- 1Functional Control Systems, Graduate School of Engineering and Science, Shibaura Institute of Technology, Saitama, Japan
- 2Department of Bio-Science and Engineering, Shibaura Institute of Technology, Saitama, Japan
B-type procyanidins, a series of catechin oligomers, are among the most ingested polyphenols in the human diet. Results of meta-analyses have suggested that intake of B-type procyanidins reduces cardiovascular disease risk. Another recent focus has been on the effects of B-type procyanidins on central nervous system (CNS) function. Although long-term B-type procyanidin ingestion is linked to health benefits, a single oral intake has been reported to cause physiological alterations in circulation, metabolism, and the CNS. Comprehensive analyses of previous reports indicate an optimal mid-range dose for the hemodynamic effects of B-type procyanidins, with null responses at lower or higher doses, suggesting hormesis. Indeed, polyphenols, including B-type procyanidins, elicit hormetic responses in vitro, but animal and clinical studies are limited. Hormesis of hemodynamic and metabolic responses to B-type procyanidins was recently confirmed in animal studies, however, and our work has linked these effects to the CNS. Here, we evaluate the hormetic response elicited by B-type procyanidins, recontextualizing the results of intervention trials. In addition, we discuss the possibility that this hormetic response to B-type procyanidins arises via CNS neurotransmitter receptors. We have verified the direction of future research for B-type procyanidins in this review.
Introduction
B-type procyanidins are characterized by a series of heteropolyflavan-3-ols, with a single interflavan bond between carbon-4 of the B-ring and either carbon-8 or carbon-6 of the C-ring (1–3). B-type procyanidins can be categorized by their degree of polymerization: monomers form linkages leading to oligomers. The most common monomeric unit is (–)-epicatechin, and the C4–C8 bond (Figure 1A) is the most prominent. B-type procyanidins containing 2–7 monomeric units are defined as oligoprocyanidins which are abundant in cocoa (4–6), apples (7, 8), grape seeds (9, 10), and red wine (11–13).
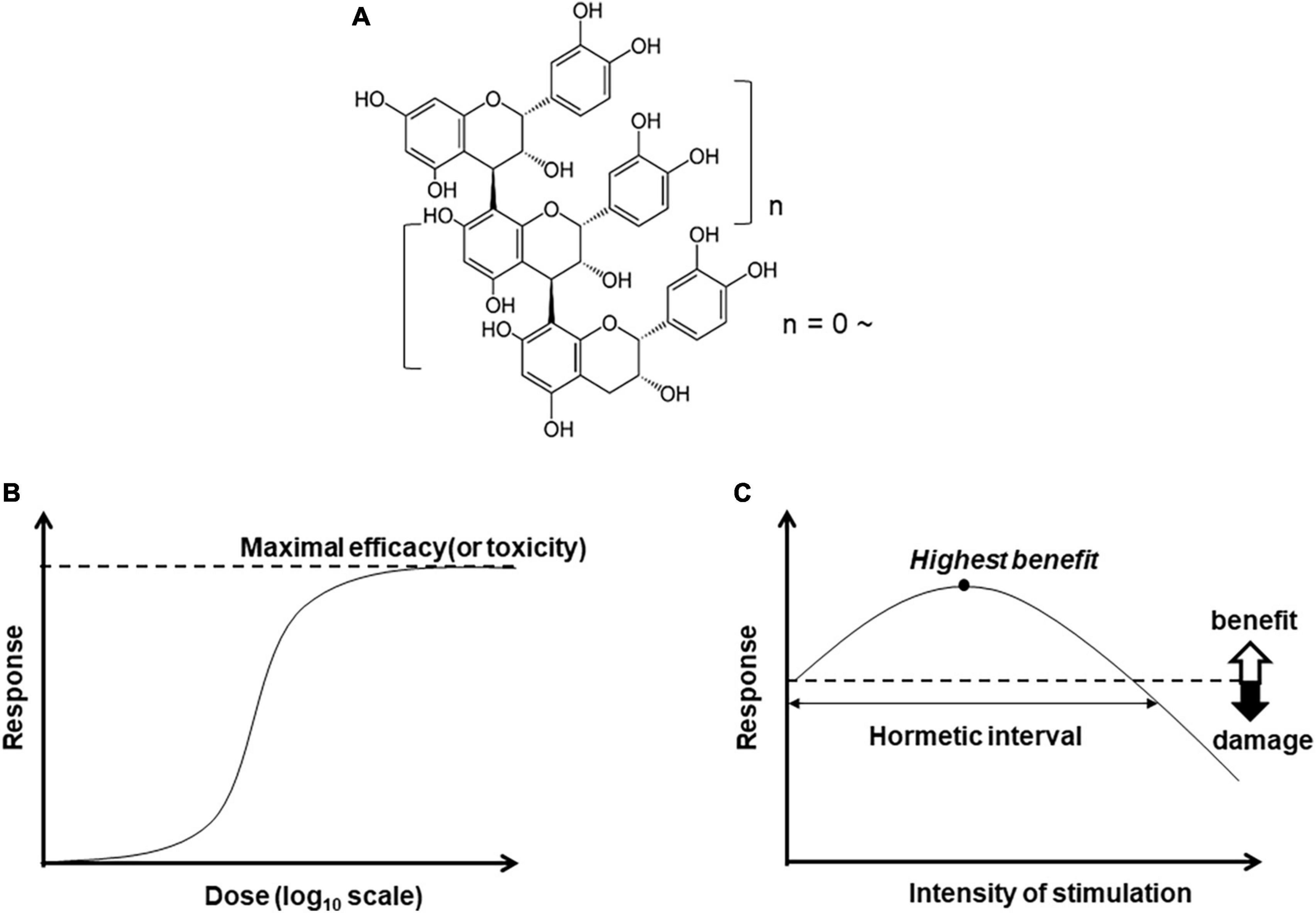
Figure 1. Chemical structure of B-type procyanidin (C4–C8) bond, (A), and dose-response curves: (B) monotonic and (C) hormesis.
Results of meta-analyses have suggested that intake of foods rich in B-type procyanidins is linked to reduced risk for cardiovascular disease, including coronary heart disease, myocardial infarction, and stroke (14–20). Randomized controlled trials and subsequent meta-analyses have confirmed that dark chocolate containing large amounts of B-type procyanidins can mitigate states related to the metabolic syndrome, including hypertension (21–23), dyslipidemia (24, 25), and glucose intolerance (25, 26). In addition, the latest large-scale randomized trial found a 27% reduction in cardiovascular death by ingestion of cocoa flavanol fraction, which is rich in B-type procyanidin monomer and oligomers, for 3.6 years (27). Recent studies have focused on the benefit of B-type procyanidin ingestion for the central nervous system (CNS). A few intervention trials have reported that B-type procyanidin might be effective in improving cognitive function (28–31).
Almost all B-type procyanidins ingested in food move into the colon, and some are degraded by the microbiome (32–34). Consequently, changes in the gut microbiome induced by ingestion of B-type procyanidins for a comparatively long period may alter the composition of metabolites in the colon (32, 35–38). One hypothesis is that these colon changes associated with gut microbiota contribute to the beneficial effects of B-type procyanidins.
Acute physiological changes have been reported to follow a single intake of foods rich in B-type procyanidins. These changes are related to hemodynamics (39–43), metabolism (44, 45), the autonomic nervous system (46), and cognitive function or cerebral blood flow (28, 47–54). These findings highlight the need to evaluate the acute and chronic physiological effects of B-type procyanidin ingestion.
