- 1Department of Medical Surgical and Health Sciences, Medical Clinic, Cattinara Hospital, University of Trieste, Trieste, Italy
- 2Hospital Pharmacy, Cattinara Hospital, Azienda Sanitaria Universitaria Giuliano Isontina, Trieste, Italy
- 3Institute for Maternal and Child Health, Istituto di Ricovero e Cura a Carattere Scientifico (IRCCS) Burlo Garofolo, Trieste, Italy
- 4Department of Advanced Medical and Surgical Sciences, University of Campania L. Vanvitelli, Naples, Italy
- 5Institute of Space Physiology and Medicine (MEDES), Toulouse, France
Muscle inactivity leads to muscle atrophy. Leucine is known to inhibit protein degradation and to promote protein synthesis in skeletal muscle. We tested the ability of a high-protein diet enriched with branched-chain amino acids (BCAAs) to prevent muscle atrophy during long-term bed rest (BR). We determined body composition (using dual energy x-ray absorptiometry) at baseline and every 2-weeks during 60 days of BR in 16 healthy young women. Nitrogen (N) balance was assessed daily as the difference between N intake and N urinary excretion. The subjects were randomized into two groups: one received a conventional diet (1.1 ± 0.03 g protein/kg, 4.9 ± 0.3 g leucine per day) and the other a high protein, BCAA-enriched regimen (1.6 ± 0.03 g protein-amino acid/kg, 11.4 ± 0.6 g leucine per day). There were significant BR and BR × diet interaction effects on changes in lean body mass (LBM) and N balance throughout the experimental period (repeated measures ANCOVA). During the first 15 days of BR, lean mass decreased by 4.1 ± 0.9 and 2.4 ± 2.1% (p < 0.05) in the conventional and high protein-BCAA diet groups, respectively, while at the end of the 60-day BR, LBM decreased similarly in the two groups by 7.4 ± 0.7 and 6.8 ± 2.4%. During the first 15 days of BR, mean N balance was 2.5 times greater (p < 0.05) in subjects on the high protein-BCAA diet than in those on the conventional diet, while we did not find significant differences during the following time intervals. In conclusion, during 60 days of BR in females, a high protein-BCAA diet was associated with an early protein-LBM sparing effect, which ceased in the medium and long term.
Introduction
Physical inactivity or bed rest (BR) frequently occurs in patients affected by several diseases, but also during physiologic aging (1–3). Muscle inactivity leads to muscle atrophy, loss of bone mineral density and decreased insulin sensitivity (2, 4–10). Beyond its well-known functional-motor structural role, skeletal muscle represents a homeostatic “organ,” fundamental for the maintenance of whole-body metabolic control (11). Indeed, skeletal muscle mass regulates the amino acid balance of organs and tissues, as well as the availability of nutrients and metabolic precursors (11–13). Moreover, it has a central role in maintaining the general energy balance, through complex interplay mechanisms, and contributes to preserve the individual’s state of health and quality of life (11).
Together, with physical activity, nutrition has a fundamental role in homeostatic regulation and maintenance of muscle mass and function (14–16). Among nutrients, essential amino acids, occupy a central place, linked not only to the formation of proteins, but also to the control of numerous and complex subcellular pathways, fundamental for maintaining cellular energy balance and, ultimately, for survival itself (17–19). The action of essential amino acids on muscle, in addition to being of a direct type on specific regulatory factors (i.e., mammalian target of rapamycin, mTOR, complex 1), is also mediated by hormonal control (i.e., insulin, glucagon, cortisol), involved in anabolic and catabolic processes, through the regulation of transcriptional sequences (19, 20). Evidence indicates that high-protein diets and essential amino acid supplementation may ameliorate muscle protein loss in healthy volunteers during experimental BR (21, 22). In addition, protein/amino acid intake has been reported to modulate insulin signaling and β-cell function in “in vivo” experiments (23, 24). Branched-chain amino acids (BCAAs), i.e., leucine, valine, and isoleucine are essential amino acids, exhibiting selective effects not only on stimulation of muscle protein synthesis, but also on insulin mediated glucose uptake, moreover they play an important role in several metabolic and signaling functions, particularly via activation of the mTOR signaling pathway (25, 26). Among BCAAs, the most noteworthy effects have been observed with leucine (26). In particular, leucine: (a) is a constituent of proteins (27); (b) regulates protein synthesis translation initiation in skeletal muscle (28–30); (c) modulates insulin/phosphoinositide 3-kinase (PI3K) signal cascade (31); (d) is a fuel for skeletal muscle cells (32); (e) is a primary nitrogen donor for the production of alanine and glutamine in skeletal muscle (33); (f) modulates the pancreatic β-cell insulin release (34). All these diverse metabolic roles allow leucine to influence the rate of muscle protein synthesis, insulin secretion and glucose homeostasis (25, 35). Evidence indicates that leucine alone may exert and anabolic response (36), while no such data exists for isoleucine or valine, although isoleucine could potentially increase muscle growth by up-regulating the protein expression of GLUT1 and GLUT4 in muscle (26).
The aim of the present study was to assess the effects of a high-protein diet enriched with BCAAs on progression of lean body mass (LBM) atrophy over 2-months experimental BR in healthy young female volunteers as an experimental model of long-term physical inactivity. LBM was assessed approximately every 2 weeks by dual-energy X-ray absorptiometry (DXA). Whole-body protein kinetics were evaluated by nitrogen (N) balance before and during BR. Healthy volunteers who underwent a prolonged BR represent a good model to investigate the effects of muscle unloading on physiological functions (37).
Materials and methods
Subjects and study design
Sixteen medically and psychologically healthy females, aged 25–40 years (32 ± 4 years), participated in the Women’s International Space Simulation for Exploration (WISE)—2005 BR study. The study was completed in two campaigns (February 2005–May 2005, September 2005–December 2005). Non-smoking volunteers were recreationally active but athletically untrained, thus competitive athletes were excluded from the study. Included volunteers met the following criteria: a body mass index between 20 and 25 kg/m2, regular menstrual cycles, no intake of oral contraceptives in the 2 months before the study, no family history of chronic diseases or psychiatric disorders, and absence of musculoskeletal, orthopedic, blood clotting, and cardiovascular disorders. Mean weight and height of the subjects were 59 ± 4 kg and 166 ± 7 cm, respectively.
The procedures were conducted in accordance with the ethical principles stated in the Declaration of Helsinki 1964. The protocol was reviewed and approved by the local ethics committee (Comité Consultatif de Protection des Personnes dans la Recherche Biomédicale, CCPPRB—Toulouse 1: dossier n°1-04-16: 20/07/04—avis n°2: p.1), by the University of California, San Diego Institutional Review Board and by the Institutional Review Boards of the National Aeronautics and Space Administration, Johnson Space Center Committee for the Protection of Human Subjects. The whole study procedures was explained to the volunteers both verbally and in writing. All participants provided a written informed consent. The study was performed at the Institute for Space Medicine and Physiology in Toulouse, France. The whole study protocol is discussed in detail elsewhere (38, 39), briefly, it consisted in a long-term BR which included a 20-day ambulatory control period followed by 60 days of strict 6° head-down-tilt (HDT) BR (40). A 20-day ambulatory recovery period followed the BR period.
