- Beijing Advanced Innovation Center for Food Nutrition and Human Health, School of Food and Health, Beijing Higher Institution Engineering Research Center of Food Additives and Ingredients, Beijing Technology and Business University, Beijing, China
Heat processed beef flavor (HPBF) is a common thermal process flavoring, whose flavor properties can be affected by lipid oxidation during storage. Addition of antioxidants is an option to avoid the changes of HPBF induced by lipid oxidation. In this study, the effects of three antioxidants, tert-butylhydroquinone (TBHQ), tea polyphenol (TP), and L-ascorbyl palmitate (L-AP), on volatile components, physicochemical properties, and antioxidant activities of HPBF were studied over 168 days at different temperatures (4, 20, and 50°C). Although all three antioxidants had little effect on browning, acidity, water activity, and secondary lipid oxidation products, L-AP and TBHQ showed greater capabilities to prevent the formation of primary lipid oxidation products than TP. According to the results of oxidation reduction potential and DPPH radical scavenging experiments, TBHQ had better antioxidant ability compared to L-AP and TP during the storage. Of note, TBHQ affected the flavor profiles of HPBF, mainly on volatile odorants produced by lipid degradation. TBHQ could mitigate the development of unfavorable odorants. This study indicated TBHQ would enhance lipid oxidation stability and maintain physicochemical properties and flavor profiles of HPBF during storage. It suggested that TBHQ could be applied as an alternative additive to improve the quality of HPBF related thermal process flavorings.
Introduction
Heat processed beef flavor (HPBF) is known as one of the “thermal process” flavorings produced by heating a mixture of two or more precursor materials (1). A major purpose of “thermal process” flavorings is to enhance the characteristic meaty note of foods (2, 3). However, the negative changes in quality during storage might be affected by storage time and temperature. It is well-known that the Maillard reaction and lipid oxidation are of the utmost importance for the development of meaty note, which occur as the main processes during thermal treatment and storage (4). Lipids determine the flavor properties of products with a meaty note (5, 6). Lipid oxidation products give generally fatty and meaty notes which determines the aroma differences between meats from different species (7). Lipid-derived compounds, such as certain aldehydes with higher odor detection threshold values, generally have a higher contribution to overall favor profiles than the sulfur or nitrogen heterocyclic compounds formed through the Maillard reaction (5, 8).
The quality changes caused by lipid oxidation reactions are more prominent than those caused by reactions with other precursors (9, 10). All lipid-containing products, even those with minimal unsaturated fatty acid contents, have essentially the potential ability to undergo lipid oxidation in highly processing or during prolonged storage (11). Fatty acids presented in animal meats, especially polyunsaturated fatty acids increase the risk of oxidation reaction, leading to undesirable flavors (12). Radical species produced by lipid oxidation may have an unfavorable effect on the physicochemical properties, such as browning (13). Thus, appropriate evaluation and control of lipid oxidation are controversial issues.
Antioxidants have been employed to improve food quality by preventing lipid oxidation (14). Tert-butylhydroquinone (TBHQ), L-ascorbyl palmitate (L-AP), and tea polyphenol (TP) are the most commonly used antioxidants in the current relevant Chinese national standard GB-2760 (15). These antioxidants have the potential ability to prevent lipid oxidation in meat-based products and maintain the flavor stability of food (16). Lipid-soluble TBHQ as one of the synthetic antioxidants has been widely utilized to prevent lipid oxidation (17). Even the addition of TP, a water-soluble antioxidant, could inhibit the lipid oxidation of sausages made of meat (18), which has shown antioxidant performance comparable to TBHQ (19). L-AP as one of the intermediate polarity antioxidants provided antioxidant protection comparable to TBHQ during a long-term storage of flaxseed oil (20).
However, the effects of antioxidants with different solubility on the performance and flavor properties of the water-oil mixtures are controversial (21, 22). Frankel et al. (23) reported that the antioxidant activity of TBHQ was higher than that of L-AP in the emulsion system, which could mitigate flavor deterioration. This finding by Frankel et al. (23) is consistent with the results obtained by Gordon and Kourkimskå (24) in deep-fried rapeseed oil (as with bulk oils). This could be due to the fact that lipophilic antioxidants are more potent in emulsions than in bulk oils (considered water-in-oil nanoemulsions) (25). In contrast, Wanasundara and Shahidi (26) found that TBHQ and butylated hydroxyanisole (BHA) were not as effective as catechins (the primary component of TP) in preventing the oxidation of seal blubber or menhaden oil, as well as the odor and flavor of lipid-containing foods. Zhang et al. (27) reported that polyphenolic antioxidants (e.g., TP) can effectively alleviate lipid oxidation while also enhancing unpleasant odors due to their astringency. Consequently, it is still challengeable to control lipid oxidation and improve aroma profiles in parallel.
Hence, it is necessary to obtain the optimum antioxidant to control lipid oxidation and mitigate flavor deterioration of HPBF within a water-oil mixture during the storage. To achieve this purpose, TBHQ, TP, and L-AP were added into HPBF as lipid soluble, water soluble, and intermediate polarity antioxidants respectively. A potential antioxidant was determined by evaluating the effects of three antioxidants on lipid oxidative stability, physicochemical properties, and volatile components of HPBF. The purpose of this study was to provide a basis for the development of thermal process flavorings with high quality.
Materials and methods
Materials and reagents
Amino acids (glycine, cysteine), monosaccharide (glucose, xylose), Protamex (120 U/mg), and Flavourzyme (20 U/mg) were obtained from Shanghai Yuanye Biotechnology Co., Ltd. (Shanghai, China). Yeast extract was purchased by Beijing Aoboxing Biotech Co., Ltd. (Beijing, China). Spices (including clove, cinnamon, and fennel), fresh beef lean meat, fresh onion, garlic, and ginger were purchased from the local supermarket.
Reagents used in the study are listed as follows: isooctane, isopropyl alcohol, potassium thiocyanate, ferrous chloride anhydrous, ethanol, and butanol were purchased from Fuchen Chemical Reagent Co., Ltd. (Tianjin, China). Trichloroacetic acid (TCA) and 2-thiobarbituric acid (TBA, biochemical reagent, purity ≥ 98.5%) were obtained from Sinopharm Chemical Reagent Co., Ltd. (Beijing, China). Ethylene diamine tetraacetic acid (EDTA) was provided by Beijing Biotopped Science and Technology Co., Ltd. (Beijing, China). 1,1-Diphenyl-2-picrylhydrazyl (DPPH) was purchased from Shanghai Yuanye Biotechnology Co., Ltd. (Shanghai, China). Tert-butylhydroquinone (TBHQ), tea polyphenols (TP), L-ascorbyl palmitate (L-AP), and 1,1,3,3-tetraethoxy propane were purchased from Shanghai Macklin Biochemistry Co., Ltd. (Shanghai, China). 2-Methyl-3-heptanone and n-alkane were purchased by Sigma-Aldrich (Shanghai, China). Methanol [purity ≥ 99.9%, Mreda Technology Inc. (United States)], and ammonium acetate (purity ≥ 98.7%, Thermo Fisher Scientific, Inc.) were of HPLC grade. In addition, n-hexane (purity ≥ 99.9%) of GC grade was purchased from Mreda Technology Inc. (United States). Distilled-deionized water used in all experiments was purified using a Milli-Q Gradient (Millipore, Bedford, MA, United States). Helium gas (99.9992% purity) was provided by Beijing Tianlirenhe Material Trade Co. Ltd. (Beijing, China).