In addition, the acute hemodynamic changes following ingestion of B-type procyanidin, such as flow-mediated dilation (FMD), do not show a monotonic dose response (55). Instead, these physiological changes follow a pattern of hormesis, with peak benefit at mid-range doses and less benefit at higher or lower doses. Comprehensive analyses of many earlier findings suggest that there is likely a mid-range optimal dose for the effects of B-type procyanidins on hemodynamics.
Polyphenols, including B-type procyanidins, elicit hormetic responses in cell culture (56–59). Cellular proliferation occurs at relatively low concentrations, but cytotoxicity is detected at high concentrations (60). In vivo animal and human dose-response findings for B-type procyanidins are relatively limited. Recently, however, results from animal studies confirmed that hemodynamics and metabolism show a hormetic dose-response to B-type procyanidin, and we found that these changes arise through sympathetic nerve activation, driven by CNS activation. Here, we review data from human intervention trials supporting a hormesis pattern of response to B-type procyanidins. Furthermore, we discuss the possibility that B-type procyanidins elicit this response via neurotransmitter receptors expressed in the CNS.
Hormesis
Bioactive compounds are expected to yield a monotonic dose-dependent response in terms of efficacy or toxicity (Figure 1B). In some cases, however, the pattern is characterized by an inverted U-shaped dose-response (Figure 1C). This pattern of hormesis can also reflect an adaptive response. For example, exposure to low amounts of a substance or stressor can induce resistance to higher doses of the same trigger. This exposure to mild levels of harmful factors can precondition a cell or an organism, inducing activation of stress resistance pathways and expanding maintenance and repair capacities (61, 62).
As an example, a moderate exercise program yields various benefits, such as decreased risk of cardiovascular disease, stronger bones and skeletal muscle, and longevity. An overly intense exercise program, however, can lead to harmful effects (63), so that the response to exercise “dose” shows a hormetic pattern. Exercise-related enhancement of cognition and mood also shows a hormetic response (64) that is reported to relate closely to adult hippocampal neurogenesis (65). Furthermore, moderate physical activity is generally accepted to be associated with cardiovascular (66–68) and metabolic benefits through sympathetic nervous system (SNS) (69–71). Recent evidence also links CNS plasticity to the effects of moderate exercise on SNS activity (72).
Polyphenols elicit a hormetic response in cell culture (73); curcumin (74), resveratrol (75) and B-type procyanidins (76). The hormesis effect of other polyphenols also has been confirmed in vitro, and these activities are considered to arise from modulation of a number of redox-based signaling pathways. Abundant evidence thus supports a hormesis effect of polyphenols in in vitro studies, but limited data illustrate these effects in vivo.
Hormetic response to B-type procyanidin in intervention and animal studies
As mentioned above, repeated ingestion of B-type procyanidins is reported to reduce the risk of cardiovascular diseases. Besides numerous intervention trials have been examined following the single ingestion of foods rich in B-type procyanidins. Regarding hemodynamics, a single ingestion was associated with increased FMD at about 2 h following ingestion (23). These results indicated that a single treatment of B-type procyanidin might improve vascular endothelial cell function. Sun et al. assessed the dose-response pattern of human endothelial function to B-type procyanidin in cocoa (55). They concluded that cocoa flavanols could significantly improve endothelial function, with an optimal dose of about 710 mg. They also observed a non-linear association (inverted U-shape) between cocoa flavanols and FMD. There were no notable adverse effects in the intervention studies using 1.4 times (1,008 mg) or 1.76 times (1,248 mg) the effective dose (710 mg) shown by Sun et al. (55). Since these intervention studies used cocoa drinks or chocolate as the test food, it was limited intake amount. Therefore, the toxicity of type B-type procyanidins may not detect.
In addition, results of a recent intervention trial indicate that repeated supplements of B-type procyanidins are associated with improvement on memory tasks that depend on dentate gyrus functions (52). Intervention trials have been conducted in young adults, examining the effects on a memory task of a single ingestion of cocoa flavanols at doses from 172 to 994 mg (77). In almost all cases, cocoa flavanols were associated with enhanced working memory or mood and reduced fatigue, but evidence of dose-response in CNS studies is limited.
These results, taken together, suggest that a hormetic physiological response following a single intake of B-type procyanidins is likely. Studies of other polyphenols, such as curcumin and resveratrol, are too limited to allow for interpretations regarding dose response (78).
Repeated oral gavage with 10 mg/kg body weight (bw) of cocoa flavanol in rats resulted in significantly decreased blood pressure and markedly increased aortic endothelial nitric oxide synthase expression (eNOS), indicating this dose as optimal (79). On the other hand, a single oral administration of 10 mg/kg cocoa flavanol, resulted in a transient increase in mean blood pressure (BP) and heart rate (HR), along with a marked increase in blood flow in the cremaster muscle arteriole soon after treatment. A significant increase in eNOS phosphorylation was also observed in aorta dissected 60 min after this treatment. Similar but weaker alterations were observed at a dose of 1 mg/kg cocoa flavanol but 100 mg/kg cocoa flavanol did not trigger any changes in hemodynamics or eNOS phosphorylation.
We also compared B-type procyanidins such as the monomer [(-)-epicatechin; EC], dimer (procyanidin B2; B2), trimer (procyanidin C1; C1), and tetramer (cinnamtannin A2; A2) on hemodynamics (80). At a dose of 10 μg/kg, A2 and B2 were associated with a marked increase in cremasteric arteriole blood flow, C1 was linked to a slight increase, and EC did not trigger any change. Based on these findings, a relative efficacy of B-type procyanidins on hemodynamics was suggested as follows: A2 > B2 > > C1 > > > EC (Figure 2). A dose-response study of A2 showed increased blood flow with a single dose of 10 μg/kg, but not with a dose of 100 μg/kg. In our dose-response study for A2 induction of thermogenic uncoupling protein (UCP)-1 expression in brown adipose tissue (BAT), we found that a single oral dose at 1 μg/kg was associated with significantly increased UCP-1 mRNA expression (Figure 2), but more than 1 μg/kg A2 (10 to 1,000 μg/kg) did not show any change (81).
