- 1Green Biotech Network, School of Agricultural Sciences, São Paulo State University, Botucatu, Brazil
- 2Laboratory of Foodomics, Institute of Food Science Research, CIAL, CSIC-UAM, Madrid, Spain
Soybeans are mainly used for food and biodiesel production. It is estimated that soy crops worldwide will leave about 651 million metric tons of branches, leaves, pods, and roots on the ground post-harvesting in 2022/23. These by-products might serve as largely available and cheap source of high added-value metabolites, such as flavonoids, isoflavonoids, and other phenolic compounds. This work aimed to explore green approaches based on the use of pressurized and gas expanded-liquid extraction combined with natural deep eutectic solvents (NADESs) to achieve phenolic-rich extracts from soy by-products. The total phenolic and flavonoid contents of the generated extracts were quantified and compared with conventional solvents and techniques. Pressurized liquid extraction (PLE) with choline chloride/citric acid/water (1:1:11 – molar ratio) at 120°C, 100 bar, and 20 min, resulted in an optimized condition to generate phenolic and flavonoid-rich fractions of soy by-products. The individual parts of soy were extracted under these conditions, with their metabolic profile obtained by UHPLC-ESI-QToF-MS/MS and potential antioxidant properties by ROS scavenging capacity. Extracts of soy roots presented the highest antioxidant capacity (207.48 ± 40.23 mg AA/g), three times higher than soybean extracts (68.96 ± 12.30). Furthermore, Hansen solubility parameters (HSPs) were applied to select natural hydrophobic deep eutectic solvents (NaHDES) as substituents for n-heptane to defat soybeans. Extractions applying NaHDES candidates achieved a similar yield and chromatography profile (GC-QToF-MS) to n-heptane extracts.
Introduction
Compounds identified in natural products have been historically used to develop new drugs, ingredients for functional foods, cosmetic products, and other applications (1). Commodity crops, such as apples, coffee, olives, and soybeans, contain several metabolites with potential bioactivity (2–5). Soybeans, the major oilseed crop worldwide, present a wide range of phenolic compounds, mainly isoflavonoids and flavonoids, resulting in the growing interest for being more than just an oilseed plant (5). Genistin, an isoflavone glycoside, contains anti-adipogenic, and anti-lipogenic in vitro properties and also prevents breast cancer (6, 7). Apigenin, a flavonoid, has protective effects against cardiometabolic diseases, and it has been suggested as biopesticide (8, 9). In addition, other bioactive metabolites identified in agro-soy by-products collected after harvesting (i.e., branches, leaves, pods, and roots) provide new findings and perspectives for using such materials (10, 11). Soybeans, the soy commodity, have been the second source of vegetable oils, with an estimated production of 61 million tons in 2022/23 (12). The defatting process of soybeans has been widely performed using hexane, a toxic and flammable solvent (13). Alternative solvents, such as ethanol, supercritical CO2, and 2-methyloxolane, have already demonstrated to be feasible and greener options to replace the conventional one (13–15). Furthermore, new alternatives as natural hydrophobic deep eutectic solvents (NaHDES) may be promising bio-based solvents for defatting procedures. Moreover, an in silico approach applying Hansen solubility parameters (HSPs) can be performed to select the most similar solvent to hexane. Based on the concepts of solubility and “like dissolves like,” HSPs promote the comparison among several solvents and generate a computational simulation of their interaction (16). Solvents with similar HSP could present a higher similarity between their chemical and/or physical properties, meaning a valuable tool to replace conventional and hazardous solvents with sustainable ones.
In this context, green analytical chemistry should be employed to add value to agro-soy by-products, reducing potential environmental problems related to their extraction and revalorization, as suggested by the goal 12, “Sustainable consumption and production” of the 17 sustainable development goals (SDGs) set by the United Nations (UN) (17). Therefore, greener solvents and techniques are required in accordance with a sustainable procedure, reducing energy, reagents, and increasing the security of the applied analytical methodology. Natural deep eutectic solvents (NADESs), which are composed of major metabolites identified in plants and other organisms, could be green alternatives to extract bioactive compounds (18). Duru et al. performed NADES extraction of isoflavones from kudzu root and soy molasses wastes, achieving fractions with higher antioxidant activity and stability than methanolic extraction (19).
To achieve a greener process, the integration of green solvents with innovative and environmentally friendly technologies can offer some advantages such as time and energy reduction, as well as solvent consumption. In this sense, pressurized liquid extraction (PLE) has shown significant benefits for extracting phenolics and other bioactive compounds from natural matrices, despite being scarcely integrated with NADES as solvents (20–23). Nevertheless, this combination was successfully performed to extract anthocyanins from a Brazilian berry processing by-product (24), as demonstrated by Benvenutti et al. (23). In addition, gas-expanded liquids (GXLs), a promising type of solvents, could be useful to decrease the viscosity of NADES, with the addition of CO2 at large amounts. Moreover, GXLs promote other modifications in the physicochemical properties of the solvents (24).
Therefore, this work aimed to develop an alternative approach to generate phenolic-rich fractions from soy by-products, i.e., branches, leaves, pods, and roots collected just after mechanical harvesting, combining PLE and GXLs with NADES. For this, (i) an in silico approach using HSP was applied to select an alternative NaHDES candidate to replace n-heptane in the defatting process, (ii) PLE and GXLs were performed with choline chloride/citric acid/water 1:1:11 (molar ratio) (ChCl:Ca:H2O) to generate phenolic-rich extracts, (iii) the optimized extraction condition was applied to extract the individual parts of agro-soy by-products and soybeans, (iv) the potential reactive oxygen species (ROS) scavenging capacity of the generated extracts was measured, and (v) the metabolite profiling was studied by ultra-high-performance liquid chromatography coupled to high-resolution mass spectrometry (UHPLC-QToF-MS/MS).
Materials and methods
Chemicals
High-performance liquid chromatography (HPLC)-grade solvents such as acetonitrile, n-heptane, and ethanol (EtOH), as well as menthol, eucalyptol, thymol, choline chloride, and citric acid were purchased from VWR Chemicals (Spain). Standards of 2,2′-azino-bis (3-ethylbenzothiazoline-6-sulphonic acid) (ABTS), sodium carbonate (Na2CO3), aluminum chloride (AlCl3), potassium persulfate (K2S2O8), disodium phosphate (Na2HPO4), monopotassium phosphate (KH2PO4), fluorescein sodium salt, gallic acid, ascorbic acid, and quercetin were obtained from Sigma-Aldrich (Spain). Folin–Ciocalteu phenol reagent was acquired from Merck (Germany). 2,2-azobis (2-amidinopropane) dihydrochloride (AAPH) was purchased from TCI Chemicals (Japan). Ultrapure water was obtained from a Millipore system (Billerica, United States).
Plant material
Soy branches, leaves, pods, roots, and soybeans (cultivar NA5909) were collected after the mechanical harvesting at the School of Agricultural Sciences of São Paulo State University—Botucatu, São Paulo State, Brazil (−22.8296354, −48.42553). All parts were grounded in a basic analytical mill (IKA® A11, Germany) and separated by granulometry on an electromagnetic sieve shaker (Bertel, Brazil). Only particles with < 0.25 mm were used for extraction.
Pressurized liquid extraction
The PLEs were made in an ASE 200 device (Dionex, United States) using an 11-ml stainless steel cell at 100 bars. PLE was performed in two steps: (i) a first step in which materials with high content of lipids (soybeans) are defatted, and (ii) a second step focused on phenolic extraction. It is worth mentioning that, for those vegetal materials with low lipid content, the PLE extraction begins on the second step.
Soy material was separated into the following: (i) a mix of equal parts of soy by-products (250 mg of branches, leaves, pods, and roots—in a total of 1 g) and (ii) 1 g of soybeans (reference material). The vegetal material was mixed with sea sand in a 1:2 w/w proportion, which facilitated the diffusion of the solvent in the material, while avoiding preferential paths. All the extractions were made in triplicate, and the samples were preserved at −18°C until further analysis. The flowchart of the extraction processes with the analyses employed in this work is shown in Figure 1.
Pressurized liquid extraction first step—Defatting procedure
First, a defatting process was performed using n-heptane as the benchmark solvent, which is greener but highly similar to hexane regarding the HSP, the conventional solvent used to defat soybeans (25). The extraction procedure was performed at three temperatures (40, 80, and 120°C) for 90 min, generating kinetic extraction curves from sample collection each 10 min. The accumulated extraction yield (%) of each extraction was measured by concentrating each extract with a continuous flow of nitrogen gas. To replace n-heptane, three NaHDES candidates were selected using HSPs, as well as considering the toxicity and availability of their components (26, 27). The HSPs of 108 NaHDES candidates, which have already been described in the literature, were calculated using the HSPiP® software v 5.0 (28–45). Therefore, the three parameters of HSP, δD, δP, and δH, which correspond to dispersion bonds, dipolar intermolecular force, and hydrogen bonds between molecules/solvents, were estimated for each NaHDES mixture (16). Additionally, Jchem (JChem for Excel 21.1.0.787, ChemAxon1 and Microsoft Excel 365 (Microsoft Corporation, United States) were applied to calculate the volume percentage of each compound of NaHDES, as well as to generate their SMILES. Then, the HSPs of the individual components of NaHDES were estimated by the “Do It Yourself” tool, and the final HSPs of NaHDES mixtures were achieved using the “Solvent Optimizer” tool in HSPiP® software v 5.0 (27). The selection of NaHDES was based on the distance (Ra—Equation 1) to n-heptane (considered as “solute” in Equation 1). Supplementary Table 1 summarizes the estimated HSP of listed NaHDES and n-heptane, as well as the distance between them. Thus, the selected NaHDESs were prepared by mixing their components with a magnetic stirring bar at 90°C until a clear liquid was reached (46). The alternative PLE first step using NaHDES was tested at three temperatures (90, 120, and 150°C) for 20 min (two extractions of 10 min each). Bottles with NaHDES were kept at 90°C in all extractions (Supplementary Figure 1). The resulting extracts were concentrated by a continuous flow of nitrogen gas until dryness.