Samples preparation
The HPBF samples (control) was prepared by mixing of an enzymatic hydrolysate and other ingredients. To prepare the enzymatic hydrolysate, the fresh beef lean meat was trimmed of its fat and connective tissue and then crushed prior to the experiment. The meat paste was hydrolyzed by 0.21 g of Protamex (120 U/mg) and 0.42 g of Flavourzyme (20 U/mg) for 60 and 240 min, respectively. After cooling of the above enzymatic hydrolysate, the ingredients of HPBF were weighed according to the proportion of ingredients including 21.0% bovine bone, 2.7% yeast extract, 0.3% glycine, 0.3% cysteine, 0.9% glucose, 0.3% xylose, 4.8% fresh onion, 1.9% garlic, 0.9% ginger, and 0.076% spice powder. Those ingredients of HPBF were completely mixed with the enzymatic hydrolysate before being placed in a steam sterilization pot at 115°C for 60 min. In the treatment group, TBHQ, TP, and L-AP were added at a level of 0.02% based on fat content.
The sterilized HPBF was filtered and filled into 40 mL glass vials (Supelco, United States) sealed with screwed top PTFE/silicone septa. The HPBF samples were stored at 4, 20 and 50°C, respectively. Among them, 4°C represents low temperature and 20°C represents room temperature. In addition to accelerate the storage, the temperature of 50°C also applied and represents the high temperature conditions. The relevant physicochemical characteristics and odorants were determined for 168 days including a total of 17 sets (1, 7, 14, 21, 28, and so on up to 168 days).
Oxidative stability
Oxidation reduction potential
Oxidation reduction potential (ORP) was measured using a calibrated hand-held ORP Electrode LE501 [Mettler Toledo International Trading (Shanghai) Co., Ltd., Shanghai, China]. The ORP readings (mV) was determined by the method described in Capuani et al. (28) with minor modifications. The redox electrode was inserted into the HPBF immediately after leaving the electrolyte solution of 3 M KCl in order to minimize the effect of air. The redox electrode was checked using a redox buffer solution of 220 mV/pH 7 from Mettler Toledo before each use.
Peroxide value
Lipid peroxides were quantitated using a modified method by Chaiyasit et al. (29). The original sample (1 mL) and 5 mL of isooctane-isopropyl alcohol (2:1, v/v) solution were stirred by eddy current for 30 s, and then centrifugation at 9000 rpm for 5 min. The clear upper layer (2 mL) was mixed thoroughly with 20 μL of potassium thiocyanate solution and 20 μL of ferrous chloride solution (0.144 M). The mixture was mixed with butanol-methanol (v/v, 2:1) solution to obtain a 5 mL mixed solution. Absorbance was measured at 510 nm after incubation away from light for 20 min at room temperature. A standard curve made up of diluted ferrous chloride solutions was used to calculate lipid peroxide concentrations in the HPBF. The results of PV value were expressed as the uptake of mEq of active oxygen per kg of lipid (mEq/kg).
Thiobarbituric acid reactive substances
The TBARS assay was performed according to a previously method described by Papastergiadis et al. (30) with slight adjustments. Briefly, 1 g HPBF was mixed with a total of 5 mL of 7.5% TCA (w/v) with 0.02% (w/v) of EDTA. The mixed solution was homogenized with a refrigerated centrifuge (Hunan Herexi Instrument and Equipment Co., Ltd., Changsha, China) for 10 min at 8000 rpm. The homogenate was treated with 5 mL TBA reagent (20 mM) at 90°C for 30 min, and then filtered through a 0.22 Millipore membrane filter (MREDA Technology Inc., Beijing, China) after cooled using running water. Ten μL mixture solution was separated by Waters 2695 HPLC on a Waters SunFireTM C18 column (4.6 × 250 mm, 5 μm) using a mobile phase of 10 mM ammonium acetate and methanol (7:3, v/v). The column temperature was 30°C. The detection wavelength was 532 nm using Waters 2996 photodiode array detector (PAD). A standard curve made up of diluted 1,1,3,3-tetraethoxy propane solutions was used to calculate the amount of TBARS. The results were given in milligrams of MDA equivalents per kilogram of the sample (mg MAD/kg).
1,1-Diphenyl-2-picrylhydrazyl radical scavenging activity
The antioxidant activity in the DPPH assay was performed in the manner of the descriptions given by previous reports (31) with minor modifications. A dilution of 1 mL HPBF (diluted 500 times with deionized water) was thoroughly mixed with 3 mL of DPPH ethanol solution (0.1 mM). The mixture was incubated at room temperature for 30 min in the dark. The ethanol control group was added as a blank for DPPH. An ultraviolet-visible spectrophotometer (UV-1240, Shimadzu, Co., Ltd., Tokyo, Japan) was used to measure the absorbance at 517 nm to estimate the radical scavenging capacity of antioxidant samples at each storage time.
Physicochemical characteristics evaluation
Browning determination
Browning degree was linked with the absorbance at 420 nm (32). Non-enzymatic browning was monitored using the ultraviolet-visible spectrophotometer (UV-1240, Shimadzu, Co., Ltd., Tokyo, Japan) against water at 420 nm.
pH level determination
The pH was measured by using a calibrated hand-held pH meter [TB-214, Mettler Toledo International Trading (Shanghai) Co., Ltd., Shanghai, China]. The pH was calibrated using pH 4, pH 6.86, and pH 9.18 calibration buffers.
Water activity (aw) determination
The water activity (aw) was measured using an AquaLab 4TEV water activity meter in capacitance mode after samples reached equilibrium at 25°C.
Volatile compounds analysis
Extraction of volatiles by HS-SPME
Volatile compounds of HPBF were analyzed by means of solid-phase microextraction-Gas chromatography/Mass spectrometry (SPME-GC/MS) with a DB-WAX column (30 m, 0.25 mm i.d., 0.25 μm film thickness, Agilent). For each sample, 10 mL of HPBF were added to a 40 mL headspace bottle (Supelco, United States) containing 1 μL of 2-methyl-3-heptanone (diluted by a factor of 1000 in hexane) as the internal standard, and allowed to stand for 30 min at 45°C. The DVB/carboxen/PDMS fiber (Supelco, United States) was then exposed to the headspace of HPBF for 30 min while the vial was maintained at 45°C, and desorbed at 250°C for 5 min in the injection port after headspace collection. The experiments were performed in triplicate.
GC-MS analysis
GC-MS analyses were performed on an Agilent 7890B gas chromatography coupled to an Agilent 5977A mass spectrometer. The DVB/carboxen/PDMS fiber was desorbed in splitless mode with a splitless inlet liner of 0.75 mm inlet diameter (Agilent) suitable at 250°C for SPME analysis. The mass spectrometer was operated in electron impact mode with the electron energy set at 70 eV to obtain the mass spectra. A mass scan from m/z 35 to m/z 400 was performed with the ion source at 230°C. Helium was used as the carrier gas at a rate of 1 mL/min. The oven program started at 40°C for 2 min, then increased at 4°C/min to 190°C, and subsequently to 240°C at 8°C/min.
Isolation and identification of the volatiles
Volatile compounds were identified by comparing mass spectrometry patterns from the NIST14 database with linear retention indices (RI) based on a homologous series of even numbered n-alkanes (C7-C40). A semi-quantitative analysis of the detected volatile compounds was performed based upon comparison of their GC-MS peak regions to the internal standard (2-methyl-3-heptanone).
An Agilent 7890B gas chromatography coupled to a sniffing port ODP-3 from Gerstel was employed to analyze HPBF under the same analytical conditions. Volatile odorants were sniffed by trained panelists (two females and one male) on the top of the sniffing port and were used for the PCA analysis.
Statistical analysis
The least significant difference among different samples was analyzed using the one-way analysis of variance (ANOVA) with SPSS Statistics 22.0 (IBM Corporation, New York, NY, United States). All analyses for significant differences set at a 5% significance level. Statistical analysis each experiment was independently triplicated to derive an average and standard deviation. The data were subjected to principal component analysis (PCA) and illustrated using Origin 2021b (OriginLab Corporation, Northampton, MA, United States).
