- School of Food and Biological Engineering, Hefei University of Technology, Hefei, China
During food processing, especially heating, the flavor and color of food change to a great extent due to Maillard reaction (MR). MR is a natural process for improving the flavor in various model systems and food products. Maillard reaction Products (MRPs) serve as ideal materials for the production of diverse flavors, which ultimately improve the flavor or reduce the odor of raw materials. Due to the complexity of the reaction, MR is affected by various factors, such as protein source, hydrolysis conditions, polypeptide molecular weight, temperature, and pH. In the recent years, much emphasis is given on conditional MR that could be used in producing of flavor-enhancing peptides and other compounds to increase the consumer preference and acceptability of processed foods. Recent reviews have highlighted the effects of MR on the functional and biological properties, without elaborating the flavor compounds obtained by the MR. In this review, we have mainly introduced the Maillard reaction-derived flavors (MF), the main substances producing MF, and detection methods. Subsequently, the main factors influencing MF, from the selection of materials (sugar sources, protein sources, enzymatic hydrolysis methods, molecular weights of peptides) to the reaction conditions (temperature, pH), are also described. In addition, the existing adverse effects of MR on the biological properties of protein are also pointed out.
Highlights
- Implicating the efficiency of MR as natural method for improving flavor in different food model systems and resulting food products.
- Introducing the different Maillard reaction-derived flavors, with an emphasis on the flavor substances.
- A well-controlled MR may be useful in the production of flavor-enhancing peptides and other compounds to increase the consumer preference and acceptability of processed foods.
- The negative effects of Maillard reaction and its solutions are proposed.
- Highlighting the recent application trend of MRPs as flavor agents.
Introduction
The Maillard reaction (MR) is a non-enzymatic reaction that occurs when the carbonyl group of reducing sugars reacts with the amino group of amino acids, polypeptides, or proteins, resulting in the natural production of Maillard reaction products (MRPs), a class of compounds with a wide range of sensory properties (1). Due to the heteroatoms in amino acids, the resultant scents are heterocyclic compounds with ring structures including an atom of N, O, S, or mixtures of these. In food industry, different flavor and color are produced during the MR, especially as food is heated. This is known not only to alter the food properties (flavor, color, and odor), but also to enhance the functional properties (antioxidant and bacteriostasis) of amino acids, peptides, and proteins (2, 3). Chiang et al. (4) explored how heat treatment affected the volatile profiles of beef bone hydrolysates, and found that MR can be used to alter the flavor characteristics of beef bone hydrolysates as a natural meat flavor product. Song et al. (5) detected the flavor substances in pepper powder and revealed that adding exogenous MR substrate can offer a mechanism to improve BPP flavor quality. In another study, sensory evaluation revealed that soybean protein-derived MRPs have higher umami and caramel traits than soybean protein controls (6). The bitter taste and off-flavor of peptides are regarded as serious challenges to their use in the food industry. The most frequent method for removing or reducing beany flavor components is heating (7). Chen et al. (8) modified the Cucumaria frondosa hydrolysate with glucose/xylooligosaccharide by MR which largely increased desirable aroma compounds and reduced off-flavor compounds, improving the overall flavor. These studies implicit the efficiency of MR as natural method for improving flavor in different food model systems and resulting food products.
In MR, flavor relates to peptides of various molecular weights present in amine group substrates. After the reaction, the content of flavor precursors in the resulting products increased significantly, and their umami and strong taste were also improved (9). Until now, over 100 umami peptides (with 2–11 amino acids) responsible for umami or kokumi taste from different sources have been distinguished, such as pea protein hydrolysates (10), sweet potato protein hydrolysates (11), edible mushrooms (12), peanut protein (13), and Takifugu rubripes (14). It has been evident that different MR conditions result in different flavor products. Since the open-chain concentrations of sugars and active forms of amino reactants depend on pH (15). Lotfy et al. (16) heated the Quinoa protein hydrolysates at varying initial pH values and increased the pH from 5 to 9 to change the sensory attributes from caramel to burnt-coffee. Furthermore, MR is made up of a chain of complex reactions, in which the sensitivity of each reaction and reactant to temperature are different. At higher temperatures, bitter and umami FAAs were present in higher concentrations (17). Thus, a well-controlled MR may be useful in the production of flavor-enhancing peptides and other compounds to increase the consumer preference and acceptability of processed foods.
Recent reviews on this research area have mainly focused on the MR during processing (18, 19), or their effects on functional and biological properties (2, 20), without elaborating the flavor compounds obtained by the MR. To bridge the knowledge gap, we aim to introduce the MR and different MF, with an emphasis on the flavor substances. The influencing factors, flavor formation mechanism of MRPs, and widely used flavor detection methods are also analyzed. Besides, we highlight the recent application trend of MRPs as flavor agents. The development direction and trend are comprehensively discussed, to provide theoretical support for expanding the existing application standing of MRPs.
Maillard reaction-derived flavors
As the foods containing proteins/peptides and carbohydrates are heated, MR (formation of covalent bonds between carbonyl groups and free amino groups) is a common reaction (17). It started in 1912 with French chemist Louis Camille Maillard, who studied the interaction between glucose and glycine and labeled the resultant dark pigment melanin. Generally, in MR there are three steps, namely primary stage, medium stage, and final stage, as shown in Figure 1.
Amadori products of the primary stage are often used as an indicator of food processing degree (21). In a simple model of cysteine and xylose, relatively stable cyclic 2-3-thiazolidine-4-carboxylic acid (TTCA) and the Amadori rearrangement product of cysteine are formed at the early stage. Gly-Amadori reacts fastest, followed by Cys-Amadori and TTCA. Free glycine accelerates the reaction of TTCA, whereas cysteine inhibits that of Gly-Amadori due to forming relatively stable thiazolidines. Cys-Amadori/Gly has the highest efficiency in developing both meaty flavors and brown products. TTCA/Gly is conducive to yield meaty flavors, whereas Gly-Amadori/Cys is conducive to generate brown products (22). Conclusively, initial formation of initial intermediates/pathways could be regulated for the maximal formation of related flavors.
In the medium stage, the ketone aldehydes and other compounds produced in this process are the flavor precursors and flavor substances of foods. For example, amino ketones could be converted to enol-amines through isomerization. Strecker aldehydes and acids, which have strong aromatic potential, produce different aroma characteristics according to the types of amino acids and act as precursors. Various special aldehydes could be generated by the MR of different α-amino acids and reducing sugars as Strecker degradation (23). Therefore, Strecker degradation is related to browning and aroma production. For example, Val reacts with glucose, chocolate aroma is produced (24). The reaction of Lys and Pro with glucose produces the aroma of toasted bread (25).
The final stage mainly includes dehydrogenation, cyclization, retro-Aldol reaction, isomerization, rearrangement and condensation. Nitrogen-containing dark compounds, namely melanoidins, are formed eventually. In addition, the carbohydrate in MR also is cracked after heating. Ketones, keto alcohols, ketoaldehydes and other crackates react with amino compounds, before the final MRPs are further formed (26).
MF refers to characteristic flavor formed by MR during food processing and storage. The flavor characteristics could be pleasant floral, nutty, caramel, attractive meat, or even spicy (5, 27, 28), depending on the types, composition or reaction pathways of aminos and carbonyls. In 1960, Morton prepared meat flavor by using reducing sugar and cysteine, and applied for the first patent on MF in the UK. Since then, the research on MFs have been continuously progressing. MF is caused by MR that produces compounds, such as aldehydes, ketones, furans, thiophenes, pyrazines, pyrrols, etc. These compounds (MRPs) contribute to the overall flavor perception in foods during the processing. Due to the edible safety of MRPs and the high efficiency of flavor enhancement, the preparation of MFs through thermal reaction has gained an immense focus from researchers engaged in flavor industry and food processing ingredients (8, 16). At present, the key technologies for thermal reaction flavors have been developed, which are composed of adipo-regulated oxidation (28), targeted biocatalysis (28), and temperature-controlled (17).
Flavor ingredients of MRPs and detection methods
Flavor ingredients of MRPs
Flavor components in MR
Flavor is an important characteristic of MRPs and flavor substances are initially formed in the middle stage of MR. The structure, formation process, and flavor characteristics of some representative flavor substances are summarized in Table 1. Most of MRPs are furans and their derivatives, and the precursors of other condiments (such as thiazole and furan mercaptan). Linoleic acid and other n-6 including 2-pentylfuran, which is a non-carboxylated molecule (28). 1-Octen-3-ol was degraded from lipids and had a mushroom and baking aroma (36), as well as antibacterial efficacy against spoilage and opportunistic microorganisms (37). The MR generated furan compounds, which are a key odor group for hibiscus flowers (38); 3-methylbutanal provides a malty scent, while pyrazines (typical MRPs) contribute a barbeque taste to food, with higher pyrazine levels owing to the possibility of lipid-derived active carbonyl and ammonia reactions (39). MR is known to significantly contribute to baked red pepper powder flavor due to these compounds (5). Meat flavor is characterized by sulfur-containing volatile compounds with sulfury and meaty aromas. Meaty tastes described with high odor in model MR containing cysteine including 2-methyl-3-furanthiol, 2-furfurylthiol, 3-thiophenethiol, 3-mercapto-2-pentanone, bis(2-methyl-3-furyl)disulfide, and 2-methylthiophene (40).