Equation 1
Pressurized liquid extraction second step—Phenolic extraction
The second step of PLE was first performed and optimized with the mix of soy by-products. Subsequently, the optimal condition was applied to extract the soy by-products individually, as well as the defatted soybean. Hence, two solvents were tested in this step: (i) EtOH-H2O 7:3 (v/v), and (ii) choline chloride/citric acid/water 1:1:11 (molar ratio) (ChCl:Ca:H2O), which has previously been applied and its constituents and ratio optimized to extract isoflavones and solubilize flavonoids (47, 48). ChCl:Ca:H2O was prepared as described in the previous section. Extractions were performed at three temperatures (60, 90, and 120°C) for 20 min (two extractions of 10 min each). Subsequently, the condition with the highest phenolic and flavonoid content was applied to extract the soy parts individually.
Conventional and alternative extraction of agro-soy by-products
Dynamic maceration (Mac) and gas-expanded liquids (GXLs) were performed to extract the mix of agro-soy by-products and compare their total phenolic and flavonoid contents with PLE. The results were evaluated by analysis of variance (ANOVA) and Tukey’s multiple comparisons test with a 5% level of significance on GraphPad Prism version 9 (GraphPad Software, United States).
Dynamic maceration as the reference extraction technique
Dynamic maceration (Mac) with magnetic stirring was selected as the conventional extraction technique (11). A mix of 100 mg of branches, leaves, pods, and roots—in a total of 400 mg—was extracted with 8 ml of (i) EtOH-H2O 7:3 (v/v), and (ii) NADES choline chloride/citric acid/water 1:1:11 (molar ratio—ChCl:Ca:H2O), at 1,500 rpm, 60°C for 35 min (IKA C-MAG HS 7, Germany).
Gas-expanded liquids
As an alternative technique, gas-expanded liquid (GXLs) extraction was combined with NADES to achieve a new methodology for phenolic extraction. To the best of our knowledge, the literature does not report such combination. Thus, a homemade compressed fluid extractor was used for gas-expanded liquid (GXLs) extractions. The equipment consisted of a PU-2080 Plus CO2 high-pressure pump (Jasco, Japan) and an Agilent 1,200 Binary Pump (Agilent, United States). For the extractions, 1 g of the mix of soy by-products (prepared as stated above) was mixed with 2 g of sea sand and added into a 20-ml stainless-steel extraction cell with glass wool packed at both ends. The cell was placed in an Adept CE 4600 column oven (Cecil, United Kingdom) at 60°C. The pressure was kept at 100 bar using a manual metering valve (Swagelok, United States) (49). The total flow rate was established at 4 ml/min, and 25, 50, and 75% CO2 in ChCl:Ca:H2O were tested. All the extractions were made in triplicate, and the samples were preserved at −18°C until further analysis.
Determination of total phenolic content
Total phenolic content (TPC) was determined by Folin-Ciocalteu method (50). First, 10 μl of extracts was mixed with 600 μl of ultrapure water and reacted with 50 μl of the reagent Folin-Ciocalteu. After 1 min, 150 μl of 20% (w/v) sodium carbonate (Na2CO3) was added, and the final volume was adjusted to 1 ml with water. The sample was mixed and incubated for 2 h at room temperature in darkness. Subsequently, 300 μl of the mixture was transferred to a 96-well microplate spectrophotometer reader (Synergy HT, United States), and the absorbance was measured at 760 nm. The values were converted to mg GAE (gallic acid equivalent) in the extracts by a calibration curve (0–2 mg/ml) prepared with standard gallic acid. Results were expressed in mg equivalents of gallic acid per g of vegetal material.
Determination of total flavonoid content
Total flavonoid content (TFC) was measured using the aluminum chloride colorimetric method with some modifications (51). First, 240 μl of methanolic solutions of quercetin (4–14 μg/ml) was used to prepare the calibration curve, and 100 μl of extracts adding 60 μl of AlCl3 8 mM was filled into each well. The absorbance of each sample was measured at 425 nm after 30 min of incubation in darkness. The values were converted to mg QE (quercetin equivalent) in the extracts. Results were expressed in μg equivalents of quercetin per g of vegetal material.
Antioxidant activity assays—Reactive oxygen species scavenging capacity
The oxygen radical absorbance capacity (ORAC) method was carried out according to Ou et al. (52) and Ninfali et al. (53). Reaction mixtures in the wells contained the following reagents: 100 μl of extract sample at different concentrations (5 μg–50 μg/ml) in EtOH-H2O 9:1 (v/v), 100 μl of AAPH (590 mM) in 30 mM phosphate-buffered saline (PBS) at pH = 7.5, 25 μl of fluorescein (10 μM) in PBS buffer, and 100 μl of PBS buffer. Fluorescence was measured (λexcitation = 485 nm; λemission = 530 nm) every 5 min at 37°C for 1 h. Ascorbic acid was used as the reference standard. The capacity of each extract for scavenging peroxyl radicals was calculated using Equation 2, where As is the area under the curve (AUC) of fluorescein in the sample, At is the AUC of the ascorbic acid, Ab is the AUC of the control (blank of the extraction), k is the dilution factor, a is the concentration of the ascorbic acid in mg/ml, and h is the ratio between the grams of extract and the grams of plant material used for the extraction (mg AA/g).
Equation 2
Gas chromatography coupled to mass spectrometry
Metabolite profiling of soybean n-heptane and NaHDES extracts (10 mg/ml) was performed using a 7890B Agilent system (Agilent Technologies, United States) coupled to a quadrupole time-of-flight (QToF) 7200 (Agilent Technologies, United States) equipped with an electronic ionization (EI) interface. Separations were achieved in a 30 m × 250μm × 0.25μm DB5- MS + 10 m Duragard Capillary Column (Zorbax, Agilent Technologies, United States). The samples were diluted at 1:10 in n-heptane and filtered with a 0.22-μm nylon syringe filter before injection. The injection volume was 1μl using a split flow of 8.4 ml/min. The helium flow rate was 0.8 ml/min. The injector temperature was 250°C. Oven temperature started at 60°C (1 min), followed by 325°C at the rate of 10°C°/min (10 min) and 11-min solvent delay. Metabolites present in the n-heptane and eucalyptol/menthol (1:1) extracts were annotated using the match of mass spectra in the Agilent Mass Hunter Unknown Analysis tool and mass spectral databases (i.e., NIST MS Search v.2.0 and Fiehn Lib).
Ultra-high-performance liquid chromatography coupled to mass spectrometry
Extracts of the individual parts of soy, i.e., branches, leaves, pods, roots, and soybeans, from the optimal condition of PLE (ChCl:Ca:H2O at 120°C), which presented the highest TPC and TFC), were diluted 1:10 in EtOH-H2O 7:3 (v/v) and filtered with a 0.22-μm nylon syringe filter before injection. Analyses were performed in an Agilent 1290 UHPLC system (Agilent Technologies, United States) coupled to an Agilent 6540 quadrupole-time-of-flight mass spectrometer (QToF-MS), equipped with an orthogonal ESI source (Agilent Jet Stream, United States). The analysis in positive and negative modes was performed using a C18 column, 100 mm × 2.1 mm; 1.8μm (Zorbax Eclipse Plus, Agilent Technologies, United States) and H2O and ACN, both acidified with 0.01% formic acid (v/v) (A and B, respectively) in the following gradient elution: 0–30% B in 0–7 min, 30–80% B in 7–9 min, 80–100% B in 9–11 min, 100% B in 11–13 min, and 0% B in 13–14 min. The flow rate was kept at 0.5 ml/min, the temperature of the column was 30°C, and the sample injection volume was 5μl. MS parameters were as follows: capillary voltage, 4,000 V; nebulizer pressure, 40 psi; drying gas flow rate, 10 L/min; gas temperature, 350°C; skimmer voltage, 45 V; fragmentor voltage, 110 V. The MS and auto-MS/MS modes were set to acquire m/z values ranging between 50 and 1,100 and 50–800, respectively, at a scan rate of 5 spectra per second. Agilent Mass Hunter Qualitative Analysis software (B.07.00) was used for post-acquisition data processing. Metabolites present in the extracts were annotated using Global Natural Product Social Molecular Networking (GNPS)2 (54). First, MS data were converted to mzML format with ProteoWizard 3.0.6002 package MSConvert software (ProteoWizard, United States). The converted files were uploaded to GNPS platform, and a molecular network was created using the online workflow.3 The data were filtered by removing all MS/MS fragment ions within ± 17 Da of the precursor m/z. MS/MS spectra were window filtered by choosing only the top six fragment ions in the ± 50Da window throughout the spectrum. The precursor ion mass and MS/MS fragment ion tolerances were set to 0.02 Da. A cosine score above 0.65 and more than four matched peaks were used to create the edges of the network. The spectra in the network were searched against GNPS’ spectral libraries. The library spectra were filtered in the same manner as the input data. The product ion spectra presented in GNPS was manually verified with previous literature annotation. In addition, isoflavonoids and flavonoids which have already been identified in soy have their m/z and MS/MS spectra searched in the chromatograms using MZmine 2.53 software4 (55, 56).
Results and discussion
Pressurized liquid extraction first step—Defatting soy with n-heptane and alternative natural hydrophobic deep eutectic solvents
The kinetic curves corresponding to the first step of PLE of soy (including soybeans and the mix of soy by-products) are shown in Figure 2. Such curves are related to the accumulated yield (%) of the defatting procedure of the soybeans (reference material - A) and of the mix of soy by-products (branches, leaves, pods, and roots - B) using n-heptane as the reference solvent. The extraction of soybeans reached equilibrium after 20 min of extraction with an accumulated yield of 21.14% at 120°C (Figure 2A). On the other hand, the defatting process for the mix of soy by-products resulted in an accumulated yield lower than 3% for all monitored temperatures and extraction times. Therefore, defatting was not applied to the mix and individual parts of soy by-products since it would involve an additional step with more solvent and energy consumption with a small benefit in terms of oil yield.
