- 1Institute of Human Genetics, University Hospital Jena, Friedrich Schiller University, Jena, Germany
- 2Department of Psychiatry, Douglas Mental Health University Institute, McGill University, Montreal, QC, Canada
- 3Institute for Biochemistry and Biophysics, Friedrich-Schiller-University Jena, Jena, Germany
- 4Laboratory of Physiological Chemistry, de Duve Institute, Brussels, Belgium
- 5Clinic for Psychiatry and Psychotherapy, University Hospital of Otto-von-Guericke-Universität Magdeburg, Magdeburg, Germany
- 6Department of Psychiatry and Psychotherapy, Jena University Hospital, Jena, Germany
GDP-Mannose Pyrophosphorylase B (GMPPB) is a key enzyme for glycosylation. Previous studies suggested a dysregulation of GMPBB and mannose in depression. Evidence, however, was sporadic and interventions to reverse these changes are unknown. Here, we show that GMPPB protein, but not RNA abundance is increased in the postmortem prefrontal cortex (PFC) of depressed patients and the chronic variable stress (CVS) mouse-model. This is accompanied by higher plasma mannose levels. Importantly, a single dose of intraperitoneally administered vitamin B12, which has previously been shown to rapidly reverse behavioral symptoms and molecular signatures of chronic stress in mice, normalized GMPPB plasma mannose levels and elevated GDP-mannose abundance. In summary, these data underline metabolic dysregulation in chronic stress and depression and provide further support for rapid effects of vitamin B12 on chronic stress.
Introduction
GDP-Mannose Pyrophosphorylase B (GMPPB) is a key enzyme in the glycosylation pathway, which catalyzes the synthesis of GDP-mannose from mannose-1-phosphate and guanosine triphosphate (1). Its activity is regulated by its enzymatically inactive homologue GMPPA, which acts as an allosteric inhibitor of GMPPB. Mutations in GMPPB and GMPPA result in complex congenital disorders of glycosylation (2–4). Recently, a protein-wide association study on depressed patients found that GMPPB protein levels are increased postmortem in the prefrontal cortex (PFC) of depressed patients (5). Accordingly, receptors for neurotransmitters that are essential to stress and depression in the PFC are regulated by glycosylation, including the NMDA receptor (6–8) and serotonin receptors (9, 10). In congenital disorders of glycosylation, depressive symptoms are relatively frequent (11, 12).
The substrate for GMPPB, mannose-1-phosphate, is reduced in the PFC in a rat model of chronic stress and depression (13). A high dose of intraperitoneally administered mannose may contribute to depression-like states in mice (14). However, it is, at least to our knowledge, unknown, whether mannose levels are intrinsically affected by depression and whether this correlates with GMPPB abundance.
We recently observed that vitamin B12 rapidly reverses depression-associated phenotypes in mice (15). Vitamin B12 (cobalamin) is an essential nutrient, which can only be synthesized by bacteria. Vitamin B12 is a cofactor in the one-carbon metabolism, which provides methyl donors, e.g., for the methylation of DNA, histones or other proteins. 15% of the human population suffers from vitamin B12 deficiency and affected individuals have an increased risk of suffering from depression (16–18). Importantly, there is also evidence that vitamin B12-supplementation in non-deficient populations may reduce depression risk (19, 20). We have previously shown that a single acute dose of vitamin B12 reduced depressive-like behavior and stress-linked biomarkers in mice in the chronic mild stress-model (15).
Here we confirm increased GMPPB protein levels in human PFC postmortem tissue of depressed patients. Furthermore, we observed increased mannose levels in plasma of depressed patients. Similar changes were found in the chronic variable stress (CVS) mouse-model, which is superior in modeling human molecular signatures of depression (21). Additionally, plasma GDP-mannose levels were increased by CVS in mice. Interestingly, an acute dose of vitamin B12 was sufficient to reverse GMPPB and plasma mannose levels. In summary, this study underlines an association between GMPPB and depression.
Methods
Animals and licenses
Mice were housed in accordance with the ethical guidelines of the Thüringer Landesamt für Verbraucherschutz (TLV). Experiments were conducted under Animal license UKJ-18-037 (Germany), which are complying to the EU Directive 2010/63/EU guidelines for animal experiments. C57Bl/6J mice were bred in the animal facility (FZL) of Jena University Hospital, Germany. Animals received standard chow (LASQCdiet Rod16-R, LASvendi GMBH, Soest, Germany), which contains 50 mg/kg chow vitamin B12. Mice were at least 10 weeks of age. Mice were housed in a 14L:10D light-cycle. For brain analysis, mice were sacrificed and PFC tissue was immediately frozen on dry ice and stored at −80°C until further use.
Drugs and chemicals
Mice were intraperitoneally (i.p.) injected with 2.7 mg/kg vitamin B12 (cyanocobalamin, #V6629, Sigma-Aldrich, Burlington, MA, USA) or saline at an injection volume of 10 ml/kg body weight and tested 24 h later as described in Engmann et al. (22).
RNA purification and quantification
RNA was purified by resuspension in Trizol and chloroform-precipitation. RNA was washed in isopropanol and 75% ethanol. After cDNA-conversion with a GoScript™ Reverse Transcriptase kit (#A5001, Promega, Madison, WI, USA), quantitative realtime-PCR was performed on a Bio-rad CFX96 Real-time system. Quantitative PCR results were processed as described (23). Primer sequences were: GMPPB/Gmppb, Fvd: 5′-CCT CACTGGCATGTGC CTC-3′, Rev: 5′-GACTTGTGGGGC AGCACG-3′; GAPDH (22), Fvd: 5′-TGGGCAGCCGTTAGG AAAG-3′, Rev: 5′-AGTTAAAAGCAGCCCTGGTGA-3′; Gapdh (22), Fvd: 5′-AACTTTGGCATTGTGGAAGG-3′, Rev: 5′-ACACATTGGGGGTAGGAACA-3′.
