- 1Department of Cell Biology, Cardiovascular Medical Science Center, Key Laboratory of Neural and Vascular Biology of Ministry of Education, Hebei Medical University, Shijiazhuang, China
- 2Hebei Key Laboratory of Forensic Medicine, College of Forensic Medicine, Hebei Medical University, Shijiazhuang, China
- 3Eco-Environmental Monitoring Center of Hebei Province, Shijiazhuang, China
- 4Hebei Food Safety Key Laboratory, Hebei Food Inspection and Research Institute, Shijiazhuang, China
Benzo(a)pyrene (BaP) is a highly toxic and carcinogenic polycyclic aromatic hydrocarbon (PAH) whose toxicological effects in the vessel-wall cells have been recognized. Many lines of evidence suggest that tobacco smoking and foodborne BaP exposure play a pivotal role in the dysfunctions of vessel-wall cells, such as vascular endothelial cell and vascular smooth muscle cells, which contribute to the formation and worsening of cardiovascular diseases (CVDs). To clarify the underlying molecular mechanism of BaP-evoked CVDs, the present study mainly focused on both cellular and animal reports whose keywords include BaP and atherosclerosis, abdominal aortic aneurysm, hypertension, or myocardial injury. This review demonstrated the aryl hydrocarbon receptor (AhR) and its relative signal transduction pathway exert a dominant role in the oxidative stress, inflammation response, and genetic toxicity of vessel-wall cells. Furthermore, antagonists and synergists of BaP are also discussed to better understand its mechanism of action on toxic pathways.
Introduction
BaP is a crystalline, aromatic hydrocarbon consisting of five fused benzene rings found in coal tar with the formula C20H12 in the nineteenth century (1). The main sources of BaP in food are from pollution materials in the environment or produced by the pyrolysis of amino acids, fatty acids, and carbohydrates (2).The BaP is formed during the incomplete combustion of organic matter at temperatures between 300 and 600°C, and is primarily found in automobile emissions, cigarette smoke, coal tar and charcoal-broiled foods (3, 4). Due to their lipophilic and hydrophobic characteristics, polycyclic aromatic hydrocarbon (PAH) finally accumulates in the food chain. Dietary exposure accounts for more than 90% of the total exposure to PAHs in the general population in various countries (5). Nowadays, BaP is the first pollution indicator of PAHs in food designated by the scientific community (6).
Cardiovascular diseases (CVDs) are associated with DNA damage, including DNA adduct and oxidative DNA damage, in both circulating and vessel-wall cells. And environmental chemical carcinogens have been identified to be as a risk factor for CVDs (7–10). In addition, collective evidence from these studies revealed that the cardiovascular toxicity of foodborne contaminants is mainly attributed to PAHs, especially BaP. However, no literature review focuses on the association between BaP and CVDs. In this review, we aimed to provide a comprehensive understanding of the mechanism of cell toxicity effects of BaP in CVDs, mainly focusing on atherosclerosis (AS), hypertension (HTN), and abdominal aortic aneurysm (AAA).
Sources, metabolism and tissue toxicity of BaP
The primary sources of PAH contamination can be divided into two groups: anthropogenic pollution and natural pollution. Natural sources of PAHs are negligible or less important. The significant determinants of PAH pollution are anthropogenic pollution sources, classified into four groups, i.e., industrial, mobile, domestic, and agricultural pollution sources (11). In fact, the main source of exposure to PAHs for non-smokers and non-occupationally exposed adults are food. Diet contributes to more than 90% of total PAHs exposures in the general population in various countries (2, 5). PAHs can generate during the food preparation procession (contaminated by environmental PAH that are present in air, soil, or water by deposition and transfer), or during processing and cooking (12). BaP is the first pollution indicator of PAHs in food. Therefore, foodborne BaP contaminants are a primary source of BaP uptake by humans.
After entering the body, except for a small part of BaP excreted in the feces in its original form, most of the BaP accumulated in the gastrointestinal tract, epididymal fat, lung, liver, brain, and kidney (13). BaP is highly lipophilic and can be easily absorbed into cells through the plasma membrane. BaP can be metabolized into dozens of metabolites through AhR and aromatic hydrocarbon metabolizing enzymes (14–16), such as 1, 2-dihydroxy-1, 2-dihydrobenzopyrene, benzopyrene diketone, and BPDE. The conversion to hydroxyl compounds or ketones is a detoxification reaction, while the conversion to epoxide is an activation reaction. About 10% of BaP is converted to BPDE in the organism (17). BPDE has a strong oxidizing capacity, which can cause oxidative damage to DNA, affect DNA replication, and induce apoptosis and gene mutation.
BaP is well known for its carcinogenic activity early in 1930, and numerous studies since the 1970s have documented links between BaP intake and cancers (1, 18, 19). It has been classified as class I carcinogen by the International Agency for Research on Cancer (www.iarc.who.int). The exposure of BaP results in extensive and selective formation of BPDE, which has high interaction activity with DNA and forms a DNA adduct, the major mutagen in tobacco smoke (7, 20). Properly speaking, BaP is a procarcinogen. Its carcinogenic effects depend on the activity of the detoxification enzymes cytochrome P450 1A1 (CYP1A1) and CYP1B1, which enzymatic metabolism BaP to BPDE (21, 22). Furthermore, BaP induces the CYP1A1 gene expression by activating the AhR nuclear translocation signal pathway (23, 24). Furthermore, most of the BPDE-DNA adducts can be removed from DNA by nucleotide excision repair mechanism within the cell. Therefore, continuous or high doses of BaP intake will inevitably cause errors during DNA replication leading to carcinogenic mutations (23–25). Besides, there is growing evidence that BaP has strong toxic effects on the nervous system (26), immune system (27, 28), and reproductive system (29).
AhR is a ligand-activated transcription factor best known for mediating carcinogen toxicity and tumor-promoting properties, including dioxin and BaP (30). AhR belongs to the basic helix-loop-helix transcription factors family. And numerous studies have revealed that the toxicity of BaP has been linked to activation of the AhR (31–33). In the absence of ligands, AhR exists predominantly in the cytosolic compartment in association with a chaperone complex (Hsp90/XAP/p23) (30). Upon BaP binding to AhR, dimerization of AhR and the aryl hydrocarbon receptor nuclear translocator (ARNT) occurs. The AhR/ARNT heterodimer then binds to xenobiotic responsive elements (XREs) (core sequence: GCGTG) in the promoters of BaP-regulated genes, such as cytochrome P450s (CYPs), intercellular cell adhesion molecule 1 (ICAM1), vascular cell adhesion molecule 1 (VCAM1), and prostaglandin endoperoxide synthase 2 (PTGS2) (33–35).
CYPs are membrane-associated proteins that use molecular oxygen and reduce the equivalents of NADPH to catalyze oxidative, peroxidative, and reductive metabolism of endogenous and exogenous substrates (36). More than 400 genes encoding CYP-associated activities have been cloned, but their relative expression exhibits remarkable tissue, gender, and developmental specificity (37). Kerzee and Ramos investigated constitutive and inducible expression of these two CYPs from AhR knockout mice. Their results show that the expression of CYP1A1 was inducible in BaP-treated AhR+/+ mice, and CYP1B1 was expressed under constitutive and inducible conditions irrespective of AhR phenotype or growth status (36). In mouse aortic smooth muscle cells, BaP increased the aryl hydrocarbon hydroxylase activity. The specific inhibitor of CYP1B1, but not CYP1A1, could reverse the BaP-induced DNA adducts formation, which may contribute to atherogenesis by PAHs (38). Furthermore, CYP1A1−/− and AhR−/− mice formed smaller atherosclerotic lesions size and oxidative stress when suffering over 10 weeks of 10 mg/kg/body weight(bw) BaP treatment (39, 40).
Vascular toxicity effects and underlying mechanism of BaP
As early as 1977, it was reported that the aorta was the target organ of BaP (41). However, it is only in recent years, the cardiovascular toxicity of BaP attracted considerable attention. Currently, as an independent risk factor for CVDs, BaP has been found to be closely related to CVDs, including AS, HTN and AAA, and shows multiple kinds of vascular toxicity (10, 11, 13, 42). Preclinical studies have found that BaP exposure is correlated with oxidative stress and vascular toxicity. In addition, investigations have discovered a relationship between BaP exposure and the occurrence and development of CVDs (11).
