- 1ICAR-Indian Institute of Maize Research, Ludhiana, India
- 2AICRP on Post Harvest Engineering and Technology, University of Agricultural Sciences, Bengaluru, India
- 3School of Agricultural Biotechnology, Punjab Agricultural University, Ludhiana, India
- 4Department of Food Science and Technology, Punjab Agricultural University, Ludhiana, India
Maize grains are consumed majorly in the form of unleavened flat bread (chapatti) in the South East Asian region. The landraces are better accepted for their chapatti-making attributes such as grain color and good organoleptic properties. However, these cultivars are low in essential amino acids, particularly lysine and tryptophan content. Hence, an investigation was performed to identify maize genotypes with high nutritional value coupled with good chapatti-making qualities. Seven genotypes, comprising two Quality Protein Maize (QPM) hybrids, two normal maize hybrids, and three normal white maize landraces were assessed for their physical characteristics, proximate composition, and chapatti-making quality. Landrace 593 showed the highest protein and ash content. Flours obtained from different genotypes were significantly different (p ≤ 0.001) in terms of protein content, color value, textural, as well as mineral content. PMH 10 and IQMH 203 exhibited the highest and lowest hydration index, respectively. Two QPM hybrids showed significantly higher lysine and tryptophan content as compared to other genotypes. QPM hybrids were identified as the promising material with improved nutritional quality with respect to chapatti making. In combination with mustard greens, maize chapatti constitutes an important traditional delicacy in north India. The enhanced nutritional quality of QPM chapattis is an added advantage. We show the differentiation of chapattis made from QPM and normal maize using a rapid protocol developed previously. This is expected to enable the development and quality control of commercial enterprises based on high protein quality QPM.
Introduction
In terms of production, maize is the most important globally and the third most important cereal in India.1 It is regarded as good for health due to its nutraceutical properties. Celiac disease is an autoimmune chronic illness characterized by small intestine inflammation and villous atrophy (1). Patients with Celiac disease are advised to take gluten-free diets. Therefore, cereal grains such as wheat and barley were excluded from the diet of patients with celiac disease (2, 3). However, it is challenging to adhere to a restrictive gluten-free diet due to various reasons. First, the choice of food becomes limited because cereal products play predominant roles in a daily diet. Second, most processed foods contain gluten-based products as a major or an additional component (4). Moreover, the replacement of gluten is also a technological challenge, as the absence of gluten exhibits quality deficiencies such as poor expansion, color, and texture in final products (5, 6). Hence, the production of gluten-free foods possessing high nutritional value and consumer acceptance can be of immense health benefit to patients with celiac disease.
Maize is one of the preferred gluten-free cereal grains, with suitability to prepare food products mainly addressed to patients with celiac disease (7). Maize flour is consumed as food (35%), mainly in the form of unleavened flat bread (‘‘chapatti,’’ also known by the name ‘‘roti’’), in South East Asia. In northern parts of India, especially in the state of Punjab, the combination of maize chapatti with mustard green is a very popular traditional dish. Government of Punjab, India, has listed this traditional delicacy in its culture section and has mentioned the availability of entrepreneurial opportunities in cuisine.2 However, maize is limited in terms of its nutritional properties as being low in essential amino acids such as lysine and tryptophan, which leads to protein-energy malnutrition (5). If biofortified maize is utilized for making traditional delicacies, it would provide the benefits of improved gluten-free, amino acid nutrition. However, the sensory quality and nutritional attributes subsequent to product development need to be ascertained to evaluate its potential deployment.
To overcome malnutrition, the fortification of staple foods such as flatbread was considered (8). To minimize the requirement and to cut the cost of fortification, quality protein maize (QPM) has received much attention owing to its well-balanced protein and also being gluten-free grain, which reduces the risk of various diseases. QPM flour can be used to prepare nutrition-enriched chapatti with improved amino acid balances, which can help to overcome the national protein-calorie malnutrition problem (9). Incorporation of QPM flour in common food systems is expected to add value to it, and also provide convenient substitutes to expensive nutritious foods, with the changing lifestyles and trends around the world.
Chapatti has served as a staple diet to a majority of households in India, Pakistan, and some parts of the Middle East (10). Traditionally, it is prepared from wheat flour dough after rolling into a circular sheet followed by baking of both sides at high temperatures for a short time duration, which results in the puffing of chapatti by rapid steam formation. The major protein (gluten) present in wheat possesses unique properties to form a cohesive dough, which can trap gases and also enable mechanical sheeting but is not tolerated by patients with celiac disease. Although maize flour is healthy and gluten-free, the absence of gluten results in weak dough-binding properties and affects the chapatti-making quality (6). The dough behavior, rheological properties, and sensory qualities such as color, flavor, texture, and aroma of chapatti directly affect the acceptability of chapatti (11). In India, most of the population consuming maize as food prefers locally available maize landrace for chapatti due to its fine texture and unique taste. Keeping in view the preference of people and nutritional aspects, the present study was conducted to evaluate chapattis made from different types of maize genotypes, viz., landraces, normal, and QPM hybrids. Overall, the study aimed to compare the chapatti-making ability and nutritional quality of seven maize genotypes.
Materials and methods
Materials
The materials consist of grains of seven maize genotypes. These genotypes represented both white and yellow maize including landraces, normal hybrids, as well as QPM hybrids. Various genotypes were grown at ICAR-IIMR Ladhowal farm, Ludhiana, Punjab. The genotypes were harvested in October 2020 and dried properly followed by storage in airtight containers at ambient temperature. A sample from each genotype was selected randomly. The details of the genotypes selected for the study are as follows:
Sr. No. | Variety | Hybrid | Developing Organization |
1 | PMH 10 | Normal Orange Maize Hybrid | PAU, Ludhiana |
2 | IQPMH 1708 | QPM Experimental Hybrid | ICAR-IIMR, Ludhiana |
3 | IQMH 203 | QPM Hybrid | ICAR-IIMR, Ludhiana |
4 | MCFL 15 | Normal White Maize Landrace | ICAR-IIMR, Ludhiana |
5 | MCFL 346 | Normal White Maize Landrace | ICAR-IIMR, Ludhiana |
6 | White Hybrid 574 | Normal White Maize Experimental Hybrid | ICAR-IIMR, Ludhiana |
7 | Landrace 593 | Normal White Maize Landrace | ICAR-IIMR, Ludhiana |
All the grains were screened to remove extraneous matter. The cleaned grains were stored in sealed packages at room temperature. Each genotype was assessed for its physical characteristics and was ground to make maize flour (<200 μ) using a laboratory mill (Perten Instruments, Hagersten, Sweden), sieved, and packed for further analysis and processing.