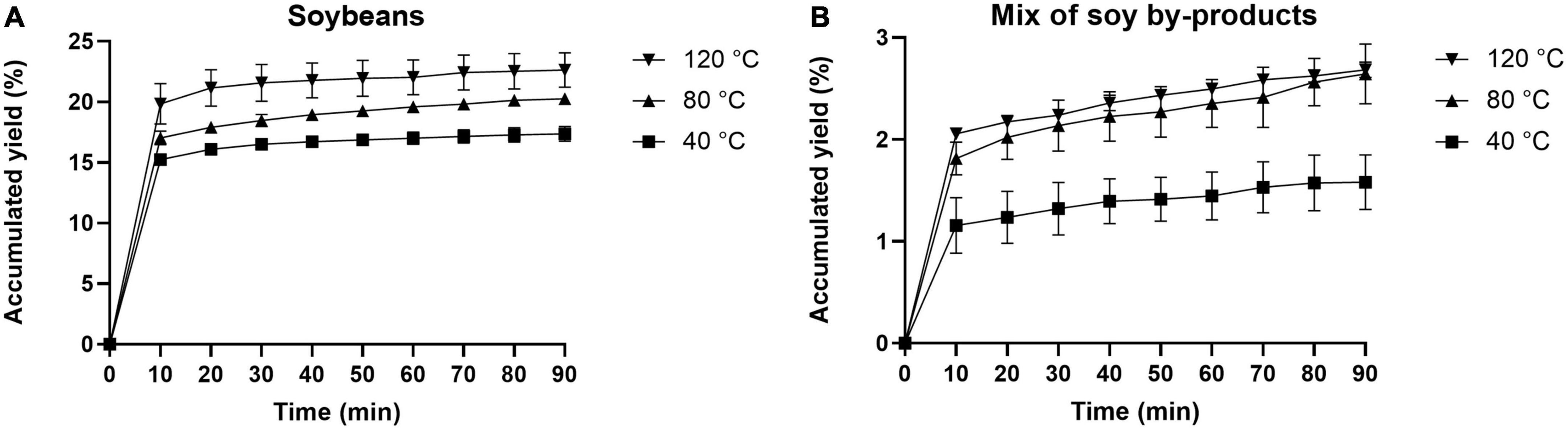
Figure 2. Kinetic curves based on the yields of soybean and mix of soy by-products extracts generated by PLE using n-heptane at three different temperatures (40, 80, and 120°C).
As an alternative to n-heptane, 108 candidates of NaHDESs, which have already been described in the literature, were screened in silico to defat soybeans (28–45). In contrast to the usual approach to HSPs, when the target is a bioactive compound, this work used a solvent, n-heptane, as a target to find other similar (and greener) options. The HSP approach was applied using HSPiP® software, allowing the calculation of HSP for NaHDES. A lower distance (Ra) between NaHDES and n-heptane means a higher similarity between them and a potential replacer to the benchmarking solvent. Supplementary Table 1 presents the HSP and Ra values of NaHDES, and Table 1 contains the referred values of the three selected NaHDES and n-heptane. Eucalyptol/menthol 1:1 (EM) presented a higher similarity to n-heptane, with a Ra of 6.33. Such NaHDES candidate has already been applied to extract astaxanthin from brown crab shell residues (45). EM was the least polar and viscous terpene-based mixture in quoted work. The second NaHDES candidate selected was camphor/menthol (1:1) (CM) as it contains one of the same constituents as the first, menthol, and similar Ra (5th place on Supplementary Table 1—Ra of 7.13). It reduces the requirement of the other two substances and contains a percentage difference of 3.63% from the second-ranked NaHDES, borneol/oleic acid (1:4). Camphor/thymol (3:2) was the third mixture chosen as it presents one of the same constituents of the latter mixture and contains intermediate properties among the other two proportions (1:1 and 2:1) of its components.
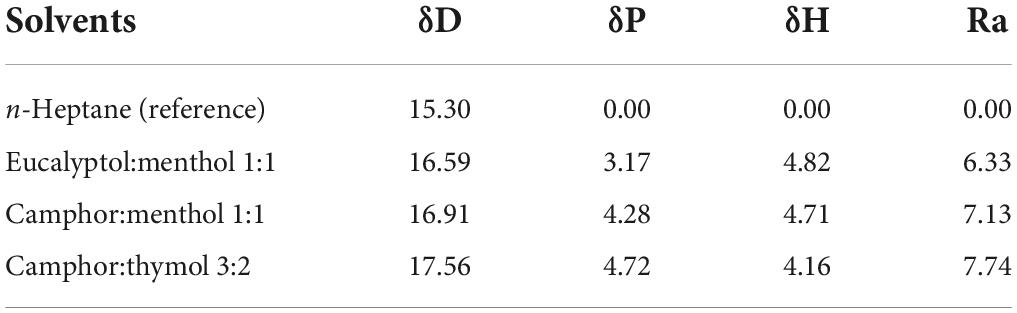
Table 1. Hansen solubility parameters (δD, δP, and δH) of n-heptane, the reference solvent, and the three selected natural hydrophobic deep eutectic solvents (NaHDES).
Then, the three selected NaHDES candidates were initially tested to defat soybean using PLE at 90, 120, and 150°C. The temperature of 120°C was set as the intermediate temperature since it was the best temperature for n-heptane. The achieved yields for the three mixtures were similar to those observed for n-heptane, with an accumulated yield of 22.34 ± 1.43% (Table 2 and Supplementary Figure 2). The extraction time was fixed at 20 min, resulting in the equilibrium of the accumulated yield with the reference solvent, as previously described. No statistical differences (p > 0.05) were observed for the three mixtures at the three temperatures. It means that extractions with NaHDES candidates at 90°C could be successfully used to replace n-heptane to defat soybean at 120°C. Similar results were achieved by Claux et al. (15). In their work, soybean defatting was performed using dry and aqueous 2-methyloxolane (2-MeTHF), resulting in a similar accumulated yield (23.7 ± 0.1) to our results. For the following steps, EM was selected as the best mixture to defat soybean as it presents similar yields compared to the other options and their components showed lower toxicity (57–60). Gasparetto et al. highlighted green alternatives to extract soybean oil (61). Solvents, such as terpenes, cyclopentyl methyl ether (CPME), and 2-MeTHF, have been applied as suitable replacers for hexane. Moreover, mixtures of ethanol with alkyl ester or ethyl acetate, as well as α-pinene were studied to defat soybean, achieving promising results (62–64). In addition to the HSP, COnductor-like Screening MOdel for Real Solvents (COSMO-RS) can also be employed for screening greener solvents for defatting process, as described by Sicaire et al. (65, 66) and Cascan et al. (67).
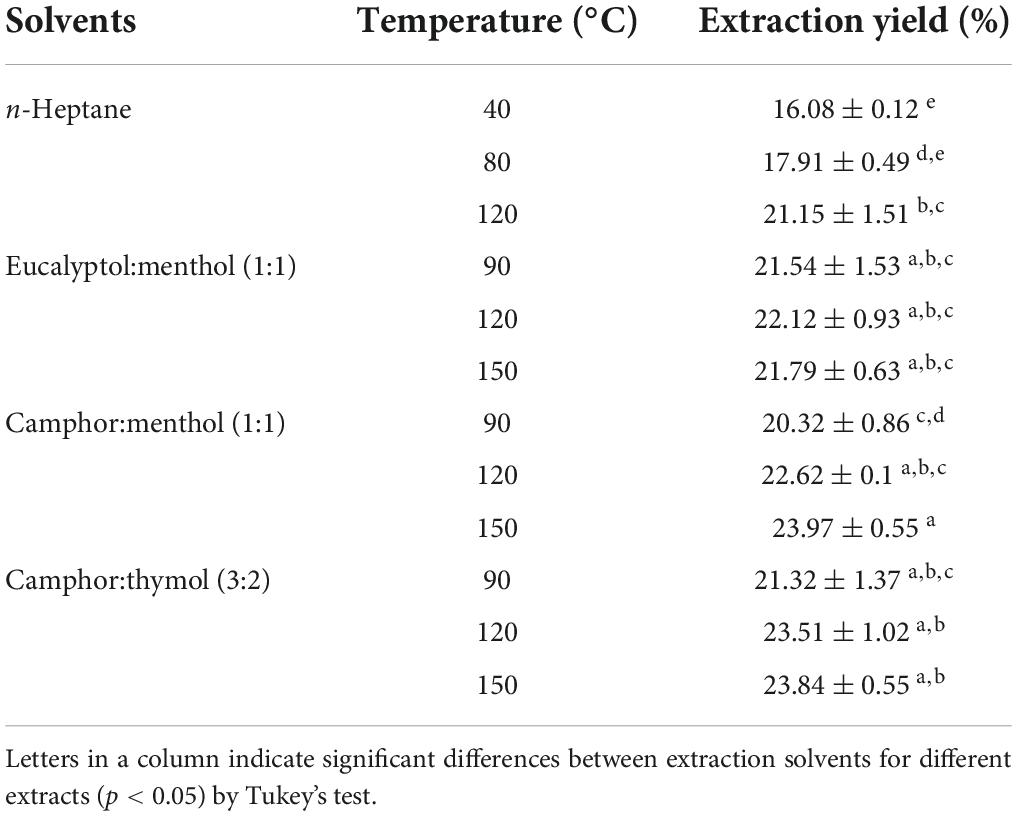
Table 2. Extraction yield of the defatting process of soybeans employing pressurized liquid extraction (PLE) with n-heptane at 40, 80, and 120°C and three selected NaHDES candidates, eucalyptol/menthol (1:1), camphor/menthol (1:1), camphor/thymol (3:2), at 90, 120, and 150°C.