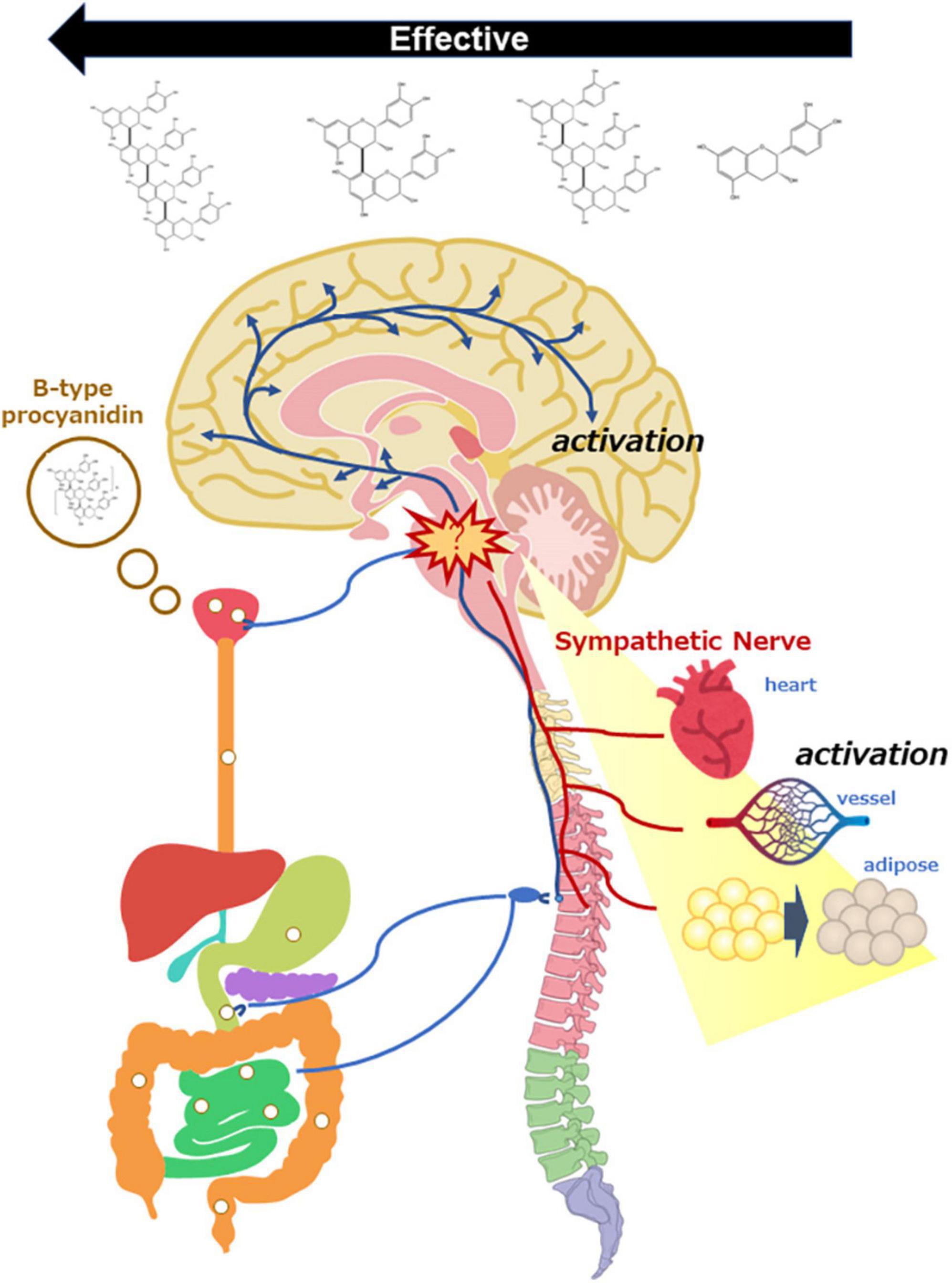
Figure 2. Scheme of sympathetic nerve activation by oral administration of B-type procyanidins, consequently activation of the central nervous system and peripheral organs.
Taken together, the results of animal studies of cocoa flavanol or the B-type procyanidins are consistent with those of intervention studies following a single intake of food rich in B-type procyanidin. The implication is that this polyphenol elicits an inverted U-shaped dose-response.
Target organ of B-type procyanidins from the perspective of bioavailability
B-type procyanidins show poor bioavailability, and intact forms in foods are hardly present in the blood (82). For this reason, how these polyphenols exert beneficial effects remains unclear. Recent studies suggest that the physiological changes following repeated B-type procyanidins ingestion may be related to alterations in gut microflora and/or their metabolites, but the mechanism for changes arising immediately after a single dose is unclear. Considering that most B-type procyanidins are present in the feces, the target organ of them is the gastrointestinal tract, including the oral cavity.
Single doses of B-type procyanidins do not draw a monotonic dose-response, and benefits are seen at the mid-range doses but not at lower or higher doses. Among various pharmacological agents, those that support social interactions or memory are reported to show biphasic reactions (83) and enhance memory (84). A single oral ingestion of cocoa flavanol has been also reported to improve cognition and mood in intervention studies. As noted, the primary target organ of B-type procyanidins appears to be the digestive tract, but activation of the CNS may be crucial to the mechanism of action.
Sympathetic nerve activation by B-type procyanidins
A single oral optimal dose of cocoa flavanol triggers an increase in blood flow in the cremaster arteriole soon after treatment in rats (85). Such a rapid response likely does not depend on absorption or distribution in the blood. The SNS is a well-known regulator of hemodynamic reflection, exerting its influence through adrenergic receptors (AdR) expressed in the myocardium, vascular smooth muscle, and vasomotor center in the medulla oblongata (86). Activation of myocardial β1 AdR, which are expressed predominantly in cardiac tissue, causes increased cardiac output and HR. Activation of the α1 AdR in vascular smooth muscle contracts blood vessels, leading to elevated BP (Figure 3) (87). For this reason, we used adrenaline blockers to examine whether the SNS is involved in the hemodynamic changes induced by B-type procyanidins. We found that a transient increase in HR caused by an optimal dose of cocoa flavanol could be markedly decreased by co-treatment with a β1 AdR blocker in rats. In addition, co-treatment with an α1 blocker inhibited the transient elevation in BP that a single oral dose of cocoa flavanol induced.
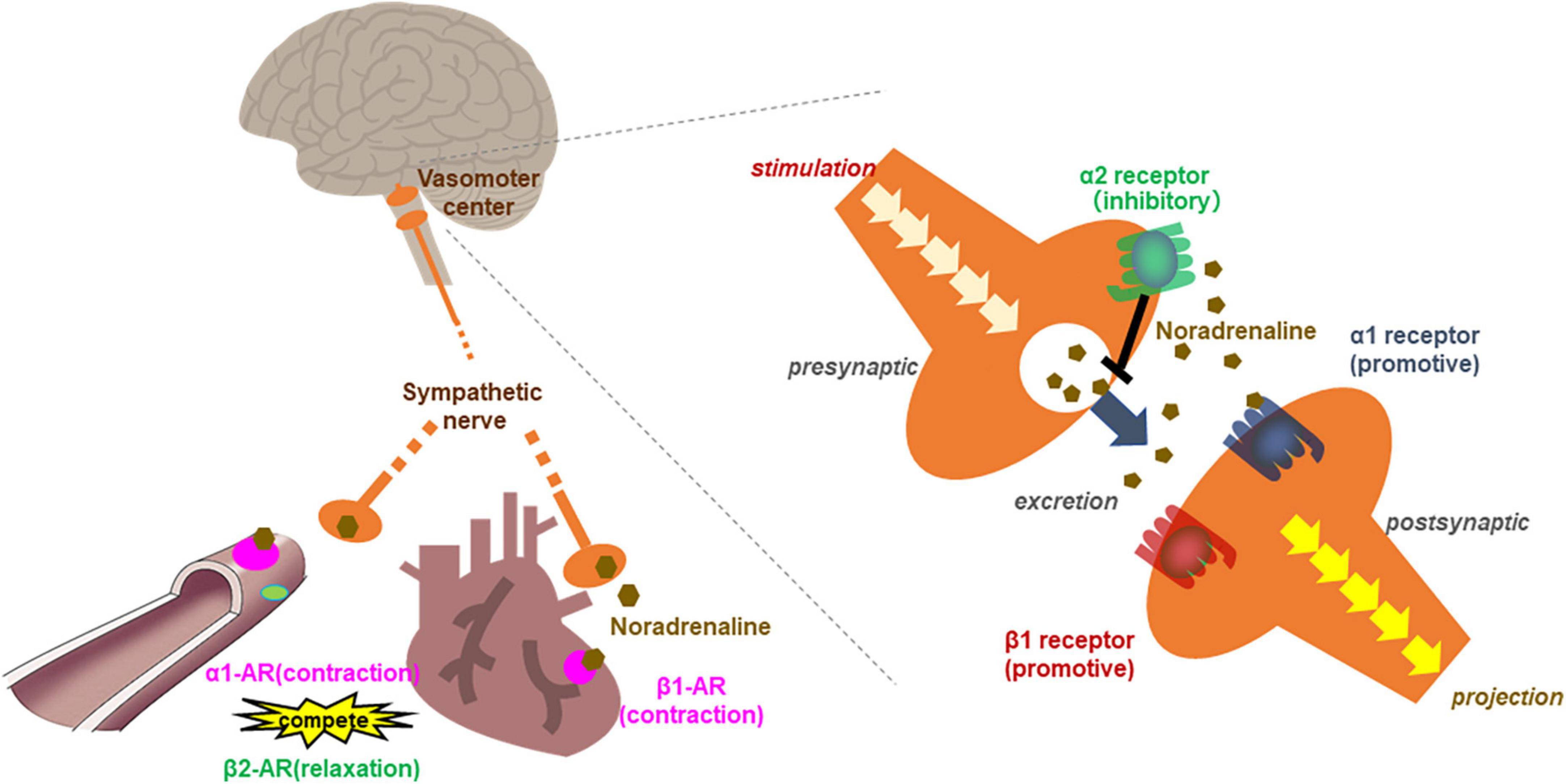
Figure 3. Scheme of the relationship between adrenergic receptors in the circulation system. β1 adrenergic receptors contracts the myocardium and increased cardiac output and heart rate. α1 adrenergic receptor in vascular smooth muscle contracts blood vessels, leading to elevated blood pressure. While β2 receptor relaxes vascular smooth muscle as a competitive effect against α1 adrenergic receptors. In the vasomotor center, presynaptic neurons release noradrenaline (NA) into the synaptic cleft upon stimulation. Noradrenaline binds the activating receptors of the postsynaptic neuron. NA also binds inhibitory autoreceptors of the presynaptic neurons, consequently, inhibited NA release from postsynaptic neurons.