Results and discussion
Oxidative stability analysis
Oxidation reduction potential
Oxidation reduction potential is a measure of a chemical species to acquire electrons and thereby be reduced. As shown in Supplementary Figures 1A–C, the ORP values increased steadily in all treatments (TBHQ, TP, and L-AP) with the increasing storage time. The results showed that lipids in HPBF were continuously oxidized during storage. Besides, three antioxidants (TBHQ, TP, and L-AP) had only a small effect on the potential redox of HPBF during storage. There was no significant difference (p > 0.05) between the treatment groups (TP or L-AP) and control. An addition of TBHQ in HPBF decreased potential redox values after 98 days compared to the control at a lower temperature (4°C). This suggested that the antioxidant effect of TBHQ in HPBF was superior to that of TP and L-AP at 4°C. As a results, TBHQ may be a potent antioxidant that produce an additive protective effect during storage, inhibiting the oxidative degradation of lipids.
Peroxide value
Peroxide value (PV) was quantified by measuring the concentration of lipid peroxides, which is normally considered as the product of primary lipid oxidation (33). As shown in Figure 1, the three antioxidants (TBHQ, TP, L-AP), as well as storage period, have a significant (p < 0.05) effect on the PV of HPBF. PV increased significantly (p < 0.05) both in the control and treated samples during the storage. The increased PV value suggested that the number of lipid peroxides produced by lipid oxidative processes increased gradually with time. A distinct drop before 28 days may be due to the unstable decomposition of primary lipid oxidation products into shorter chain hydrocarbon (34).
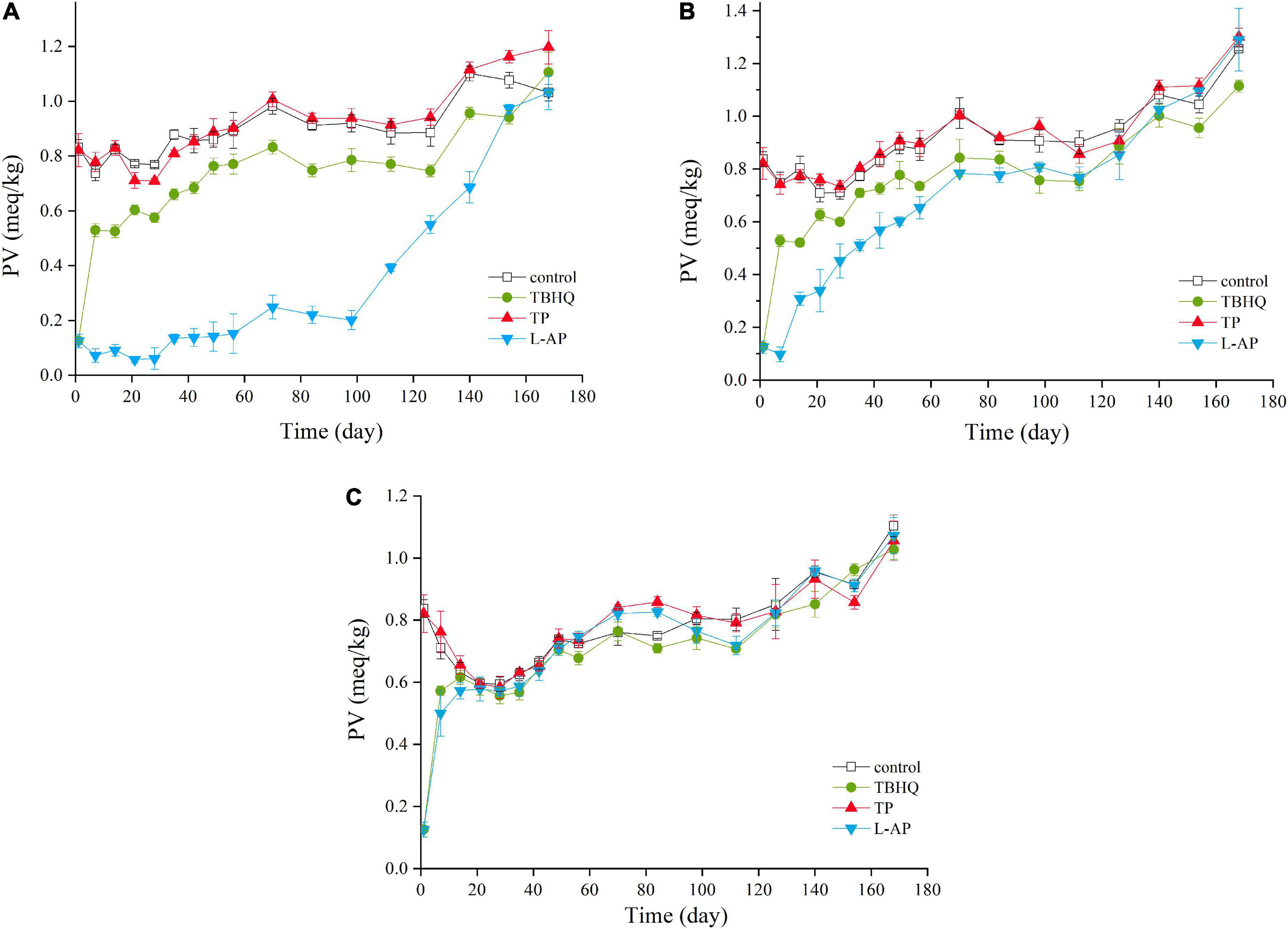
Figure 1. PV values of HPBF with the addition of TBHQ, TP, and L-AP during the storage display at different temperature [(A) 4°C; (B) 20°C; (C) 50°C]. The color discrimination is to distinguish four groups including control test, TBHQ, TP, and L-AP.
In the initial stage of storage, the PV of the L-AP treatment group was lower than that of the control group at any storage temperatures, followed by the TBHQ treatment group and TP treatment group. There was no clear distinction between the control group and the TP treatment group. The results indicated that TBHQ, followed by L-AP, had an antioxidant effect on primary lipid oxidation products for HPBF during storage. According to the findings of Wang et al. (35), both TBHQ and L-AP showed a significant (p < 0.05) antioxidant effect, which effectively retarded the oxidative degradation of polyunsaturated fatty acids. However, the antioxidant activity of TBHQ was better than that of L-AP in oil and fat, which was different from our results. This could be as a consequence that L-AP with compatible properties had a better antioxidant activity than TBHQ for a water-oil mixture of the HPBF. Besides, the antioxidant effect of TP was lower than TBHQ. A similar effect of TBHQ and TP was observed by Rababah et al. (36) who confirmed that TBHQ was the most effective in preventing lipid oxidation in cooked chicken, followed by TP. Although TBHQ had a higher lipid solubility than TP and L-AP, it was clear that L-AP had the best antioxidant impact on primary oxidation products of HPBF among three antioxidants in the study, followed by TBHQ.
Thiobarbituric acid reactive substance value
Thiobarbituric acid reactive substance are used as an estimate of the concentration of secondary lipid oxidation products and the results of TBARS are shown in Supplementary Figures 1D–F for HPBF with different treatments (TBHQ, TP, L-AP). As shown in Supplementary Figures 1D–F, TBARS values of HPBF are significantly different between storage temperatures during storage. TBARS values increased faster at a higher temperature (50°C) compared to that at lower temperature (4 and 20°C), which was consistent with the production of primary lipid oxidation (PV value) as mentioned above. Interestingly, TBARS values gradually increased during the first 14 days at 50°C and then decreased during the following stages. The decrease of TBARS values with time was attributed to the advanced reactions of secondary lipid oxidation products with protein residues (33). The result of TBARS increased and then decreased during storage, which have previously been reported by Koutina et al. (33) who explained that interesting observations were a consequence of secondary lipid oxidation products being consumed by proteins to produce oxidized modified proteins.
