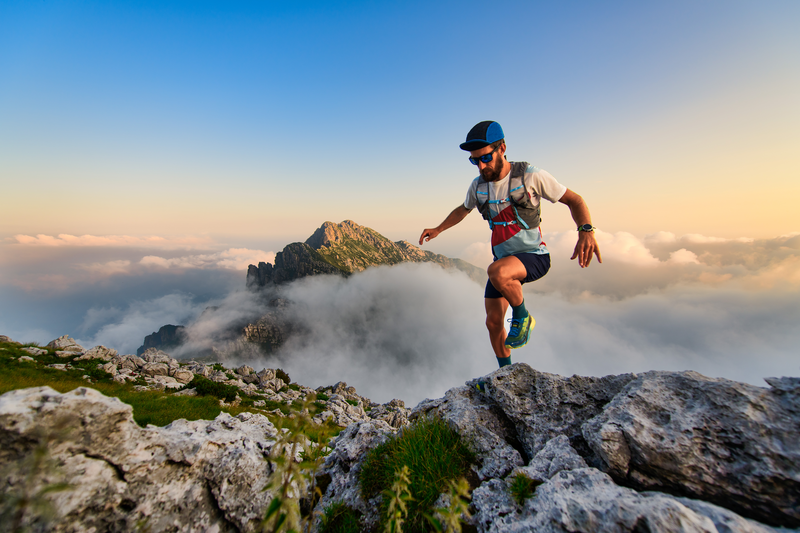
94% of researchers rate our articles as excellent or good
Learn more about the work of our research integrity team to safeguard the quality of each article we publish.
Find out more
REVIEW article
Front. Nutr. , 25 August 2022
Sec. Nutritional Immunology
Volume 9 - 2022 | https://doi.org/10.3389/fnut.2022.974896
This article is part of the Research Topic The Nutritional Immunological Effects and Mechanisms of Chemical Constituents from the Homology of Medicine and Food View all 11 articles
Cancer is a major public health problem that threatens human life worldwide. In recent years, immunotherapy has made great progress in both clinical and laboratory research. But the high heterogeneity and dynamics of tumors makes immunotherapy not suitable for all cancers. Dietary polyphenols have attracted researchers' attention due to their ability to induce cancer cell pyroptosis and to regulate the tumor immune microenvironment (TIME). This review expounds the regulation of dietary polyphenols and their new forms on cancer cell pyroptosis and the TIME. These dietary polyphenols include curcumin (CUR), resveratrol (RES), epigallocatechin gallate (EGCG), apigenin, triptolide (TPL), kaempferol, genistein and moscatilin. New forms of dietary polyphenols refer to their synthetic analogs and nano-delivery, liposomes. Studies in the past decade are included. The result shows that dietary polyphenols induce pyroptosis in breast cancer cells, liver cancer cells, oral squamous cells, carcinoma cells, and other cancer cells through different pathways. Moreover, dietary polyphenols exhibit great potential in the TIME regulation by modulating the programmed cell death protein 1(PD-1)/programmed death-ligand 1 (PD-L1) axis, enhancing antitumor immune cells, weakening the function and activity of immunosuppressive cells, and targeting tumor-associated macrophages (TAMs) to reduce their tumor infiltration and promote their polarization toward the M1 type. Dietary polyphenols are also used with radiotherapy and chemotherapy to improve antitumor immunity and shape a beneficial TIME. In conclusion, dietary polyphenols induce cancer cell pyroptosis and regulate the TIME, providing new ideas for safer cancer cures.
The incidence of cancer has continually risen to 25% since the 20th century. The ever-increasing incidence of cancer is associated with increased production patterns, lifestyles, and life expectancy (1). According to the latest projections from the American Cancer Bureau, 1,918,030 people will be diagnosed with cancer every day in the United States in 2022. In the end, 609,630 people will die of cancer (2). Going back to the nature of cancer itself, cancer can originate in any organ and structure of the body. Unlike other cells, cancer cells can continue to proliferate, replicate indefinitely, and resist death. Even cancer cells can lure immune cells to escape immune evasion (3). Therefore, it is especially difficult to cure cancer. As the three most important treatments, surgery, radiotherapy and chemotherapy have long been and will continue to be the weapons in the fight against cancer. However, all three methods have their own shortcomings and limitations. There is an urgent need to develop more effective anticancer approaches.
Cancer treatment has made landmark achievements in the past decade. In fact, cancer is not a direct cause of death. The weakened immunity and complications of cancer are. Immunotherapy aims to promote the “rebuilding” of the immune system. Some cancer-fighting immune cells are engineered to fight cancer. This involves a new concept of the TIME. In short, the TIME refers to the microenvironment formed by the interaction of tumor cells and immune cells. Some immune checkpoints and cytokines are also included. The TIME is the foundation of immunotherapy. In recent years, research related to immunotherapy has grown exponentially. Clinically, dendritic cells (DC) vaccines, CAR-T cell therapy, adoptive cell transfer, and immune checkpoint inhibitors have achieved surprising results (4).
Immunotherapy is not perfect due to tumor heterogeneity and dynamics. Therefore, it is particularly important to deepen the understanding of the TIME and to find drugs to enhance anti-tumor immunity. In view of this situation, dietary polyphenols have attracted the attention of some researchers due to their superior anti-inflammatory, anticancer, and immunomodulatory functions. Moreover, dietary polyphenols are widely present in everyday foods. They are safe and easily available.
Pyroptosis is a type of programmed cell death. Pro-inflammatory is the most significant feature of pyroptosis that distinguishes it from other programmed cell death such as apoptosis and autophagy (5). Is it possible to artificially induce cancer cell pyroptosis to make cancer regress? This issue is widely discussed. In the study, it is surprisingly found that dietary polyphenols not only induce cancer cell pyroptosis, but also drive a favorable anticancer immunity in the TIME.
Pyroptosis is an inflammatory programmed cell death performed by a gasdermin (GSDM) protein family. When pyroptosis is activated, GSDM is cleaved into an auto-inhibitory C-terminal and an active N-terminal (NT). GSDM-NT then punches holes in the cell membrane, causing the cell to swell until it bursts. And a large amount of cellular contents, such as pro-inflammatory factors and lysosomes, are released, resulting in an inflammatory cascade (see Figure 1).
Figure 1. Regulation of dietary polyphenols on cancer cell pyroptosis and the TIME. Dietary polyphenols are ingested orally and interact with food in the stomach. Subsequently, dietary polyphenols undergo phase I and phase II metabolism in the liver. In the gut, dietary polyphenols are metabolized and absorbed by intestinal epithelial cells and gut microbes. The absorbed and digested dietary polyphenols then travel with the blood to the TIME. Immune cells in the TIME are influenced by dietary polyphenols. At the same time, cancer cell pyroptosis was activated by dietary polyphenols. The blue arrow represents promotion and the red arrow represents inhibition.
GSDM protein family includes GSDMA, GSDMB, GSDMC, GSDMD, and GSDME. In cancer, GSDMD and GSDME are the most studied. GSDMD induces pyroptosis mainly through two pathways (6). In the classical pyroptosis pathway, inflammasomes recognize pathogen-associated molecular patterns (PAMPs) or damage associated molecular pattern molecules (DAMPs). Then caspase-1 is activated and upregulated, and cleaves GSDMD into GSDMD-NT. This is the first pyroptosis pathway to be studied. In the non-canonical pyroptotic pathway, GSDMD cleavage is performed by caspase-4, 5 (human) and caspase-11 (mouse). Like GSDMD, GSDME also has two pathways. Typically, chemotherapeutic drugs induce caspase-3 to cleave GSDME to perform pyroptosis (7). Recently granzyme B (GZMB) was found to cleave GSDME at D270 to induce pyroptosis instead of caspase-3 (8).
Each GSDM protein has a high degree of expression variability and tissue specificity (8). This is rare in mammals. In different cancers, GSDMD and GSDME have different expression levels and functions. In gastric cancer cells, GSDMD is underexpressed and promotes proliferation (9). In non-small cell carcinoma, GSDMD is highly expressed and indicates higher invasiveness (10). In human colorectal cancer, GSDMD is underexpressed, which is detrimental to patient survival (11). In addition, high expression of GSDMD is associated with poor prognosis of lung adenocarcinoma and osteosarcoma (12). It can be seen that the expression and role of GSDMD in cancer are complex and variable. Even the subcellular localization of GSDMD affects cancer progression and immune response (13). Therefore, the idea of targeting GSDMD to induce cancer cell pyroptosis requires more careful selection and more in-depth exploration.
Different from GSDMD, GSDME acts more as a tumor suppressor. GSDME is normally expressed in the heart, kidney and brain. In most cancers, epigenetics and mutations lead to silencing of the GSDME gene (14). Different expression levels of GSDME determine whether cancer cells tend to apoptosis or pyroptosis during chemotherapy. In cancer cells with high expression of GSDME, caspase-3 specifically cleaves GSDME to convert apoptosis into pyroptosis (7, 15). However, caspase-3 tends to induce apoptosis in cancer cells with low GSDME expression. This phenomenon provides a new idea for anti-apoptotic cancer therapy. In addition to inhibiting cancer cell proliferation, GSDME promotes immune cell infiltration (16, 17). Its expression in tumors converts immunologically “cold” tumors into “hot” tumors, thereby activating antitumor immunity (18).