After a 20-day ambulatory adaptation to a controlled diet (i.e., conventional diet), subjects were randomized into two eucaloric diet groups (n = 8, each): a control group who continued the conventional diet and a protein group on a high protein diet enriched with BCAA. During the 20 days pre-BR (ambulatory period, AMB), resting metabolic rate was determined by indirect calorimetry using Deltatrac II (General Electric, Indianapolis, IN) according to the manufacturer. The prescribed caloric intake for the two groups was 140% of their resting metabolic rate during the pre-BR. During BR, energy intake was adjusted downward to 110% of resting metabolic rate both in the control (conventional diet) and protein (high protein-BCAA diet) groups. In the control group on the conventional diet, the prescribed daily protein intake was 1.1 ± 0.03 g⋅kg–1⋅day–1 of body weight; while, in the group on the high protein-BCAA diet, dietary protein content was increased to about 1.45 g⋅kg–1⋅day–1 and enriched with 0.15 g⋅kg–1⋅day–1 of BCAAs (leucine/valine/isoleucine = 2/1/1). Free BCAAs (3.6 g/day free leucine, 1.8 g/day free isoleucine, and 1.8 g/day free valine) were added as a supplement (Friliver, Bracco, Italy) during the three main meals. Thus, the total protein/amino acid daily intake for this group was of 1.6 ± 0.03 g⋅kg–1 body weight. To compensate for the additional increase in energy intake from protein in this group, carbohydrate content was reduced during the BR period. Fat mass was monitored every 15 days and maintained at basal levels by changing energy intake, if necessary. All subjects were always restricted to the HDT position except for mealtime when they were allowed to elevate on one elbow. Body weight, urine production, intake of fluids and body temperature were regularly monitored.
Body mass and composition
Body weight was measured daily during pre-BR and during BR. Lean and fat mass were determined by DXA with a Hologic QDR 4,500 W, Software Version 11.1 (Hologic, Bedford, MA). At baseline, measurements were obtained twice, respectively, on days −14 and −2 before the start of BR in each group. In the ambulatory condition DXA measurements obtained at days −14 and −2 were averaged. DXA scans were performed four times during the 60-day BR, respectively, on days 15, 31, 43, and 60 (days BR 15, BR 31, BR 43, and BR 60). DXA scans were executed in the morning in the fasting state. The bladder was emptied before the scan. The subjects had nothing to eat or drink after dinner the night before.
Nitrogen balance during the bed rest
Nitrogen (N) balance reflects the equilibrium between protein N intake and N losses to define anabolic and catabolic conditions of whole-body protein kinetics (i.e., difference between rates of synthesis and degradation). N balance is calculated as the difference between N intake and N losses. In this study, N balance was estimated from the difference between N protein/amino acid intake and urinary N excretion (41), with the addition of a constant value of 4 g/day to account for N losses from the skin and feces (41, 42). A total of 24-h urine samples were collected, aliquoted in 10 mL samples and at −20°C. Urinary N from these aliquots was measured by chemo-luminescence (Antek 7000, Antek Instruments, U.S.) in an accredited laboratory (Central Biochemical Laboratory, Université de Lyon, Lyon, France). Protein intake was assessed by entering all food eaten including all ingredients used to prepare complex recipes, into the Nutrilog Edition Expert software, version 2.0 (Marans, France). All food and leftovers were weighed individually. N balance [g] was then calculated daily according to the following equation: N balance = (24-h protein/BCAA intake/6.25) – (24-h urinary urea N + 4). In the ambulatory condition N balance of days −1 and −2 were averaged. During the BR period the average daily N balance values were calculated in the time intervals between consecutive LBM measurements. Values of N balance were expressed as mg/kg LBM per day.
Data and statistical analysis
The numerical data are presented as means ± standard deviation (SD). The differences between pre-BR and during 60-days BR were analyzed by repeated-measures ANCOVA with time (AMB or BR days) and diet (conventional or high protein-BCAA diet) as the two factors using ambulatory values as covariates. Post hoc analysis was performed, when appropriate, by using paired t-test or Mann-Whitney test with Bonferroni’s adjustments. Levene’s test showed normal distribution and equal variance of ambulatory values of LBM, N intake, N excretion and N balance in the two groups. All p-values were considered significant when < 0.05. All analyses were performed using the IBM SPSS statistic 21 software (Version 21.0, SPSS Inc., Chicago, IL, USA).
Results
Table 1 shows mean values of nutrient intakes in the AMB and BR periods in the conventional and high protein-BCAA diet groups. During BR, energy intake decreased by about 8% in both groups. Protein intake increased during BR by about 11 and 53% in the conventional and high protein-BCAA diet groups, respectively. Leucine intake increased by about 100% during the BR period in the high protein-BCAA diet group.
Changes in body weight and composition during the 60-day BR are shown in Table 2. Body weight significantly decreased following BR. At the end of the BR period body weight decreased by 5.9 ± 1.1% in the conventional diet group and by 4.1 ± 1.7% in the high protein-BCAA diet group (p = 0.01). Fat mass did not change significantly during the BR period in both groups. Lean mass significantly decreased during the first 31 days of BR and did not change during the following 29 days of BR, both in the high protein-BCAA and the conventional diet groups. There were significant BR effect and BR × diet interaction on changes in LBM.
After 15 days from the beginning of the BR, the decrease in lean mass was about twice greater in the conventional diet group than that in the high protein-BCAA diet group (Figure 1). Nonetheless, at the end of the 60 days of BR the total changes in LBM were similar in the two groups.
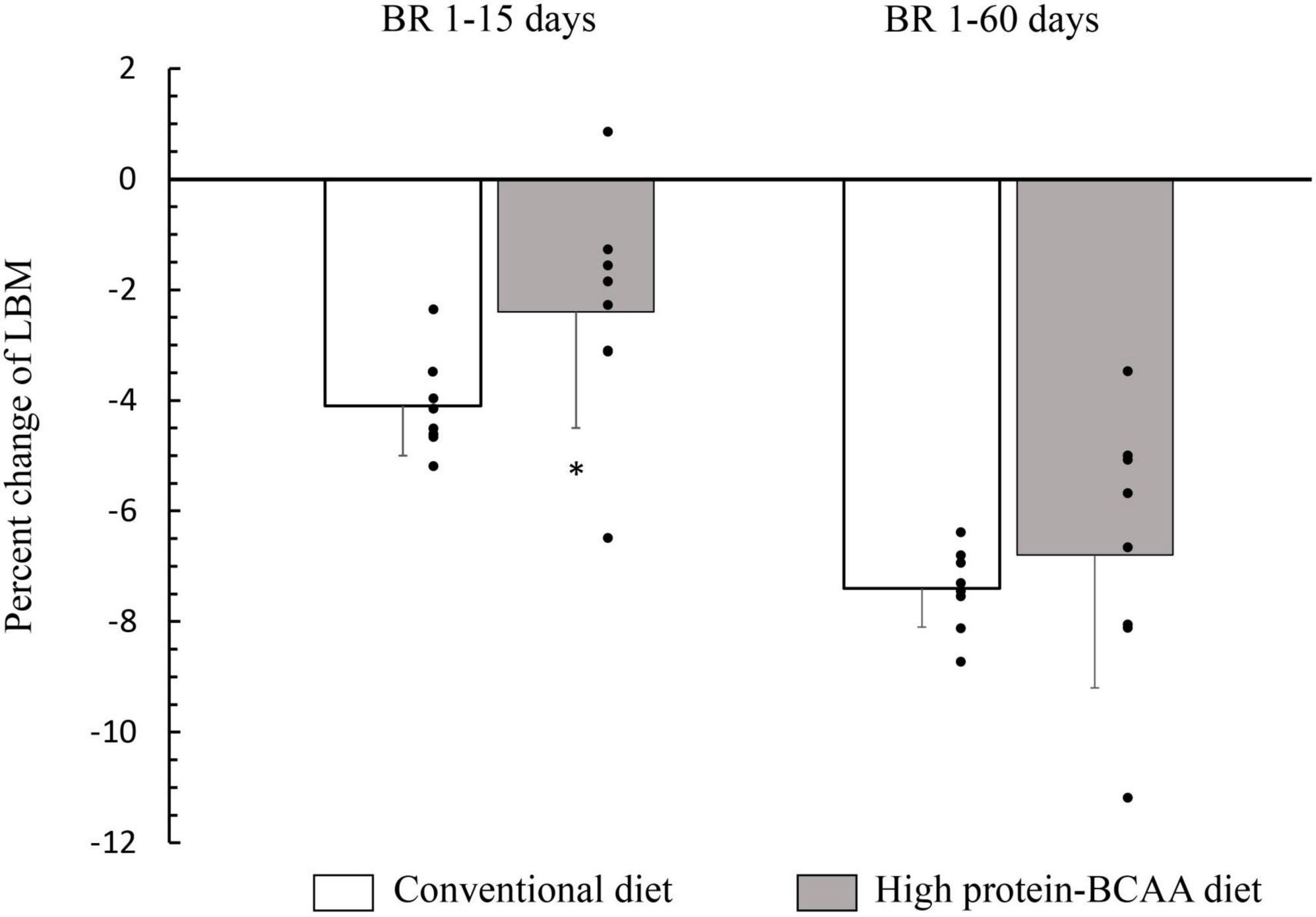
Figure 1. Effects of high protein-BCAA diet on lean body mass (LBM) during the first 15 days and during entire bed rest (BR) period of 60 days. All data were expressed as means ± SD. Individual data are additionally shown. All data were analyzed by repeated-measures ANCOVA with time (BR 1–15 days or BR 1–60 days) and diet (conventional or high protein-BCAA diet) as the two factors. BR effect, p < 0.001; BR × diet interaction, p = 0.036. Post hoc analysis was performed by using Mann-Whitney test with Bonferroni’s adjustment. *p < 0.05, high protein-BCAA vs. normal protein (conventional diet); SD, standard deviation.