Atherogenic effect of BaP
Atherosclerosis (AS) is a chronic pathological process in the large artery wall and is characterized by the accumulation of oxidized lipid, fibrous elements, and calcification. This process is initiated by endothelium injury, followed by a cascade of events, which causes the vessel narrowing and activation of inflammatory responses leading to atheroma plaque formation (43–45). As a result, these processes result in multiple cardiovascular complications, including myocardial infarction, heart failure, stroke, and claudication, which remain the leading cause of death globally (46). Moreover, this complex disease is caused by the interaction of multiple genetic and environmental risk factors, which include western pattern diet, tobacco smoking, and air pollution (45).
In ApoE−/− background atherosclerotic animal models, 4 days of 5–8 mg/kg/bw BaP grave treatment can increase the serum epsilon A and high-density lipoprotein (HDL) level (47). Moreover, the BPDE-DNA adduct could also be observed in the aorta tissues (47), while compared to the control groups, 12–24 weeks of treatment increased the expression of monocyte chemoattractant protein 1 (MCP1), and promoted the release of transforming growth factor beta (TGFβ) and tumor necrosis factor α (TNFα) in vascular wall cells (48–50). In response to the inflammatory mediator, the atherosclerosis lesion may be heavily infiltrated with pro-inflammation cells, including macrophages, T cells and neutrophils (49), and the lesion size began to enlarge (13, 50).
Exposure to BaP plays a vital role in the etiology of atherosclerosis (Table 1). The endothelium represents the inner cell layer of blood vessels and is supported by smooth muscle cells and pericytes, which form the vessel structure (51). Due to direct blood contact, the blood vessel endothelium is inevitably exposed to genotoxic substances that are systemically taken up by the body, including BaP (17). One key step in the development of atherosclerosis is vascular endothelial dysfunction, followed by cell death and a local inflammatory response (48, 53, 67). Besides, there is strong evidence to suggest oxidative stress is one of the most potent inductors of vascular inflammation in atherogenesis. nuclear factor-κB (NF-κB) may respond directly to BaP-induced oxidative stress, and the activation of NF-κB is a key redox-sensitive event associated with vascular dysfunction (54).
Caveolae are non-clathrin-coated plasma membrane microdomains enriched in cholesterol and glycosphingolipids. They are particularly abundant in endothelial cells and play an important role in membrane traffic and cellular signal transduction (52, 68). Oesterling and his collage observed that caveolin-1 mediated the BaP-induced ICAM1 expression in primary human endothelial cells. They also illustrated that β-naphthoflavone/BaP induced ICAM1 expression by signaling through MEK, MAPK, and AP-1 leading to increased adhesion of monocytes to the activated endothelium (52, 68).
Besides, BaP-induced bulky DNA adducts and the consequent DNA mutations in vascular cells are considered to be involved in AS (31). BaP forms BPDE through a three-step activation process catalyzed by human cytochrome P450 enzymes, notably CYP1A1 and CYP1B1, and by epoxide hydrolase (17). A screening for DNA repair factors in BPDE treated human umbilical vein endothelial cells (HUVECs) revealed that the nucleotide excision repair (NER) proteins excision repair cross-complementation (ERCC) 1, ERCC 4 and ligase I were expressed at lower levels in HUVECs compared with human umbilical artery smooth muscle cells (HUASMCs) and haemopoietic progenitor cell (HPCs), which corresponds with the impaired NER-mediated removal of BPDE adducts from DNA (17). These data revealed that HUVECs is more sensitive to BPDE than HPCs and HUASMCs and displays an unexpected DNA repair-impaired phenotype.
Vascular smooth muscle cells (VSMCs) are located in the mid-membrane of the vascular wall and are responsible for the structural characteristics of the vessel wall. Abnormal proliferation, migration, and invasion of VSMCs have been suggested to be the major contributor to the development of atherosclerotic lesions (57, 58, 69). Evidence shows that BaP could activate interleukin 6 (IL6) production and suppress nitric oxide-induced apoptosis in VSMCs. A significant role of IL6 in the pathophysiology of atherosclerosis has also been suggested, and atherosclerosis even has been suggested to be an inflammatory disease (69, 70). Furthermore, BaP was capable of inducing the activation of NF-κB and MAPK in VSMCs. Both NF-κB inhibitor and MAPK inhibitor significantly reversed the anti-apoptotic effect of BaP on NO-induced VSMCs apoptosis (57).
Matrix metalloproteinases (MMPs) are a family of over 20 different endopeptidases that each degrades several extracellular matrix proteins and non-matrix substrates. MMPs play a major role in cell migration, differentiation, angiogenesis, and host defense (71). Expression of various MMPs was found to increase in BaP-induced transcriptional activation of MMPs, especially MMP3, is not through activator protein 1 (AP-1) or NF-κB, and the expression of MMPs increased the migration and invasion ability of VSMCs in rats (58). Besides, when treated with BaP, the expression level of T-cadherin, an atypical glycosylphosphatidylinositol-anchored member of the cadherin superfamily of adhesion molecules, is significantly repressed (59). However, further investigation of the relationship between the upregulation of MMPs and T-cadherin degradation still needs further exploration.
Cyclooxygenase (COX2), also known as prostaglandin-endoperoxide synthase (PTGS2), is a rate-limiting enzyme responsible for prostaglandins forming and plays both physiologic and pathologic roles in vascular function. In BaP-treated VSMCs, ERK and NF-κB signal pathways are involved in the expression of COX2, which may participate in the genesis of AS (60).
Miller and his colleagues observed that treatment of VSMC with BaP induced reactive oxygen species (ROS) accumulation which leads to a variety of different outcomes, including activation of nuclear proteins to bind antioxidant response element/electrophilic response element (ARE/EpRE), activation of cytosolic proteins that translocate and bind ARE/EpRE, or redox sensor that interacts with cellular proteins to activate binding to ARE/EpRE. As a result, oxidative intermediates of BaP mediate activation of nuclear protein binding to ARE/EpRE and contribute to transcriptional de-regulation of c-Ha-ras (62). Kerzee et al. (63) found the upregulation of oxidative stress and c-Ha-ras in VSMCs could be reserved by CYPs and AhR inhibitor ellipticine. Chen et al. (61) observed another nuclear protein CCAAT/enhancer-binding protein alpha and beta (C/EBP-α, β) which was activated by AhR signal pathway and lead to the inhibition of glutathione S-transferase (GST)-Ya subunit gene expression. In addition, overexpression of antioxidant enzymes suppressed BaP-accelerated atherosclerosis in ApoE-deficient mice (66, 72).
BaP and hypertension
Hypertension (HTN), also known as high blood pressure, is a long-term medical condition in which the blood pressure in the arteries is persistently elevated (7). HTN usually does not cause noticeable symptoms. However, long-term untreated HTN is a major risk factor for heart attacks, stroke, atrial fibrillation, heart failure, and peripheral arterial disease. HTN is a major cause of premature death worldwide (73, 74). Over 90% of HTN is classified as a primary type and is usually caused by unhealthy lifestyles such as a high salt diet, overweight, alcohol drinking, and smoking. The remaining cases are categorized as secondary types due to identifiable causes, including chronic kidney disease, narrowing of the kidney arteries, and an endocrine disorder (73).
Studies have shown that systolic blood pressure is significantly increased, and aortic responsiveness to phenylephrine is enhanced in rats exposed to BaP (Table 2). Inhibitors of protein kinase C (PKC), MAPK, myosin light-chain kinase (MLCK) and Rho kinases significantly inhibit BaP-enhanced vasoconstriction (Table 2). BaP induces ROS production in vascular smooth muscle cells in a time-dependent manner (7). Heart rate was not affected in BaP-treated sprague-dawley rats, however, weight loss, markedly elevated blood pressure (14). BaP exposure may affecte cardiovascular development and increased systolic blood pressure. Jules et al. (76) show that exposure to BaP results in functional deficits in offspring during cardiovascular development, which may lead to cardiovascular dysfunction later in life. BaP exposure altered the circadian pattern of blood pressure, with a reduction in the normal dipping pattern during sleep. This was associated with increased neutrophil recruitment in the lungs of BaP-exposed rats (75). Intraperitoneal injection of BaP up regulated the expression of CYP1A, CYP1B1, CYP1C1, CYP1C2, and COX1 in zebrafish mesenteric arteries suggesting that BaP is associated with changes in cardiovascular function (77).