Sympathetic nervous system also regulates non-shivering thermogenesis through β3 AdR in BAT via UCP-1 (88). We have found that UCP-1 mRNA upregulation in BAT after an optimal dose of cocoa flavanol is markedly attenuated by co-administration of β3 blocker. These results implicate the SNS in the acute hemodynamic and metabolic changes following a single oral dose of B-type procyanidins.
In hormesis, effects at high doses can be less than effects at optimal doses. We evaluated the hormetic pattern of response to B-type procyanidins in vivo and found that a single oral administration of 10-fold the optimal dose of cocoa flavanol in rats yielded no transient hemodynamic alterations (79). In addition, as noted, an optimal dose of cocoa flavanol increased UCP-1 mRNA expression in BAT, but this change was markedly dampened at doses 10-fold the optimal level (89). Based on our findings linking the hemodynamic and thermogenic effects of B-type procyanidins to SNS activation, we focused on why optimal dose elicit these effects but not high doses.
Blood pressure and heart rate are regulated competitively by inhibitory and activating AdR. Activation of the β2 receptor relaxes vascular smooth muscle as a competitive effect against the vasoconstrictive action of α1 AdR and thus decreases BP (Figure 3) (87). In our co-administration study with a high dose of B-type procyanidins and α1 blocker in rats, although, we found no changes in BP (79). Besides, inhibitory α2 AdR, which are expressed in the preganglionic sympathetic fibers and vasomotor center in the CNS, down-regulate the SNS. Yohimbine is an α2 blocker that is reported to be more effective in CNS than SNS. Given this pattern, we conducted a co-administration study with a high dose of cocoa flavanol (100 mg/kg) and yohimbine. A single high dose of cocoa flavanol alone elicited no change in BP, but BP increased markedly and transiently by co-administered with yohimbine. Similar results were observed co-administration of B-type procyanidin tetramer A2 (100 μg/kg) and yohimbine (80).
As mentioned above, whereas a single oral dose of 1 μg/kg of A2 significantly increased UCP-1 mRNA expression in BAT, doses from 10 to 1,000 μg/kg A2 did not (81). In contrast, co-administration of a high dose (100 μg/kg) of A2 and yohimbine markedly increased UCP-1 mRNA expression. A recent report suggested that the premotor neurons controlling thermogenic effector activation lie primarily within the medullary rostral raphe pallidus (90). Non-shivering thermogenesis through the β3 receptor is inhibited by α2AR activation in this region (91).
α2 AdR are present on noradrenergic terminals in the peripheral nervous system and the CNS (92). α2 adrenergic autoreceptors lie in the presynaptic membrane of adrenergic neurons, inhibiting exocytosis of their neurotransmitters (mostly noradrenaline) as part of a negative feedback loop (93, 94) (Figure 3). Feedback inhibition of noradrenaline release from sympathetic nerves by α2-autoreceptors limits its exocytosis and protects heart under normal conditions (95). The reduced hemodynamic and metabolic output at a high dose of B-type procyanidins observed in our previous studies may cause activation of autoreceptor α2. Thus, SNS deactivation may be induced by a high dose of B-type procyanidins.
Stress and hormetic response to B-type procyanidins
The relationship between stress and hormetic responses is well known. Various factors induce the stress response, which involves rapid activation of the sympathetic–adreno–medullar (SAM) axis and the hypothalamus–pituitary–adrenal (HPA) axis (96). In the SAM, rapid physiological adaptation mediated mainly by noradrenaline results in transient responses, such as alertness, and appraisal of the situation, enabling a strategic decision. Sympathetic modulations induced by stressors rely on direct projections from the paraventricular nucleus of the hypothalamus (PVN), locus coeruleus, and rostral ventrolateral medulla to pre-ganglionic sympathetic neurons present in the dorsal intermediolateral cellular column of the spinal cord (97). As a result, noradrenaline is secreted from sympathetic nerve terminals, leading to activation of signaling pathways that evoke changes in blood vessels, glands, visceral organs, and smooth muscle. Considering the previous results following a single oral administration of B-type procyanidins, these changes may be induced by activation of the SNS.
The PVN, which also has a role in eliciting activation of the HPA, synthesizes oxytocin, vasopressin, and corticotropin-releasing hormone (CRH), depending on the target (98). CRH excreted from the PVN to the anterior pituitary induces release of adrenocorticotropic hormone, which drives the responses associated with release of cortisol (corticosterone in rodents) from the adrenal gland in the hours following stress. When blood cortisol exceeds a certain level, it exerts negative feedback on the hypothalamic release of CRH and the pituitary release of adrenocorticotropic hormone (99). Activation of these pathways results in adaptive conditions that mediate long-term memories of the experience. Therefore, HPA activation induced by optimal stress has a strong positive effect on memory, cognition, and stress resilience (100).
If the outcome following a single dose of B-type procyanidins arises as stress response, HPA activation is expected to occur at the same time as sympathetic hyperactivity. Therefore, we examined the activation of HPA following a single dose of B-type procyanidins. In mouse PVN, the optimal dose of cocoa flavanol (10 mg/kg bw) markedly upregulated CRH mRNA, as detected by in situ hybridization, 240 min after administration. A dose of 50 mg/kg cocoa flavanol also showed similar alterations 60 min after administration, with a significant elevation in plasma corticosterone (101). In addition, CRH mRNA in mouse PVN was increased significantly 60 min after administration of an optimal dose of A2 (10 μg/kg), and a similar change only 15 min after administration of a 10-fold oral dose of A2 (102). Few reports have described the relationship between stress intensity and the duration of response, but our results suggested that the reaction is faster with exposure to more severe stress. Taken together, these findings indicate that stimulation with an oral dose of B-type procyanidin might be a stressor for mammals, resulting in SNS activation (Figure 2).