GSDMA, GSDMB, GSDMC are less studied in cancer. But that doesn't mean they are not important (see Table 1). Using drugs to induce pyroptosis of cancer cells is an important research direction at present. Various studies have shown that pyroptosis has broad prospects in cancer therapy.
The TIME is a complex ecosystem that acts as a double-edged sword in the progression of cancer. On the one hand, antitumor cells such as NK cells and cytotoxic T lymphocytes (CTLs, mainly CD8+ T cells) can identify and eliminate cancer cells. They play a role in cancer immune monitoring (21–23). On the other hand, immunosuppressive cells such as regulatory T cells (Tregs), TAMs, and myeloid-derived suppressor cells (MDSCs) protect cancer cells by evading immune surveillance. Subsequently, cancer cells can continue to invade, metastasize and induce angiogenesis (21–23). At the same time, cancer cells can induce, expand and recruit a large number of tumor-promoting myeloid cells to establish a tumor immunosuppressive microenvironment by driving immunosuppression, regulating the generation of immune cell subtypes (21, 22). With the joint efforts of anti-tumor immune cells and cancer cells, cancer develops to malignant. However, the role of immune cells in the TIME is not immutable. For example, TAMs, signals in the TIME can directly affect the differentiation of TAMs and polarization between M1 and M2. The interaction between antitumor cells, immunosuppressive cells, and tumor cells is mainly achieved through exosomes, chemokines, and cytokines (see Figure 2) (24–31). This interaction plays a key role in the development of cancer.
Figure 2. The interaction between antitumor cells, immunosuppressive cells and tumor cells. a: ADCC/apoptosis/pyroptosis; b: IFN-γ, TNF-α: anti-proliferative, anti-angiogenic, pro-apoptosis; c: TGF-β, IL-10, IL-35, GZMB, perforin; d: TGF-β, IL-10, IL-35, GZMB, perforin, CTLA-4, PD-1; e: PD-L1↑, HIF-1α↑, TGF-β, IL-10, CCL4, CCL5; f: IFN-γ: induce activation; g: IFN-γ, LTα, IL-2; h: M2a: IL-4, IL-13; M2b: immune complex with IL-1β/LPS; M2c: IL-10, TGF-β; IL-6, LIF; i: dual blockade of PI3K-γ pathway and CSF-1/CSF-1R; CD40 agonist; j: pro-proliferative, pro-invasion, pro-metastasis, angiogenesis; k: IL-4 → IFN-γ↓; l: MHCI↓ → escape; m: PD-1/PD-L1 → hijack; miR-214 → proliferation; n: cytokine↓; degranulation↓; metabolism↓; mTOR signal↓. ADCC, antibody-dependent cell-mediated cytotoxicity; TGF-β, transforming growth factor-β; CTLA-4, cytotoxic T lymphocyte-associated antigen-4; LIF, leukemia inhibitory; CSF-1, colony-stimulating factor 1 factor; mTOR, mammalian target of rapamycin.
All kinds of evidence show that pyroptosis is closely related to the TIME. For example, GSDMB/E can induce pyroptosis after specific cleavage by GZM secreted by CTLs. It was also recently found that macrophage-derived TNF-α activates caspase-8 to cleave GSDMC, resulting in cancer cell pyroptosis (20). However, the role of pyroptosis in the TIME is ambiguous. On the one hand, inflammatory factors released accompanying cancer cell pyroptosis form a chronic inflammatory microenvironment, including NLRP3, IL-18 and IL-1β. This chronic inflammatory microenvironment has been shown to help cancer cells evade innate immune responses and promote cancer progression (10, 32–36). On the other hand, pyroptosis triggers strong antitumor immunity. Pyroptosis significantly increases the accumulation of immune cells and immune factors in solid tumors (37–39). Targeting pyroptosis and stimulating the TIME is a new idea for cancer treatment (40, 41).
The PD-1/PD-L1 axis is a core immunosuppression pathway in the TIME. For a long time, the PD-1/PD-L1 axis has been widely studied due to its immune checkpoint function. However, non-immune checkpoint functions of the PD-1/PD-L1 axis have been identified in studies of pyroptosis. Antibiotic chemotherapeutics induce GSDMC-mediated pyroptosis in hypoxic tumor environments by upregulating the nPD-L1/pro-signal transducer and activator of transcription 3 (p-STAT3) complex (20). In addition, the inflammatory environment created by pyroptosis may enhance the efficacy of anti-PD-L1 therapy. Whether pyroptosis have adverse effects on the immunity of cancer patients? No clear conclusions have been drawn. Is there a substance that can induce cancer cell pyroptosis, and promote anti-tumor immunity at the same time? Dietary polyphenols caught our eye.
Polyphenols, which literally means “having multiple phenolic groups,” are mostly found in plant foods. They are widely found in the daily human diet, including nuts, vegetables, fruits, dark chocolate, tea, red wine, and some natural Chinese herbal medicines. They are called “the seventh type of nutrient.” Most natural dietary polyphenols exist in the form of glycoside esters or free aglycones and are biotransformed mainly in the gastrointestinal tract, liver, and gut microbiota (see Table 2) (42–54). Due to factors such as chemical structure and biometabolic properties of dietary polyphenols, their low bioavailability has become a major factor that limits their efficacy in vivo and in clinical trials. Chinese herbal medicines rich in polyphenols have been widely used clinically. For example, turmeric, Polygonum cuspidatum, and Tripterygium wilfordii are used to treat cardiovascular disease, rheumatoid arthritis, and systemic lupus erythematosus (55, 56). In recent years, dietary polyphenols have been proven to have a wide range of biological activities. And their effective anti-inflammatory, antioxidant, anticancer, immunomodulatory, and cardiovascular protective properties have made them widely studied and used in food additives, skin care, medicine, health care (57). The anticancer properties of dietary polyphenols are mainly manifested in inhibiting tumor development (proliferation, growth, invasion, metastasis, and angiogenesis), regulating programmed cell death (apoptosis and pyroptosis), inhibiting chemoresistance, enhancing anticancer immune response, and regulating the TIME. In this review, we focus on the properties of dietary polyphenols that induce pyroptosis and regulate the TIME in cancer.
Table 2. Dietary polyphenols involved in this review and their names, chemical formulas, structural formulas and metabolic absorption.
Clinically, insufficient intake of dietary polyphenols does not cause a certain disease. However, dietary polyphenol intake is positively correlated with body health (58). As the study of pyroptosis becomes more and more extensive, the inner link between pyroptosis and disease is being revealed. It was found that dietary polyphenols have different effects on pyroptosis in different diseases. Microglia are phagocytic cells that reside in the brain. They play an important role in the immune response after central nervous system injury. Studies have shown that dietary polyphenols protect microglia by inhibiting pyroptosis. This is beneficial for Parkinson's patients and those with spinal cord injuries (59–61). In addition, dietary polyphenols can reduce cardiomyocyte pyroptosis caused by chemotherapeutic drugs (doxorubicin) and adverse environment (ischemia/hypoxia) (62, 63). Studies have also reported that dietary polyphenols inhibit the pathological pyroptosis of liver and kidney cell (64, 65). Dietary polyphenols reduce cell pyroptosis induced by toxic heavy metals. It is of great significance for heavy metal exposure-related diseases. Therefore, we can conclude that dietary polyphenols protect the body by resisting pyroptosis. However, in cancer, dietary polyphenols tend to induce cancer cell pyroptosis to promote the body's victory over cancer. In this section, we discuss how dietary polyphenols induce cancer cell pyroptosis (see Figure 1).
CUR induces pyroptosis in MCF-7 breast cancer cells through the autophagy/cathepsin B (CTSB)/NLRP3/caspase-1/GSDMD signaling pathway (66). Anthocyanin activates pyroptosis of Tca8113 and SCC15 oral squamous cell carcinoma cells through the NLRP3/caspase-1/GSDMD pathway. Besides, anthocyanin inhibits cancer cell viability, invasion, and metastasis (67). Similar to anthocyanin, chrysophanol up-regulated the expression of NLRP3 in gastric cancer cells. Subsequently, the caspase-1/GSDMD pathway is activated to induce cancer cell pyroptosis (68).