Additionally, Figure 3 shows the GC-QToF-MS chromatograms of the fractions corresponding to the lower and higher temperature of n-heptane (40 and 120°C) and EM (90 and 150°C) pressurized extractions. As represented in the chromatograms, the temperature did not promote the differences in the metabolite profile. Compounds 1 and 2 were identified as linolenic acid and δ-tocopherol, respectively. In n-heptane chromatograms, higher intensity of δ-tocopherol was achieved, and in contrast, the peak related to linolenic acid was more intense in EM chromatograms. The peaks marked with (*) were related to phthalate contaminants identified in NaHDES candidate extracts. Further analysis should be employed focused on replacing n-heptane (hexane) to defat soybeans as proposed by Claux et al. (15). The analysis of fatty acid profile, neutral lipids, phospholipids, and unsaponifiable compounds will comprehensively determine the potential application of NaHDES candidates in the defatting process.
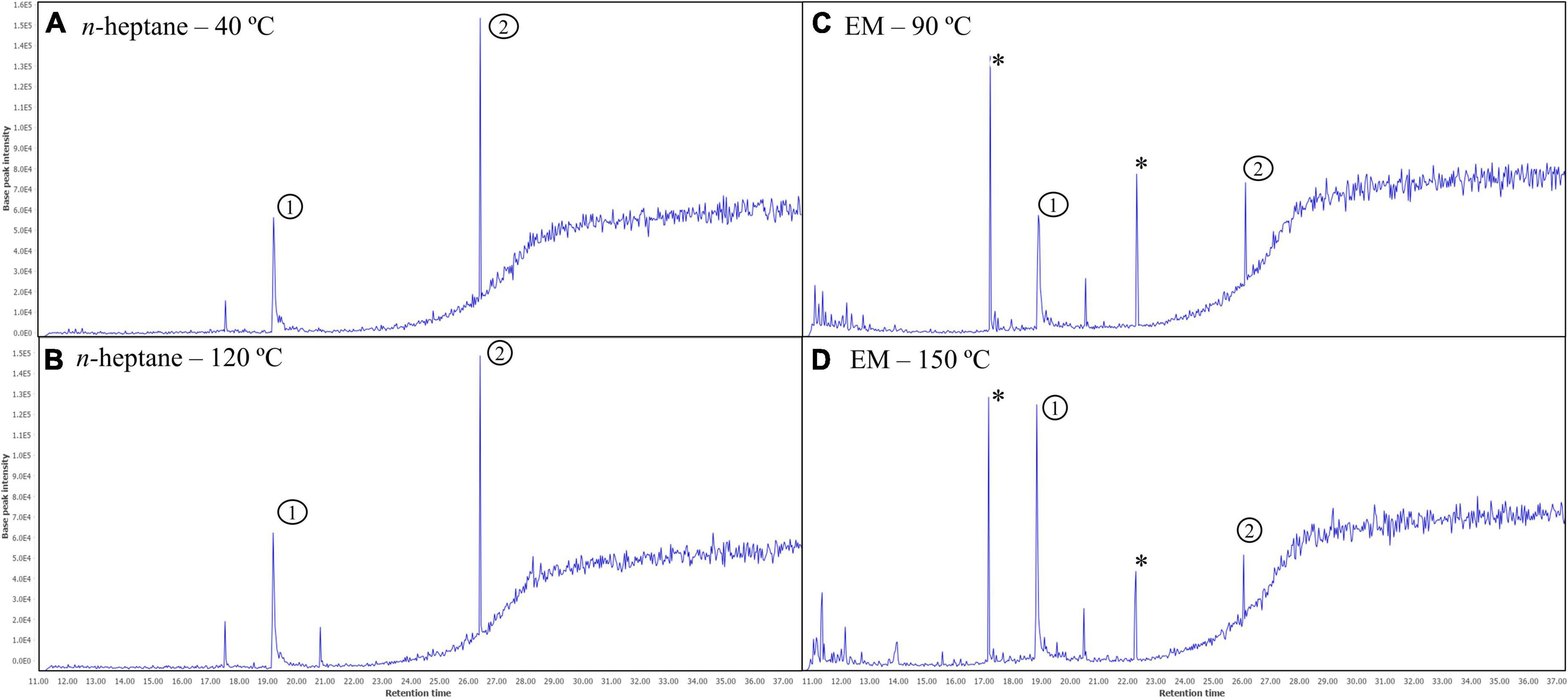
Figure 3. GC-QToF-MS chromatograms of the lower and higher temperature of soybean n-heptane extracts at 40 (A) and 120°C (B) and eucalyptol/menthol (1:1) – EM at 90 (C) and 150°C (D) performed by PLE. The peaks marked with * were related to phthalate contaminants identified in NaHDES candidate extracts.
Pressurized liquid extraction second step—Extraction of phenolic compounds
The total flavonoid (TFC) and phenolic (TPC) content of the mix of soy by-products were set as the response to optimize the pressurized conditions to extract the individual parts of soy and soybeans, as presented hereafter. First, PLE was performed using three temperatures: 60, 90, and 120°C; two solvents: (i) EtOH-H2O 7:3 (v/v) and the NADES (ii) choline chloride/citric acid/water (1:1:11) (ChCl:Ca:H2O); 20 min of extraction was set since it achieved the best result in the first step of PLE (Supplementary Figure 3). Such NADES was selected as its constituents and ratio have already been optimized to extract isoflavones and solubilize flavonoids under low-pressure conditions (47, 48). Bajkacz and Adamek determined the best conditions to extract daidzin, genistin, genistein, and daidzein from soy products with choline chloride/citric acid (molar ratio of 1:1 with 30% of water) at 60°C in ultrasound-assisted extraction (UAE) (48). Tang et al. found that a similar NADES ratio and constituents, with a slight modification in water content (37%, which means ChCl:Ca:H2O - 1:1:11), presents a higher capacity to extract, separate, or purify flavonoids, such as phloretin, phlorizin, and naringin DC (47). Our work selected the NADES ratio with 37% of water because it provides a lower viscosity solvent, enabling their pressurization by the PLE pump. Moreover, the TPC and TFC of PLE extractions were compared with dynamic maceration using (i) ChCl:Ca:H2O (at the same ratio as above) and (ii) EtOH-H2O 7:3 (v/v) at 60°C as the reference method. Additionally, GXLs with ChCl:Ca:H2O were tested with three CO2 percentages (25, 50, and 75%) at 60°C to reduce the amount of NADES to be employed while favoring viscosity and diffusivity of the compounds in the solvent. Mechanical problems to maintain the pressure at lower and higher CO2 percentages determined the utilization of just 50% in the viscous NADES. To the best of our knowledge, the literature does not report the combination of GXLs with NADES. Then, an updated GXLs equipment could be proposed as a potential innovation to deal with the solvents with a higher viscosity since, as mentioned, it could decrease their viscosity, modify the pH of the extraction media, and change other chemical and/or physical properties (24).
The results of TFC and TPC of pressurized ChCl:Ca:H2O and EtOH-H2O 7:3 (v/v), as well as GXLs and dynamic maceration extractions, are displayed in Table 3. Pressurized ChCl:Ca:H2O at 90 and 120°C and pressurized EtOH-H2O 7:3 (v/v) at 120°C presented similar results (p > 0.05). However, the highest average of TFC and TPC was achieved at 120°C and ChCl:Ca:H2O, which represents 369.74 ± 8.78 μg QE/g and 5.63 ± 1.11 mg GAE/g, respectively. The lowest TFC and TPC were achieved at 60°C by dynamic maceration with EtOH-H2O 7:3 (v/v), 136.30 ± 23.8 μg QE/g, and gas-expanded ChCl:Ca:H2O, which content was not detected, respectively. Aboushanab et al. extracted isoflavones from kudzu roots and soy molasses using choline chloride and citric acid at a 1:2 molar ratio (68). They achieved the TPC and TFC of 15.20 ± 3.47 mg GAE/g and 6.12 ± 0.51 mg QE/g, respectively, in soy molasses extracts. The comparison between the quoted results with our work is inadequate since they washed three times their NADES extracts with ethyl acetate 1:3 (v/v) ratio and concentrated such fractions. The dried extracts were used to perform the assays, promoting a higher concentration of TPC and TFC (68). Combining pressurized hot water extraction (PHWE) and NADES as modifiers, Loarce et al. achieved a promising approach to extract anthocyanins from grape pomace (22). In total, eight NADES mixtures were tested, and the best NADES composition was added at three percentages in PHWE (10, 20, and 30%). Subsequently, the best composition was studied at four temperatures (40, 60, 80, and 100°C). A PHWE with 30% choline chloride/oxalic acid 1:1 (molar ratio) resulted in the best results. In their work, higher concentrations of NADES demonstrated a higher content of anthocyanin and pyroanthocyanins. In contrast, extractions made at 60, 80, and 100°C did not present significant differences (p > 0.05) in quoted responses. Rachmaniah et al. applied pressurized NADES to extract alkaloids from Narcissus pseudonarcissus. The best results were accomplished using malic acid/sucrose/water (1:1:5) at 50 bar and 50°C, with similar results to the exhaustive Soxhlet extraction with methanol (69). Moreover, Benvenutti et al. performed pressurized DES extractions of Brazilian berry processing by-product resulting in fractions with potential antioxidant, anti-diabetic, and anti-obesity properties (23). DES solution of choline chloride with propylene glycol or malic acid combined with PLE promoted extracts with yields 50% higher than water, acidified water (pH 1.5), and hydroethanolic solution (47% of EtOH in water), which represent the conventional solvents for anthocyanins extraction.