Western blot
Tissue lysates were prepared with the Potter S tissue homogenizer (Sartorius, #S14492) in TBS-buffer (20 mM Tris, 150 mM NaCl, 1% (v/v) TritonX-100, complete protease inhibitor and complete phosphatase inhibitor (#04693124001, Sigma-Aldrich, Burlington, MA, USA). After sonication, homogenates were spun down at 16,900 g to remove nuclei and insoluble debris. The supernatant was stored at −80°C until further use. Proteins were denatured at 90°C for 5 min in Laemmli buffer (4X Laemmli buffer: 50% glycerol, 5% SDS, 0.25%1.5M Tris pH 6.8, 30% β-mercaptoethanol, 0.001% bromophenol blue, ddH2O). After separation by SDS-PAGE (8% polyacrylamide glycine gels, run for 1.5 h at 80 V) proteins were transferred onto 0.45 μm PVDF membranes (#10600023, GE Healthcare) at 290 mA for 100 min. Membranes were blocked in 2% BSA for 1 h at RT and incubated with primary antibodies at appropriate dilutions in tris-buffered saline supplemented with 0.1% Tween-20 (TBS-T) overnight at 4°C. The following primary antibodies were used: rabbit anti-GMPPA (#15517-1-AP, Proteintech, Rosemont, IL, USA) 1:500, rabbit anti-GMPPB (#15094-1-AP, Proteintech, Rosemont, IL, USA) 1:500, rabbit anti-GAPDH (#10494-1-AP, Proteintech, Rosemont, IL, USA) 1:1,000, self-made mouse anti-oligomannose antibody (6–9 terminal mannose residues) 1:50 [gift of Rüdiger Horstkorte, Halle (24)]. Membranes were washed in TBS-T and primary antibodies were detected with horseradish peroxidase-conjugated secondary antibodies in an appropriate dilution. Following secondary antibodies were used: donkey anti-rabbit IgG-HRP (#NA 9340V, Amersham, Buckinghamshire, UK) 1:4,000 and goat anti-mouse IgM # (31440, Thermo Fisher Scientific, Waltham, MA, USA) 1:4,000.
Measurement of plasma sugar concentrations
Human plasma samples were obtained in agreement with the Ethics committee of Magdeburg University Hospital, Germany (110/07). Patients did not receive psychopharmacological substances other than benzodiazepines for at least 6 weeks prior to testing. Blood plasma was collected by centrifuging blood immediately after collection for 10 min at 3,000 rpm (Hettich centrifuge EBA 2, type 2002). The supernatant consisting of plasma was aliquoted into DNA LoBind tubes (Eppendorf, Hamburg, Germany) and stored at −80°C. Samples from depressed patients and age-matched controls were collected at day 0 (T0) and 6 weeks after the beginning of treatment (T6). Treatment differed between patients and was individually tailored to their needs. As no difference was found in plasma sugar levels for patients between days T0 and T6 (data not shown), the average of both time points was calculated for each participant to reduce variability. Mouse blood was obtained from unfasted animals and incubated on ice for 15 min. Samples were centrifuged for 10 min at 4°C and 4,000 g. Sugars were measured in the supernatant with the D-mannose, D-fructose, D-glucose kit following manufacturer’s instructions (#K-MANGL, Megazyme, Wicklow, Ireland).
GDP-mannose measurements
Plasma samples were obtained as described above and stored at −80°C. For sample preparations, 10 μL of plasma was added to 190 μL of Methanol/water/Chloroform mixture (8:1:1), followed by a vigorous shacking and then centrifuged at 13,200 rpm during 15 min at 4°C. Supernatant was dried by speed Vacuum and samples were re-suspended in 70 μL of methanol 50%, and finally were analyzed by LC/MS as described previously (2). Tissue quality control showed no differences in GMP, GDP, or GTP levels between treatments (data not shown).
Chronic variable stress induction
The CVS-protocol was performed as described (21). In brief, mice received 21 days of stress with one of three stressors presented in a semi-random order, where the same stressor does not occur on two consecutive days. The following stressors were used: 1 h of tube restraint, tail suspension or 100 mild electric random foot shocks. If only female experimenters were present, a used male t-shirt was wrapped in clean protective clothing from the animal unit and placed into the experimental room in order to avoid variability due to sex-specific scents of the scientists (3). All experiments were conducted in the light phase of the light-cycle to allow comparability with previous experiments (15). For CVS-groups, vitamin B12 was injected at the last day of CVS (day 21), just prior to the stressor.
Postmortem brain samples
Samples were generously provided by the Douglas-Bell Canada Brain Bank. Experiments were conducted in agreement with the Ethics committee of Jena University Hospital, Germany (Reg.-Nr. 2020-1862-Material) and Douglas Institute REB Approval #04/21.
Statistics
Statistical analysis was performed in GraphPrism. Two-tailed Student’s t-test was used for comparison of two groups. Two-way ANOVA with Bonferroni post-hoc test was used, when two factors were varied. Outliers were removed when data points were more than two standard deviations away from the average.
Results
GDP-mannose pyrophosphorylase B and plasma mannose are increased in depressed patients and in a mouse model of chronic stress
We obtained PFC postmortem tissue from depressed patients to assess whether altered GMPPB levels observed by Wingo et al. (5) can be reproduced and whether this was associated with altered mannosylation (Figure 1A). Additionally, we obtained samples from the CVS mouse-model (Figure 1B). This model is the gold standard in mimicking molecular and behavioral changes linked to depression and is well suited for functional and interventional studies (21, 25).
