- 1The First School of Clinical Medicine, Zhejiang Chinese Medical University, Hangzhou, China
- 2Department of Medical Oncology, Hangzhou TCM Hospital of Zhejiang Chinese Medical University (Hangzhou Hospital of Traditional Chinese Medicine), Hangzhou, China
- 3Department of Medical Oncology, The First Affiliated Hospital of Zhejiang Chinese Medical University (Zhejiang Provincial Hospital of Traditional Chinese Medicine), Hangzhou, China
The peel and fruit of Citrus varieties have been a raw material for some traditional Chinese medicine (TCM). Pure total flavonoids from Citrus maxima (Burm.) Merr. (PTFC), including naringin, hesperidin, narirutin, and neohesperidin, have been attracted increasing attention for their multiple clinical efficacies. Based on existing in vitro and in vivo research, this study systematically reviewed the biological functions of PTFC and its components in preventing or treating liver metabolic diseases, cardiovascular diseases, intestinal barrier dysfunction, as well as malignancies. PTFC and its components are capable of regulating glycolipid metabolism, blocking peroxidation and persistent inflammation, inhibiting tumor progression, protecting the integrity of intestinal barrier and positively regulating intestinal microbiota, while the differences in fruit cultivation system, picking standard, manufacturing methods, delivery system and individual intestinal microecology will have impact on the specific therapeutic effect. Thus, PTFC is a promising drug for the treatment of some chronic diseases, as well as continuous elaborate investigations are necessary to improve its effectiveness and bioavailability.
Introduction
Citrus maxima (Burm.) Merr. (Changshanhuyou Tangelo) belongs to the genus Citrus of the Rutaceae family. It is a locally characteristic Citrus species planted in Changshan County, Zhejiang Province, China. Moreover, it refers to a hybrid between pomelo and sweet orange conducted by natural process and human intervention, which is considered a novel species retaining all the abilities of its “parents.” Geographical conditions (e.g., the humid monsoon climate and fertile red soil) contribute to the unique planting. Accordingly, Citrus varieties have been recognized as pillars of local agriculture with the largest output value in southeastern China. As the explorations on C. maxima (Burm.) Merr. have been deepened, its clinical efficacy in reducing accumulation and promoting Qi has been increasingly recognized, and it was officially accepted as a traditional Chinese medicine (TCM) in 2015. It is bitter, pungent, and sour with slight cold nature, as well as enter into spleen and stomach meridians. During the processing of C. maxima (Burm.) Merr., most of the pulp is removed for consumption, and the peel is fried to deep yellow, which acts as the valuable part enriching bioactive ingredients. The remaining parts of peel that are damaged, worm-eaten or wasted can be burned and used as soil fertilizer to nourish fruit trees.
The comprehensive development of Citrus species leads to an increase in economic income while triggering the transformation and upgrading of the traditional agricultural product. The rapid development of Citrus peel is primarily dependent on its massive availability and cost-effectiveness. Accumulating evidence suggests that there are abundant flavonoids with multiple beneficial effects in the peel of Citrus varieties (1). Epidemiological evidence suggests that the intake of food with high-level flavonoids and low-level fatty acids (e.g., fruit and vegetables) is directly correlated with a decreased risk of human diseases [e.g., cardiovascular diseases (CVD), diabetes, and cancers] (2–4). In addition, existing research has suggested that flavonoids play a vital role in the aging process by providing antioxidants and avoiding a vicious cycle of oxidative stress, tissue damage, and inflammatory processes (5). Nevertheless, the above effects have been controversial due to the individualized differences in the absorption and metabolism caused by the varied transformation of gut microbiota (6). A previous study has indicated that the urinary excretion level of flavonoid metabolism in healthy volunteers compared with the absorbed flavonoids reaches 45.9, 64.2, and 100%, respectively, implying that individualized differences in gut microbiota directly affects the bioavailability (7, 8). The result of high-performance liquid chromatography (HPLC) indicated that four pure flavonoids compounds exerted from Citrus maxima (Burm.) Merr. (PTFC) were found, including naringin, neohesperidin, narirutin, and hesperidin, and the content were 243.78 ± 2.69, 5.96 ± 0.06, 12.11 ± 0.12, and 136.04 ± 4.24 mg/g, respectively (9). Since PTFC is regarded as the major active ingredient of C. maxima (Burm.) Merr., the analysis of its biological functions is of great significance in clarifying the potential treatment fields. Accordingly, this study aims to systematically review the therapeutic efficiency and possible utilization of pure total flavonoids isolated and purified from the dry and ripe peels of C. maxima (Burm.) Merr., which is exhibited in Figure 1.
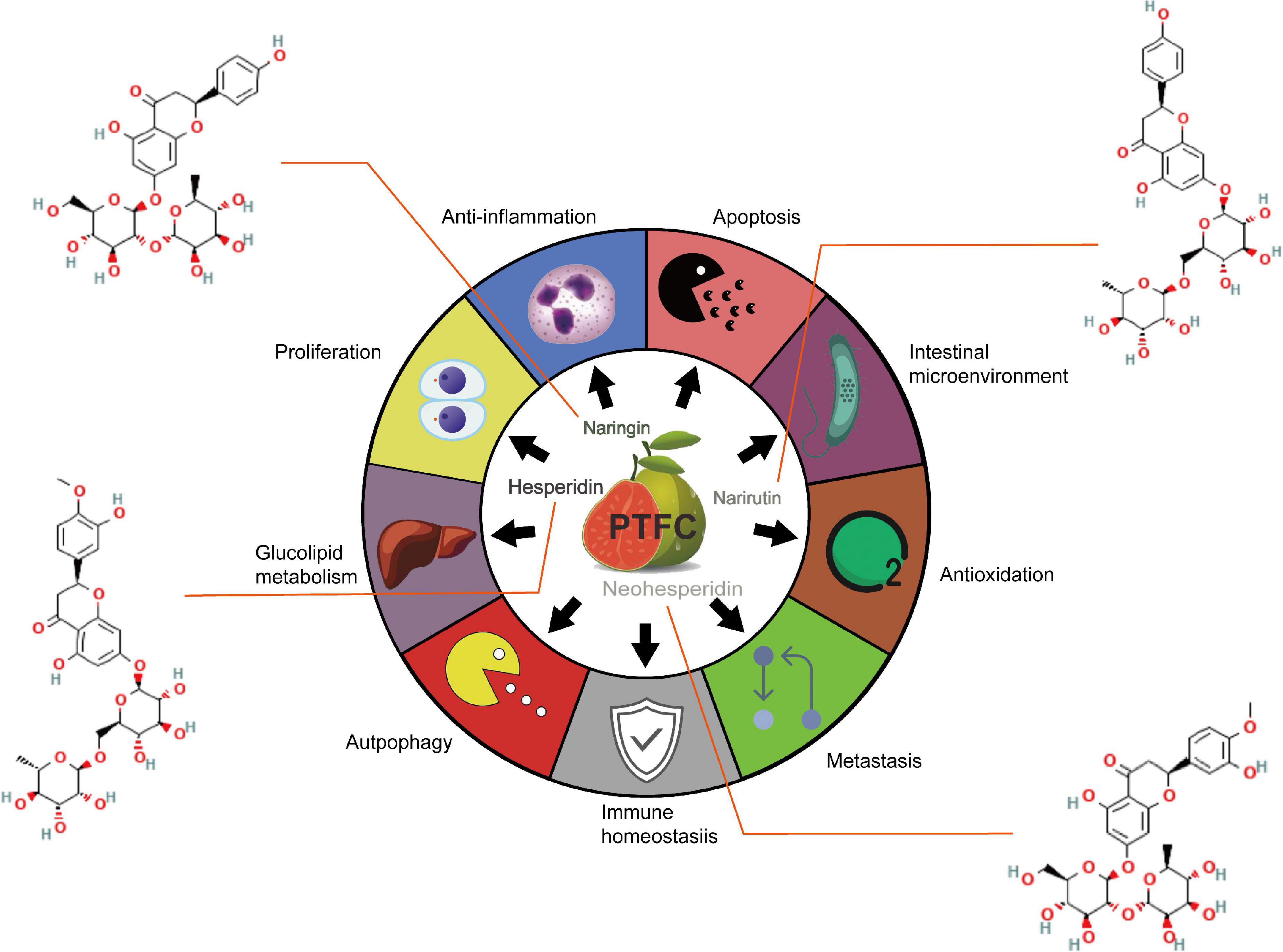
Figure 1. Summary of the therapeutic potentials of PTFC administration on hepatic metabolic disorder, cardiovascular events, malignancies development and intestinal barrier dysfunction.
Chemistry
Flavonoids are yellow pigments structured by two benzene rings (A and B) connected with a heterocyclic ring containing oxygen (C). The classification of subclasses is dependent on the degree of unsaturation and oxidation of the C ring (10). Flavanone (2,3-dihydro-2-phenylchromen-4-one) refers to the major class of flavonoids with the saturated double bond between positions 2 and 3. Flavanone exists in the forms of glycosides and aglycones. The components of PTFC belong to the former, in which naringin and neohesperidin belong to neohesperidosides, and narirutin and hesperidin belong to rutinosides. In general, the metabolism of glycosides comprises gastrointestinal hydrolysis and liver metabolism. The glucose, xylose, rhamnose, and other glycosyl are hydrolyzed in the gastrointestinal tract to form aglycones. Naringenin and hesperetin have been found as the critical flavanones in aglycone forms (11). Citrus taste properties comprise sweetness, bitterness, and sourness (12). The flavanone-7-O-neohesperidosides partly account for the bitter taste of naringin and neohesperidin, while the tasteless flavanone-7-O-rutinosides account for the non-bitter species, hesperidin and narirutin (13).