As shown in Table 3, during the BR period N intake and urine N excretion were 55 ± 8 and 52 ± 1% greater in subjects on the high protein-BCAA diet than in those on the conventional diet. There were significant BR effect and BR × diet interaction on N balance in both groups. Between the 1st and the 15th day of BR, N balance was significantly greater in the high protein-BCAA diet group than in the conventional diet group, while we did not find significant differences during the following time intervals.
Discussion
We have studied healthy young women during 60 days of BR in eucaloric conditions at different levels of protein intake. In the high protein-BCAA and conventional diet groups protein/amino acid intakes were about 1.6 and 1.1 g⋅kg–1⋅day–1, respectively. The high protein diet was enriched with 0.15 g⋅kg–1⋅day–1 of BCAAs with the following BCAA proportions, leucine/valine/isoleucine: 2/1/1. The diet high in protein and BCAA caused a slowing down of the loss of lean mass secondary to BR of about 42%, only in the first 15 days of inactivity. This lean mass saving effect was abolished in the later stages of BR. In fact, at the end of long-BR the total changes in LBM were similar in the two groups. The results of N balance agreed with changes in LBM.
The subjects were in energy balance during the whole study period as demonstrated by the absence of significant changes in fat mass. This finding is important as our previous studies showed that a positive or negative energy balance accelerated BR-induced muscle atrophy (43, 44). In fact, we have demonstrated that during 5 weeks of BR a positive energy balance was associated with greater loss of LBM and activation of the systemic inflammatory response and antioxidant defenses (44–48). Several pieces of literature provide evidence to support the role of inflammation in impairing muscle homeostasis with a consequent loss of skeletal muscle (49–51). We have also shown that a negative energy balance (hypocaloric nutrition) during 2 weeks of BR leads to a greatest wasting of LBM by increasing protein catabolism in the postabsorptive period and by impairing postprandial anabolic utilization of free amino acids (43).
Muscle atrophy depends on an imbalance between muscle protein synthesis and breakdown. It has been suggested that disuse atrophy in humans is caused mainly by a decreased basal protein synthesis, with no changes in protein degradation, at least in the early stages of BR or immobilization (52–54). In fact, the rate of basal protein synthesis declines immediately after unloading and stays at a suppressed level for the duration of the disuse (52–59). The most important role in the loss of muscle mass during a period of disuse has been explained elsewhere (60, 61) as a decline in both post-absorptive and postprandial muscle protein synthesis rates. This response now called anabolic resistance, is a state of diminished muscle protein synthesis, despite provision of an adequate amount of essential amino acids to elicit an appropriate synthesis response (54, 60–66). In addition, during limb immobilization and BR, a decreased post-absorptive muscle protein synthesis has been reported (55, 60, 62, 67, 68).
In our study, we speculated that the beneficial effect of a high protein-BCAA diet on LBM in the early phase of BR was due to leucine supplementation while maintaining an adequate intake of protein and of the other BCAAs, valine and isoleucine. In fact, several studies have shown that leucine, considered a strong stimulator of protein synthesis (30, 69, 70), has anabolic effects on protein metabolism by increasing the rate of protein synthesis and by decreasing the rate of protein degradation in resting human muscle (26, 30, 35, 71–73). On one hand, leucine increases muscle protein synthesis by modulating the activation of mTOR signaling pathway (25, 74, 75), on the other it reduces protein degradation by regulating autophagy through the acetyl-coenzyme on mTOR complex 1 and by diminishing oxidative stress (76, 77).
In the current study, we have demonstrated that an anti-catabolic action of the high protein-BCAA diet ceases after 15 days (Figure 1). In agreement with our study, English et al. have demonstrated that a diet with leucine supplementation (0.06 g/kg per meal) protected against muscle loss after 7-day BR but not after 14 days in middle-aged males; showing that the beneficial effects of leucine supplementation may not be maintained through a prolonged muscle disuse (78). It is not clear why the anabolic action on protein metabolism by chronic leucine supplementation, is not maintained for prolonged periods, despite its powerful effect on acute muscle protein synthesis.
Among several potential mechanisms, including a leucine “desynchronization effect” (63) one possibility is that beyond a certain level, excess of leucine may stimulate key enzymes in BCAA catabolism (i.e., BCAA aminotransferase and branched-chain alpha-keto acid dehydrogenase) thus increasing the oxidation of serum leucine (79, 80). Moreover, a surplus in leucine intake can decrease the plasma concentration of the other EAAs, an effect called BCAA antagonism (81–83). Further investigations are needed to confirm the “desynchronization effect” hypothesis. During immobilization/BR or aging, another important mechanism explaining a reduced muscle protein synthesis, and subsequent smaller increases in lean mass in response to protein feeding, may be due to a decreased amino acid transporter expression. Among them, the large neutral amino acid transporter 1 (LAT1), which preferentially transports leucine and the other BCAAs into the cells, together with the sodium-coupled neutral amino acid transporter 2 (SNAT2), have been shown to activate mTOR signaling (84). In physiological condition, these important amino acid transporters (LAT1 and SNAT2) are sensitive to changes in nutrient status and are associated with activation of mTOR signaling and muscle protein synthesis (85). In fact, mRNA expression and protein content of LAT1 and SNAT2 are increased by EAAs and resistance exercise (86, 87). However, Drummond et al. also found that the EAA-induced increase in LAT1 and SNAT2 proteins was abolished by 7 days of BR (88). Therefore, we hypothesized that the loss of effectiveness of the high protein-BCAA diet could be associated with a decreased muscle protein synthesis by a mechanism involving reduced mTOR signaling pathway, and amino acid transporter expression, thus causing a “desynchronization effect” of leucine in the first 15 days of inactivity. Taken together, these findings suggest limited effect of increasing protein supplementation with EAAs, leucine or BCAAs in offsetting muscle loss in states of long-term BR or leg immobilization, as disuse models.
N balance has been traditionally used to estimate whole body protein balance in response to nutritional interventions (41). In this study, we have shown a consistency of the results obtained every 15 days with DXA scan measurements with those obtained with daily monitoring of the N balance. In order to compare N balance and DXA results, the average daily N balance values were calculated in the time intervals between consecutive LBM measurements. Results of N balance were consistent with those of DXA. During the first 15 days of BR, as compared with the conventional diet group, the high protein-BCAA group exhibited 42% lower LBM loss and 2.5 times greater N balance. During the remaining part of the study, no significant differences were observed between the two groups in both lean mass loss and N balance. Koyama et al. have previously compared changes in lean tissue mass measured by DXA with N balance studies in obese women, studied over two periods of treatment with a very low-energy diet (89). There was a moderate correlation between the changes in lean mass measured by the two methods (r = 0.40, p < 0.05) (89).