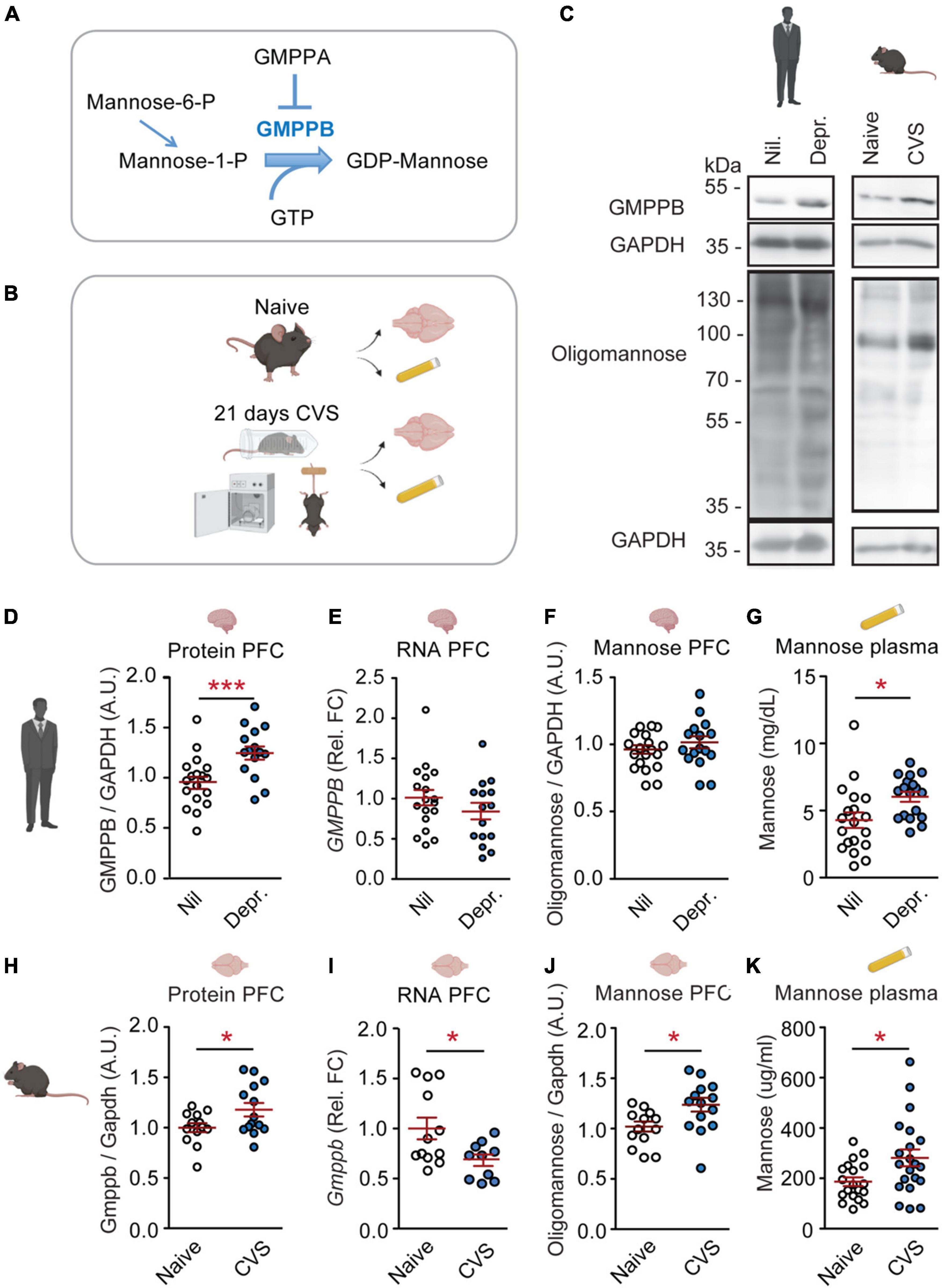
Figure 1. GMPPB and plasma mannose are increased by chronic stress and depression. (A) Cartoon illustrating the regulation of GDP-mannose production. (B) Experimental setup for CVS-experiment. (C) Representative western blots. (D–K) Statistics: Student’s t-test. (D–G) Results from human cohorts. (D–F,H–J) Prefrontal cortex. (G,K) Plasma. (D) Increased GMPPB protein-levels in depressed patients (Depr.) vs. controls (Nil); n = 15–18 per group; t31 = 3.32, **P < 0.01. (E) No association between depression and GMPPB RNA-levels; n = 15–18 per group; t31 = 1.22, P = 0.23. (F) No association between depression and oligomannose-levels. n = 15–19 per group; t32 = 0.59, P = 0.56. (G) Increased plasma mannose in depressed patients; n = 18–19 per group; t35 = 2.51, *P < 0.05. (H–K) Results from the CVS mouse-model. (H) Increased GMPPB protein-levels in stressed mice; n = 13–14 per group; t25 = 2.18, *P < 0.05. (I) Reduced Gmppb-RNA levels in CVS-group; n = 10–12 per group; t20 = 2.13, *P < 0.05. (J) Increased oligomannose-levels in stressed mice; n = 13–14 per group; t25 = 2.53, *P < 0.05. (K) Increased plasma mannose in CVS-group. n = 19–21 per group; t38 = 2.44, *P < 0.05. (D–K) Individual data points are plotted and means ± s.e.m. are shown. A.U., Arbitrary units; Rel. FC, Relative fold change. Illustrations were generated with biorender.com.
Increased GMPPB protein levels in postmortem PFC-tissue from depressed patients were confirmed in our cohort (Figures 1C,D). The change in GMPPB protein abundance was not accompanied by increased GMPPB transcripts, suggesting a regulation of the protein itself (Figure 1E). As GMPPB is a mediator of mannosylation, oligomannose levels in the PFC and plasma mannose levels were measured as well. While PFC oligomannose levels were not affected in depressed patients (Figure 1F), plasma mannose levels were increased (Figure 1G and Supplementary Figure 1).
In the CVS mouse-model, increased GMPPB was observed as well (Figure 1H). Here, too, Gmppb RNA-levels did not match the observed increase in protein abundance (Figure 1I). In the mouse model, both, PFC oligomannose and plasma mannose were increased (Figures 1J,K).
These data suggest that chronic stress and depression are indeed linked to altered PFC GMPPB and mannose metabolism. GMPPB and oligomannose changes in the CVS-model occurred in the PFC. No changes were observed in the hippocampus (Supplementary Figure 2). Moreover, GDP-mannose levels were significantly increased in murine plasma samples (Supplementary Figure 3). Mannose can be converted from other carbohydrates such as fructose and glucose (Supplementary Figure 4). Hence, plasma fructose and glucose levels were assessed in mice and human cohorts as well. While fructose was not affected in depressed patients or by CVS, glucose amounts were significantly reduced in depressed patients (Supplementary Figure 4).
Vitamin B12 rapidly reverses GDP-mannose pyrophosphorylase B- and plasma mannose-levels in chronically stressed mice
Previously, we have observed that a single dose of vitamin B12 at the end of a chronic stress paradigm can ameliorate symptoms associated with depression (15).