Analysis of maize kernel, maize flour, and chapatti was performed by following methods
Assessment of physical properties of maize kernels
Maize genotypes were assessed for their physical characteristics such as kernel type (flint, dent) and kernel color (white, orange, and yellow), as well as other physical and quality parameters described below.
Thousand kernel weight
Thousand kernel weight was noted by weighing a hundred grains on an electronic weighing balance and multiplied by 10 and results were expressed in grams (g).
Specific gravity
A measuring cylinder (100 ml) was filled with water up to a mark. Pre-weighed corn grains were poured into the cylinder and a rise in the volume of water was noted.
Linear dimensions
The linear dimensions (in triplicates) such as length (L), breadth (b), and thickness (t) of the corn kernel were measured by a vernier caliper (12).
Shape index
The shape index is a measure of the kernel shape that is oval or spherical. The data are computed according to the following equation:
where, b = breadth and t = thickness.
If the shape index is greater than 1.5, the kernel is considered oval and if it is less than 1.5, the kernel will be of spherical shape (13).
Hydration capacity (%) and hydration index
Hydration capacity and hydration index were determined according to the method described by Williams et al. (14). To measure hydration capacity, a known weight of grains is transferred into a beaker containing water. Beaker was covered with aluminum foil and left overnight at room temperature. On the next day, the water was drained and the weight of wet grains was noted and calculated as follows:
Analysis of maize flour
Proximate analysis
Proximate composition of maize flour was determined using the standard method (15).
Moisture content
The moisture content of the flour was analyzed by the hot air oven method after drying at 100°C for 2 h and the percent moisture content is calculated from loss in moisture from the sample (15).
Fat content
Fat content of the flour samples was analyzed by FOSS instrument-Soxtec 2045 (Sweden). Approximately 2 g of flour sample was added in a thimble followed by the addition of petroleum ether (70 ml) in pre-weighed extraction beakers. The instrument was pre-heated prior to analysis at a temperature of 130–135°C. After a pre-determined temperature, extraction beakers were attached and allowed to boil for 20 min followed by rinsing for 20 min. After the solvent was recovered for 10 min, the extraction beakers were removed and weighed after cooling at room temperature. Crude fat (%) was calculated from the increase in the weight of the extraction beaker (15).
Protein content
The protein content of flour samples was determined by the micro-Kjeldahl method. The macro-Kjeldhal method was used to determine the nitrogen content for all raw materials (15). A general composite conversion factor of 6.25 was used to calculate the percent crude protein content.
Ash content
The sample was taken in pre-weighed crucibles followed by charring at a hot plate until no fumes come out. Charred samples were placed in a muffle furnace at 550°C for 5 h and were then placed in the desiccator. The weight of the final crucible is noted as ash content (15).
Carbohydrate content
Carbohydrate content was calculated using a subtraction method, that is, 100 – moisture, ash, fat, and protein contents.
Pasting properties of maize flour
The pasting properties of the maize flour samples were determined by using the Rapid Visco Analyzer (RVA) model starch Master (Newport Scientific, Warrie Wood, Australia). The operation procedure is followed as given below: The RVA was allowed to warm up for 30 min prior to the experiment. The pre-weighed sample was poured into a canister followed by the addition of water (25 ml). The paddle was inserted into the canister and vigorously shaken up and down 10 times through the sample until it mixes properly. Insert the canister into the pre-adjusted instrument. The programmed heating and cooling cycle were given. After the completion of the test, the pasting properties such as peak viscosity, final viscosity, breakdown, and setback were noted. The canister was removed from the instrument and the sample was discarded.
Mineral estimation
The mineral content of maize flour was determined for five different minerals viz. Fe, Zn, Ca, Mg, and K using the OptimaTM2100DV Spectrometer (Perkin Elmer). The mineral concentrations were recorded as ppm, which can be represented as mg of mineral per 100 g of sample.
Amino acid analysis
Amino acid analysis (tryptophan and lysine) of the maize flour samples was carried out by following a previously described method (16).
Color analysis
Color analysis of flour samples was carried out using a Hunter lab colorimeter on the basis of L*, a*, and b* values. The colorimeter was calibrated with the standard black and white plate to set zero. The samples were uniformly packed in clean petri plates. The different places on the surface were given three exposures by the colorimeter. Readings were displayed as a*, b*, and L* where the ‘a’ value indicates the redness to greenness, the ‘b’ value measures the blueness to yellowness, while the ‘L’ value ranges from 0 (black) to 100 (white) which indicates the measure of lightness (17).
Chapatti-making
Preparation of chapatti
Chapatti was prepared by adopting the method as described by previous researchers with slight modifications (18). Corn flour was mixed with an optimum amount of lukewarm water to form a smooth dough. Dough balls of similar weight were prepared, placed on a rolling board, and round sheeted using a rolling pin to make chapatti. The raw chapatti was immediately placed on a hot plate (tawa) and baked at 220°C on one side and then on the other side. It was again turned until fully baked. The chapattis prepared from different genotypes (Figure 1) were allowed to cool for 10 min at 25°C and then packed in polythene pouches and placed in an airtight container for further analysis.
Physico-chemical properties of chapatti
Water absorption capacity
The water absorption capacity of maize flour to form dough was measured by employing the method outlined by Gujral and Gaur (19). The calculated amount of water was added to the flour (200 g) to form the smooth and non-stick dough, appropriate for sheeting without exhibiting any cracks. Then, the optimum amount of water added was noted.
Texture analysis of chapatti
A strip of each chapatti was tested (in triplicates) for Shear value and Texture Profile Analysis (TPA) on the TA/XT2 Texture analyzer (Stable Micro Systems, Surrey, England) by following the method described below.
Texture Profile Analysis (TPA): Texture Profile Analysis parameters including adhesiveness, cohesiveness, springiness, hardness, chewiness, and gumminess were measured. Samples were cut into uniform sizes and a cylindrical aluminum (P25) probe was used to exert pressure. The instrumental condition used is as follows: Pre-test speed: 10.0 mm/min, Post-test speed: 10.0 mm/min Trigger: 15.0 g, Load cell: 20.0 kg (20).
Shear Value: Shear value was measured by cutting the strip (4 cm × 2 cm) of chapatti (taken from the center of the chapatti) using Warner Bratzler Blade (HDP/BSK). The following conditions were employed: load cell—50 kg, target mode distance—4.5 mm, pre-test speed—1 mm/s, test speed—2 mm/s, post-test speed—10 mm/s, and trigger force—10 g. The force required to shear the strip of chapatti into two pieces was noted. Three measurements were taken for each sample in triplicates and average values are reported (21).
Proximate, amino acid, and mineral content of chapatti
Chapatti was analyzed for proximate composition, amino acid content, mineral content, and color as per the previously described methods for flour.