Conclusion
Various stressors such as radiation, reactive oxygen species, calorie restriction, temperature, chemicals, and exercise elicit hormetic responses (103). Hormesis and the underlying biochemical pathways induced by the stressors confer protection against a range of pathological or aging processes (62). In this review, we especially focused on the hormetic alterations induced by B-type procyanidins, which are electrophilic compounds that easily cause redox reactions. The relationship between CNS activation and the chemical characteristics of B-type procyanidins remains unclear and requires further clarification. B-type procyanidins or related compounds may contribute to the beneficial effects of eating fruits and vegetables through hormetic responses induced by neuromodulation.
Author contributions
TF and YF collected the sources and drafted this manuscript. NO constructed the conception and finally approved of this manuscript. All authors contributed to the article and approved the submitted version.
Funding
This work was supported by JSPS KAKENHI (Grant Number: 19H04036).
Conflict of interest
The authors declare that the research was conducted in the absence of any commercial or financial relationships that could be construed as a potential conflict of interest.
Publisher’s note
All claims expressed in this article are solely those of the authors and do not necessarily represent those of their affiliated organizations, or those of the publisher, the editors and the reviewers. Any product that may be evaluated in this article, or claim that may be made by its manufacturer, is not guaranteed or endorsed by the publisher.
References
1. Rue EA, Rush MD, van Breemen RB. Procyanidins: a comprehensive review encompassing structure elucidation via mass spectrometry. Phytochem Rev. (2018) 17:1–16. doi: 10.1007/s11101-017-9507-3
2. Rauf A, Imran M, Abu-Izneid T, Iahtisham Ul H, Patel S, Pan X, et al. Proanthocyanidins: a comprehensive review. Biomed Pharmacother. (2019) 116:108999. doi: 10.1016/j.biopha.2019.108999
3. Smeriglio A, Barreca D, Bellocco E, Trombetta D. Proanthocyanidins and hydrolysable tannins: occurrence, dietary intake and pharmacological effects. Br J Pharmacol. (2017) 174:1244–62. doi: 10.1111/bph.13630
4. Hatano T, Miyatake H, Natsume M, Osakabe N, Takizawa T, Ito H, et al. Proanthocyanidin glycosides and related polyphenols from cacao liquor and their antioxidant effects. Phytochemistry. (2002) 59:749–58. doi: 10.1016/s0031-9422(02)00051-1
5. Natsume M, Osakabe N, Yamagishi M, Takizawa T, Nakamura T, Miyatake H, et al. Analyses of polyphenols in cacao liquor, cocoa, and chocolate by normal-phase and reversed-phase HPLC. Biosci Biotechnol Biochem. (2000) 64:2581–7. doi: 10.1271/bbb.64.2581
6. Pedan V, Fischer N, Bernath K, Huhn T, Rohn S. Determination of oligomeric proanthocyanidins and their antioxidant capacity from different chocolate manufacturing stages using the NP-HPLC-online-DPPH methodology. Food Chem. (2017) 214:523–32. doi: 10.1016/j.foodchem.2016.07.094
7. Shoji T, Yanagida A, Kanda T. Gel permeation chromatography of anthocyanin pigments from Rose cider and red wine. J Agric Food Chem. (1999) 47:2885–90. doi: 10.1021/jf981311k
8. Shoji T, Obara M, Takahashi T, Masumoto S, Hirota H, Miura T. The differences in the Flavan-3-ol and procyanidin contents of the Japanese ‘fuji’ and ‘orin’ apples using a rapid quantitative high-performance liquid chromatography method: estimation of the japanese intake of flavan-3-ols and procyanidins from apple as case study. Foods. (2021) 10:274. doi: 10.3390/foods10020274
9. Muñoz-González C, Criado C, Pérez-Jiménez M, Pozo-Bayón M. Evaluation of the effect of a grape seed tannin extract on wine ester release and perception using in vitro and in vivo instrumental and sensory approaches. Foods. (2021) 10:93. doi: 10.3390/foods100100937
10. Rajakumari R, Volova T, Oluwafemi OS, Rajesh Kumar S, Thomas S, Kalarikkal N. Grape seed extract-soluplus dispersion and its antioxidant activity. Drug Dev Ind Pharm. (2020) 46:1219–29. doi: 10.1080/03639045.2020.1788059
11. Carando S, Teissedre PL. Catechin and procyanidin levels in French wines: contribution to dietary intake. Basic Life Sci. (1999) 66:725–37.
12. Bertelli AA, Das DK. Grapes, wines, resveratrol, and heart health. J Cardiovasc Pharmacol. (2009) 54:468–76. doi: 10.1097/FJC.0b013e3181bfaff3
13. Merkytė V, Longo E, Jourdes M, Jouin A, Teissedre PL, Boselli E. High-Performance liquid chromatography-hydrogen/deuterium exchange-high-resolution mass spectrometry partial identification of a series of tetra- and pentameric cyclic procyanidins and prodelphinidins in wine extracts. J Agric Food Chem. (2020) 68:3312–21. doi: 10.1021/acs.jafc.9b06195
14. Buitrago-Lopez A, Sanderson J, Johnson L, Warnakula S, Wood A, Di Angelantonio E, et al. Chocolate consumption and cardiometabolic disorders: systematic review and meta-analysis. BMJ. (2011) 343:d4488. doi: 10.1136/bmj.d4488
15. Larsson SC, Virtamo J, Wolk A. Chocolate consumption and risk of stroke: a prospective cohort of men and meta-analysis. Neurology. (2012) 79:1223–9. doi: 10.1212/WNL.0b013e31826aacfa
16. McCullough ML, Peterson JJ, Patel R, Jacques PF, Shah R, Dwyer JT. Flavonoid intake and cardiovascular disease mortality in a prospective cohort of US adults. Am J Clin Nutr. (2012) 95:454–64. doi: 10.3945/ajcn.111.016634
17. Quinones M, Miguel M, Aleixandre A. Beneficial effects of polyphenols on cardiovascular disease. Pharmacol Res. (2013) 68:125–31. doi: 10.1016/j.phrs.2012.10.018
18. Yuan S, Li X, Jin Y, Lu J. Chocolate consumption and risk of coronary heart disease, stroke, and diabetes: a meta-analysis of prospective studies. Nutrients. (2017) 9:688. doi: 10.3390/nu9070688
19. Larsson SC, Åkesson A, Gigante B, Wolk A. Chocolate consumption and risk of myocardial infarction: a prospective study and meta-analysis. Heart. (2016) 102:1017–22. doi: 10.1136/heartjnl-2015-309203
20. Gong F, Yao S, Wan J, Gan X. Chocolate consumption and risk of heart failure: a meta-analysis of prospective studies. Nutrients. (2017) 9:402. doi: 10.3390/nu9040402
21. Desch S, Schmidt J, Kobler D, Sonnabend M, Eitel I, Sareban M, et al. Effect of cocoa products on blood pressure: systematic review and meta-analysis. Am J Hypertens. (2010) 23:97–103. doi: 10.1038/ajh.2009.213
22. Taubert D, Berkels R, Roesen R, Klaus W. Chocolate and blood pressure in elderly individuals with isolated systolic hypertension. JAMA. (2003) 290:1029–30. doi: 10.1001/jama.290.8.1029
23. Ried K, Fakler P, Stocks NP. Effect of cocoa on blood pressure. Cochrane Database Syst Rev. (2017) 4:Cd008893. doi: 10.1002/14651858.CD008893.pub3
24. Darand M, Hajizadeh Oghaz M, Hadi A, Atefi M, Amani R. The effect of cocoa/dark chocolate consumption on lipid profile, glycemia, and blood pressure in diabetic patients: a meta-analysis of observational studies. Phytother Res. (2021) 35:5487–501. doi: 10.1002/ptr.7183
25. Lin X, Zhang I, Li A, Manson JE, Sesso HD, Wang L, et al. Cocoa flavanol intake and biomarkers for cardiometabolic health: a systematic review and meta-analysis of randomized controlled trials. J Nutr. (2016) 146:2325–33. doi: 10.3945/jn.116.237644