Limitations of our study are that only women were investigated and that sample size for each group was small (n = 8 per group, conventional vs. high protein-BCAA diets). Therefore, further studies should have a cross design and include larger sample sizes to investigate if there is a difference between sex that could affect the potential ergogenic benefit provided by leucine supplementation during BR. The results of the WISE—2005 study in women, were compared to those derived from many studies on long-term BR (55 days and more) in males (90–95). This is important to evaluate any sex differences in muscle atrophy and in the effectiveness of countermeasures. In a recent review, Gao and Chilibeck examined the results of previous nutritional interventions during BR for prevention of muscle loss (96). Findings were mixed, among the 11 protein/amino acid supplementation studies included in the review, 4 failed to find any beneficial effects on muscle mass (96). These discrepancies have been attributed to differences in dietary protein quantity and quality (96). Therefore, the difference between diet groups in our study may be due to differences in leucine content or in protein intake between groups (1.1 g⋅kg–1⋅day–1 vs. 1.45 g⋅kg–1⋅day–1). In fact, we believe that the apparent heterogeneity between studies (especially in short-term BR) could be the consequence of differences in the diet composition (quantity of protein), experimental models (i.e., leg immobilization vs. bed rest) and bed rest duration rather that to sex-related factors and age (Table 4) (38, 41, 78, 97–106). In addition, as referred by Stein and Blanc, baseline protein intake was different between studies failing to report a beneficial effect and those finding a positive effect (107).
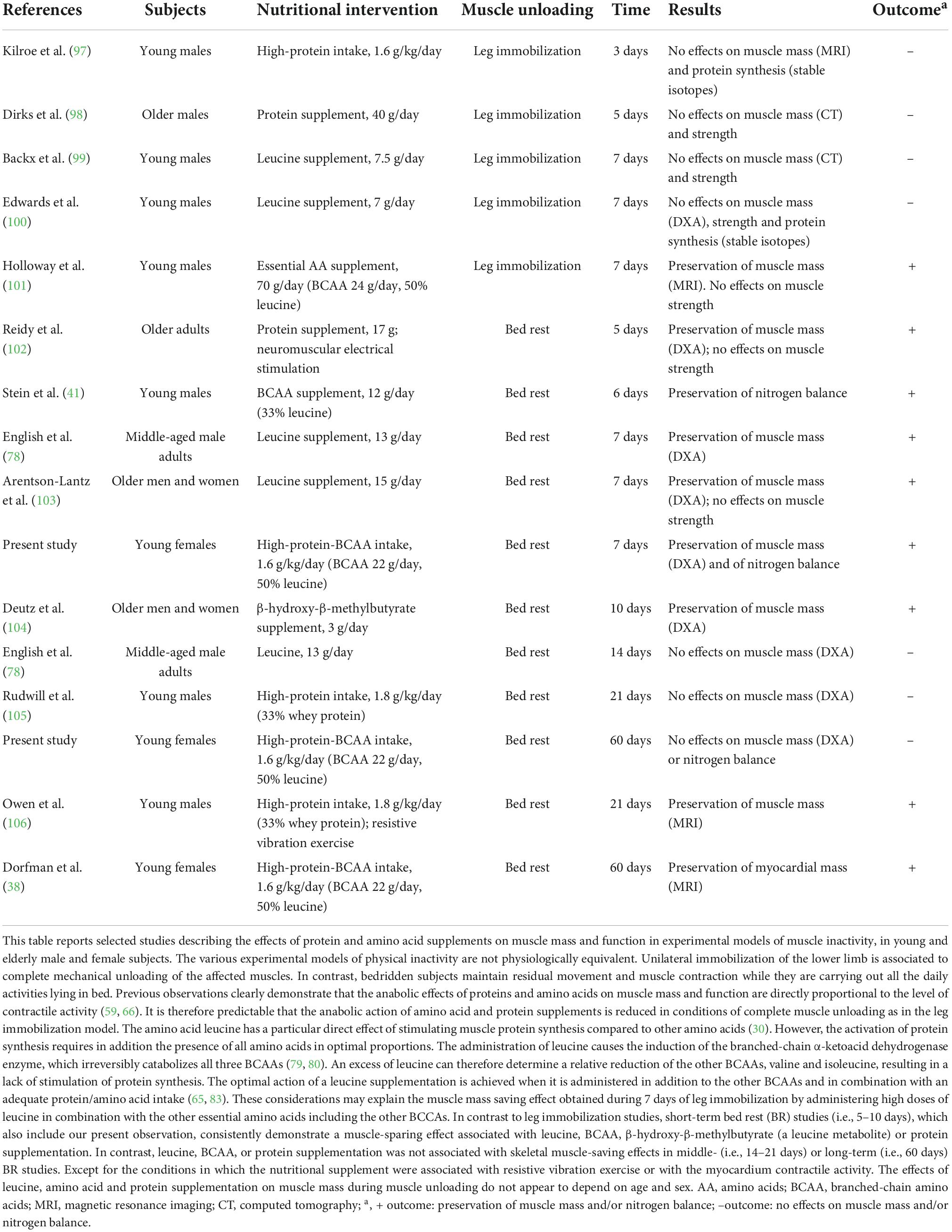
Table 4. Effects of amino acid and protein supplements on muscle mass and function in different models of muscle unloading in healthy volunteers of different ages and sex.
From the results of our study, it can be assumed that it is useful to administer a high-protein diet with BCAAs in the short-term BR, such as in acute illness of short duration, whereas in long-lasting pathological conditions, this nutritional approach appears useless. Nevertheless, a limitation of this study is that it has been carried out in healthy participants free of medical conditions that may exacerbate muscle loss. Prolonged immobility is harmful with rapid reductions in muscle mass, bone mineral density and impairment in other body systems. These effects are further exacerbated in individuals with critical illness. From the results of our study, we believe that if BR is absolutely recommended, as clinical intervention for a variety of health problems, a high-protein diet strategy could be helpful in mitigating short-term disuse muscle atrophy.
Data availability statement
The raw data supporting the conclusions of this article will be made available by the authors, without undue reservation.
Ethics statement
The studies involving human participants were reviewed and approved by the Institutional Review Boards of National Aeronautics and Space Administration, Johnson Space Center Committee for the Protection of Human Subjects, the University of California-San Diego Institutional Review Board, and Comité consultatif de Protection des Personnes dans la Recherche Biomédicale of Toulouse, France. The patients/participants provided their written informed consent to participate in this study.
Author contributions
GB, FDG, and AM designed the study, analyzed the results, and wrote the manuscript. GB, PV, NF, and M-PB provided resources, expertise, and critically reviewed the manuscript. FM, AN, and MG prepared the figures and tables and edited the manuscript. All authors contributed to the article and approved the submitted version.
Funding
This study, Women International Space Simulation for Exploration (WISE), was sponsored by the European Space Agency and the Italian Space Agency (ASI). This study has been performed by MEDES, Institute for Space Physiology and Medicine in Toulouse, France.
Acknowledgments
We thank Mariella Sturma for her assistance in laboratory analysis. Moreover, we thank the nurses, staff, and entire research team at the MEDES Space Clinic (Toulouse Rangueil Hospital) for their exceptional work. Finally, we thank the outstanding women who volunteered for this bed rest investigation.
Conflict of interest
The authors declare that the research was conducted in the absence of any commercial or financial relationships that could be construed as a potential conflict of interest.
Publisher’s note
All claims expressed in this article are solely those of the authors and do not necessarily represent those of their affiliated organizations, or those of the publisher, the editors and the reviewers. Any product that may be evaluated in this article, or claim that may be made by its manufacturer, is not guaranteed or endorsed by the publisher.