Furfural, 5-methyl furfural, 2-acetyl furan, maltol, iso-maltol, and other substances have strong caramel and fruit aroma. 2, 5-Dimethyl-4-hydroxy-3-furanone and its 5-methyl homolog have a pleasant caramel taste and roasted pineapple smell. Dicarbonyl compounds (such as butanedione) with buttery aroma are obtained by deoxyglucone rearrangement and dehydration. Aldehydes, such as 3-methyl-butyraldehyde, are formed through Strecker degradation by dicarbonyl compounds, and present a refreshing malt aroma. Pyrazines, which are a kind of flavor compound with pleasant mood and nutty aroma, have attracted much attention from flavor chemistry researchers since the 1960s. They're generally produced by condensation of -aminoketone, which is formed through Strecker degradation. Similarly, pyrrole and its derivatives formed by dehydration and cyclization of 3-deoxyketone with amino compounds are also important flavor substances. When proteins react with methylglyoxal or dihydroxyacetone, 2-acetyl-1-pyrroline with bread aroma is produced. The sulfur-containing flavor substances formed directly relate to sulfur-containing amino acids in the reaction system. Hydrogen sulfide and ammonia are easily produced by cysteine hydrolyzation or Strecker degradation. Similarly, thiophenes are formed by hydrogen sulfide\deoxyglucose after dehydration and oxidation. Recently, MRPs derived from protein hydrolysates/peptides and carbs have been shown in numerous researches to impact the flavor properties of food (8, 28, 41). Table 2 summarizes the flavor attributes of MRPs generated from different protein hydrolysates/peptides and carbs.
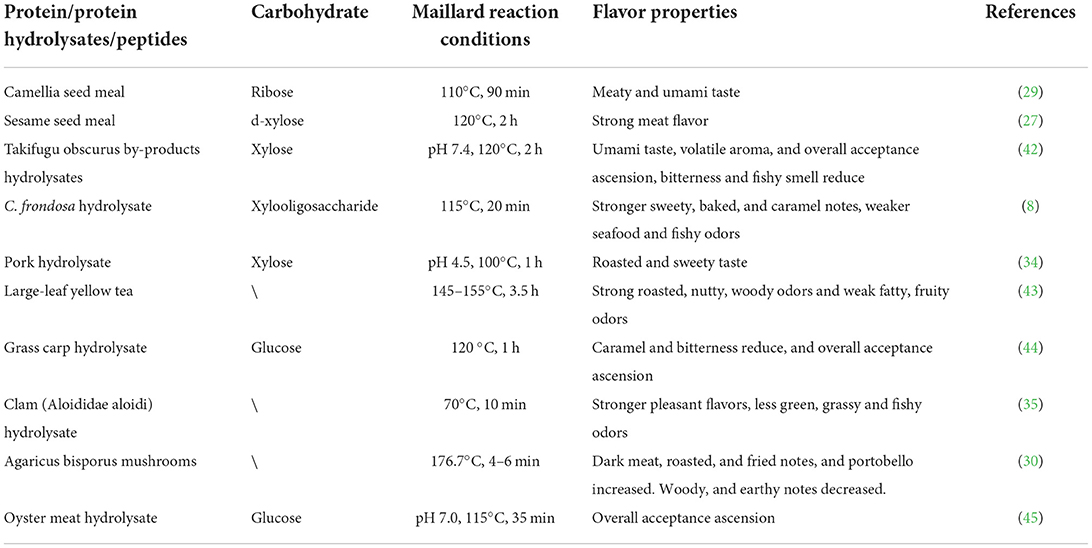
Table 2. Summary of the recent studies on flavor properties of Maillard reaction products generated from various protein/ protein hydrolysates/peptides and carbohydrate sources.
In the heated cysteine-xylic-glycine system, the possible processes of different compounds are summarized in Figure 2. 2-Methyltetrahydrothiophene-3-ketone could be formed from 1-deoxypentanone through reduction. Furfural may be generated from 3-deoxypentosone via cyclization and dehydration, while 2-furfurylthiol could be formed from the reaction of ffurfural and H2S. Besides, 2-methylfuran could be formed after the reduction of furfural. After 2,3-pentadione reacting with H2S, 3-mercapto-2-pentanone may be generated. Analogously, after cyclization and dehydration with hydrogen sulfide, 3-thiophene mercaptan is formed. Subsequently, 2-ethylthiophene is formed through dehydration and reduction. As aromatization occurs, 2,5-dimethylthiophene is formed (40).
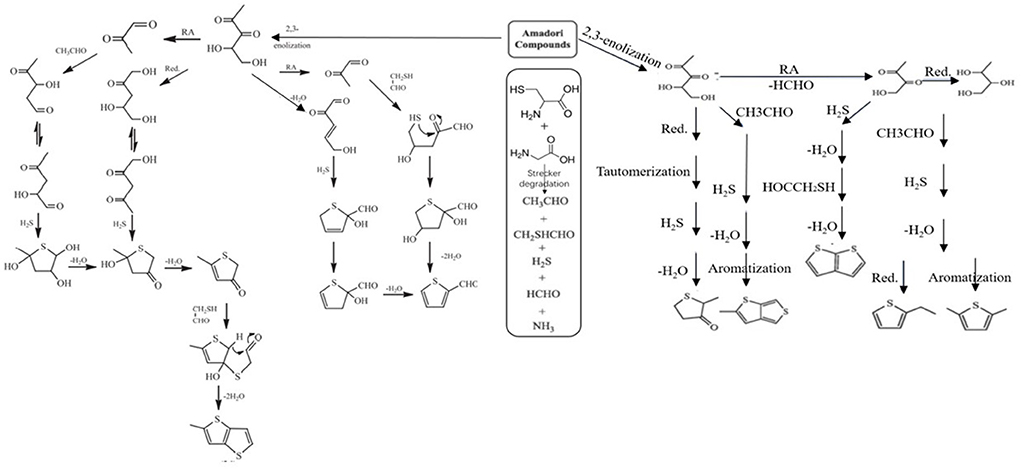
Figure 2. Possible formation pathways for compounds in the Gly-Cys reaction systems (Red., reduction reaction; RA, retro-aldol reaction) (40).
Flavor components in foods associated with MR
In heat processed foods, such as coffee, cocoa, bread, peanuts, pork, beef, chicken, fish and potatoes, most aromatic substances are produced by MR. Over 400 volatile compounds including pyrazines, thiazoles, oxazoles, pyrrole derivatives, furans, and pyridine derivatives have been identified in roasted cocoa. Furthermore, the pattern of alkyl pyrazines generation depends on oligopeptides and free amino acids presented in the particular foods before roasting (46). Kocadagli et al. (32) selected precursors to add to potato dough before baking for improving the baked flavor of low-acrylamide potato products. Due to the reactive complexities of tea, noticeable changes such as pigment destruction, oxidative polymerization of catechin, and oxidative degradation of amino acids have been recorded following high-temperature esterification and long-term storage. Compared with the roasted spring and autumn tea leaves, the color, stewing aroma, taste and chemical composition of pan-fired spring tea leaves changed to least, indicating that baking enhanced the flavor stability of tea drinks (47).
In addition to improving the food taste, MR is also used to improve the food smell. For example, shellfish hydrolysates usually produce strong unpleasant odors (such as fishy odors), which is the key reason for their limited application (47). After MR, the number of volatile components detected in the enzymatic hydrolysate of clams was changed insignificantly. Nevertheless, there were differences in the types, and the contents of aldehydes, ketones and esters were also increased. After enzymatic hydrolysis, MR eliminated the unpleasant smell of oyster, and a pleasant smell of milk, nut and meat was generated at the same time (48).
Extraction and detection methods of MRPs
Extraction and analysis are important technical strategies to study the food flavor components. Flavor components are mainly composed of many volatile substances with complex structures and different chemical properties. They act on olfactory organs to transmit information, and their contents are usually below the nanogram level. Therefore, it is relatively difficult to analyze their chemical composition by conventional chemical methods. Analysis of the chemical composition usually includes separation, enrichment, desorption, and identification of volatile components. All the processes should avoid oxidation, thermal degradation and other biochemical reactions, which are the prerequisites for pretreatment of volatile compounds. Common methods for samples pretreatments include solvent extraction (SE), simultaneous distillation extraction (SDE), headspace extraction (HS), solvent assisted flavor evaporation (SAFE), super-critical fluid extraction (SFE), purge and trap method (PT), solid phase microextraction (SPME), etc. The commonly used analytical instruments for the identification of volatile aroma compounds in food systems include electronic nose (E-nose), gas chromatography (GC), and high-performance liquid chromatography (HPLC).
E-nose is a rapid detection system suitable for detecting large number of samples, without the need to separate the volatiles. Using E-nose as a human sensory simulator, sensory evaluation could be conducted conveniently and sensitively. Currently, E-nose is used to analyze the overall level of aroma components for MR conditions optimization (49). However, the electronic nose cannot detect the quantity and content of each substance in MRPs. An useful analytical technique for identifying MRPs is HPLC or anion-exchange chromatography in combination with a diode array detector (DAD) and an evaporative light scattering detector (ELSD) (50). The majority of these approaches, nonetheless, did not succeed in clearly identifying each MRP with good peak shape and resolution. The high polarity of MRPs, which results in early elution with many compounds in the reversed phase (RP) column and substantial retention in the normal phase (NP) column, is attributed as one major cause. Satisfactory separation of polar substances is now possible thanks to the recent invention of the hydrophilic interaction liquid chromatography (HILIC) column, which strikes a fair compromise between RP and NP columns. Its use concurrently separates the amino acids glycine, diglycine, and triglycine as well as the associated MR intermediate (51).
These analytical techniques have limitations, such as low selectivity, especially when compared to cutting-edge analytical techniques utilizing mass spectrometry (MS) and nuclear magnetic resonance (NMR) spectroscopy (52). For the analysis of both tiny and large organic compounds, MS is regarded as one of the fundamental methods (53). Sensory assessment, GC-MS and E-nose were used to investigate the flavor differences of five sections of the Chinese blanched chicken (CBC): skin, breast, thigh, head, and butt. This examination of several aspects of CBC might give guidance on how to cater consumers' preferences and forecast the quality changes that may occur. Additionally, using a combination of GC-MS and E-nose, the volatile and taste components of foods (such as golden pompano filets, roasted coffee beans, apple fruits, and so on) were evaluated to gain complete information on flavor profiles. Nowadays, GC-MS is a popular approach for analyzing aroma that has been widely applied in meat science. For example, the investigation of volatile profiles of fermented sausages, dry-cured hams, and bacons (54, 55). It provides an accurate approach to qualitatively and quantitatively analyze the volatile and semi-volatile compounds (56). GC-MS, on the other hand, requires extra derivatization and is not appropriate for large-scale detection (57).