26. Veronese N, Demurtas J, Celotto S, Caruso MG, Maggi S, Bolzetta F, et al. Is chocolate consumption associated with health outcomes? An umbrella review of systematic reviews and meta-analyses. Clin Nutr. (2019) 38:1101–8. doi: 10.1016/j.clnu.2018.05.019
27. Sesso HD, Manson JE, Aragaki AK, Rist PM, Johnson LG, Friedenberg G, et al. Effect of cocoa flavanol supplementation for prevention of cardiovascular disease events: the COSMOS randomized clinical trial. Am J Clin Nutr. (2022) 115:1490–1500. doi: 10.1093/ajcn/nqac055
28. Desideri G, Kwik-Uribe C, Grassi D, Necozione S, Ghiadoni L, Mastroiacovo D, et al. Benefits in cognitive function, blood pressure, and insulin resistance through cocoa flavanol consumption in elderly subjects with mild cognitive impairment: the Cocoa. Cognition, and Aging (CoCoA) study. Hypertension. (2012) 60:794–801. doi: 10.1161/hypertensionaha.112.193060
29. Mastroiacovo D, Kwik-Uribe C, Grassi D, Necozione S, Raffaele A, Pistacchio L, et al. Cocoa flavanol consumption improves cognitive function, blood pressure control, and metabolic profile in elderly subjects: the cocoa, cognition, and aging (CoCoA) study–a randomized controlled trial. Am J Clin Nutr. (2015) 101:538–48. doi: 10.3945/ajcn.114.092189
30. Brickman AM, Khan UA, Provenzano FA, Yeung LK, Suzuki W, Schroeter H, et al. Enhancing dentate gyrus function with dietary flavanols improves cognition in older adults. Nat Neurosci. (2014) 17:1798–803. doi: 10.1038/nn.3850
31. Sloan RP, Wall M, Yeung LK, Feng T, Feng X, Provenzano F, et al. Insights into the role of diet and dietary flavanols in cognitive aging: results of a randomized controlled trial. Sci Rep. (2021) 11:3837. doi: 10.1038/s41598-021-83370-2
32. Wiese S, Esatbeyoglu T, Winterhalter P, Kruse HP, Winkler S, Bub A, et al. Comparative biokinetics and metabolism of pure monomeric, dimeric, and polymeric flavan-3-ols: a randomized cross-over study in humans. Mol Nutr Food Res. (2015) 59:610–21. doi: 10.1002/mnfr.201400422
33. Sorrenti V, Ali S, Mancin L, Davinelli S, Paoli A, Scapagnini G. Cocoa polyphenols and gut microbiota interplay: bioavailability, prebiotic effect, and impact on human health. Nutrients. (2020) 12:1908. doi: 10.3390/nu12071908
34. Gómez-Juaristi M, Sarria B, Martínez-López S, Bravo Clemente L, Mateos R. Flavanol bioavailability in two cocoa products with different phenolic content. A comparative study in humans. Nutrients. (2019) 11:1441. doi: 10.3390/nu11071441
35. Urpi-Sarda M, Monagas M, Khan N, Lamuela-Raventos RM, Santos-Buelga C, Sacanella E, et al. Epicatechin, procyanidins, and phenolic microbial metabolites after cocoa intake in humans and rats. Anal Bioanal Chem. (2009) 394:1545–56. doi: 10.1007/s00216-009-2676-1
36. Martin FP, Montoliu I, Nagy K, Moco S, Collino S, Guy P, et al. Specific dietary preferences are linked to differing gut microbial metabolic activity in response to dark chocolate intake. J Proteome Res. (2012) 11:6252–63. doi: 10.1021/pr300915z
37. Urpi-Sarda M, Monagas M, Khan N, Llorach R, Lamuela-Raventós RM, Jáuregui O, et al. Targeted metabolic profiling of phenolics in urine and plasma after regular consumption of cocoa by liquid chromatography-tandem mass spectrometry. J Chromatogr A. (2009) 1216:7258–67. doi: 10.1016/j.chroma.2009.07.058
38. Marhuenda-Muñoz M, Laveriano-Santos EP, Tresserra-Rimbau A, Lamuela-Raventós RM, Martínez-Huélamo M, Vallverdú-Queralt A. Microbial phenolic metabolites: which molecules actually have an effect on human health? Nutrients. (2019) 11:2725. doi: 10.3390/nu11112725
39. Farouque HM, Leung M, Hope SA, Baldi M, Schechter C, Cameron JD, et al. Acute and chronic effects of flavanol-rich cocoa on vascular function in subjects with coronary artery disease: a randomized double-blind placebo-controlled study. Clin Sci. (2006) 111:71–80. doi: 10.1042/cs20060048
40. Monahan KD, Feehan RP, Kunselman AR, Preston AG, Miller DL, Lott ME. Dose-dependent increases in flow-mediated dilation following acute cocoa ingestion in healthy older adults. J Appl Physiol. (2011) 111:1568–74. doi: 10.1152/japplphysiol.00865.2011
41. Dower JI, Geleijnse JM, Kroon PA, Philo M, Mensink M, Kromhout D, et al. Does epicatechin contribute to the acute vascular function effects of dark chocolate? A randomized, crossover study. Mol Nutr Food Res. (2016) 60:2379–86. doi: 10.1002/mnfr.201600045
42. Engler MB, Engler MM, Chen CY, Malloy MJ, Browne A, Chiu EY, et al. Flavonoid-rich dark chocolate improves endothelial function and increases plasma epicatechin concentrations in healthy adults. J Am Coll Nutr. (2004) 23:197–204. doi: 10.1080/07315724.2004.10719361
43. Heiss C, Dejam A, Kleinbongard P, Schewe T, Sies H, Kelm M. Vascular effects of cocoa rich in flavan-3-ols. JAMA. (2003) 290:1030–1. doi: 10.1001/jama.290.8.1030
44. Basu A, Betts NM, Leyva MJ, Fu D, Aston CE, Lyons TJ. Acute cocoa supplementation increases postprandial HDL cholesterol and insulin in obese adults with type 2 diabetes after consumption of a high-fat breakfast. J Nutr. (2015) 145:2325–32. doi: 10.3945/jn.115.215772
45. Naissides M, Mamo JC, James AP, Pal S. The effect of acute red wine polyphenol consumption on postprandial lipaemia in postmenopausal women. Atherosclerosis. (2004) 177:401–8. doi: 10.1016/j.atherosclerosis.2004.07.025
46. Spaak J, Merlocco AC, Soleas GJ, Tomlinson G, Morris BL, Picton P, et al. Dose-related effects of red wine and alcohol on hemodynamics, sympathetic nerve activity, and arterial diameter. Am J Physiol Heart Circ Physiol. (2008) 294:H605–12. doi: 10.1152/ajpheart.01162.2007
47. Decroix L, Tonoli C, Soares DD, Tagougui S, Heyman E, Meeusen R. Acute cocoa flavanol improves cerebral oxygenation without enhancing executive function at rest or after exercise. Appl Physiol Nutr Metab. (2016) 41:1225–32. doi: 10.1139/apnm-2016-0245
48. Karabay A, Saija JD, Field DT, Akyürek EG. The acute effects of cocoa flavanols on temporal and spatial attention. Psychopharmacology. (2018) 235:1497–511. doi: 10.1007/s00213-018-4861-4
49. Scholey AB, French SJ, Morris PJ, Kennedy DO, Milne AL, Haskell CF. Consumption of cocoa flavanols results in acute improvements in mood and cognitive performance during sustained mental effort. J Psychopharmacol. (2010) 24:1505–14. doi: 10.1177/0269881109106923
50. Field DT, Williams CM, Butler LT. Consumption of cocoa flavanols results in an acute improvement in visual and cognitive functions. Physiol Behav. (2011) 103:255–60. doi: 10.1016/j.physbeh.2011.02.013
51. Gratton G, Weaver SR, Burley CV, Low KA, Maclin EL, Johns PW, et al. Dietary flavanols improve cerebral cortical oxygenation and cognition in healthy adults. Sci Rep. (2020) 10:19409. doi: 10.1038/s41598-020-76160-9
52. Decroix L, van Schuerbeek P, Tonoli C, van Cutsem J, Soares DD, Heyman E, et al. The effect of acute cocoa flavanol intake on the BOLD response and cognitive function in type 1 diabetes: a randomized, placebo-controlled, double-blinded cross-over pilot study. Psychopharmacology. (2019) 236:3421–8. doi: 10.1007/s00213-019-05306-z
