTS assays, respectively) was higher than lipophilic antioxidant values (24.1–40.3 and 63.1–87.3 μmol TE/100 g DW, with DPPH and ABTS assays, respectively) which may be due to the higher phenolic concentrations found in Cabanita maize than lipophilic phytochemicals such as carotenoids. Significant positive correlations between the total free UHPLC phenolic contents and the hydrophilic antioxidant capacity (r = 0.6492, r = 0.7820, p < 0.05, DPPH and ABTS methods, respectively) were obtained. In case of the lipophilic antioxidant capacity, results showed moderate correlation with the total carotenoid levels (r = 0.4443, p < 0.05, ABTS method). Other minor lipophilic compounds not evaluated in this study may partially contribute to the lipophilic antioxidant property in Cabanita maize. Tocols and phytostherols have been also detected in maize (76). Recently, Lux et al. (50) found tocopherols and tocotrienols in similar ranges as those shown by total carotenoids in yellow maize.
Cabanita maize from Castilla showed higher total antioxidant capacity (free + bound) than Caylloma samples, and differences were high with the ABTS method. Ranges varied from 3410.6 to 4103.6 μmol TE/100 g DW and from 2626.0 to 3331.9 μmol TE/100 g DW for Castilla and Caylloma maize samples, respectively. CAY and CCR samples showed the highest total antioxidant capacity among Castilla and Caylloma maize, respectively. The hydrophilic and lipophilic antioxidant capacity results were in general similar in both provinces, and CAY sample (Castilla) showed the highest values (1068.9 and 87.3 μmol TE/100 g DW for the hydrophilic and lipophilic antioxidant capacity, ABTS assay). Current hydrophilic antioxidant capacity results are much higher than values reported in commercial Chinese maize (28 μmol TE/100 g DW, ABTS method), and other cereal grains such as sorghum (40 μmol TE/100 g DW, ABTS method), wheat (83 μmol TE/100 g DW, ABTS method), and barley (210–250 μmol TE/100 g DW, ABTS method) (77, 78). Comparable values have been shown in quinoa seeds (1280 and 22 μmol TE/100 g DW, hydrophilic and lipophilic fractions, respectively; ABTS method) (79). Differences may be attributed to the specific composition of hydrophilic and lipophilic phytochemicals associated with each grain food.
Principal component analysis
Underlying relationships based on all studied variables were explored through the PCA descriptive model (Figure 3). The score plot is shown considering the sample code and the district of origin of Cabanita samples. This approach retained two principal components (PC1 and PC2) which expressed 48% of the total variance of the sample data set likely indicating a certain grade of homogeneity among all evaluated maize samples from both provinces. This degree of homogeneity could be explained by the endemism of Cabanita race. Higher variability with the first two PC (61–71%) was reported by Ranilla et al. (24) when evaluating different maize races from a germplasm bank and collected in situ from the Arequipa region in Peru. In the same study, a clear discrimination based on the phenolic composition and some in vitro functional properties was found among the Kculli (purple-pigmented kernel), Granada (red-pigmented kernels), Arequipeño (white-yellow kernels) and Cabanita maize races (mostly white-pigmented kernels) (24).