MS is a standard mass detector that detects ionized chemicals and delivers mass spectra and compound structures at each location. MS/MS or even MS3 approaches improved selectivity and structure identification by analyzing each ion fragment of a molecular ion (58). In a recent investigation, the standards of glycine-glucose and proline-glucose-Schiff bases, Amadori, and Heyns compounds were well separated by ESI-MS/MS (59). Additionally, techniques utilizing LC-MS/MS and stable isotope dilution assay were proposed (60). The identification of tiny molecules like ARPs in a complex matrix becomes feasible and reliable, which is favorable and has a wide range of applications, despite certain hurdles brought by the high cost of MS detection and onerous technique.
A key component of MS approach is the MS Library; flavor analysis, for instance, benefits from the NIST library (61). A dedicated library for Maillard reaction chemicals does not exist, though. On the basis of ion fragments, researchers must infer the molecule and/or further confirmed by NMR. Only a few attempts have been made to create tiny libraries of sulfur-methyl thioesters so far, and they haven't been put into too much use. Therefore, the creation of a thorough library of MRPs would be a significant addition.
Influencing factors of MF
Influencing factors of MF
The sort of amino acids and reducing sugars, as well as the reaction circumstances (such as temperature, time and pH), play a big role in flavor development via MR. The sugar has only a limited influence on flavor character, while the amino acid has a major impact. Rather than altering the flavor character, sugars have a significant impact on the reaction rate. The influence of different influencing factors of MRPs is showed in Table 3.
Reducing sugar
The MR refers to the reaction between an amino group (e.g., amino acids) and a carbonyl group (e.g., reducing sugars), a non-enzymatic modification of proteins with reducing sugars. Therefore, sugars were a key participant of MR, and the content and structure of reducing sugars have an important effect on the MR and the structure and flavor of MRPs. The level of the reducing sugars inside the beans has a potential impact on coffee aroma, flavor and color during roasting process (64). In the searing-cooked steak, the reducing sugar was lower and MRPs were higher than oven-cooked steak, which had higher scores for overall flavor and roasted meat flavor (65). Nevertheless, one of the limitations of these studies is that they only studied the effect of the total reducing sugar content in the system on the flavor produced by the Maillard reaction. It is not explained which reducing sugar plays the key role. Deng et al. (66) found reducing sugars such as glucose and ribose could react with α-amino acids via the Maillard reaction and promote the formation of the cooked meat aroma compound. Furan is a necessary intermediate in some chemical systems and composed of pentose and generated by Amadori intermediate 1, 2-enolization. In the Maillard reaction with xylose, the content of furfural and meat related volatile flavor substances was higher than that with ribose and glucose (29). Furthermore, the addition of reducing sugars could induce protein glycation and facilitate the solubilization of myosin, which in turn might increase the water holding capacity of meat (66). However, only the sensory evaluation of the products after adding different sugars is not enough. According to the current research, it can only be confirmed that the addition of reducing sugar is beneficial to the formation of flavor, and xylose is relatively widely used. These studies would be more relevant if the effects of sugars on the flavor orientation produced by the MR were explored more broadly.
Source of proteins
Proteins of different sources react with sugars and further produce different flavor of MRPs. In recent years, more and more vegetable proteins or peptides have been used in the production of flavor enhancers by MR. Soybean peptides have been used to generate a range of flavors, including umami, sweet, salty, and meaty, as a potential flavor precursor (67). MRPs were made utilizing a xylose and soybean peptide method after heating at 100–140°C and pH 7.6 for 2 h. MRPs with cysteine addition presented the minimal bitterness, after heating at 140°C, and the highest umami and saltiness at 100°C (68). Not only soybeans, but other plant-derived proteins are also reported to change the flavor through MR. In umami soup, MRPs of flaxseed protein hydrolysates could enhance the mouthfulness and continuity (68). And hydrolysates or extracts from meat have also been employed to improve the meat-like flavors of food products. However, the main drawback of these studies is that the resulting Maillard reaction products have no clear directivity (chicken, beef, or fish flavor).
To further enhance the flavor, Kang et al. (69) reported that after addition of xylose and L-cysteine to beef hydrolysate, kokumi, meaty, umami, umami-enhancing and kokumi-enhancing capacity were improved. However, In comparison to meat hydrolysates, meat extracts have poor flavor and aromatic qualities. Enzymatic hydrolysis and MR could turn animal bone extracts into taste components. To solve this problem, ribose was utilized to investigate MRPs generated from beef bone hydrolysates. Enzymatic hydrolysis and heat treatment are reported to promote the production of meat characteristic flavor substance (2-methylpyrazine, dimethyl disulphide, and dimethyl trisulphide) and inhibit the burning taste and formation of bitter substance (2-furanmethanol) (4). Similar results were also observed in swine lard and goat by-product protein hydrolysates, in which aroma compounds were conducive to an increase in the hydrolysis process (70). By markedly increasing the contents of pyrazine and sulfur compounds in the hydrolysate, Flavourzyme could improve the flavor of chicken bone extracts. MR significantly decreased the bitter taste and increased the flavor acceptability of hydrolyzed chicken bone extracts by using Protamex® and Flavourzyme® in a sequential hydrolysis procedure (71). Using chicken as a source of proteins, after thermal treatment with xylose, meat flavor was generated, and the thick, kokumi and freshness of the freshness solution were significantly enhanced (72). Moreover, in the recent study, strong barbecue beef flavor was generated with bone protein hydrolysate (73, 74). To improve the surplus value of fish, heating the low-valued fish hydrolysates with meat hydrolysates, xylose and other additives were optimal for sauce flavor synthesis, in which meaty smell was strengthened and fishy odor was reduced. For example, after xylose reacted with the hydrolysate of shrimp, a product with rich seafood taste and freshness was obtained. After xylose was heated with the polypeptide hydrolysis of shrimp, the resulting MRPs had a rich seafood taste and freshness (75). The hydrolysate of fermented Tilapia fish head could develop a flavor concentrate (31). Overall, a convergence between meat proteins and the flavors of their MRPs was showed, which indicated that we could target the raw materials for MR. However, these studies mainly focused on meat flavor and lacked systematic research on other flavors.
Hydrolysis conditions of raw material
The specific substances with flavor characteristics in MR are affected by specific amino acids, peptides, and proteins. The hydrolyzed products obtained by different peptidases contain different peptide spectral and free amino acids, its MRPs also have different sensory properties. A. Melleus contains a large number of peptides below 1,355 Da (probably corresponding to dipeptides or aromatic amino acids). In the meantime, under the hydrolysis of some proteases such as chymotrypsin or pepsin, the proteins were cleaved specifically to produce peptides that had a c-terminal amino acid (76). Grossmann et al. (77) found that the bitter and umami taste of the cricket and mealworm protein samples had an increasing tendency after enzymatic hydrolyses, which coule be clarified by the liberation of amino acids. However, not all proteases play decisive roles in flavor. Five kinds of bovine bone hydrolysates were genarated by single (P-Protamex®, B-bromelain, F-Flavourzyme®) and simultaneous (P+F and B+F) enzymatic hydrolysis, then were heated at 113°C to form MRPs. The proportion of most volatile compounds in P+F-MRPs and B+F-MRPs differed insignificantly as Flavourzyme® reacted with Protamex® or bromelain simultaneously. Volatile components (such as 2-methylpyrazine, dimethyl disulfide and dimethyl trisulfide) were detected in B-MRPs after heating, and did not appear in P/F-MRPs. They contributed to baking, sulfur, and meat flavor, but the difference was not significant. Based on it, comparing the single hydrolysis of F-MRPs with the simultaneous hydrolysis of P+F-MRPs and B+F-MRPs, there was no remarkable difference in the main volatile compounds of the three MRPs. This suggested that only addition of Flavournzyme® was effective in producing major aroma compounds during MR (4). Various studies have fully demonstrated that protease specific cleavage is one of the means to improve the sensory quality of MRPs. However, the mechanism of flavor enhancement by enzymatic hydrolysis was not considered. It would be more meaningful to further study the characteristic precursor peptides that generate flavor substances in MR. Other enzymes besides proteases also contribute to the formation of flavor in MR. For example, meat-like flavors can be created by myrosinase and lipoxygenase in the two-step brom of Brassica proteins (78). Reducing sugars produced by enzymatic hydrolysis of starch play a role in MR, which can alter the production of taste components directly or indirectly (79). The content and reactivity of MR substrate were increased by enzymatic hydrolysis, which provided the prerequisite for the production of flavor. After the selection of raw materials, the type of enzyme can be selected according to the requirements of the reaction and material characteristics. When dealing with substances with high protein content, proteases can be selected for enzymatic digestion to obtain more highly active peptides. As raw materials with high carbohydrate content were utilized, amylase and glycosidase could be used to increase the content of reducing sugar that can participate in MR.