By treating different head and neck cancer cells with TPL, it has been found that TPL selectively induced pyroptosis in an HK1 squamous cell carcinoma cell line and a FaDu hypopharyngeal carcinoma cell line. This pyroptosis was achieved by inhibiting the expression of c-myc and mitochondrial hexokinase II (HK-II) in cancer cells, leading to the activation of the BAD/BAX-caspase-3 cascade, and then cleaves GSDME (69). CUR up-regulates ROS levels, down-regulates pro-caspase-3 expression, and up-regulates GSDME-NT expression in a time- and dose-dependent manner to promote pyroptosis in HepG2 hepatoma cells (70). Kaempferol has been shown to trigger GSDME-mediated U87 MG and U251 glioblastoma cell line pyroptosis by inducing high levels of ROS autophagy and activating inflammasome/caspase-1/IL-1β signaling in vitro and in vivo experiments (71). In another study on glioblastoma, researchers used genomic data to find that human gliomas express higher levels of GSDME than normal brains. Subsequently, in vivo and in vitro tests were carried out. They found that galangin induces GSDME-mediated pyroptosis in glioblastoma cells (72). Neobractatin is a kind of dietary polyphenol extracted from Garcinia bracteata. In esophageal cancer cells with high GSDME expression, neobractatin induces pyroptosis through the caspase-3/GSDME pathway. After GSDME was knocked out, pyroptosis transformed into apoptosis. Neobractatin treatment of esophageal cancer showed significant tumor regression (73). Spatholobus suberectus Dunn is called “Ji Xue Teng” in Chinese. Its percolation extract contains various dietary polyphenols such as catechin, procyanidin B2, epicatechin, genistein, and formononetin. Spatholobus suberectus Dunn percolation extract induced pyroptosis of triple-negative breast cancer cells is also mediated by GSDME. However, GSDME is activated by caspase-4 rather than caspase-1 (74).
In some studies, dietary polyphenols have been shown to induce cancer cell pyroptosis. But the pyroptosis pathway was not revealed. These findings are also included in this review.
CUR delivers a double whammy against malignant mesothelioma cells. It induces pyroptosis through activation of caspase-1 by ROS. Moreover, CUR significantly down-regulated the expression levels of inflammasome-related genes such as NF-κB, toll-like receptor (TLR), and IL-1β (75). Researchers linked CUR to sound photodynamic therapy (SPDT). In vitro experiments showed that HepG2 cells underwent pyroptosis and apoptosis with CUR-PLGA-MB-SPDT (CUR-loaded poly (L-lactide-co-glycolide)-microbubble (MB)-mediated SPDT) treatment. The underlying mechanism is related to the loss of mitochondrial membrane potential (MMP) and the increase of ROS (76).
Moscatilin and RES can act as radiosensitizers in combination with 1 Gy X-ray or 200 J/m2 UV-C radiation. Overlapping cell death pathways were activated by this combination in HepG2, SH-SY5Y and HaCaT cell lines, including necroptosis and pyroptosis (77). Moscatiline induces immunogenic death of cancer cells. The combination of Moscatiline and radiation induces pyroptosis of cancer cells, eventually leading to necroptosis (77). These findings validate the fact that dietary polyphenols induce cancer cell pyroptosis (see Table 3).
In fact, dietary polyphenols induce not only cancer cell pyroptosis but also various regulated cell deaths such as apoptosis, ferroptosis and autophagy (78–80). While one of cell death is inhibited, dietary polyphenols enhance other cell death (72). This is due to the crosstalk between different cell deaths. Therefore, targeting pyroptosis may be a potential therapy for some apoptosis-resistant cancer. And as mentioned in the section 3, pyroptosis may have a positive effect on the TIME. Taken together, targeting cancer cell pyroptosis is undoubtedly important and meaningful. So, we focused on the role of dietary polyphenols on cancer cell pyroptosis in this review. However, it is extremely important that dietary polyphenol-induced cancer cell death is not single.
Among all immune checkpoint inhibition points, the PD-L1/PD-1 axis was the most well-studied. Because of its value as a therapeutic target for patients with multiple malignancies and advanced cancers, the PD-L1/PD-1 axis has received extensive attention. PD-L1 binds to PD-1 on the surface of antigen-specific T cells, and suppress antitumor immunity and maintain self-tolerance by regulating the number and activity of antigen-specific T cells in the TIME (81). Some studies have shown that dietary polyphenols can regulate the PD-1/PD-L1 axis between tumor cells and immune cells, driving the TIME to develop in an antitumor direction.
Studies have found that RES directly destroys the N-linked glycan modification and dimerization of PD-L1 in JIMT-1 breast cancer cells, inhibits the correct localization of glycosylated PD-L1 to the cell membrane, blocks the PD-1/PD-L1 axis between CTLs and cancer cells. The destruction of PD-1/PD-L1 axis by RES reduces the immune escape of cancer cells and enhances the immune activity of CTLs (82). RES upregulated PD-L1 expression in lung cancer cells in vitro and significantly induced apoptosis in Jurkat T cells with high PD-1 expression. The mechanism is related to the activation of the canonical Wnt signaling pathway and the reduction of IFN-γ in Jurkat T cells (83). Both luteolin and apigenin can inhibit STAT3 phosphorylation in non-small cell lung cancer (NSCLC) cells, down-regulate IFN-γ-induced PD-L1 expression, increase the activity and function of CD8+ T cells, and enhance the infiltration of CD8+ T cells in tumors (84). Like luteolin and apigenin, CUR can reduce STAT1 phosphorylation and inhibit IFN-γ-induced PD-L1 up-regulation in A375 human melanoma cells in vitro (85). For tongue squamous cell carcinoma, CUR down-regulates PD-L1 expression in vitro and in vivo and inhibits immunosuppressive signaling. CUR also increases CD8 + T cells in immune infiltration, promotes anti-tumor immunity (86).
It is not difficult to see the synergistic effect of dietary polyphenols and anti-PD-1. And this inference has been confirmed in multiple studies. For luteolin and apigenin, anti-PD-1 can amplify the TIME-regulating effect of luteolin and apigenin and significantly enhance the anticancer effect, providing a prospective treatment strategy for KRAS-mutated NSCLC (84). CUR can reduce the expression of TGF-β1 and PD-L1 on the surface of hepatoma cells and inhibit the PD-1/PD-L1 axis both in vitro and in vivo. At the same time, the proportion of CD8+ T cells increased and the expression of Foxp3+ Tregs decreased, which promoted anti-tumor immunity. Besides, the combination of anti-PD-1 and CUR significantly enhanced the TIME regulation function of CUR (87).
As mentioned in section 2, antitumor cell immunity and immunosuppression in the TIME are in a state of mutual confrontation that is like two sides of a scale. Regardless of the form of action, dietary polyphenols always tip the balance in favor of the antitumor side (see Figure 1). CUR inhibits the maturation and immunosuppressive function of MDSCs by inhibiting the levels of arginase-1 (Arg-1), ROS, and IL-6 in the Lewis lung cancer model, reducing the inhibitory effect of MDSCs on T cell proliferation (88). The proportion of IFN-γ and CD8+ T cells increased and the proportion of CD4+ T cells decreased when huh-7 human hepatoma cells were treated with CUR. The addition of taurine significantly enhanced the effect of CUR (89, 90). In another study, it was found that curcuminoids promoted pancreatic cancer cell apoptosis by enhancing the cytotoxicity of NK cells (90, 91). Subsequent experiments showed that the combination of CUR, omega-3 fatty acids and Smartfish (antioxidant-rich lipid emulsion) further enhanced the toxic effects of NK cells on pancreatic cancer cells. And CUR prevents NK cells from producing IFN-γ (90, 91). The researchers attempted to compare and combine CUR with Poly I: C (Toll-like receptor 3 agonist, PIC). It was demonstrated that CUR effectively inhibited PIC-dependent NF-κB activation and Tregs recruitment in head and neck squamous cell carcinoma, demonstrating a beneficial TIME regulation (92). In the co-culture system of MDA-MB-231 and NK-92, CUR bidirectionally enhanced the anticancer activity of NK-9 cells. CUR increases the expression and proportion of Stat4 and STAT5 in CD16+ and CD56dim NK-9 cells. In addition, the expression of pErk and PI3K in MDA-MB-231 was significantly downregulated by CUR (93). In the A375 mouse xenograft model, apigenin was observed to inhibit melanoma growth by significantly enhancing immune cell infiltration and T-cell-mediated tumor-cell killing (85). By comparison, CUR also has the same TIME-regulating effect as apigenin but is not as significant as that of apigenin (85).
