- 1Animal Nutritional Genome and Germplasm Innovation Research Center, College of Animal Science and Technology, Hunan Agricultural University, Changsha, China
- 2Key Laboratory of Systems Health Science of Zhejiang Province, School of Life Sciences, Hangzhou Institute for Advanced Study, University of Chinese Academy of Sciences, Hangzhou, China
- 3Institute of Bast Fiber Crops, Chinese Academy of Agricultural Sciences, Changsha, China
Arbutin has been widely studied in whitening, anti-inflammatory, and antioxidant. However, the interaction between arbutin and intestinal microbes has been rarely studied. Thus, mice were treated with arbutin concentrations of 0, 0.1, 0.2, 0.4, and 1 mg/ml. We found that arbutin promoted gut development such as villus length, villus areas, and villus length/crypt depth (L/D). Total cholesterol (TC), high-density lipoprotein (HDL), and low-density lipoprotein (LDL) were significantly reduced by low concentrations of arbutin. Importantly, we analyzed the microbial composition in the control and 0.4 mg/ml arbutin group and found that the abundance of Lactobacillus intestinalis (L. intestinalis) was highest and enhanced in arbutin. Further, mice were fed with oral antibiotics and antibiotics + 0.4 mg/ml arbutin and then we transplanted fecal microbes from oral 0.4 mg/ml arbutin mice to mice pretreated with antibiotics. Our results showed that arbutin improves gut development, such as villus width, villus length, L/D, and villus areas. In addition, L. intestinalis monocolonization was carried out after a week of oral antibiotics and increased villus length, crypt depth, and villus areas. Finally, in vitro arbutin and L. intestinalis co-culture showed that arbutin promoted the growth and proliferation of L. intestinalis. Taken together, our results suggest that arbutin improves gut development and health of L. intestinalis. Future studies are needed to explore the function and mechanism of L. intestinalis affecting gut development.
Introduction
Arbutin is a natural phytochemical active substance, which is extracted from the bearberry leaves of Ericaceae and Saxifragaceae families (1, 2). It inhibits the activity of tyrosinase to reduce the production of melanin in the host (3), thereby lowering the deposition of melanin (4, 5). Meanwhile, arbutin is also associated with antioxidant (6, 7) and anti-inflammatory (8, 9). Additionally, arbutin has been widely studied for its role in protecting against nerve injury or other diseases caused by nerve injury (10, 11). However, arbutin regulation of gut development and host metabolism through gut microbiota has rarely been reported. The intestinal villi were directly contacted with nutrients and absorbed small molecules into the blood (12, 13), whereas the crypt was genetically regulated to shrink and invaginate (14), which was not conducive to nutrient absorption. Goblet cells secrete mucins and mucopolysaccharides to form the mucous system and were the site of colonization by gut microbes (15). Arbutin was rarely absorbed by the small intestine, but the majority was used by gut microbiota. Numerous studies reported that the role of phytochemicals was weakened by low bioavailability (16). Arbutin is a β-glucoside derived from hydroquinone (HQ) (1,4-dihydroxybenzene) (2, 3), its bioactivity and bioavailability were altered by gut microbes secreting glycoside hydrolase (17), and gut microbes have been identified as closely related to host metabolic disorders and diseases (18, 19).
Whether arbutin regulates gut development and host metabolism by altering gut microbes is unclear. Thus, we speculated that the interaction between arbutin and intestinal microbiome influences the pathological status and development of the gastrointestinal tract. Our results indicated that arbutin directly affects the composition of gut microbiota and development; further, Lactobacillus intestinalis (L. intestinalis) may serve as the potential mechanism.
Materials and methods
Bacterial strains
The L. intestinalis (ATCC49335) used in this study was purchased by Beijing Beina Chuanglian Biotechnology Research Institute (Beijing, China). Unless otherwise stated, bacterial strains were grown in MRS Broth (MRSB) (Qingdao Hope Bio-technology Corporation Ltd.) or on MRS Agar (MRSA) plates at 37°C.
Animal studies
Fifty female C57BL/6 mice (aged 6 weeks, 17 ± 0.5 g and aged 4 weeks, 14 ± 0.5 g) were randomly divided into 5 groups with arbutin solution of 0, 0.1, 0.2, 0.4, and 1 mg/ml (20) and fed maintenance diet lasted 3 weeks. We found that arbutin 0.4 mg/ml was most effective in improving intestinal index; thus, twenty mice were treated with antibiotics and antibiotics + 0.4 mg/ml arbutin for 3 weeks. Then, we transplanted fecal microbes from oral 0.4 mg/ml arbutin mice to mice pretreated with antibiotics for 1 week and then normal feeding for 2 weeks. Finally, twenty mice were pretreated with antibiotics for a week, 1.3 × 109 colony-forming unit (CFU)/ml L. intestinalis was intragastric to mice for 1 week, and then normal feeding for 2 weeks. All the animals were purchased from Hunan SJA Laboratory Animal Corporation Ltd. (Changsha, China) and used in this study. All the experimental animals were allowed free access to food and drinking water, and subjected to 12-h light-dark cycles, controlled temperature (23 ± 2°C), and humidity (45–60%) during the experiment. The basic diet was described in our previous study (21).