Molecular weight of peptides
Peptides have been considered to be very critical taste active ingredients in foods. Peptides of molecular weight ranging from 1 to 5 kDa have been found to strongly influence the flavor enhancing attributes (umami, continuity, and mouthfulness), and they are called Maillard peptides; which mainly contribute to the kokumi taste (41, 80). The scent of chicken was provided by a peptide fraction of 2–5 kDa in chicken enzymatic hydrolysate. Meanwhile, in MRPs of chicken enzymatic hydrolysate, a peptide fraction < 500 Da contributed to the roasted scent of chicken, a peptide fraction > 1 kDa formed by cross-linking the peptide fraction 500 Da contributed a kokumi flavor, and a peptide fraction > 3 kDa imparted a bitter taste (81). Low molecular weight (LMW) peptides (<1 kDa) generated in meat during chilled conditioning can act as flavor precursors in the MR with a potential contribution to key volatile organic compound (VOC). The majority of nitrogen-containing volatiles, pyrazines and pyridines, dominated the carnosine mixture, while sulfur-containing VOCs dominated the GSH and Cys Gly peptide mixtures. The peptides with LMW (<1 kDa) are mainly the products of degradation of High molecular weight (HMW) peptides, which are considered as the main contributor of the formation of pyrazines and 2-furfurylpyrrole due to the higher reactivity of the amidogen in these compounds. HMW peptides are the products of cross-linking and polymerization of LMW peptides, which may be formed during the MR. However, peptides with a molecular weight of 128–1,000 Da were found to be primarily responsible for the meat-like flavor, as well as influencing other sensory characteristics (68). Various studies have well demonstrated that peptides with molecular weights < 5 kDa are more suitable, and HMW peptides tend to bring bitter and other odors. Thus, specific enzymatic hydrolysis or ultrafiltration can be used to increase the content of LMW in the reaction system, to increase the consumer preference and acceptability of processed foods.
Reaction conditions of MR
Since the open-chain concentrations of sugars and active forms of amino reactants depend on pH, the parameters significantly influenced the reaction rate and formation of MRPs (15). Firstly, high pH provided favorable conditions for the molecular rearrangement of sugars, and could promote the occurrence of nucleophilic addition reaction (82). Secondly, Amadori compounds are prone to form 1, 2-enolization at pH 8, and tend to react with 2, 3-enolization at pH 9.7. Thirdly, increasing initial reaction pH was beneficial to caramelization, but it had little effect in the range of 6.7–8.0 (83). Therefore, pH might alter the route of MR, causing in variations in the types and contents of flavor compounds in products. During the MR, the consumption of amino groups, the degradation of sugars, free amino acids (FAAs), and peptides facilitated the formation of acidic compounds. For example, after the addition of cysteine to the soybean peptide and D-xylose system, terminal pH was decreased, because cysteine accelerated the formation of formic acid and acetic acid (68). For the first time, Lotfy et al. (16) used enzymatically hydrolyzed quinoa protein as a major precursor for producing thermal process flavorings. By increasing pH from 5 to 9, the odor sensory attributes of the generated process flavor changed from caramel to burnt-coffee. This was due to the fact that low pH favored the production of furans, while high pH preferred the formation of pyrazines. However, pH does not have a significant effect on MRPs in all the cases. Li and Liu (34) discovered that the volatile profiles of heat-treated pork hydrolysate might not unaffected by pH. Volatile compounds such as furfural and furans were formed in similar amounts in heated samples with varying pH values.
MR is composed of a series of complex reactions, in which the sensitivity of each reaction and reactant to temperature are different. Therefore, temperature is also known to directly or indirectly influence the reaction degree and product composition. The MR rate increased significantly with the increase of temperature. For every 10°C increase of temperature, the reaction rate increases 3–5 times. At temperature < 110°C, the system of polypeptide and xylose was given priority to cross-linking reaction. At temperature > 110°C, a series of small molecular compounds of degradation were formed in the peptides system. These small molecular compounds accelerate the transition from pyrazine, sulfur-containing compounds and pyrrole to volatile compounds. Furans are sugar-derived compounds that are commonly generated under high thermal treatment (84). The concentration of furans in the soybean peptides-xylose system rose, as the temperature climbed from 80 to 140°C, demonstrating that furans were formed as a result of severe thermal treatment (68). Similarly, as xylose and chicken hydrolytic peptides were heated at 80–100°C for 60–90 min, the formation of umami-flavor and thick-flavor substances was conducive. While pyrazine, furan and pyridine were heated at high temperature (100–140°C) for 30–60 min, the formation of volatile compounds such as with barbecue and meat flavor was conducive (81). On one hand, after high-temperature treatment, ketones and phenols may be converted into intermediates for heterocyclic compounds or volatilized, and esters could be hydrolyzed into acids and alcohols. On the other hand, Protein hydrolysis may produce more amino acids, peptides, and small molecule compounds to accelerate the MR process. Lower heating temperature (80–100°C) with cysteine added was beneficial to forming umami FAAs, while bitter FAAs were likely formed at temperature ranging from 100 to 120°C which was consistent with the recent study for stewed pork with the high-temperature processing methods (63). However, excessively high temperature would not only generate pungent odor substances such as thiazole, but also destroy the enzyme binding sites of polypeptides and generate some cellulose analogs. The analogs are not easy to be decomposed and absorbed by the human body, thus reducing the nutritional values of food. In addition, at temperature > 110°C, some toxic substances (such as acrylamide and AGEs) are formed, which have certain toxic effects on human body.
MF assistive technology
Recently, new emerging technologies, including pulsed electric field, microwave radiation, high-pressure homogenization and high pressure, have been applied to promote the Maillard reaction with less processing time (35). MR could be significantly accelerated, since ultrasound provided more energy during proteins grafting (85). Furthermore, ultrasonic treatment has the potential to alter the secondary and tertiary structures of proteins, reducing glycation time and increasing the functional properties of conjugates (86). Under ultrasound treatment, the reactivity between proteins and sugars was extremely low. The protein-dense quaternary and tertiary structures protect the active amino groups, and make the protein graft difficult. In contrast, this process usually requires higher temperature and longer time (usually several days) without ultrasound treatment. As an alternative strategy, ultrasound is an appropriate choice for promoting protein saccharification. MRPs had a higher content of conjugated amino acids after ultrasonic pretreatment, indicating that ultrasonic pretreatment of soybean protein/sugar mixture before heating accelerated the reaction (87). Ultrasound assisting MR has been a promising approach for improving the functional properties of mung bean protein isolates with glucose (88). It could accelerate the MR rate, and improve the content of sulfur containing volatile flavor compounds and antioxidant properties of the products (89). Many studies have found that ultrasonic pretreatment of proteins before enzymolysis hydrolyzed the protein into smaller fragments, resulting in a considerable increase in reaction rate (90, 91). Comparing energy aggregating ultrasound (EGU) and energy dispersing ultrasound (EDU) pretreatments, the taste and overall acceptability of MRPs were improved. Due to the low intensity and uniformity of the ultrasonic waves generated by the EDU, the protein molecule distribution was more uniform, which increased the irregular curvature and surface hydrophobicity of the protein. It also resulted in the increase of the content of peptides and amino acids in the hydrolyzed protein as well as the ratio of small molecule peptides to amino acids, thus promoting the MR between hydrolyzed protein and glucose (11).
Traditional production methods of black garlic could not meet the industrial demand because of long-time, high production cost, high-energy consumption, and uneven product quality. The most important chemical reaction in the production of black garlic is MR, and the major substrates are reducing sugars and amino acids (92). The reducing sugars produced by the degradation of polysaccharides and enzymes are important parts of MR, but the enzymes and substrates are usually isolated at different locations in intact cells. The destruction of cellular structures by high-pressure pretreatment affected the intracellular environment, and especially accelerated the degradation of fructan. MR substrates accumulated rapidly in the early stage of processing, which led to the accelerated arrival of the reducing sugar equilibrium point (RSBP) during the heat treatment. High pressure reduced the production time of black garlic from 24 to 15 d, and the taste was also improved (93).
A new non-thermal food processing method is pulse electric field (PEF). Electric field strength, polarity, pulse time, pulse count, and pulse shape are the basic PEF process parameters. PEF processing has so far been widely employed in the extraction of physiologically active compounds, alteration of biomacromolecules, augmentation of chemical processes, and other aspects (94). PEF processing has the ability to change the microstructure and macromolecular interactions of biomacromolecules while reducing the overall processing time with great efficiency when compared to conventional heating techniques. PEF is particularly vital for improving interactions between proteins and polysaccharides (95). Researchers claim that PEF processing might boost the carbon backbone's internal energy fluctuation, enhance mass transfer, and lower the chemical reaction's activation energy (94). The molecular chains of proteins would be attacked by the free radicals produced by electrolysis or electrochemical reactions when the protein-polysaccharide mixed solution is subjected to the PEF processing, leading to the partial-unfolding of proteins' secondary structure and improved protein-water interactions. Additionally, several previously hidden cationic, surface-free sulfhydryl, and hydrophobic groups on proteins are revealed (95). The delivered electric energy simultaneously induces the depolymerization and breakdown of carbohydrate chains. These modifications facilitate the binding of various reactants and increase the likelihood of collisions between reactive molecules. When the free -NH2 of proteins links with the -C = O of polysaccharides, covalent interactions take place. By-products of Rainbow Trout (Oncorhynchus mykiss), Dover Sole (Dover Sole) as well as other animal protein (96) and non-dairy plant-based Beverages (97) have been demonstrated to taste better and be more widely accepted when subjected to pulsed electric fields. However, there is presently no large-scale PEF technology available to generate MRPs for the food business. To fully comprehend the interaction between the critical intensity and the thermal consequences of PEF processing, more study is required.
Negative effects
It is widely known that the average human diet contains large amounts of MRPs. A certain degree of browning plays an important role in developing the sensory properties (such as color, aroma, and flavor) which are needed in certain foods. For example, many baked goods, such as cookies and bread, have desired brown color, giving the consumer an impression of high quality. However, excessive MR might also undermine the sensory, flavor and nutritional values of foods, and even produce anti-nutritional or toxic substances such as acrylamide, heterocyclic amine, and advanced glycation final.