In contrast to our suspicion, there were no variations in lipid oxidation (p > 0.05) among the three control treatments at any storage temperatures. It demonstrated that the three antioxidants had a limited ability to inhibit secondary lipid oxidation products in HPBF during the storage. A similar finding was reported by Jin et al. (37) who affirmed that TBHQ or L-AP alone did not show many protective properties on heat-treated corn oil with the TBARS assay.
1,1-Diphenyl-2-picrylhydrazyl scavenging activity
The assay of DPPH scavenging activity is a simple method to assess the ability of antioxidants to trap free radicals (38). The DPPH scavenging activity is related to the delocalization of the unpaired electron throughout the molecule, such as peroxide-free radical, hydroxyl radicals and reactive oxygen species (38). The antioxidant effectiveness of TBHQ, TP, and L-AP evaluated in HPBF during the storage are shown in Figure 2. Based on the results in Figure 2, the DPPH scavenging ability increased first and then decreased during storage. One unanticipated discovery was that the values of DPPH scavenging ability were always the largest in the storage condition of 50°C. This was mainly owing to the ease with the Maillard reaction that occurs as the temperature rises. The Maillard reaction products had an effective antioxidant effect, which resulted in enhanced DPPH scavenging ability through the conversion of DPPH into a stable DPPH-H form (39, 40). The values gradually decreased and tended to be flat as storage time increased indicating that the oxidation reaction of HPBF was caused by the consumption of antioxidants. These findings would be confirmed by the results of oxidative stability analysis.
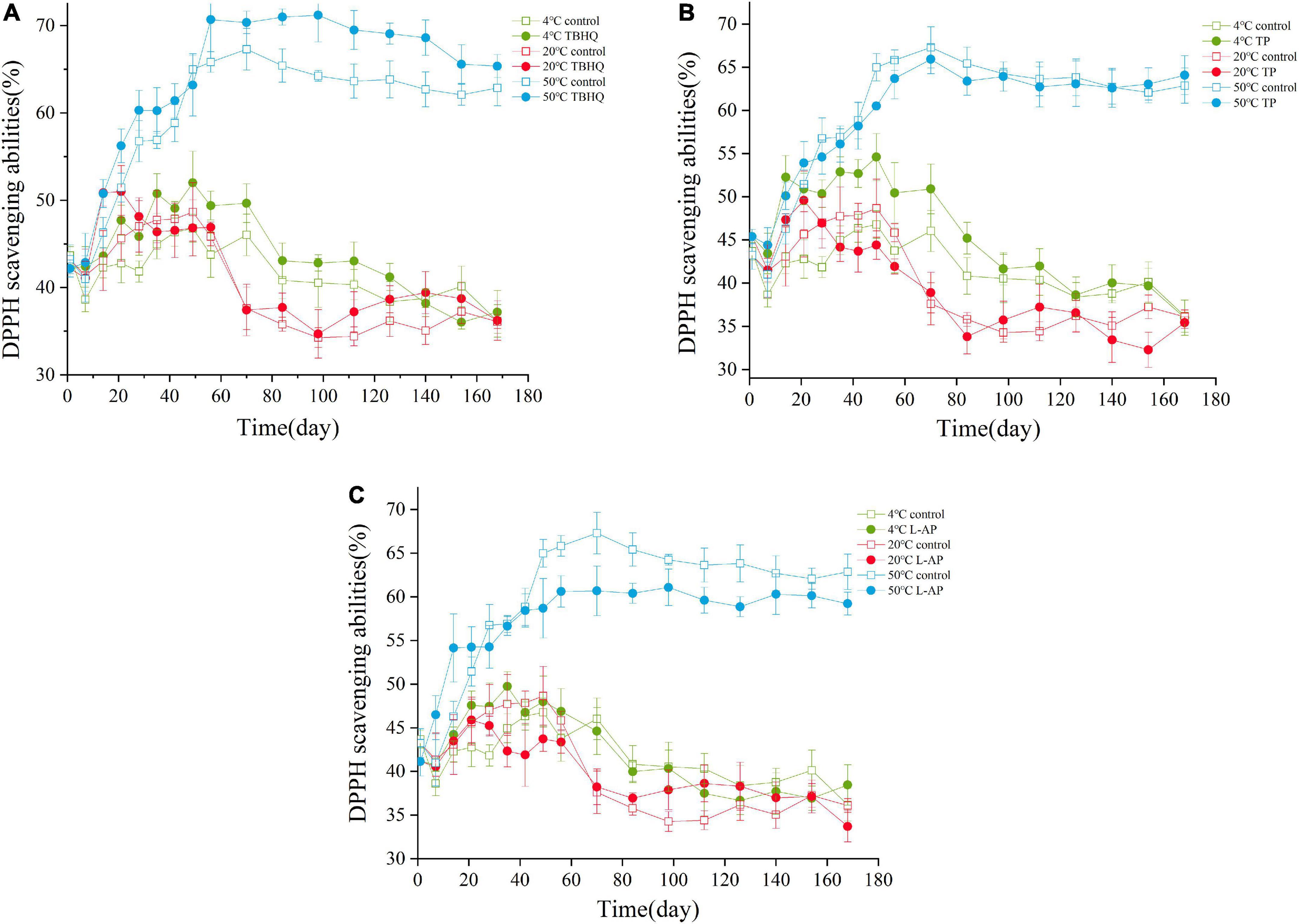
Figure 2. Levels of DPPH during the storage of HPBF with the addition of TBHQ (A), TP (B), and L-AP (C). The discrimination of the shape is shown to distinguish the control group and the treatment groups. The color discrimination represents various temperature (4, 20, and 50°C).
Compared with the control group, the addition of TBHQ, TP, and L-AP in HPBF could efficiently scavenge DPPH radicals and inhibit oil oxidation after being stored at a low temperature (4°C) for a short period. There was no significant difference in free radical scavenging between the three treatment groups at room temperature (20°C). TBHQ had an effective DPPH scavenging ability at higher temperatures (50°C), which indicted that its antioxidant impact was substantially greater than that of TP and L-AP. The results were consistent with previous research findings reported by Jin (41). TBHQ as one outstanding synthetic antioxidant reported by Liang et al. (42) who found that TBHQ can effectively inhibit the formation of free radicals and hence contributes to the stabilization of lipids. More unexpectedly, HPBF with the addition of L-AP (as another synthetic antioxidant), exhibited a lower DPPH scavenging activity than that of the control group at a higher storage temperature (50°C). This could be associated with the initial acidity of HPBF or the stability and structure of L-AP. Under acidic conditions, L-AP could be entirely hydrolyzed to ascorbic acid and their respective fatty acids in the reporter of EFSA and ANS (43), which affect its own free radical scavenging ability. Comparatively, TBHQ had a noticeable effect on scavenging free radicals terminating the free radical chain reaction, which could be attributed to its molecular structure, high thermal resistance and stability.