CUR is the most dietary polyphenol in regulating the TIME, but other dietary polyphenols such as RES, EGCG, anthocyanins, and genistein are not inferior. RES enhances the overall cytotoxicity of NK cells, resulting in strong activation of NK cells in the TIME. The addition of IL-2 enhanced the activation of NK cells in tumors (94). When non-cytotoxic concentrations of RES were used to treat hepatocellular carcinoma mice, RES was found to enhance anti-tumor immunity by reducing levels of Tregs and M2 macrophages and up-regulating levels of CD8+ T cells (95). Meanwhile, RES also modulated the TIME by regulating related cytokines, for example, up-regulating the anti-tumor cytokines TNF-α and IFN-γ, and down-regulating the immunosuppressive cytokines TGF-β1 and IL-10 (95). Anthocyanin treatment of N-nitrosomethylbenzylamine-induced esophageal papilloma in rats showed good antitumor properties (96). Anthocyanins significantly reduced the recruitment and infiltration of CD68+/CD163- macrophages and CD163+ macrophages in tumors and reduced the accumulation of neutrophils (96). EGCG increased the proportion of CD4+ Tregs and CD8+ T cells in the breast cancer TIME and enhanced the antitumor immune response (83). In addition, EGCG targeted MDSCs in tumors through the Arg-1/iNOS/Nox2/NF-κB/STAT3 classical pathway and non-classical pathways, such as the PI3K-Akt signaling pathway, focal adhesions, and ECM-receptor interactions, reducing their proportion (97). Genistein significantly reduced immune avoidance marker Foxp3 in tumors and upregulated cytotoxic T cell marker Cd8a at mRNA level. And lifetime intake of genistein improves anti-tumor immune response (98).
TAMs affect tumor progression in different ways. They can be roughly divided into two distinct polarization states: antitumor M1 type and pro-tumor immunosuppressive M2 type. In recent years, TAMs targeting strategies have become a hot spot in antitumor therapy. Some dietary polyphenols inhibit the infiltration and polarization of TAMs to M2 type or promote TAMs polarization from M2 type to M1 type in the TIME. Studies found that apigenin restored SHIP-1 expression in pancreatic cancer mice, promoted MDSCs homeostasis, increased M1 expression and M2 polarized to M1, resulting in tumor regression (99). EGCG up-regulates Mir-16 in breast cancer cell exosomes both in vitro and in vivo. Subsequently, TAMs received mir-16 transferred from exosomes. Finally, TAMs infiltration and M2-type polarization were inhibited to inhibit NF-κB activity through the IKKα/Iκ B/NF-κB pathway (100). Hs-1793, a synthetic RES analog, induces upregulation of IFN-γ in tumor tissue in breast cancer mice, leading to a significant reduction in M2 invasion and reprogramming. Favorable TIME changes were associated, such as decreased infiltration of Tregs and decreased immunosuppressive mediators (101).
Due to low solubility and bioavailability of dietary polyphenols, it is difficult to reach serum concentrations in clinical experimental models. Therefore, dietary polyphenols have certain shortcomings in clinical applications. In recent years, researchers have used liposome coating, nano-delivery systems, and synthetic analogs to improve this problem. The anticancer and the TIME-regulating effects of dietary polyphenols were significantly enhanced.
Cur-loaded nanomicelles (CUR@PPC) showed good TIME-regulating activity against melanoma in vitro in two aspects. First, it affects the expression of cytokines, including down-regulation of CCL-22, PD-L1, TGF-β and IL-10, and up-regulation of IFN-γ and TNF-α. Second, it affects immune cell infiltration, including reducing Tregs and enhancing CD8+ T cell immune infiltration (102). This study also found that anti-PD-1 and T cell-delivered NF-κB inhibitors synergized and promoted each other with CUR@PPC, showing good TIME regulation and antitumor effects. This founding provides a new idea for the combined treatment of cancer with dietary polyphenols and immunotherapy (102). In a study of glioblastoma (GBM), researchers found that both liposomal TriCurin (CUR: EGCG: RES = 4:1:12.5) and phytosomal CUR (CCP) therapy induced GBM cells, GBM stem cells apoptosis, and M2-to-M1 polarization (103, 104). In studies using CCP, a large number of activated NK cells were found in the TIME, accompanied by M2-to-M1 polarization. It is speculated that monocyte chemoattractant protein-1 (MCP-1/CCL2) produced by M1 microglia in CCP-induced GBM first acts to induce M1 activation and release IL-12. Subsequently, IL-12 stimulates NK cells to express Cc chemokine receptor 2 and recruit NK cells to the TIME (104). In another experiment using tricurin, the researchers found similar results. Tricurin induces M2 to M1 polarization in human papillomavirus tumors, and IL-12-dependent NK cells and CTLs were recruited to the TIME (105), Nano-curcumin and RES work together to arrest the cell cycle reduce cell viability in rectal cancer cells. In vivo experiments, nanocurcumin and RES promoted macrophage recruitment and enhanced T lymphocyte infiltration (106). In vitro experiments, nano-curcumin was reported to support antitumor cells against pancreatic cancer through various pathways, including enhancing the expression of CD86 and driving DC maturation; significantly reducing the levels of various pro-inflammatory cytokines, such as TNF-α, IL-8, IL-6, IL-10, and IL-1 in activated T cells; and down-regulating IL-8 and up-regulating IFN-γ expression in CTLs (107, 108). CUR analog GO-Y030 exerts potent anticancer effects by reducing the generation, stability, and secretion (TGF-β, IL-10) of Tregs in the melanoma TIME (109). Trans-Scirpusin A (TSA), a natural oligomer of RES. It reduces the number and ratio of Tregs and MDSCs in mouse colorectal cancer tumor tissue and induces antitumor immunity (108).
Although immunotherapy and precision medicine have risen rapidly with the deepening of research in recent years. Surgery, chemotherapy, and radiotherapy are still the most utilized cancer treatments in clinical practice. Some scholars have tried to use dietary polyphenols to alleviate the interference of chemotherapy resistance and radiotherapy on the TIME, and have made some progress.
TPL reduces cisplatin (DDP) resistance in epithelial ovarian cancer mice and synergizes with DDP (110). Both TPL and TPL combined with DDP significantly increased the levels of NK cell-related proteins CD16 and CD56 in the TIME, and promoted cancer cell apoptosis. It provides a new possibility for improving the survival rate of patients with chemotherapy-resistant advanced ovarian cancer (110). Mammary chimeric mice that received sparse ionizing radiation (SIR) and dense ionizing radiation (DIR) had higher tumor incidence and tumor growth rates, accompanied by a distinct tumor immunosuppressive microenvironment (111). It manifested as a lack of lymphocyte infiltration, increased immunosuppressive myeloid cells, a lack of CD8+ T cells in some aged and fast-growing tumor mice, and a high expression of COX-2, PD-L1, and TGF-β (111). However, Phenyl caffeate was reported to effectively reverse this adverse effect of irradiation on the TIME (111). Joong Sun Kim et al. combined HS-1793 with radiotherapy. They found that HS-1793 could effectively alleviate the adverse effects of radiotherapy on the TIME in FM3A tumor-bearing mice by reducing the number and the infiltration of Tregs in tumor tissue. Meanwhile, HS-1793 upregulated the number of CD8+ T cells and upregulated IFN-γ secretion to attenuate TAM-induced immunosuppression (112). In another study, it was also found that modulated electrothermal therapy could create a more favorable TIME and significantly enhance the immunomodulatory and antitumor effects of nanocurcumin and RES (106) (see Supplementary Table 1).
In this review, we systematically reviewed the effects of dietary polyphenols on cancer cell pyroptosis and the TIME. The results showed that dietary polyphenols induced pyroptosis in cancer cells mainly through the GSDMD and GSDME pathways. Furthermore, dietary polyphenols regulate the TIME by enhancing antitumor immune cells and weakening immunosuppressive cells. At the same time, TAMs are targeted by dietary polyphenols and reduce their tumor infiltration and promote their polarization from M2 to M1 type. Of course, there are also some issues that deserve to be considered.
Elevated IL-18, IL-1β, NLRP3 are one of the main features of pyroptosis. These three inflammatory factors are often used as indicators of pyroptosis. However, overproduction of IL-18/1β and NLRP3 leads to neonatal-onset multisystem inflammatory disease (NOMID) which damages the spleen, skin, liver, and bone a lot. Studies have shown that the pathogenesis of NOMID is GSDMD-dependent (113). The concomitant effects of IL-18, IL-1β and NLRP3 elevation were ignored in studies claiming that GSDMD mediates pyroptosis in cancer cells. The same problem exists in all studies targeting pyroptosis to treat cancer. Some studies of dietary polyphenols inducing pyroptosis in cancer cells were performed only in cells. Unfortunately, in the in vitro studies, the effects of pyroptosis on the TIME and inflammation were ignored too. This may affect the prognosis and complications of cancer patients. There is no doubt that dietary polyphenols are beneficial for anti-tumor immunity in patients. But does this conclusion hold when using drugs to induce pyroptosis in cancer? It is a pity that this question has not been explored in depth.
Dietary polyphenols can reduce levels of pro-inflammatory mediators. This conclusion has been verified in many experiments. Taking CUR as an example, CUR alleviates inflammatory diseases by reducing major markers of inflammation, such as IL-6 and TNF-α, malondialdehyde, sensitive C-reactive protein, MCP-1 (114–116). The anti-inflammatory effect of dietary polyphenols is the basis of their biological activity. Are dietary polyphenols beneficial to the release of inflammatory factors induced by pyroptosis in cancer cells? This requires further research and discussion. Perhaps, we should consider the inflammatory nature of pyroptosis, the TIME, and pyroptosis as a whole. The global effects of dietary polyphenols targeting pyroptosis need to be discussed systematically. Accelerate the pace of dietary polyphenols from laboratory to clinical.