References
1. Biolo G, De Cicco M, Lorenzon S, Dal Mas V, Fantin D, Paroni R, et al. Treating hyperglycemia improves skeletal muscle protein metabolism in cancer patients after major surgery. Crit Care Med. (2008) 36:1768–75. doi: 10.1097/CCM.0b013e318174de32
2. Bauer J, Biolo G, Cederholm T, Cesari M, Cruz-Jentoft AJ, Morley JE, et al. Evidence-based recommendations for optimal dietary protein intake in older people: a position paper from the PROT-AGE Study Group. J Am Med Dir Assoc. (2013) 14:542–59. doi: 10.1016/j.jamda.2013.05.021
3. Di Girolamo FG, Fiotti N, Milanovic Z, Situlin R, Mearelli F, Vinci P, et al. The aging muscle in experimental bed rest: a systematic review and meta-analysis. Front Nutr. (2021) 8:633987. doi: 10.3389/fnut.2021.633987
4. Biolo G, Antonione R, Barazzoni R, Zanetti M, Guarnieri G. Mechanisms of altered protein turnover in chronic diseases: a review of human kinetic studies. Curr Opin Clin Nutr Metab Care. (2003) 6:55–63. doi: 10.1097/00075197-200301000-00009
5. Hamburg NM, McMackin CJ, Huang AL, Shenouda SM, Widlansky ME, Schulz E, et al. Physical inactivity rapidly induces insulin resistance and microvascular dysfunction in healthy volunteers. Arterioscler Thromb Vasc Biol. (2007) 27:2650–6. doi: 10.1161/ATVBAHA.107.153288
6. Ferrucci L, Baroni M, Ranchelli A, Lauretani F, Maggio M, Mecocci P, et al. Interaction between bone and muscle in older persons with mobility limitations. Curr Pharm Des. (2014) 20:3178–97. doi: 10.2174/13816128113196660690
7. Biolo G, Cederholm T, Muscaritoli M. Muscle contractile and metabolic dysfunction is a common feature of sarcopenia of aging and chronic diseases: from sarcopenic obesity to cachexia. Clin Nutr. (2014) 33:737–48. doi: 10.1016/j.clnu.2014.03.007
8. Barazzoni R, Deutz NEP, Biolo G, Bischoff S, Boirie Y, Cederholm T, et al. Carbohydrates and insulin resistance in clinical nutrition: recommendations from the ESPEN expert group. Clin Nutr. (2017) 36:355–63. doi: 10.1016/j.clnu.2016.09.010
9. Di Girolamo FG, Situlin R, Biolo G. What factors influence protein synthesis and degradation in critical illness? Curr Opin Clin Nutr Metab Care. (2017) 20:124–30. doi: 10.1097/MCO.0000000000000347
10. Di Girolamo FG, Fiotti N, Sisto UG, Nunnari A, Colla S, Mearelli F, et al. Skeletal muscle in hypoxia and inflammation: insights on the covid-19 pandemic. Front Nutr. (2022) 9:865402. doi: 10.3389/fnut.2022.865402
11. Mukund K, Subramaniam S. Skeletal muscle: a review of molecular structure and function, in health and disease. Wiley Interdiscip Rev Syst Biol Med. (2020) 12:e1462. doi: 10.1002/wsbm.1462
12. Kim KM, Jang HC, Lim S. Differences among skeletal muscle mass indices derived from height-, weight-, and body mass index-adjusted models in assessing sarcopenia. Korean J Intern Med. (2016) 31:643–50. doi: 10.3904/kjim.2016.015
13. Biolo G, Pisot R, Mazzucco S, Di Girolamo FG, Situlin R, Lazzer S, et al. Anabolic resistance assessed by oral stable isotope ingestion following bed rest in young and older adult volunteers: relationships with changes in muscle mass. Clin Nutr. (2017) 36:1420–6. doi: 10.1016/j.clnu.2016.09.019
14. Deutz NE, Bauer JM, Barazzoni R, Biolo G, Boirie Y, Bosy-Westphal A, et al. Protein intake and exercise for optimal muscle function with aging: recommendations from the ESPEN Expert Group. Clin Nutr. (2014) 33:929–36. doi: 10.1016/j.clnu.2014.04.007
15. Argiles JM, Campos N, Lopez-Pedrosa JM, Rueda R, Rodriguez-Manas L. Skeletal muscle regulates metabolism via interorgan crosstalk: roles in health and disease. J Am Med Dir Assoc. (2016) 17:789–96. doi: 10.1016/j.jamda.2016.04.019
16. Di Girolamo FG, Guadagni M, Fiotti N, Situlin R, Biolo G. Contraction and nutrition interaction promotes anabolism in cachectic muscle. Curr Opin Clin Nutr Metab Care. (2019) 22:60–7. doi: 10.1097/MCO.0000000000000527
17. Broer S, Broer A. Amino acid homeostasis and signalling in mammalian cells and organisms. Biochem J. (2017) 474:1935–63. doi: 10.1042/BCJ20160822
18. Di Girolamo FG, Situlin R, Fiotti N, Tence M, De Colle P, Mearelli F, et al. Higher protein intake is associated with improved muscle strength in elite senior athletes. Nutrition. (2017) 42:82–6. doi: 10.1016/j.nut.2017.05.003
19. Takahara T, Amemiya Y, Sugiyama R, Maki M, Shibata H. Amino acid-dependent control of mTORC1 signaling: a variety of regulatory modes. J Biomed Sci. (2020) 27:87. doi: 10.1186/s12929-020-00679-2
20. Cucca A, Mazzucco S, Bursomanno A, Antonutti L, Di Girolamo FG, Pizzolato G, et al. Amino acid supplementation in l-dopa treated Parkinson’s disease patients. Clin Nutr. (2015) 34:1189–94. doi: 10.1016/j.clnu.2014.12.007
21. Stein TP, Donaldson MR, Leskiw MJ, Schluter MD, Baggett DW, Boden G. Branched-chain amino acid supplementation during bed rest: effect on recovery. J Appl Physiol. (2003) 94:1345–52. doi: 10.1152/japplphysiol.00481.2002
22. Paddon-Jones D, Sheffield-Moore M, Urban RJ, Sanford AP, Aarsland A, Wolfe RR, et al. Essential amino acid and carbohydrate supplementation ameliorates muscle protein loss in humans during 28 days bedrest. J Clin Endocrinol Metab. (2004) 89:4351–8. doi: 10.1210/jc.2003-032159
23. Newsholme P, Krause M. Nutritional regulation of insulin secretion: implications for diabetes. Clin Biochem Rev. (2012) 33:35–47.
24. Newsholme P, Cruzat V, Arfuso F, Keane K. Nutrient regulation of insulin secretion and action. J Endocrinol. (2014) 221:R105–20. doi: 10.1530/JOE-13-0616
25. Holecek M. Branched-chain amino acids in health and disease: metabolism, alterations in blood plasma, and as supplements. Nutr Metab. (2018) 15:33. doi: 10.1186/s12986-018-0271-1
26. Zhang S, Zeng X, Ren M, Mao X, Qiao S. Novel metabolic and physiological functions of branched chain amino acids: a review. J Anim Sci Biotechnol. (2017) 8:10. doi: 10.1186/s40104-016-0139-z
27. Nie C, He T, Zhang W, Zhang G, Ma X. Branched chain amino acids: beyond nutrition metabolism. Int J Mol Sci. (2018) 19:954. doi: 10.3390/ijms19040954
28. Kimball SR, Jefferson LS. New functions for amino acids: effects on gene transcription and translation. Am J Clin Nutr. (2006) 83:500S–7S. doi: 10.1093/ajcn/83.2.500S
29. Boutry C, El-Kadi SW, Suryawan A, Wheatley SM, Orellana RA, Kimball SR, et al. Leucine pulses enhance skeletal muscle protein synthesis during continuous feeding in neonatal pigs. Am J Physiol Endocrinol Metab. (2013) 305:E620–31. doi: 10.1152/ajpendo.00135.2013
30. Ham DJ, Caldow MK, Lynch GS, Koopman R. Leucine as a treatment for muscle wasting: a critical review. Clin Nutr. (2014) 33:937–45. doi: 10.1016/j.clnu.2014.09.016
31. Peyrollier K, Hajduch E, Blair AS, Hyde R, Hundal HS. L-leucine availability regulates phosphatidylinositol 3-kinase, p70 S6 kinase and glycogen synthase kinase-3 activity in L6 muscle cells: evidence for the involvement of the mammalian target of rapamycin (mTOR) pathway in the L-leucine-induced up-regulation of system A amino acid transport. Biochem J. (2000) 350(Pt 2.):361–8.