Hematoxylin and eosin staining
Intestinal HE staining was performed. The jejunal and ileal segments were fixed in 4% paraformaldehyde solution. The sections were first treated with xylene and ethanol solution for 15 and 5 min, respectively, then stained with hematoxylin for 5 min, rinsed with water for 5 min, then stained with eosin solution for 1–3 min, and then washed with ethanol and sealed. Finally, the villi, and crypt morphology were observed under a microscope.
Serum biochemical parameters
Serum samples were separated after centrifugation at 1,500 × g for 10 min at 4°C and 100 μl serum was transferred into another tube. Serum biochemical parameters were determined using an Automatic Biochemistry Analyzer (Cobas c 311, Roche).
Antibiotic treatment and fecal microflora transplantation
To eradicate commensal bacteria, filter-sterilized drinking water was supplemented with ampicillin (0.5 mg/ml, Meilunbio), gentamicin (0.5 mg/ml, Meilunbio), metronidazole (0.5 mg/ml, Meilunbio), neomycin (0.5 mg/ml, Meilunbio), and vancomycin (0.25 mg/ml, Meilunbio) for 1 week. Antibiotics were purchased from Dalian Meilun Biotechnology Corporation Ltd. (Dalian, China). Before fecal microbiota transplantation, the native gut microbiota in one group of C57 female mice (n = 10 biologically independent animals per group) was deleted by administering drinking water containing a cocktail of antibiotics for 1 week. Fecal samples of ∼200 mg were then collected from arbutin (0.4 mg/ml)-fed mice and resuspended in 2.0 ml normal saline. Fecal samples were mixed and centrifuged at 1,000 × g, and the microbiota supernatants were transplanted into the microbiota-depleted mice by gavaging with 0.2 ml per mice for 1 week. After transplantation, two groups of mice were administrated with a standard diet and regular water.
Gut microbiota profiling
Total genome DNA from ileal chyme and mucosa was extracted using cetyltrimethylammonium bromide (CTAB) method. DNA concentration and purity were monitored on 1% agarose gels. According to the concentration, DNA was diluted to 1 ng/μl using sterile water. 16S rDNA genes of distinct regions (16S V3-V4) were amplified using a specific primer (515F-806R) with the barcode. All the PCR reactions were carried out with 15 μl of the Phusion® High-Fidelity PCR Master Mix (New England Biolabs), 2 μM of forward and reverse primers, and about 10 ng of template DNA. Sequencing libraries were generated using the TruSeq® DNA PCR-Free Sample Preparation Kit (Illumina, United States) following the manufacturer’s recommendations and index codes were added according to our previous study (22). Microbial communities were investigated by iTag sequencing of 16S rDNA genes (23, 24).
Statistical analysis
All the statistical analyses were performed using the one-way ANOVA and t-test analysis in SPSS version 20.0 software (SPSS Incorporation, Chicago, IL, United States). The data are expressed as the means ± SEM). P < 0.05 was considered statistically significant. All the figures in this study were drawn using GraphPad Prism version 8.0.
Results
Arbutin administration improves gut development
Final body weight was not obviously changed (Figure 1A), but the relative weight and weight/length of the intestine were significantly increased by arbutin administration at 0.2 and 0.4 mg/ml (P < 0.05) (Figures 1B,D), and arbutin did not alter the intestinal length, villus width and crypt depth (Figures 1C,F,H). Thus, we continued to investigate the intestinal pathology section; the results showed that the villus length was increased by 0.4 and 1.0 mg/ml arbutin, villus area was enhanced by 0.2 and 0.4 mg/ml arbutin, and villus length/crypt depth (L/D) was higher at 0.4 mg/ml arbutin (P < 0.05) (Figures 1E,G,I,J).