There is no doubt that the TIME is a huge, complex system. How to adjust the TIME to make it a tool to fight cancer will be a research hotspot into the future. Deeper research and exploration are required on other pathways and mechanisms of pyroptosis incancer, the role of pyroptosis in tumor development, the impact of cancer cell pyroptosis on the TIME, and the appropriate degree of cancer cell pyroptosis. Among the above issues, dietary polyphenols deserve further attention. Of course, when it comes to dietary polyphenols, their limited bioavailability cannot be ignored. Related researchers have greatly improved the bioavailability of dietary polyphenols by using nano-delivery systems, liposome coating. Dietary polyphenols are one step closer to clinic.
Pyroptosis is a new field. Targeting pyroptosis to treat cancer holds a aboard future. Dietary polyphenols offer a safer option. Based on the current research, it is also a good idea to combine dietary polyphenols with other drugs. Dietary polyphenols are also excellent as adjuvants for chemotherapy and radiotherapy.
JD and LL conceived and designed the study. XH and YW collected the literature and drafted the manuscript. JD, LL, and WY helped to review and revise the manuscript. All authors read and approved the final paper.
This work was supported by the National Natural Science Foundation of China (No. 32102736), Ruipeng Foundation and New Ruipeng Pet Medical Group Co., LTD (No. RPJJ2020033), Liaoning Province Xing Liao Talents Program Project (No. XLYC1807120), Shenyang Young and Middle-aged Scientific and Technological Innovation Talent Support Program (No. RC200431), Liaoning Province High-level Innovation Team Overseas Training Project (No. 2018LNGXJWPY-YB017), and Liaoning Province General Undergraduate Intercollegiate Joint Training Project (No. 2021-24).
The authors declare that the research was conducted in the absence of any commercial or financial relationships that could be construed as a potential conflict of interest.
All claims expressed in this article are solely those of the authors and do not necessarily represent those of their affiliated organizations, or those of the publisher, the editors and the reviewers. Any product that may be evaluated in this article, or claim that may be made by its manufacturer, is not guaranteed or endorsed by the publisher.
The Supplementary Material for this article can be found online at: https://www.frontiersin.org/articles/10.3389/fnut.2022.974896/full#supplementary-material
1. Roy PS, Saikia BJ. Cancer and cure: a critical analysis. Indian J Cancer. (2016) 53:441–2. doi: 10.4103/0019-509X.200658
2. Siegel RL, Miller KD, Fuchs HE, Jemal A. Cancer statistics, 2022. CA Cancer J Clin. (2022) 72:7–33. doi: 10.3322/caac.21708
3. Hanahan D, Weinberg RA. Hallmarks of cancer: the next generation. Cell. (2011) 144:646–74. doi: 10.1016/j.cell.2011.02.013
4. Zhang Y, Zhang Z. The history and advances in cancer immunotherapy: understanding the characteristics of tumor-infiltrating immune cells and their therapeutic implications. Cell Mol Immunol. (2020) 17:807–21. doi: 10.1038/s41423-020-0488-6
5. Green DR. The coming decade of cell death research: five Riddles. Cell. (2019) 177:1094–107. doi: 10.1016/j.cell.2019.04.024
6. Shi J, Zhao Y, Wang K, Shi X, Wang Y, Huang H, et al. Cleavage of GSDMD by inflammatory caspases determines pyroptotic cell death. Nature. (2015) 526:660–5. doi: 10.1038/nature15514
7. Wang Y, Gao W, Shi X, Ding J, Liu W, He H, et al. Chemotherapy drugs induce pyroptosis through caspase-3 cleavage of a gasdermin. Nature. (2017) 547:99–103. doi: 10.1038/nature22393
8. Zhang Z, Zhang Y, Xia S, Kong Q, Li S, Liu X, et al. Gasdermin E suppresses tumour growth by activating anti-tumour immunity. Nature. (2020) 579:415–20. doi: 10.1038/s41586-020-2071-9
9. Wang WJ, Chen D, Jiang MZ, Xu B, Li XW, Chu Y, et al. Downregulation of gasdermin D promotes gastric cancer proliferation by regulating cell cycle-related proteins. J Dig Dis. (2018) 19:74–83. doi: 10.1111/1751-2980.12576
10. Gao J, Qiu X, Xi G, Liu H, Zhang F, Lv T, et al. Downregulation of GSDMD attenuates tumor proliferation via the intrinsic mitochondrial apoptotic pathway and inhibition of EGFR/Akt signaling and predicts a good prognosis in nonsmall cell lung cancer. Oncol Rep. (2018) 40:1971–84. doi: 10.3892/or.2018.6634
11. Wu LS, Liu Y, Wang XW, Xu B, Lin YL, Song Y, et al. LPS enhances the chemosensitivity of oxaliplatin in HT29 cells via GSDMD-mediated pyroptosis. Cancer Manag Res. (2020) 12:10397–409. doi: 10.2147/CMAR.S244374
12. Lin R, Wei H, Wang S, Huang Z, Chen H, Zhang S, et al. Gasdermin D expression and clinicopathologic outcome in primary osteosarcoma patients. Int J Clin Exp Pathol. (2020) 13:3149–57.
13. Wang J, Kang Y, Li Y, Sun L, Zhang J, Qian S, et al. Gasdermin D in different subcellular locations predicts diverse progression, immune microenvironment and prognosis in colorectal cancer. J Inflamm Res. (2021) 14:6223–35. doi: 10.2147/JIR.S338584
14. Wang Y, Peng J, Xie X, Zhang Z, Li M, Yang M. Gasdermin E-mediated programmed cell death: an unpaved path to tumor suppression. J Cancer. (2021) 12:5241–8. doi: 10.7150/jca.48989
15. Rogers C, Fernandes-Alnemri T, Mayes L, Alnemri D, Cingolani G, Alnemri ES. Cleavage of DFNA5 by caspase-3 during apoptosis mediates progression to secondary necrotic/pyroptotic cell death. Nat Commun. (2017) 8:14128. doi: 10.1038/ncomms14128
16. Hu K, Xu Z, Yao L, Yan Y, Zhou L, Li J. Integrated analysis of expression, prognostic value and immune infiltration of GSDMs in hepatocellular carcinoma. Aging. (2021) 13:24117–35. doi: 10.18632/aging.203669
17. Zhang Z, Zhao S, Yang H, Chen Y, Feng H, An M, et al. Prognostic and immunological role of gasdermin E in pan-cancer analysis. Front Oncol. (2021) 11:706266. doi: 10.3389/fonc.2021.706266
18. Zhang Z, Zhang Y, Lieberman J. Lighting a fire: can we harness pyroptosis to ignite antitumor immunity? Cancer Immunol Res. (2021) 9:2–7. doi: 10.1158/2326-6066.CIR-20-0525
19. Zhou Z, He H, Wang K, Shi X, Wang Y, Su Y, et al. Granzyme A from cytotoxic lymphocytes cleaves GSDMB to trigger pyroptosis in target cells. Science. (2020) 368:eaaz7548. doi: 10.1126/science.aaz7548
20. Hou J, Zhao R, Xia W, Chang CW, You Y, Hsu JM, et al. PD-L1-mediated gasdermin C expression switches apoptosis to pyroptosis in cancer cells and facilitates tumour necrosis. Nat Cell Biol. (2020) 22:1264–75. doi: 10.1038/s41556-020-0575-z
21. Li MO, Wolf N, Raulet DH, Akkari L, Pittet MJ, Rodriguez PC, et al. Innate immune cells in the tumor microenvironment. Cancer Cell. (2021) 39:725–9. doi: 10.1016/j.ccell.2021.05.016
22. Liu Y, Cao X. Immunosuppressive cells in tumor immune escape and metastasis. J Mol Med. (2016) 94:509–22. doi: 10.1007/s00109-015-1376-x
23. Fu X, He Y, Li M, Huang Z, Najafi M. Targeting of the tumor microenvironment by curcumin. Biofactors. (2021) 47:914–32. doi: 10.1002/biof.1776
24. Ohue Y, Nishikawa H. Regulatory T (Treg) cells in cancer: can treg cells be a new therapeutic target? Cancer Sci. (2019) 110:2080–9. doi: 10.1111/cas.14069
25. Melaiu O, Lucarini V, Cifaldi L, Fruci D. Influence of the tumor microenvironment on NK cell function in solid tumors. Front Immunol. (2019) 10:3038. doi: 10.3389/fimmu.2019.03038
26. Myers JA, Miller JS. Exploring the NK cell platform for cancer immunotherapy. Nat Rev Clin Oncol. (2021) 18:85–100. doi: 10.1038/s41571-020-0426-7
27. Kumar V, Patel S, Tcyganov E, Gabrilovich DI. The nature of myeloid-derived suppressor cells in the tumor microenvironment. Trends Immunol. (2016) 37:208–20. doi: 10.1016/j.it.2016.01.004
28. Farhood B, Najafi M, Mortezaee K. CD8(+) cytotoxic T lymphocytes in cancer immunotherapy: a review. J Cell Physiol. (2019) 234:8509–21. doi: 10.1002/jcp.27782