Evaluation of physicochemical profiles
Browning
Browning is one of the most common natural phenomena during the processing and storage of food (44). As shown in Supplementary Figures 1G–I, the brown color (A420) of samples increased over time, particularly when stored at 50°C; A420 values of samples stored at 50°C were higher compared to that of samples stored at 4 and 20°C at each time point during storage. The results suggested that Maillard browning depended greatly on temperature and time. However, it was worth noting that the growing trend of browning in the three treatment groups (TBHQ, TP, and L-AP) did not differ substantially. The similarity of A420 values between TBHQ, TP, and L-AP groups indicated that the addition of antioxidants had little effect on the browning of HPBF during storage. In fact, products of the Maillard browning reaction during storage were also regarded as antioxidants and could interfere with the antioxidant effect from TBHQ, TP, and L-AP (45, 46). For example, Mshayisa (47) revealed that Maillard reaction products showed higher antioxidant capacity than TBHQ (0.02% w/w) according to the TBARs assay in a glucose-casein model system. As also demonstrated by Kirigaya et al. (48), melanoidin pigment, a component of Maillard browning, played an important role in the antioxidant activity. Accordingly, it may be suspected that the impact of three antioxidants on browning was much lower than that of Maillard reaction products during the storage.
pH
An examination of the acidity of HPBF with three antioxidants (TBHQ, TP, L-AP) treatment during storage (Supplementary Figures 2A–C) indicated that TBHQ, TP and L-AP did not alter (p > 0.05) the acidity values, except after 112 days during the low temperature (4°C) storage. TBHQ, TP, and L-AP may be able to limit the hydrolytic and oxidative rancidity of lipid to a certain extent in the long term at low temperatures. However, the activities of three antioxidants to control the change of acidity value was not significantly different (p > 0.05).
Water activity
Water activity (aw) is another important parameter to evaluate the physicochemical quality that governs storage stability (49). The effects of three antioxidants (TBHQ, TP, L-AP) on HPBF are shown in Supplementary Figures 2D–F. Based on the results, the aw values of the TBHQ and L-AP groups were similar at all temperatures. The aw of the HPBF samples in either TBHQ group or L-AP group equilibrated to the control indicating that the addition TBHQ or L-AP had little effect on the aw of HPBF during storage. However, the TP group exhibited higher aw values than other treatment groups and the control at both low (4°C) and room (20°C) temperature after 14 days of storage. No distinguished difference in aw was found between the three treatment groups and the control at 50°C. The HPBF samples with the addition of TP or stored at 50°C may increase the value of aw, which may adversely affect the physicochemical profiles and stability of the HPBF samples.
Analysis of volatility odorants
Lipid oxidation rancidity affects flavor quality. It was attributed to the results that TBHQ had a better effect on controlling lipid oxidation than TP and L-AP, based on the evaluation of lipid oxidation stability and physicochemical profiles. The results of volatile odorants detected by SPME-GC-MS only discussed the effect of TBHQ on flavor profiles during storage. Volatile flavor compounds in HPBF were detected during storage (Table 1). As shown in Table 1, alcohols, terpenes, and terpenoids, nitrogenous heterocyclic compounds (thiophenes, thiazoles, pyrazines, pyrrole, and pyrimidines) and sulfur compounds accounted for a large percentage of volatility odorants. The concentration and amount of volatile odorants in HPBF altered with the storage environment, which was depicted in Figures 3A–D. The concentration of volatile components in HPBF did not exhibit significant changes at different temperatures (4 and 20°C). All of alcohols, acids, and sulfur compounds were significantly decreasing, when HPBF is held at 50°C. The accelerate experiment at the high temperature could give a negative impact on the total concentration of volatile chemicals. Interestingly, the concentration of aldehydes and furans in HPBF, and the number of pyrazines and sulfur compounds increased slightly with the storage time.
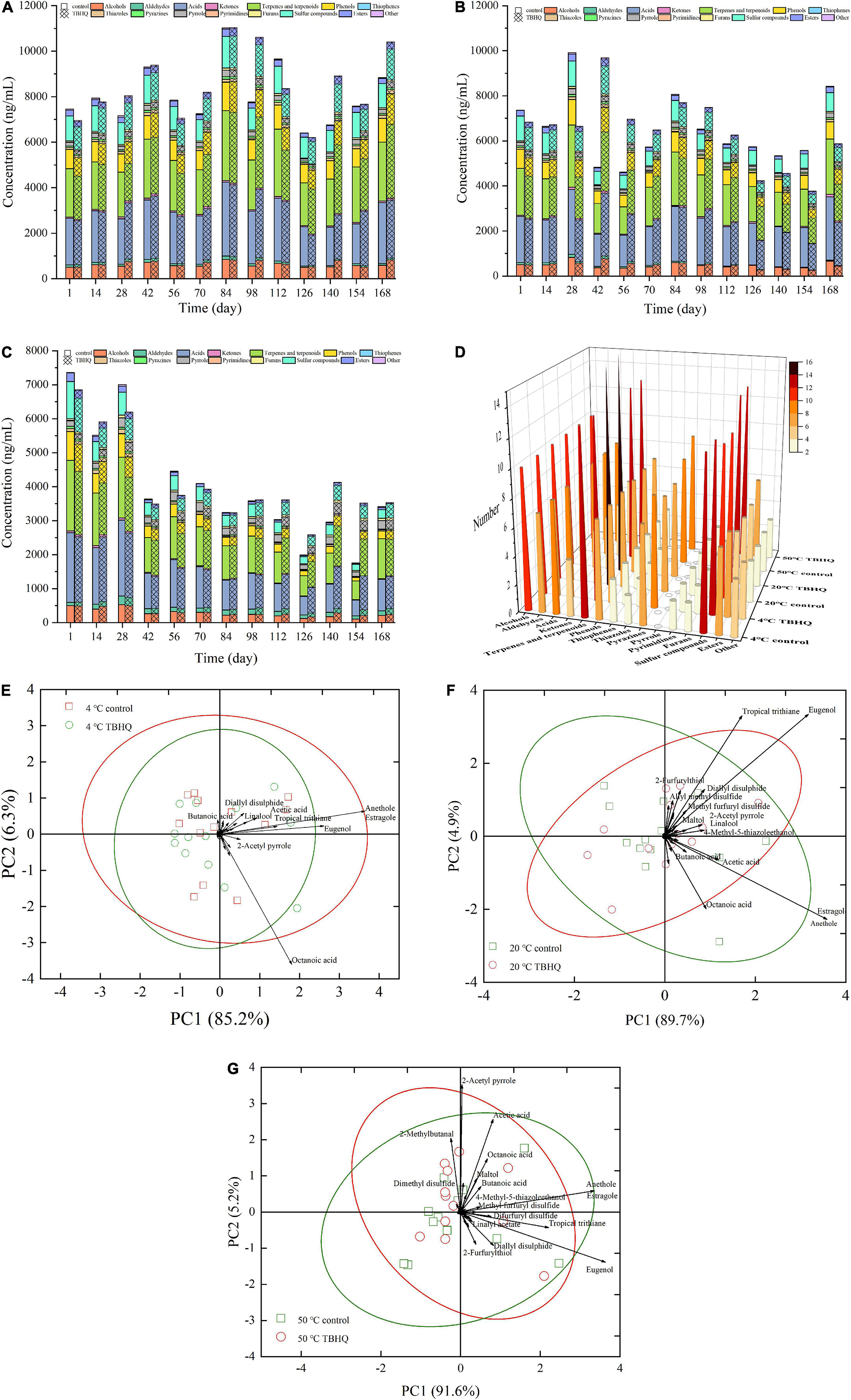
Figure 3. Effect of TBHQ on the quantity [(A) 4°C; (B) 20°C; (C) 50°C] and concentration (D) of volatile odorants in HPBF during storage. PCA diagram shows the difference of volatile odorants between TBHQ group and control test group [(E) 4°C; (F) 20°C; (G) 50°C].
The decrease of alcohols and the increase of aldehydes were reported to be due to oxidation reactions under high temperature conditions, which promoted the conversion of alcohols to aldehydes, or the generation of aldehydes attributed to lipid oxidation or thermal degradation of amino acids (50). As reported by Frank et al. (51), the increase of 2-methylbutanal was produced through thermal Strecker degradation of isoleucine. The protein oxidation and Maillard reaction were responsible for Strecker aldehydes have been reported by Zhou et al. (52) and Wen et al. (53). However, alcohols and aldehydes in the TBHQ treatment group were higher than those in the control group as storage time went on, indicating that the antioxidant impact of TBHQ was able to stabilize their reaction activity throughout storage.