BaP and abdominal aortic aneurysm
Abdominal aortic aneurysm (AAA) is defined as a localized enlargement of the abdominal aorta more than 50% of its diameter. The aortic wall continues to weaken and becomes unable to hold the forces of the luminal blood pressure, resulting in progressive dilatation and rupture. They usually cause no symptoms, except during rupture. Smoking and advanced age are the primary risk factors for AAA; if ruptured, the mortality is 85−90% (42, 92).
Co-stimulation of male C57BL/6J mice with angiotensin (Ang) II and BaP induced AAA significantly increased rates of formation and mean aortic diameter. The samples were subjected to circRNA expression analysis, and a circRNA-miRNA co-expression network was established based on six apoptosis-related circRNAs. Genes regulated by this network map to multiple pathways, including apoptosis, IL-17 signaling, and vascular endothelial growth factor signaling, all of which are involved in AAA formation (78). BaP increases macrophage infiltration, activates NF-κB, upregulates MMP2, MMP9, and MMP12 expression, elastic lamina disorder, and VSMCs loss (79), which increased AAA formation and rupture in C57/B6J mice (80). Furthermore, the metabolites of BaP such as 7,8-dihydrodiol, 3,6-, and 6,12-dione metabolites are reported involvement in BaP induced abdominal aortic toxicity via elevating plasma ROS levels and increased protein expression of TNFα, CYP1A1, and MMP9 (42).
BaP and myocardial injury
In BaP-treated offspring rats, microarray and quantitative real-time PCR analysis revealed that the up-regulated gene expression of AngII, angiotensinogen and, eNOS, which are associated with the dysregulate cardiovascular development (76). BaP increased heart-to-body weight ratios, as well as hypertrophy markers, atrial natriuretic peptide and brain natriuretic peptide. BaP treatment increased the gene expression of CYP1A1, CYP1B1, CYP2E1, CYP4F4, CYP4F5 and soluble epoxide hydrolase. BaP treatment increased the ratios of dihydroxyeicosatrienoic acid and 20-hydroxyeicosatetraenoic acid in total epoxyeicosatrienoic acid. Benzo(e)pyrene, an isomer of BaP and a poor ligand for AhR, did not cause cardiac hypertrophy in rats, confirming the role of AhR in the development of cardiac hypertrophy (82). Zebrafish exposed to solutions containing 5 μmol/L BaP treatment exhibit cardiovascular malformations. Microarray analysis was performed to identify heart-specific transcriptomic changes in BaP/fluoranthene (FL) during early development, with Ca2+ cycling and muscle contraction genes being the most differentially expressed class of transcripts (Table 2). BaP/FL may affect cellular Ca2+ levels, which subsequently affect myocardial function and may underlie BaP/FL cardiotoxicity (84). Exposure of zebrafish embryos to BaP for 72 h resulted in defective cardiac development in zebrafish embryos (85). BaP co-exposure with alpha-naphthoflavone or resveratrol resulted in the most dramatic changes in heart and vessel morphology, with decreased ventricular length and width, increased ventricular wall thickness, and increased vessel lumen diameter. In addition, decreased expression of COX2, which is inversely associated with cardiac malformations and vasodilation (86). Moreover, BaP-induced NCF1/p47(phox) expression enhances superoxide anion production in an AhR-dependent manner in PMA-treated human macrophages; regulation of NCF1/NADPH oxidase by such PAHs may be involved in atherosclerotic heart disease associated with vascular disease such as sclerosis (81).
BaP and angiogenesis
BaP exposure is also associated with angiogenesis both in vivo and in vitro (Table 2). Evidence shows that BaP reduced corpus luteum number, disrupted steroid secretion, affected the corpus luteum vascular network in pregnant female mice, and significantly decreased angiogenic factors (VEGFR, Ang-1, and Tie2), and increased the anti-angiogenic factor THBS1. The BaP metabolite BPDE also interfered with the expression levels of angiogenesis-related factors, such as Notch signaling molecules in HUVECs in vitro (10). The expression of several decidua-related factors was altered, including FOXO1, HoxA10, and BMP2 (Table 2). BaP reduced CD34 expression, suggesting that BaP treatment inhibited decidual angiogenesis. Furthermore, BaP induced the downregulation of vascular endothelial growth factors suggesting that oral administration of BaP impairs decidualization and decidual angiogenesis (91).
After exposure to BaP, the expression of metallothionein was upregulated in the ischemic hindlimb of wild-type mice and markedly inhibited ischemia-induced angiogenesis. The mRNA amount of IL6 and VEGF was reduced in the ischemic hindlimb of wild-type mice (90). BaP-treated HUVECs reduced endothelial capillary formation, cell migration, MAPK phosphorylation, and integrin expression when stimulated by angiogenic factors. Angiogenesis was also inhibited in the chorionic villus assay (87).
Using zebrafish embryos model, 6H-benzo[cd]pyren-6-one induced developmental and cardiovascular toxicity at lower doses, including reduced heart rate and blood flow. The mixture of PAHs and oxy-PAHs may lead to increased developmental and cardiovascular toxicity of zebrafish embryos through an AhR-dependent mechanism (88). Embryonic teratogenicity and developmental toxicity of BaP in Japanese medaka, BaP was efficiently incorporated into embryos by nanosecond pulsed electric field treatment. Embryos containing BaP exhibited typical teratogenic and developmental effects, such as cardiovascular abnormalities, dysplasia, and spinal curvature (89).
BaP and other CVDs
Two blood-testis barrier proteins, Claudin-11 and Connexin 43, were impaired by treatment with a mixture of 1 μg/L streptozotocin and 1 μg/L BaP (93). The function of blood brain barrier was assessed by measuring the transendothelial electrical resistance of mouse brain microvascular endothelial cells, and the viability of cells was altered in the presence of BaP (94). Besides, BaP elicited mouse testicular sertoli cells apoptosis and blood-testis barrier disruption, which involves mitochondrial dysfunction and oxidative stress (95). These evidence imply that BaP can damage vascular barrier which in turn cause other injury includes neurotoxicity and reproductive toxicity.
Chronic BaP exposure did not alter hepatocellular carcinoma cell (HCC) growth, but promoted cell migration and invasion both in vitro and in vivo. There was a negative correlation between BaP exposure and survival in tumor-bearing mice. In addition, BaP-treated HCC cells recruited vascular endothelial cells and promoted tumor angiogenesis, possibly by increasing the secretion of vascular endothelial growth factor. The NF-κB pathway may be an adverse outcome pathway related to the cumulative effect of BaP on HCC metastasis (96).
Antagonist and synergistic agents of BaP
Numerous reports examined different types of agents in interfering BaP metabolism and molecular signing alteration, which have potential therapeutic implications in BaP induced CVDs (Table 3). Gdula-Argasińska et al. observed that resolvin D1, a product of transcellular biosynthesis with leukocytes and endothelial cells from docosa-hexaenoic acid, reversed the overexpression of COX2, cPGES and repression of GSTM1 protein after co-cultured with BaP incubated HUVEC. Besides, an increase of cPLA2 and a decrease of CYP1A1 activity were also noted in RvD1 and BaP co-treatment (97). These data suggested that resolvin D1 could contribute to vascular function and alleviate the harmful effects caused by BaP, which might potentially aid in repairing the injured endothelium. In addition, flavonoids that contain a 40 B-ring hydroxyl substitution and a 2-3 C-ring double bond can decrease proinflammatory molecular ICAM1 expression in endothelial cells (98). Two latest reports show that bioactive compounds, hesperidin, and phaeodactylum tricornutum extracts can also inhibit the pro-inflammation mediators, including IL1β, TNFα, and IL8 (99, 100). Moreover, Budesonide-poly(lactide-co-glycolide), Soluble epoxide hydrolase inhibitor, and Ginkgo biloba extract have been proved to have anti-oxidative effects. In BaP-treated animals, the repression of ROS, NO, and CYP1A1 level and multiple cell protection effects were observed (100, 102, 103). Collectively, the above observations indicate that the anti-oxidative and anti-inflammation natural extract or inhibitors have potential protective effects on BaP-induced damage on vascular wall cells.