29. Wu K, Lin K, Li X, Yuan X, Xu P, Ni P, et al. Redefining tumor-associated macrophage subpopulations and functions in the tumor microenvironment. Front Immunol. (2020) 11:1731. doi: 10.3389/fimmu.2020.01731
30. Pan Y, Yu Y, Wang X, Zhang T. Tumor-associated macrophages in tumor immunity. Front Immunol. (2020) 11:583084. doi: 10.3389/fimmu.2020.583084
31. Zhu J. T helper cell differentiation, heterogeneity, and plasticity. Cold Spring Harb Perspect Biol. (2018) 10:a030338. doi: 10.1101/cshperspect.a030338
32. Chen L, Huang CF, Li YC, Deng WW, Mao L, Wu L, et al. Blockage of the NLRP3 inflammasome by MCC950 improves anti-tumor immune responses in head and neck squamous cell carcinoma. Cell Mol Life Sci. (2018) 75:2045–58. doi: 10.1007/s00018-017-2720-9
33. Daley D, Mani VR, Mohan N, Akkad N, Pandian G, Savadkar S, et al. NLRP3 signaling drives macrophage-induced adaptive immune suppression in pancreatic carcinoma. J Exp Med. (2017) 214:1711–24. doi: 10.1084/jem.20161707
34. Wang F, Li G, Ning J, Chen L, Xu H, Kong X, et al. Alcohol accumulation promotes esophagitis via pyroptosis activation. Int J Biol Sci. (2018) 14:1245–55. doi: 10.7150/ijbs.24347
35. Barber G, Anand A, Katarzyna O, Phelan JJ, Heeran AB, Flis E, et al. Characterizing caspase-1 involvement during esophageal disease progression. Cancer Immunol Immunother. (2020) 69:2635–49. doi: 10.1007/s00262-020-02650-4
36. Pachathundikandi SK, Blaser N, Bruns H, Backert S. Helicobacter pylori avoids the critical activation of NLRP3 inflammasome-mediated production of oncogenic mature IL-1beta in human immune cells. Cancers. (2020) 12:803. doi: 10.3390/cancers12040803
37. Zhao P, Wang M, Chen M, Chen Z, Peng X, Zhou F, et al. Programming cell pyroptosis with biomimetic nanoparticles for solid tumor immunotherapy. Biomaterials. (2020) 254:120142. doi: 10.1016/j.biomaterials.2020.120142
38. Bollino D, Colunga A, Li B, Aurelian L. DeltaPK oncolytic activity includes modulation of the tumour cell milieu. J Gen Virol. (2016) 97:496–508. doi: 10.1099/jgv.0.000353
39. Wang Q, Wang Y, Ding J, Wang C, Zhou X, Gao W, et al. A bioorthogonal system reveals antitumour immune function of pyroptosis. Nature. (2020) 579:421–6. doi: 10.1038/s41586-020-2079-1
40. Gao Y, Zhang H, Zhou N, Xu P, Wang J, Gao Y, et al. Methotrexate-loaded tumour-cell-derived microvesicles can relieve biliary obstruction in patients with extrahepatic cholangiocarcinoma. Nat Biomed Eng. (2020) 4:743–53. doi: 10.1038/s41551-020-0583-0
41. Peng Z, Wang P, Song W, Yao Q, Li Y, Liu L, et al. GSDME enhances Cisplatin sensitivity to regress non-small cell lung carcinoma by mediating pyroptosis to trigger antitumor immunocyte infiltration. Signal Transduct Target Ther. (2020) 5:159. doi: 10.1038/s41392-020-00274-9
42. Springer M, Moco S. Resveratrol and its human metabolites-effects on metabolic health and obesity. Nutrients. (2019) 11:143. doi: 10.3390/nu11010143
43. Tang D, Chen K, Huang L, Li J. Pharmacokinetic properties and drug interactions of apigenin, a natural flavone. Expert Opin Drug Metab Toxicol. (2017) 13:323–30. doi: 10.1080/17425255.2017.1251903
44. Wang M, Firrman J, Liu L, Yam K. A review on flavonoid apigenin: dietary intake, ADME, antimicrobial effects, and interactions with human gut microbiota. Biomed Res Int. (2019) 2019:7010467. doi: 10.1155/2019/7010467
45. Adiwidjaja J, McLachlan AJ, Boddy AV. Curcumin as a clinically-promising anti-cancer agent: pharmacokinetics and drug interactions. Expert Opin Drug Metab Toxicol. (2017) 13:953–72. doi: 10.1080/17425255.2017.1360279
46. Tsuda T. Curcumin as a functional food-derived factor: degradation products, metabolites, bioactivity, and future perspectives. Food Funct. (2018) 9:705–14. doi: 10.1039/C7FO01242J
47. Fang J. Bioavailability of anthocyanins. Drug Metab Rev. (2014) 46:508–20. doi: 10.3109/03602532.2014.978080
48. Scholl C, Lepper A, Lehr T, Hanke N, Schneider KL, Brockmoller J, et al. Population nutrikinetics of green tea extract. PLoS ONE. (2018) 13:e0193074. doi: 10.1371/journal.pone.0193074
49. Gan RY, Li HB, Sui ZQ, Corke H. Absorption, metabolism, anti-cancer effect and molecular targets of epigallocatechin gallate (EGCG): an updated review. Crit Rev Food Sci Nutr. (2018) 58:924–41. doi: 10.1080/10408398.2016.1231168
50. Li Y, Yao J, Han C, Yang J, Chaudhry MT, Wang S, et al. Quercetin, inflammation and immunity. Nutrients. (2016) 8:167. doi: 10.3390/nu8030167
51. Liu J, Zhou X, Chen XY, Zhong DF. Excretion of [3H]triptolide and its metabolites in rats after oral administration. Acta Pharmacol Sin. (2014) 35:549–54. doi: 10.1038/aps.2013.192
52. Li XJ, Jiang ZZ, Zhang LY. Triptolide: progress on research in pharmacodynamics and toxicology. J Ethnopharmacol. (2014) 155:67–79. doi: 10.1016/j.jep.2014.06.006
53. Yang Z, Kulkarni K, Zhu W, Hu M. Bioavailability and pharmacokinetics of genistein: mechanistic studies on its ADME. Anticancer Agents Med Chem. (2012) 12:1264–80. doi: 10.2174/187152012803833107
54. Dabeek WM, Marra MV. Dietary quercetin and kaempferol: bioavailability and potential cardiovascular-related bioactivity in humans. Nutrients. (2019) 11:2288. doi: 10.3390/nu11102288
55. Bar-Sela G, Epelbaum R, Schaffer M. Curcumin as an anti-cancer agent: review of the gap between basic and clinical applications. Curr Med Chem. (2010) 17:190–7. doi: 10.2174/092986710790149738
56. Law SK, Simmons MP, Techen N, Khan IA, He MF, Shaw PC, et al. Molecular analyses of the Chinese herb leigongteng (Tripterygium wilfordii Hook.f.). Phytochemistry. (2011) 72:21–6. doi: 10.1016/j.phytochem.2010.10.015
57. Yahfoufi N, Alsadi N, Jambi M, Matar C. The immunomodulatory and anti-inflammatory role of polyphenols. Nutrients. (2018) 10:1618. doi: 10.3390/nu10111618
58. Fraga CG, Croft KD, Kennedy DO, Tomas-Barberan FA. The effects of polyphenols and other bioactives on human health. Food Funct. (2019) 10:514–28. doi: 10.1039/C8FO01997E
59. Cai M, Zhuang W, Lv E, Liu Z, Wang Y, Zhang W, et al. Kaemperfol alleviates pyroptosis and microglia-mediated neuroinflammation in Parkinson's disease via inhibiting p38MAPK/NF-kappaB signaling pathway. Neurochem Int. (2022) 152:105221. doi: 10.1016/j.neuint.2021.105221
60. Liu Z, Yao X, Sun B, Jiang W, Liao C, Dai X, et al. Pretreatment with kaempferol attenuates microglia-mediate neuroinflammation by inhibiting MAPKs-NF-kappaB signaling pathway and pyroptosis after secondary spinal cord injury. Free Radic Biol Med. (2021) 168:142–54. doi: 10.1016/j.freeradbiomed.2021.03.037
61. Tufekci KU, Eltutan BI, Isci KB, Genc S. Resveratrol Inhibits NLRP3 inflammasome-induced pyroptosis and miR-155 expression in microglia through sirt1/AMPK pathway. Neurotox Res. (2021) 39:1812–29. doi: 10.1007/s12640-021-00435-w
62. Yu W, Qin X, Zhang Y, Qiu P, Wang L, Zha W, et al. Curcumin suppresses doxorubicin-induced cardiomyocyte pyroptosis via a PI3K/Akt/mTOR-dependent manner. Cardiovasc Diagn Ther. (2020) 10:752–69. doi: 10.21037/cdt-19-707
63. Li W, Chen L, Xiao Y. Apigenin protects against ischemia-/hypoxia-induced myocardial injury by mediating pyroptosis and apoptosis. In Vitro Cell Dev Biol Anim. (2020) 56:307–12. doi: 10.1007/s11626-020-00434-9
64. Ding T, Zhao T, Li Y, Liu Z, Ding J, Ji B, et al. Vitexin exerts protective effects against calcium oxalate crystal-induced kidney pyroptosis in vivo and in vitro. Phytomedicine. (2021) 86:153562. doi: 10.1016/j.phymed.2021.153562
65. He C, Yang J, Jiang X, Liang X, Yin L, Yin Z, et al. Kaempferol alleviates LPS-ATP mediated inflammatory injury in splenic lymphocytes via regulation of the pyroptosis pathway in mice. Immunopharmacol Immunotoxicol. (2019) 41:538–48. doi: 10.1080/08923973.2019.1666405
66. Duan HN. Curcumin induces pyroptosis of human breast cancer cells (MCF-7) through autophagy-CTSB-inflammasome signaling pathway. Dalian Med Univ. (2021).