32. Rennie MJ, Bohe J, Smith K, Wackerhage H, Greenhaff P. Branched-chain amino acids as fuels and anabolic signals in human muscle. J Nutr. (2006) 136(1 Suppl.):264S–8S. doi: 10.1093/jn/136.1.264S
33. van Zanten AR. Glutamine and antioxidants: status of their use in critical illness. Curr Opin Clin Nutr Metab Care. (2015) 18:179–86. doi: 10.1097/MCO.0000000000000152
34. Yang J, Chi Y, Burkhardt BR, Guan Y, Wolf BA. Leucine metabolism in regulation of insulin secretion from pancreatic beta cells. Nutr Rev. (2010) 68:270–9. doi: 10.1111/j.1753-4887.2010.00282.x
35. Nair KS, Short KR. Hormonal and signaling role of branched-chain amino acids. J Nutr. (2005) 135(6 Suppl.):1547S–52S. doi: 10.1093/jn/135.6.1547S
36. Wilkinson DJ, Hossain T, Hill DS, Phillips BE, Crossland H, Williams J, et al. Effects of leucine and its metabolite beta-hydroxy-beta-methylbutyrate on human skeletal muscle protein metabolism. J Physiol. (2013) 591:2911–23. doi: 10.1113/jphysiol.2013.253203
37. Biolo G, Heer M, Narici M, Strollo F. Microgravity as a model of ageing. Curr Opin Clin Nutr Metab Care. (2003) 6:31–40. doi: 10.1097/00075197-200301000-00006
38. Dorfman TA, Levine BD, Tillery T, Peshock RM, Hastings JL, Schneider SM, et al. Cardiac atrophy in women following bed rest. J Appl Physiol. (2007) 103:8–16. doi: 10.1152/japplphysiol.01162.2006
39. Jost PD. Simulating human space physiology with bed rest. Hippokratia. (2008) 12(Suppl 1.):37–40.
40. Hargens AR, Vico L. Long-duration bed rest as an analog to microgravity. J Appl Physiol. (2016) 120:891–903. doi: 10.1152/japplphysiol.00935.2015
41. Stein TP, Schluter MD, Leskiw MJ, Boden G. Attenuation of the protein wasting associated with bed rest by branched-chain amino acids. Nutrition. (1999) 15:656–60. doi: 10.1016/s0899-9007(99)00120-3
42. Irwin MI, Hegsted DM. A conspectus of research on protein requirements of man. J Nutr. (1971) 101:387–429. doi: 10.1093/jn/101.3.385
43. Biolo G, Ciocchi B, Stulle M, Bosutti A, Barazzoni R, Zanetti M, et al. Calorie restriction accelerates the catabolism of lean body mass during 2 wk of bed rest. Am J Clin Nutr. (2007) 86:366–72. doi: 10.1093/ajcn/86.2.366
44. Biolo G, Agostini F, Simunic B, Sturma M, Torelli L, Preiser JC, et al. Positive energy balance is associated with accelerated muscle atrophy and increased erythrocyte glutathione turnover during 5 wk of bed rest. Am J Clin Nutr. (2008) 88:950–8. doi: 10.1093/ajcn/88.4.950
45. Di Girolamo FG, Mazzucco S, Situlin R, Mohorko N, Jenko-Praznikar Z, Petelin A, et al. Roasting intensity of naturally low-caffeine Laurina coffee modulates glucose metabolism and redox balance in humans. Nutrition. (2016) 32:928–36. doi: 10.1016/j.nut.2016.02.001
46. Biolo G, Di Girolamo FG, McDonnell A, Fiotti N, Mearelli F, Situlin R, et al. Effects of hypoxia and bed rest on markers of cardiometabolic risk: compensatory changes in circulating trail and glutathione redox capacity. Front Physiol. (2018) 9:1000. doi: 10.3389/fphys.2018.01000
47. Biolo G, Massolino B, Di Girolamo FG, Fiotti N, Mearelli F, Mazzucco S, et al. Intensive insulin therapy increases glutathione synthesis rate in surgical ICU patients with stress hyperglycemia. PLoS One. (2018) 13:e0190291. doi: 10.1371/journal.pone.0190291
48. Biolo G, Di Girolamo FG, Heer M, Sturma M, Mazzucco S, Agostini F, et al. Alkalinization with potassium bicarbonate improves glutathione status and protein kinetics in young volunteers during 21-day bed rest. Clin Nutr. (2019) 38:652–9. doi: 10.1016/j.clnu.2018.04.006
49. Londhe P, Guttridge DC. Inflammation induced loss of skeletal muscle. Bone. (2015) 80:131–42. doi: 10.1016/j.bone.2015.03.015
50. Costamagna D, Costelli P, Sampaolesi M, Penna F. Role of inflammation in muscle homeostasis and myogenesis. Mediators Inflamm. (2015) 2015:805172. doi: 10.1155/2015/805172
51. Guadagni M, Biolo G. Effects of inflammation and/or inactivity on the need for dietary protein. Curr Opin Clin Nutr Metab Care. (2009) 12:617–22. doi: 10.1097/MCO.0b013e32833193bd
52. Phillips SM, Glover EI, Rennie MJ. Alterations of protein turnover underlying disuse atrophy in human skeletal muscle. J Appl Physiol. (2009) 107:645–54. doi: 10.1152/japplphysiol.00452.2009
53. Brocca L, Cannavino J, Coletto L, Biolo G, Sandri M, Bottinelli R, et al. The time course of the adaptations of human muscle proteome to bed rest and the underlying mechanisms. J Physiol. (2012) 590:5211–30. doi: 10.1113/jphysiol.2012.240267
54. Kilroe SP, Fulford J, Holwerda AM, Jackman SR, Lee BP, Gijsen AP, et al. Short-term muscle disuse induces a rapid and sustained decline in daily myofibrillar protein synthesis rates. Am J Physiol Endocrinol Metab. (2020) 318:E117–30. doi: 10.1152/ajpendo.00360.2019
55. de Boer MD, Selby A, Atherton P, Smith K, Seynnes OR, Maganaris CN, et al. The temporal responses of protein synthesis, gene expression and cell signalling in human quadriceps muscle and patellar tendon to disuse. J Physiol. (2007) 585(Pt 1.):241–51. doi: 10.1113/jphysiol.2007.142828
56. Agostini F, Heer M, Guarnieri G, Biolo G. Physical inactivity decreases whole body glutamine turnover independently from changes in proteolysis. J Physiol. (2008) 586:4775–81. doi: 10.1113/jphysiol.2008.153783
57. Rennie MJ, Selby A, Atherton P, Smith K, Kumar V, Glover EL, et al. Facts, noise and wishful thinking: muscle protein turnover in aging and human disuse atrophy. Scand J Med Sci Sports. (2010) 20:5–9. doi: 10.1111/j.1600-0838.2009.00967.x
58. Glover EI, Yasuda N, Tarnopolsky MA, Abadi A, Phillips SM. Little change in markers of protein breakdown and oxidative stress in humans in immobilization-induced skeletal muscle atrophy. Appl Physiol Nutr Metab. (2010) 35:125–33. doi: 10.1139/H09-137
59. Biolo G, Tipton KD, Klein S, Wolfe RR. An abundant supply of amino acids enhances the metabolic effect of exercise on muscle protein. Am J Physiol. (1997) 273(1 Pt 1.):E122–9. doi: 10.1152/ajpendo.1997.273.1.E122
60. Wall BT, Dirks ML, Snijders T, van Dijk JW, Fritsch M, Verdijk LB, et al. Short-term muscle disuse lowers myofibrillar protein synthesis rates and induces anabolic resistance to protein ingestion. Am J Physiol Endocrinol Metab. (2016) 310:E137–47. doi: 10.1152/ajpendo.00227.2015
61. Wall BT, Snijders T, Senden JM, Ottenbros CL, Gijsen AP, Verdijk LB, et al. Disuse impairs the muscle protein synthetic response to protein ingestion in healthy men. J Clin Endocrinol Metab. (2013) 98:4872–81. doi: 10.1210/jc.2013-2098
62. Glover EI, Phillips SM, Oates BR, Tang JE, Tarnopolsky MA, Selby A, et al. Immobilization induces anabolic resistance in human myofibrillar protein synthesis with low and high dose amino acid infusion. J Physiol. (2008) 586:6049–61. doi: 10.1113/jphysiol.2008.160333
63. Dardevet D, Remond D, Peyron MA, Papet I, Savary-Auzeloux I, Mosoni L. Muscle wasting and resistance of muscle anabolism: the “anabolic threshold concept” for adapted nutritional strategies during sarcopenia. ScientificWorldJournal. (2012) 2012:269531. doi: 10.1100/2012/269531
64. Biolo G, Ciocchi B, Lebenstedt M, Heer M, Guarnieri G. Sensitivity of whole body protein synthesis to amino acid administration during short-term bed rest. J Gravit Physiol. (2002) 9:197–8.