Sensory evaluation
Chapatti prepared from each genotype were analyzed for sensory scores in terms of color, appearance, taste, mouth feel, and overall acceptability in order to find the best genotype for the development of chapatti. Semi-trained and untrained panelists were selected to evaluate the Chapatti. Chapatti was placed on white paper and labeled with numbers to avoid any bias. A total of 10 semi-trained panelists (five men and five women, between the age group of 25 and 55 years) were selected for sensory evaluation. All the panelists were instructed to rinse their mouths properly with water after tasting every sample and to score the chapatti samples based on the acceptance. A 9-point hedonic scale presenting a score of 1 for extremely disliking and 9 for extremely liking was used. The final score was calculated by averaging the scores provided by all the panelists (22).
Rapid differentiation
A process was designed to rapidly differentiate normal maize grains from QPM grains utilizing molecular differences in the two groups (Figure 2). An Indian Patent application (No. 202211015547) has been filed for this process. The same process was used to differentiate between the normal maize chapattis from QPM chapattis. The method records OD at 595 nm for nutritionally poor protein to act as a proxy for maize protein quality.
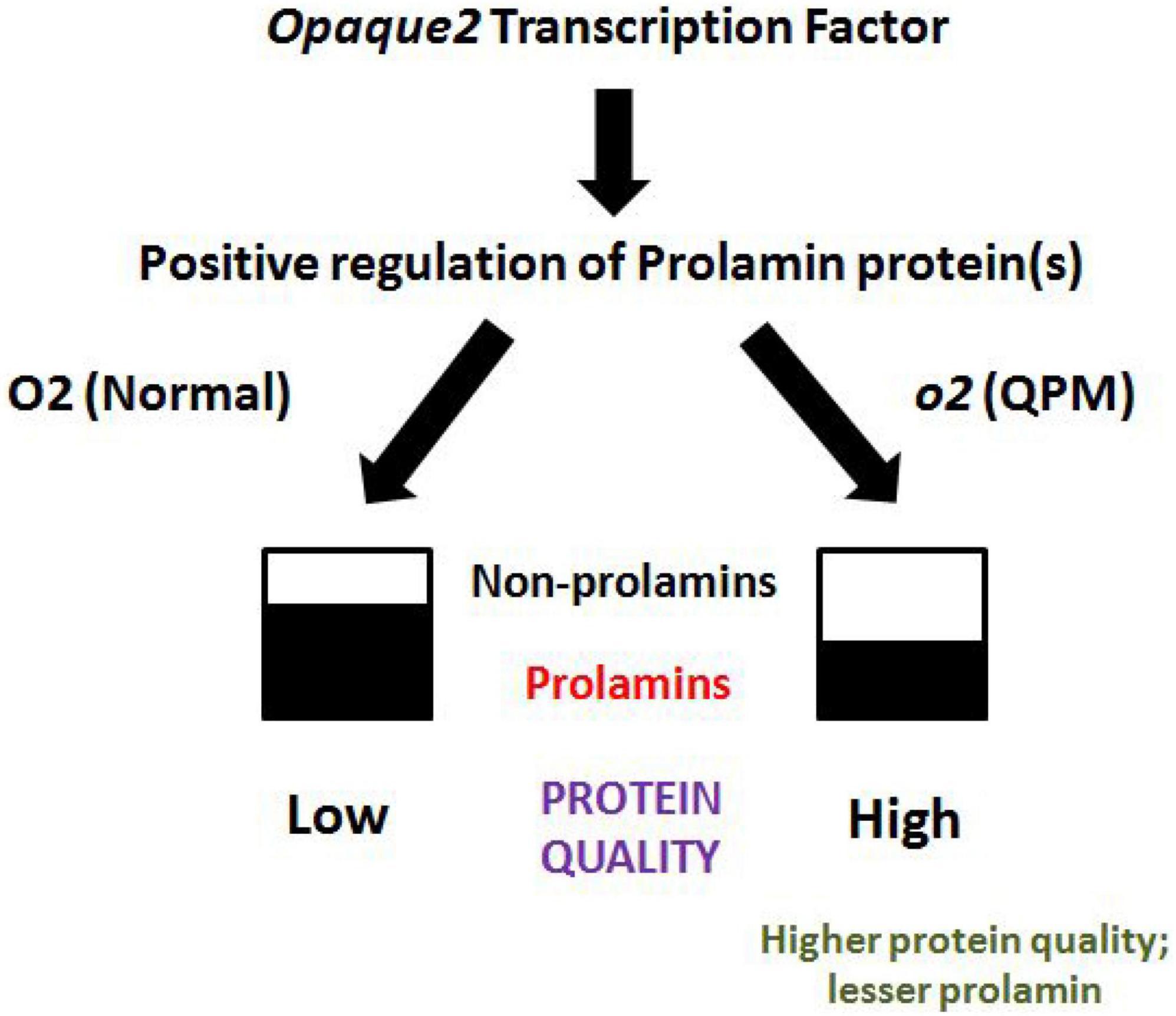
Figure 2. Protein quality in normal maize and QPM. By virtue of its replacement by higher quality non-prolamins, the lower expression of prolamins increases protein quality on one hand and decreases the chances of any adverse reactions in some patients with celiac disease as observed in normal maize.
Statistical analysis
Data were recorded in triplicates and presented as mean ± standard deviation. The data were analyzed using SAS version 9.4 software. The least significant difference (LSD) was used as the test for significance for different measured traits among the treatments/genotypes. Paired ‘t’ test was used to test the significant changes in different attributes between maize raw flour and chapatti made out of it.
Results and discussion
The physical properties of maize are important for milling and processing industries which usually prefer large grains. The greater the size of grains the more would be the extraction of starch and oil (23).