53. Francis ST, Head K, Morris PG, Macdonald IA. The effect of flavanol-rich cocoa on the fMRI response to a cognitive task in healthy young people. J Cardiovasc Pharmacol. (2006) 47(Suppl. 2):S215–20. doi: 10.1097/00005344-200606001-00018
54. Jackson PA, Wightman EL, Veasey R, Forster J, Khan J, Saunders C, et al. A randomized, crossover study of the acute cognitive and cerebral blood flow effects of phenolic, nitrate and botanical beverages in young, healthy humans. Nutrients. (2020) 12:2254. doi: 10.3390/nu12082254
55. Sun Y, Zimmermann D, De Castro CA, Actis-Goretta L. Dose-response relationship between cocoa flavanols and human endothelial function: a systematic review and meta-analysis of randomized trials. Food Funct. (2019) 10:6322–30. doi: 10.1039/c9fo01747j
56. Tamir S, Eizenberg M, Somjen D, Stern N, Shelach R, Kaye A, et al. Estrogenic and antiproliferative properties of glabridin from licorice in human breast cancer cells. Cancer Res. (2000) 60:5704–9.
57. Maggiolini M, Bonofiglio D, Marsico S, Panno ML, Cenni B, Picard D, et al. Estrogen receptor alpha mediates the proliferative but not the cytotoxic dose-dependent effects of two major phytoestrogens on human breast cancer cells. Mol Pharmacol. (2001) 60:595–602.
58. Elattar TM, Virji AS. The inhibitory effect of curcumin, genistein, quercetin and cisplatin on the growth of oral cancer cells in vitro. Anticancer Res. (2000) 20:1733–8.
59. Oh SM, Kim YP, Chung KH. Biphasic effects of kaempferol on the estrogenicity in human breast cancer cells. Arch Pharm Res. (2006) 29:354–62. doi: 10.1007/bf02968584
60. Martel J, Ojcius DM, Ko YF, Ke PY, Wu CY, Peng HH, et al. Hormetic effects of phytochemicals on health and longevity. Trends Endocrinol Metab. (2019) 30:335–46. doi: 10.1016/j.tem.2019.04.001
62. Gems D, Partridge L. Stress-Response hormesis and aging: “that which does not kill us makes us stronger”. Cell Metab. (2008) 7:200–3. doi: 10.1016/j.cmet.2008.01.001
63. Gradari S, Pallé A, McGreevy KR. Fontán-Lozano Á, Trejo JL. Can exercise make you smarter, happier, and have more neurons? A hormetic perspective. Front Neurosci. (2016) 10:93. doi: 10.3389/fnins.2016.00093
64. Mattson MP. Energy intake and exercise as determinants of brain health and vulnerability to injury and disease. Cell Metab. (2012) 16:706–22. doi: 10.1016/j.cmet.2012.08.012
65. Holmes MM, Galea LA, Mistlberger RE, Kempermann G. Adult hippocampal neurogenesis and voluntary running activity: circadian and dose-dependent effects. J Neurosci Res. (2004) 76:216–22. doi: 10.1002/jnr.20039
66. Cechetto DF, Shoemaker JK. Functional neuroanatomy of autonomic regulation. Neuroimage. (2009) 47:795–803. doi: 10.1016/j.neuroimage.2009.05.024
67. Charkoudian N, Wallin BG. Sympathetic neural activity to the cardiovascular system: integrator of systemic physiology and interindividual characteristics. Compr Physiol. (2014) 4:825–50. doi: 10.1002/cphy.c130038
68. Halliwill JR, Morgan BJ, Charkoudian N. Peripheral chemoreflex and baroreflex interactions in cardiovascular regulation in humans. J Physiol. (2003) 552(Pt. 1):295–302. doi: 10.1113/jphysiol.2003.050708
69. Fu Q, Levine BD. Exercise and the autonomic nervous system. Handb Clin Neurol. (2013) 117:147–60. doi: 10.1016/b978-0-444-53491-0.00013-4
70. Brito NA, Brito MN, Bartness TJ. Differential sympathetic drive to adipose tissues after food deprivation, cold exposure or glucoprivation. Am J Physiol Regul Integr Comp Physiol. (2008) 294:R1445–52. doi: 10.1152/ajpregu.00068.2008
71. Labbé SM, Caron A, Chechi K, Laplante M, Lecomte R, Richard D. Metabolic activity of brown, “beige,” and white adipose tissues in response to chronic adrenergic stimulation in male mice. Am J Physiol Endocrinol Metab. (2016) 311:E260–8. doi: 10.1152/ajpendo.00545.2015
72. Mueller PJ. Exercise training and sympathetic nervous system activity: evidence for physical activity dependent neural plasticity. Clin Exp Pharmacol Physiol. (2007) 34:377–84. doi: 10.1111/j.1440-1681.2007.04590.x
73. Kanner J. Polyphenols by generating H2O2, affect cell redox signaling, inhibit PTPs and activate Nrf2 axis for adaptation and cell surviving: in vitro, in vivo and human health. Antioxidants. (2020) 9:797. doi: 10.3390/antiox9090797
74. Concetta Scuto M, Mancuso C, Tomasello B, Laura Ontario M, Cavallaro A, Frasca F, et al. Curcumin, hormesis and the nervous system. Nutrients. (2019) 11:2417. doi: 10.3390/nu11102417
75. Zhao YN, Li WF, Li F, Zhang Z, Dai YD, Xu AL, et al. Resveratrol improves learning and memory in normally aged mice through microRNA-CREB pathway. Biochem Biophys Res Commun. (2013) 435:597–602. doi: 10.1016/j.bbrc.2013.05.025
76. Fujii Y, Suhara Y, Sukikara Y, Teshima T, Hirota Y, Yoshimura K, et al. Elucidation of the interaction between flavan-3-ols and bovine serum albumin and its effect on their in-vitro cytotoxicity. Molecules. (2019) 24:3667. doi: 10.3390/molecules24203667
77. Martín MA, Goya L, de Pascual-Teresa S. Effect of cocoa and cocoa products on cognitive performance in young adults. Nutrients. (2020) 12:3691. doi: 10.3390/nu12123691
78. Leri M, Scuto M, Ontario ML, Calabrese V, Calabrese EJ, Bucciantini M, et al. Healthy effects of plant polyphenols: molecular mechanisms. Int J Mol Sci. (2020) 21:1250. doi: 10.3390/ijms21041250
79. Saito A, Inagawa K, Ebe R, Fukase S, Horikoshi Y, Shibata M, et al. Onset of a hypotensive effect following ingestion of flavan 3-ols involved in the activation of adrenergic receptors. Free Radic Biol Med. (2016) 99:584–92. doi: 10.1016/j.freeradbiomed.2016.09.008
80. Koizumi R, Fushimi T, Sato Y, Fujii Y, Sato H, Osakabe N. Relationship between hemodynamic alteration and sympathetic nerve activation following a single oral dose of cinnamtannin A2. Free Radic Res. (2020) 55:491–8. doi: 10.1080/10715762.2020.1759805
81. Nakagawa Y, Ishimura K, Oya S, Kamino M, Fujii Y, Nanba F, et al. Comparison of the sympathetic stimulatory abilities of B-type procyanidins based on induction of uncoupling protein-1 in brown adipose tissue (BAT) and increased plasma catecholamine (CA) in mice. PLoS One. (2018) 13:e0201203. doi: 10.1371/journal.pone.0201203
82. Osakabe N, Terao J. Possible mechanisms of postprandial physiological alterations following flavan 3-ol ingestion. Nutr Rev. (2018) 76:174–86. doi: 10.1093/nutrit/nux070
83. Calabrese EJ. An assessment of anxiolytic drug screening tests: hormetic dose responses predominate. Crit Rev Toxicol. (2008) 38:489–542. doi: 10.1080/10408440802014238
84. Calabrese EJ. Alzheimer’s disease drugs: an application of the hormetic dose-response model. Crit Rev Toxicol. (2008) 38:419–51. doi: 10.1080/10408440802003991
85. Ingawa K, Aruga N, Matsumura Y, Shibata M, Osakabe N. Alteration of the systemic and microcirculation by a single oral dose of flavan-3-ols. PLoS One. (2014) 9:e94853. doi: 10.1371/journal.pone.0094853
86. Sear JW. 26-Antihypertensive drugs and vasodilators. In: HC Hemmings, TD Egan editors. Pharmacology and Physiology for Anesthesia (Second Edition). Philadelphia: Elsevier (2019). p. 535–55.