Technology
The extraction and utilization of effective fractions in Citrus have attracted wide attention based on the abundant germplasm resources of Citrus in southeast Asia. Water extraction is the earliest process used for extraction and exhibits the characteristic of low cost and high safety. Since the decoction should follow the rigorous standards of liquid-material ratio, soaking time, and extraction temperature, water extraction has been replaced by organic solution extraction. The addition of ethanol significantly increases the solubility of Citrus peel since most flavonoids have phenolic hydroxyl groups that are soluble and precipitated after acidification (14). Moreover, the leaching capacity of alkaline diluted alcohol is significantly lower than that of alkaline solution, which facilitates purification since it is not easily attached to impurities. To further transfer flavonoids from plants, Xiong et al. (15) used the above methods and obtained 14.65 g PTFC dry powder through soaking, heating, concentrating, separating, purifying, and drying processes. On that basis, the purity of total flavonoids of 88% and the yield of ketone extraction of 2.93% were achieved. After verification, the extraction requirements are confirmed as follows: Mass concentration of ethanol = 70%, solid-liquid ratio = 1:14, decoction time = 60 min and decoction for twice.
Later, Zeng et al. (16) proposed that the single ethanol method combined with ultrasonic-assisted extraction (UAE) and microwave-assisted extraction (MAE) as auxiliary techniques is capable of saving energy and time, and significantly increasing the yield of PTFC, thus revealing that the application of UAE facilitates the extraction process and reduces solvent consumption (17). MAE has also been well accepted for the extraction of bioactive compounds from non-edible parts due to the short time and less solvent consumption and the extracted amount of the above compounds is reported to be comparable to or higher than that of the edible part of the same plant (18, 19). In the future, more well-designed parameter settings are required, and technical research should be conducted to increase efficiency, reduce cost consumption, and protect environmental safety.
Exploration on biological function of pure flavonoids compounds exerted from Citrus maxima (Burm.) Merr.
The following sections summarize the existing main efficacy of PTFC, discussing its treatment on non-alcoholic liver disease, cancer, and intestinal barrier damage and related mechanisms based on studies listed in Table 1.
Non-alcoholic fatty liver disease
The unique metabolism of the liver and its correlation with the gastrointestinal tract take on a critical significance in the human physiological function. A wide variety of factors can lead to chronic liver damage (e.g., alcoholism, viral infections, obesity, diabetes, and dyslipidemia), eventually causing steatohepatitis and liver fibrosis (20). Steatosis characterized by the accumulation of lipid in hepatocytes primarily leads to non-alcoholic fatty liver disease (NAFLD) (21). Both oxidative stress and inflammatory response can be beneficial to the aggravation of NAFLD to non-alcoholic steatohepatitis (NASH) (22). Yang et al. (23) found that PTFC is capable of alleviating steatosis by reducing the body weight, lowing serum total cholesterol (TC), low-density lipoprotein cholesterol (LDL-C) and triglyceride (TG), and actively regulating peroxisome proliferator-activated receptor α (PPAR-α) in hyperlipidemic rats. Also, PTFC is capable of correcting dyslipidemia by regulating superoxide dismutase (SOD) and malondialdehyde (MDA) levels (24, 25), thus inhibiting lipid peroxidation. Jiang et al. found the beneficial effects of PTFC on NASH in two studies. They suggested that PTFC intervention can upregulate SIRTI-mediated deacetylated PGC-1α and SOD and downregulate MDA and 8-hydroxy-2′-deoxyguanosine (8-OHdG), which are biomarkers for lipid peroxidation and free radical level, respectively (26). In addition, they also confirmed that PTFC has hepatoprotective and anti-inflammatory functions by inhibiting phosphorylated NF-κB and MAPKs, as well as the abnormal activation of proinflammatory factors (e.g., IL-1β, IL-6, IL-12, TNF-α, and IFN-γ) (27). Furthermore, PTFC is conductive to maintaining the balance of Th17/Treg expressions and their transcriptional regulators RORγt and Foxp3 (28), which relieves the intrahepatic inflammatory response by limiting the immunopathological reaction and inhibiting the secretion of IL-17 and IL-10 (29).
Above all, existing research has concluded that the potential efficiency of PTFC can arise from the regulatory effect on lipid metabolism, anti-oxidation, and anti-inflammation properties. PTFC can be a curative agent for preventing NAFLDs.
Cancer
Malignancies are initiated by the destruction of DNA replication and aggravated by immune escape, which further results in distant metastasis. Previous research has demonstrated that dietary intake of flavonoids is beneficial to reduce the risk of malignancies (30). Dai et al. (31) uncovered that PTFC invention dose-dependently and time-dependently inhibits proliferation of neoplastic cells and triggers cell apoptosis by upregulating activated poly adenosine diphosphate-ribose polymerase and downregulating Mcl-1. Overexpression of XIAP is common in carcinomas, usually suggesting an unfavorable response to chemotherapy, increased recurrence rate and shorter overall survival. Wu et al. (32) demonstrated that PTFC at a particular concentration range can downregulate XIAP, and the inhibition promotes apoptosis of cancer cells by activating caspases-3, -7, and -9.
Nearly the whole approaches target to remove or kill the neoplastic cells to control the tumor growth and to minimize the number of metastatic cells. These results revealed that PTFC is beneficial to inhibit cell proliferation and induce apoptosis in malignancies, and not involved in the resistance to cancer cell migration and invasion, which needs to be supplemented by more experimental and clinical evidences.
Intestinal barrier damage
The gastrointestinal tract plays a critical role in regulating the immune homeostasis since it exhibits the largest interface in the body (33). It is accepted that intestinal barrier is the first line of defense against the entry of pathogenic microorganisms into human intestines. Due to the long-term administration of non-steroidal anti-inflammatory drugs (NSAIDs) for tumor chemoprophylaxis and cardiocerebrovascular diseases, compounds and their metabolites are subjected to a prolonged and repeated exposure of the intestinal mucosa, thereby impairing the intestinal barrier (34). Toxins, bile acids, and proteolytic enzymes all aggravate the intestinal barrier with the increase in intestinal mucosal permeability (35). Chen et al. (9) applied PTFC to rats with NSAID-induced small intestine injury and observed that Atg5, LC3-II, TJ, and the LC3-II/LC3-I ratio are upregulated, while p-PI3K and p-Akt are downregulated, suggesting that PTFC maintains the intestinal barrier integrity by promoting autophagy through the PI3K/AKT signaling pathway. They also claimed that PTFC intervention significantly reduces the content of toxic bile acids (e.g., TDCA, DCA, TCA, and CA), and enriches the phylogenetic diversity of gut microbiota in high-fat diet (HFD)-induced rats (e.g., Bacteroidaceae and Christensenellaceae) (36). That is to say, PTFC has a promising role in protecting human intestinal barrier, and its major effects include optimization of barrier permeability, positive balance of gut microbiota, and improvement of bile acid metabolism, which are considered to be vital in intestinal immunomodulation.
Extension of pure flavonoids compounds exerted from Citrus maxima (Burm.) Merr. treatment based on its components
According to the above exploration of PTFC treatment, the following sections further excavate the promising effects of its components on liver damage, NAFLD-associated CVDs, malignancies, and intestinal microecological disorder based on studies listed in Table 2, providing new perspectives on the potential functions of PTFC. All the related mechanisms are shown in Figure 2.
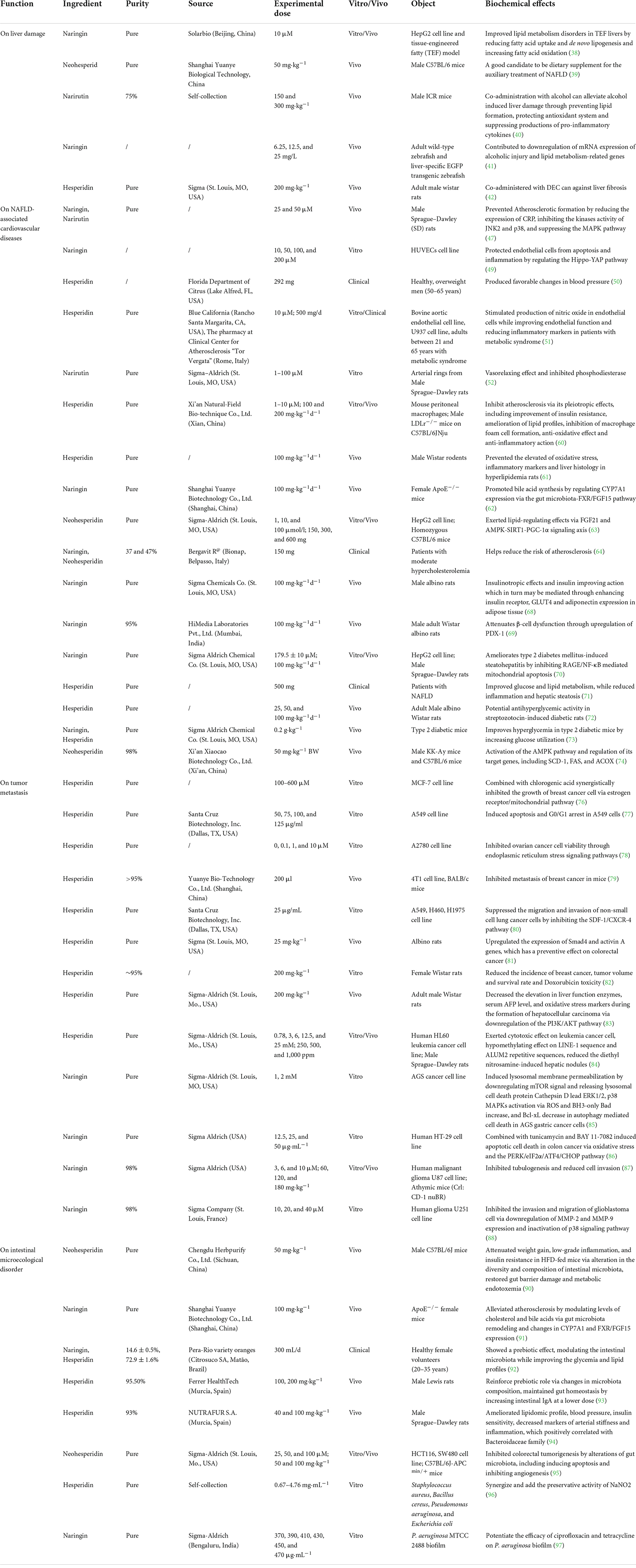
Table 2. Summary of biochemical effects exerted by PTFC components, including naringin, hesperidin, neohesperidin, and narirutin.