Physical and dimensional properties of maize kernels
The physical characteristics such as color, grain type, dimensions, thousand kernel weight (TKW), specific gravity, hydration capacity, hydration index, and shape index of different genotypes have been mentioned in Table 1. Each of the maize genotypes recorded significantly different thousand kernel weights (TKWs). It was observed that the kernel weight of White Hybrid 574 was highest (368.47 g) followed by Landrace 593 (356.43 g), MCFL 346 (322.33 g), MCFL 15 (298.83 g), and PMH 10 (291.66 g). Karthik et al. (13) reported that the TKW of different maize genotypes ranged from 80.50 to 321.85 g, which is in agreement with the present study. Maize genotypes having TKW greater than 290 gm are appropriate for industrial applications because they provide high yields in different products (23). The dimensions such as length, breadth, and thickness of various corn genotypes varied significantly (p ≤ 0.001) between 9.45 and 11.28 mm, 7.20 and 9.53 mm, and 3.46 and 5.56 mm, respectively. QPM (IQPMH 1708) genotype had the smallest grain size out of the seven genotypes under study. The Thousand Kernel Weight (TKW) of QPM hybrids (HQPM 1 and HQPM 7) was observed to be in the range of 275.5 and 288.3 g by Sangeeta and Grewal (24). The shape index is important in determining the productivity of various genotypes as flat grains are considered desirable grain quality to meet the requirement of high productivity (25). The data showed that two genotypes namely MCFL 15 and MCFL 346 were of spherical shape whereas White Hybrid 574 and IQPMH 1708 showed no significant difference in shape index and were oval-shaped with a higher yield. The study of Srinivas et al. (25) stated that the factors contributing to shaping variation could be the position of grain on the cob, varietal or environmental difference, and distorted or twisted pattern of rows within the cobs. The results of Bolade (26) with respect to TKW, length, and width of the maize ranged from 223.7 to 284.2 g, 9.1 to 11.9 mm, and 8.1 to 9.5 mm, respectively.
The hydration index is a process of water absorption by grains that increases their moisture content and could affect their physicochemical, nutritional, as well as textural properties (27). The hydration index of corn genotypes significantly varied from 0.194 to 0.432 (p ≤ 0.001). The hydration index was higher in PMH 10 and a lower value was found in IQMH 203. The lower hydration index might help to extend the shelf life of maize grain during storage (27).
Pasting properties of maize flour
The pasting properties of flours obtained from seven genotypes are presented in Table 2. A significant difference (p ≤ 0.001) was observed for pasting properties, viz., peak viscosity (cP), hold viscosity (cP), final viscosity (cP), breakdown (cP), set back (cP), and water absorption capacity (ml) among flours from different maize genotypes depending on the rigidity of starch granules which in turn affect the granule swelling potential (28). Peak viscosity ranged from 207 (IQPMH 1708) to 1,097 cP (PMH 10), indicating the water binding capacity of starch or mixture, which often correlates to the quality of the final product, respectively. The higher peak viscosity may be associated with a high proportion of ungelatinized starch, whereas the lower values might be due to greater degradation through depolymerization and molecular entanglement during processing conditions (29). Breakdown value varied significantly and was higher in MCFL 346 (202 cP) followed by MCFL 15 (117 cP). It is related to the starch response to shear with continuous heating, causing a rupture and resulting in a decrease in viscosity (30). The setback viscosity is related to starch retrogradation and reordering (31) and varied from 908 to 1,696.33 cP. PMH 10 was reported to exhibit a low rate of syneresis and retrogradation of starch molecules (32). The low setback viscosity value of IQPMH 1708 and Landrace 593 flour indicates the lower value of retrogradation. Hence, chapattis prepared from IQPMH 1708 and Landrace 593 genotypes would remain fresh for a longer time (8). Sagbo et al. (33) found the range of peak viscosity and setback viscosity of different maize genotypes varied from 438-1,271.5 cP and 362-2,534 cP, respectively. IQMH-based flour can be used to replace wheat flour for chapatti preparation, which can complement as a source of essential amino acids as well as a gluten-free diet.
Water absorption is the addition of lukewarm water to flour to obtain desired consistency of the dough and indicate the baking quality of the flour. A significant difference was observed for the water absorption capacity of different maize genotypes, however, similar water absorption was observed for MCFL 15 and MCFL 346, and PMH 10 and IQMH 203 (Table 2). White hybrid 574 required a higher amount of water (159.3 ml) followed by MCFL 346 (155 ml) for the preparation of dough to make chapatti, which could be attributed to the molecular structure of starch, variation in protein content, and presence of high hydrophilic constituents (34). The lowest absorption was found in Landrace 593 (p ≤ 0.001). It shows that the genotype White hybrid 574 has a higher ability to retain water during the baking process which provides a desirable soft texture in final products (35). However, the QPM genotypes IQPMH 1708 and IQMH 203 had recorded medium water absorption, i.e., 146 and 135 ml. This indicates that this genotype had soaked a good amount of water which is desirable for the baking of chapattis.
Nutritional composition of maize flour and chapattis
Maize genotypes varied significantly with respect to their proximate composition such as moisture, fat, and protein contents (Table 3). The protein content in chapattis was observed to be higher in Landrace 593 followed by MCFL 15. The concentration of protein varied from 6.19 to 8.39% as stated in the previous study conducted by Vaswani et al. (36). MCFL 15 flour had lower moisture (3.02%), and higher ash (1.99%) and crude fiber (1.36%) contents. Sandhu et al. (37) also reported ash, protein, fiber, and carbohydrate contents of 1.66%, 5.18–7.82%, 1.56–2.42%, and 87.6–92.5% for corn flour. The composition of chapatti also differed significantly among different genotypes (Table 3). The chapatti prepared from genotype IQPMH 1708 showed higher moisture content (31.15%), which is a desirable property to impart softness in chapattis, whereas MCFL 15-based chapatti had lower moisture content (24.67%). IQMH 203-based chapattis were recorded for the highest ash (1.71%) and lowest crude fiber (0.18%) contents. The t-value indicates that there was a highly significant difference between maize flours and chapatti for the parameters such as moisture (−21.93), fiber (2.79), and carbohydrate (14.09), whereas fat (−0.79), ash (−2.08), and protein (−0.50) showed no significant difference between flour and chapatti.
The significantly higher content of minerals such as K (1929.04 ppm) and P (4188.85 ppm) was noticed in IQMH 203 and MCFL 15 genotypes, respectively (Table 4). Mineral contents such as copper (2.21–2.36 ppm), zinc (37.05–52.40 ppm), calcium (410–590 ppm), and potassium (2,915–3,471 ppm) were also reported in earlier studies (38). Similar results for Zn content (30.51–42.18 ppm) in maize varieties were also observed by Kabir et al. (39). The difference observed in the mineral composition might be due to the varietal difference, environmental effect, or type of irrigation or fertilizer used. Vaswani et al. (36) stated that the genotypic effect is more prominent in the composition than other environmental factors. The mineral content of chapatti revealed that cooking greatly affects the composition of the minerals. Zn, Cu, Mn, P, Ca, and K contents of chapatti varied from 38.10 to 46.30 ppm; 2.05–3.51 ppm; 4.50-7.40 ppm; 3,156.85–4,128.35 ppm; 219.46–491 ppm; and 1,546.72–1,942.55 ppm, respectively. It was observed that the chapatti samples had significantly higher Zn, Cu, Mn, Ca, and K contents except for P in comparison to flour samples. It might be due to the cooking process involved during the preparation of chapatti. The Zn (46.30 ppm) and Ca (491 ppm) contents of chapatti prepared from PMH 10 were much higher.