We observed that an acute dose of vitamin B12 reduced GMPPB protein-amounts in stressed mice (Figures 2A–C). Gmppb RNA-levels and PFC oligomannose were not affected (Figures 2D,E). Additionally, vitamin B12 decreased plasma mannose levels (Figure 2F) without affecting plasma fructose and glucose (Supplementary Figure 4). Moreover, plasma GDP-mannose levels were not altered by vitamin B12 (Supplementary Figure 3). These data suggest that in mice, a single dose of vitamin B12 can, at least in part, counteract chronic stress-induced changes in mannose metabolism.
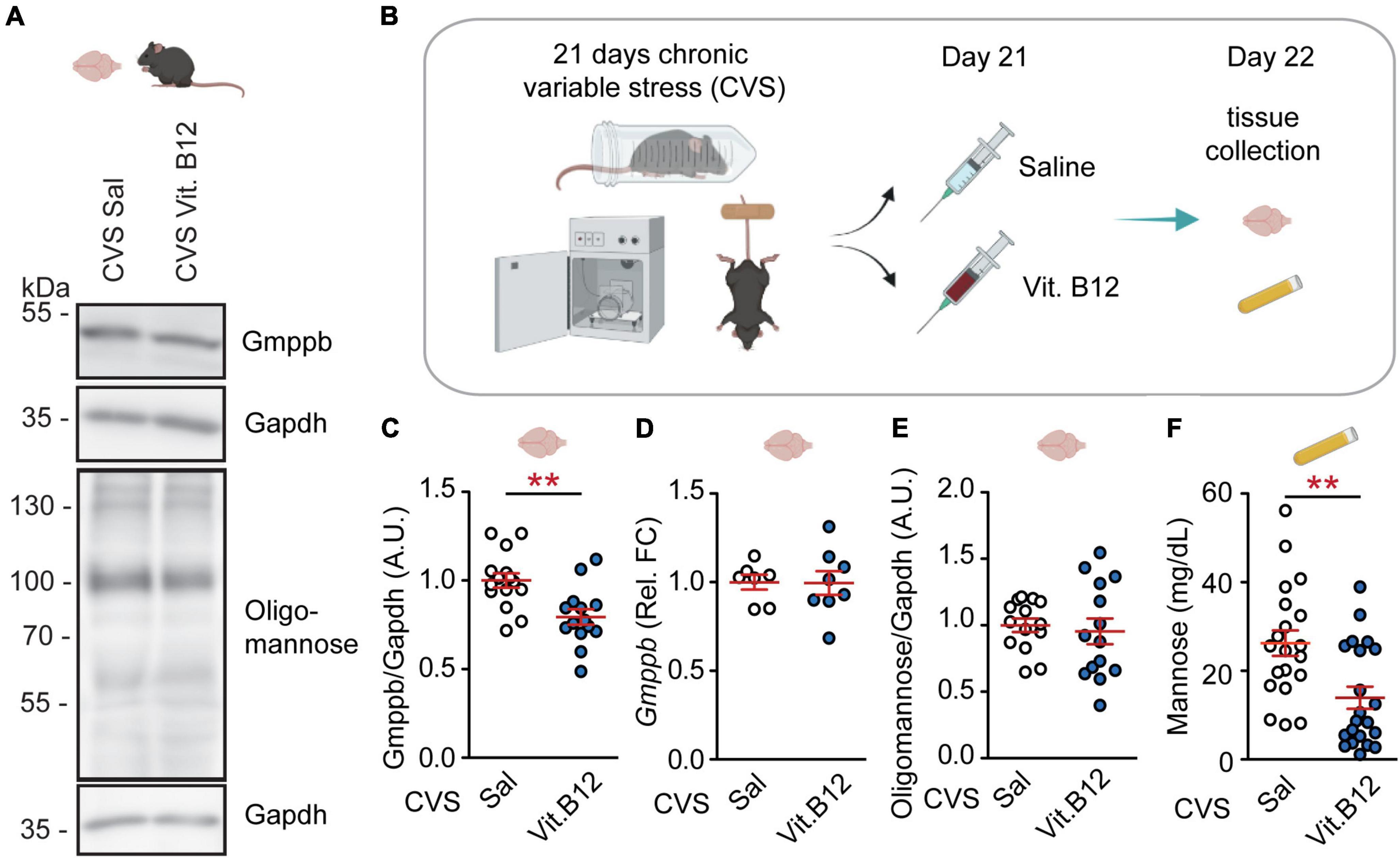
Figure 2. Vitamin B12 rapidly reverses GMPPB levels and plasma mannose in stressed mice. (A) Representative western blots. (B) Experimental setup for Vitamin B12-experiment. (C–F) Statistics: Student’s t-test. (C) Vitamin B12 reduces GMPPB protein-levels in the PFC of stressed mice; n = 14–15 per group; t27 = 3.43, **P < 0.01. (D) No effect of vitamin B12 on Gmppb transcription; n = 7–8 per group; t13 = 0.05, P = 0.96. (E) No effect of vitamin B12 on oligomannose abundance in the PFC; n = 14 per group; t26 = 0.42, P = 0.68. (F) Vitamin B12 reduces plasma mannose in CVS-treated mice; n = 20–21 per group; t39 = 3.23, **P < 0.01. (C–F) Individual data points are plotted and means ± s.e.m. are shown. A.U., Arbitrary units. Illustrations were generated with biorender.com.
GMPPA, an allosteric inhibitor of GDP-mannose pyrophosphorylase B, is affected by depression, but not chronic stress or vitamin B12
GMPPA is the allosteric feedback inhibitor of GMPPB (Figure 1A). GMPPA protein levels were increased in depressed patients (Figures 3A,D). However, they were not affected by chronic stress in untreated mice or by vitamin B12 in stressed mice (Figures 3B–F and Supplementary Figure 5). Hence, the observed GMPPB-changes appear to be selective in mice. In human depression cohorts, GMPPB-associated elevations in mannosylation might be counteracted by an increase in GMPPA abundance as a compensatory mechanism.