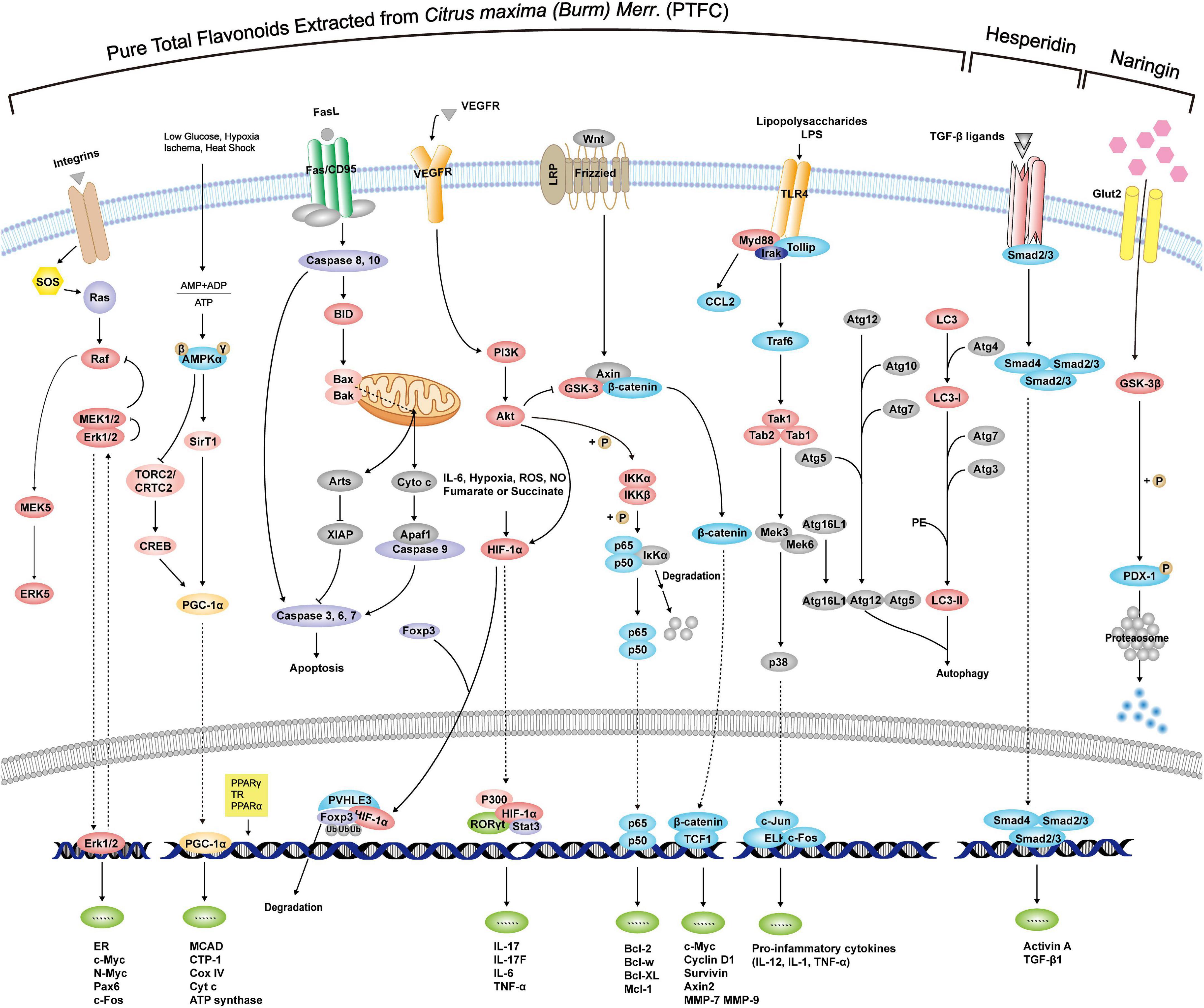
Figure 2. Mechanisms of PTFC and its components through interference with signaling pathways exerting effects of anti-oxidation, anti-inflammation, anti-cancer, and glycolipid metabolism.
Liver damage
Studies have shown that the prevalence of a HFD, a sedentary lifestyle and alcoholic abuse are the risk factors for liver damage (37). The therapeutic effects of PTFC on liver metabolism affected by overnutrition has been achieved. Furthermore, it is found that naringin could reduce TG and very low-density lipoprotein (VLDL) in the fatty liver model by activating PPAR-α (38), and neohesperidin could facilitate PGC-1α-mediated mitochondrial biogenesis, thus alleviating hepatic steatosis in mice fed with a HFD (39), which further highlights the main contributor in PTFC efficacy.
On the other hand, the effects of narirutin, naringin, and hesperidin on alcoholic liver diseases has also been reported, providing new references for expanding the therapeutic field of PTFC treatment. In mice with a long-term consumption of alcohol, narirutin intake can reduce the serum transaminase, pro-inflammatory cytokines, TG, and TC (40). Zhou et al. confirmed that naringin protects against alcohol-induced liver damage by reducing lipid accumulation and oxidative stress (41). The combination of hesperidin with an anti-inflammatory drug synergistically inhibits alcoholic liver fibrosis (42). As a result, the treatment of PTFC components prevents steatosis caused by chronic alcohol ingestion, thus protecting alcoholic liver diseases. It is therefore suggested that PTFC alleviates alcoholic-induced liver diseases by preventing excessive lipid formation and protecting the antioxidant system, which should be validated in depth through animal studies and clinical trials.
Non-alcoholic fatty liver disease-associated cardiovascular diseases
Non-alcoholic fatty liver disease increases the risk of CVD, and it has been found as the most common cause of death of non-alcoholic liver diseases (43). Myocardial infarction, stroke, and revascularization are common risks of CVD, which are significantly correlated with NAFLD (44). However, a post hoc analysis indicated that the hazard risk of NAFLD population with body mass index ≥23 kg/m2 for incident CVD is lower than those non-obese population (45), thus suggesting that myocardial infarction or stroke-induced vascular dysfunction is likely to be the pivotal element for CVD rather than obesity. Atherosclerosis in NASH patients is usually confirmed through the measurement of calcification and aortic stiffness (46). Naringin and narirutin are found to block the formation of scar tissue and vascular stenosis in atherosclerosis cases by downregulating pro-inflammatory factors (47). Similarly, naringin is able to prevent endothelial dysfunction with a decrease in the expressions of inflammatory cytokines (IL-6, TNF-α, and IL-1β) via Nox4/NF-κB, PI3K/AKT and Hippo-YAP pathways, thus relieving atherosclerosis (48, 49). Existing research has found that hesperidin intervention leads to favorable changes in endothelium-dependent microvascular reactivity by decreasing the concentrations of circulating inflammatory biomarkers (e.g., high-sensitivity C-reactive protein, serum amyloid A protein, and soluble E-selectin) and regulating nitric oxide bioactivity (50, 51). Narirutin relaxes the vascular system by stimulating the phosphorylation of endothelial nitric oxide synthase and voltage-dependent potassium current in arterial smooth muscle cells (52). Accordingly, the effects of PTFC compounds on NAFLD-associated CVD are primarily attributed to the inhibition of systematic inflammation. The persistent inflammatory responses trigger the aggravation of hepatic steatosis, NASH to liver fibrosis, thus significantly increasing the risk of CVD. An early intervention for NASH may reduce the incidence of cardiovascular events. Liver biopsy has been employed as the gold standard for the diagnosis of NASH though it is not highly acceptable due to the invasive examination and high medical costs. Although emerging blood makers and non-invasive imaging methods have been applied to the diagnosis of fibrosis and cirrhosis (53, 54), steatosis identification and inflammation evaluation should be conducted to make the progression of NASH. There have been rare typical manifestations of early stage NASH, so it is urgent to search for a reliable and accurate diagnostic method.
Interestingly, abnormal lipid metabolism and its transportation trigger the development of atherosclerosis (55, 56). NAFLD-induced excessive lipid uptake results in the abundant synthesis of hepatic TG and overproduction of VLDL particles assisting the mobilization of liver fat to peripheral tissues (57, 58). The accumulation of VLDL and TG on the arterial wall, alongside inflammation response and oxidative stress, leads to a heavy burden of atherosclerotic plaques (59). Free fatty acids (FFAs) are the product of lipolysis in the portal circulation. Sun et al. (60) built a lipoprotein receptor deficient model in atherosclerosis mice fed with HFD and discovered that hesperidin can suppress the formation of atherosclerotic plaque and macrophage foam cells by inhibiting the activities of enzymes involved in hepatic FFAs and TG synthesis, thus further suppressing the cholesterol transportation from peripheral tissues to the liver and then preventing hepatic steatosis. Also, hesperidin could maintain the redox homeostasis and prevent lipidemic stress in hyperlipidemia rats (61). Wang et al. (62) suggested that naringin more significantly mitigates atherosclerosis than hesperidin (55.92% vs. 42.87%). Their data revealed that the concentration of naringin in the gastrointestinal tract is higher than that of hesperidin, and a small amount of naringin in the liver is subsequently absorbed. Naringin facilitates the transformation from cholesterol to bile acids and promots their excretion from the liver by regulating the gut microbiota structure. Moreover, some naringin absorbed in the liver facilitates the reverse transport of cholesterol (62). Neohesperidin consistently exerts a potent hypolipidemic effect on HepG2 cells enriched in FFAs and reverses lipid-related pathological changes in the acute or chronic dyslipidemia mouse model (63). A kind of citrus juice containing neohesperidin and naringin significantly alleviates subclinical atherosclerosis by reducing lipoprotein content and carotid intima-media thickness within 6 months (64).