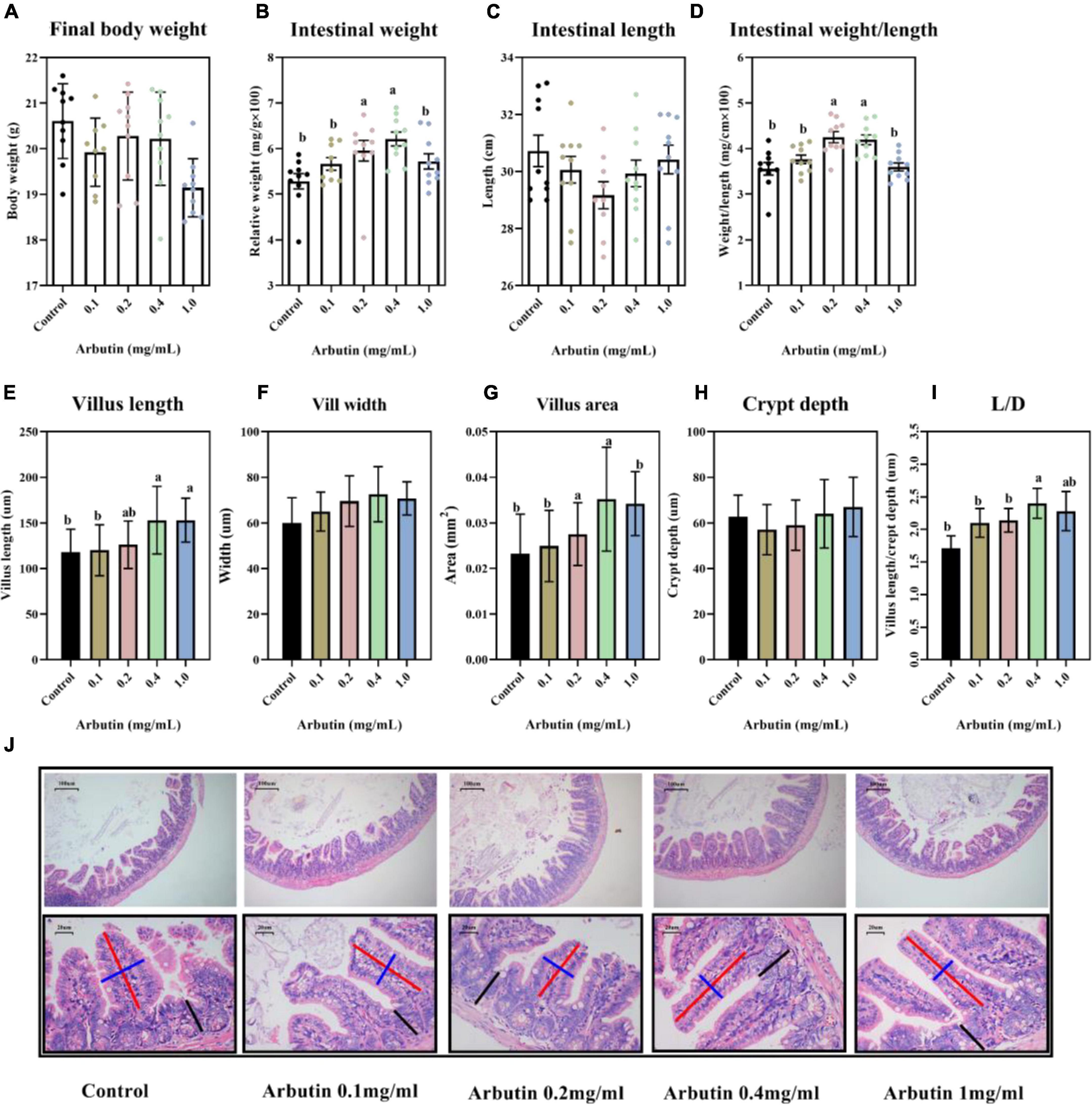
Figure 1. Oral arbutin improves gut development. Final body weight (A), relative intestinal weight (B), intestinal length (C), intestinal weight/length (D), villus length (E), villus width (F), villus areas (G), crypt depth (H), L/D (I), and HE staining of jejunum and ileum (J). Values are presented as the means ± SEMs. Differences were assessed by one-way ANOVA and denoted as follows: a and b indicate significant differences in each group.
Effects of arbutin on serum biochemical parameters
To further understand the role of arbutin, lipid parameters in serum were determined (Figures 2A–F). Arbutin at 0.4 mg/ml significantly enhanced the content of serum glucose (Glu) (P < 0.05) (Figure 2A). Nevertheless, arbutin at 0.2 mg/ml lowered the content of total cholesterol (TC) and high-density lipoprotein (HDL) (P < 0.05) (Figures 2C,D), and low-density lipoprotein (LDL) was lowered at 0.1 and 0.2 mg/ml (P < 0.05) (Figure 2E). These results suggested that arbutin can improve intestinal development and serum lipid parameters.