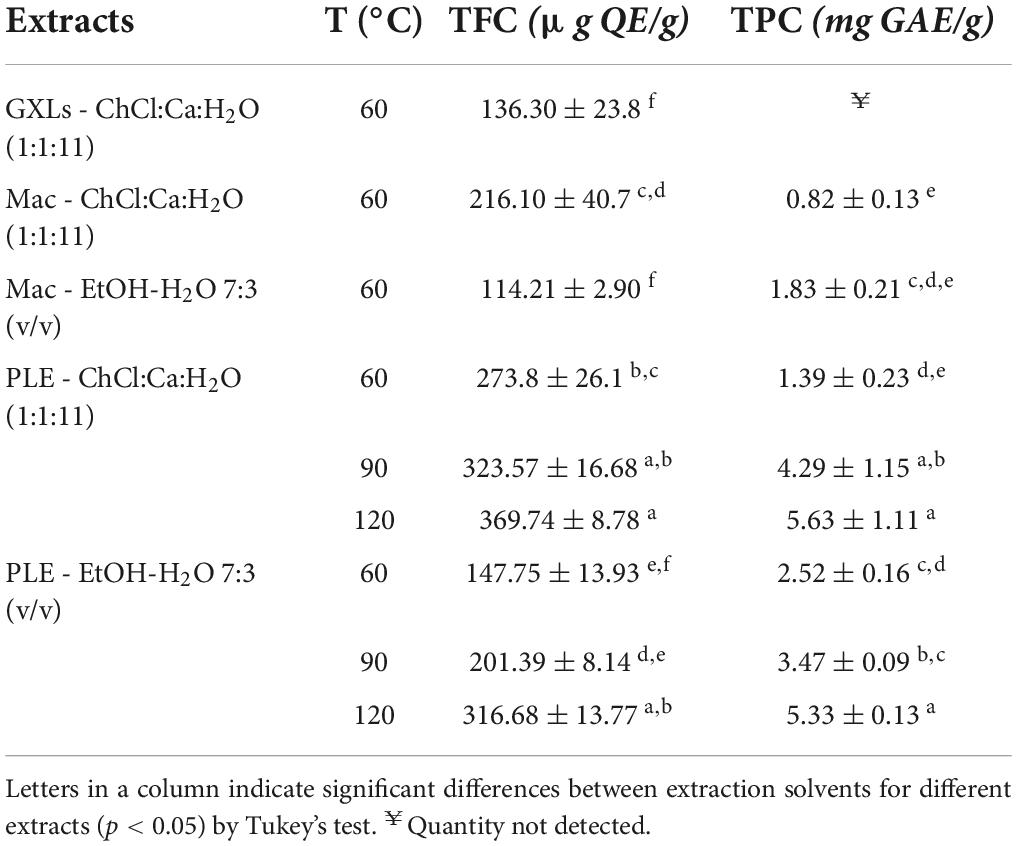
Table 3. Total flavonoids (TFC) and phenolic content (TPC) of the mix of agro-soy by-products (i.e., branches, leaves, pods, and roots) from different extraction conditions.
Analysis of the individual parts of soy by-products and soybean
The highest TPC and TFC for the extracts prepared from the mix of soy by-products were achieved using PLE at 120°C with ChCl:Ca:H2O. Then, this condition was applied to extract soy by-products individually and soybeans (previously defatted by pressurized EM); results are presented in Table 4. Extracts of soy leaves followed by pods presented the higher TFC, 419.10 ± 33.8 and 250.10 ± 20.00 μg QE/g, respectively. Soy branches, roots, and soybeans did not present a significant difference (p > 0.05) in TFC. For soy by-products, the average value of TPC was 8.45 ± 2.26 mg GAE/g, with no difference among them. However, soybeans contained the lowest TPC value, 1.01 ± 0.18 mg GAE/g. Comparisons between the content of bioactive compounds, specifically isoflavones, of soy by-products and soybeans were reported by Carneiro et al. (10). The authors found that soy by-products have 132% of the total isoflavones quantified in the soybeans, representing that soy by-products are potential sources of phenolic compounds. In addition, Cabezudo et al. achieved a higher content of phenolic compounds in soy hull, a soy by-product, than in soybeans (70). They performed a green extraction based on an alkaline hydrolysis treatment, resulting in a TPC of 0.72 g gallic acid equivalents per 100 g of soybean hull. Gupta and Chen promoted a higher content of daidzein and genistein in fermented okara (soymilk by-product) extracts using Rhizopus oligosporus compared to the unfermented material (71). Moreover, the fermented extracts presented a TPC and TFC of 709.33 ± 2.92 and 10.77 ± 1.25 mg of gallic acid and quercetin equivalents per g of okara samples, respectively. Regarding the antioxidant properties of the soy by-products and commodity extracts, ORAC was determined by ROS scavenging capacity method (Table 4). Soy root extracts presented the highest antioxidant capacity, 207.48 ± 40.23 mg AA/g, with three times more activity than soybeans extracts, 68.96 ± 12.30. Leaves and branches contained similar antioxidant capacity, with 124.75 ± 12.71 and 108.33 ± 20.61 mg AA/g. In contrast, pods had the lowest value, 60.74 ± 13.60 mg AA/g. Dorta et al. compared the TPC and ORAC of mango by-products, concluding that both responses did not present a correlation (72). In contrast, applying a combination of PLE and NADES, Benvenutti et al. found a correlation between TPC and antioxidant capacity of Brazilian berry processing by-product extracts (23). In addition, intermediate DES concentrations, flow rate, and temperature increased the antioxidant potential of the extracts. Performing subcritical water with NADES, Loarce et al. accomplished extracts with a higher content of catechins, tannins, hydroxycinnamic acids, and flavonols from winemaking by-products (21). Besides, they referred that quoted bioactive metabolites contribute to antioxidant capacity. According to the best of our knowledge, the literature does not report the combination of PLE and NADES to generate phenolic-rich fractions with high antioxidant capacity from agro-soy by-products, which have been described as the promising sources of bioactive compounds and sparsely explored (11).
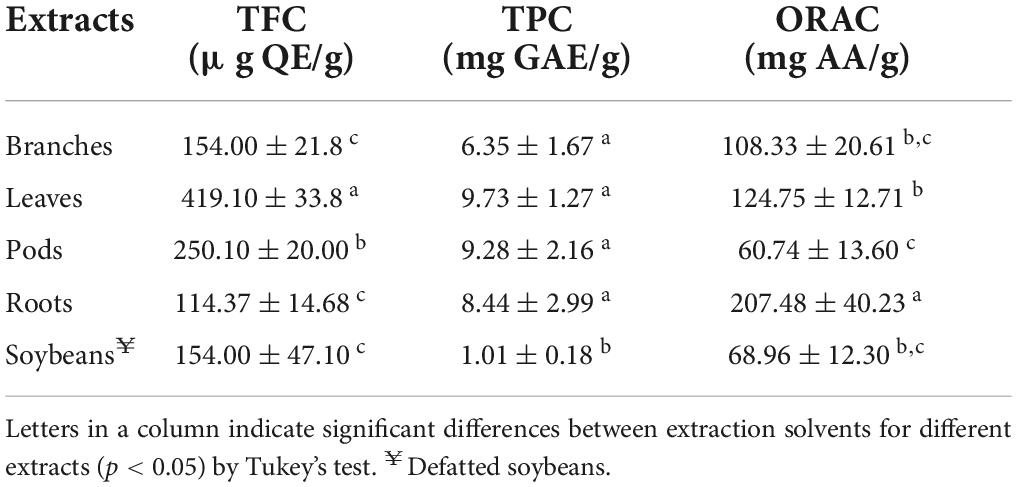
Table 4. Total content of flavonoids (TFC) and phenolics (TPC), as well as antioxidant capacity (ORAC) of the individual extracts of soy by-products (i.e., branches, leaves, pods, and roots) and soybeans resulted from pressurized choline chloride/citric acid/water (1:1:11) at 120°C, 100 bar, and 20 min of extraction.
Pressurized ChCl:Ca:H2O extracts were also analyzed by UHPLC-ESI-QToF-MS/MS. The annotation of the MS/MS spectra was performed by comparing its data against the GNPS spectral reference library and manually checking by MZmine (54). Additionally, the candidates obtained by GNPS had their compatibility challenged with the acquired high-resolution mass and with previous reports of their occurrence in soy by-products as organized in a database published elsewhere (5). Table 5 summarizes the annotation of identified metabolites, focusing on the most important metabolites in soy, flavonoids, and isoflavonoids. Isoflavonoids such as daidzin and genistin, as well as flavonoid apigenin, were found in all soy parts. Such metabolites were related to potential bioactive properties against cancer and anti-inflammatory capacity (7, 73–75). Moreover, bioactive isoflavonoids, such as formononetin 7-O-glucoside (ononin) and biochanin A 7-O-glucoside (astroside), were identified in at least one soy by-product. Ononin has been related as an anti-parasitic drug, and astroside contains potential properties to maximize the microbial community function in animals (76, 77).