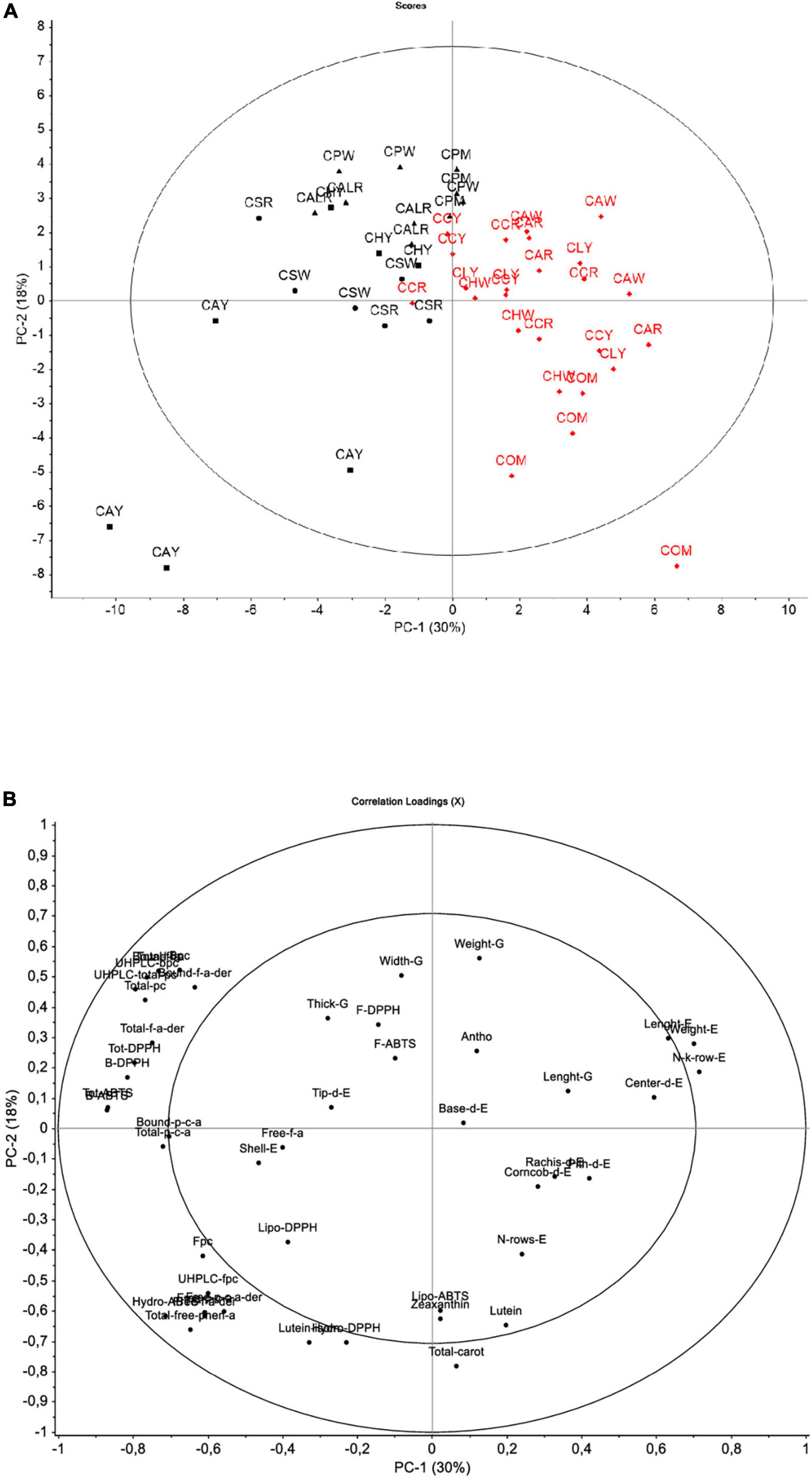
Figure 3. Score plot (A) and loading plot (B) for the principal component analysis (PCA) model for the first two factors considering of all data. In (A) Districts: box (Andahua), dot (Ayo), triangle (Chachas), diamond (Cabanaconde). Provinces: black (Castilla), red (Caylloma).
Despite the observed low retained variability of the model, PC1 (30% of explained variability) separated samples from the Castilla (Chachas, Ayo, Andahua districts) and Caylloma (Cabanaconde district) provinces (from left to right, Figure 3A). The PC2 (18% of explained variability) separated maize grown in Chachas district (samples CALR, CPW, CPM) within the Castilla province. Differences between both provinces were increased by samples CAY (Andahua district, Castilla province) and COM (Cabanaconde district, Caylloma province). A positive correlation among the phenolic compounds including the bound and total UHPLC phenolic contents, bound ferulic acid, bound ferulic acid derivatives, total bound ferulic acid, bound and total p-coumaric acid was observed. These variables were correlated with the bound and total antioxidant capacity measured with the ABTS and DPPH free radical inhibition methods. All these variables were higher in samples from the Castilla province, specially from the districts of Chachas (CALR, CAPW, CPM), Ayo (CSW, CSR), and Andahua (CHY). CAY sample (Andahua district, Castilla) showed a different pattern among the other maize samples from Castilla (bottom left side of the score plot). This sample that showed higher TPCs as previously highlighted, also had the highest free UHPLC phenolic compounds including free ferulic acid derivatives, free p-coumaric acid derivatives and total free phenolic acids. These variables had a direct relation with higher ABTS hydrophilic antioxidant capacity in CAY sample (Figure 3B).
The loading plot also shows that yield-relevant physical characteristics were inversely correlated with phenolic concentrations and the in vitro antioxidant capacity indicating that Caylloma samples (right side of the score plot) are linked to lower phenolic contents but had better agronomic yield. Data from sample COM grouped differently compared with the rest of Caylloma maize (bottom right side of the score plot, Figure 3A). Variables such as lutein, and total carotenoid concentrations were the highest in this sample and were associated with high ABTS lipophilic antioxidant capacity. Further PCA analysis excluding data from CAY and COM samples was performed and results are shown in Supplementary Figure 5. The explained variability with the first two PC decreased to 42% indicating higher homogeneity among evaluated maize samples based on studied variables. However, PC1 (29% of explained variability) consistently separated data according to the province of origin (Castilla and Caylloma samples were in the left and right side of the score plot, respectively, Supplementary Figure 5A). Samples from Castilla continued to be linked to higher phenolic contents. Overall, most of the physical kernel and ear parameters (excepting those linked to the yield), and the carotenoid contents were not significant variables (Supplementary Figure 5B).