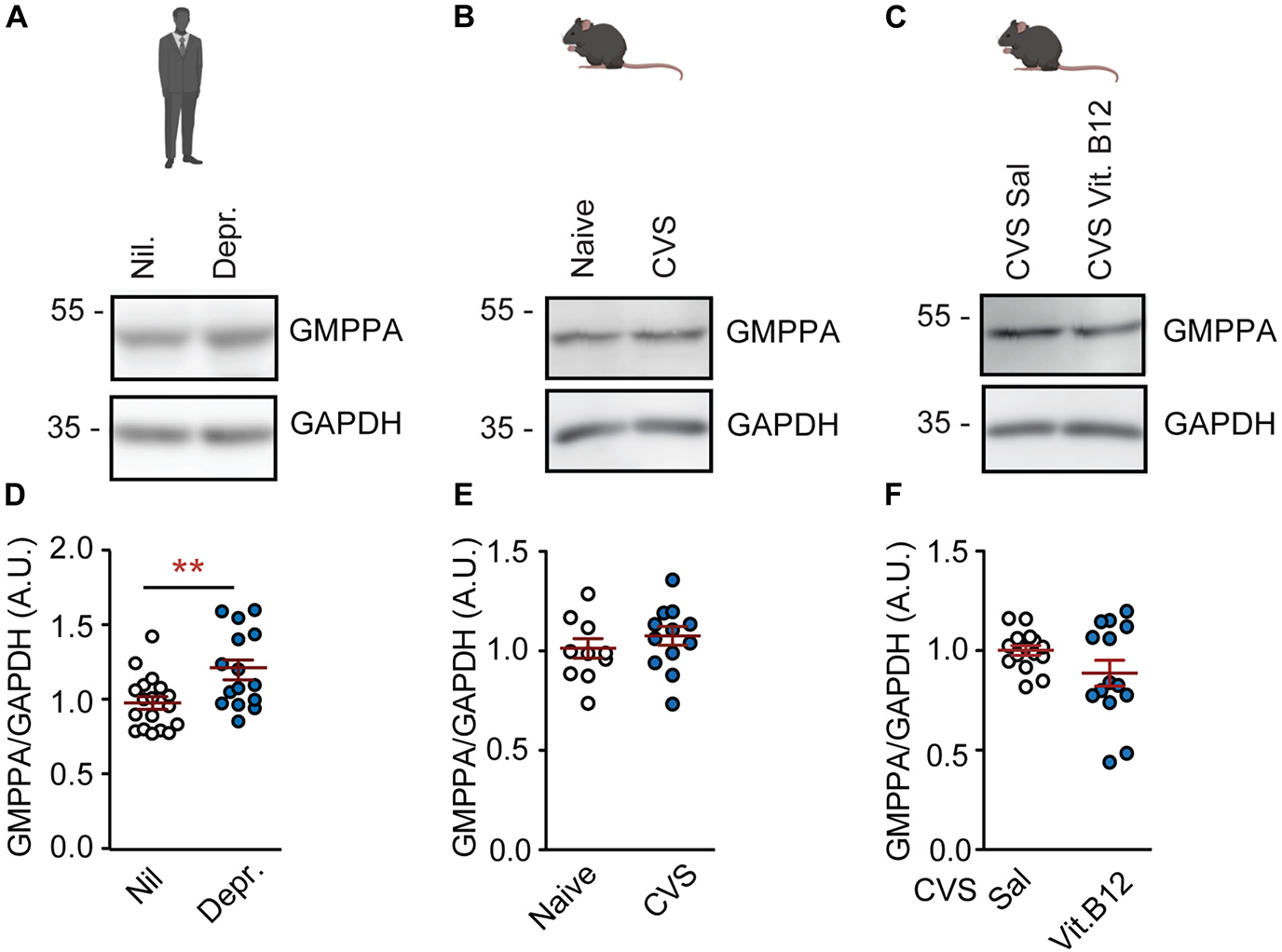
Figure 3. GMPPA levels are not associated with depression or chronic stress in the PFC. (A) Representative western blots for GMPPA and housekeeping gene, GAPDH on prefrontal cortex tissue. (A) Human cohorts. (B) Naïve mice and CVS model. (C) Vitamin B12 treatment in stressed mice. (D–F) Statistics: Student’s t-test. (D) Increased GMPPA abundance in depression; n = 15–18 per group; t31 = 2.91, **P < 0.01. (E) GMPPA is not altered by chronic stress; n = 13–15 per group; t26 = 1.10, P = 0.28. (F) Vitamin B12 does not affect GMPPA levels; n = 13–15 per group; t26 = 1.95, P = 0.06. (D–F) Individual data points are plotted and means ± s.e.m. are shown. A.U., Arbitrary units. Illustrations were generated with biorender.com.
Discussion
Here we confirm a previous observation that altered GMPPB levels are increased in the postmortem PFC of depressed patients. We found similar changes in a mouse model of chronic stress and depression. Changes in mice were specific to the PFC and were not accompanied by altered GMPPA-levels. Notably, in a brain proteome-wide association study, Wingo et al. found not only GMPPB levels to be altered, but also beta 3-glucosyltransferase (B3GALTL). This indicates that various enzymes in the glycosylation process might be changed upon depression. We further observed increased plasma mannose-levels in patients suffering from depression. Unfortunately, it was not possible to obtain correlative data as the samples had to be taken from different alive and postmortem cohorts. Furthermore, we found that vitamin B12 can rapidly decrease GMPPB and plasma mannose in stressed mice.
While the CVS-model reflected main observations from human depressed patients, it differed in several details. For instance, Gmppb transcript-levels were reduced in stressed mice but not in depressed patients. Stressed mice, but not depressed patients, showed altered protein-bound oligo-mannose residues in the PFC. Plasma glucose-levels were decreased in depressed patients but not in stressed mice. In humans, but not mice, GMPPA levels were altered. These differences need to be considered when exploring functional links between GMPPB metabolism and depression. Causes may be species-differences or secondary biases due to life style-changes in depressed patients or sampling methods (e.g., altered dietary choices, fasting prior to testing in humans vs. mice, longer postmortem intervals in human samples). Furthermore, depressed patients were under medication (benzodiazepines for plasma cohort, various pharmaceuticals for the postmortem cohort), which may affect metabolic markers as well.
Based on the availability of samples, our data sets were skewed toward males and Caucasians. In order to ensure a wider applicability of findings, such biases should be avoided whenever possible in the future.