MR might affect the color of agricultural products, causing economic losses. The color deterioration of shrimp was noticed due to the multiple effects of lipid oxidation, phenol oxidation, MR, and astaxanthin degradation (98). Pyrazine and 5-hydroxymethyl furfural (5-HMF) were increased in a time-dependent way. Similarly, the internal browning depreciated the economic value of sweet potatoes due to the generation levels of reactive oxygen species, active polyphenols in the browning area were higher than those in the normal area. Intracellular glucose accumulation initiated the MR and led to ROS aggregation (99).
Moreover, MR played a key role in browning during the instant controlled pressure drop-assisted hot air drying of the apple slices which reduced the sensory quality of the slices (100). As one of the carcinogenic MRPs, acrylamide should be prevented from generation in food systems containing reducing sugars and asparagine. To control the acryl amide generation, ultrasound-MR could be used to accelerate MR in the asparagine-glucose model system (100).
The stability of flavor and flavorings is receiving more attention these days. Flavor loss and deterioration are significant restrictions during food processing and storage, particularly at temperatures above room temperature. Because of their instability (such as pyranone and unsaturated diones), MRPs are extremely prone to flavor loss during storage and processing (101). The flavor quality and customer acceptance of food were both lowered as a result of the loss of favorable fragrance components (such as acetaldehyde, butanal, and furfural). The contents of taste components in MRPs were drastically reduced after storage, and the solution's clarity deteriorated. The stability and transparency of Maillard reaction intermediates as a spice precursor also make them a potential substitute for MRPs (102).
In addition to the food system, MR also occurs in the human body, and could pose a health risk. It is known that the superoxides product might oxidize biological macromolecules (such as lipids and proteins) in each stage of MR in the medical field. Diabetes is related to advanced glycation end products (AGEs), as a result of the fact that MR is triggered by an increase in blood sugar level (103). Furthermore, AGEs accumulation in follicles by activating ATF4 in the follicular microenvironment, which triggers inflammation and reduces oocyte competence (104). In a concentration-dependent manner, AGEs significantly accelerated BMSC senescence, induced mitochondrial dysfunction, and blocked mitophagy (43). Therefore, it is necessary to imply intervention measures to control MR under these situations.
Application of MRPs as flavor enhancers
Table 4 showed the latest studies on the role of the Maillard reaction in the production of flavor. By studying the taste characteristics of 1–5 kDa peptides MRPs, the Maillard peptide could enhance the umami and continuity of umami solution and consommé soup (43). Heo et al. (111) investigated on the molecular and sensory properties of y-glutamyl peptides, which are key factors to the Kokumi taste of edible beans (Phaseolus vulgaris L.). As added to a savory matrix like sodium chloride and monosodium glutamate solutions or chicken broth, the y-glutamyl peptides greatly improved mouthfullness, complexity, and shelf life. Similarly, the Maillard-peptide and the taste enhancer from long-term ripening of miso (soybean paste) were deemed essential substances, suggesting the characteristic flavor (mouthfulness and continuity) of long-ripened miso. Purified di-, tri-, tetra-, and other short peptides have also been extensively studied in MR systems to provide various flavor and taste characteristics. According to previous study, the cross-linking of peptides in the thermal reaction process played an important role in the aroma and mellow feeling. MR study of rapeseed peptides, cysteine and Xylose showed that high temperature reduced the bitter value of the product, and low temperature promoted the salty and umami-tasting (85). Kang et al. (69) reported that interaction between enzymatic hydrolysis and MR improved the quality of beef flavor by regulating the formation of characteristic aroma precursors. In addition, the possible pathway and mechanism of the characteristic flavor of natural meat were further clarified, forming a new technology for the preparation of high-fidelity MF.
In the recent years, the promising industrial avenues for MFs have a good potential in China due to the rich flavor, however, there is still scope to develop their application. In view of this characteristic, strategies can be applied to obtain MFs directly by chemical synthesis after analyzing the structural characteristics of key flavor compounds. Zhai et al. (112) synthesized 2, 5-dimethylpyrazine, 2,3, 5-trimethylpyrazine and benzyl alcohol by palladium-catalyzed synthesis of 2-enylpyrazine oxides. Kocadagli et al. (32) also prepared 2-acetyl-1-pyrroline, 6-acetyl-1,2,3,4-tetrahydropyridine and 5-acetyl-2,3-dihydro4H-1,4-thiazide to enhance the flavor characteristics of baked foods. However, resulting MRPs were often unstable and highly volatile, especially at higher temperatures (101). In addition, flavor substances with active properties are often derived into other substances through peroxidation, polymerization and condensation, thus changing the overall flavor profile. Among the MRPs, pyrazine is regarded as the most vulnerable key aroma substances. Followed by pyrrole, mercaptans and aldehydes, oxidation and polymerization are the most important factors for the loss. Therefore, during the application and storage of MFs, it is difficult to maintain a stable concentration and flavor enhancing effect, especially in the thermal processing. It has become an important research direction for food scientists to analyze the mechanism of flavor generation and loss, and improve the controllability of flavor substances escape. In addition to high stability, MRIs can also reduce the bitterness of raw materials and increase the umami taste. It also improves the body's perception of salt, which helps reduce salt intake in daily life. But the mechanism of saltiness enhancement of vegetable protein enzymatic hydrolysates warrants future studies (10).
Conclusion
The MRPs not only alter food properties (stability, flavor, color, etc.), but also enhance the functional properties (anti-oxidant, anti-microbial, and anti-browning) of amino acids, peptides and proteins. MRPs have been used as flavor enhancers because of good mouthfeel and umami. Their flavor characteristics could be pleasant floral, nutty, caramel, attractive meat, or even spicy. E-nose, E-tongue, and GC-MS have become the most representative tools to detect flavor components, helping researchers to obtain comprehensive flavor profile. MF depends largely on the proteins, reducing sugars, and conditions (time, temperature, pH, vacuum degree, etc.). After proteins and sugars obtained from different sources react, MRPs of different flavor are obtained. Meanwhile, under different enzymatic hydrolysis conditions, proteins are enzymolyzed into different peptide fragments. In MR, since the open chain concentration of sugars and active form of amino reactants depend on pH, each reaction and reactant has a different sensitivity to temperature and affects the reaction differently.
Except flavor, MR currently has many limitations in terms of the difficulty in controlling the reaction degree, and the instability of the final product. Undesirable substances, which could reduce the flavor and nutrition, might be formed during the reaction. To address these issues, MRI has become a new research focus and has contributed to improving stability and flavor, nevertheless, the generation and mechanism of MRPs still need to be further exploited.
Author contributions
SL, GM, and LW designed the topic. LG and SL prepared the manuscript. HP, XL, and TZ prepared the figures. SL, GM, TZ, and HS reviewed and revised the manuscript. All authors contributed to the article and approved the submitted version.
Funding
This work was funded by Technology Project of Anhui Province 201903a06020024, Technology Project of Anhui Province 202203a06020029, Eight Major Industrial Chain Strengthening and Chain Reinforcing Projects 2021GJ010, Science and Technology Plan Project of Huangshan City 2020KN-04, and Key Research and Development Projects from Anhui Province 202104a06020013.
Conflict of interest
The authors declare that the research was conducted in the absence of any commercial or financial relationships that could be construed as a potential conflict of interest.
Publisher's note
All claims expressed in this article are solely those of the authors and do not necessarily represent those of their affiliated organizations, or those of the publisher, the editors and the reviewers. Any product that may be evaluated in this article, or claim that may be made by its manufacturer, is not guaranteed or endorsed by the publisher.