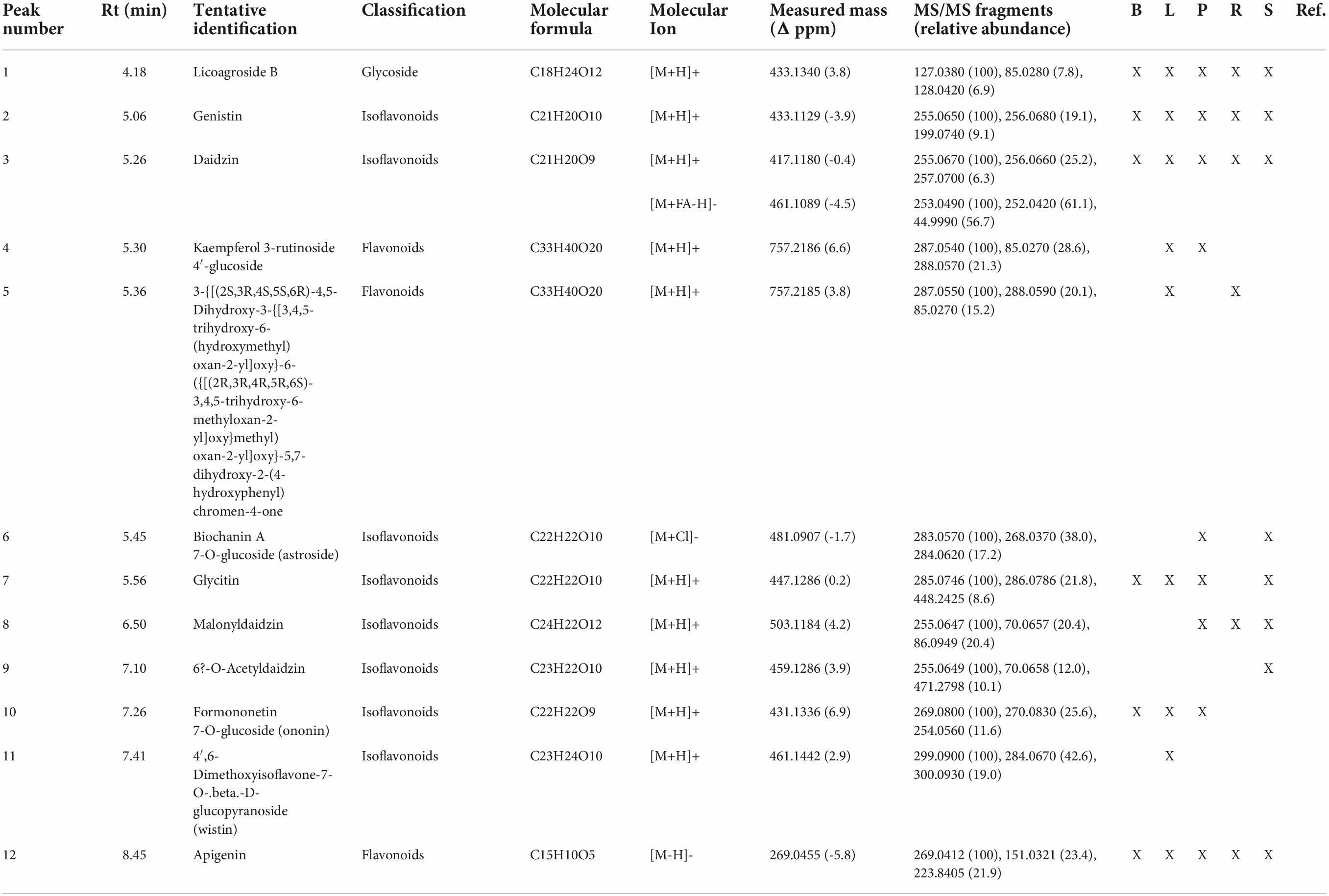
Table 5. List of tentatively identified compounds in the pressurized choline chloride/citric acid/water (1:1:11) extracts of soy branches (B), leaves (L), pods (P), roots (R), and soybeans (S) by UHPLC-ESI-QToF-MS/MS.
Despite the high temperature, 120°C, which could affect the glucoside forms of the flavonoids and isoflavonoids, 6 -O-acetyldaidzin, malonyldaidzin, daidzin, genistin, glycitin, and kaempferol 3-rutinoside 4′-glucoside were also identified in the extracts. Rostagno et al. found that the degradation of malonyl-glucoside and glucosides occur over 100 and 150°C, respectively (78). Therefore, NADES composition may have interfered with the temperature effect on flavonoids and isoflavonoids. Nevertheless, PLE-NADES-based extracts were diluted before the injection in the MS systems as a precautionary step, which could have decreased the identification of minority compounds. Alternative methodologies for recovery of phenolic compounds from NADES extracts, such as liquid–liquid extraction (LLE), solid-phase extraction (SPE), and supercritical carbon dioxide extraction (SFC), may be feasible approaches to concentrate target metabolites and reduce the interference of NADES components, as mentioned by Mišan et al. (79).
Conclusion
PLE using choline chloride/citric acid/water (1:1:11—molar ratio) at 120°C, 100 bar, and 20 min (2 cycles of 10 min) resulted in an optimized conditions to extract phenolics and flavonoids from agro-soy by-products. Extracts of soy leaves obtained under these conditions contained the highest TFC, followed by pods, with 419.10 ± 33.8 and 250.10 ± 20.00 μg QE/g, respectively. Regarding TPC, soy by-products contain similar content and are higher than soybeans, whereas soy root extracts presented the highest antioxidant capacity, 207.48 ± 40.23 mg AA/g. This was three times higher than that observed for soybeans extracts, with 68.96 ± 12.30 mg AA/g. Moreover, bioactive compounds, such as apigenin (flavonoid) and daidzin and genistin (isoflavonoids), were identified in PLE-NADES-based extracts of all parts of soy. Additionally, a new defatting process was developed using pressurized NaHDES candidates, with a similar yield and chromatography profile compared to n-heptane, becoming a new and greener alternative to conventional soybean oil extraction.
This work promotes new insights into the use of pressurized and gas-expanded NADES, which have been sparsely combined and present promising applications. Such solvents can be green alternatives to extract bioactive compounds and, combined with pressurized techniques, could be a powerful approach to generate phenolic-rich fractions with human health benefits. In addition, the use of an abundant agricultural by-product, i.e., soy branches, leaves, pods, and roots, which contains several bioactive compounds, is related to a circular and greener economy, as suggested by the goal 12, “Sustainable consumption and production” of the 17 sustainable development goals (SDGs) set by the United Nations (UN).
Data availability statement
The original contributions presented in this study are included in the article/Supplementary material, further inquiries can be directed to the corresponding author.
Author contributions
FB, BS-R, JM, AC, CF, and EI: conceptualization and methodology. FB, CF, and EI: writing – original draft preparation, review, and editing. FB: software and data curation. All authors have read and agreed to the published version of the manuscript.
Funding
The authors thank the São Paulo Research Foundation—FAPESP (2020/09500-0, 2018/21128-9, 2018/01786-1, 2017/06216-6, and 2014/50926-0) and the Coordenação de Aperfeiçoamento de Pessoal de Nível Superior—Brasil—CAPES (Finance Code 001). BS-R also thank the Ministry of Science, Innovation and Universities for her “Juan de la Cierva” postdoctoral grant.
Conflict of interest
The authors declare that the research was conducted in the absence of any commercial or financial relationships that could be construed as a potential conflict of interest.
Publisher’s note
All claims expressed in this article are solely those of the authors and do not necessarily represent those of their affiliated organizations, or those of the publisher, the editors and the reviewers. Any product that may be evaluated in this article, or claim that may be made by its manufacturer, is not guaranteed or endorsed by the publisher.
Supplementary material
The Supplementary Material for this article can be found online at: https://www.frontiersin.org/articles/10.3389/fnut.2022.953169/full#supplementary-material
Footnotes
- ^ https://www.chemaxon.com
- ^ http://gnps.ucsd.edu
- ^ https://ccms-ucsd.github.io/GNPSDocumentation/
- ^ https://mzmine.github.io/
References
1. Newman DJ, Cragg GM. Natural products as sources of new drugs over the nearly four decades from 01/1981 to 09/2019. J Nat Prod. (2020) 83:770–803. doi: 10.1021/acs.jnatprod.9b01285
2. Zhang F, Wang T, Wang X, Lü X. Apple pomace as a potential valuable resource for full-components utilization: a review. J Clean Prod. (2021) 329:129676. doi: 10.1016/J.JCLEPRO.2021.129676
3. da Silva MR, Bragagnolo FS, Carneiro RL, de Oliveira Carvalho Pereira I, Ribeiro JAA, Martins C, et al. Metabolite characterization of fifteen by-products of the coffee production chain: from farm to factory. Food Chem. (2021) 369:130753. doi: 10.1016/J.FOODCHEM.2021.130753
4. Suárez Montenegro ZJ, Álvarez-Rivera G, Mendiola JA, Ibáñez E, Cifuentes A. Extraction and mass spectrometric characterization of terpenes recovered from olive leaves using a new adsorbent-assisted supercritical CO2 process. Foods. (2021) 10:1301. doi: 10.3390/FOODS10061301
5. Bragagnolo FS, Funari CS, Ibáñez E, Cifuentes A. Metabolomics as a tool to study underused soy parts: in search of bioactive compounds. Foods. (2021) 10:1308. doi: 10.3390/FOODS10061308
6. Choi YR, Shim J, Kim MJ. Genistin: a novel potent anti-adipogenic and anti-lipogenic agent. Molecules. (2020) 25:2042. doi: 10.3390/MOLECULES25092042
7. Hwang ST, Yang MH, Baek SH, Um JY, Ahn KS. Genistin attenuates cellular growth and promotes apoptotic cell death breast cancer cells through modulation of ERalpha signaling pathway. Life Sci. (2020) 263:118594. doi: 10.1016/j.lfs.2020.118594
8. Xu Y, Li X, Wang H. Protective roles of apigenin against cardiometabolic diseases: a systematic review. Front Nutr. (2022) 9:875826. doi: 10.3389/FNUT.2022.875826
9. Schnarr L, Segatto ML, Olsson O, Zuin VG, Kümmerer K. Flavonoids as biopesticides – systematic assessment of sources, structures, activities and environmental fate. Sci Total Environ. (2022) 824:153781. doi: 10.1016/J.SCITOTENV.2022.153781
10. Carneiro AM, Moreira EA, Bragagnolo FS, Borges MS, Pilon AC, Rinaldo D, et al. Soya agricultural waste as a rich source of isoflavones. Food Res Int. (2020) 130:108949. doi: 10.1016/j.foodres.2019.108949
11. Bragagnolo FS, Álvarez-Rivera G, Breitkreitz MC, Ibáñez E, Cifuentes A, Funari CS. Metabolite profiling of soy by-products: a comprehensive approach. J Agric Food Chem. (2022) 70:7321–41. doi: 10.1021/ACS.JAFC.2C01050
12. USDA Foreign Agricultural Service.Oilseeds: world markets and trade. (2022). Available online at: https://www.fas.usda.gov/data/oilseeds-world-markets-and-trade (accessed May 15, 2022).