The discrepancy between regulation of Gmppb transcripts vs. proteins suggest a regulation on a protein level, e.g., via posttranslational modifications or proteasomal degradation. Furthermore, a clear link between vitamin B12 and carbohydrate mechanisms has not been explored. Being a regulator of methyl donors, it is conceivable that vitamin B12 affects the methylation of GMPPB or its’ regulators, for instance on it’s R357 residue (26). This possibility should be further investigated.
Currently it is unclear whether increased GMPPB abundance in the PFC will lead to increased plasma mannose levels, e.g., via degradation of glycoproteins. It is conceivable that, despite the observed brain-region specific alterations, GMPPB abundance may be altered in other tissues such as liver (27). We recently showed that hyperglycosylation of proteins is correlated with increased plasma mannose levels (2). Moreover, we showed that increased GMPPB levels correlate with protein hypermannosylation and enhanced plasma mannose levels (24). Altered glycosylation may affect protein stability and conformation, protein interactions and adhesion, as well as protein activity and localization (28).
In depressed patients and CVS mice, we found plasma mannose concentrations to be strongly increased, which may reflect increased release from glycans or increased generation of mannose from glucose or fructose. Although only approximately 2% of mannose entering the cell is used for glycosylation (29), the higher systemic mannose levels may contribute to the larger pool of GDP-mannose/hypermannosylated proteins in CVS mice and patients and thus hyperglycosylation.
In agreement with this, we detected increased plasma GDP-mannose levels in stressed mice. Plasma GDP-mannose levels are probably derived from blood cells or dead peripheral cells and not from an efflux from cells as reported for blood mannose (30). Plasma GDP-mannose levels were not affected by vitamin B12 in stressed mice. This may be due to previously observed tissue-specific effects of vitamin B12. Future studies might address blood glycoproteins and their regulation through vitamin B12 as well.
It has been shown that reduced GMPPB abundance leads to decreased GDP-mannose levels and thus affects neuronal and muscle development (4, 31, 32). For example, motor neurons were shortened (4), and an early marker of pan-neuronal cells was remarkably decreased in GMPPB knockdown zebrafish (33). Notably, GDP-mannose supplementation restored GDP-mannose levels, protein mannosylation and thus muscle and neuronal defects (4). Another study showed that GDP-fucose supplementation in a GDP-mannose 4,6 dehydratase mutant zebrafish where fucosylation was decreased restored protein fucosylation and the neuronal phenotype (34). However, it has been shown that extremely elevated GDP-mannose levels affect neuron morphology and development (4). Thus, normal neuron function likely depends on a balanced GDP-mannose homeostatis mediated by GMPPB and GMPPA.
A possible link between GMPPB and plasma mannose levels may be addressed using GMPPB mutant mice. Furthermore, the impact of a mannose-enriched or mannose-depleted diet on symptoms of chronic stress and depression could be investigated.
This study further supports the rapid stress-ameliorating effects of vitamin B12. The current findings add a metabolic and possible protein-regulatory level to the previously observed changes in behavioral and transcriptional markers, highlighting a multidimensional impact of vitamin B12. To date, the vitamin B12 induced stress reversal has been observed in two different mouse models of chronic stress.
Hence, vitamin B12 should be tested as a rapid dietary intervention to treat symptoms associated with chronic stress and depression in human cohorts. Blood samples may be taken to investigate an impact on plasma mannose in addition to mood-related measures. This would allow in patient-correlations and may perhaps provide a therapeutic approach using a widely available, affordable, well-tolerated and rapid acting molecule to improve symptoms of stress and depression.
Despite the missing functional link between vitamin B12 and mannose metabolism, this study provides several novel insights: (1) Systemic mannose levels are altered in depressed patients and in a mouse model. The fact that vitamin B12 rapidly reverses plasma mannose changes in stressed mice demonstrates the dynamic nature of this marker. (2) Vitamin B12 rapidly normalizes metabolic correlates of depression in a mouse model. Together with previous behavioral and molecular data, this study further encourages testing of vitamin B12 as a fast-acting intervention to chronic stress.
Data availability statement
The raw data supporting the conclusions of this article will be made available by the authors, without undue reservation. Raw data western blot images are shown in Supplementary Figure 5.
Ethics statement
The studies involving human participants were reviewed and approved by the 2020-1862-Material. The patients/participants provided their written informed consent to participate in this study. The animal study was reviewed and approved by the UKJ-18-037. Human plasma samples were obtained in agreement with the Ethics committee of Magdeburg University Hospital, Germany (110/07).
Author contributions
OE provided the study design and ideas, conducted chronic-stress and RNA-experiments, and wrote the manuscript. PF performed the western blots and sugar measurements in plasma and performed the statistical analyses. SC performed the qPCR. GT and JS provided the human samples. TK analyzed the GDP-mannose levels. CH and MW provided the financial support and mentorship. All authors contributed to the article and approved the submitted version.
Funding
This study was supported by the Advanced Medical Scientist Award from the Interdisciplinary Center for Clinical Research (IZKF) at the Jena University Hospital, Germany (#973685). Furthermore, this project was made possible by funding from the Carl-Zeiss-Foundation (IMPULS #P2019-01-0006). CH was supported by the DFG grant HU 800/15-1 and RTG ProMoage 2155.
Conflict of interest
The authors declare that the research was conducted in the absence of any commercial or financial relationships that could be construed as a potential conflict of interest.
Publisher’s note
All claims expressed in this article are solely those of the authors and do not necessarily represent those of their affiliated organizations, or those of the publisher, the editors and the reviewers. Any product that may be evaluated in this article, or claim that may be made by its manufacturer, is not guaranteed or endorsed by the publisher.
Supplementary material
The Supplementary Material for this article can be found online at: https://www.frontiersin.org/articles/10.3389/fnut.2022.981511/full#supplementary-material
Supplementary Figure 1 | Demography of human cohorts. (A) Postmortem prefrontal cortex tissue. (B) Plasma samples. (C) Plasma-mannose levels (from Figure 1) analyzed by sex; n = 9 per group; 2-way ANOVA: effect of depr.: F(1, 32) = 13.68, P = 0.0008; effect of sex: F(1, 32) = 0.02, P = 0.88; no interaction: F(1, 32) = 2.14, P = 0.15; Bonferroni post hoc test: effect of depr. within females: **P < 0.01; all other comparisons: P > 0.05. (D) Legend with abbreviations. (C) Individual data points are plotted and means ± s.e.m. are shown. Illustrations were generated with biorender.com.