Using the zebrafish model, Duan and Asweto et al. found that co-exposure of silica nanoparticles and BaP activate the AP-1/c-Jun and MAPK/PI3K signaling pathway, upregulate the expression of proinflammatory and procoagulant genes. As a result, silica nanoparticles help trigger the inflammation response and ROS generation, which could cause cardiac toxicity and erythrocyte aggregation (106–108). Interestingly, the protective effects of vitamin D on cardiovascular disease are principally mediated by the conversion of vitamin D to the active form, 1α,25-dihydroxy vitamin D3 [1,25(OH)2D3]. However, combined treatment with BaP and 1,25(OH)2D3 enhances BaP toxicity, including BaP-DNA adduct formation and ROS production (104).
Conclusions
The present review summarizes the molecular mechanisms underlying the vascular cell toxicity effects of BaP in CVDs as follows: (1) numerous studies have demonstrated that BaP can accelerate the pathological progress of AS, and many lines of evidence show that excessive daily BaP intake is a potent risk factor which accompanied by AAA, HTN, and MI. (2) BaP binds to the ligand-activated transcription factor AhR, which evokes oxidative stress and inflammation response molecules in ECs via activating the AhR/ARNT/XRE, MEK/c-Jun, and MAPK/NF-kB/AP-1 signaling pathway. (3) BaP exposures increase the cell proliferation, migration, and invasion ability of VSMC by a similar signaling pathway in BaP-treated ECs. (4) The activation of CYP1A1 actually increased the genetic toxicity on vessel-wall cells through the metabolism of BaP into BPDE, which can form DNA adducts and induce mutation (Figure 1). Furthermore, compounds with AhR activation activity probability exert synergism effects on BaP vascular toxicity. In contrast, this toxicity might be alleviated by bioactive materials which process anti-oxidative and anti-inflammation action.
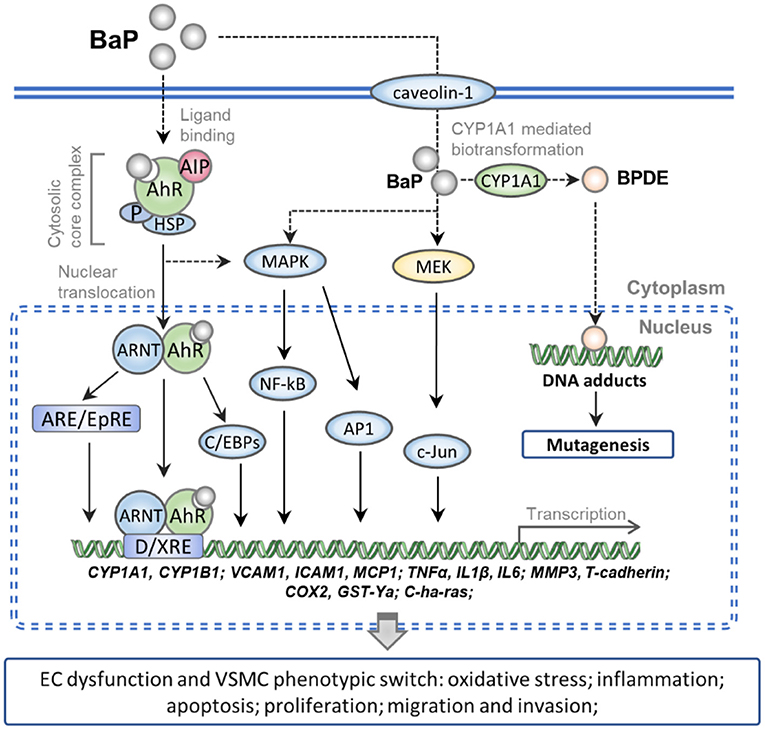
Figure 1. Schematic representation of the molecular mechanism of BaP exposure-induced CVDs. Numerous pieces of evidence show that the caveolin-1 can facilitate entry of the BaP into vessel-wall cells. The AhR complex is translocated into nuclear after binding with intracellular BaP. And then the BaP-AhR complex could activate the AhR/ARNT/XRE, MEK/c-Jun, and MAPK/NF-kB/AP-1 signaling pathway which can up-regulate the gene of CYPs, cell adhesion molecules, pro-inflammatory factors, and peroxidase. Besides, the activated CYP1A1 actually increased the metabolism of BaP into BPDE, which can form DNA adducts and induce mutation. As a result, BaP exposure increases the ECs dysfunction and VSMC phenotypic switch which accelerates the pathological progress of CVDs.
Author contributions
PL and YZ: ideas, conceptualization, supervision, and funding acquisition. CF and YL: resources, literature collection, and writing-review and editing. HX, ZN, NC, RW, YY, XG, MW, and WZ: literature collection and initial draft writing. All authors contributed to the article and approved the submitted version.
Funding
This work was supported by the National Key Research Project of China (2019YFC1606400), Major Public Welfare Projects in Henan Province (201300110200), National Key Research Project of Hebei Province (20375502D), Fund of National R&D Center for Edible Fungus Processing Technology, Henan University (20200109), Postdoctoral Research Funds of Hebei Medical University (30705010016-3759), and University Science and Technology Research Project of Hebei Province (QN2017107).
Conflict of interest
The authors declare that the research was conducted in the absence of any commercial or financial relationships that could be construed as a potential conflict of interest.
Publisher's note
All claims expressed in this article are solely those of the authors and do not necessarily represent those of their affiliated organizations, or those of the publisher, the editors and the reviewers. Any product that may be evaluated in this article, or claim that may be made by its manufacturer, is not guaranteed or endorsed by the publisher.