13. Potrich E, Miyoshi SC, Machado PFS, Furlan FF, Ribeiro MPA, Tardioli PW, et al. Replacing hexane by ethanol for soybean oil extraction: modeling, simulation, and techno-economic-environmental analysis. J Clean Prod. (2020) 244:118660. doi: 10.1016/J.JCLEPRO.2019.118660
14. Jokić S, Nagy B, Zeković Z, Vidović S, Bilić M, Velić D, et al. Effects of supercritical CO2 extraction parameters on soybean oil yield. Food Bioprod Process. (2012) 90:693–9. doi: 10.1016/J.FBP.2012.03.003
15. Claux O, Rapinel V, Goupy P, Patouillard N, Vian MA, Jacques L, et al. Dry and aqueous 2-methyloxolane as green solvents for simultaneous production of soybean oil and defatted meal. ACS Sustain Chem Eng. (2021) 9:7211–23. doi: 10.1021/ACSSUSCHEMENG.0C09252
16. Sánchez-Camargo AD, Bueno M, Parada-Alfonso F, Cifuentes A, Ibáñez E. Hansen solubility parameters for selection of green extraction solvents. Trends Analyt Chem. (2019) 118:227–37. doi: 10.1016/J.TRAC.2019.05.046
17. UNDP. Goal 12: responsible consumption and production. New York, NY: United Nations Development Programme (2016).
18. Choi YH, van Spronsen J, Dai Y, Verberne M, Hollmann F, Arends IWCE, et al. Are natural deep eutectic solvents the missing link in understanding cellular metabolism and physiology? Plant Physiol. (2011) 156:1701–5. doi: 10.1104/PP.111.178426
19. Duru KC, Slesarev GP, Aboushanab SA, Kovalev IS, Zeidler DM, Kovaleva EG, et al. An eco-friendly approach to enhance the extraction and recovery efficiency of isoflavones from kudzu roots and soy molasses wastes using ultrasound-assisted extraction with natural deep eutectic solvents (NADES). Ind Crops Prod. (2022) 182:114886. doi: 10.1016/J.INDCROP.2022.114886
20. Alvarez-Rivera G, Bueno M, Ballesteros-Vivas D, Mendiola JA, Ibañez E. Pressurized liquid extraction. In: CF Poole editor. Liquid-Phase Extraction. Handbooks in Separation Science. (Amsterdam: Elsevier) (2019). p. 375–98. doi: 10.1016/B978-0-12-816911-7.00013-X
21. Loarce L, Oliver-Simancas R, Marchante L, Díaz-Maroto MC, Alañón ME. Implementation of subcritical water extraction with natural deep eutectic solvents for sustainable extraction of phenolic compounds from winemaking by-products. Food Res Int. (2020) 137:109728. doi: 10.1016/J.FOODRES.2020.109728
22. Loarce L, Oliver-Simancas R, Marchante L, Díaz-Maroto MC, Alañón ME. Modifiers based on natural deep eutectic mixtures to enhance anthocyanins isolation from grape pomace by pressurized hot water extraction. LWT. (2021) 149:111889. doi: 10.1016/J.LWT.2021.111889
23. Benvenutti L, Zielinski AAF, Ferreira SRS. Pressurized aqueous solutions of deep eutectic solvent (DES): a green emergent extraction of anthocyanins from a Brazilian berry processing by-product. Food Chem X. (2022) 13:100236. doi: 10.1016/J.FOCHX.2022.100236
24. Herrero M, Mendiola JA, Ibáñez E. Gas expanded liquids and switchable solvents. Curr Opin Green Sustain Chem. (2017) 5:24–30. doi: 10.1016/J.COGSC.2017.03.008
25. Prat D, Wells A, Hayler J, Sneddon H, McElroy CR, Abou-Shehada S, et al. CHEM21 selection guide of classical- and less classical-solvents. Green Chem. (2015) 18:288–96. doi: 10.1039/c5gc01008j
26. Nowak PM, Wietecha-Posłuszny R, Pawliszyn J. White analytical chemistry: an approach to reconcile the principles of green analytical chemistry and functionality. Trends Analyt Chem. (2021) 138:116223. doi: 10.1016/J.TRAC.2021.116223
27. del Pilar Sánchez-Camargo A, Pleite N, Herrero M, Cifuentes A, Ibáñez E, Gilbert-López B. New approaches for the selective extraction of bioactive compounds employing bio-based solvents and pressurized green processes. J Supercrit Fluids. (2017) 128:112–20. doi: 10.1016/J.SUPFLU.2017.05.016
28. van Osch DJGP, Dietz CHJT, van Spronsen J, Kroon MC, Gallucci F, van Sint Annaland M, et al. A search for natural hydrophobic deep eutectic solvents based on natural components. ACS Sustain Chem Eng. (2019) 7:2933–42. doi: 10.1021/acssuschemeng.8b03520
29. Florindo C, Romero L, Rintoul I, Branco LC, Marrucho IM. From phase change materials to green solvents: hydrophobic low viscous fatty acid-based deep eutectic solvents. ACS Sustain Chem Eng. (2018) 6:3888–95. doi: 10.1021/acssuschemeng.7b04235
30. Ribeiro BD, Florindo C, Iff LC, Coelho MAZ, Marrucho IM. Menthol-based eutectic mixtures: hydrophobic low viscosity solvents. ACS Sustain Chem Eng. (2015) 3:2469–77. doi: 10.1021/acssuschemeng.5b00532
31. Morelli DC, Bernardi G, Morés L, Pierri ME, Carasek E. A green - high throughput –extraction method based on hydrophobic natural deep eutectic solvent for the determination of emerging contaminants in water by high performance liquid chromatography – diode array detection. J Chromatogr A. (2020) 1626:461377. doi: 10.1016/j.chroma.2020.461377
32. Ye X, Ye B, Xu J, Fang M, Dong D, Wu C, et al. A modified quick, easy, cheap, effective, rugged, and safe method with hydrophobic natural deep eutectic solvent as extractant and analyte protectant for analyzing pyrethroid residues in tomatoes. J Sep Sci. (2020) 43:3546–54. doi: 10.1002/jssc.202000547
33. Nia NN, Hadjmohammadi MR. Amino acids- based hydrophobic natural deep eutectic solvents as a green acceptor phase in two-phase hollow fiber-liquid microextraction for the determination of caffeic acid in coffee, green tea, and tomato samples. Microchem J. (2021) 164:106021. doi: 10.1016/j.microc.2021.106021
34. Haider MB, Dwivedi M, Jha D, Kumar R, Marriyappan Sivagnanam B. Azeotropic separation of isopropanol-water using natural hydrophobic deep eutectic solvents. J Environ Chem Eng. (2021) 9:104786. doi: 10.1016/j.jece.2020.104786
35. Sereshti H, Semnani Jazani S, Nouri N, Shams G. Dispersive liquid–liquid microextraction based on hydrophobic deep eutectic solvents: application for tetracyclines monitoring in milk. Microchem J. (2020) 158:105269. doi: 10.1016/j.microc.2020.105269
36. Sas OG, Castro M, Domínguez Á, González B. Removing phenolic pollutants using deep eutectic solvents. Sep Purif Technol. (2019) 227:115703. doi: 10.1016/j.seppur.2019.115703
37. Hansen FA, Santigosa-Murillo E, Ramos-Payán M, Muñoz M, Leere Øiestad E, Pedersen-Bjergaard S. Electromembrane extraction using deep eutectic solvents as the liquid membrane. Anal Chim Acta. (2021) 1143:109–16. doi: 10.1016/j.aca.2020.11.044
38. Florindo C, Branco LC, Marrucho IM. Development of hydrophobic deep eutectic solvents for extraction of pesticides from aqueous environments. Fluid Ph Equilibria. (2017) 448:135–42. doi: 10.1016/j.fluid.2017.04.002
39. Fan Y, Wu H, Cai D, Yang T, Yang L. Effective extraction of harmine by menthol/anise alcohol-based natural deep eutectic solvents. Sep Purif Technol. (2020) 250:117211. doi: 10.1016/j.seppur.2020.117211
40. Li Y, Li X, Tang S, Yang Y. Emulsification liquid–liquid micro-extraction based on natural deep eutectic solvent for (triarylmethane) dyes determination. Chem Pap. (2020) 74:3617–26. doi: 10.1007/s11696-020-01184-4
41. Lo C, Semerel J, van den Berg C, Wijffels RH, Eppink MHM. Eutectic solvents with tuneable hydrophobicity: lipid dissolution and recovery. RSC Adv. (2021) 11:8142–9. doi: 10.1039/d1ra00306b
42. Majidi SM, Hadjmohammadi MR. Hydrophobic borneol-based natural deep eutectic solvents as a green extraction media for air-assisted liquid-liquid micro-extraction of warfarin in biological samples. J Chromatogr A. (2020) 1621:461030. doi: 10.1016/j.chroma.2020.461030
43. Ortega-Zamora C, González-Sálamo J, González-Sálamo J, Hernández-Sánchez C, Hernández-Sánchez C, Hernández-Borges J, et al. Menthol-based deep eutectic solvent dispersive liquid-liquid microextraction: a simple and quick Approach for the analysis of phthalic acid esters from water and beverage samples. ACS Sustain Chem Eng. (2020) 8:8783–94. doi: 10.1021/acssuschemeng.0c02603
44. Santana-Mayor Á, Socas-Rodríguez B, Rodríguez-Ramos R, Herrera-Herrera AV, Rodríguez-Delgado MÁ. Quality assessment of environmental water by a simple and fast non-ionic hydrophobic natural deep eutectic solvent-based extraction procedure combined with liquid chromatography tandem mass spectrometry for the determination of plastic migrants. Anal Bioanal Chem. (2021) 413:1967–81. doi: 10.1007/s00216-021-03166-1
45. Rodrigues LA, Pereira CV, Leonardo IC, Fernández N, Gaspar FB, Silva JM, et al. Terpene-Based natural deep eutectic systems as efficient solvents to recover astaxanthin from brown crab shell residues. ACS Sustain Chem Eng. (2020) 8:2246–59. doi: 10.1021/acssuschemeng.9b06283
46. Fernandes CC, Haghbakhsh R, Marques R, Paiva A, Carlyle L, Duarte ARC. Evaluation of deep eutectic systems as an alternative to solvents in painting conservation. ACS Sustain Chem Eng. (2021) 9:15451–60. doi: 10.1021/ACSSUSCHEMENG.1C04591
47. Tang N, Zhong J, Yan W. Solubilities of three flavonoids in different natural deep eutectic solvents at T = (288.15 to 328.15) K. J Chem Eng Data. (2016) 61:4203–8. doi: 10.1021/acs.jced.6b00552
48. Bajkacz S, Adamek J. Evaluation of new natural deep eutectic solvents for the extraction of isoflavones from soy products. Talanta. (2017) 168:329–35. doi: 10.1016/J.TALANTA.2017.02.065
49. Reyes FA, Mendiola JA, Ibañez E, del Valle JM. Astaxanthin extraction from Haematococcus pluvialis using CO2-expanded ethanol. J Supercrit Fluids. (2014) 92:75–83. doi: 10.1016/J.SUPFLU.2014.05.013
50. Koşar M, Dorman HJD, Hiltunen R. Effect of an acid treatment on the phytochemical and antioxidant characteristics of extracts from selected lamiaceae species. Food Chem. (2005) 91:525–33. doi: 10.1016/J.FOODCHEM.2004.06.029
51. Woisky RG, Salatino A. Analysis of propolis: some parameters and procedures for chemical quality control. J Apic Res. (2015) 37:99–105. doi: 10.1080/00218839.1998.11100961
52. Ou B, Hampsch-Woodill M, Prior RL. Development and Validation of an improved oxygen radical absorbance capacity assay using fluorescein as the fluorescent probe. J Agric Food Chem. (2001) 49:4619–26. doi: 10.1021/JF010586O
53. Ninfali P, Mea G, Giorgini S, Rocchi M, Bacchiocca M. Antioxidant capacity of vegetables, spices and dressings relevant to nutrition. Br J Nutr. (2005) 93:257–66. doi: 10.1079/BJN20041327
54. Wang M, Carver JJ, Phelan VV, Sanchez LM, Garg N, Peng Y, et al. Sharing and community curation of mass spectrometry data with global natural products social molecular networking. Nat Biotechnol. (2016) 34:828–37. doi: 10.1038/nbt.3597
55. Katajamaa M, Miettinen J, Orešič M. MZmine: toolbox for processing and visualization of mass spectrometry based molecular profile data. Bioinformatics. (2006) 22:634–6. doi: 10.1093/bioinformatics/btk039
56. Pluskal T, Castillo S, Villar-Briones A, Orešič M. MZmine 2: modular framework for processing, visualizing, and analyzing mass spectrometry-based molecular profile data. BMC Bioinformatics. (2010) 11:395. doi: 10.1186/1471-2105-11-395/TABLES/3
57. Registration Dossier – ECHA. Camphor Tree, Ext. (2021). Available online at: https://echa.europa.eu/registration-dossier/-/registered-dossier/10569/7/3/2 (accessed November 24, 2021).