87. Grassi G. Assessment of sympathetic cardiovascular drive in human hypertension: achievements and perspectives. Hypertension. (2009) 54:690–7. doi: 10.1161/hypertensionaha.108.119883
88. Bartness TJ, Vaughan CH, Song CK. Sympathetic and sensory innervation of brown adipose tissue. Int J Obes. (2010) 34, Suppl. 1:S36–42. doi: 10.1038/ijo.2010.182
89. Kamio N, Suzuki T, Watanabe Y, Suhara Y, Osakabe N. A single oral dose of flavan-3-ols enhances energy expenditure by sympathetic nerve stimulation in mice. Free Radic Biol Med. (2015) 91:256–63. doi: 10.1016/j.freeradbiomed.2015.12.030
90. Morrison SF, Madden CJ, Tupone D. Central control of brown adipose tissue thermogenesis. Front Endocrinol. (2012) 3:5. doi: 10.3389/fendo.2012.00005
91. Madden CJ, Tupone D, Cano G, Morrison SF. α2 Adrenergic receptor-mediated inhibition of thermogenesis. J Neurosci. (2013) 33:2017–28. doi: 10.1523/jneurosci.4701-12.2013
92. Langer SZ. Presynaptic regulation of catecholamine release. Biochem Pharmacol. (1974) 23:1793–800. doi: 10.1016/0006-2952(74)90187-7
93. Starke K. Presynaptic autoreceptors in the third decade: focus on alpha2-adrenoceptors. J Neurochem. (2001) 78:685–93. doi: 10.1046/j.1471-4159.2001.00484.x
94. Trendelenburg AU, Philipp M, Meyer A, Klebroff W, Hein L, Starke K. All three alpha2-adrenoceptor types serve as autoreceptors in postganglionic sympathetic neurons. Naunyn Schmiedebergs Arch Pharmacol. (2003) 368:504–12. doi: 10.1007/s00210-003-0829-x
95. Kurnik D, Muszkat M, Li C, Sofowora GG, Solus J, Xie HG, et al. Variations in the alpha2A-adrenergic receptor gene and their functional effects. Clin Pharmacol Ther. (2006) 79:173–85. doi: 10.1016/j.clpt.2005.10.006
96. Godoy LD, Rossignoli MT, Delfino-Pereira P, Garcia-Cairasco N, de Lima Umeoka EHA. Comprehensive overview on stress neurobiology: basic concepts and clinical implications. Front Behav Neurosci. (2018) 12:127. doi: 10.3389/fnbeh.2018.00127
97. Ulrich-Lai YM, Herman JP. Neural regulation of endocrine and autonomic stress responses. Nat Rev Neurosci. (2009) 10:397–409. doi: 10.1038/nrn2647
98. Sawchenko PE, Brown ER, Chan RK, Ericsson A, Li HY, Roland BL, et al. The paraventricular nucleus of the hypothalamus and the functional neuroanatomy of visceromotor responses to stress. Prog Brain Res. (1996) 107:201–22. doi: 10.1016/s0079-6123(08)61866-x
99. Seeman TE, Singer B, Wilkinson CW, Bruce M. Gender differences in age-related changes in HPA axis reactivity. Psychoneuroendocrinology. (2001) 26:225–40. doi: 10.1016/S0306-4530(00)00043-3
100. Finsterwald C, Alberini CM. Stress and glucocorticoid receptor-dependent mechanisms in long-term memory: from adaptive responses to psychopathologies. Neurobiol Learn Mem. (2014) 112:17–29. doi: 10.1016/j.nlm.2013.09.017
101. Fujii Y, Suzuki K, Hasegawa Y, Nanba F, Toda T, Adachi T, et al. Single oral administration of flavan 3-ols induces stress responses monitored with stress hormone elevations in the plasma and paraventricular nucleus. Neuroscience Lett. (2018) 682:106–11. doi: 10.1016/j.neulet.2018.06.015
102. Fujii Y, Suzuki K, Adachi T, Taira S, Osakabe N. Corticotropin-releasing hormone is significantly upregulated in the mouse paraventricular nucleus following a single oral dose of cinnamtannin A2 as an (-)-epicatechin tetramer. J Clin Biochem Nutr. (2019) 65:29–33. 103, doi: 10.3164/jcbn.19-19
Keywords: B-type procyanidin, hormesis, sympathetic nervous system (SNS), central nervous system, hemodynamics, stress
Citation: Osakabe N, Fushimi T and Fujii Y (2022) Hormetic response to B-type procyanidin ingestion involves stress-related neuromodulation via the gut-brain axis: Preclinical and clinical observations. Front. Nutr. 9:969823. doi: 10.3389/fnut.2022.969823
Received: 15 June 2022; Accepted: 22 August 2022;
Published: 07 September 2022.
Edited by:
Cátia Vaz, University of Beira Interior, PortugalReviewed by:
Halyna Semchyshyn, Vasyl Stefanyk Precarpathian National University, UkraineCopyright © 2022 Osakabe, Fushimi and Fujii. This is an open-access article distributed under the terms of the Creative Commons Attribution License (CC BY). The use, distribution or reproduction in other forums is permitted, provided the original author(s) and the copyright owner(s) are credited and that the original publication in this journal is cited, in accordance with accepted academic practice. No use, distribution or reproduction is permitted which does not comply with these terms.
*Correspondence: Naomi Osakabe, bmFvLW9zYUBzaGliYXVyYS1pdC5hYy5qcA==