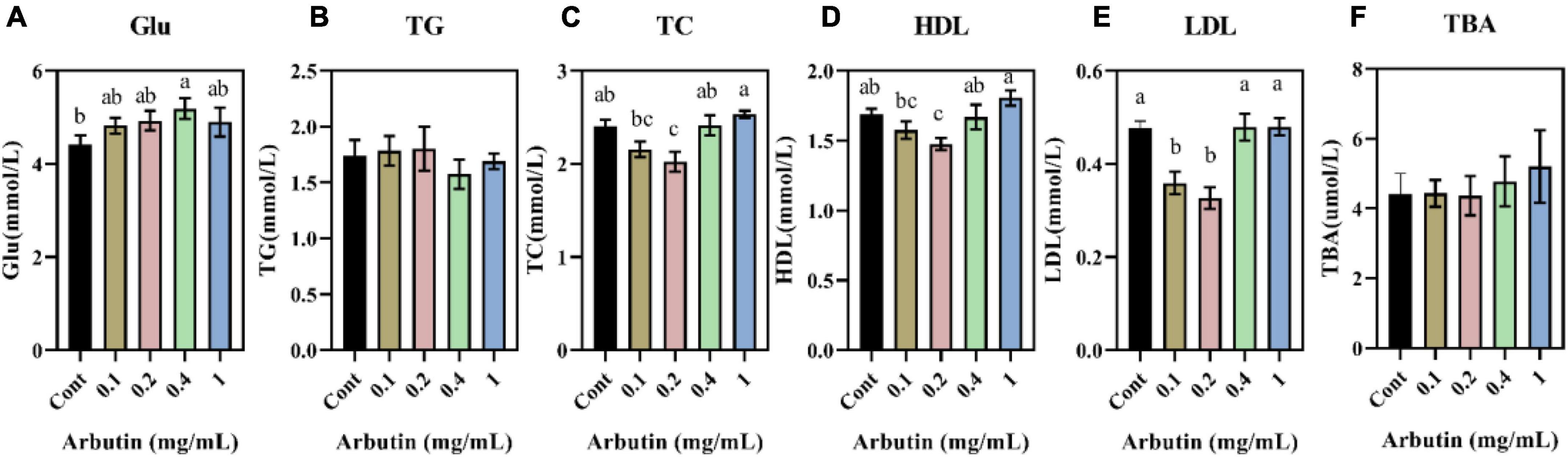
Figure 2. Effects of oral arbutin on serum lipids. Glucose (A), total triglycerides (B), total cholesterol (C), high-density lipoprotein (D), low-density lipoprotein (E), and total bile acid (F) (n = 10). Values are presented as the means ± SEMs. Differences were assessed by one-way ANOVA and denoted as follows: a and b indicate significant differences in each group.
Arbutin alters the composition of gut microbiota
To investigate the effects of arbutin on gut microbes, we determined the microbiome by 16S rDNA sequencing at 0.4 mg/ml (Figures 3, 4). Venn diagram showed that 597 and 111 different operational taxonomic units (OTUs) were found in the control and arbutin groups and contained the same 540 OTUs (Figure 3A), rarefaction curve indicated that the sample capacity and sample depth were reasonable (Figure 4B). Arbutin significantly decreased the α-diversity index [observed species, Shannon index, phylogenetic diversity (PD), Simpson index, Chao1, and abundance-based coverage estimator (ACE)] (P < 0.05) (Figures 3B–D, 4A,C–E). Meanwhile, the β-diversity index was reduced (P < 0.05) (Figure 3E), and principal component analysis showed that there were different zones of intestinal microflora between the control group and arbutin (Figure 3F). At the phylum level, the relative abundance of Actinobacteria and Proteobacteria was clearly lowered by arbutin (P < 0.05) (Figure 3G). At the species level, 0.4 mg/ml arbutin markedly increased the abundance of Lactobacillus intestinalis (P < 0.05) (Figures 3H, 4F), while the abundance of Bifidobacterium animalis, Bacillus velezensis, Lachnospiraceae bacterium_M18-1, Eubacterium sp_14-2, Helicobacter ganmani, Lachnospiraceae bacterium_10-1, Lachnospiraceae bacterium_615, Planoglabratella opercularis, Pseudoflavonifractor sp_Marseille-P3106, Clostridium leptum, Clostridium sp_ASF356, Dubosiella newyorkensis, Burkholderiales bacterium_YL145, Desulfovibrio sp_ABHU2SB, Firmicutes_bacterium CAG_194_44_15, Clostridium sp_Culture-27, and Ruminiclostridium sp_KB18 was lowered compared to control (P < 0.05) (Figures 3H, 4F).