65. Magne H, Savary-Auzeloux I, Migne C, Peyron MA, Combaret L, Remond D, et al. Unilateral hindlimb casting induced a delayed generalized muscle atrophy during rehabilitation that is prevented by a whey or a high protein diet but not a free leucine-enriched diet. PLoS One. (2013) 8:e70130. doi: 10.1371/journal.pone.0070130
66. Biolo G, Ciocchi B, Lebenstedt M, Barazzoni R, Zanetti M, Platen P, et al. Short-term bed rest impairs amino acid-induced protein anabolism in humans. J Physiol. (2004) 558(Pt 2.):381–8. doi: 10.1113/jphysiol.2004.066365
67. Ferrando AA, Lane HW, Stuart CA, Davis-Street J, Wolfe RR. Prolonged bed rest decreases skeletal muscle and whole body protein synthesis. Am J Physiol. (1996) 270(4 Pt 1.):E627–33. doi: 10.1152/ajpendo.1996.270.4.E627
68. Kortebein P, Ferrando A, Lombeida J, Wolfe R, Evans WJ. Effect of 10 days of bed rest on skeletal muscle in healthy older adults. JAMA. (2007) 297:1772–4. doi: 10.1001/jama.297.16.1772-b
69. Katsanos CS, Kobayashi H, Sheffield-Moore M, Aarsland A, Wolfe RR. A high proportion of leucine is required for optimal stimulation of the rate of muscle protein synthesis by essential amino acids in the elderly. Am J Physiol Endocrinol Metab. (2006) 291:E381–7. doi: 10.1152/ajpendo.00488.2005
70. Stipanuk MH. Leucine and protein synthesis: mTOR and beyond. Nutr Rev. (2007) 65:122–9. doi: 10.1111/j.1753-4887.2007.tb00289.x
71. Alvestrand A, Hagenfeldt L, Merli M, Oureshi A, Eriksson LS. Influence of leucine infusion on intracellular amino acids in humans. Eur J Clin Invest. (1990) 20:293–8. doi: 10.1111/j.1365-2362.1990.tb01858.x
72. Louard RJ, Barrett EJ, Gelfand RA. Effect of infused branched-chain amino acids on muscle and whole-body amino acid metabolism in man. Clin Sci. (1990) 79:457–66. doi: 10.1042/cs0790457
73. Nair KS, Schwartz RG, Welle S. Leucine as a regulator of whole body and skeletal muscle protein metabolism in humans. Am J Physiol. (1992) 263(5 Pt 1.):E928–34. doi: 10.1152/ajpendo.1992.263.5.E928
74. Drummond MJ, Rasmussen BB. Leucine-enriched nutrients and the regulation of mammalian target of rapamycin signalling and human skeletal muscle protein synthesis. Curr Opin Clin Nutr Metab Care. (2008) 11:222–6. doi: 10.1097/MCO.0b013e3282fa17fb
75. Manifava M, Smith M, Rotondo S, Walker S, Niewczas I, Zoncu R, et al. Dynamics of mTORC1 activation in response to amino acids. Elife. (2016) 5:e19960. doi: 10.7554/eLife.19960
76. Son SM, Park SJ, Stamatakou E, Vicinanza M, Menzies FM, Rubinsztein DC. Leucine regulates autophagy via acetylation of the mTORC1 component raptor. Nat Commun. (2020) 11:3148. doi: 10.1038/s41467-020-16886-2
77. Arentson-Lantz EJ, Mikovic J, Bhattarai N, Fry CS, Lamon S, Porter C, et al. Leucine augments specific skeletal muscle mitochondrial respiratory pathways during recovery following 7 days of physical inactivity in older adults. J Appl Physiol. (2021) 130:1522–33. doi: 10.1152/japplphysiol.00810.2020
78. English KL, Mettler JA, Ellison JB, Mamerow MM, Arentson-Lantz E, Pattarini JM, et al. Leucine partially protects muscle mass and function during bed rest in middle-aged adults. Am J Clin Nutr. (2016) 103:465–73. doi: 10.3945/ajcn.115.112359
79. Harper AE, Miller RH, Block KP. Branched-chain amino acid metabolism. Annu Rev Nutr. (1984) 4:409–54. doi: 10.1146/annurev.nu.04.070184.002205
80. Aftring RP, Block KP, Buse MG. Leucine and isoleucine activate skeletal muscle branched-chain alpha-keto acid dehydrogenase in vivo. Am J Physiol. (1986) 250(5 Pt 1.):E599–604. doi: 10.1152/ajpendo.1986.250.5.E599
81. May RC, Piepenbrock N, Kelly RA, Mitch WE. Leucine-induced amino acid antagonism in rats: muscle valine metabolism and growth impairment. J Nutr. (1991) 121:293–301. doi: 10.1093/jn/121.3.293
82. Cemin HS, Tokach MD, Woodworth JC, Dritz SS, DeRouchey JM, Goodband RD. Branched-chain amino acid interactions in growing pig diets. Transl Anim Sci. (2019) 3:1246–53. doi: 10.1093/tas/txz087
83. Wolfe RR. Branched-chain amino acids and muscle protein synthesis in humans: myth or reality? J Int Soc Sports Nutr. (2017) 14:30. doi: 10.1186/s12970-017-0184-9
84. Pinilla J, Aledo JC, Cwiklinski E, Hyde R, Taylor PM, Hundal HS. SNAT2 transceptor signalling via mTOR: a role in cell growth and proliferation? Front Biosci. (2011) 3:1289–99. doi: 10.2741/e332
85. Nicklin P, Bergman P, Zhang B, Triantafellow E, Wang H, Nyfeler B, et al. Bidirectional transport of amino acids regulates mTOR and autophagy. Cell. (2009) 136:521–34. doi: 10.1016/j.cell.2008.11.044
86. Drummond MJ, Glynn EL, Fry CS, Timmerman KL, Volpi E, Rasmussen BB. An increase in essential amino acid availability upregulates amino acid transporter expression in human skeletal muscle. Am J Physiol Endocrinol Metab. (2010) 298:E1011–8. doi: 10.1152/ajpendo.00690.2009
87. Drummond MJ, Fry CS, Glynn EL, Timmerman KL, Dickinson JM, Walker DK, et al. Skeletal muscle amino acid transporter expression is increased in young and older adults following resistance exercise. J Appl Physiol. (2011) 111:135–42. doi: 10.1152/japplphysiol.01408.2010
88. Drummond MJ, Dickinson JM, Fry CS, Walker DK, Gundermann DM, Reidy PT, et al. Bed rest impairs skeletal muscle amino acid transporter expression, mTORC1 signaling, and protein synthesis in response to essential amino acids in older adults. Am J Physiol Endocrinol Metab. (2012) 302:E1113–22. doi: 10.1152/ajpendo.00603.2011
89. Koyama H, Nishizawa Y, Yamashita N, Furumitsu Y, Hagiwara S, Ochi H, et al. Measurement of composition changes using dual-photon absorptiometry in obese patients undergoing semistarvation. Metabolism. (1990) 39:302–6. doi: 10.1016/0026-0495(90)90051-d