Increasing data have indicated that obesity is not the necessary factor for cardiovascular events in NAFLD population, but boosts the development of atherosclerotic plaques to a certain extent. Notably, NAFLD population with dyslipidemia is highly risky for cardiovascular events. The ingredients of PTFC, especially hesperidin and naringin, possess therapeutic efficiency on lipid deposition and adiposity, which dramatically inhibit the formation of atherosclerosis via blocking the secretion and transport of redundant lipid.
Glucose abnormality induced by insulin resistance and beta cell dysfunction is a hallmark of NAFLD, which takes on a great significance in the pathogenesis of CVD (65, 66). Elevated FFAs released by enlarged adipose mass remarkably restrict the anti-lipid effect of insulin and even lead to insulin resistance, which in turn aggravates dyslipidemia and pathoglycemia (67). Naringin exerts the insulinotropic effect by increasing the expression levels of insulin receptors and adiponectin in adipose tissues (68). Also, naringin attenuates mellitus-mediated steatohepatitis by upregulating the transcription factor PDX-1 that regulates insulin secretion and maintains β-cells mass (69), and inhibiting hyperglycemia-mediated oxidative stress and pro-inflammatory cytokine secretion (70). Yari et al. (71) suggested that hesperidin supplementation controls fatty liver indexes by inhibiting inflammation, while enhancing insulin sensitivity and fasting glucose. Meanwhile, hesperidin and naringin contribute to glucose uptake by enhancing hepatic glycolysis and glycogen content, and lowering hepatic gluconeogenesis (72, 73). Neohesperidin suppresses fat accumulation and reduces the size of adipocytes, which further improves oral glucose tolerance and insulin sensitivity (74).
A conclusion is drawn that, PTFC, at least in part, plays a positive role in the treatment of CVDs by suppressing vascular senescence, improving lipid profile and maintaining glucose homeostasis for its active ingredients. Moreover, their activities of controlling persistent inflammatory response and oxidative stress facilitate the prevention of CVD. However, inconsistent results on glucolipid metabolism have been obtained in some randomized controlled trials (RCTs). Motallaei et al. (75) reported that the meta-analysis on the intake of orange beverage associated with relieving serum TC and insulin resistance is poorly qualified, which fails to show a significant effect of citrus drinks on cardiometabolic risk factors. The above phenomenon can be explained by the poor bioavailability of flavonoids in the human body. Since the absorption of flavonoids focuses on the colon, individualized differences in gut microbiota compositions and activities result in varied therapeutic efficiency. Therefore, in addition to early intervention of steatosis to prevent CVD, it is also essential to mediate the intestinal microecology, which can facilitate the absorption of PTFC.
Tumor metastasis
The growth of the primary tumors often do not pose major health threats except for those growing in sensitive and restrictive organs, such as the brain. Transformation into a cell capable of metastasis, acquiring the capabilities to escape the primary tumor, to enter vascular systems, to invade, and to colonize secondary organs, is more of a concern. Hesperidin is reported to control tumor growth by regulating mitochondria production (76), arresting cell cycle progression (77), and inhibiting cell viability via the endoplasmic reticulum stress signaling pathway (78). It also can inhibit the metastatic potential by suppressing metastases growth in vivo and cell migration and invasion in vitro (79, 80). Existing research indicated that hesperidin contributes to chemotherapy by modulating Smad4 and the activin A signaling in colon cancer (81), and downregulating Ki-67 expression in breast cancer (82). During the process of diethyl nitrosamine-induced hepatocellular carcinoma, the addition of hesperidin preserves liver tissue integrity, improves liver function (83), and exerts a hypomethylating effect (84). As the major component in PTFC, naringin is considered an anti-tumor agent and adjuvant in the combination therapy by inducing lysosomal permeabilization and autophagy in gastric cancer (85). It induces mitochondrial and cellular apoptosis in colon cancer via inhibiting NF-κB and endoplasmic reticulum stress (86). Though downregulating MMP-2 and MMP-9 and inactivating the p38 signaling, naringin inhibits angiogenesis and cell invasion in glioblastoma cancer (87, 88).
Due to the anti-tumor capacities of hesperidin and naringin in inhibiting metastatic potential, blocking neovascularization, and strengthening chemical protection, the anti-tumor function of PTFC appears to be consistent and reliable. Considering some uncertain aspects of cancer treatment, more in vivo and in vitro studies are needed to validate the anti-tumor effect of PTFC.
Intestinal microecological disorder
Besides the physical barrier created by intestinal epithelial cells, the symbiotic relationship between intestinal microbiota and host also contributes to the intestinal immunity. The gut microbiota complements human genome functions based on its wide range of metabolic properties. However, the individualized difference in gut microbiota changes significantly due to diet, antibiotic use, and lifestyle (89).
It is found that neohesperidin administration changes the structure of gut microbiota by decreasing the intestinal ratio of Firmicutes to Bacteroidetes and enhances gut barrier integrity by mitigating serum metabolic endotoxemia in obese mice, which is reversed by the antibiotic treatment. Increasing evidence suggested that the profitable effect of neohesperidin on the obese population is largely dependent on gut microbiota (90). Naringin alleviates atherosclerosis by modulating the abundances of Bifidobacterium, Bacteroidetes, Clostridium, and Eubacterium (91). Fidélix et al. (92) revealed that the absorption and metabolism of hesperidin and naringin increase the abundance of probiotics, thus ameliorating the glycemia and lipid profiles. The immunomodulatory effect of hesperidin on the gut-associated lymphoid tissue is achieved by increasing the proportions of Lactobacillus and Bifidobacterium (93). Furthermore, an increased dose of hesperidin supplementation reduces the risk of CVD by modulating the metabolism of the Bacteroidaceae family (94). Feeding with fecal of neohesperidin-treated mice yielded a considerable inhibition of colon cancer, suggesting that the adjustment of neohesperidin on gut microbiota may be a promising strategy for cancer (95).
Notably, knowledge of PTFC compounds as antimicrobial agent is equally attractive. The current research indicates that hesperidin combining with widely used NaNO2 has synergistic antibacterial activity against Bacillus cereus, Staphylococcus aureus, Escherichia coli, and Pseudomonas aeruginosa (96), showing the advantages of nutrients as safe natural bio-preservatives to reduce the hazards of overuse of chemical preservatives. Ciprofloxacin and tetracycline are antibiotics for P. aeruginosa, and the addition of naringin potentiates their efficacies to further manage bacterial infection (97). As for methicillin-resistant S. aureus that has developed resistance against most of the antibiotics and resulted in life-threatening outbreaks, naringin, hesperidin and neohesperidin are all strongly supported to be the adjuvant antimicrobial agent via the docking interactions (98).
Overall, the regulatory effect of its components on raising the proportion of beneficial bacteria implies the improvement of PTFC on chronic diseases may begin from the impact of its components on gut microbiota, and protecting intestinal microecological homeostasis can be the priority of human health. Based on the efforts on bacteriostasis by its components, the positive effect of PTFC on intestinal immunity has been affirmed again. Regional factors and personal habits lead to individualized differences in the dynamic equilibrium of intestinal microecology, which may be eliminated by the long-term administration of PTFC through regulating the structure of intestinal microbiota or clearing relevant pathological factors.
Bioavailability
Most flavonoids are extensively absorbed in the intestine and then transported to the liver for the further metabolization. The metabolites formed in the liver can re-enter enterohepatic circulation through hydrolysis of bile excretion to aglycones via gut microbiota or being directly excreted in urine or feces (99). However, flavonoids are poorly absorbed through the gastrointestinal tract (100), and great efforts have been made on enhancing the bioavailability of flavonoids by inhibiting relevant enzymes, altering food intakes, and increasing dissolution rate (101–103). Xia et al. (104) demonstrated that neohesperidin modified with an immobilized lipase (40 mg/ml catalyst, 50°C of reaction temperature and 18 h of reaction time) can enhance the lipophilicity, thus improving the applicability in lipophilic media and enhance bioavailability in vivo.
Additionally, the low activity of α-rhamnosidase serves as a limiting step for hesperidin degraded by colonic microbiota (105, 106). Pereira-Caro et al. (107) reported that Lactobacillus rhamnosus exhibits the rhamnosidase activity, which is cooperated with Bifidobacterium in the in vivo colonic catabolism of hesperidin. They also highlighted that the chronic intake of Bifidobacterium is significantly beneficial to the enhancement of hesperidin bioavailability (108), suggesting that the long-term intake of PTFC assists the expansion of probiotic communities, also in turn promotes the absorption of PTFC. Unlike the large difference of hesperidin content in PTFC, plasma concentration of narirutin is significantly lower than that of hesperidin (109). The α-rhamnosidase serves as the catalyst to boost the absorption of naringin via the conversion from rutinoside to glucoside (110). Accordingly, α-rhamnosidase activity is still the critical issue to limit bioavailability. The commensal intestinal microecology and its substantial gene pool has been validated to significantly regulate the bioavailability and metabolism of nutrients (111). In addition to increasing the activity of α-rhamnosidase, microbiome profiling combined with powerful machine learning algorithms can be employed to select more appropriate biomarkers for flavonoid metabolism from microorganisms, thus enhancing the clinical response and efficacy to PTFC.
A clinical study suggested that the solubility of hesperidin in juice is a vital factor for bioavailability since its excretion and maximal plasma concentration are correlated with the soluble hesperidin concentration in juice, instead of the total hesperidin intake (6, 112). As a result, encapsuling hesperidin by the nanotechnology is confirmed as a promising strategy to enhance the bioavailability of hesperidin. Also, a reduced particle size facilitates the interaction with intestinal cells and gut microbiota, thus weakening the demand of α-rhamnosidase hydrolysis (113). Meanwhile, the nanoparticulate systems were employed for the naringin formulations to prevent drug cleavage in the lumen or the gut under harsh pH and enzymatic conditions of gastrointestinal tract, providing a sustained delivery of naringin (114).