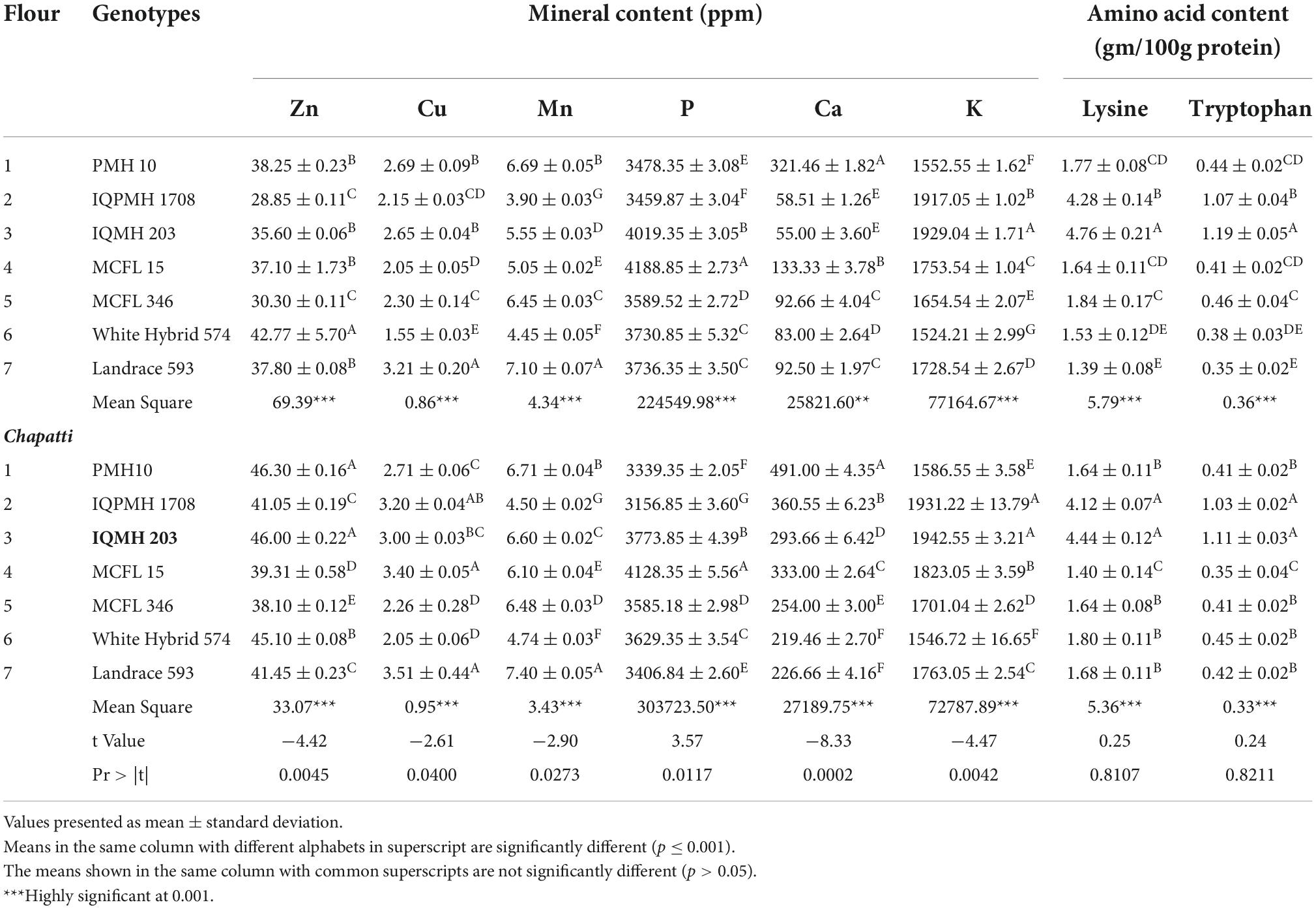
Table 4. Mineral and amino acid content of maize flours and chapatti prepared from different genotypes.
Lysine and tryptophan contents of maize flours and chapattis are summarized in Table 4. The lysine and tryptophan contents were observed to be in the range of 1.39–4.76 g and 0.35–1.19 g per 100 g of protein and significantly differed among various genotypes. IQMH 203 showed a higher value of lysine followed by IQPMH 1708. Landrace 593 showed the lowest lysine content which is 1.39 g/100 g of protein. A similar trend in amino acid content was observed in chapatti prepared from different genotypes. The lysine and tryptophan contents of chapatti prepared from QPM hybrids, viz., IQPMH 1708 and IQMH 203 were recorded to be 4.12 and 1.03, and 4.44 and 1.11 g/100 g, respectively. However, the lysine and tryptophan contents in chapatti were lower as compared to flours which could be due to the effect of baking conditions (40). A study by Gallego-Castillo et al. (41) in non-QPM and QPM-based processed products, namely tortillas, arepas, and mazamorra, showed a true retention value of tryptophan content that is 62.27, 16.67, 15.91%, and 66.29, 23.44, and 19.69%, respectively. During processing, the reduction in lysine content might be due to the occurrence of the Maillard reaction, which modifies the starch and protein structures and leads to more availability of reducing sugars and reactive sites of protein, respectively (42). It was also found that the lysine and tryptophan contents are more than double in the QPM-based chapattis as compared to normal and landrace genotypes-based chapattis. Hence, chapattis prepared from QPM genotypes are more nutritious and beneficial for human consumption than chapattis prepared from normal maize.
Color parameter of chapatti
Hunter color laboratory parameters such as L*, a*, and b* values among flours and chapattis prepared from different maize genotypes were observed (Table 5). The L* value of flour and chapatti from different maize genotypes significantly varied from 84.88 to 90.63 and 47.09–74.96, respectively. In the case of flour and chapattis, the highest L * value (lightness) was observed for MCFL 346 and the lowest for PMH 10. L* values of IQPMH 1708 and IQMH 203 were observed to be 87.38 and 89.18 indicating that the color of chapattis was acceptable and preferred by the consumer. L* value of 81.94 to 86.96 for corn flours from various genotypes have been reported by Sandhu et al. (37). Kathuria et al. (43) analyzed the color value of maize flour to be around 70.05 ± 0.02. The a* value presents the redness or greenness which ranged from −0.57 to 1.48 and 2.25–5.31 in flour and chapatti, respectively. The highest a* and b* values in PMH 10-based flour and chapatti might be due to the high level of anthocyanins and carotenoids, respectively (17). ΔL, Δa, Δb, and ΔE values indicate the color difference for lightness, redness-greenness, blueness-yellowness, and total color difference, respectively, for different genotypes-based corn flour and these values ranged from −13.99 to −8.24, −0.43 to 1.62, 8.15 to 22.19, and 12.16 to 26.28, respectively. Genotypes, viz., White Hybrid 574, MCFL 15, and MCFL 346 exhibited no significant difference in total color difference value. IQMH 203 and IQPMH 1708 were not significantly different with respect to the b* value in maize flours. Highly significant differences for parameters such as L (8.46), a*(−9.18), b* (−12.54), ΔL (9.83), Δa (−10.35), Δb (−9.61), and ΔE (−10.84) were observed among corn flours and chapatti for different genotypes.