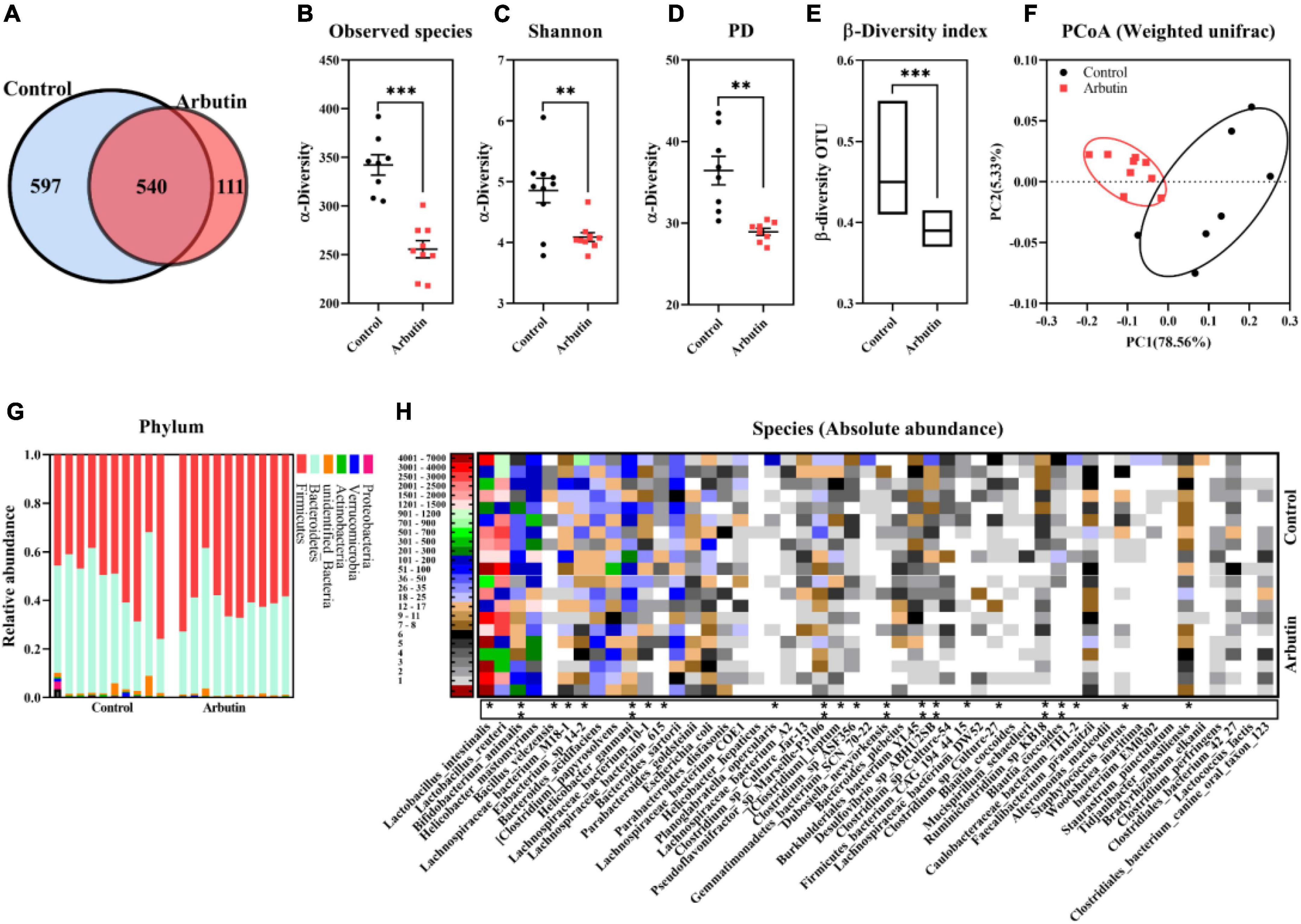
Figure 3. Arbutin alters the gut microbiota. Venn diagram (A), observed species (B), Shannon index (C), PD_whole_tree (D), β-diversity index (E), principal component analysis (F), phylum (G), and species (H) were analyzed at 0.4 mg/ml. Differences were assessed by t-test and denoted as follows: *P < 0.05, ** P < 0.01, ***P < 0.001.