That is to say, nanotechnology is capable of increasing the encounter area and reducing gastric lysis, which can be extensively used to modulate the release and absorption of bioactive fractions. What’s more, its physicochemical properties should be further modified to enhance the solubility and permeability with the use of nanotechnology. As a result, efforts should be made to assess the effects of nanotechnology on the metabolism, bioavailability, and efficacy of PTFC.
According to studies on the comparison of various Citrus fruits (115), the difference of efficacy lies in the total flavonoids content and the main component object. It is reported that the kind of C. maxima (Burm.) Merr. has the relatively stronger anti-inflammation, anti-oxidant and bactericidal effects due to it contains a large amount of naringin and hesperidin (116, 117). The total flavonoids content is found to increase with maturity stages (118), so standard planning for the shape, weight and skin luster of C. maxima (Burm.) Merr. before picking may be the new focus associated with the absorption of nutrients.
Conclusion
In brief, the effects of lipid-lowering, anti-inflammation, anti-oxidation, anti-cancer, anti-bacterial, and intestinal barrier protection of PTFC were reviewed and novel prospects were put forward in accordance with the findings on its components. Besides, paying attention to the effects of PTFC on gut microbiota may contribute to the enhancement of therapeutic efficacy, while well-designed experiments and clinical trials are still required to further clarify the specific application of PTFC in clinical practices. Meanwhile, it is found that the mature stage of raw material affects the total flavonoids content, the manufacturing methods affects PTFC purity, and delivery system and individual intestinal microecology affect the specific bioavailability. Thus, optimization on fruit cultivation system and picking standard, and more elaborate investigations on facilitating their controlled release and actual potency in blood should be further conducted to address the poor bioavailability, as well as effective productive and easier methods to separate and extract PTFC should be explored to improve purity.
To the best of our knowledge, this study has summarized the extraction technology, chemical properties, and biological effects of PTFC, which may boost the development of their biological profiles in human disease treatment. Of course, studies on pharmacokinetic parameters, toxicity testing, effective dose assessment, and adverse reaction are of significance before PTFC is officially used as a clinical therapeutic agent.
Author contributions
SD and PW conducted the data disposal. XP, LZ, and LQ wrote the manuscript. XJ and WC participated in the discussion. SD, PW, SR, and LS revised and edited the manuscript. All authors have read and agreed to the published version of the manuscript.
Funding
This work was supported by the Natural Science Foundation of Zhejiang Province (LS, No. LQ22H270008), National Natural Science Foundation Youth Fund (LS, No. 82204824), Young Elite Scientists Sponsorship Program by CACM (LS, No. 2021-QNRC2-B13), “the Postgraduate Scientific Research Fund” of Zhejiang Chinese Medical University (PW, No. 2021YKJ01), and Top-Notch Innovative Talents for Postgraduates of Zhejiang Chinese Medical University in 2022 (SD, No. 721100G00730).
Acknowledgments
We thank the specialists from The First Affiliated Hospital of Zhejiang Chinese Medical University and Zhejiang Chinese Medical University for their support to this work.
Conflict of interest
The authors declare that the research was conducted in the absence of any commercial or financial relationships that could be construed as a potential conflict of interest.
Publisher’s note
All claims expressed in this article are solely those of the authors and do not necessarily represent those of their affiliated organizations, or those of the publisher, the editors and the reviewers. Any product that may be evaluated in this article, or claim that may be made by its manufacturer, is not guaranteed or endorsed by the publisher.
Abbreviations
PTFC, pure total flavonoids extracted from Citrus maxima (Burm.) Merr.; TCM, traditional Chinese medicine; HPLC, high-performance liquid chromatography; UAE, ultrasonic-assisted extraction; MAE, microwave-assisted extraction; NAFLD, non-alcoholic fatty liver disease; NASH, non-alcoholic steatohepatitis; TC, total cholesterol; LDL-C, low-density lipoprotein cholesterol; TG, triglyceride; PPAR-α, peroxisome proliferator-activated receptor α; SOD, superoxide dismutase; MDA, malondialdehyde; 8-OHdG, 8-hydroxy-2′-deoxyguanosine; VLDL, very low-density lipoprotein; HFD, high-fat diet; VEGF-C, vascular endothelial growth factor-C; CVD, cardiovascular disease; FFAs, free fatty acids; NSAIDs, non-steroidal anti-inflammatory drugs.
References
1. Nair SA, Sr RK, Nair AS, Baby S. Citrus peels prevent cancer. Phytomedicine. (2018). 50:231–7. doi: 10.1016/j.phymed.2017.08.011
2. Ottaviani JI, Heiss C, Spencer JPE, Kelm M, Schroeter H. Recommending flavanols and procyanidins for cardiovascular health: revisited. Mol Aspects Med. (2018) 61:63–75. doi: 10.1016/j.mam.2018.02.001
3. Hazafa A, Rehman KU, Jahan N, Jabeen Z. The role of polyphenol (flavonoids) compounds in the treatment of cancer cells. Nutr Cancer. (2020) 72:386–97.
4. Dinda B, Dinda M, Roy A, Dinda S. Dietary plant flavonoids in prevention of obesity and diabetes. Adv Protein Chem Struct Biol. (2020) 120:159–235.
5. Jiang J, Shan L, Chen Z, Xu H, Wang J, Liu Y, et al. Evaluation of antioxidant-associated efficacy of flavonoid extracts from a traditional Chinese medicine Hua Ju Hong (peels of Citrus grandis (L.) Osbeck). J Ethnopharmacol. (2014) 158:158 Pt A:325–30. doi: 10.1016/j.jep.2014.10.039
6. Aschoff JK, Riedl KM, Cooperstone JL, Högel J, Bosy-Westphal A, Schwartz SJ, et al. Urinary excretion of Citrus flavanones and their major catabolites after consumption of fresh oranges and pasteurized orange juice: a randomized cross-over study. Mol Nutr Food Res. (2016) 60:2602–10. doi: 10.1002/mnfr.201600315
7. Bao W, Bowers K, Tobias DK, Olsen SF, Chavarro J, Vaag A, et al. Prepregnancy low-carbohydrate dietary pattern and risk of gestational diabetes mellitus: a prospective cohort study. Am J Clin Nutr. (2014) 99:1378–84.
8. Pereira-Caro G, Polyviou T, Ludwig IA, Nastase AM, Moreno-Rojas JM, Garcia AL, et al. Bioavailability of orange juice (poly)phenols: the impact of short-term cessation of training by male endurance athletes. Am J Clin Nutr. (2017) 106:791–800. doi: 10.3945/ajcn.116.149898
9. Chen S, Jiang J, Chao G, Hong X, Cao H, Zhang S. Pure total flavonoids from Citrus protect against nonsteroidal anti-inflammatory drug-induced small intestine injury by promoting autophagy in vivo and in vitro. Front Pharmacol. (2021) 12:622744. doi: 10.3389/fphar.2021.622744
10. Wang TY, Li Q, Bi KS. Bioactive flavonoids in medicinal plants: structure, activity and biological fate. Asian J Pharm Sci. (2018) 13:12–23. doi: 10.1016/j.ajps.2017.08.004
11. Najmanová I, Vopršalová M, Saso L, Mladìnka P. The pharmacokinetics of flavanones. Crit Rev Food Sci Nutr. (2020) 60:3155–71.
12. Lubinska-Szczygeł M, Polkowska Ż, Dymerski T, Gorinstein S. Comparison of the physical and sensory properties of hybrid Citrus fruit Jaffa(®) sweetie in relation to the parent fruits. Molecules. (2020) 25:2748. doi: 10.3390/molecules25122748
13. Frydman A, Weisshaus O, Bar-Peled M, Huhman DV, Sumner LW, Marin FR, et al. Citrus fruit bitter flavors: isolation and functional characterization of the gene Cm1,2RhaT encoding a 1,2 rhamnosyltransferase, a key enzyme in the biosynthesis of the bitter flavonoids of Citrus. Plant J. (2004) 40:88–100. doi: 10.1111/j.1365-313X.2004.02193.x
14. Zheng YZ, Zhou Y, Liang Q, Chen DF, Guo R. A theoretical study on the hydrogen-bonding interactions between flavonoids and ethanol/water. J Mol Model. (2016) 22:95. doi: 10.1007/s00894-016-2968-2
15. Xiong Y, Jiang J, Zhang C, Xu C. Preparation Process and Application of Pure Total Flavonoids From Citrus Changshan-Huyou. CN. Patent No CN102302592B. Hangzhou: China National Intellectual Property Administration (2021).
16. Zeng H, Xu T, Shi L, Lv L, Li J. Study on the technology of extracting flavonoids from huyou pomelo peel and comprehensive utilization of residues. J Anhui Agric Sci. (2019) 47:185–7.