Several studies have emphasized that the genotype has more influence on the bioactive composition of maize than differences in the agroecological factors. Giordano et al. (80) found no differences in the main phenolic acids, anthocyanins, carotenoids (β-cryptoxanthin and β-carotene) and the antioxidant capacity from several pigmented Italian landraces under different nitrogen rates. However, the genotype showed a significant effect in same study (80). Some free and bound phenolic compounds along with lipophilic compounds such as carotenoids and tocochromanols from yellow maize were increased by sowing time, but not affected by phosphate fertilization (50). Furthermore, Paulsmeyer et al. (54) pointed out that the anthocyanin profiles and concentrations were strongly influenced by genetic factors with minimal influence of environmental conditions. Based on the PCA analysis in current study (low retained variability of the models), evaluated maize from Caylloma and Castilla provinces would belong to the same landrace population or race (Cabanita); however, the heterogenous Andean climatic factors seem to affect the bioactive composition of Cabanita maize to a higher extent than the sample type. Differences between maize from both provinces could be attributed to different agroecological factors. Districts from Castilla provinces showed more extreme climatic conditions than Caylloma locations (Supplementary Figures 1–4). The phenylpropanoid metabolism in plants has shown an extreme plasticity under changes in the environmental conditions redirecting the metabolic flux to produce phenolic-derived metabolites for plant protection (81). Increased levels of TPCs, bound ferulic acid, and DPPH antioxidant capacity have been reported in kernels from Peruvian purple maize grown at highland Andean locations (with lower temperature ranges and high UV radiation) compared with maize from lowland sites (18). The biosynthesis of ferulic acid and its derivatives was induced in maize seedlings and roots, stems, and leaves of maize plants under salt stress (82). The increase of phenolic metabolites under several abiotic stress factors has been previously reported in other cereal crops (83, 84). Cold stress has also shown to impair the photosynthesis leading to a decrease in grain yield (85). This may explain the lower yield physical parameters in maize from Castilla. Nevertheless, specific agricultural management differences observed in each province as previously stated could also play a role and should be investigated in future studies.
Conclusion
The diversity of the Peruvian Andean maize race Cabanita from two provinces (Caylloma and Castilla) in the Arequipa region is a promising source of phenolic compounds with in vitro antioxidant capacity. Major free phenolic compounds in all maize samples were p-coumaric and ferulic acid derivatives whereas anthocyanins were only detected in samples with partially red and purple-pigmented kernels (CCR, CAR, CHW, CHY, CSR, CPM, CALR). The bound phenolic fractions were rich in ferulic acid and its derivatives, followed by p-coumaric acid. The hydrophilic antioxidant capacity was correlated with the free phenolic fraction, whereas bound phenolic acids highly contributed to the bound antioxidant capacity. Orange (COM) and white-yellow pigmented maize (CCY, CLY, CHW, CAY, CPW) showed carotenoid compounds mostly xanthophylls such as lutein and zeaxanthin. The multivariate analysis (PCA) revealed a low variability of integrated data indicating a grade of similarity among evaluated maize samples based on their physical, phytochemical, and antioxidant properties. However, Caylloma samples were characterized by their more uniform physical characteristics and higher yield than Castilla maize which exhibited higher phenolic contents and antioxidant capacity. Samples CAY (Castilla) and COM (Caylloma) were remarkable due to their highest phenolic and carotenoid concentrations among all samples (246.7 mg/100 g DW and 1.95 μg/g DW, respectively). The heterogeneous environmental conditions in the Andean region along with differences in the pre-harvest agricultural practices may play a role, but genetic factors may also be involved and should be further investigated. This research provides the foundations of metabolomic base for future molecular studies to better characterize the ethnic-relevant maize race Cabanita. Results from this research contribute to current efforts to ensure a good characterization of the high Peruvian maize diversity to give extra value to Peruvian biodiversity for the development of Andean indigenous health-targeted food systems.
Data availability statement
The original contributions presented in this study are included in the article and Supplementary material. Further inquiries can be directed to the corresponding authors.
Author contributions
LR and GZ conceived and designed the study. LR directed the research and wrote the manuscript. IF-C and RC-P performed the experiments and analyzed the data. SM-T helped with the experiments. HB-G coordinated and helped with the sample collection. CF performed the multivariate statistical analysis. GZ, CF, and KS critically reviewed the manuscript. All authors have read and approved the final manuscript.
Funding
This research was supported by PROCIENCIA-CONCYTEC (Peru) under the Basic Research Program 2018-01, Contract N° 114-2018-FONDECYT, and by the Vice-presidency of Research of Universidad Catolica de Santa Maria (Peru), grant number 26572-R-2019.
Acknowledgments
The authors thank Engineer Diego Vargas-Yana for the technical support along the development of the project. Evaluated Cabanita maize samples are from Peru and have been accessed under the contract N° 002-2021-MIDAGRI-INIA/DGIA (Peru) with Resolución Directoral N°0006-2021-INIA-DGIA. Information about Cabanita maize is shown in Table 1 and Figures 1, 2 of current manuscript. Access and Benefit-Sharing Clearing-House (ABSCH) ABSCH-IRCC-PE-256874-1. Internationally recognized certificate of compliance constituted from information on the permit, or its equivalent made available to the Access and Benefit-sharing Clearing-House of the Convention on Biological Diversity (CBD) (https://absch.cbd.int/en/database/ABSCH-IRCC-PE-256874).
Conflict of interest
The authors declare that the research was conducted in the absence of any commercial or financial relationships that could be construed as a potential conflict of interest.
Publisher’s note
All claims expressed in this article are solely those of the authors and do not necessarily represent those of their affiliated organizations, or those of the publisher, the editors and the reviewers. Any product that may be evaluated in this article, or claim that may be made by its manufacturer, is not guaranteed or endorsed by the publisher.