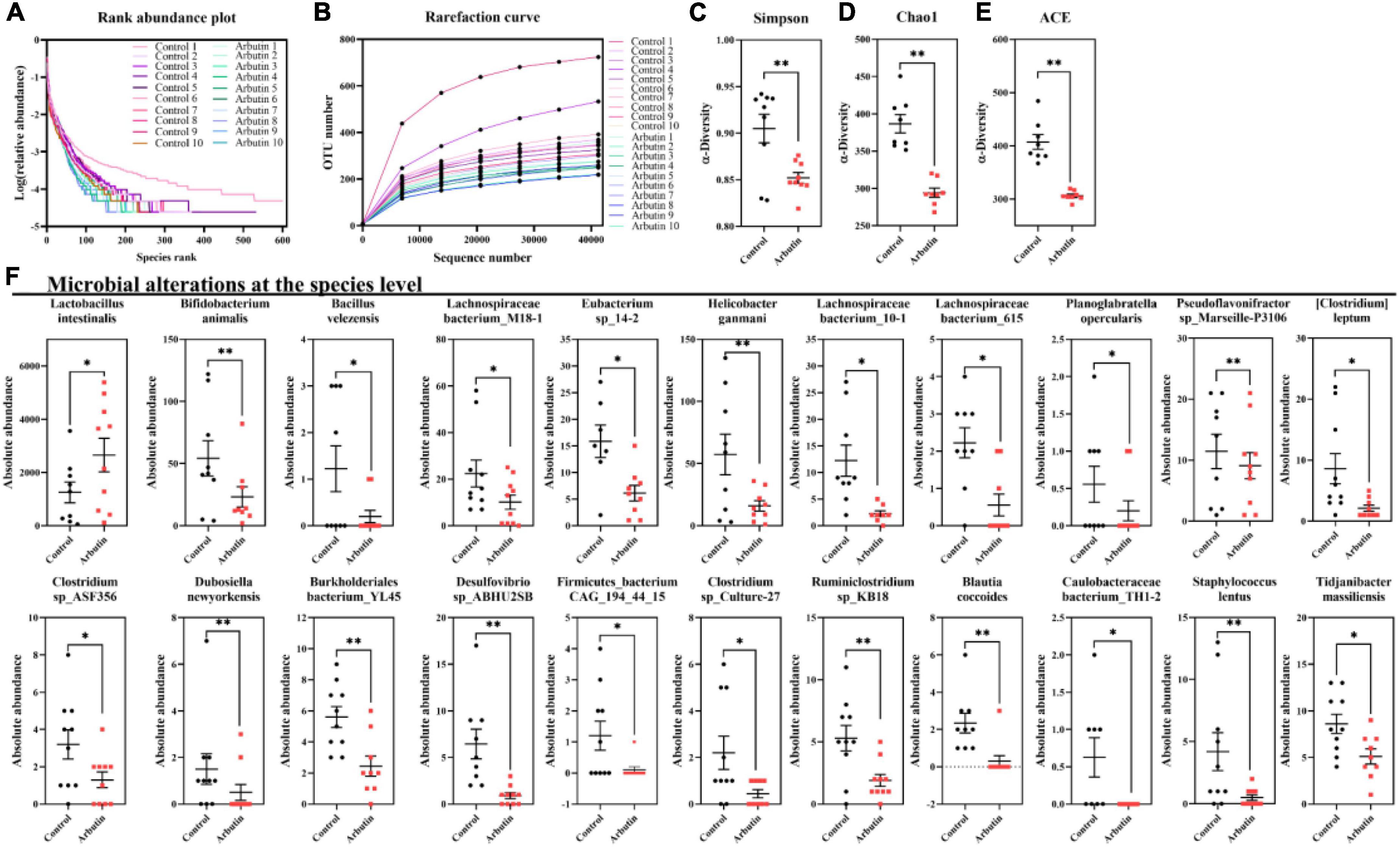
Figure 4. Arbutin alters the gut microbiota. Rank abundance plot (A), rarefaction curve (B), Simpson index (C), Chao1 (D), ACE (E), and species (F) were analyzed at 0.4 mg/ml. Differences were assessed by t-test and denoted as follows: *P < 0.05, **P < 0.01.
Arbutin improves gut development with an antibiotics cocktail and fecal microflora transplantation
The intestinal microbiota has been shown to regulate intestinal development (25) and host metabolism (18). To further determine the role of intestinal microbiota, 4 weeks mice were given an antibiotics cocktail for 1 week with oral arbutin solution (0.4 mg/ml). Predictably, arbutin significantly enriched the villi width compared to the antibiotics group in the jejunum (P < 0.05) (Figures 5A,C), but villus length, villus area, crypt depth, L/D were not changed (Figure 5B,D–F), and there was a tendency to enhance the ileal villi index (Figures 5G–L). Then, we further collected feces from mice administered with arbutin 0.4 mg/ml and transplanted them to mice with an antibiotics cocktail. Fecal microflora transplantation significantly improved intestinal pathologies, such as jejunal villus length (Figures 6A,B), jejunal villus length/villus width (L/D) (Figures 6A,F), and ieal villus areas (Figure 6J). But jejunal villus width, jejunal villus area, jejunal crypt depth, ileal villus legth, ileal villus width, ileal crypt depth and ileal L/D were uninfluential (Figures 6C–E,H,I,K,L). In summary, gut microbes contributed improving intestinal development.