Supplementary Figure 2 | Hippocampal GMPPB and oligomannose are not affected by chronic stress or depression. (A) Representative western blots on hippocampal tissue. (B–D) Statistics: Student’s t-test. (B) No difference in GMPPB-levels; n = 9 per group; t16 = 1.10, P = 0.29. (C) No difference in GMPPA-levels; n = 10 per group; t18 = 0.69, P = 0.50. (D) No difference in oligomannose-levels; n = 9–10 per group; t17 = 0.18, P = 0.86. (B–D) Individual data points are plotted and means ± s.e.m. are shown. A.U., Arbitrary units. Illustrations were generated with biorender.com.
Supplementary Figure 3 | GDP-Mannose is increased in plasma of chronically stressed mice but not altered by vitamin B12. (A,B) Statistics: Student’s t-test. (A) GDP-mannose is affected by CVS. n = 9–10 per group; t17 = 2.95, **P < 0.01. (A) GDP-mannose is not affected by vitamin B12 in the CVS group. n = 9–10 per group; t17 = 1.21, P = 0.24. Individual data points are plotted and means ± s.e.m. are shown. A.U., Arbitrary units.
Supplementary Figure 4 | Fructose and glucose levels in chronic stress and depression. (A) Overview of conversion pathway between mannose, fructose and glucose. (B–G) Statistics: Student’s t-test. (B,C) Plasma sugar levels in human cohorts. (B) Fructose-levels are not significantly altered in depressed patients; n = 19–20 per group; t37 = 1.86, P = 0.07. (C) Glucose levels are reduced in depressed patients. n = 19 per group; t36 = 3.80, ***P < 0.001. (D,E) Plasma sugar levels in mice. (D) Fructose levels are not affected by CVS; n = 19–20 per group; t37 = 1.15, P = 0.26. (E) Glucose levels are not altered by CVS; n = 19–21 per group; t38 = 1.47, P = 0.15. (F) Vitamin B12 does not affect plasma fructose levels; n = 20–21 per group; t39 = 0.13, P = 0.90. (G) Plasma glucose levels are not affected by vitamin B12 in stressed mice; n = 20 per group; t38 = 1.96, P = 0.06. (B–G) Individual data points are plotted and means ± s.e.m. are shown. Illustrations were generated with biorender.com.
Supplementary Figure 5 | Full length western blots.
References
1. Ning B, Elbein AD. Cloning, expression and characterization of the pig liver GDP-mannose pyrophosphorylase: evidence that GDP-mannose and GDP-Glc pyrophosphorylases are different proteins. Eur J Biochem. (2000) 267:6866–74. doi: 10.1046/j.1432-1033.2000.01781.x
2. Franzka P, Henze H, Jung MJ, Schüler SC, Mittag S, Biskup K, et al. GMPPA defects cause a neuromuscular disorder with α-dystroglycan hyperglycosylation. J Clin Invest. (2021) 131:e139076. doi: 10.1172/JCI139076
3. Sorge RE, Martin LJ, Isbester KA, Sotocinal SG, Rosen S, Tuttle AH, et al. Olfactory exposure to males, including men, causes stress and related analgesia in rodents. Nat Methods. (2014) 11:629–32. doi: 10.1038/nmeth.2935
4. Zheng L, Liu Z, Wang Y, Yang F, Wang J, Huang W, et al. Cryo-EM structures of human GMPPA–GMPPB complex reveal how cells maintain GDP-mannose homeostasis. Nat Struct Mol Biol. (2021) 28:1–12. doi: 10.1038/s41594-021-00591-9
5. Wingo TS, Liu Y, Gerasimov ES, Gockley J, Logsdon BA, Duong DM, et al. Brain proteome-wide association study implicates novel proteins in depression pathogenesis. Nat Neurosci. (2021) 24:810–7. doi: 10.1038/s41593-021-00832-6
6. Kaniakova M, Lichnerova K, Skrenkova K, Vyklicky L, Horak M. Biochemical and electrophysiological characterization of N-glycans on NMDA receptor subunits. J Neurochem. (2016) 138:546–56. doi: 10.1111/jnc.13679
7. Skrenkova K, Lee S, Lichnerova K, Kaniakova M, Hansikova H, Zapotocky M, et al. N-glycosylation regulates the trafficking and surface mobility of GluN3A-containing NMDA receptors. Front Mol Neurosci. (2018) 11:188. doi: 10.3389/fnmol.2018.00188
8. Tucholski J, Simmons MS, Pinner AL, McMillan LD, Haroutunian V, Meador-Woodruff JH. N-linked glycosylation of cortical N-methyl-D-aspartate and kainate receptor subunits in schizophrenia. Neuroreport. (2013) 24:688–91. doi: 10.1097/WNR.0b013e328363bd8a
9. Monk SA, Williams JM, Hope AG, Barnes NM. Identification and importance of N-glycosylation of the human 5-hydroxytryptamine3A receptor subunit. Biochem Pharmacol. (2004) 68:1787–96. doi: 10.1016/j.bcp.2004.06.034
10. Carrel D, Hamon M, Darmon M. Role of the C-terminal di-leucine motif of 5-HT1A and 5-HT1B serotonin receptors in plasma membrane targeting. J Cell Sci. (2006) 119:4276–84. doi: 10.1242/jcs.03189
11. Gardeitchik T, Wyckmans J, Morava E. Complex phenotypes in inborn errors of metabolism: overlapping presentations in congenital disorders of glycosylation and mitochondrial disorders. Pediatr Clin North Am. (2018) 65:375–88. doi: 10.1016/j.pcl.2017.11.012
12. Morava E, Tiemes V, Thiel C, Seta N, de Lonlay P, de Klerk H, et al. ALG6-CDG: a recognizable phenotype with epilepsy, proximal muscle weakness, ataxia and behavioral and limb anomalies. J Inherit Metab Dis. (2016) 39:713–23.