References
2. Domingo JL, Nadal M. Human dietary exposure to polycyclic aromatic hydrocarbons: a review of the scientific literature. Food Chem Toxicol. (2015) 86:144–53. doi: 10.1016/j.fct.2015.10.002
3. Hu G, Cai K, Li Y, Hui T, Wang Z, Chen C, et al. Significant inhibition of garlic essential oilon benzo[a]pyrene formation in charcoal-grilled pork sausagesrelates to sulfide compounds. Food Res Int. (2021) 141:110127. doi: 10.1016/j.foodres.2021.110127
4. Liao K, Yu JZ. Abundance and sources of benzo[a]pyrene and other PAHs in ambient air in Hong Kong: a review of 20-year measurements (1997-2016). Chemosphere. (2020) 259:127518. doi: 10.1016/j.chemosphere.2020.127518
5. Alomirah H, Al-Zenki S, Al-Hooti S, Zaghloul S, Sawaya W, Ahmed N, et al. Concentrations and dietary exposure to polycyclic aromatic hydrocarbons (PAHs) from grilled and smoked foods. Food Control. (2011) 22:2028–35. doi: 10.1016/j.foodcont.2011.05.024
6. Zhang Y, Chen X, Zhang Y. Analytical chemistry, formation, mitigation, and risk assessment of polycyclic aromatic hydrocarbons: from food processing to in vivo metabolic transformation. Compr Rev Food Sci Food Saf. (2021) 20:1422–56. doi: 10.1111/1541-4337.12705
7. Tzeng H-P, Yang T-H, Wu C-T, Chiu H-C, Liu S-H, Lan K-C. Benzo[a]pyrene alters vascular function in rat aortas ex vivo and in vivo. Vascul Pharmacol. (2019) 121:106578. doi: 10.1016/j.vph.2019.106578
8. Pulliero A, Godschalk R, Andreassi MG, Curfs D, Van Schooten FJ, Izzotti A. Environmental carcinogens and mutational pathways in atherosclerosis. Int J Hyg Environ Health. (2015) 218:293–312. doi: 10.1016/j.ijheh.2015.01.007
9. Vayssier-Taussat M, Camilli T, Aron Y, Meplan C, Hainaut P, Polla BS, et al. Effects of tobacco smoke and benzo[a]pyrene on human endothelial cell and monocyte stress responses. Am J Physiol Heart Circ Physiol. (2001) 280:H1293–300. doi: 10.1152/ajpheart.2001.280.3.H1293
10. Liu M, Deng T, He J, Ding Y, Liu X, Xu H, et al. Exposure to Benzo[a]pyrene impairs the corpus luteum vascular network in rats during early pregnancy. Environ Pollut. (2020) 259:113915. doi: 10.1016/j.envpol.2020.113915
11. Mallah MA, Changxing L, Mallah MA, Noreen S, Liu Y, Saeed M, et al. Polycyclic aromatic hydrocarbon and its effects on human health: an overeview. Chemosphere. (2022) 296:133948. doi: 10.1016/j.chemosphere.2022.133948
12. Singh L, Varshney JG, Agarwal T. Polycyclic aromatic hydrocarbons' formation and occurrence in processed food. Food Chem. (2016) 199:768–81. doi: 10.1016/j.foodchem.2015.12.074
13. Jin X, Hua Q, Liu Y, Wu Z, Xu D, Ren Q, et al. Organ and tissue-specific distribution of selected polycyclic aromatic hydrocarbons (PAHs) in ApoE-KO mouse. Environ Pollut. (2021) 286:117219. doi: 10.1016/j.envpol.2021.117219
14. Gan TE, Xiao SP, Jiang Y, Hu H, Wu YH, Duerksen-Hughes PJ, et al. Effects of benzo(a)pyrene on the contractile function of the thoracic aorta of Sprague-Dawley rats. Biomed Environ Sci. (2012) 25:549–56. doi: 10.3967/0895-3988.2012.05.008
15. Bolton JL, Trush MA, Penning TM, Dryhurst G, Monks TJ. Role of quinones in toxicology. Chem Res Toxicol. (2000) 13:135–60. doi: 10.1021/tx9902082
16. Shimada T, Guengerich FP. Inhibition of human cytochrome P450 1A1-, 1A2-, and 1B1-mediated activation of procarcinogens to genotoxic metabolites by polycyclic aromatic hydrocarbons. Chem Res Toxicol. (2006) 19:288–94. doi: 10.1021/tx050291v
17. Kress JM, Dio LD, Heck L, Pulliero A, Izzotti A, Laarmann K, et al. Human primary endothelial cells are impaired in nucleotide excision repair and sensitive to benzo[a]pyrene compared with smooth muscle cells and pericytes. Sci Rep. (2019) 9:13800. doi: 10.1038/s41598-019-49953-w
18. Myers JN, Harris KL, Rekhadevi PV, Pratap S, Ramesh A. Benzo(a)pyrene-induced cytotoxicity, cell proliferation, DNA damage, and altered gene expression profiles in HT-29 human colon cancer cells. Cell Biol Toxicol. (2021) 37:891–913. doi: 10.1007/s10565-020-09579-5
19. Xue J, Zhao Q, Sharma V, Nguyen LP, Lee YN, Pham KL, et al. Aryl hydrocarbon receptor ligands in cigarette smoke induce production of interleukin-22 to promote pancreatic fibrosis in models of chronic pancreatitis. Gastroenterology. (2016) 151:1206–17. doi: 10.1053/j.gastro.2016.09.064
20. Weng MW, Lee HW, Park SH, Hu Y, Wang HT, Chen LC, et al. Aldehydes are the predominant forces inducing DNA damage and inhibiting DNA repair in tobacco smoke carcinogenesis. Proc Natl Acad Sci U S A. (2018) 115:E6152–e6161. doi: 10.1073/pnas.1804869115
21. Abbass M, Chen Y, Arlt VM, Stürzenbaum SR. Benzo[a]pyrene and Caenorhabditis elegans: defining the genotoxic potential in an organism lacking the classical CYP1A1 pathway. Arch Toxicol. (2021) 95:1055–69. doi: 10.1007/s00204-020-02968-z
22. Jee SC, Kim M, Kim KS, Kim HS, Sung JS. Protective effects of myricetin on benzo[a]pyrene-induced 8-hydroxy-2'-deoxyguanosine and BPDE-DNA ADduct. Antioxidants. (2020) 9:446 doi: 10.3390/antiox9050446
23. Ghosh J, Chowdhury AR, Srinivasan S, Chattopadhyay M, Bose M, Bhattacharya S, et al. Cigarette smoke toxins-induced mitochondrial dysfunction and pancreatitis involves aryl hydrocarbon receptor mediated cyp1 gene expression: protective effects of resveratrol. Toxicol Sci. (2018) 166:428–40. doi: 10.1093/toxsci/kfy206
24. Vázquez-Gómez G, Rocha-Zavaleta L, Rodríguez-Sosa M, Petrosyan P, Rubio-Lightbourn J. Benzo[a]pyrene activates an AhR/Src/ERK axis that contributes to CYP1A1 induction and stable DNA adducts formation in lung cells. Toxicol Lett. (2018) 289:54–62. doi: 10.1016/j.toxlet.2018.03.012
25. Mescher M, Haarmann-Stemmann T. Modulation of CYP1A1 metabolism: From adverse health effects to chemoprevention and therapeutic options. Pharmacol Ther. (2018) 187:71–87. doi: 10.1016/j.pharmthera.2018.02.012
26. Liu D, Zhao Y, Qi Y, Gao Y, Tu D, Wang Y, et al. Benzo(a)pyrene exposure induced neuronal loss, plaque deposition, and cognitive decline in APP/PS1 mice. J Neuroinflammation. (2020) 17:258. doi: 10.1186/s12974-020-01925-y
27. Ma J, Liu X, Yang Y, Qiu J, Dong Z, Ren Q, et al. Binding of benzo[a]pyrene alters the bioreactivity of fine biochar particles toward macrophages leading to deregulated macrophagic defense and autophagy. ACS Nano. (2021) 15:9717–31. doi: 10.1021/acsnano.1c00324
28. Cella M, Colonna M. Aryl hydrocarbon receptor: linking environment to immunity. Semin Immunol. (2015) 27:310–4. doi: 10.1016/j.smim.2015.10.002
29. Rahmani Z, Karimpour Malekshah A, Zargari M, Talebpour Amiri F. Effect of prenatal exposure to Benzo[a]pyrene on ovarian toxicity and reproductive dysfunction: protective effect of atorvastatin in the embryonic period. Environ Toxicol. (2021) 36:1683–93. doi: 10.1002/tox.23164
30. Murray IA, Patterson AD, Perdew GH. Aryl hydrocarbon receptor ligands in cancer: friend and foe. Nat Rev Cancer. (2014) 14:801–14. doi: 10.1038/nrc3846