Textural properties of chapatti
The textural properties directly affect the overall acceptability of chapatti (20). The results indicated that the different maize genotypes exhibited significant differences in the shear force of the chapatti. The shear force value is mainly related to the freshness and pliability of the final product. The value of shear force was found to be in the range of 3.36 (Landrace 593) − 6.96 N (White hybrid 574), (Table 6). A decrease in shear force resulted in an increase in pliability and soft texture which might be due to the higher retention of moisture in chapatti (20).
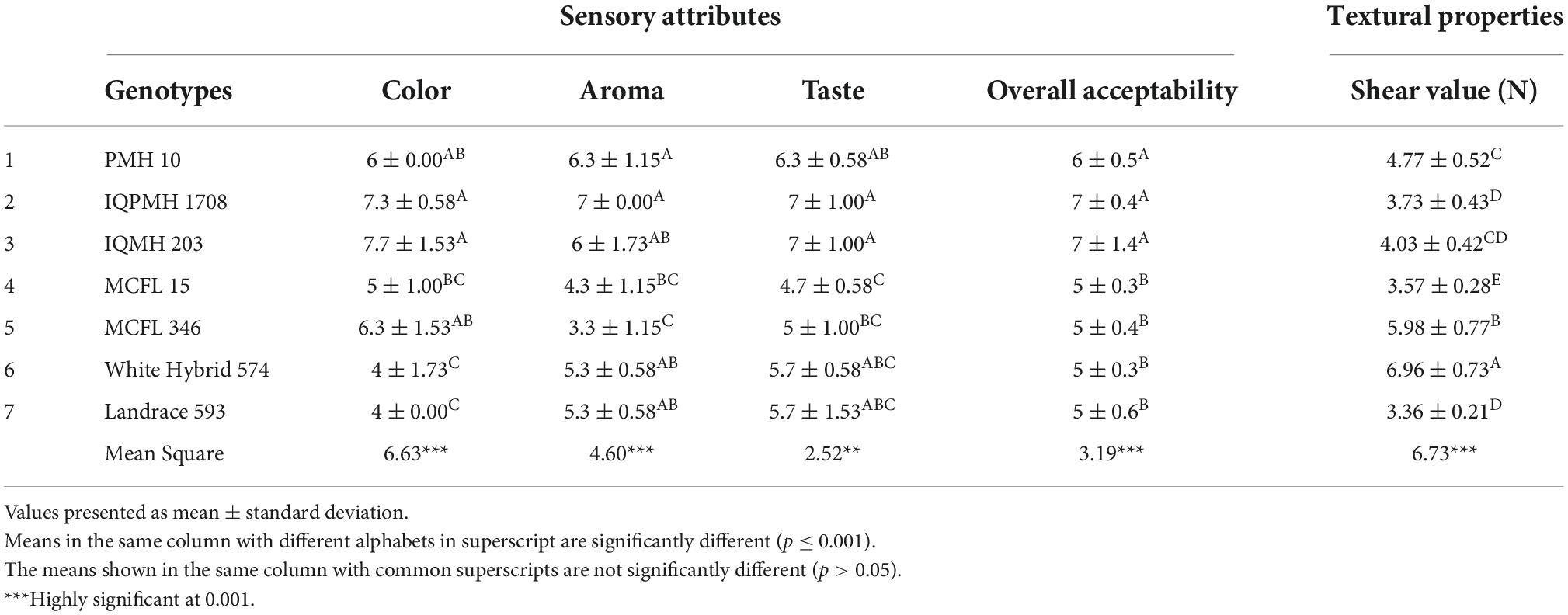
Table 6. Sensory attributes and textural properties (Shear Value) of Chapatti from different genotypes.
Sensory attributes of maize-based chapatti
The sensory score of chapatti made from various maize genotypes is elucidated in Table 6. Maize chapatti prepared from IQMH 203 and IQPMH 1708 was rated highest in terms of color, taste, aroma, and overall acceptability and were not significantly different from each other. Chapatti prepared from White Hybrid 574 and Landrace 593 was not highly acceptable in terms of sensory attributes. Hence, due to the relatively higher sensory score of IQMH 203 and IQPMH 1708 coupled with their relative nutritional value in terms of mineral profile and essential amino acids, they were considered the most appropriate varieties for the production of maize-based nutritious flat breads.
Differentiation of QPM chapattis from normal maize
Figure 2 provides a schematic representation of the mechanism, by which QPM results in higher protein quality as compared to normal maize. The opaque-2 gene positively regulates low-quality prolamin proteins in normal maize, whereas its mutation in QPM increases higher-quality non-prolamin proteins, including albumins, globulins, and glutelins. By virtue of its replacement by higher quality non-prolamins, the lower expression of prolamins increases protein quality on one hand and decreases the chances of any adverse reactions in some patients with celiac disease as observed in normal maize (44). In order to enable commercialization of the biofortified products in the market, it is necessary to employ a rapid method for Quality Control and consumer empowerment. Using a previously standardized process (Indian patent applied), we quantified protein quality in chapattis made from normal maize and QPM. The samples were read at 595 nm after processing. A lower value indicates less nutritionally poor protein fraction, thereby higher overall maize protein quality. Conversely, a higher amount of nutritionally poor protein indicates overall lower maize protein quality. The readings of IQPMH 1708 and IQMH 203 at 595 nm were 0.135 and 0.152, respectively, while the readings for normal genotypes were above 0.25. This indicates that a cut-off of 0.2 at 595 nm is indicative of protein quality in maize chapattis. This process requires less than 10 min to complete, providing a good tool for the quality control of the product.
Conclusion
With respect to modern lifestyles and healthy eating trends, traditional and nutritional food products are gaining popularity. Chapatti is a major staple baked food in most households and could bring the combination of nutrition and goodness of maize. Hence, the present study was executed for a better understanding of the nutritional and chapatti-making quality attributes of different maize genotypes. Chapattis prepared from QPM showed higher lysine and tryptophan content as compared to other genotypes. The overall quality score of chapatti prepared from IQMH 203 and IQPMH 1708 scored higher and imparted a desirable aroma coupled with chapatti of better texture, taste, and acceptability. Therefore, such cultivars need to be popularized for nutritional security at low cost in midday meals and other nutrition schemes of the government as well as for catering to patients with celiac disease. Given the listing of maize chapatti in traditional delicacies, there is ample scope for entrepreneurship development in this sector using QPM. The availability of a rapid protocol to differentiate the products made from QPM from those of normal maize is an added advantage to ensure quality control and empower consumers. Overall, the study provides a comparative assessment of different maize types for chapatti-making and shows the ability of rapid differentiation to categorize and confirm the final product based on protein quality.