67. Yue E, Tuguzbaeva G, Chen X, Qin Y, Li A, Sun X, et al. Anthocyanin is involved in the activation of pyroptosis in oral squamous cell carcinoma. Phytomedicine. (2019) 56:286–94. doi: 10.1016/j.phymed.2018.09.223
68. Binfen H, Zhao L, Deng M. Chrysophanol inhibits the progression of gastric cancer by activating NLRP3. Res Square. (2021). doi: 10.21203/rs.3.rs-1073406/v1
69. Cai J, Yi M, Tan Y, Li X, Li G, Zeng Z, et al. Natural product triptolide induces GSDME-mediated pyroptosis in head and neck cancer through suppressing mitochondrial hexokinase-IotaIota. J Exp Clin Cancer Res. (2021) 40:190. doi: 10.1186/s13046-021-01995-7
70. Liang WF, Gong YX, Li HF, Sun FL, Li WL, Chen DQ, et al. Curcumin activates ROS signaling to promote pyroptosis in hepatocellular carcinoma HepG2 cells. In Vivo. (2021) 35:249–57. doi: 10.21873/invivo.12253
71. Chen S, Ma J, Yang L, Teng M, Lai ZQ, Chen X, et al. Anti-glioblastoma activity of kaempferol via programmed cell death induction: involvement of autophagy and pyroptosis. Front Bioeng Biotechnol. (2020) 8:614419. doi: 10.3389/fbioe.2020.614419
72. Kong Y, Feng Z, Chen A, Qi Q, Han M, Wang S, et al. The natural flavonoid galangin elicits apoptosis, pyroptosis, and autophagy in glioblastoma. Front Oncol. (2019) 9:942. doi: 10.3389/fonc.2019.00942
73. Tan JQ, Li Z, Chen G, Wu M, Feng JL, Kong SY, et al. The natural compound from garcinia bracteata mainly induces GSDME-mediated pyroptosis in esophageal cancer cells. Phytomedicine. (2022) 102:154142. doi: 10.1016/j.phymed.2022.154142
74. Zhang F, Liu Q, Ganesan K, Kewu Z, Shen J, Gang F, et al. The antitriple negative breast cancer efficacy of spatholobus suberectus dunn on ROS-Induced noncanonical inflammasome pyroptotic pathway. Oxid Med Cell Longev. (2021) 2021:5187569. doi: 10.1155/2021/5187569
75. Miller JM, Thompson JK, MacPherson MB, Beuschel SL, Westbom CM, Sayan M, et al. Curcumin: a double hit on malignant mesothelioma. Cancer Prev Res. (2014) 7:330–40. doi: 10.1158/1940-6207.CAPR-13-0259
76. Zhu JX, Zhu WT, Hu JH, Yang W, Liu P, Liu QH, et al. Curcumin-Loaded Poly(L-lactide-co-glycolide) microbubble-mediated sono-photodynamic therapy in liver cancer cells. Ultrasound Med Biol. (2020) 46:2030–43. doi: 10.1016/j.ultrasmedbio.2020.03.030
77. Pujari I, Thomas A, Thomas J, Jhawar N, Guruprasad KP, Rai PS, et al. Cytotoxicity and radiosensitizing potency of Moscatilin in cancer cells at low radiation doses of X-ray and UV-C. Biotech. (2021) 11:281. doi: 10.1007/s13205-021-02827-3
78. Sharma A, Kaur M, Katnoria JK, Nagpal AK. Polyphenols in food: cancer prevention and apoptosis induction. Curr Med Chem. (2018) 25:4740–57. doi: 10.2174/0929867324666171006144208
79. Lesjak M, Simin N, Srai SKS. Can polyphenols inhibit ferroptosis? Antioxidants. (2022) 11:150. doi: 10.3390/antiox11010150
80. Musial C, Siedlecka-Kroplewska K, Kmiec Z, Gorska-Ponikowska M. Modulation of autophagy in cancer cells by dietary polyphenols. Antioxidants. (2021) 10:123. doi: 10.3390/antiox10010123
81. Sun C, Mezzadra R, Schumacher TN. Regulation and function of the PD-L1 checkpoint. Immunity. (2018) 48:434–52. doi: 10.1016/j.immuni.2018.03.014
82. Verdura S, Cuyas E, Cortada E, Brunet J, Lopez-Bonet E, Martin-Castillo B, et al. Resveratrol targets PD-L1 glycosylation and dimerization to enhance antitumor T-cell immunity. Aging. (2020) 12:8–34. doi: 10.18632/aging.102646
83. Yang M, Li Z, Tao J, Hu H, Li Z, Zhang Z, et al. Resveratrol induces PD-L1 expression through snail-driven activation of Wnt pathway in lung cancer cells. J Cancer Res Clin Oncol. (2021) 147:1101–13. doi: 10.1007/s00432-021-03510-z
84. Jiang ZB, Wang WJ, Xu C, Xie YJ, Wang XR, Zhang YZ, et al. Luteolin and its derivative apigenin suppress the inducible PD-L1 expression to improve anti-tumor immunity in KRAS-mutant lung cancer. Cancer Lett. (2021) 515:36–48. doi: 10.1016/j.canlet.2021.05.019
85. Xu L, Zhang Y, Tian K, Chen X, Zhang R, Mu X, et al. Apigenin suppresses PD-L1 expression in melanoma and host dendritic cells to elicit synergistic therapeutic effects. J Exp Clin Cancer Res. (2018) 37:261. doi: 10.1186/s13046-018-0929-6
86. Liao F, Liu L, Luo E, Hu J. Curcumin enhances anti-tumor immune response in tongue squamous cell carcinoma. Arch Oral Biol. (2018) 92:32–7. doi: 10.1016/j.archoralbio.2018.04.015
87. Guo L, Li H, Fan T, Ma Y, Wang L. Synergistic efficacy of curcumin and anti-programmed cell death-1 in hepatocellular carcinoma. Life Sci. (2021) 279:119359. doi: 10.1016/j.lfs.2021.119359
88. Liu D, You M, Xu Y, Li F, Zhang D, Li X, et al. Inhibition of curcumin on myeloid-derived suppressor cells is requisite for controlling lung cancer. Int Immunopharmacol. (2016) 39:265–72. doi: 10.1016/j.intimp.2016.07.035
89. Abd-Rabou AA, Zoheir KM, Ahmed HH. Potential impact of curcumin and taurine on human hepatoma cells using Huh-7 cell line. Clin Biochem. (2012) 45:1519–21. doi: 10.1016/j.clinbiochem.2012.06.032
90. Halder RC, Almasi A, Sagong B, Leung J, Jewett A, Fiala M. Curcuminoids and omega-3 fatty acids with anti-oxidants potentiate cytotoxicity of natural killer cells against pancreatic ductal adenocarcinoma cells and inhibit interferon gamma production. Front Physiol. (2015) 6:129. doi: 10.3389/fphys.2015.00129
91. Fiala M. Curcumin and omega-3 fatty acids enhance NK cell-induced apoptosis of pancreatic cancer cells but curcumin inhibits interferon-gamma production: benefits of omega-3 with curcumin against cancer. Molecules. (2015) 20:3020–6. doi: 10.3390/molecules20023020
92. Kotting C, Hofmann L, Lotfi R, Engelhardt D, Laban S, Schuler PJ, et al. Immune-stimulatory effects of curcumin on the tumor microenvironment in head and neck squamous cell carcinoma. Cancers. (2021) 13:1335. doi: 10.3390/cancers13061335
93. Lee HH, Cho H. Improved anti-cancer effect of curcumin on breast cancer cells by increasing the activity of natural killer cells. J Microbiol Biotechnol. (2018) 28:874–82. doi: 10.4014/jmb.1801.01074
94. Lee Y, Shin H, Kim J. In vivo anti-cancer effects of resveratrol mediated by NK cell activation. J Innate Immun. (2021) 13:94–106. doi: 10.1159/000510315
95. Zhang Q, Huang H, Zheng F, Liu H, Qiu F, Chen Y, et al. Resveratrol exerts antitumor effects by downregulating CD8(+)CD122(+) Tregs in murine hepatocellular carcinoma. Oncoimmunology. (2020) 9:1829346. doi: 10.1080/2162402X.2020.1829346
96. Peiffer DS, Wang LS, Zimmerman NP, Ransom BW, Carmella SG, Kuo CT, et al. Dietary consumption of black raspberries or their anthocyanin constituents alters innate immune cell trafficking in esophageal cancer. Cancer Immunol Res. (2016) 4:72–82. doi: 10.1158/2326-6066.CIR-15-0091