31. Wang Z, Yang H, Ramesh A, Roberts LJ, Zhou L, Lin X, et al. Overexpression of Cu/Zn-superoxide dismutase and/or catalase accelerates benzo(a)pyrene detoxification by upregulation of the aryl hydrocarbon receptor in mouse endothelial cells. Free Radic Biol Med. (2009) 47:1221–9. doi: 10.1016/j.freeradbiomed.2009.08.001
32. N'Diaye M, Le Ferrec E, Lagadic-Gossmann D, Corre S, Gilot D, Lecureur V, et al. Aryl hydrocarbon receptor- and calcium-dependent induction of the chemokine CCL1 by the environmental contaminant benzo[a]pyrene. J Biol Chem. (2006) 281:19906–15. doi: 10.1074/jbc.M601192200
33. Palatnik A, Xin H, Su EJ. Dichotomous effects of aryl hydrocarbon receptor (AHR) activation on human fetoplacental endothelial cell function. Placenta. (2016) 44:61–8. doi: 10.1016/j.placenta.2016.06.004
34. Chavan H, Krishnamurthy P. Polycyclic aromatic hydrocarbons (PAHs) mediate transcriptional activation of the ATP binding cassette transporter ABCB6 gene via the aryl hydrocarbon receptor (AhR). J Biol Chem. (2012) 287:32054–68. doi: 10.1074/jbc.M112.371476
35. Dolwick KM, Swanson HI, Bradfield CA. In vitro analysis of Ah receptor domains involved in ligand-activated DNA recognition. Proc Natl Acad Sci U S A. (1993) 90:8566–70. doi: 10.1073/pnas.90.18.8566
36. Kerzee JK, Ramos KS. Constitutive and inducible expression of Cyp1a1 and Cyp1b1 in vascular smooth muscle cells: role of the Ahr bHLH/PAS transcription factor. Circ Res. (2001) 89:573–82. doi: 10.1161/hh1901.097083
37. Zanger UM, Schwab M. Cytochrome P450 enzymes in drug metabolism: regulation of gene expression, enzyme activities, and impact of genetic variation. Pharmacol Ther. (2013) 138:103–41. doi: 10.1016/j.pharmthera.2012.12.007
38. Moorthy B, Miller KP, Jiang W, Williams ES, Kondraganti SR, Ramos KS. Role of cytochrome P4501B1 in benzo[a]pyrene bioactivation to DNA-binding metabolites in mouse vascular smooth muscle cells: evidence from 32P-postlabeling for formation of 3-hydroxybenzo[a]pyrene and benzo[a]pyrene-3,6-quinone as major proximate genotoxic intermediates. J Pharmacol Exp Ther. (2003) 305:394–401. doi: 10.1124/jpet.102.044271
39. Uno S, Sakurai K, Nebert DW, Makishima M. Protective role of cytochrome P450 1A1 (CYP1A1) against benzo[a]pyrene-induced toxicity in mouse aorta. Toxicology. (2014) 316:34–42. doi: 10.1016/j.tox.2013.12.005
40. Kerley-Hamilton JS, Trask HW, Ridley CJ, Dufour E, Lesseur C, Ringelberg CS, et al. Inherent and benzo[a]pyrene-induced differential aryl hydrocarbon receptor signaling greatly affects life span, atherosclerosis, cardiac gene expression, and body and heart growth in mice. Toxicol Sci. (2012) 126:391–404. doi: 10.1093/toxsci/kfs002
41. Bond JA, Kocan RM, Benditt EP, Juchau MR. Metabolism of benzo[a]pyrene and 7,12-dimethylbenz[a]anthracene in cultured human fetal aortic smooth muscle cells. Life Sci. (1979) 25:425–30. doi: 10.1016/0024-3205(79)90574-5
42. Ramesh A, Prins PA, Perati PR, Rekhadevi PV, Sampson UK. Metabolism of benzo(a)pyrene by aortic subcellular fractions in the setting of abdominal aortic aneurysms. Mol Cell Biochem. (2016) 411:383–91. doi: 10.1007/s11010-015-2600-2
43. Borén J, Chapman MJ, Krauss RM, Packard CJ, Bentzon JF, Binder CJ, et al. Low-density lipoproteins cause atherosclerotic cardiovascular disease: pathophysiological, genetic, and therapeutic insights: a consensus statement from the European atherosclerosis society consensus panel. Eur Heart J. (2020) 41:2313–30. doi: 10.1093/eurheartj/ehz962
44. Jebari-Benslaiman S, Galicia-García U, Larrea-Sebal A, Olaetxea JR, Alloza I, Vandenbroeck K, et al. Pathophysiology of Atherosclerosis. Int J Mol Sci. (2022) 23:3346. doi: 10.3390/ijms23063346
45. Falk E. Pathogenesis of atherosclerosis. J Am Coll Cardiol. (2006) 47:C7–12. doi: 10.1016/j.jacc.2005.09.068
46. Frostegård J. Immunity, atherosclerosis and cardiovascular disease. BMC Med. (2013) 11:117. doi: 10.1186/1741-7015-11-117
47. Godschalk R, Curfs D, Bartsch H, Van Schooten FJ, Nair J. Benzo[a]pyrene enhances lipid peroxidation induced DNA damage in aorta of apolipoprotein E knockout mice. Free Radic Res. (2003) 37:1299–305. doi: 10.1080/10715760310001621333
48. Knaapen AM, Curfs DM, Pachen DM, Gottschalk RW, Winther MP, de Daemen MJ, et al. The environmental carcinogen benzo[a]pyrene induces expression of monocyte-chemoattractant protein-1 in vascular tissue: a possible role in atherogenesis. Mutat Res. (2007) 621:31–41. doi: 10.1016/j.mrfmmm.2006.12.010
49. Curfs DM, Lutgens E, Gijbels MJ, Kockx MM, Daemen MJ, van Schooten FJ. Chronic exposure to the carcinogenic compound benzo[a]pyrene induces larger and phenotypically different atherosclerotic plaques in ApoE-knockout mice. Am J Pathol. (2004) 164:101–8. doi: 10.1016/S0002-9440(10)63101-X
50. Curfs DM, Knaapen AM, Pachen DM, Gijbels MJ, Lutgens E, Smook ML, et al. Polycyclic aromatic hydrocarbons induce an inflammatory atherosclerotic plaque phenotype irrespective of their DNA binding properties. FASEB J. (2005) 19:1290–2. doi: 10.1096/fj.04-2269fje
51. Sroczyńska K, Totoń-Zurańska J, Czepiel J, Zajac-Grabiec A, Jurczyszyn A, Wołkow P, et al. Therapeutic role of eicosapentaenoic and arachidonic acid in benzo(a) pyrene-induced toxicity in HUVEC endothelial cells. Life Sci. (2022) 293:120345. doi: 10.1016/j.lfs.2022.120345
52. Oesterling E, Toborek M, Hennig B. Benzo[a]pyrene induces intercellular adhesion molecule-1 through a caveolae and aryl hydrocarbon receptor mediated pathway. Toxicol Appl Pharmacol. (2008) 232:309–16. doi: 10.1016/j.taap.2008.07.001
53. Gu J, Chan LS, Wong CK, Wong NS, Wong CK, Leung KN, et al. Effect of benzo[a]pyrene on the production of vascular endothelial growth factor by human eosinophilic leukemia EoL-1 cells. J Environ Pathol Toxicol Oncol. (2011) 30:241–9. doi: 10.1615/JEnvironPatholToxicolOncol.v30.i3.70
54. Ji K, Xing C, Jiang F, Wang X, Guo H, Nan J, et al. Benzo[a]pyrene induces oxidative stress and endothelial progenitor cell dysfunction via the activation of the NF-κB pathway. Int J Mol Med. (2013) 31:922–30. doi: 10.3892/ijmm.2013.1288
55. van Grevenynghe J, Monteiro P, Gilot D, Fest T, Fardel O. Human endothelial progenitors constitute targets for environmental atherogenic polycyclic aromatic hydrocarbons. Biochem Biophys Res Commun. (2006) 341:763–9. doi: 10.1016/j.bbrc.2006.01.028
56. Tithof PK, Elgayyar M, Cho Y, Guan W, Fisher AB, Peters-Golden M. Polycyclic aromatic hydrocarbons present in cigarette smoke cause endothelial cell apoptosis by a phospholipase A2-dependent mechanism. FASEB J. (2002) 16:1463–4. doi: 10.1096/fj.02-0092fje
57. Tzeng HP, Lan KC, Yang TH, Chung MN, Liu SH. Benzo[a]pyrene activates interleukin-6 induction and suppresses nitric oxide-induced apoptosis in rat vascular smooth muscle cells. PLoS ONE. (2017) 12:e0178063. doi: 10.1371/journal.pone.0178063
58. Meng D, Lv DD, Zhuang X, Sun H, Fan L, Shi XL, et al. Benzo[a]pyrene induces expression of matrix metalloproteinases and cell migration and invasion of vascular smooth muscle cells. Toxicol Lett. (2009) 184:44–9. doi: 10.1016/j.toxlet.2008.10.016