58. Registration Dossier – ECHA. Cineole. (2021). Available online at: https://echa.europa.eu/registration-dossier/-/registered-dossier/13231/7/3/2 (accessed November 24, 2021).
59. Registration Dossier – ECHA. Menthol. (2021). Available online at: https://echa.europa.eu/registration-dossier/-/registered-dossier/13758/7/3/4 (accessed November 24, 2021).
60. Registration Dossier – ECHA. Thymol. (2021). Available online at: https://echa.europa.eu/registration-dossier/-/registered-dossier/11030/7/3/4 (accessed November 24, 2021).
61. Gasparetto H, de Castilhos F, Paula Gonçalves Salau N. Recent advances in green soybean oil extraction: a review. J Mol Liq. (2022) 361:119684. doi: 10.1016/J.MOLLIQ.2022.119684
62. Dagostin JLA, Carpiné D, Corazza ML. Extraction of soybean oil using ethanol and mixtures with alkyl esters (biodiesel) as co-solvent: kinetics and thermodynamics. Ind Crops Prod. (2015) 74:69–75. doi: 10.1016/J.INDCROP.2015.04.054
63. Dagostin JLA, Carpiné D, dos Santos PRS, Corazza ML. Liquid-liquid equilibrium and kinetics of ethanolic extraction of soybean oil using ethyl acetate as co-solvent. Braz J Chem Eng. (2018) 35:415–28. doi: 10.1590/0104-6632.20180352S20160175
64. Bertouche S, Tomao V, Hellal A, Boutekedjiret C, Chemat F. First approach on edible oil determination in oilseeds products using alpha-pinene. J Essent Oil Res. (2013) 25:439–43. doi: 10.1080/10412905.2013.782473
65. Sicaire AG, Vian M, Fine F, Joffre F, Carré P, Tostain S, et al. Alternative Bio-based solvents for extraction of fat and oils: solubility prediction, global yield, extraction kinetics, chemical composition and cost of manufacturing. Int J Mol Sci. (2015) 16:8430–53. doi: 10.3390/IJMS16048430
66. Sicaire AG, Abert Vian M, Fine F, Carré P, Tostain S, Chemat F. Experimental approach versus COSMO-RS assisted solvent screening for predicting the solubility of rapeseed oil. OCL. (2015) 22:D404. doi: 10.1051/OCL/2015010
67. Cascant MM, Breil C, Garrigues S, de la Guardia M, Fabiano-Tixier AS, Chemat F. A green analytical chemistry approach for lipid extraction: computation methods in the selection of green solvents as alternative to hexane. Anal Bioanal Chem. (2017) 409:3527–39. doi: 10.1007/S00216-017-0323-9/FIGURES/7
68. Aboushanab SA, Shevyrin VA, Slesarev GP, Melekhin VV, Shcheglova AV, Makeev OG, et al. Antioxidant and cytotoxic activities of kudzu roots and soy molasses against pediatric tumors and phytochemical analysis of isoflavones using HPLC-DAD-ESI-HRMS. Plants. (2022) 11:741. doi: 10.3390/PLANTS11060741
69. Rachmaniah O, Wilson EG, Choi YH, Witkamp G-J, Verpoorte R. Pressurized natural deep eutectic solvent extraction of galanthamine and related alkaloids from Narcissus pseudonarcissus. Planta Med. (2022) 88:814–25. doi: 10.1055/A-1803-3259
70. Cabezudo I, Meini MR, di Ponte CC, Melnichuk N, Boschetti CE, Romanini D. Soybean (Glycine max) hull valorization through the extraction of polyphenols by green alternative methods. Food Chem. (2021) 338:128131. doi: 10.1016/j.foodchem.2020.128131
71. Gupta S, Chen WN. A metabolomics approach to evaluate post-fermentation enhancement of daidzein and genistein in a green okara extract. J Sci Food Agric. (2021) 101:5124–31. doi: 10.1002/jsfa.11158
72. Dorta E, Rodríguez-Rodríguez EM, Jiménez-Quezada A, Fuentes-Lemus E, Speisky H, Lissi E, et al. Use of the oxygen radical absorbance capacity (ORAC) assay to predict the capacity of mango (Mangifera indica L.) by-products to inhibit meat protein oxidation. Food Anal Methods. (2017) 10:330–8. doi: 10.1007/S12161-016-0584-5/FIGURES/4
73. Li B, Hu Y, Wu T, Feng Y, Jiang C, Du H, et al. Apigenin-oxymatrine binary co-amorphous mixture: enhanced solubility, bioavailability, and anti-inflammatory effect. Food Chem. (2022) 373:131485. doi: 10.1016/J.FOODCHEM.2021.131485
74. Jheng HF, Hayashi K, Matsumura Y, Kawada T, Seno S, Matsuda H, et al. Anti-inflammatory and antioxidative properties of isoflavones provide renal protective effects distinct from those of dietary soy proteins against diabetic nephropathy. Mol Nutr Food Res. (2020) 64:2000015. doi: 10.1002/MNFR.202000015
75. Ji X, Liu K, Li Q, Shen Q, Han F, Ye Q, et al. Mini-review of flavone isomers apigenin and genistein in prostate cancer treatment. Front Pharmacol. (2022) 13:269. doi: 10.3389/FPHAR.2022.851589/BIBTEX
76. Harlow BE, Flythe MD, Kagan IA, Goodman JP, Klotz JL, Aiken GE. Isoflavone supplementation, via red clover hay, alters the rumen microbial community and promotes weight gain of steers grazing mixed grass pastures. PLoS One. (2020) 15:e0229200. doi: 10.1371/JOURNAL.PONE.0229200
77. Yang Z, Tan X, Zhang Z, Han J, Qu S, Liu T, et al. Ononin: a candidate anti-parasitic drug isolated from Spatholobi caulis against infections of Dactylogyrus intermedius (Monogenea). Parasitol Int. (2022) 88:102535. doi: 10.1016/J.PARINT.2021.102535
78. Rostagno MA, Palma M, Barroso CG. Pressurized liquid extraction of isoflavones from soybeans. Anal Chim Acta. (2004) 522:169–77. doi: 10.1016/J.ACA.2004.05.078
Keywords: green chemistry, bioactive compounds, bioeconomy, biorefinery, NADES, soy by-products, pressurized liquid
Citation: Bragagnolo FS, Socas-Rodríguez B, Mendiola JA, Cifuentes A, Funari CS and Ibáñez E (2022) Pressurized natural deep eutectic solvents: An alternative approach to agro-soy by-products. Front. Nutr. 9:953169. doi: 10.3389/fnut.2022.953169
Received: 25 May 2022; Accepted: 11 August 2022;
Published: 08 September 2022.
Edited by:
De-qiang Li, Second Hospital of Hebei Medical University, ChinaReviewed by:
Ling Xu, Zhejiang Sci-Tech University, ChinaAleksandra Mišan, University of Novi Sad, Serbia
Copyright © 2022 Bragagnolo, Socas-Rodríguez, Mendiola, Cifuentes, Funari and Ibáñez. This is an open-access article distributed under the terms of the Creative Commons Attribution License (CC BY). The use, distribution or reproduction in other forums is permitted, provided the original author(s) and the copyright owner(s) are credited and that the original publication in this journal is cited, in accordance with accepted academic practice. No use, distribution or reproduction is permitted which does not comply with these terms.
*Correspondence: Elena Ibáñez, elena.ibanez@csic.es