Pyrazines are usually produced at higher temperatures and contribute to the roast and nutty aroma of overall flavor attributes (54). Changes in concentration and amount during storage may due to the decomposition of proteins and amino acids (55) or the generation of Maillard reaction via sugar dehydration or fragmentation (56), instead of lipid oxidation. This confirmed that TBHQ had no significant effect on pyrazines.
Sulfur compounds are considered to be essential volatile aroma active compounds due to their lower odor threshold (57). The decrease or increase of sulfur compounds had a direct effect on the overall flavor profiles of HPBF during storage. Some sulfur compounds include 2-methyl-3-furanthiol, dipropyl disulfide, 2-furfurylthiol, diallyl disulfide, tropical trithiane, methyl furfuryl disulfide, and difurfuryl disulfide decreased over the time. The reaction of lipid oxidation degradation products with H2S produced by Strecker degradation of cysteine, interfering with the reaction pathway and reducing the reactants of sulfur compounds, which resulted in a large reduction in the overall content of sulfur compounds (54). Thiols and other sulfur compounds with oxygen heterocycles were extremely reactive, susceptible to oxidation, and easily affected by the environment (58). They can degrade thermally to produce a variety of carbonyl and hydroxyl carbonyl components (59). However, an addition of TBHQ increased the total concentration of sulfur compounds during storage, which could attribute to that TBHQ was more effective in inhibiting lipid oxidation and maintaining greater oxidation stability of HPBF than control group. Notably, in the TBHQ treatment group and the control group, dimethyl disulfide and dimethyl trisulfide increased in the later stage of storage and accounted for a large proportion. These volatiles had been previously linked to meat spoilage and strong objectionable odors (51, 60).
Furans as oxygen-containing heterocyclics are major intermediate products of the Maillard reaction, mostly from sugar degradation (61). It had little correlation with fat oxidation during storage. The concentration of 2-acetylfuran (one of the oxygenated heterocyclic compounds) gradually increased with storage time. However, this odorant with a high odor threshold had little effect on the flavor profiles (62).
Generally speaking, only a few volatile components were important aroma active compounds, which contributed effectively to the overall flavor properties. A total of 51 odorants were sniffed and semi-quantified from the headspace of the HPBF during the storage (Table 1). In order to more accurately and intuitively analyze the influence of the TBHQ treatment group on volatile aroma components in HPBF, PCA applied to reveal patterns in the dataset was shown in Figures 3E–G. At 4, 20, and 50°C, the Bi-plot of the sum of first two principal components accounted for 91.5, 94.6, and 96.8% of the total variance in raw data, respectively, which explained fully the variation trend of the volatile odorants. The data points with distinct markers belonged to different treatments, according to the distribution of data mapped on the principal components. There was a hazy demarcation between HPBF samples from the blank and TBHQ treatment groups during the storage at any temperature. The importance of different volatile odorants to HPBF with different treatments could be distinguished by the visual loading matrix (the black lines). Closer inspection of Figures 3E–G revealed that clove-smelling eugenol, anise-smelling anethole, and estragole had a higher contribution value to the overall flavor attribute of HPFB during storage due to their positions far from the coordinate origin. The loading matrix of 2-furfurylthiol, diallyl disulfide, tropical trithiane, and methyl furfuryl disulfide increased were larger than that of control group with the increasing of temperature at 4 and 20°C, implying that these odorants have a higher contribution to the flavor profiles of TBHQ treatment group. 2-Methylbutanal, 2-acetyl pyrrole, and difurfuryl disulfide were more prominent in the TBHQ treatment group at 50°C. This was possible because TBHQ could maintain the oxidation stability of HPBF during the storage, owing to the antioxidant effect. The loss of some active aroma components (2-methylbutanal, 2-methyl-3-furanthiol, 2-furfurylthiol, diallyl disulfide, tropical trithiane, methyl furfuryl disulfide, and difurfuryl disulfide) in HPBF during storage was minimized in the TBHQ treatment group. It was interesting to find that the contribution value of octanoic acid gradually decreases, indicating that it was oxidized due to the influence of storage temperature and time. In short, these findings provide vital information about that several volatile odorants were affected by TBHQ contributing to the overall flavor qualities during storage. Meanwhile, these odorants were the main differences between the blank and TBHQ treatment group.
Conclusion
The effect of three antioxidants (TBHQ, TP, and L-AP) on the flavor profiles, oxidative stability and the physicochemical profiles in HPBF at different temperatures during the storage was analyzed. The results indicated that TBHQ, TP, and L-AP had a little influence on the browning, aw, and acidity of HPBF. Based on the results of an oxidative stability assessment, L-AP had a better ability to limit the production of primary oxidation products than TBHQ, although both L-AP and TBHQ exhibited poor control over secondary lipid oxidation products regardless of storage temperature. In particular, TBHQ performed better in the DPPH radical scavenging assays, inhibiting lipid oxidation during storage with a higher DPPH radical scavenging ability. According to the results of oxidative stability and physicochemical profiles, HPBF with the addition of TBHQ exhibited greater lipid stability than L-AP, followed by TP. In addition, TBHQ could inhibit high-activity compounds from being oxidized and forming other objectionable odorants. However, an addition antioxidant (i.e., TBHQ) had a lower impact on flavor qualities during storage than storage temperature and storage time. It was undeniable that TBHQ may play an antioxidant role in HPBF during storage. The developed compound additive containing TBHQ had the potential to be employed as an effective additive in a complex system of beef flavor with the capacity to postpone oxidation reactions thereby enhancing flavor quality.
Data availability statement
The original contributions presented in this study are included in the article/Supplementary material, further inquiries can be directed to the corresponding authors.
Author contributions
ZZ: data curation, methodology, writing – original draft preparation, investigation, and validation. FM: writing – review and editing and funding acquisition. BW: writing – review and editing, project administration, and funding acquisition. YC: conceptualization, supervision, and project administration. All authors contributed to the article and approved the submitted version.
Funding
This work was supported by National Natural Science Foundation of China (project no. 32072345; Beijing) and Young Teachers Scientific Research Start-up Fund of Beijing Technology and Business University (Project No: QNJJ2022-04).
Conflict of interest
The authors declare that the research was conducted in the absence of any commercial or financial relationships that could be construed as a potential conflict of interest.
Publisher’s note
All claims expressed in this article are solely those of the authors and do not necessarily represent those of their affiliated organizations, or those of the publisher, the editors and the reviewers. Any product that may be evaluated in this article, or claim that may be made by its manufacturer, is not guaranteed or endorsed by the publisher.
Supplementary material
The Supplementary Material for this article can be found online at: https://www.frontiersin.org/articles/10.3389/fnut.2022.966697/full#supplementary-material
Abbreviations
HPBF, heat processed beef flavor; TBHQ, tert-butylhydroquinone; TP, tea polyphenol; L-AP, L-ascorbyl palmitate; BHA, butylated hydroxyanisole; ORP, oxidation reduction potential; PV, peroxide value; TBARS, thiobarbituric acid reactive substances; aw, water activity; SPME-GC/MS, solid phase microextraction-gas chromatography/mass spectrometry.
References
1. Lotfy SN, Fadel HH, El-Ghorab AH, Shaheen MS. Stability of encapsulated beef-like flavourings prepared from enzymatically hydrolysed mushroom proteins with other precursors under conventional and microwave heating. Food Chem. (2015) 187:7–13. doi: 10.1016/j.foodchem.2015.04.027
2. Song S, Zhang X, Hayat K, Huang M, Liu P, Karangwa E, et al. Contribution of beef base to aroma characteristics of beeflike process flavour assessed by descriptive sensory analysis and gas chromatography olfactometry and partial least squares regression. J Chromatogr A. (2010) 1217:7788–99.