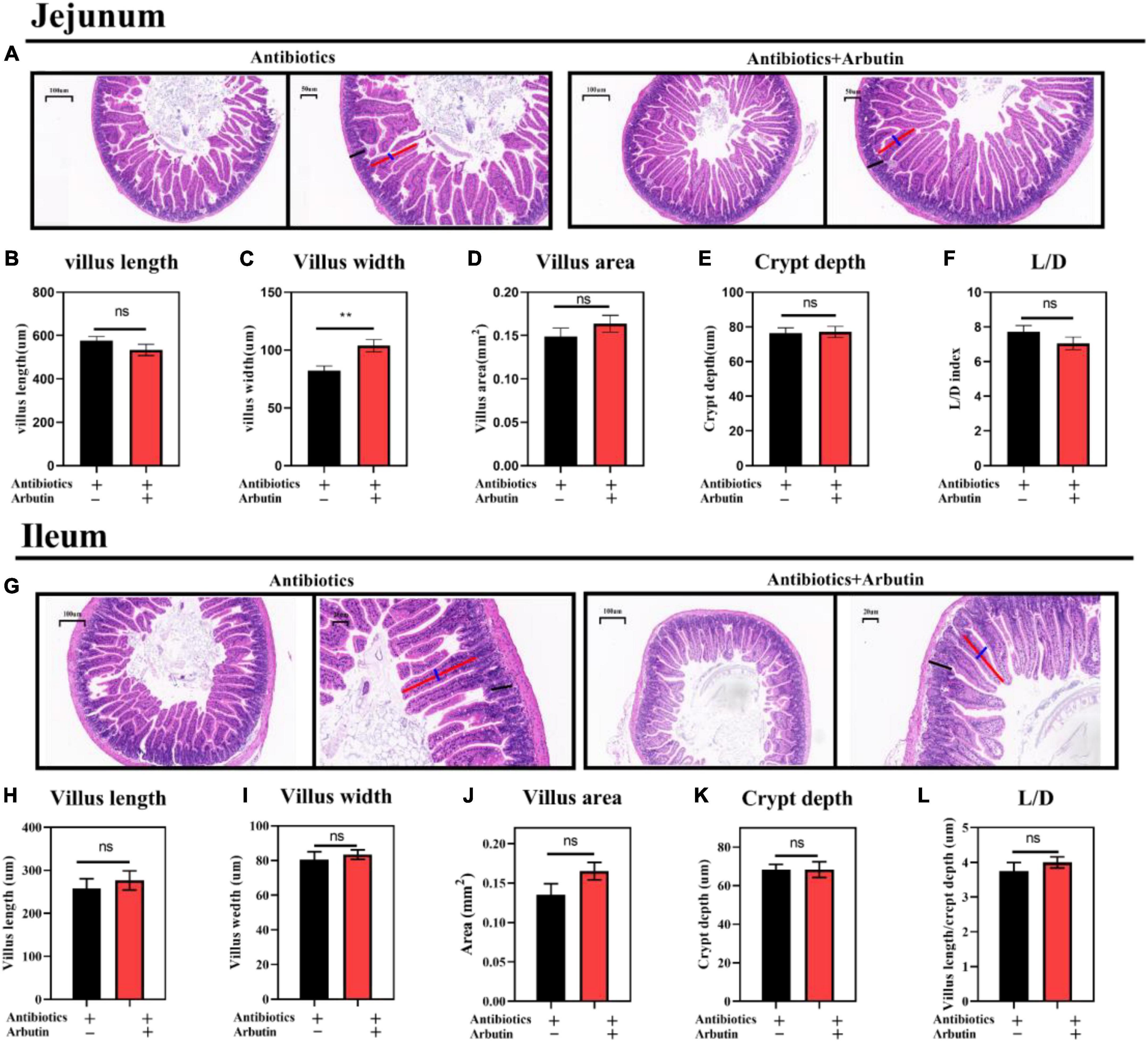
Figure 5. Arbutin administration improved jejunal and ileal gut development after oral cocktails. HE staining of the jejunum (A), villus length in the jejunum (B), villus width in the jejunum (C), villus areas in the jejunum (D), crypt depth in the jejunum (E), L/D in the jejunum (F), HE staining of the ileum (G), villus length in the ileum (H), villus width in the ileum (I), villus areas in the ileum (J), crypt depth in the ileum (K), and L/D in the ileum (L) (n = 10). Differences were assessed by t-test and denoted as follows: **P < 0.01.