Supplementary material
The Supplementary Material for this article can be found online at: https://www.frontiersin.org/articles/10.3389/fnut.2022.983208/full#supplementary-material
References
1. United Nations, General Assembly. Annex to the letter dated 5 April 2002 from the Permanent Representative of Mexico to the United Nations addressed to the Secretary-General. Cancun Declaration of Like-Minded Megadiverse Countries. (2002). Available online at: https://digitallibrary.un.org/record/463140?ln=es (accessed April 4, 2022).
2. Peruvian Ministry of Environment. Executive summary climate scenarios for Peru to 2030. (2009). Available online at: https://repositoriodigital.minam.gob.pe/handle/123456789/311 (accessed April 4, 2022).
3. Peruvian Ministry of Environment. Peru, megadiverse country. (2008). Available online at: https://repositoriodigital.minam.gob.pe/bitstream/handle/123456789/169/BIV01165_en.pdf?sequence=2&isAllowed=y (accessed April 4, 2022).
4. Espinoza JC, Garreaud R, Poveda G, Arias PA, Molina-Carpio J, Masiokas M, et al. Hydroclimate of the Andes part I: main climatic features. Front Earth Sci. (2020) 8:64. doi: 10.3389/feart.2020.00064
5. Ranilla LG. The application of metabolomics for the study of cereal corn (Zea mays L.). Metabolites. (2020) 10:300. doi: 10.3390/metabo10080300
6. Anderson E, Cutler HC. Races of Zea mays: I. Their recognition and classification. Ann Missouri Bot Gard. (1942) 29:69–88. doi: 10.2307/2394331
7. Flint-Garcia SA. Genetics and consequences of crop domestication. J Agric Food Chem. (2013) 61:8267–76. doi: 10.1021/jf305511d
8. Salazar-Suazo ER, León-Lobos P, Rosas-Cerda M, Schick CM. Estado de la Conservación Ex Situ de los Recursos Fitogenéticos Cultivados y Silvestres en Chile. Santiago: Instituto de Investigaciones Agropecuarias-INIA (2006). p. 17–8.
9. Villa TCC, Maxted N, Scholten M, Ford-Lloyd B. Defining and identifying crop landraces. Plant Genet Resour. (2005) 3:373–84. doi: 10.1079/PGR200591
10. Harlan J, De Wet J. Toward a rational classification of cultivated plants. Taxon (1971) 40:509–17. doi: 10.2307/1218252
11. Peruvian Ministry of Environment. Informe final. Colecta, elaboración de mapas de distribución y estudio socioeconómico de la diversidad genética del maíz. (2014). Available online at: https://bioseguridad.minam.gob.pe/wp-content/uploads/2018/07/ldb_maiz_diezregiones_14.pdf (accessed April 8, 2022).
12. Guzzon F, Arandia Rios LW, Caviedes Cepeda GM, Céspedes Polo M, Chavez Cabrera A, Muriel Figueroa J, et al. Conservation and use of Latin American maize diversity: Pillar of nutrition security and cultural heritage of humanity. Agron. (2021) 11:172. doi: 10.3390/agronomy11010172
13. Sánchez JJ, Goodman MM, MckBird R, Stuber CW. Isozyme and morphological variation in maize of five Andean countries. Maydica. (2006) 51:25–42.
14. Peruvian Ministry of Environment. Línea de base de la diversidad genética del maíz peruano con fines de bioseguridad. (2018). Available online at: https://bioseguridad.minam.gob.pe/wp-content/uploads/2019/01/Linea-de-base-ma%C3%ADz-LowRes.pdf (accessed April 8, 2022).
15. Grobman A, Salhuana W, Sevilla R. Races of maize in Peru. Washington D.C: National Academy of Sciences-National Research Council (1961). 374 p.