3. Cui H, Yu J, Xia S, Duhoranimana E, Huang Q, Zhang X. Improved controlled flavor formation during heat-treatment with a stable maillard reaction intermediate derived from xylose-phenylalanine. Food Chem. (2019) 271:47–53. doi: 10.1016/j.foodchem.2018.07.161
4. Wellner A, Huettl C, Henle T. Formation of maillard reaction products during heat treatment of carrots. J Agric Food Chem. (2011) 59:7992–8. doi: 10.1021/jf2013293
5. Mottram DS. Flavour formation in meat and meat products: a review. Food Chem. (1998) 62:415–24. doi: 10.1016/S0308-8146(98)00076-4
6. Flores M. The Eating Quality of Meat: III—Flavor. Lawrie’ S Meat Science. Duxford: Elsevier (2017). p. 383–417.
7. Van Ba H, Hwang I, Jeong D, Touseef A. Principle of meat aroma flavors and future prospect. Latest Res Qual Control. (2012) 2:145–76. doi: 10.5772/51110
8. Khan MI, Jo C, Tariq MR. Meat flavor precursors and factors influencing flavor precursors-a systematic review. Meat Sci. (2015) 110:278–84. doi: 10.1016/j.meatsci.2015.08.002
10. Gray J, Gomaa E, Buckley D. Oxidative quality and shelf life of meats. Meat Sci. (1996) 43:111–23. doi: 10.1016/0309-1740(96)00059-9
11. Vieira SA, Zhang G, Decker EA. Biological implications of lipid oxidation products. J Am Oil Chem Soc. (2017) 94:339–51. doi: 10.1007/s11746-017-2958-2
12. Wood J, Richardson R, Nute G, Fisher A, Campo M, Kasapidou E, et al. Effects of fatty acids on meat quality: a review. Meat Sci. (2004) 66:21–32. doi: 10.1016/S0309-1740(03)00022-6
13. Huang X, Ahn DU. Lipid oxidation and its implications to meat quality and human health. Food Sci Biotechnol. (2019) 28:1275–85. doi: 10.1007/s10068-019-00631-7
14. Tao L. Oxidation of polyunsaturated fatty acids and its impact on food quality and human health. Adv Food Technol Nutr Sci. (2015) 1:135–42.
15. Huali W, Jiyue Z, Jianbo Z. Revision on the “National food safety standard-standards for uses of food additives” (GB 2760–2011). China Food Addit. (2013) 67–71.
16. Min DB, Wen J. Qualitative and quantitative effects of antioxidants on the flavor stability of oil. J Food Sci. (1983) 48:1172–4. doi: 10.1111/j.1365-2621.1983.tb09185.x
17. Sohaib M, Anjum FM, Sahar A, Arshad MS, Rahman UU, Imran A, et al. Antioxidant proteins and peptides to enhance the oxidative stability of meat and meat products: a comprehensive review. Int J Food Prop. (2017) 20:2581–93. doi: 10.1080/10942912.2016.1246456
18. Meng FB, Lei YT, Zhang Q, Li YC, Chen WJ, Liu DY. Encapsulation of Zanthoxylum bungeanum essential oil to enhance flavor stability and inhibit lipid oxidation of Chinese-style sausage. J Sci Food Agric. (2022) 102:4035–45. doi: 10.1002/jsfa.11752
19. Cisneros-Yupanqui M, Lante A. Tea from the food science perspective: an overview. Open Biotechnol J. (2020) 14:78–83. doi: 10.2174/1874070702014010078
20. Mohanan A, Nickerson MT, Ghosh S. Oxidative stability of flaxseed oil: effect of hydrophilic, hydrophobic and intermediate polarity antioxidants. Food Chem. (2018) 266:524–33. doi: 10.1016/j.foodchem.2018.05.117
21. Chen B, McClements DJ, Decker EA. Minor components in food oils: a critical review of their roles on lipid oxidation chemistry in bulk oils and emulsions. Crit Rev Food Sci Nutr. (2011) 51:901–16. doi: 10.1080/10408398.2011.606379
22. Chastain M, Huffman D, Hsieh W, Cordray J. Antioxidants in restructured beef/pork steaks. J Food Sci. (1982) 47:1779–82. doi: 10.1111/j.1365-2621.1982.tb12881.x
23. Frankel EN, Huang S-W, Kanner J, German JB. Interfacial phenomena in the evaluation of antioxidants: bulk oils vs emulsions. J Agric Food Chem. (1994) 42:1054–9. doi: 10.1021/jf00041a001
24. Gordon MH, Kourkimskå L. The effects of antioxidants on changes in oils during heating and deep frying. J Sci Food Agric. (1995) 68:347–53. doi: 10.1002/jsfa.2740680314
25. Budilarto ES, Kamal-Eldin A. The supramolecular chemistry of lipid oxidation and antioxidation in bulk oils. Eur J Lipid Sci Technol. (2015) 117:1095–137. doi: 10.1002/ejlt.201400200
26. Wanasundara U, Shahidi F. Stabilization of seal blubber and menhaden oils with green tea catechins. J Am Oil Chem Soc. (1996) 73:1183–90. doi: 10.1007/BF02523382
27. Zhang L, McClements DJ, Wei Z, Wang G, Liu X, Liu F. Delivery of synergistic polyphenol combinations using biopolymer-based systems: advances in physicochemical properties, stability and bioavailability. Crit Rev Food Sci Nutr. (2020) 60:2083–97. doi: 10.1080/10408398.2019.1630358
28. Capuani A, Behr J, Vogel RF. Influence of lactic acid bacteria on the oxidation–reduction potential of buckwheat (Fagopyrum esculentum Moench) sourdoughs. Eur Food Res Technol. (2012) 235:1063–9. doi: 10.1007/s00217-012-1834-4
29. Chaiyasit W, Silvestre MPC, McClements DJ, Decker EA. Ability of surfactant hydrophobic tail group size to alter lipid oxidation in oil-in-water emulsions. J Agric Food Chem. (2000) 48:3077–80. doi: 10.1021/jf000323e
30. Papastergiadis A, Mubiru E, Van Langenhove H, De Meulenaer B. Malondialdehyde measurement in oxidized foods: evaluation of the spectrophotometric thiobarbituric acid reactive substances (Tbars) test in various foods. J Agric Food Chem. (2012) 60:9589–94. doi: 10.1021/jf302451c
31. Zhang YY, Zhang F, Thakur K, Ci AT, Wang H, Zhang JG, et al. Effect of natural polyphenol on the oxidative stability of pecan oil. Food Chem Toxicol. (2018) 119:489–95. doi: 10.1016/j.fct.2017.10.001
32. Chen XM, Kitts DD. Correlating changes that occur in chemical properties with the generation of antioxidant capacity in different sugar-amino acid maillard reaction models. J Food Sci. (2011) 76:C831–7. doi: 10.1111/j.1750-3841.2011.02215.x
33. Koutina G, Jongberg S, Skibsted LH. Protein and lipid oxidation in Parma ham during production. J Agric Food Chem. (2012) 60:9737–45. doi: 10.1021/jf3026887
34. Farajzadeh F, Motamedzadegan A, Shahidi S-A, Hamzeh S. The effect of chitosan-gelatin coating on the quality of shrimp (Litopenaeus vannamei) under refrigerated condition. Food Control. (2016) 67:163–70. doi: 10.1016/j.foodcont.2016.02.040
35. Wang L, Liu G, Cao L-X. Optimization of L-ascorbate oleate production using response surface methodology and its properties. Sci Technol Food Industry. (2016) 11:226–31.