Data availability statement
The original contributions presented in this study are included in the article/supplementary material, further inquiries can be directed to the corresponding author.
Author contributions
NK performed the experiments and prepared the manuscript. RK planned the experiments and reviewed the manuscript. AS performed the experiments on Rapid Kit and reviewed the manuscript. DS and DC reviewed the manuscript. BS planned the experiments. AD analyzed the results. PK and YK performed the experiments. PS performed the mineral content analysis. All authors contributed to the article and approved the submitted version.
Acknowledgments
The facilities provided by ICAR—Indian Institute of Maize Research Ludhiana are hereby acknowledged. AS acknowledges the funding received from Science for Equity, Empowerment, and Development (SEED), Department of Science and Technology, Government of India for a research grant (SP/YO/217/2018).
Conflict of interest
The authors declare that the research was conducted in the absence of any commercial or financial relationships that could be construed as a potential conflict of interest.
Publisher’s note
All claims expressed in this article are solely those of the authors and do not necessarily represent those of their affiliated organizations, or those of the publisher, the editors and the reviewers. Any product that may be evaluated in this article, or claim that may be made by its manufacturer, is not guaranteed or endorsed by the publisher.
Footnotes
References
1. Ludvigsson JF, Leffler DA, Bai J, Biagi F, Fasano A, Green PHR, et al. The Oslo definitions for coeliac disease and related terms. Gut. (2013) 62:43–52. doi: 10.1136/gutjnl-2011-301346
2. Rubio-Tapia A, Murray JA. Celiac disease. Curr Opin Gastroenterol. (2010) 26:116. doi: 10.1097/MOG.0b013e3283365263
3. Ciacci C, Ciclitira P, Hadjivassiliou M, Kaukinen K, Ludvigsson F, McGough N, et al. The gluten-free diet and its current application in coeliac disease and dermatitis herpetiformis. United Eur Gastroenterol J. (2015) 3:121–35. doi: 10.1177/2050640614559263
4. Missbach B, Schwingshackl L, Billmann A, Mystek A, Hickelsberger M, Bauer G, et al. Gluten-free food database: the nutritional quality and cost of packaged gluten-free foods. Peer J. (2015) 3:e1337. doi: 10.7717/peerj.1337
5. Segura MEM, Rosell CM. Chemical composition and starch digestibility of different Gluten-free breads. Plant Foods Hum Nutr. (2011) 66:224–30. doi: 10.1007/s11130-011-0244-2
6. Patil S, Arya SS. Optimization and characterization of Gluten-freeformulation for the development of gluten free flatbread using underutilized sources. Curr Res Nutr Food Sci. (2018) 6:678–89. doi: 10.12944/CRNFSJ.6.3.10
7. Volta U, Caio G, Tovoli F, De Giorgio R. Non-celiac gluten sensitivity: questions still to be answered despite increasing awareness. Cell Mol Immunol. (2013) 10:383–92. doi: 10.1038/cmi.2013.28
8. Wani IA, Sogi DS, Sharma P, Gill BS. Physicochemical and pasting properties of unleavened wheat flat bread (Chapatti) as affected by addition of pulse flour. Cogent Food Agric. (2016) 2:1124486. doi: 10.1080/23311932.2015.1124486
9. Abiose SH, Ikujenlola AV. Comparison of chemical composition, functional properties and amino acids composition of quality protein maize and common maize (Zea may L). Afr J Food Sci Technol. (2014) 5:81–9.
10. Chilkunda N, Salimath V. Carbohydrate composition of wheat, wheat bran, sorghum and bajra with good chapati/roti (Indian flat bread) making quality. Food Chem. (2001) 73:197–203. doi: 10.1016/S0308-8146(00)00278-8
11. Prabhasankar P, Manohar R, Gowda L. Physicochemcial and biochemical characterization of selected wheat cultivars and their correlation to chapatti making quality. Eur Food Res Technol. (2002) 214:131–7. doi: 10.1007/s00217-001-0435-4
12. Ghaffari H, Marghoub N, Sheikhdarabadi MS, Hakimi A, Abbasi F. Physical properties of three Iranian onion genotypes. Int Res J Appl Basic Sci. (2013) 7:587–93.
13. Karthik SK, Mahesh T, Sumanth B, Tanmay M. Study of physical and engineering properties of corn (Zea mays). Bull Env Pharmacol Life Sci. (2017) 6:404–9.
14. Williams PC, Nakoul H, Singh KB. Relationship between cooking time and some physical characteristics in chickpea (Cicer arietinum L). J Sci Food Agric. (1983) 34:492–6. doi: 10.1002/jsfa.2740340510
15. AACC. Approved Laboratory Methods. St. Paul, MN: American Association of Cereal Chemists (2000).
16. Scott IMP, Bhatnagar S, Betran J. Tryptophan and methionine levels in quality protein maize breeding germplasm. Maydica. (2004) 49:303–11.
17. Moreira R, Chenlo F, Arufe S, Rubinos SN. Physicochemical characterization of white, yellow and purple maize flours and rheological characterization of their doughs. J Food Sci Technol. (2015) 52:7954–63. doi: 10.1007/s13197-015-1953-6
18. Kadam ML, Salve RV, Mehrajfatema ZM, More SG. Development and evaluation of composite flour for missi roti/chapatti. J Food Process Technol. (2012) 3:134.
19. Gujral HS, Gaur S. Effects of barley flour, wet gluten and liquid shortening on the texture and storage characteristics of chapatti. J Texture Stud. (2002) 33:461–9. doi: 10.1111/j.1745-4603.2002.tb01360.x
20. Yadav DN, Patki PE, Mahesh C, Sharma GK, Bawa AS. Optimization of baking parameters of chapatti with respect to vitamin B1 and B2 retention and quality. Int J Food Sci Technol. (2008) 43:1474–83. doi: 10.1111/j.1365-2621.2008.01712.x
21. Shah TR, Prasad K, Abhijit Pardeep Kumar Das P. Development and parameter optimization of maize flat bread supplemented with asparagus bean flour. Food Sci Technol. (2018) 38:148–56. doi: 10.1590/1678-457x.36616
22. Larmond E. Methods for Sensory Evaluation of Food. Ottawa: Canada Department of Agriculture Publications (1970).
23. Gongora IG, Dunoyer AT, García-Zapateiro LA. Physical, chemical and biological properties of maize variety Fr –28. Contemp Eng Sci. (2018) 11:257–68. doi: 10.12988/ces.2018.8114
24. Sangeeta Grewal RB. Pasting properties of maize flour from variety HQPM-1 and HQPM-7. J Pharmacogn Phytochem. (2018) 7:223–5.