References
1. Fu Y, Zhang YH, Soladoye OP, Aluko RE. Maillard reaction products derived from food protein-derived peptides: insights into flavor and bioactivity. Crit Rev Food Sci Nutr. (2020) 60:3429–42. doi: 10.1080/10408398.2019.1691500
2. Nooshkam M, Varidi M, Verma DK. Functional and biological properties of Maillard conjugates and their potential application in medical and food: a review. Food Res Int. (2020) 131:109003. doi: 10.1016/j.foodres.2020.109003
3. Xu Z, Huang G, Xu T, Liu L, Xiao J. Comparative study on the Maillard reaction of chitosan oligosaccharide and glucose with soybean protein isolate. Int J Biol Macromol. (2019) 131:601–7. doi: 10.1016/j.ijbiomac.2019.03.101
4. Chiang JH, Eyres GT, Silcock PJ, Hardacre AK, Parker ME. Changes in the physicochemical properties and flavour compounds of beef bone hydrolysates after Maillard reaction. Food Res Int. (2019) 123:642–9. doi: 10.1016/j.foodres.2019.05.024
5. Song Y, Du B, Ding Z, Yu Y, Wang Y. Baked red pepper (Capsicum annuum L.) powder flavor analysis and evaluation under different exogenous Maillard reaction treatment. LWT. (2020) 139:110525. doi: 10.1016/j.lwt.2020.110525
6. Habinshuti I, Chen X, Yu J, Mukeshimana O, Duhoranimana E, Karangwa E, et al. Antimicrobial, antioxidant and sensory properties of Maillard reaction products (MRPs) derived from sunflower, soybean and corn meal hydrolysates. LWT. (2019) 101:694–702. doi: 10.1016/j.lwt.2018.11.083
7. Navicha W, Hua Y, Masamba KG, Kong X, Zhang C. Effect of soybean roasting on soymilk sensory properties. Br Food J. (2018) 120:2832–42. doi: 10.1108/BFJ-11-2017-0646
8. Chen F, Lin L, Zhao M, Zhu Q. Modification of Cucumaria frondosa hydrolysate through maillard reaction for sea cucumber peptide based-beverage. LWT. (2021) 136:110329. doi: 10.1016/j.lwt.2020.110329
9. Wang W, Zhang L, Wang Z, Wang X, Liu Y. Physicochemical and sensory variables of Maillard reaction products obtained from Takifugu obscurus muscle hydrolysates. Food Chem. (2019) 290:40–6. doi: 10.1016/j.foodchem.2019.03.065
10. Zhou X, Cui H, Zhang Q, Hayat K, Yu J, Hussain S, et al. Taste improvement of Maillard reaction intermediates derived from enzymatic hydrolysates of pea protein. Food Res Int. (2021) 140:109985. doi: 10.1016/j.foodres.2020.109985
11. Habinshuti I, Mu T-H, Zhang M. Structural, antioxidant, aroma, and sensory characteristics of Maillard reaction products from sweet potato protein hydrolysates as influenced by different ultrasound-assisted enzymatic treatments. Food Chem. (2021) 361:130090. doi: 10.1016/j.foodchem.2021.130090
12. Sun L-b, Zhang Z-y, Xin G, Sun B-x, Bao X-j, Wei Y-y, et al. Advances in umami taste and aroma of edible mushrooms. Trends Food Sci Technol. (2020) 96:176–87. doi: 10.1016/j.tifs.2019.12.018
13. He S, Zhang Z, Sun H, Zhu Y, Zhao J, Tang M, et al. Contributions of temperature and l-cysteine on the physicochemical properties and sensory characteristics of rapeseed flavor enhancer obtained from the rapeseed peptide and d-xylose Maillard reaction system. Ind Crops Prod. (2019) 128:455–63. doi: 10.1016/j.indcrop.2018.11.048
14. Liu Z, Zhu Y, Wang W, Zhou X, Chen G, Liu Y. Seven novel umami peptides from Takifugu rubripes and their taste characteristics. Food Chem. (2020) 330:127204. doi: 10.1016/j.foodchem.2020.127204
15. Geng J-T, Takahashi K, Kaido T, Kasukawa M, Okazaki E. Relationship among pH, generation of free amino acids, and Maillard browning of dried Japanese common squid Todarodes pacificus meat. Food Chem. (2019) 283:324–30. doi: 10.1016/j.foodchem.2019.01.056
16. Lotfy SN, Saad R, El-Massrey KF, Fadel HHM. Effects of pH on headspace volatiles and properties of Maillard reaction products derived from enzymatically hydrolyzed quinoa protein-xylose model system. LWT. (2021) 145:111328. doi: 10.1016/j.lwt.2021.111328
17. Spotti MJ, Loyeau PA, Marangón A, Noir H, Rubiolo AC, Carrara CR. Influence of Maillard reaction extent on acid induced gels of whey proteins and dextrans. Food Hydrocoll. (2019) 91:224–31. doi: 10.1016/j.foodhyd.2019.01.020
18. Singh P, Rao PS, Sharma V, Arora S. Physico-chemical aspects of lactose hydrolysed milk system along with detection and mitigation of maillard reaction products. Trends Food Sci Technol. (2021) 107:57–67. doi: 10.1016/j.tifs.2020.11.030
19. Zhou Z, Langrish T. A review of Maillard reactions in spray dryers. J Food Eng. (2021) 305:110615. doi: 10.1016/j.jfoodeng.2021.110615
20. Hafsa J, Smach MA, Mrid RB, Sobeh M, Majdoub H, Yasri A. Functional properties of chitosan derivatives obtained through Maillard reaction: A novel promising food preservative. Food Chem. (2021) 349:129072. doi: 10.1016/j.foodchem.2021.129072
21. Ahmed T, Wang CK. Black garlic and its bioactive compounds on human health diseases: a review. Molecules. (2021) 26:5028. doi: 10.3390/molecules26165028
22. Hou L, Xie J, Zhao J, Zhao M, Fan M, Xiao Q, et al. Roles of different initial Maillard intermediates and pathways in meat flavor formation for cysteine-xylose-glycine model reaction systems. Food Chem. (2017) 232:135–44. doi: 10.1016/j.foodchem.2017.03.133
23. Lomelí-Martín A, Martínez LM, Welti-Chanes J, Escobedo-Avellaneda Z. Induced changes in aroma compounds of foods treated with high hydrostatic pressure: a review. Foods. (2021) 10:878. doi: 10.3390/foods10040878
24. Deuscher Z, Gourrat K, Repoux M, Boulanger R, Labouré H, Le Quéré JL. Key aroma compounds of dark chocolates differing in organoleptic properties: a GC-O comparative study. Molecules. (2020) 25:1809. doi: 10.3390/molecules25081809
25. Bertrand E, Boustany PE, Faulds CB, Berdagué J. The Maillard reaction in food: an introduction. In: Reference Module in Food Science (2018).
26. Rufián-Henares JA, Pastoriza S. Browning: non-enzymatic browning. In: Encyclopedia of Food and Health, eds B. Caballero, P. M. Finglas, and F. Toldrá. Oxford: Academic Press (2016). p. 515–21.
27. Shen Y, Hu L-T, Xia B, Ni Z-J, Elam E, Thakur K, et al. Effects of different sulfur-containing substances on the structural and flavor properties of defatted sesame seed meal derived Maillard reaction products. Food Chem. (2021) 365:130463. doi: 10.1016/j.foodchem.2021.130463
28. Xiao Q, Woo MW, Hu J, Xiong H, Zhao Q. The role of heating time on the characteristics, functional properties and antioxidant activity of enzyme-hydrolyzed rice proteins-glucose Maillard reaction products. Food Biosci. (2021) 43:101225. doi: 10.1016/j.fbio.2021.101225
29. Ni Z-J, Liu X, Xia B, Hu L-T, Thakur K, Wei Z-J. Effects of sugars on the flavor and antioxidant properties of the Maillard reaction products of camellia seed meals. Food Chem. (2021) 11:100127. doi: 10.1016/j.fochx.2021.100127
30. Du X, Sissons J, Shanks M, Plotto A. Aroma and flavor profile of raw and roasted agaricus bisporus mushrooms using a panel trained with aroma chemicals. LWT. (2021) 138:110596. doi: 10.1016/j.lwt.2020.110596
31. Gao P, Xia W, Li X, Liu S. Optimization of the Maillard reaction of xylose with cysteine for modulating aroma compound formation in fermented tilapia fish head hydrolysate using response surface methodology. Food Chem. (2020) 331:127353. doi: 10.1016/j.foodchem.2020.127353
32. Kocadagli T, Methven L, Kant A, Parker JK. Targeted precursor addition to increase baked flavour in a low-acrylamide potato-based matrix. Food Chem. (2021) 339:128024. doi: 10.1016/j.foodchem.2020.128024
33. Heo J, Adhikari K, Choi KS, Lee J. Analysis of caffeine, chlorogenic acid, trigonelline, and volatile compounds in cold brew coffee using high-performance liquid chromatography and solid-phase microextraction-gas chromatography-mass spectrometry. Foods. (2020) 9:1746. doi: 10.3390/foods9121746
34. Li X, Liu S-Q. Effect of pH, xylose content and heating temperature on colour and flavour compound formation of enzymatically hydrolysed pork trimmings. LWT. (2021) 150:112017. doi: 10.1016/j.lwt.2021.112017
35. Li Z, Zheng Y, Sun Q, Wang J, Zheng B, Guo Z. Structural characteristics and emulsifying properties of myofibrillar protein-dextran conjugates induced by ultrasound Maillard reaction. Ultrason Sonochem. (2021) 72:105458. doi: 10.1016/j.ultsonch.2020.105458
36. Fan M, Xiao Q, Xie J, Cheng J, Sun B, Du W, et al. Aroma compounds in chicken broths of Beijing Youji and commercial broilers. J Agric Food Chem. (2018) 66:10242–51. doi: 10.1021/acs.jafc.8b03297
37. Xiong C, Qiang L, Li S, Cheng C, Chen Z, Huang W. In vitro antimicrobial activities and mechanism of 1-Octen-3-ol against food-related bacteria and pathogenic fungi. J Oleo Sci. (2017) 66:1041–9. doi: 10.5650/jos.ess16196
38. Zannou O, Kelebek H, Selli S. Elucidation of key odorants in Beninese Roselle (Hibiscus sabdariffa L.) infusions prepared by hot and cold brewing. Food Res. Int. (2020) 133:109133. doi: 10.1016/j.foodres.2020.109133
39. Hidalgo FJ, Lavado-Tena CM, Zamora R. Formation of 3-hydroxypyridines by lipid oxidation products in the presence of ammonia and ammonia-producing compounds. Food Chem. (2020) 328:127100. doi: 10.1016/j.foodchem.2020.127100
40. Zhao J, Wang T, Xie J, Xiao Q, Du W, Wang Y, et al. Meat flavor generation from different composition patterns of initial Maillard stage intermediates formed in heated cysteine-xylose-glycine reaction systems. Food Chem. (2019) 274:79–88. doi: 10.1016/j.foodchem.2018.08.096
41. Zhan H, Hayat K, Cui H, Hussain S, Ho C-T, Zhang X. Characterization of flavor active non-volatile compounds in chicken broth and correlated contributing constituent compounds in muscle through sensory evaluation and partial least square regression analysis. LWT. (2020) 118:108786. doi: 10.1016/j.lwt.2019.108786