17. Pham DC, Nguyen HC, Nguyen TL, Ho HL, Trinh TK, Riyaphan J, et al. Optimization of Ultrasound-assisted extraction of flavonoids from Celastrus hindsii leaves using response surface methodology and evaluation of their antioxidant and antitumor activities. Biomed Res Int. (2020) 2020:3497107. doi: 10.1155/2020/3497107
18. Bagade SB, Patil M. Recent advances in microwave assisted extraction of bioactive compounds from complex herbal samples: a review. Crit Rev Anal Chem. (2021) 51:138–49. doi: 10.1080/10408347.2019.1686966
19. Chaves JO, de Souza MC, da Silva LC, Lachos-Perez D, Torres-Mayanga PC, Machado A, et al. Extraction of flavonoids from natural sources using modern techniques. Front Chem. (2020) 8:507887. doi: 10.3389/fchem.2020.507887
20. Dhanasekaran R, Felsher DW. A tale of two complications of obesity: NASH and hepatocellular carcinoma. Hepatology. (2019) 70:1056–8. doi: 10.1002/hep.30649
21. Malhotra P, Gill RK, Saksena S, Alrefai WA. Disturbances in cholesterol homeostasis and non-alcoholic fatty liver diseases. Front Med (Lausanne). (2020) 7:467. doi: 10.3389/fmed.2020.00467
22. Pierantonelli I, Svegliati-Baroni G. Nonalcoholic fatty liver disease: basic pathogenetic mechanisms in the progression from NAFLD to NASH. Transplantation. (2019) 103:e1–13. doi: 10.1097/TP.0000000000002480
23. Yang PL, Jiang JP, Li QQ, Wu XM, Chen ZY. Effects of pure total flavonoids from Citrus changshan-huyou on blood lipid metabolism in hyperlipidemic rats. Zhongguo Zhong Yao Za Zhi. (2017) 42:936–43. doi: 10.19540/j.cnki.cjcmm.20170121.008
24. Yachida S, Mizutani S, Shiroma H, Shiba S, Nakajima T, Sakamoto T, et al. Metagenomic and metabolomic analyses reveal distinct stage-specific phenotypes of the gut microbiota in colorectal cancer. Nat Med. (2019) 25:968–76. doi: 10.1038/s41591-019-0458-7
25. Moore M, Gill S, Asmis T, Berry S, Burkes R, Zbuk K, et al. Randomized phase II study of modified FOLFOX-6 in combination with ramucirumab or icrucumab as second-line therapy in patients with metastatic colorectal cancer after disease progression on first-line irinotecan-based therapy. Ann Oncol. (2016) 27:2216–24. doi: 10.1093/annonc/mdw412
26. Chen ZY, Li JS, Jiang JP, Yan MX, He BH. Effect of pure total flavonoids from Citrus on hepatic SIRT1/PGC-1alpha pathway in mice with NASH. Zhongguo Zhong Yao Za Zhi. (2014) 39:100–5.
27. Jiang J, Yan L, Shi Z, Wang L, Shan L, Efferth T. Hepatoprotective and anti-inflammatory effects of total flavonoids of Qu Zhi Ke (peel of Citrus changshan-huyou) on non-alcoholic fatty liver disease in rats via modulation of NF-κB and MAPKs. Phytomedicine. (2019) 64:153082. doi: 10.1016/j.phymed.2019.153082
28. Li JS, Chen ZY, Jiang JP, He BH. Regulation of pure total flavonoids from Citrus on TH17/Treg balance in mice with NASH. Zhongguo Zhong Yao Za Zhi. (2015) 40:2644–8.
29. Lee GR. The balance of Th17 versus treg cells in autoimmunity. Int J Mol Sci. (2018) 19:730. doi: 10.3390/ijms19030730
30. Li G, Ding K, Qiao Y, Zhang L, Zheng L, Pan T, et al. Flavonoids regulate inflammation and oxidative stress in cancer. Molecules. (2020) 25:5628. doi: 10.3390/molecules25235628
31. Dai TY, Wang B, Lin SY, Jiang JP, Wu LQ, Qian WB. Pure total flavonoids from Citrus paradisi Macfad induce leukemia cell apoptosis in vitro. Chin J Integr Med. (2017) 23:370–5. doi: 10.1007/s11655-016-2593-z
32. Wu L, Zhang X, Lin X, Wang B, Huang C, Qin Y, et al. Inhibition of X-linked inhibitor of apoptosis protein enhances anti-tumor potency of pure total flavonoids on the growth of leukemic cells. Exp Ther Med. (2018) 15:2020–6. doi: 10.3892/etm.2017.5627
33. Takiishi T, Fenero CIM, Câmara NOS. Intestinal barrier and gut microbiota: shaping our immune responses throughout life. Tissue Barriers. (2017) 5:e1373208.
34. Utzeri E, Usai P. Role of non-steroidal anti-inflammatory drugs on intestinal permeability and nonalcoholic fatty liver disease. World J Gastroenterol. (2017) 23:3954–63. doi: 10.3748/wjg.v23.i22.3954
35. van de Peppel IP, Verkade HJ, Jonker JW. Metabolic consequences of ileal interruption of the enterohepatic circulation of bile acids. Am J Physiol Gastrointest Liver Physiol. (2020) 319:G619–25.
36. He B, Jiang J, Shi Z, Wu L, Yan J, Chen Z, et al. Pure total flavonoids from Citrus attenuate non-alcoholic steatohepatitis via regulating the gut microbiota and bile acid metabolism in mice. Biomed Pharmacother. (2021) 135:111183. doi: 10.1016/j.biopha.2020.111183
37. Singh S, Osna NA, Kharbanda KK. Treatment options for alcoholic and non-alcoholic fatty liver disease: a review. World J Gastroenterol. (2017) 23:6549–70.
38. Zhang X, Zhang Y, Gao W, Guo Z, Wang K, Liu S, et al. Naringin improves lipid metabolism in a tissue-engineered liver model of NAFLD and the underlying mechanisms. Life Sci. (2021) 277:119487. doi: 10.1016/j.lfs.2021.119487
39. Wang SW, Sheng H, Bai YF, Weng YY, Fan XY, Lou LJ, et al. Neohesperidin enhances PGC-1α-mediated mitochondrial biogenesis and alleviates hepatic steatosis in high fat diet fed mice. Nutr Diabetes. (2020) 10:27. doi: 10.1038/s41387-020-00130-3
40. Park HY, Ha SK, Eom H, Choi I. Narirutin fraction from Citrus peels attenuates alcoholic liver disease in mice. Food Chem Toxicol. (2013) 55:637–44. doi: 10.1016/j.fct.2013.01.060
41. Zhou C, Lai Y, Huang P, Xie L, Lin H, Zhou Z, et al. Naringin attenuates alcoholic liver injury by reducing lipid accumulation and oxidative stress. Life Sci. (2019) 216:305–12. doi: 10.1016/j.lfs.2018.07.031
42. El-Sisi AEE, Sokar SS, Shebl AM, Mohamed DZ. Antifibrotic effect of diethylcarbamazine combined with hesperidin against ethanol induced liver fibrosis in rats. Biomed Pharmacother. (2017) 89:1196–206. doi: 10.1016/j.biopha.2017.03.013
43. Mantovani A, Scorletti E, Mosca A, Alisi A, Byrne CD, Targher G. Complications, morbidity and mortality of nonalcoholic fatty liver disease. Metabolism. (2020) 111s:154170.
44. Sinn DH, Kang D, Chang Y, Ryu S, Cho SJ, Paik SW, et al. Non-alcoholic fatty liver disease and the incidence of myocardial infarction: a cohort study. J Gastroenterol Hepatol. (2020) 35:833–9.
45. Yoshitaka H, Hamaguchi M, Kojima T, Fukuda T, Ohbora A, Fukui M. Nonoverweight nonalcoholic fatty liver disease and incident cardiovascular disease: a post hoc analysis of a cohort study. Medicine (Baltimore). (2017) 96:e6712. doi: 10.1097/MD.0000000000006712
46. Kapuria D, Takyar VK, Etzion O, Surana P, O’Keefe JH, Koh C. Association of hepatic steatosis with subclinical atherosclerosis: systematic review and meta-analysis. Hepatol Commun. (2018) 2:873–83.
47. Mao Z, Gan C, Zhu J, Ma N, Wu L, Wang L, et al. Anti-atherosclerotic activities of flavonoids from the flowers of Helichrysum arenarium L. MOENCH through the pathway of anti-inflammation. Bioorg Med Chem Lett. (2017) 27:2812–7. doi: 10.1016/j.bmcl.2017.04.076
48. Li W, Wang C, Peng J, Liang J, Jin Y, Liu Q, et al. Naringin inhibits TNF-α induced oxidative stress and inflammatory response in HUVECs via Nox4/NF-κ B and PI3K/Akt pathways. Curr Pharm Biotechnol. (2014) 15:1173–82. doi: 10.2174/1389201015666141111114442
49. Zhao H, Liu M, Liu H, Suo R, Lu C. Naringin protects endothelial cells from apoptosis and inflammation by regulating the Hippo-YAP Pathway. Biosci Rep. (2020) 40:BSR20193431.
50. Morand C, Dubray C, Milenkovic D, Lioger D, Martin JF, Scalbert A, et al. Hesperidin contributes to the vascular protective effects of orange juice: a randomized crossover study in healthy volunteers. Am J Clin Nutr. (2011) 93:73–80. doi: 10.3945/ajcn.110.004945
51. Rizza S, Muniyappa R, Iantorno M, Kim JA, Chen H, Pullikotil P, et al. Citrus polyphenol hesperidin stimulates production of nitric oxide in endothelial cells while improving endothelial function and reducing inflammatory markers in patients with metabolic syndrome. J Clin Endocrinol Metab. (2011) 96:E782–92. doi: 10.1210/jc.2010-2879
52. Wong ES, Li RW, Li J, Li R, Seto SW, Lee SM, et al. Relaxation effect of narirutin on rat mesenteric arteries via nitric oxide release and activation of voltage-gated potassium channels. Eur J Pharmacol. (2021) 905:174190. doi: 10.1016/j.ejphar.2021.174190
53. Loomba R, Jain A, Diehl AM, Guy CD, Portenier D, Sudan R, et al. Validation of serum test for advanced liver fibrosis in patients with nonalcoholic steatohepatitis. Clin Gastroenterol Hepatol. (2019) 17:1867–76.e3.
54. Loomba R, Cui J, Wolfson T, Haufe W, Hooker J, Szeverenyi N, et al. Novel 3D magnetic resonance elastography for the noninvasive diagnosis of advanced fibrosis in NAFLD: a prospective study. Am J Gastroenterol. (2016) 111:986–94. doi: 10.1038/ajg.2016.65
55. Generoso G, Janovsky C, Bittencourt MS. Triglycerides and triglyceride-rich lipoproteins in the development and progression of atherosclerosis. Curr Opin Endocrinol Diabetes Obes. (2019) 26:109–16.