97. Xu P, Yan F, Zhao Y, Chen X, Sun S, Wang Y, et al. Green tea polyphenol EGCG attenuates MDSCs-mediated immunosuppression through canonical and non-canonical pathways in a 4T1 murine breast cancer model. Nutrients. (2020) 12:1042. doi: 10.3390/nu12041042
98. Zhang X, Cook KL, Warri A, Cruz IM, Rosim M, Riskin J, et al. Lifetime genistein intake increases the response of mammary tumors to tamoxifen in rats. Clin Cancer Res. (2017) 23:814–24. doi: 10.1158/1078-0432.CCR-16-1735
99. Villalobos-Ayala K, Ortiz Rivera I, Alvarez C, Husain K, DeLoach D, Krystal G, et al. Apigenin increases SHIP-1 expression, promotes tumoricidal macrophages and anti-tumor immune responses in murine pancreatic cancer. Cancers. (2020) 12:3631. doi: 10.3390/cancers12123631
100. Jang JY, Lee JK, Jeon YK, Kim CW. Exosome derived from epigallocatechin gallate treated breast cancer cells suppresses tumor growth by inhibiting tumor-associated macrophage infiltration and M2 polarization. BMC Cancer. (2013) 13:421. doi: 10.1186/1471-2407-13-421
101. Jeong SK, Yang K, Park YS, Choi YJ, Oh SJ, Lee CW, et al. Interferon gamma induced by resveratrol analog, HS-1793, reverses the properties of tumor associated macrophages. Int Immunopharmacol. (2014) 22:303–10. doi: 10.1016/j.intimp.2014.07.004
102. Xiao Z, Su Z, Han S, Huang J, Lin L, Shuai X. Dual pH-sensitive nanodrug blocks PD-1 immune checkpoint and uses T cells to deliver NF-kappaB inhibitor for antitumor immunotherapy. Sci Adv. (2020) 6:eaay7785. doi: 10.1126/sciadv.aay7785
103. Mukherjee S, Fried A, Hussaini R, White R, Baidoo J, Yalamanchi S, et al. Phytosomal curcumin causes natural killer cell-dependent repolarization of glioblastoma (GBM) tumor-associated microglia/macrophages and elimination of GBM and GBM stem cells. J Exp Clin Cancer Res. (2018) 37:168. doi: 10.1186/s13046-018-0792-5
104. Mukherjee S, Baidoo JNE, Sampat S, Mancuso A, David L, Cohen LS, et al. Liposomal TriCurin, a synergistic combination of curcumin, epicatechin gallate and resveratrol, repolarizes tumor-associated microglia/macrophages, and eliminates glioblastoma (GBM) and GBM stem cells. Molecules. (2018) 23:201. doi: 10.3390/molecules23010201
105. Mukherjee S, Hussaini R, White R, Atwi D, Fried A, Sampat S, et al. TriCurin, a synergistic formulation of curcumin, resveratrol, and epicatechin gallate, repolarizes tumor-associated macrophages and triggers an immune response to cause suppression of HPV+ tumors. Cancer Immunol Immunother. (2018) 67:761–74. doi: 10.1007/s00262-018-2130-3
106. Kuo IM, Lee JJ, Wang YS, Chiang HC, Huang CC, Hsieh PJ, et al. Potential enhancement of host immunity and anti-tumor efficacy of nanoscale curcumin and resveratrol in colorectal cancers by modulated electro- hyperthermia. BMC Cancer. (2020) 20:603. doi: 10.1186/s12885-020-07072-0
107. Milano F, Mari L, van de Luijtgaarden W, Parikh K, Calpe S, Krishnadath KK. Nano-curcumin inhibits proliferation of esophageal adenocarcinoma cells and enhances the T cell mediated immune response. Front Oncol. (2013) 3:137. doi: 10.3389/fonc.2013.00137
108. Hong EH, Heo EY, Song JH, Kwon BE, Lee JY, Park Y, et al. Trans-scirpusin A showed antitumor effects via autophagy activation and apoptosis induction of colorectal cancer cells. Oncotarget. (2017) 8:41401–11. doi: 10.18632/oncotarget.17388
109. MaruYama T, Kobayashi S, Nakatsukasa H, Moritoki Y, Taguchi D, Sunagawa Y, et al. The curcumin analog GO-Y030 controls the generation and stability of regulatory T cells. Front Immunol. (2021) 12:687669. doi: 10.3389/fimmu.2021.687669
110. Hu H, Huang G, Wang H, Li X, Wang X, Feng Y, et al. Inhibition effect of triptolide on human epithelial ovarian cancer via adjusting cellular immunity and angiogenesis. Oncol Rep. (2018) 39:1191–6. doi: 10.3892/or.2017.6158
111. Omene C, Ma L, Moore J, Ouyang H, Illa-Bochaca I, Chou W, et al. Aggressive mammary cancers lacking lymphocytic infiltration arise in irradiated mice and can be prevented by dietary intervention. Cancer Immunol Res. (2020) 8:217–29. doi: 10.1158/2326-6066.CIR-19-0253
112. Kim JS, Jeong SK, Oh SJ, Lee CG, Kang YR, Jo WS, et al. The resveratrol analogue, HS1793, enhances the effects of radiation therapy through the induction of antitumor immunity in mammary tumor growth. Int J Oncol. (2020) 56:1405–16. doi: 10.3892/ijo.2020.5017
113. Xiao J, Wang C, Yao JC, Alippe Y, Xu C, Kress D, et al. Gasdermin D mediates the pathogenesis of neonatal-onset multisystem inflammatory disease in mice. PLoS Biol. (2018) 16:e3000047. doi: 10.1371/journal.pbio.3000047
114. Liu Y, Wang Z, Gan Y, Chen X, Zhang B, Chen Z, et al. Curcumin attenuates prostatic hyperplasia caused by inflammation via up-regulation of bone morphogenetic protein and activin membrane-bound inhibitor. Pharm Biol. (2021) 59:1026–35. doi: 10.1080/13880209.2021.1953539
115. Mokgalaboni K, Ntamo Y, Ziqubu K, Nyambuya TM, Nkambule BB, Mazibuko-Mbeje SE, et al. Curcumin supplementation improves biomarkers of oxidative stress and inflammation in conditions of obesity, type 2 diabetes and NAFLD: updating the status of clinical evidence. Food Funct. (2021) 12:12235–49. doi: 10.1039/D1FO02696H
Keywords: tumor immune microenvironment, dietary polyphenols, pyroptosis, curcumin, antitumor immunity
Citation: Huang X, Wang Y, Yang W, Dong J and Li L (2022) Regulation of dietary polyphenols on cancer cell pyroptosis and the tumor immune microenvironment. Front. Nutr. 9:974896. doi: 10.3389/fnut.2022.974896
Received: 21 June 2022; Accepted: 29 July 2022;
Published: 25 August 2022.
Edited by:
Guiyan Yang, University of California, Davis, United StatesReviewed by:
Hongxiu Diao, China Agricultural University, ChinaCopyright © 2022 Huang, Wang, Yang, Dong and Li. This is an open-access article distributed under the terms of the Creative Commons Attribution License (CC BY). The use, distribution or reproduction in other forums is permitted, provided the original author(s) and the copyright owner(s) are credited and that the original publication in this journal is cited, in accordance with accepted academic practice. No use, distribution or reproduction is permitted which does not comply with these terms.
*Correspondence: Jing Dong, ZG9uZ2ppbmc5ODM0QHN5YXUuZWR1LmNu; Lin Li, bGlsaW42MTk2MTlAc3lhdS5lZHUuY24=
Disclaimer: All claims expressed in this article are solely those of the authors and do not necessarily represent those of their affiliated organizations, or those of the publisher, the editors and the reviewers. Any product that may be evaluated in this article or claim that may be made by its manufacturer is not guaranteed or endorsed by the publisher.
Research integrity at Frontiers
Learn more about the work of our research integrity team to safeguard the quality of each article we publish.