42. Zhang N, Yang Y, Wang W, Fan Y, Liu Y. A potential flavor seasoning from aquaculture by-products: an example of takifugu obscurus. LWT. (2021) 151:112160. doi: 10.1016/j.lwt.2021.112160
43. Guo Y, Jia X, Cui Y, Song Y, Wang S, Geng Y, et al. Sirt3-mediated mitophagy regulates AGEs-induced BMSCs senescence and senile osteoporosis. Redox Biol. (2021) 41:101915. doi: 10.1016/j.redox.2021.101915
44. Yang X, Li Y, Li S, Ren X, Oladejo AO, Lu F, et al. Effects and mechanism of ultrasound pretreatment of protein on the Maillard reaction of protein-hydrolysate from grass carp (Ctenopharyngodon idella). Ultrason Sonochem. (2020) 64:104964. doi: 10.1016/j.ultsonch.2020.104964
45. He S, Chen Y, Brennan C, Young DJ, Chang K, Wadewitz P, et al. Antioxidative activity of oyster protein hydrolysates Maillard reaction products. Food Sci Nutr. (2020) 8:3274–86. doi: 10.1002/fsn3.1605
46. Andruszkiewicz PJ, D'Souza RN, Corno M, Kuhnert N. Novel Amadori and Heyns compounds derived from short peptides found in dried cocoa beans. Food Res Int. (2020) 133:109164. doi: 10.1016/j.foodres.2020.109164
47. Liang S, Zhang T, Fu X, Zhu C, Mou H. Partially degraded chitosan-based flocculation to achieve effective deodorization of oyster (Crassostrea gigas) hydrolysates. Carbohydr Polym. (2020) 234:115948. doi: 10.1016/j.carbpol.2020.115948
48. Wang Z, Zheng C, Huang F, Liu C, Huang Y, Wang W. Effects of radio frequency pretreatment on quality of tree peony seed oils: process optimization and comparison with microwave and roasting. Foods. (2021) 10:3062. doi: 10.3390/foods10123062
49. Zhang Z, Elfalleh W, He S, Tang M, Zhao J, Wu Z, et al. Heating and cysteine effect on physicochemical and flavor properties of soybean peptide Maillard reaction products. Int J Biol Macromol. (2018) 120:2137–46. doi: 10.1016/j.ijbiomac.2018.09.082
50. Nakagawa K, Maeda H, Yamaya Y, Tonosaki Y. Maillard reaction intermediates and related phytochemicals in black garlic determined by EPR and HPLC analyses. Molecules. (2020). 25:4578. doi: 10.3390/molecules25194578
51. Luo Y, Li S, Ho CT. Key aspects of amadori rearrangement products as future food additives. Molecules. (2021) 26:4314. doi: 10.3390/molecules26144314
52. Prud'homme SM, Hani YMI, Cox N, Lippens G, Nuzillard JM, Geffard A. The zebra mussel (Dreissena polymorpha.) as a model organism for ecotoxicological studies: a prior 1H NMR spectrum interpretation of a whole body extract for metabolism monitoring. Metabolites. (2020) 10:256. doi: 10.3390/metabo10060256
53. Neagu AN, Jayathirtha M, Baxter E, Donnelly M, Petre BA, Darie CC. Applications of tandem mass spectrometry (MS/MS) in protein analysis for biomedical research. Molecules. (2022) 27:2411. doi: 10.3390/molecules27082411
54. Saldaña E, Saldarriaga L, Cabrera J, Siche R, Behrens JH, Selani MM, et al. Relationship between volatile compounds and consumer-based sensory characteristics of bacon smoked with different Brazilian woods. Food Res Int. (2019) 119:839–49. doi: 10.1016/j.foodres.2018.10.067
55. Petričević S, Marušić Radovčić N, Lukić K, Listeš E, Medić H. Differentiation of dry-cured hams from different processing methods by means of volatile compounds, physico-chemical and sensory analysis. Meat Sci. (2018) 137:217–27. doi: 10.1016/j.meatsci.2017.12.001
56. Routray W, Rayaguru K. 2-Acetyl-1-pyrroline: a key aroma component of aromatic rice and other food products. Food Rev Int. (2018) 34:539–65. doi: 10.1080/87559129.2017.1347672
57. Cambeiro-Perez N, Gonzalez-Gomez X, Gonzalez-Barreiro C, Perez-Gregorio MR, Fernandes I, Mateus N, et al. Metabolomics insights of the immunomodulatory activities of phlorizin and phloretin on human THP-1 macrophages. Molecules. (2021) 26:787. doi: 10.3390/molecules26040787
58. Ahluwalia K, Ebright B, Chow K, Dave P, Mead A, Poblete R, et al. Lipidomics in understanding pathophysiology and pharmacologic effects in inflammatory diseases: considerations for drug development. Metabolites. (2022) 12:333. doi: 10.3390/metabo12040333
59. Xing H, Mossine VV, Yaylayan V. Diagnostic MS/MS fragmentation patterns for the discrimination between Schiff bases and their Amadori or Heyns rearrangement products. Carbohydr Res. (2020) 491:107985. doi: 10.1016/j.carres.2020.107985
60. Feskens E, Brennan L, Dussort P, Flourakis M, Lindner LME, Mela D, et al. Potential markers of dietary glycemic exposures for sustained dietary interventions in populations without diabetes. Adv Nutr. (2020) 11:1221–36. doi: 10.1093/advances/nmaa058
61. Hwang IS, Chon SY, Bang WS, Kim MK. Influence of roasting temperatures on the antioxidant properties, β-glucan content, and volatile flavor profiles of shiitake mushroom. Foods. (2020) 10:54. doi: 10.3390/foods10010054
62. Xie Q, Xu B, Xu Y, Yao Z, Zhu B, Li X, et al. Effects of different thermal treatment temperatures on volatile flavour compounds of water-boiled salted duck after packaging. LWT. (2022) 154:112625. doi: 10.1016/j.lwt.2021.112625
63. Han D, Zhang C-H, Fauconnier M-L, Jia W, Wang J-F, Hu F-F, et al. Characterization and comparison of flavor compounds in stewed pork with different processing methods. LWT. (2021) 144:111229. doi: 10.1016/j.lwt.2021.111229
64. Elhalis H, Cox J, Frank D, Zhao J. Microbiological and chemical characteristics of wet coffee fermentation inoculated with hansinaspora uvarum and Pichia kudriavzevii and their impact on coffee sensory quality. Front Microbiol. (2021) 12:713969. doi: 10.3389/fmicb.2021.713969
65. Yoo JH, Kim JW, Yong HI, Baek KH, Lee HJ, Jo C. Effects of searing cooking on sensory and physicochemical properties of beef steak. Food Sci Anim Resources. (2020) 40:44–54. doi: 10.5851/kosfa.2019.e80
66. Deng S, Xing T, Li C, Xu X, Zhou G. The effect of breed and age on the growth performance, carcass traits and metabolic profile in breast muscle of Chinese indigenous chickens. Foods. (2022) 11, 483. doi: 10.3390/foods11030483
67. Tu Y, Xu Y, Ren F, Zhang H. Characteristics and antioxidant activity of Maillard reaction products from α-lactalbumin and 2′-fucosyllactose. Food Chem. (2020) 316:126341. doi: 10.1016/j.foodchem.2020.126341
68. Wei CK, Thakur K, Liu DH, Zhang JG, Wei ZJ. Enzymatic hydrolysis of flaxseed (Linum usitatissimum L.) protein and sensory characterization of Maillard reaction products. Food Chem. (2018) 263:186–93. doi: 10.1016/j.foodchem.2018.04.120
69. Kang L, Alim A, Song H. Identification and characterization of flavor precursor peptide from beef enzymatic hydrolysate by Maillard reaction. J Chromatogr B. (2019) 1104:176–81. doi: 10.1016/j.jchromb.2018.10.025
70. de Araújo Cordeiro ARR, de Medeiros LL, Bezerra TKA, Pacheco MTB, de Sousa Galvão M, Madruga MS. Effects of thermal processing on the flavor molecules of goat by-product hydrolysates. Food Res Int. (2020) 138:109758. doi: 10.1016/j.foodres.2020.109758
71. Sun HM, Wang JZ, Zhang CH, Li X, Xu X, Dong XB, et al. Changes of flavor compounds of hydrolyzed chicken bone extracts during Maillard reaction. Food Sci. (2015) 79:C2415–26. doi: 10.1111/1750-3841.12689
72. Zhang L, Chen Q, Liu Q, Xia X, Wang Y, Kong B. Effect of different types of smoking materials on the flavor, heterocyclic aromatic amines, and sensory property of smoked chicken drumsticks. Food Chem. (2022) 367:130680. doi: 10.1016/j.foodchem.2021.130680
73. Shen DY, Li M-k, Song H-L, Zou T-t, Zhang L, Xiong J. Characterization of aroma in response surface optimized no-salt bovine bone protein extract by switchable GC/GC × GC-olfactometry-mass spectrometry, electronic nose, and sensory evaluation. LWT. (2021) 147:111559. doi: 10.1016/j.lwt.2021.111559
74. Xu X, Yu M, Raza J, Song H, Gong L, Pan W. Study of the mechanism of flavor compounds formed via taste-active peptides in bovine bone protein extract. LWT. (2021) 137:110371. doi: 10.1016/j.lwt.2020.110371
75. Cai L, Li D, Dong Z, Cao A, Lin H, Li J. Change regularity of the characteristics of Maillard reaction products derived from xylose and Chinese shrimp waste hydrolysates. LWT Food Sci Technol. (2016) 65:908–16. doi: 10.1016/j.lwt.2015.09.007
76. Hou X, Yuan Z, Wang X, Cheng R, Zhou X, Qiu J. Peptidome analysis of cerebrospinal fluid in neonates with hypoxic-ischemic brain damage. Mol Brain. (2020) 13:133. doi: 10.1186/s13041-020-00671-9
77. Grossmann KK, Merz M, Appel D, De Araujo MM, Fischer L. New insights into the flavoring potential of cricket (Acheta domesticus) and mealworm (Tenebrio molitor) protein hydrolysates and their Maillard products. Food Chem. (2021) 364:130336. doi: 10.1016/j.foodchem.2021.130336
78. Marcinkowska M, Jeleń HH. Inactivation of thioglucosidase from Sinapis alba (white mustard) seed by metal salts. Molecules. (2020) 25:4363. doi: 10.3390/molecules25194363
79. Ma R, Jin Z, Wang F, Tian Y. Contribution of starch to the flavor of rice-based instant foods. Crit Rev Food Sci Nutr. (2021) 1–12. doi: 10.1080/10408398.2021.1931021
80. Rhyu M-R, Lyall V. Interaction of taste-active nutrients with taste receptors. Curr Opin Physiol. (2021) 20:64–9. doi: 10.1016/j.cophys.2020.12.008
81. Liu J, Liu M, He C, Song H, Chen F. Effect of thermal treatment on the flavor generation from Maillard reaction of xylose and chicken peptide. LWT Food Sci Technol. (2015) 64:316–25. doi: 10.1016/j.lwt.2015.05.061
82. Hrynets Y, Bhattacherjee A, Betti M. Nonenzymatic Browning Reactions: Overview. Amsterdam: Academic Press (2019). p. 233–44.