56. Yu XH, Zhang DW, Zheng XL, Tang CK. Cholesterol transport system: an integrated cholesterol transport model involved in atherosclerosis. Prog Lipid Res. (2019) 73:65–91. doi: 10.1016/j.plipres.2018.12.002
57. Lechner K, McKenzie AL, Kränkel N, Von Schacky C, Worm N, Nixdorff U, et al. High-risk atherosclerosis and metabolic phenotype: the roles of ectopic adiposity, atherogenic dyslipidemia, and inflammation. Metab Syndr Relat Disord. (2020) 18:176–85. doi: 10.1089/met.2019.0115
58. Iqbal Chowdhury I, Rahman MA, Hashem MA, Bhuiyan MMH, Hajjar D, Alelwani W, et al. Supplements of an aqueous combination of Justicia adhatoda and Ocimum tenuiflorum boost antioxidative effects and impede hyperlipidemia. Animal Model Exp Med. (2020) 3:140–51. doi: 10.1002/ame2.12115
59. Goldstein JL, Brown MS. A century of cholesterol and coronaries: from plaques to genes to statins. Cell. (2015) 161:161–72. doi: 10.1016/j.cell.2015.01.036
60. Sun YZ, Chen JF, Shen LM, Zhou J, Wang CF. Anti-atherosclerotic effect of hesperidin in LDLr(-/-) mice and its possible mechanism. Eur J Pharmacol. (2017) 815:109–17. doi: 10.1016/j.ejphar.2017.09.010
61. Kumar R, Akhtar F, Rizvi SI. Protective effect of hesperidin in poloxamer-407 induced hyperlipidemic experimental rats. Biol Futur. (2021) 72:201–10. doi: 10.1007/s42977-020-00053-1
62. Wang F, Zhao C, Yang M, Zhang L, Wei R, Meng K, et al. Four Citrus flavanones exert atherosclerosis alleviation effects in ApoE(-/-) mice via different metabolic and signaling pathways. J Agric Food Chem. (2021) 69:5226–37. doi: 10.1021/acs.jafc.1c01463
63. Wu H, Liu Y, Chen X, Zhu D, Ma J, Yan Y, et al. Neohesperidin exerts lipid-regulating effects in vitro and in vivo via fibroblast growth factor 21 and AMP-activated protein kinase/Sirtuin Type 1/peroxisome proliferator-activated receptor Gamma coactivator 1α signaling axis. Pharmacology. (2017) 100:115–26. doi: 10.1159/000452492
64. Toth PP, Patti AM, Nikolic D, Giglio RV, Castellino G, Biancucci T, et al. Bergamot reduces plasma lipids, atherogenic small dense LDL, and subclinical atherosclerosis in subjects with moderate hypercholesterolemia: A 6 months prospective study. Front Pharmacol. (2015) 6:299. doi: 10.3389/fphar.2015.00299
65. Stahl EP, Dhindsa DS, Lee SK, Sandesara PB, Chalasani NP, Sperling LS. Nonalcoholic fatty liver disease and the heart: JACC State-of-the-Art review. J Am Coll Cardiol. (2019) 73:948–63.
66. White MG, Shaw JA, Taylor R. Type 2 diabetes: the pathologic basis of reversible β-Cell dysfunction. Diabetes Care. (2016) 39:2080–8. doi: 10.2337/dc16-0619
67. Ghosh A, Gao L, Thakur A, Siu PM, Lai CWK. Role of free fatty acids in endothelial dysfunction. J Biomed Sci. (2017) 24:50.
68. Ahmed OM, Hassan MA, Abdel-Twab SM, Abdel Azeem MN. Navel orange peel hydroethanolic extract, naringin and naringenin have anti-diabetic potentials in type 2 diabetic rats. Biomed Pharmacother. (2017) 94:197–205. doi: 10.1016/j.biopha.2017.07.094
69. Subramanian M, Thotakura B, Chandra Sekaran SP, Jyothi AK, Sundaramurthi I. Naringin (4’,5,7-trihydroxyflavanone 7-rhamnoglucoside) attenuates β-cell dysfunction in diabetic rats through upregulation of PDX-1. Cells Tissues Organs. (2018) 206:133–43. doi: 10.1159/000496506
70. Syed AA, Reza MI, Shafiq M, Kumariya S, Singh P, Husain A, et al. Naringin ameliorates type 2 diabetes mellitus-induced steatohepatitis by inhibiting RAGE/NF-κB mediated mitochondrial apoptosis. Life Sci. (2020) 257:118118. doi: 10.1016/j.lfs.2020.118118
71. Yari Z, Cheraghpour M, Alavian SM, Hedayati M, Eini-Zinab H, Hekmatdoost A. The efficacy of flaxseed and hesperidin on non-alcoholic fatty liver disease: an open-labeled randomized controlled trial. Eur J Clin Nutr. (2021) 75:99–111. doi: 10.1038/s41430-020-0679-3
72. Sundaram R, Nandhakumar E, Haseena Banu H. Hesperidin, a Citrus flavonoid ameliorates hyperglycemia by regulating key enzymes of carbohydrate metabolism in streptozotocin-induced diabetic rats. Toxicol Mech Methods. (2019) 29:644–53. doi: 10.1080/15376516.2019.1646370
73. Jung UJ, Lee MK, Jeong KS, Choi MS. The hypoglycemic effects of hesperidin and naringin are partly mediated by hepatic glucose-regulating enzymes in C57BL/KsJ-db/db mice. J Nutr. (2004) 134:2499–503. doi: 10.1093/jn/134.10.2499
74. Jia S, Hu Y, Zhang W, Zhao X, Chen Y, Sun C, et al. Hypoglycemic and hypolipidemic effects of neohesperidin derived from Citrus aurantium L. in diabetic KK-A(y) mice. Food Funct. (2015) 6:878–86. doi: 10.1039/c4fo00993b
75. Motallaei M, Ramezani-Jolfaie N, Mohammadi M, Shams-Rad S, Jahanlou AS, Salehi-Abargouei A. Effects of orange juice intake on cardiovascular risk factors: a systematic review and meta-analysis of randomized controlled clinical trials. Phytother Res. (2021) 35:5427–39. doi: 10.1002/ptr.7173
76. Hsu PH, Chen WH, Juan-Lu C, Hsieh SC, Lin SC, Mai RT, et al. Hesperidin and chlorogenic acid synergistically inhibit the growth of breast cancer cells via estrogen receptor/mitochondrial pathway. Life (Basel). (2021) 11:950. doi: 10.3390/life11090950
77. Xia R, Sheng X, Xu X, Yu C, Lu H. Hesperidin induces apoptosis and G0/G1 arrest in human non-small cell lung cancer A549 cells. Int J Mol Med. (2018) 41:464–72. doi: 10.3892/ijmm.2017.3250
78. Zhao J, Li Y, Gao J, De Y. Hesperidin inhibits ovarian cancer cell viability through endoplasmic reticulum stress signaling pathways. Oncol Lett. (2017) 14:5569–74. doi: 10.3892/ol.2017.6873
79. Pang S, Jia M, Gao J, Liu X, Guo W, Zhang H. Effects of dietary patterns combined with dietary phytochemicals on breast cancer metastasis. Life Sci. (2021) 264:118720.
80. Xia R, Xu G, Huang Y, Sheng X, Xu X, Lu H. Hesperidin suppresses the migration and invasion of non-small cell lung cancer cells by inhibiting the SDF-1/CXCR-4 pathway. Life Sci. (2018) 201:111–20. doi: 10.1016/j.lfs.2018.03.046
81. El-Deek SEM, Abd-Elghaffar SKH, Hna RS, Mohamed HG, El-Deek HEM. Effect of hesperidin against induced colon cancer in rats: impact of Smad4 and activin a signaling pathway. Nutr Cancer. (2021) 74:697–714. doi: 10.1080/01635581.2021.1907424
82. Patel P, Shah J. Protective effects of hesperidin through attenuation of Ki67 expression against DMBA-induced breast cancer in female rats. Life Sci. (2021) 285:119957. doi: 10.1016/j.lfs.2021.119957
83. Mo’men YS, Hussein RM, Kandeil MA. Involvement of PI3K/Akt pathway in the protective effect of hesperidin against a chemically induced liver cancer in rats. J Biochem Mol Toxicol. (2019) 33:e22305. doi: 10.1002/jbt.22305
84. Fernández-Bedmar Z, Anter J, Alonso-Moraga A, Martín de Las Mulas J, Millán-Ruiz Y, Guil-Luna S. Demethylating and anti-hepatocarcinogenic potential of hesperidin, a natural polyphenol of Citrus juices. Mol Carcinog. (2017) 56:1653–62. doi: 10.1002/mc.22621
85. Raha S, Kim SM, Lee HJ, Yumnam S, Saralamma VV, Ha SE, et al. naringin induces lysosomal permeabilization and autophagy cell death in AGS gastric cancer cells. Am J Chin Med. (2020) 48:679–702. doi: 10.1142/S0192415X20500342
86. Albayrak D, Doğanlar O, Erdoğan S, Meraklı M, Doğan A, Turker P, et al. Naringin combined with NF-κB inhibition and endoplasmic reticulum stress induces apoptotic cell death via oxidative stress and the PERK/eIF2α/ATF4/CHOP Axis in HT29 colon cancer cells. Biochem Genet. (2021) 59:159–84. doi: 10.1007/s10528-020-09996-5
87. Aroui S, Fetoui H, Kenani A. Natural dietary compound naringin inhibits glioblastoma cancer neoangiogenesis. BMC Pharmacol Toxicol. (2020) 21:46. doi: 10.1186/s40360-020-00426-1
88. Aroui S, Najlaoui F, Chtourou Y, Meunier AC, Laajimi A, Kenani A, et al. Naringin inhibits the invasion and migration of human glioblastoma cell via downregulation of MMP-2 and MMP-9 expression and inactivation of p38 signaling pathway. Tumour Biol. (2016) 37:3831–9. doi: 10.1007/s13277-015-4230-4
89. Rinninella E, Cintoni M, Raoul P, Lopetuso LR, Scaldaferri F, Pulcini G, et al. Food components and dietary habits: keys for a healthy gut microbiota composition. Nutrients. (2019) 11:2393. doi: 10.3390/nu11102393
90. Lu JF, Zhu MQ, Zhang H, Liu H, Xia B, Wang YL, et al. Neohesperidin attenuates obesity by altering the composition of the gut microbiota in high-fat diet-fed mice. FASEB J. (2020) 34:12053–71. doi: 10.1096/fj.201903102RR
91. Wang F, Zhao C, Tian G, Wei X, Ma Z, Cui J, et al. Naringin alleviates atherosclerosis in ApoE(-/-) Mice by regulating cholesterol metabolism involved in gut microbiota remodeling. J Agric Food Chem. (2020) 68:12651–60. doi: 10.1021/acs.jafc.0c05800
92. Fidélix M, Milenkovic D, Sivieri K, Cesar T. Microbiota modulation and effects on metabolic biomarkers by orange juice: a controlled clinical trial. Food Funct. (2020) 11:1599–610.