13. Duan L, Fan R, Li T, Yang Z, Hu E, Yu Z, et al. Metabolomics analysis of the prefrontal cortex in a rat chronic unpredictable mild stress model of depression. Front Psychiatry. (2022) 13:815211. doi: 10.3389/fpsyt.2022.815211
14. Xu K, Wang M, Zhou W, Pu J, Wang H, Xie P. Chronic d-ribose and d-mannose overload induce depressive/anxiety-like behavior and spatial memory impairment in mice. Transl Psychiatry. (2021) 11:90. doi: 10.1038/s41398-020-01126-4
15. Trautmann C, Bock A, Urbach A, Hübner A, Engmann O. Acute vitamin B12 supplementation evokes antidepressant response and alters Ntrk-2. Neuropharmacology. (2020) 171:108112. doi: 10.1016/j.neuropharm.2020.108112
16. Penninx BW, Guralnik JM, Ferrucci L, Fried LP, Allen RH, Stabler SP. Vitamin B(12) deficiency and depression in physically disabled older women: epidemiologic evidence from the Women’s Health and Aging Study. Am J Psychiatry. (2000) 157:715–21. doi: 10.1176/appi.ajp.157.5.715
17. Tiemeier H, Ruud van Tuijl H, Hofman A, Meijer J, Kiliaan AJ, Breteler MMB. Vitamin B12, folate, and homocysteine in depression: the Rotterdam study. Am J Psychiatry. (2002) 159:2099–101.
18. Moorthy D, Peter I, Scott TM, Parnell LD, Lai C-Q, Crott JW, et al. Status of vitamins B-12 and B-6 but not of folate, homocysteine, and the methylenetetrahydrofolate reductase C677T polymorphism are associated with impaired cognition and depression in adults. J Nutr. (2012) 142:1554–60.
19. Almeida OP, Marsh K, Alfonso H, Flicker L, Davis TME, Hankey GJ. B-vitamins reduce the long-term risk of depression after stroke: the VITATOPS-DEP trial. Ann Neurol. (2010) 68:503–10. doi: 10.1002/ana.22189
20. Syed E, Wasay M, Awan S. Vitamin B12 supplementation in treating major depressive disorder: a randomized controlled trial. Open Neurol J. (2013) 7:44–8.
21. Labonte B, Engmann O, Purushothaman I, Hodes G, Lorsch ZS, Hamilton PJ. Sex-specific transcriptional signatures in human depression. Nat Med. (2017) 23:1102–11.
22. Engmann O, Labonté B, Mitchell A, Bashtrykov P, Calipari ES, Rosenbluh C, et al. Cocaine-induced chromatin modifications associate with increased expression and three-dimensional looping of Auts2. Biol Psychiatry. (2017) 82:794–805. doi: 10.1016/j.biopsych.2017.04.013
23. Trautmann C, Burek D, Huebner C, Girault J, Engmann O. A regulatory pathway linking caffeine action, mood and the circadian clock. Neuropharmacology. (2020) 172:108133. doi: 10.1016/j.neuropharm.2020.108133
24. Franzka P, Krüger L, Schurig MK, Olecka M, Hoffmann S, Blanchard V, et al. Altered glycosylation in the aging heart. Front Mol Biosci. (2021) 8:673044. doi: 10.3389/fmolb.2021.673044
25. Scarpa J, Fatma M, Loh Y-HE, Traore SR, Stefan T, Chen TH, et al. Shared transcriptional signatures in major depressive disorder and mouse chronic stress models. Biol Psychiatry. (2020) 88:159–68.
26. Larsen SC, Sylvestersen KB, Mund A, Lyon D, Mullari M, Madsen MV, et al. Proteome-wide analysis of arginine monomethylation reveals widespread occurrence in human cells. Sci Signal. (2016) 9:rs9. doi: 10.1126/scisignal.aaf7329
29. Sharma V, Ichikawa M, Freeze HH. Mannose metabolism: more than meets the eye. Biochem Biophys Res Commun. (2014) 453:220–8. doi: 10.1016/j.bbrc.2014.06.021
30. Sharma V, Freeze HH. Mannose efflux from the cells: a potential source of mannose in blood. J Biol Chem. (2011) 286:10193–200. doi: 10.1074/jbc.M110.194241
31. Carss KJ, Stevens E, Foley AR, Cirak S, Riemersma M, Torelli S, et al. Mutations in GDP-mannose pyrophosphorylase B cause congenital and limb-girdle muscular dystrophies associated with hypoglycosylation of α-dystroglycan. Am J Hum Genet. (2013) 93:29–41. doi: 10.1016/j.ajhg.2013.05.009
32. Liu Z, Wang Y, Yang F, Yang Q, Mo X, Burstein E, et al. GMPPB-congenital disorders of glycosylation associate with decreased enzymatic activity of GMPPB. Mol Biomed. (2021) 2:13. doi: 10.1186/s43556-021-00027-2
33. Song Y, Willer JR, Scherer PC, Panzer JA, Kugath A, Skordalakes E, et al. Neural and synaptic defects in slytherin, a zebrafish model for human congenital disorders of glycosylation. PLoS One. (2010) 5:e13743. doi: 10.1371/journal.pone.0013743
Keywords: stress, vitamin B12, depression, glycosylation, mannose
Citation: Franzka P, Turecki G, Cubillos S, Kentache T, Steiner J, Walter M, Hübner CA and Engmann O (2022) Altered mannose metabolism in chronic stress and depression is rapidly reversed by vitamin B12. Front. Nutr. 9:981511. doi: 10.3389/fnut.2022.981511
Received: 29 June 2022; Accepted: 15 September 2022;
Published: 13 October 2022.
Edited by:
Nafisa M. Jadavji, Midwestern University, United StatesReviewed by:
Rongchun Han, Anhui University of Chinese Medicine, ChinaDa Jia, Sichuan University, China
Copyright © 2022 Franzka, Turecki, Cubillos, Kentache, Steiner, Walter, Hübner and Engmann. This is an open-access article distributed under the terms of the Creative Commons Attribution License (CC BY). The use, distribution or reproduction in other forums is permitted, provided the original author(s) and the copyright owner(s) are credited and that the original publication in this journal is cited, in accordance with accepted academic practice. No use, distribution or reproduction is permitted which does not comply with these terms.
*Correspondence: Olivia Engmann, olivia.engmann@uni-jena.de