59. Niermann T, Schmutz S, Erne P, Resink T. Aryl hydrocarbon receptor ligands repress T-cadherin expression in vascular smooth muscle cells. Biochem Biophys Res Commun. (2003) 300:943–9. doi: 10.1016/S0006-291X(02)02970-4
60. Yan Z, Subbaramaiah K, Camilli T, Zhang F, Tanabe T, McCaffrey TA, et al. Benzo[a]pyrene induces the transcription of cyclooxygenase-2 in vascular smooth muscle cells. Evidence for the involvement of extracellular signal-regulated kinase and NF-kappaB. J Biol Chem. (2000) 275:4949–55. doi: 10.1074/jbc.275.7.4949
61. Chen YH, Ramos KS. A CCAAT/enhancer-binding protein site within antioxidant/electrophile response element along with CREB-binding protein participate in the negative regulation of rat GST-Ya gene in vascular smooth muscle cells. J Biol Chem. (2000) 275:27366–76. doi: 10.1016/S0021-9258(19)61520-6
62. Miller KP, Chen YH, Hastings VL, Bral CM, Ramos KS. Profiles of antioxidant/electrophile response element (ARE/EpRE) nuclear protein binding and c-Ha-ras transactivation in vascular smooth muscle cells treated with oxidative metabolites of benzo[a]pyrene. Biochem Pharmacol. (2000) 60:1285–96. doi: 10.1016/S0006-2952(00)00439-1
63. Kerzee JK, Ramos KS. Activation of c-Ha-ras by benzo(a)pyrene in vascular smooth muscle cells involves redox stress and aryl hydrocarbon receptor. Mol Pharmacol. (2000) 58:152–8. doi: 10.1124/mol.58.1.152
64. Karyala S, Guo J, Sartor M, Medvedovic M, Kann S, Puga A, et al. Different global gene expression profiles in benzo[a]pyrene- and dioxin-treated vascular smooth muscle cells of AHR-knockout and wild-type mice. Cardiovasc Toxicol. (2004) 4:47–73. doi: 10.1385/CT:4:1:47
65. Raveendran M, Senthil D, Utama B, Shen Y, Dudley D, Wang J, et al. Cigarette suppresses the expression of P4Halpha and vascular collagen production. Biochem Biophys Res Commun. (2004) 323:592–8. doi: 10.1016/j.bbrc.2004.08.129
66. Yang H, Zhou L, Wang Z, Roberts LJ, Lin X, Zhao Y, et al. Overexpression of antioxidant enzymes in ApoE-deficient mice suppresses benzo(a)pyrene-accelerated atherosclerosis. Atherosclerosis. (2009) 207:51–8. doi: 10.1016/j.atherosclerosis.2009.03.052
67. Wang JC, Bennett M. Aging and atherosclerosis: mechanisms, functional consequences, and potential therapeutics for cellular senescence. Circ Res. (2012) 111:245–59. doi: 10.1161/CIRCRESAHA.111.261388
68. Luchetti F, Crinelli R, Nasoni MG, Benedetti S, Palma F, Fraternale A, et al. LDL receptors, caveolae and cholesterol in endothelial dysfunction: oxLDLs accomplices or victims? Br J Pharmacol. (2021) 178:3104–14. doi: 10.1111/bph.15272
69. Bennett MR, Sinha S, Owens GK. Vascular Smooth Muscle Cells in Atherosclerosis. Circ Res. (2016) 118:692–702. doi: 10.1161/CIRCRESAHA.115.306361
70. Hartman J, Frishman WH. Inflammation and atherosclerosis: a review of the role of interleukin-6 in the development of atherosclerosis and the potential for targeted drug therapy. Cardiol Rev. (2014) 22:147–51. doi: 10.1097/CRD.0000000000000021
71. Visse R, Nagase H. Matrix metalloproteinases and tissue inhibitors of metalloproteinases: structure, function, and biochemistry. Circ Res. (2003) 92:827–39. doi: 10.1161/01.RES.0000070112.80711.3D
72. Holme JA, Brinchmann BC, Refsnes M, Låg M, Øvrevik J. Potential role of polycyclic aromatic hydrocarbons as mediators of cardiovascular effects from combustion particles. Environ Health. (2019) 18:74. doi: 10.1186/s12940-019-0514-2
73. Lackland DT, Weber MA. Global burden of cardiovascular disease and stroke: hypertension at the core. Can J Cardiol. (2015) 31:569–71. doi: 10.1016/j.cjca.2015.01.009
74. Lau DH, Nattel S, Kalman JM, Sanders P. Modifiable Risk Factors and Atrial Fibrillation. Circulation. (2017) 136:583–96. doi: 10.1161/CIRCULATIONAHA.116.023163
75. Gentner NJ, Weber LP. Intranasal benzo[a]pyrene alters circadian blood pressure patterns and causes lung inflammation in rats. Arch Toxicol. (2011) 85:337–46. doi: 10.1007/s00204-010-0589-6
76. Jules GE, Pratap S, Ramesh A, Hood DB. In utero exposure to benzo(a)pyrene predisposes offspring to cardiovascular dysfunction in later-life. Toxicology. (2012) 295:56–67. doi: 10.1016/j.tox.2012.01.017
77. Bugiak B, Weber LP. Hepatic and vascular mRNA expression in adult zebrafish (Danio rerio) following exposure to benzo-a-pyrene and 2,3,7,8-tetrachlorodibenzo-p-dioxin. Aquat Toxicol. (2009) 95:299–306. doi: 10.1016/j.aquatox.2009.03.009
78. Wang J, Sun H, Zhou Y, Huang K, Que J, Peng Y, et al. RNA microarray expression profile in 3,4-benzopyrene/angiotensin II-induced abdominal aortic aneurysm in mice. J Cell Biochem. (2019) 120:10484–94. doi: 10.1002/jcb.28333
79. Zhang Y, Ramos KS. The development of abdominal aortic aneurysms in mice is enhanced by benzo(a)pyrene. Vasc Health Risk Manag. (2008) 4:1095–102. doi: 10.2147/VHRM.S3038
80. Ji K, Zhang Y, Jiang F, Qian L, Guo H, Hu J, et al. Exploration of the mechanisms by which 3,4-benzopyrene promotes angiotensin II-induced abdominal aortic aneurysm formation in mice. J Vasc Surg. (2014) 59:492–9. doi: 10.1016/j.jvs.2013.03.022
81. Pinel-Marie ML, Sparfel L, Desmots S, Fardel O. Aryl hydrocarbon receptor-dependent induction of the NADPH oxidase subunit NCF1/p47 phox expression leading to priming of human macrophage oxidative burst. Free Radic Biol Med. (2009) 47:825–34. doi: 10.1016/j.freeradbiomed.2009.06.025
82. Aboutabl ME, Zordoky BN, El-Kadi AO. 3-methylcholanthrene and benzo(a)pyrene modulate cardiac cytochrome P450 gene expression and arachidonic acid metabolism in male sprague dawley rats. Br J Pharmacol. (2009) 158:1808–19. doi: 10.1111/j.1476-5381.2009.00461.x
83. Greer JB, Magnuson JT, McGruer V, Qian L, Dasgupta S, Volz DC, et al. miR133b microinjection during early development targets transcripts of cardiomyocyte ion channels and induces oil-like cardiotoxicity in zebrafish (danio rerio) embryos. Chem Res Toxicol. (2021) 34:2209–15. doi: 10.1021/acs.chemrestox.1c00238
84. Jayasundara N, Van Tiem Garner L, Meyer JN, Erwin KN, Di Giulio RT. AHR2-Mediated transcriptomic responses underlying the synergistic cardiac developmental toxicity of PAHs. Toxicol Sci. (2015) 143:469–81. doi: 10.1093/toxsci/kfu245
85. Huang L, Wang C, Zhang Y, Li J, Zhong Y, Zhou Y, et al. Benzo[a]pyrene exposure influences the cardiac development and the expression of cardiovascular relative genes in zebrafish (Danio rerio) embryos. Chemosphere. (2012) 87:369–75. doi: 10.1016/j.chemosphere.2011.12.026
86. Bugiak BJ, Weber LP. Phenotypic anchoring of gene expression after developmental exposure to aryl hydrocarbon receptor ligands in zebrafish. Aquat Toxicol. (2010) 99:423–37. doi: 10.1016/j.aquatox.2010.06.003
87. Li CH, Cheng YW, Hsu YT, Hsu YJ, Liao PL, Kang JJ, et al. Benzo[a]pyrene inhibits angiogenic factors-induced alphavbeta3 integrin expression, neovasculogenesis, and angiogenesis in human umbilical vein endothelial cells. Toxicol Sci. (2010) 118:544–53. doi: 10.1093/toxsci/kfq279