83. Yu M, He S, Tang M, Zhang Z, Zhu Y, Sun H. Antioxidant activity and sensory characteristics of Maillard reaction products derived from different peptide fractions of soybean meal hydrolysate. Food Chem. (2018) 243:249–57. doi: 10.1016/j.foodchem.2017.09.139
84. Zhang H, Chen H, Wang W, Jiao W, Chen W, Zhong Q, et al. Characterization of volatile profiles and marker substances by HS-SPME/GC-MS during the concentration of coconut jam. Foods. (2020) 9:347. doi: 10.3390/foods9030347
85. Chen WJ, Ma XB, Wang WJ, Lv RL, Guo MM, Ding T, et al. Preparation of modified whey protein isolate with gum acacia by ultrasound maillard reaction. Food Hydrocoll. (2019) 95:298–307. doi: 10.1016/j.foodhyd.2018.10.030
86. Chen L, Chen J, Wu K, Yu L. Improved low pH emulsification properties of glycated peanut protein isolate by ultrasound Maillard reaction. J Agric Food Chem. (2016) 64:5531–8. doi: 10.1021/acs.jafc.6b00989
87. Yu H, Zhong Q, Liu Y, Guo Y, Xie Y, Zhou W, et al. Recent advances of ultrasound-assisted Maillard reaction. Ultrason Sonochem. (2020) 64:104844. doi: 10.1016/j.ultsonch.2019.104844
88. Abdelhedi O, Mora L, Jemil I, Jridi M, Toldra F, Nasri M, et al. Effect of ultrasound pretreatment and Maillard reaction on structure and antioxidant properties of ultrafiltrated smooth-hound viscera proteins-sucrose conjugates. Food Chem. (2017) 230:507–15. doi: 10.1016/j.foodchem.2017.03.053
89. Gouda M, El-Din Bekhit A, Tang Y, Huang Y, Huang L, He Y, et al. Recent innovations of ultrasound green technology in herbal phytochemistry: a review. Ultrason Sonochem. (2021) 73:105538. doi: 10.1016/j.ultsonch.2021.105538
90. Jin J, Okagu OD, Yagoub AEA, Udenigwe CC. Effects of sonication on the in vitro digestibility and structural properties of buckwheat protein isolates. Ultrason Sonochem. (2021) 70:105348. doi: 10.1016/j.ultsonch.2020.105348
91. Lan M, Li W, Chang C, Liu L, Li P, Pan X, et al. Enhancement on enzymolysis of pigskin with ultrasonic assistance. Bioengineered. (2020) 11:397–407. doi: 10.1080/21655979.2020.1736736
92. Ríos-Ríos KL, Montilla A, Olano A, Villamiel M. Physicochemical changes and sensorial properties during black garlic elaboration: a review. Trends Food Sci Technol. (2019) 88:459–67. doi: 10.1016/j.tifs.2019.04.016
93. Li F, Cao J, Liu Q, Hu X, Liao X, Zhang Y. Acceleration of the Maillard reaction and achievement of product quality by high pressure pretreatment during black garlic processing. Food Chem. (2020) 318:126517. doi: 10.1016/j.foodchem.2020.126517
94. Niu D, Zeng XA, Ren EF, Xu FY, Li J, Wang MS, et al. Review of the application of pulsed electric fields (PEF) technology for food processing in China. Food Res Int. (2020) 137:109715. doi: 10.1016/j.foodres.2020.109715
95. Gieteru SG, Ali MA, Oey I. Optimisation of pulsed electric fields processing parameters for developing biodegradable films using zein, chitosan and poly(vinyl alcohol). Innov Food Sci Emerg Technol. (2020) 60:102287. doi: 10.1016/j.ifset.2020.102287
96. Wang M, Zhou J, Collado MC, Barba FJ. Accelerated solvent extraction and pulsed electric fields for valorization of rainbow trout (Oncorhynchus mykiss) and sole (Dover sole) by-products: protein content, molecular weight distribution and antioxidant potential of the extracts. Marine Drugs. (2021) 19:207. doi: 10.3390/md19040207
97. Munekata PES, Domínguez R, Budaraju S, Roselló-Soto E, Barba FJ, Mallikarjunan K, et al. Effect of innovative food processing technologies on the physicochemical and nutritional properties and quality of non-dairy plant-based beverages. Foods. (2020) 9:288. doi: 10.3390/foods9030288
98. Li D-Y, Yuan Z, Liu Z-Q, Yu M-M, Guo Y, Liu X-Y, et al. Effect of oxidation and maillard reaction on color deterioration of ready-to-eat shrimps during storage. LWT. (2020) 131:109696. doi: 10.1016/j.lwt.2020.109696
99. Fukuoka N, Miyata M, Hamada T, Takeshita E. Histochemical observations and gene expression changes related to internal browning in tuberous roots of sweet potato (Ipomea batatas). Plant Sci. (2018) 274:476–84. doi: 10.1016/j.plantsci.2018.07.004
100. Gao Z, Zheng J, Chen L. Ultrasonic accelerates asparagine-glucose non-enzymatic browning reaction without acrylamide formation. Ultrason Sonochem. (2017) 34:626–30. doi: 10.1016/j.ultsonch.2016.06.041
101. He F, Qian YL, Qian MC. Flavor and chiral stability of lemon-flavored hard tea during storage. Food Chem. (2018) 239:622–30. doi: 10.1016/j.foodchem.2017.06.136
102. Cui H, Yu J, Xia S, Duhoranimana E, Huang Q, Zhang X. Improved controlled flavor formation during heat-treatment with a stable Maillard reaction intermediate derived from xylose-phenylalanine. Food Chem. (2019) 271:47–53. doi: 10.1016/j.foodchem.2018.07.161
103. Fukuoka N, Hirabayashi H, Hamada T. Oxidative stress via the Maillard reaction is associated with the occurrence of internal browning in roots of sweetpotato (Ipomoea batatas). Plant Physiol Biochem. (2020) 154:21–9. doi: 10.1016/j.plaphy.2020.05.009
104. Takahashi N, Harada M, Azhary JMK, Kunitomi C, Nose E, Terao H, et al. Accumulation of advanced glycation end products in follicles is associated with poor oocyte developmental competence. Mol Hum Reprod. (2019) 25:684–94. doi: 10.1093/molehr/gaz050
105. Hu LT, Elam E, Ni ZJ, Shen Y, Xia B, Thakur K, et al. The structure and flavor of low sodium seasoning salts in combination with different sesame seed meal protein hydrolysate derived Maillard reaction products. Food Chem.: X. (2021) 121:100148. doi: 10.1016/j.fochx.2021.100148
106. Sun F, Cui H, Zhan H, Xu M, Hayat K, Tahir MU, et al. Aqueous preparation of maillard reaction intermediate from glutathione and xylose and its volatile formation during thermal treatment. J Food Sci. (2019) 84:3584–93. doi: 10.1111/1750-3841.14911
107. Yin M, Shao S, Zhou Z, Chen M, Zhong F, Li Y. Characterization of the key aroma compounds in dog foods by gas chromatography-mass spectrometry, acceptance test, and preference test. J Agri Food Chem. (2020) 68:9195–204. doi: 10.1021/acs.jafc.0c03088
108. Wang L, Qiao K, Huang Y, Zhang Y, Xiao J, Duan W. Optimization of beef broth processing technology and isolation and identification of flavor peptides by consecutive chromatography and LC-QTOF-MS/MS. Food Sci Nutr. (2020) 8:4463–71. doi: 10.1002/fsn3.1746
109. Li Q, Sun H, Zhang M, Wu T. (2020). Characterization of the flavor compounds in wheat bran and biochemical conversion for application in food. J of Food Sci, 85, 1427–1437.
110. Bak KH, Waehrens SS, Fu Y, Chow CY, Petersen MA, Ruiz-Carrascal J, et al. Flavor characterization of animal hydrolysates and potential of glucosamine in flavor modulation. Foods. (2021)10:3008. doi: 10.3390/foods10123008
111. Heo S, Kim JH, Kwak MS, Sung MH, Jeong DW. Functional annotation genome unravels potential probiotic Bacillus velezensis strain KMU01 from traditional Korean fermented Kimchi. Foods. (2021) 10:563. doi: 10.3390/foods10030563
Keywords: Maillard reaction, Maillard reaction products, flavor, flavor mechanism, potential application
Citation: Liu S, Sun H, Ma G, Zhang T, Wang L, Pei H, Li X and Gao L (2022) Insights into flavor and key influencing factors of Maillard reaction products: A recent update. Front. Nutr. 9:973677. doi: 10.3389/fnut.2022.973677
Received: 20 June 2022; Accepted: 11 August 2022;
Published: 12 September 2022.
Edited by:
Yanyan Zhang, University of Hohenheim, GermanyReviewed by:
Majid Nooshkam, Ferdowsi University of Mashhad, IranChao-Kun Wei, Ningxia University, China
Copyright © 2022 Liu, Sun, Ma, Zhang, Wang, Pei, Li and Gao. This is an open-access article distributed under the terms of the Creative Commons Attribution License (CC BY). The use, distribution or reproduction in other forums is permitted, provided the original author(s) and the copyright owner(s) are credited and that the original publication in this journal is cited, in accordance with accepted academic practice. No use, distribution or reproduction is permitted which does not comply with these terms.
*Correspondence: Hanju Sun, sunhanjv@163.com