25. Srinivas T, Bhashyam MK, Chand N, Bhattacharya S, Murthy SS, Narasimha HV. Relationship of cob characters with grain morphology in maize (Zea mays, Poaceae). Econ Bot. (1991) 45:503–10. doi: 10.1007/BF02930714
26. Bolade MK. Evaluation of suitability of commercially available maize grains for ‘tuwo’ production in Nigeria. Afr J Food Sci. (2010) 4:371–81.
27. Miano AC, Augusto PED. The hydration of grains: a critical review from description of phenomena to process improvements. Compr Rev Food Sci Food Saf. (2017) 17:352–70. doi: 10.1111/1541-4337.12328
28. Sandhya Rani MR, Bhattacharya KR. Rheology of rice-flour pastes: effect of variety, concentration, and temperature and time of cooking. J Texture Stud. (1989) 20:127–37. doi: 10.1111/j.1745-4603.1989.tb00427.x
29. Hagenimana A, Ding X, Fang T. Evaluation of rice flour modified by extrusion cooking. J Cereal Sci. (2006) 43:38–46. doi: 10.1016/j.jcs.2005.09.003
30. Mishra S, Rai T. Morphology and functional properties of corn, potato and tapioca starches. Food Hydrocoll. (2006) 20:557–66. doi: 10.1016/j.foodhyd.2005.01.001
31. Gray D, Abdel-Aal EM, Seetharaman K, Kakuda Y. Differences in viscosity and textural properties of selected barley cultivars as influenced by pearling and cooking. Food Chem. (2010) 120:402–9. doi: 10.1016/j.foodchem.2009.10.020
32. Duarte G, Carvalho CWP, Ascheri JLR. Effect of soybean hull, screw speed and temperature on expanded maize extrudates. Braz J Food Technol. (2009) 12:205–12. doi: 10.4260/BJFT2009800900014
33. Sagbo FSY, Aissi MV, Hounkpati WA, Houedo C, Dansi A, Soumanou MM. Phyico-chemical and pasting properties of some local and improved maize genotypes cultivated in Benin. Int J Biol Chem Sci. (2017) 11:1753–65. doi: 10.4314/ijbcs.v11i4.27
34. Butt MS, Batool R. Nutritional and functional properties of some promising legumes proteins isolates. Pak J Nutr. (2010) 9:373–9. doi: 10.3923/pjn.2010.373.379
35. Kundu M, Gulia N, Khatkar BS. Relationship of dough rheological and pasting characteristics with chapatti quality of Indian wheat varieties. Int J Innov Res Sci Eng Technol. (2016) 5:17595–9.
36. Vaswani S, Kumar R, Kumar V, Roy D, Kumar M. Nutritional and mineral composition of different genotypes of normal and high quality protein maize fodder at post-cob stage. Int J Environ Sci Technol. (2016) 5:2719–27.
37. Sandhu KS, Singh N, Malhi NS. Some properties of corn grains and their flours I: physicochemical, functional and chapati-making properties of flours. Food Chem. (2007) 101:938–46. doi: 10.1016/j.foodchem.2006.02.040
38. Ullah I, Ali M, Farooqi A. Chemical and nutritionalproperties of some maize (Zea mays L.) genotypes grown in NWFP, Pakistan. Pak J Nutr. (2010) 9:1113–7. doi: 10.3923/pjn.2010.1113.1117
39. Kabir SH, Das AK, Rahman MS, Singh SK, Morshed M, Marma ASH. Effect of genotype on proximate composition and biological yield of maize (Zea mays L.). Arch Agric Environ Sci. (2019) 4:185–9. doi: 10.26832/24566632.2019.040209
40. Anjum FM, Ahmad L, Butt MS, Sheikh MA. Amino acid composition of spring wheats and losses of lysine during chapatti baking. J Food Comp Anal. (2005) 18:523–32. doi: 10.1016/j.jfca.2004.04.009
41. Gallego-Castillo S, Taleon V, Talsma EF, Rosales-Nolasco A, Palacios-Rojas N. Effect of maize processing methods on the retention of minerals, phytic acid and amino acids when using high kernel-zinc maize. Curr Res Food Sci. (2021) 4:279–86. doi: 10.1016/j.crfs.2021.03.007
42. Paes MCD, Maga J. Effect of extrusion on essential amino acids profile and color of whole-grain flours of quality protein maize (QPM) and normal maize cultivars. Rev Bras Milho Sorgo. (2004) 3:10–20. doi: 10.18512/1980-6477/rbms.v3n1p10-20
43. Kathuria P, Kaur G, Kanojia V. Physico-chemical and functional properties of whole maize flour blended with wheat and gram flour. Asian J Dairy Food Res. (2021) 40:301–8. doi: 10.18805/ajdfr.DR-1584
Keywords: Quality Protein Maize, landrace, mineral content, amino acid, unleavened flat bread, Indian traditional flat bread, chapatti
Citation: Kaur N, Kumar R, Singh A, Shobha D, Das AK, Chaudhary D, Kaur Y, Kumar P, Sharma P and Singh B (2022) Improvement in nutritional quality of traditional unleavened flat bread using Quality Protein Maize. Front. Nutr. 9:963368. doi: 10.3389/fnut.2022.963368
Received: 07 June 2022; Accepted: 24 October 2022;
Published: 23 November 2022.
Edited by:
Arun Jyoti Nath, Assam University, IndiaReviewed by:
Shuvasish Choudhury, Assam University, IndiaKarthikeyan Adhimoolam, Jeju National University, South Korea
Nemera Geleta Shargie, Agricultural Research Council of South Africa (ARC-SA), South Africa
Chandrakala Hanagi, University of Agricultural Sciences, India
Copyright © 2022 Kaur, Kumar, Singh, Shobha, Das, Chaudhary, Kaur, Kumar, Sharma and Singh. This is an open-access article distributed under the terms of the Creative Commons Attribution License (CC BY). The use, distribution or reproduction in other forums is permitted, provided the original author(s) and the copyright owner(s) are credited and that the original publication in this journal is cited, in accordance with accepted academic practice. No use, distribution or reproduction is permitted which does not comply with these terms.
*Correspondence: Ramesh Kumar, ramesh.kumar@icar.gov.in, rk.phagna@gmail.com