88. Cunha V, Vogs C, Le Bihanic F, Dreij K. Mixture effects of oxygenated PAHs and benzo[a]pyrene on cardiovascular development and function in zebrafish embryos. Environ Int. (2020) 143:105913. doi: 10.1016/j.envint.2020.105913
89. Yamaguchi A, Uchida M, Ishibashi H, Hirano M, Ichikawa N, Arizono K, et al. Potential mechanisms underlying embryonic developmental toxicity caused by benzo[a]pyrene in Japanese medaka (Oryzias latipes). Chemosphere. (2020) 242:125243. doi: 10.1016/j.chemosphere.2019.125243
90. Ichihara S, Yamada Y, Gonzalez FJ, Nakajima T, Murohara T, Ichihara G, et al. Inhibition of ischemia-induced angiogenesis by benzo[a]pyrene in a manner dependent on the aryl hydrocarbon receptor. Biochem Biophys Res Commun. (2009) 381:44–9. doi: 10.1016/j.bbrc.2009.01.187
91. Li X, Shen C, Liu X, He J, Ding Y, Gao R, et al. Exposure to benzo[a]pyrene impairs decidualization and decidual angiogenesis in mice during early pregnancy. Environ Pollut. (2017) 222:523–31. doi: 10.1016/j.envpol.2016.11.029
92. Kent KC. Clinical practice. abdominal aortic aneurysms. N Engl J Med. (2014) 371:2101–8. doi: 10.1056/NEJMcp1401430
93. Durand P, Blondet A, Martin G, Carette D, Pointis G, Perrard MH. Effects of a mixture of low doses of atrazine and benzo[a]pyrene on the rat seminiferous epithelium either during or after the establishment of the blood-testis barrier in the rat seminiferous tubule culture model. Toxicol In Vitro. (2020) 62:104699. doi: 10.1016/j.tiv.2019.104699
94. Ho DH, Burggren WW. Blood-brain barrier function, cell viability, and gene expression of tight junction-associated proteins in the mouse are disrupted by crude oil, benzo[a]pyrene, and the dispersant COREXIT. Comp Biochem Physiol C Toxicol Pharmacol. (2019) 223:96–105. doi: 10.1016/j.cbpc.2019.05.011
95. Zhang L, Ji X, Ding F, Wu X, Tang N, Wu Q, et al. Apoptosis and blood-testis barrier disruption during male reproductive dysfunction induced by PAHs of different molecular weights. Environ Pollut. (2022) 300:118959. doi: 10.1016/j.envpol.2022.118959
96. Ba Q, Li J, Huang C, Qiu H, Li J, Chu R. Effects of benzo[a]pyrene exposure on human hepatocellular carcinoma cell angiogenesis, metastasis, and NF-κB signaling. Environ Health Perspect. (2015) 123:246–54. doi: 10.1289/ehp.1408524
97. Gdula-Argasińska J, Czepiel J, Totoń-Zurańska J, Jurczyszyn A, Wołkow P, Librowski T, et al. Resolvin D1 down-regulates CYP1A1 and PTGS2 gene in the HUVEC cells treated with benzo(a)pyrene. Pharmacol Rep. (2016) 68:939–44. doi: 10.1016/j.pharep.2016.05.005
98. Owens EO, Toborek M, Hennig B. Flavonoids protect against intercellular adhesion molecule-1 induction by benzo[a]pyrene. Bull Environ Contam Toxicol. (2009) 83:4–7. doi: 10.1007/s00128-009-9664-1
99. Duan J, Chen C, Li H, Ju G, Gao A, Sun Y, et al. Multifaceted protective effects of hesperidin by aromatic hydrocarbon receptor in endothelial cell injury induced by Benzo[a]Pyrene. Nutrients. (2022) 14:574. doi: 10.3390/nu14030574
100. Goff ML, Delbrut A, Quinton M, Pradelles R, Bescher M, Burel A, et al. Protective action of ostreococcus tauri and phaeodactylum tricornutum extracts towards benzo[a]pyrene-induced cytotoxicity in endothelial cells. Mar Drugs. (2019) 18. doi: 10.3390/md18010003
101. Bandi N, Ayalasomayajula SP, Dhanda DS, Iwakawa J, Cheng PW, Kompella UB, et al. Intratracheal budesonide-poly(lactide-co-glycolide) microparticles reduce oxidative stress, VEGF expression, and vascular leakage in a benzo(a)pyrene-fed mouse model. J Pharm Pharmacol. (2005) 57:851–60. doi: 10.1211/0022357056334
102. Aboutabl ME, Zordoky BN, Hammock BD, El-Kadi AO. Inhibition of soluble epoxide hydrolase confers cardioprotection and prevents cardiac cytochrome P450 induction by benzo(a)pyrene. J Cardiovasc Pharmacol. (2011) 57:273–81. doi: 10.1097/FJC.0b013e3182055baf
103. Agha AM, El-Fattah AA, Al-Zuhair HH, Al-Rikabi AC. Chemopreventive effect of Ginkgo biloba extract against benzo(a)pyrene-induced forestomach carcinogenesis in mice: amelioration of doxorubicin cardiotoxicity. J Exp Clin Cancer Res. (2001) 20:39–50.
104. Nakagawa M, Uno S, Iriyama N, Matsunawa M, Makishima M, Takeuchi J, et al. Combined treatment with benzo[a]pyrene and 1α,25-dihydroxyvitamin D(3) induces expression of plasminogen activator inhibitor 1 in monocyte/macrophage-derived cells. Toxicol Appl Pharmacol. (2018) 345:48–56. doi: 10.1016/j.taap.2018.03.003
105. Pink M, Verma N, Rettenmeier AW, Schmitz-Spanke S. Integrated proteomic and metabolomic analysis to assess the effects of pure and benzo[a]pyrene-loaded carbon black particles on energy metabolism and motility in the human endothelial cell line EA.hy926. Arch Toxicol. (2014) 88:913–34. doi: 10.1007/s00204-014-1200-3
106. Asweto CO, Wu J, Hu H, Feng L, Yang X, Duan J, et al. Combined effect of silica nanoparticles and benzo[a]pyrene on cell cycle arrest induction and apoptosis in human umbilical vein endothelial cells. Int J Environ Res Public Health. (2017) 14:289. doi: 10.3390/ijerph14030289
107. Duan J, Yu Y, Li Y, Wang Y, Sun Z. Inflammatory response and blood hypercoagulable state induced by low level co-exposure with silica nanoparticles and benzo[a]pyrene in zebrafish (Danio rerio) embryos. Chemosphere. (2016) 151:152–62. doi: 10.1016/j.chemosphere.2016.02.079
108. Asweto CO, Hu H, Liang S, Wang L, Liu M, Yang H, et al. Gene profiles to characterize the combined toxicity induced by low level co-exposure of silica nanoparticles and benzo[a]pyrene using whole genome microarrays in zebrafish embryos. Ecotoxicol Environ Saf. (2018) 163:47–55. doi: 10.1016/j.ecoenv.2018.07.059
Keywords: Benzo(a)pyrene, cardiovascular diseases, AhR, oxidative stress, inflammation, genotoxicity
Citation: Fu C, Li Y, Xi H, Niu Z, Chen N, Wang R, Yan Y, Gan X, Wang M, Zhang W, Zhang Y and Lv P (2022) Benzo(a)pyrene and cardiovascular diseases: An overview of pre-clinical studies focused on the underlying molecular mechanism. Front. Nutr. 9:978475. doi: 10.3389/fnut.2022.978475
Received: 26 June 2022; Accepted: 14 July 2022;
Published: 04 August 2022.
Edited by:
Jie Zheng, Jinan University, ChinaReviewed by:
Chunqing Guo, School of Medicine, Virginia Commonwealth University, United StatesHou-Zao Chen, Chinese Academy of Medical Sciences and Peking Union Medical College, China
Copyright © 2022 Fu, Li, Xi, Niu, Chen, Wang, Yan, Gan, Wang, Zhang, Zhang and Lv. This is an open-access article distributed under the terms of the Creative Commons Attribution License (CC BY). The use, distribution or reproduction in other forums is permitted, provided the original author(s) and the copyright owner(s) are credited and that the original publication in this journal is cited, in accordance with accepted academic practice. No use, distribution or reproduction is permitted which does not comply with these terms.
*Correspondence: Pin Lv, bHZwaW4mI3gwMDA0MDtoZWJtdS5lZHUuY24=; Yan Zhang, c25vd3dpbmdsdiYjeDAwMDQwOzEyNi5jb20=
†These authors have contributed equally to this work