16. Domínguez-Hernández E, Gaytán-Martínez M, Gutiérrez-Uribe JA, Domínguez-Hernández ME. The nutraceutical value of maize (Zea mays L.) landraces and the determinants of its variability: A review. J Cereal Sci. (2022) 103:103399. doi: 10.1016/j.jcs.2021.103399
17. Salvador-Reyes R, Clerici MTPS. Peruvian Andean maize: General characteristics, nutritional properties, bioactive compounds, and culinary uses. Food Res Int. (2020) 130:108934. doi: 10.1016/j.foodres.2019.108934
18. Ranilla LG, Rios-Gonzales BA, Ramírez-Pinto MF, Fuentealba C, Pedreschi R, Shetty K. Primary and phenolic metabolites analyses, in vitro health-relevant bioactivity and physical characteristics of purple corn (Zea mays L.) grown at two Andean geographical locations. Metabolites. (2021) 11:722. doi: 10.3390/metabo11110722
19. Monroy YM, Rodrigues RA, Sartoratto A, Cabral FA. Purple corn (Zea mays L.) pericarp hydroalcoholic extracts obtained by conventional processes at atmospheric pressure and by processes at high pressure. Braz J Chem Eng. (2020) 37:237–48. doi: 10.1007/s43153-020-00009-x
20. Ramos-Escudero F, Muñoz AM, Alvarado-Ortíz C, Alvarado A, Yánez JA. Purple corn (Zea mays L.) phenolic compounds profile and its assessment as an agent against oxidative stress in isolated mouse organs. J Med Food. (2012) 15:206–15. doi: 10.1089/jmf.2010.0342
21. Ranilla LG, Christopher A, Sarkar D, Shetty K, Chirinos R, Campos D. Phenolic composition and evaluation of the antimicrobial activity of free and bound phenolic fractions from a Peruvian purple corn (Zea mays L.) accession. J Food Sci. (2017) 82:2968–76. doi: 10.1111/1750-3841.13973
22. Finkel ML, Sanchez S, Mak T, Granstein J, Lefkowitz A. Anthocyanin-rich purple corn extract and its effects on the blood pressure of adults. J Evid Based Complement Altern Med. (2013) 18:237–42. doi: 10.1177/2156587213482942
23. Jing P, Bomser JA, Schwartz SJ, He J, Magnuson BA, Giusti MM. Structure- function relationships of anthocyanins from various anthocyanin-rich extracts on the inhibition of colon cancer cell growth. J Agric Food Chem. (2008) 56:9391–8. doi: 10.1021/jf8005917
24. Ranilla LG, Huamán-Alvino C, Flores-Báez O, Aquino-Méndez EM, Chirinos R, Campos D, et al. Evaluation of phenolic antioxidant-linked in vitro bioactivity of Peruvian corn (Zea mays L.) diversity targeting for potential management of hyperglycemia and obesity. J. Food Sci Technol. (2019) 56:2909–24. doi: 10.1007/s13197-019-03748-z
25. Aoac International. Official Methods of Analysis of AOAC International. 20th ed. Rockville, MD: Association of Official Analytical Chemists (2016). 3172 p.
26. Catálogo del Germoplasma de Maíz del Perú. Catálogo de Recursos Genéticos de Maíz de Sudamérica. Lima: Programa Cooperativo de Investigación en Maíz (PCIM) (1986). 237 p.
27. International Maize and Wheat Improvement Center (Cimmyt). Mexico City/International Board for Plant Genetic Resources (IBPGR), Rome. Descriptores para Maíz. (1991). Available online at: http://archive-ecpgr.cgiar.org/fileadmin/bioversity/publications/pdfs/104_Descriptors_for_maize.Descriptores_para_maiz.Descripteurs_pour_le_mais-cache=1415188810.pdf (accessed May 2, 2022).
28. Egesel CO, Wong JC, Lambert RJ, Rocheford TR. Combining ability of maize inbreds for carotenoids and tocopherols. Crop Sci. (2003) 43:818–23. doi: 10.2135/cropsci2003.8180
29. Fuentealba C, Ranilla LG, Cobos A, Olaeta JA, Defilippi BG, Chirinos R, et al. Characterization of main primary and secondary metabolites and in vitro antioxidant and antihyperglycemic properties in the mesocarp of three biotypes of Pouteria lucuma. Food Chem. (2016) 190:403–11. doi: 10.1016/j.foodchem.2015.05.111
30. Rivera S, Canela R. Influence of sample processing on the analysis of carotenoids in maize. Molecules. (2012) 17:11255–68. doi: 10.3390/molecules170911255
31. Singleton VL, Rossi JA. Colorimetry of total phenolics with phosphomolybdic-phosphotungstic acid reagents. Am J Enol Vitic. (1965) 16:144–58. doi: 10.1038/nprot.2007.102
32. Vargas-Yana D, Aguilar-Morón B, Pezo-Torres N, Shetty K, Ranilla LG. Ancestral Peruvian ethnic fermented beverage “Chicha” based on purple corn (Zea mays L.): unraveling the health-relevant functional benefits. J Ethnic Foods. (2020) 7:1–12. doi: 10.1186/s42779-020-00063-3
33. Campos D, Teran-Hilares F, Chirinos R, Aguilar-Galvez A, García-Ríos D, Pacheco-Avalos A, et al. Bioactive compounds and antioxidant activity from harvest to edible ripeness of avocado cv. Hass (Persea americana) throughout the harvest seasons. Int J Food Sci Technol. (2020) 55:2208–18. doi: 10.1111/ijfs.14474
34. Duarte-Almeida JM, Santos RJD, Genovese MI, Lajolo FM. Avaliação da atividade antioxidante utilizando sistema beta-caroteno/ácido linoléico e método de seqüestro de radicais DPPH. Food Sci Technol. (2006) 26:446–52. doi: 10.1590/S0101-20612006000200031
35. World Health Organization. (2022). Available online at: https://www.who.int/news-room/questions-and-answers/item/radiation-the-ultraviolet-(uv)-index (accessed April 11, 2022).