36. Rababah TM, Ereifej KI, Al-Mahasneh MA, Al-Rababah MA. Effect of plant extracts on physicochemical properties of chicken breast meat cooked using conventional electric oven or microwave. Poult Sci. (2006) 85:148–54. doi: 10.1093/ps/85.1.148
37. Jin W, Shoeman DW, Shukla VKS, Csallany AS. Retardation of 4-hydroxy-2-transnonenal (Hne), a toxic aldehyde formation by antioxidants in heat-treated corn oil at frying temperature. Food Nutr Sci. (2020) 11:869–83. doi: 10.4236/fns.2020.117048
38. Shanmugapriya K, Saravana P, Payal H, Mohammed SP, Binnie W. Antioxidant activity, total phenolic and flavonoid contents of Artocarpus heterophyllus and Manilkara zapota seeds and its reduction potential. Int J Pharm Pharmaceut Sci. (2011) 3:256–60.
39. Benjakul S, Lertittikul W, Bauer F. Antioxidant activity of maillard reaction products from a porcine plasma protein–sugar model system. Food Chem. (2005) 93:189–96. doi: 10.1016/j.foodchem.2004.10.019
40. Phisut N, Jiraporn B. Characteristics and antioxidant activity of maillard reaction products derived from chitosan-sugar solution. Int Food Res J. (2013) 20:1077.
41. Jin Q. Effects of Antioxidants on the Oxidative Stability of Gardenia Fruit Oil. Hangzhou: Zhejiang A&F University (2018).
42. Liang Y, May C, Foon C, Ngan M, Hock C, Basiron Y. The effect of natural and synthetic antioxidants on the oxidative stability of palm diesel. Fuel. (2006) 85:867–70. doi: 10.1016/j.fuel.2005.09.003
43. EFSA. Scientific opinion on the re-evaluation of ascorbyl palmitate (E 304 (I)) and ascorbyl stearate (E 304 (II)) as food additives. EFSA J. (2015) 13:4289. doi: 10.2903/j.efsa.2015.4289
44. Manzocco L, Calligaris S, Mastrocola D, Nicoli MC, Lerici CR. Review of non-enzymatic browning and antioxidant capacity in processed foods. Trends Food Sci Technol. (2000) 11:340–6. doi: 10.1016/S0924-2244(01)00014-0
45. Yu X, Zhao M, Liu F, Zeng S, Hu J. Antioxidants in volatile maillard reaction products: identification and interaction. LWT Food Sci Technol. (2013) 53:22–8. doi: 10.1016/j.lwt.2013.01.024
46. Alfawaz M, Smith JS, Jeon IJ. Maillard reaction products as antioxidants in pre-cooked ground beef. Food Chem. (1994) 51:311–8. doi: 10.1016/0308-8146(94)90032-9
47. Mshayisa VV. Antioxidant Effects of Maillard Reaction Products (MRPS) Derived from Glucose-Casein Model Systems. Cape Town: Cape Peninsula University of Technology (2016).
48. Kirigaya N, Kato H, Fujimaki M. Studies on antioxidant activity of nonenzymic browning reaction products part I. Relations of color intensity and reductones with antioxidant activity of browning reaction products. Agric Biol Chem. (1968) 32:287–90. doi: 10.1271/bbb1961.32.287
49. Kek SP, Chin NL, Yusof YA, Tan SW, Chua LS. Classification of entomological origin of honey based on its physicochemical and antioxidant properties. Int J Food Prop. (2018) 20(Suppl. 3):S2723–38. doi: 10.1080/10942912.2017.1359185
50. Zamora R, Hidalgo FJ. Coordinate contribution of lipid oxidation and maillard reaction to the nonenzymatic food browning. Crit Rev Food Sci Nutr. (2005) 45:49–59. doi: 10.1080/10408690590900117
51. Frank D, Hughes J, Piyasiri U, Zhang Y, Kaur M, Li Y, et al. Volatile and non-volatile metabolite changes in 140-day stored vacuum packaged chilled beef and potential shelf life markers. Meat Sci. (2020) 161:108016.
52. Zhou Y, Wang Y, Pan Q, Wang XX, Li PJ, Cai KZ, et al. Effect of salt mixture on flavor of reduced-sodium restructured bacon with ultrasound treatment. Food Sci Nutr. (2020) 8:3857–71. doi: 10.1002/fsn3.1679
53. Wen R, Hu Y, Zhang L, Wang Y, Chen Q, Kong B. Effect of Nacl substitutes on lipid and protein oxidation and flavor development of Harbin dry sausage. Meat Sci. (2019) 156:33–43. doi: 10.1016/j.meatsci.2019.05.011
54. Wei CK, Ni ZJ, Thakur K, Liao AM, Huang JH, Wei ZJ. Aromatic effects of immobilized enzymatic oxidation of chicken fat on flaxseed (Linum usitatissimum L.) derived maillard reaction products. Food Chem. (2020) 306:125560. doi: 10.1016/j.foodchem.2019.125560
55. Li X, Wang K, Yang R, Dong Y, Lin S. Mechanism of aroma compounds changes from sea cucumber peptide powders (Scpps) under different storage conditions. Food Res Int. (2020) 128:108757. doi: 10.1016/j.foodres.2019.108757
56. Gao X, Feng T, Sheng M, Wang B, Wang Z, Shan P, et al. Characterization of the aroma-active compounds in black soybean sauce, a distinctive soy sauce. Food Chem. (2021) 364:130334. doi: 10.1016/j.foodchem.2021.130334
57. McGorrin RJ. The significance of volatile sulfur compounds in food flavors: An overview. In: Qian M, Xuetong F, Kanjana M editors. Volatile Sulfur Compounds in Food. Washington, DC: ACS Publications (2011). p. 3–31. doi: 10.1080/10408398.2010.536918
58. Dulsat-Serra N, Quintanilla-Casas B, Vichi S. Volatile thiols in coffee: a review on their formation, degradation, assessment and influence on coffee sensory quality. Food Res Int. (2016) 89:982–8. doi: 10.1016/j.foodres.2016.02.008
59. Weerawatanakorn M, Wu JC, Pan MH, Ho CT. Reactivity and stability of selected flavor compounds. J Food Drug Anal. (2015) 23:176–90. doi: 10.1016/j.jfda.2015.02.001
60. Casaburi A, Piombino P, Nychas GJ, Villani F, Ercolini D. Bacterial populations and the volatilome associated to meat spoilage. Food Microbiol. (2015) 45(Pt A):83–102. doi: 10.1016/j.fm.2014.02.002
61. Jousse F, Jongen T, Agterof W, Russell S, Braat P. Simplified kinetic scheme of flavor formation by the maillard reaction. J Food Sci. (2002) 67:2534–42. doi: 10.1111/j.1365-2621.2002.tb08772.x
Keywords: heat processed beef flavor, antioxidant, odorant, physicochemical, lipid oxidation
Citation: Zhang Z, Meng F, Wang B and Cao Y (2022) Effects of antioxidants on physicochemical properties and odorants in heat processed beef flavor and their antioxidant activity under different storage conditions. Front. Nutr. 9:966697. doi: 10.3389/fnut.2022.966697
Received: 11 June 2022; Accepted: 11 August 2022;
Published: 30 August 2022.
Edited by:
Yanyan Zhang, University of Hohenheim, GermanyReviewed by:
Oluwaseun Bamidele, University of Mpumalanga, South AfricaXiufang Dong, Qingdao University of Science and Technology, China
Copyright © 2022 Zhang, Meng, Wang and Cao. This is an open-access article distributed under the terms of the Creative Commons Attribution License (CC BY). The use, distribution or reproduction in other forums is permitted, provided the original author(s) and the copyright owner(s) are credited and that the original publication in this journal is cited, in accordance with accepted academic practice. No use, distribution or reproduction is permitted which does not comply with these terms.
*Correspondence: Bei Wang, d2FuZ2JlaUB0aC5idGJ1LmVkdS5jbg==; Yanping Cao, Y2FveXBAdGguYnRidS5lZHUuY24=