90. Blottner D, Salanova M, Puttmann B, Schiffl G, Felsenberg D, Buehring B, et al. Human skeletal muscle structure and function preserved by vibration muscle exercise following 55 days of bed rest. Eur J Appl Physiol. (2006) 97:261–71. doi: 10.1007/s00421-006-0160-6
91. Belavy DL, Miokovic T, Armbrecht G, Richardson CA, Rittweger J, Felsenberg D. Differential atrophy of the lower-limb musculature during prolonged bed-rest. Eur J Appl Physiol. (2009) 107:489–99. doi: 10.1007/s00421-009-1136-0
92. Armbrecht G, Belavy DL, Gast U, Bongrazio M, Touby F, Beller G, et al. Resistive vibration exercise attenuates bone and muscle atrophy in 56 days of bed rest: biochemical markers of bone metabolism. Osteoporos Int. (2010) 21:597–607. doi: 10.1007/s00198-009-0985-z
93. Moriggi M, Vasso M, Fania C, Capitanio D, Bonifacio G, Salanova M, et al. Long term bed rest with and without vibration exercise countermeasures: effects on human muscle protein dysregulation. Proteomics. (2010) 10:3756–74. doi: 10.1002/pmic.200900817
94. Belavy DL, Armbrecht G, Richardson CA, Felsenberg D, Hides JA. Muscle atrophy and changes in spinal morphology: is the lumbar spine vulnerable after prolonged bed-rest? Spine. (2011) 36:137–45. doi: 10.1097/BRS.0b013e3181cc93e8
95. Miokovic T, Armbrecht G, Gast U, Rawer R, Roth HJ, Runge M, et al. Muscle atrophy, pain, and damage in bed rest reduced by resistive (vibration) exercise. Med Sci Sports Exerc. (2014) 46:1506–16. doi: 10.1249/MSS.0000000000000279
96. Gao R, Chilibeck PD. Nutritional interventions during bed rest and spaceflight: prevention of muscle mass and strength loss, bone resorption, glucose intolerance, and cardiovascular problems. Nutr Res. (2020) 82:11–24. doi: 10.1016/j.nutres.2020.07.001
97. Kilroe SP, Fulford J, Jackman S, Holwerda A, Gijsen A, van Loon L, et al. Dietary protein intake does not modulate daily myofibrillar protein synthesis rates or loss of muscle mass and function during short-term immobilization in young men: a randomized controlled trial. Am J Clin Nutr. (2021) 113:548–61. doi: 10.1093/ajcn/nqaa136
98. Dirks ML, Wall BT, Nilwik R, Weerts DH, Verdijk LB, van Loon LJ. Skeletal muscle disuse atrophy is not attenuated by dietary protein supplementation in healthy older men. J Nutr. (2014) 144:1196–203. doi: 10.3945/jn.114.194217
99. Backx EMP, Horstman AMH, Marzuca-Nassr GN, van Kranenburg J, Smeets JS, Fuchs CJ, et al. Leucine supplementation does not attenuate skeletal muscle loss during leg immobilization in healthy, young men. Nutrients. (2018) 10:635. doi: 10.3390/nu10050635
100. Edwards SJ, Smeuninx B, McKendry J, Nishimura Y, Luo D, Marshall RN, et al. High-dose leucine supplementation does not prevent muscle atrophy or strength loss over 7 days of immobilization in healthy young males. Am J Clin Nutr. (2020) 112:1368–81. doi: 10.1093/ajcn/nqaa229
101. Holloway TM, McGlory C, McKellar S, Morgan A, Hamill M, Afeyan R, et al. A novel amino acid composition ameliorates short-term muscle disuse atrophy in healthy young men. Front Nutr. (2019) 6:105. doi: 10.3389/fnut.2019.00105
102. Reidy PT, McKenzie AI, Brunker P, Nelson DS, Barrows KM, Supiano M, et al. Neuromuscular electrical stimulation combined with protein ingestion preserves thigh muscle mass but not muscle function in healthy older adults during 5 days of bed rest. Rejuvenation Res. (2017) 20:449–61. doi: 10.1089/rej.2017.1942
103. Arentson-Lantz EJ, Fiebig KN, Anderson-Catania KJ, Deer RR, Wacher A, Fry CS, et al. Countering disuse atrophy in older adults with low-volume leucine supplementation. J Appl Physiol. (2020) 128:967–77. doi: 10.1152/japplphysiol.00847.2019
104. Deutz NE, Pereira SL, Hays NP, Oliver JS, Edens NK, Evans CM, et al. Effect of beta-hydroxy-beta-methylbutyrate (HMB) on lean body mass during 10 days of bed rest in older adults. Clin Nutr. (2013) 32:704–12. doi: 10.1016/j.clnu.2013.02.011
105. Rudwill F, O’Gorman D, Lefai E, Chery I, Zahariev A, Normand S, et al. Metabolic inflexibility is an early marker of bed-rest-induced glucose intolerance even when fat mass is stable. J Clin Endocrinol Metab. (2018) 103:1910–20. doi: 10.1210/jc.2017-02267
106. Owen PJ, Armbrecht G, Bansmann M, Zange J, Pohle-Frohlich R, Felsenberg D, et al. Whey protein supplementation with vibration exercise ameliorates lumbar paraspinal muscle atrophy in prolonged bed rest. J Appl Physiol. (2020) 128:1568–78. doi: 10.1152/japplphysiol.00125.2020
Keywords: lean body mass, high-protein diet, branched-chain amino acids, leucine, long-term bed rest
Citation: Vinci P, Di Girolamo FG, Mangogna A, Mearelli F, Nunnari A, Fiotti N, Giordano M, Bareille M-P and Biolo G (2022) Early lean mass sparing effect of high-protein diet with excess leucine during long-term bed rest in women. Front. Nutr. 9:976818. doi: 10.3389/fnut.2022.976818
Received: 23 June 2022; Accepted: 09 November 2022;
Published: 24 November 2022.
Edited by:
Daniel Moore, University of Toronto, CanadaReviewed by:
Maria Montserrat Diaz Pedrosa, State University of Maringá, BrazilChris McGlory, Queen’s University, Canada
Tyler A. Churchward-Venne, McGill University, Canada
Copyright © 2022 Vinci, Di Girolamo, Mangogna, Mearelli, Nunnari, Fiotti, Giordano, Bareille and Biolo. This is an open-access article distributed under the terms of the Creative Commons Attribution License (CC BY). The use, distribution or reproduction in other forums is permitted, provided the original author(s) and the copyright owner(s) are credited and that the original publication in this journal is cited, in accordance with accepted academic practice. No use, distribution or reproduction is permitted which does not comply with these terms.
*Correspondence: Gianni Biolo, YmlvbG9AdW5pdHMuaXQ=
†These authors have contributed equally to this work and share first authorship