36. Garcia M, Raes D, Jacobsen SE, Michel T. Agroclimatic constraints for rainfed agriculture in the Bolivian Altiplano. J Arid Environ. (2007) 71:109–21. doi: 10.1016/j.jaridenv.2007.02.005
37. Stromberg PM, Pascual U, Bellon MR. Seed systems and farmers’ seed choices: The case of maize in the Peruvian Amazon. Hum Ecol. (2010) 38:539–53. doi: 10.1007/s10745-010-9333-3
38. Phiri S, Barrios E, Rao IM, Singh BR. Changes in soil organic matter and phosphorus fractions under planted fallows and a crop rotation system on a Colombian volcanic-ash soil. Plant Soil. (2001) 231:211–23. doi: 10.1023/A:1010310300067
39. Meza K, Vanek SJ, Sueldo Y, Olivera E, Ccanto R, Scurrah M, et al. Grass–legume mixtures show potential to increase above-and belowground biomass production for Andean forage-based fallows. Agron. (2022) 12:142. doi: 10.3390/agronomy12010142
40. Rolando JL, Dubeux JCB, Ramirez DA, Ruiz-Moreno M, Turin C, Mares V, et al. Land use effects on soil fertility and nutrient cycling in the Peruvian High-Andean puna grasslands. Soil Sci Soc Am J. (2018) 82:463–74. doi: 10.2136/sssaj2017.09.0309
41. Boots-Haupt L, Brasier K, Saldivar-Menchaca R, Estrada S, Prieto-Garcia J, Jiang J, et al. Exploration of global faba bean germplasm for agronomic and nitrogen fixation traits. Crop Sci. (2022):1–12. doi: 10.1002/csc2.20794
42. Zhang P, Sun J, Li L, Wang X, Li X, Qu J. Effect of soybean and maize rotation on soil microbial community structure. Agron. (2019) 9:42. doi: 10.3390/agronomy9020042
43. Dang P, Li C, Lu C, Zhang M, Huang T, Wan C, et al. Effect of fertilizer management on the soil bacterial community in agroecosystems across the globe. Agric Ecosyst Environ. (2022) 326:107795. doi: 10.1016/j.agee.2021.107795
44. Kresoviæ B, Gajiæ B, Tapanarova A, Dugalic G. How irrigation water affects the yield and nutritional quality of maize (Zea mays L.) in a temperate climate. Pol J Environ Stud. (2018) 27:1123–31. doi: 10.15244/pjoes/76674
45. Musokwa M, Mafongoya PL. Effects of improved pigeonpea fallows on biological and physical soil properties and their relationship with maize yield. Agrofor Syst. (2021) 95:443–57. doi: 10.1007/s10457-021-00598-7
46. Liu J, Chen X, Li D, Xu C, Wu M, Liu M, et al. Variation of soil dissolved organic carbon under long-term different fertilizations and its correlation with maize yields. J Soils Sediments. (2020) 20:2761–70. doi: 10.1007/s11368-020-02631-1
47. Gonzaìlez-Munoz A, Quesille-Villalobos AM, Fuentealba C, Shetty K, Ranilla LG. Potential of Chilean native corn (Zea mays L.) accessions as natural sources of phenolic antioxidants and in vitro bioactivity for hyperglycemia and hypertension management. J Agric Food Chem. (2013) 61:10995–1007. doi: 10.1021/jf403237p
48. Yuan Y, Xiang J, Zheng B, Sun J, Luo D, Li P, et al. Diversity of phenolics including hydroxycinnamic acid amide derivatives, phenolic acids contribute to antioxidant properties of proso millet. LWT. (2022) 154:112611. doi: 10.1016/j.lwt.2021.112611
49. Collison A, Yang L, Dykes L, Murray S, Awika JM. Influence of genetic background on anthocyanin and copigment composition and behavior during thermoalkaline processing of maize. J Agric Food Chem. (2015) 63:5528–38. doi: 10.1021/acs.jafc.5b00798
50. Lux PE, Freiling M, Stuetz W, von Tucher S, Carle R, Steingass CB, et al. (Poly) phenols, carotenoids, and tocochromanols in corn (Zea mays L.) kernels as affected by phosphate fertilization and sowing time. J Agric Food Chem. (2020) 68:612–22. doi: 10.1021/acs.jafc.9b07009
51. Burt AJ, Arnason JT, García-Lara S. Natural variation of hydroxycinnamic acid amides in maize landraces. J. Cereal Sci. (2019) 88:145–9. doi: 10.1016/j.jcs.2019.06.002
52. Roumani M, Besseau S, Gagneul D, Robin C, Larbat R. Phenolamides in plants: An update on their function, regulation, and origin of their biosynthetic enzymes. J Exp Bot. (2021) 72:2334–55. doi: 10.1093/jxb/eraa582
53. Righetti L, Gottwald S, Tortorella S, Spengler B, Bhandari DR. Mass spectrometry imaging disclosed spatial distribution of defense-related metabolites in Triticum spp. Metabolites. (2022) 12:48. doi: 10.3390/metabo12010048
54. Paulsmeyer M, Chatham L, Becker T, West M, West L, Juvik J. Survey of anthocyanin composition and concentration in diverse maize germplasms. J Agric Food Chem. (2017) 65:4341–50. doi: 10.1021/acs.jafc.7b00771
55. Santos MCB, da Silva Lima LR, Nascimento FR, do Nascimento TP, Cameron LC, Ferreira MSL. Metabolomic approach for characterization of phenolic compounds in different wheat genotypes during grain development. Food Res Int. (2019) 124:118–28. doi: 10.1016/j.foodres.2018.08.034
56. Pang Y, Ahmed S, Xu Y, Beta T, Zhu Z, Shao Y, et al. Bound phenolic compounds and antioxidant properties of whole grain and bran of white, red and black rice. Food Chem. (2018) 240:212–21. doi: 10.1016/j.foodchem.2017.07.095