93. Estruel-Amades S, Massot-Cladera M, Pérez-Cano FJ, Franch À, Castell M, Camps-Bossacoma M. Hesperidin effects on gut microbiota and gut-associated lymphoid tissue in healthy rats. Nutrients. (2019) 11:E324. doi: 10.3390/nu11020324
94. Guirro M, Gual-Grau A, Gibert-Ramos A, Alcaide-Hidalgo JM, Canela N, Arola L, et al. Metabolomics elucidates dose-dependent molecular beneficial effects of hesperidin supplementation in rats fed an obesogenic diet. Antioxidants (Basel). (2020) 9:79. doi: 10.3390/antiox9010079
95. Gong Y, Dong R, Gao X, Li J, Jiang L, Zheng J, et al. Neohesperidin prevents colorectal tumorigenesis by altering the gut microbiota. Pharmacol Res. (2019) 148:104460. doi: 10.1016/j.phrs.2019.104460
96. Attia GH, Marrez DA, Mohammed MA, Albarqi HA, Ibrahim AM, Raey MAE. Synergistic effect of mandarin peels and hesperidin with sodium nitrite against some food pathogen microbes. Molecules. (2021) 26:3186. doi: 10.3390/molecules26113186
97. Dey P, Parai D, Banerjee M, Hossain ST, Mukherjee SK. Naringin sensitizes the antibiofilm effect of ciprofloxacin and tetracycline against Pseudomonas aeruginosa biofilm. Int J Med Microbiol. (2020) 310:151410. doi: 10.1016/j.ijmm.2020.151410
98. Verma AK, Ahmed SF, Hossain MS, Bhojiya AA, Mathur A, Upadhyay SK, et al. Molecular docking and simulation. studies of flavonoid compounds against PBP-2a of methicillin-resistant Staphylococcus aureus. J Biomol Struct Dyn. (2021) 1–17.
99. Cassidy A, Minihane AM. The role of metabolism (and the microbiome) in defining the clinical efficacy of dietary flavonoids. Am J Clin Nutr. (2017) 105:10–22. doi: 10.3945/ajcn.116.136051
100. Zhao J, Yang J, Xie Y. Improvement strategies for the oral bioavailability of poorly water-soluble flavonoids: an overview. Int J Pharm. (2019) 570:118642. doi: 10.1016/j.ijpharm.2019.118642
101. Ravisankar S, Agah S, Kim H, Talcott S, Wu C, Awika J. Combined cereal and pulse flavonoids show enhanced bioavailability by downregulating phase II metabolism and ABC membrane transporter function in Caco-2 model. Food Chem. (2019) 279:88–97. doi: 10.1016/j.foodchem.2018.12.006
102. Oliveira H, Perez-Gregório R, de Freitas V, Mateus N, Fernandes I. Comparison of the in vitro gastrointestinal bioavailability of acylated and non-acylated anthocyanins: purple-fleshed sweet potato vs red wine. Food Chem. (2019) 276:410–8. doi: 10.1016/j.foodchem.2018.09.159
103. Khan AW, Kotta S, Ansari SH, Sharma RK, Ali J. Enhanced dissolution and bioavailability of grapefruit flavonoid Naringenin by solid dispersion utilizing fourth generation carrier. Drug Dev Ind Pharm. (2015) 41:772–9. doi: 10.3109/03639045.2014.902466
104. Xia N, Wan W, Zhu S, Liu Q. Synthesis of hydrophobic propionyl neohesperidin ester using an immobilied enzyme and description of its anti-proliferative and pro-apoptotic effects on MCF-7 human breast cancer cells. Front Bioeng Biotechnol. (2020) 8:1025. doi: 10.3389/fbioe.2020.01025
105. Amaretti A, Raimondi S, Leonardi A, Quartieri A, Rossi M. Hydrolysis of the rutinose-conjugates flavonoids rutin and hesperidin by the gut microbiota and bifidobacteria. Nutrients. (2015) 7:2788–800. doi: 10.3390/nu7042788
106. Actis-Goretta L, Dew TP, Lévèques A, Pereira-Caro G, Rein M, Teml A, et al. Gastrointestinal absorption and metabolism of hesperetin-7-O-rutinoside and hesperetin-7-O-glucoside in healthy humans. Mol Nutr Food Res. (2015) 59:1651–62. doi: 10.1002/mnfr.201500202
107. Pereira-Caro G, Fernández-Quirós B, Ludwig IA, Pradas I, Crozier A, Moreno-Rojas JM. Catabolism of Citrus flavanones by the probiotics Bifidobacterium longum and Lactobacillus rhamnosus. Eur J Nutr. (2018) 57:231–42. doi: 10.1007/s00394-016-1312-z
108. Pereira-Caro G, Oliver CM, Weerakkody R, Singh T, Conlon M, Borges G, et al. Chronic administration of a microencapsulated probiotic enhances the bioavailability of orange juice flavanones in humans. Free Radic Biol Med. (2015) 84:206–14. doi: 10.1016/j.freeradbiomed.2015.03.010
109. Manach C, Morand C, Gil-Izquierdo A, Bouteloup-Demange C, Rémésy C. Bioavailability in humans of the flavanones hesperidin and narirutin after the ingestion of two doses of orange juice. Eur J Clin Nutr. (2003) 57:235–42.
110. Bredsdorff L, Nielsen IL, Rasmussen SE, Cornett C, Barron D, Bouisset F, et al. Absorption, conjugation and excretion of the flavanones, naringenin and hesperetin from alpha-rhamnosidase-treated orange juice in human subjects. Br J Nutr. (2010) 103:1602–9. doi: 10.1017/S0007114509993679
111. Bashiardes S, Godneva A, Elinav E, Segal E. Towards utilization of the human genome and microbiome for personalized nutrition. Curr Opin Biotechnol. (2018) 51:57–63.
112. Aschoff JK, Kaufmann S, Kalkan O, Neidhart S, Carle R, Schweiggert RM. In vitro bioaccessibility of carotenoids, flavonoids, and vitamin C from differently processed oranges and orange juices [Citrus sinensis (L.) Osbeck]. J Agric Food Chem. (2015) 63:578–87.
113. Tomás-Navarro M, Vallejo F, Borrego F, Tomás-Barberán FA. Encapsulation and micronization effectively improve orange beverage flavanone bioavailability in humans. J Agric Food Chem. (2014) 62:9458–62. doi: 10.1021/jf502933v
114. Mohamed EA, Abu H II, Yusif RM, Shaaban AAA, El-Sheakh AR, Hamed MF, et al. Polymeric micelles for potentiated antiulcer and anticancer activities of naringin. Int J Nanomedicine. (2018) 13:1009–27. doi: 10.2147/IJN.S154325
115. Zang W. Identification and Determination of Flavonoids from Different Citrus Cultivars Fruit and their Antioxidant Activity. Hangzhou: Zhejiang University (2019).
116. Miles EA, Calder PC. Effects of Citrus fruit juices and their bioactive components on inflammation and immunity: a narrative review. Front Immunol. (2021) 12:712608. doi: 10.3389/fimmu.2021.712608
117. Zhu T, Wang H, Chen J, Wang Y, Cao J, Li X, et al. Separation and purification of flavonoids from different Citrus fruits and their antioxidant activities. J Zhejiang Univ. (2021) 47:704–18.
Keywords: flavonoids, extraction, metabolism, gut microbiota, Citrus maxima (Burm.) merr.
Citation: Ding S, Wang P, Pang X, Zhang L, Qian L, Jia X, Chen W, Ruan S and Sun L (2022) The new exploration of pure total flavonoids extracted from Citrus maxima (Burm.) Merr. as a new therapeutic agent to bring health benefits for people. Front. Nutr. 9:958329. doi: 10.3389/fnut.2022.958329
Received: 06 July 2022; Accepted: 25 August 2022;
Published: 06 October 2022.
Edited by:
J. Fernando Ayala-Zavala, Centro de Investigación en Alimentación y Desarrollo, Consejo Nacional de Ciencia y Tecnología (CONACYT), MexicoReviewed by:
Melvin R. Tapia-Rodriguez, Instituto Tecnológico de Sonora (ITSON), MexicoAriadna Thalia Bernal Mercado, University of Sonora, Mexico
Copyright © 2022 Ding, Wang, Pang, Zhang, Qian, Jia, Chen, Ruan and Sun. This is an open-access article distributed under the terms of the Creative Commons Attribution License (CC BY). The use, distribution or reproduction in other forums is permitted, provided the original author(s) and the copyright owner(s) are credited and that the original publication in this journal is cited, in accordance with accepted academic practice. No use, distribution or reproduction is permitted which does not comply with these terms.
*Correspondence: Leitao Sun, sunnylt@zcmu.edu.cn; Shanming Ruan, shanmingruan@zcmu.edu.cn
†These authors have contributed equally to this work