57. Deng J, Xiang Z, Lin C, Zhu Y, Yang K, Liu T, et al. Identification and quantification of free, esterified, and insoluble-bound phenolics in grains of hulless barley varieties and their antioxidant activities. LWT. (2021) 151:112001. doi: 10.1016/j.lwt.2021.112001
58. Zavala-López M, Flint-García S, García-Lara S. Compositional variation in trans-ferulic, p-coumaric, and diferulic acids levels among kernels of modern and traditional maize (Zea mays L.) hybrids. Front Nutr. (2020) 7:600747. doi: 10.3389/fnut.2020.600747
59. Prior RL, Wu X, Schaich K. Standardized methods for the determination of antioxidant capacity and phenolics in foods and dietary supplements. J Agric Food Chem. (2005) 53:4290–302. doi: 10.1021/jf0502698
60. Pico J, Pismag RY, Laudouze M, Martinez MM. Systematic evaluation of the Folin–Ciocalteu and Fast Blue BB reactions during the analysis of total phenolics in legumes, nuts and plant seeds. Food Funct. (2020) 11:9868–80. doi: 10.1039/d0fo01857k
61. De Oliveira KG, Queiroz VAV, de Almeida Carlos L, de Morais Cardoso L, Pinheiro-Sant’Ana HM, Anunciação PC, et al. Effect of the storage time and temperature on phenolic compounds of sorghum grain and flour. Food Chem. (2017) 216:390–8. doi: 10.1016/j.foodchem.2016.08.047
62. Shahidi F, Danielski R, Ikeda C. Phenolic compounds in cereal grains and effects of processing on their composition and bioactivities: A review. J Food Bioact. (2021) 15:39–50. doi: 10.31665/JFB.2021.15281
63. De la Parra C, Saldivar SOS, Liu RH. Effect of processing on the phytochemical profiles and antioxidant activity of corn for production of masa, tortillas, and tortilla chips. J Agric Food Chem. (2007) 55:4177–83. doi: 10.1021/jf063487p
64. Martínez-Martínez R, Vera-Guzmán AM, Chávez-Servia JL, Bolaños ENA, Carrillo-Rodríguez JC, Pérez-Herrera A. Bioactive compounds and antioxidant activities in pigmented maize landraces. Interciencia. (2019) 44:549–56.
65. Trehan S, Singh N, Kaur A. Characteristics of white, yellow, purple corn accessions: phenolic profile, textural, rheological properties and muffin making potential. J Food Sci Technol. (2018) 55:2334–43. doi: 10.1007/s13197-018-3171-5
66. Kuhnen S, Menel Lemos PM, Campestrini LH, Ogliari JB, Dias PF, Maraschin M. Carotenoid and anthocyanin contents of grains of Brazilian maize landraces. J Sci Food Agric. (2011) 91:1548–53. doi: 10.1002/jsfa.4346
67. Hwang T, Ndolo VU, Katundu M, Nyirenda B, Bezner-Kerr R, Arntfield S, et al. Provitamin A potential of landrace orange maize variety (Zea mays L.) grown in different geographical locations of central Malawi. Food Chem. (2016) 196:1315–24. doi: 10.1016/j.foodchem.2015.10.067
68. Uarrota VG, Severino RB, Malinowsky C, de Oliveira SK, Kuhnen S, Yunes RA, et al. Biochemical profile of leaf, silk and grain samples of eight maize landraces (Zea mays L.) cultivated in two low-input agricultural systems. J Food Biochem. (2014) 38:551–62. doi: 10.1111/jfbc.12087
69. Capocchi A, Bottega S, Spano C, Fontanini D. Phytochemicals and antioxidant capacity in four Italian traditional maize (Zea mays L.) varieties. Int J Food Sci Nutr. (2017) 68:515–24. doi: 10.1080/09637486.2016.1261809
70. Ryu SH, Werth L, Nelson S, Scheerens JC, Pratt RC. Variation of kernel anthocyanin and carotenoid pigment content in USA/Mexico borderland land races of maize. Econ Bot. (2013) 67:98–109. doi: 10.1007/s12231-013-9232-9
71. Sun X, Ma L, Lux PE, Wang X, Stuetz W, Frank J, et al. The distribution of phosphorus, carotenoids and tocochromanols in grains of four Chinese maize (Zea mays L.) varieties. Food Chem. (2022) 367:130725. doi: 10.1016/j.foodchem.2021.130725
72. Paznocht L, Kotíková Z, Šulc M, Lachman J, Orsák M, Eliášová M, et al. Free and esterified carotenoids in pigmented wheat, tritordeum and barley grains. Food Chem. (2018) 240:670–8. doi: 10.1016/j.foodchem.2017.07.151
73. Landoni M, Puglisi D, Cassani E, Borlini G, Brunoldi G, Comaschi C, et al. Phlobaphenes modify pericarp thickness in maize and accumulation of the fumonisin mycotoxins. Sci Rep. (2020) 10:1–9. doi: 10.1038/s41598-020-58341-8
74. Rodriguez-Salinas PA, Zavala-Garcia F, Urias-Orona V, Muy-Rangel D, Heredia JB, Niño-Medina G. Chromatic, nutritional and nutraceutical properties of pigmented native maize (Zea mays L.) genotypes from the northeast of Mexico. Arabian J Sci Eng. (2020) 45:95–112. doi: 10.1007/s13369-019-04086-0
75. Harakotr B, Suriharn B, Scott MP, Lertrat K. Genotypic variability in anthocyanins, total phenolics, and antioxidant activity among diverse waxy corn germplasm. Euphytica. (2015) 203:237–48. doi: 10.1007/s10681-014-1240-z
76. Ranilla LG. Bioactive ingredients from corn and lactic acid bacterial biotransformation. In: K Shetty, D Sarkar editors. Functional Foods and Biotechnology. Boca Raton, FL: CRC Press (2019). p. 19–45.
77. Deng GF, Xu XR, Guo YJ, Xia EQ, Li S, Wu S, et al. Determination of antioxidant property and their lipophilic and hydrophilic phenolic contents in cereal grains. J Funct Foods. (2012) 4:906–14. doi: 10.1016/j.jff.2012.06.008
78. Mareèek V, Mikyška A, Hampel D, Èejka P, Neuwirthová J, Malachová A, et al. ABTS and DPPH methods as a tool for studying antioxidant capacity of spring barley and malt. J Cereal Sci. (2017) 73:40–5. doi: 10.1016/j.jcs.2016.11.004
79. Laus MN, Gagliardi A, Soccio M, Flagella Z, Pastore D. Antioxidant activity of free and bound compounds in quinoa (Chenopodium quinoa Willd.) seeds in comparison with durum wheat and emmer. J Food Sci. (2012) 77:C1150–5. doi: 10.1111/j.1750-3841.2012.02923.x
80. Giordano D, Beta T, Vanara F, Blandino M. Influence of agricultural management on phytochemicals of colored corn genotypes (Zea mays L.). part 1: nitrogen fertilization. J Agric Food Chem. (2018) 66:4300–8. doi: 10.1021/acs.jafc.8b00325
81. Dong NQ, Lin HX. Contribution of phenylpropanoid metabolism to plant development and plant–environment interactions. J Integr Plant Biol. (2021) 63:180–209. doi: 10.1111/jipb.13054
82. Oliveira DM, Mota TR, Salatta FV, Sinzker RC, Konèitíková R, Kopeènı D, et al. Cell wall remodeling under salt stress: Insights into changes in polysaccharides, feruloylation, lignification, and phenolic metabolism in maize. Plant Cell Environ. (2020) 43:2172–91. doi: 10.1111/pce.13805
83. Guo X, Xin Z, Yang T, Ma X, Zhang Y, Wang Z, et al. Metabolomics response for drought stress tolerance in Chinese wheat genotypes (Triticum aestivum). Plants. (2020) 9:520. doi: 10.3390/plants9040520
84. De Freitas GM, Thomas J, Liyanage R, Lay JO, Basu S, Ramegowda V, et al. Cold tolerance response mechanisms revealed through comparative analysis of gene and protein expression in multiple rice genotypes. PLoS One. (2019) 14:e0218019. doi: 10.1371/journal.pone.0218019
Keywords: Peruvian maize, phenolic compounds, carotenoids, antioxidant capacity, biodiversity, Zea mays L.
Citation: Fuentes-Cardenas IS, Cuba-Puma R, Marcilla-Truyenque S, Begazo-Gutiérrez H, Zolla G, Fuentealba C, Shetty K and Ranilla LG (2022) Diversity of the Peruvian Andean maize (Zea mays L.) race Cabanita: Polyphenols, carotenoids, in vitro antioxidant capacity, and physical characteristics. Front. Nutr. 9:983208. doi: 10.3389/fnut.2022.983208
Received: 30 June 2022; Accepted: 29 August 2022;
Published: 26 September 2022.
Edited by:
Dejian Huang, National University of Singapore, SingaporeReviewed by:
Yafang Shao, China National Rice Research Institute (CAAS), ChinaJanet Alejandra Gutierrez-Uribe, Monterrey Institute of Technology and Higher Education (ITESM), Mexico
Copyright © 2022 Fuentes-Cardenas, Cuba-Puma, Marcilla-Truyenque, Begazo-Gutiérrez, Zolla, Fuentealba, Shetty and Ranilla. This is an open-access article distributed under the terms of the Creative Commons Attribution License (CC BY). The use, distribution or reproduction in other forums is permitted, provided the original author(s) and the copyright owner(s) are credited and that the original publication in this journal is cited, in accordance with accepted academic practice. No use, distribution or reproduction is permitted which does not comply with these terms.
*Correspondence: Lena Gálvez Ranilla, bGdhbHZlekB1Y3NtLmVkdS5wZQ==