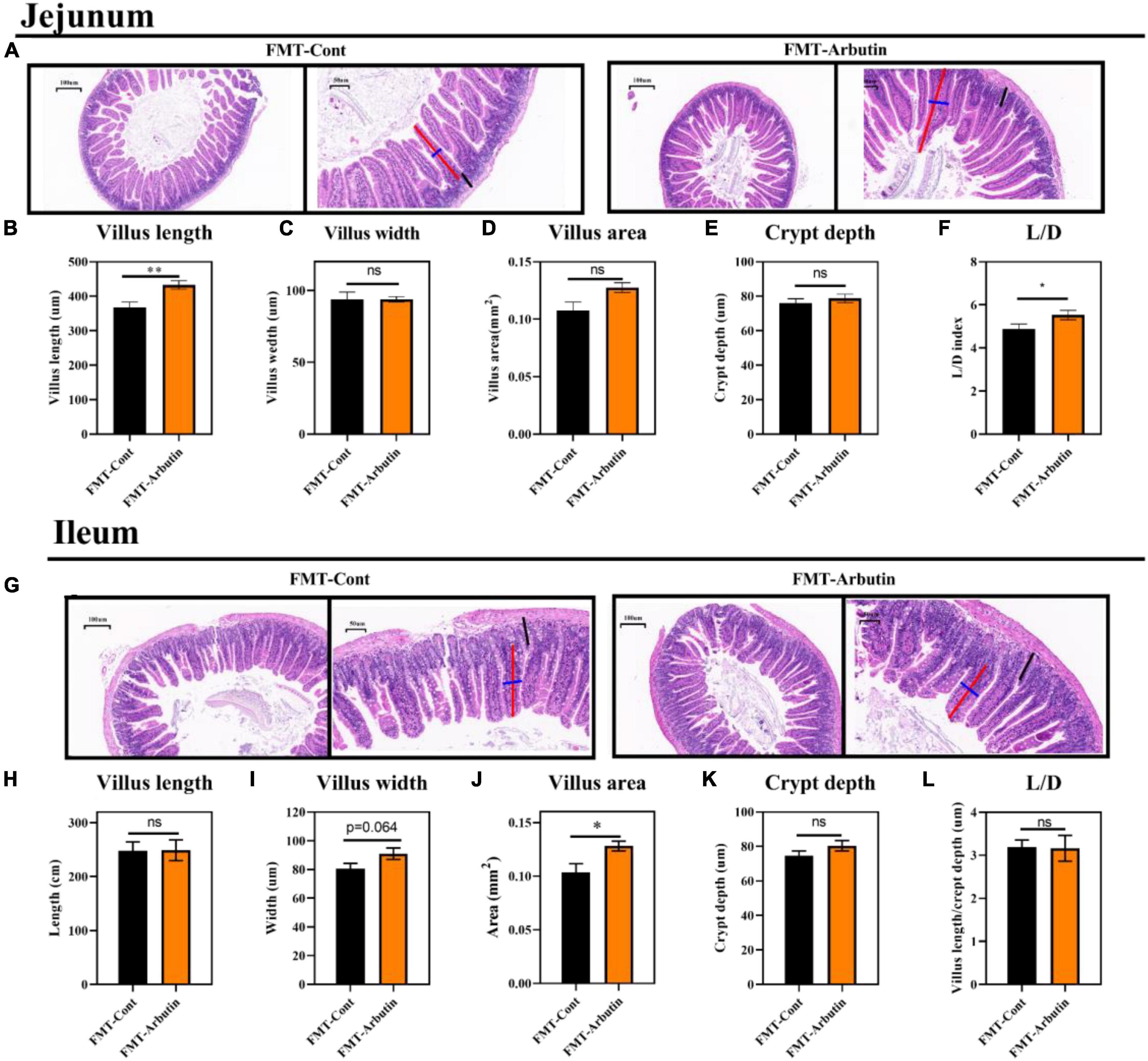
Figure 6. Fecal microflora transplantation improved gut development in mice. HE staining of the jejunum (A), villus length in the jejunum (B), villus width in the jejunum (C), villus areas in the jejunum (D), crypt depth in the jejunum (E), L/D in the jejunum (F), HE staining of the ileum (G), villus length in the ileum (H), villus width in the ileum (I), villus areas in the ileum (J), crypt depth in the ileum (K), and L/D in the ileum (L) (n = 10). Differences were assessed by t-test and denoted as follows: *P < 0.05, **P < 0.01.
Lactobacillus intestinalis colonization reduces gut damage after an antibiotics cocktail
We have found that the abundance of L. intestinalis (Lin) was markedly enhanced by arbutin and was the most abundant bacterium in the gut (Figures 3H, 4F). L. intestinalis was often found in the gut of the host, which was treated for various diseases (26–28) and metabolic disorders (29, 30), but the effect of L. intestinalis on gut development and host lipid metabolism was unclear. Thus, we investigated the role of Lin on intestinal pathology and used Lin monocolonization (31) with an antibiotics cocktail for 1 week. Interestingly, after an antibiotics cocktail for 1 week, Lin monocolonization clearly increased the villus length, crypt depth, and villus areas (Figures 7A–C,E), and there was a tendency to elevate the number of goblet cells (Figures 7A,G). Whereas villus width and L/D were not changed by L. intestinalis (Figures 7D,F).
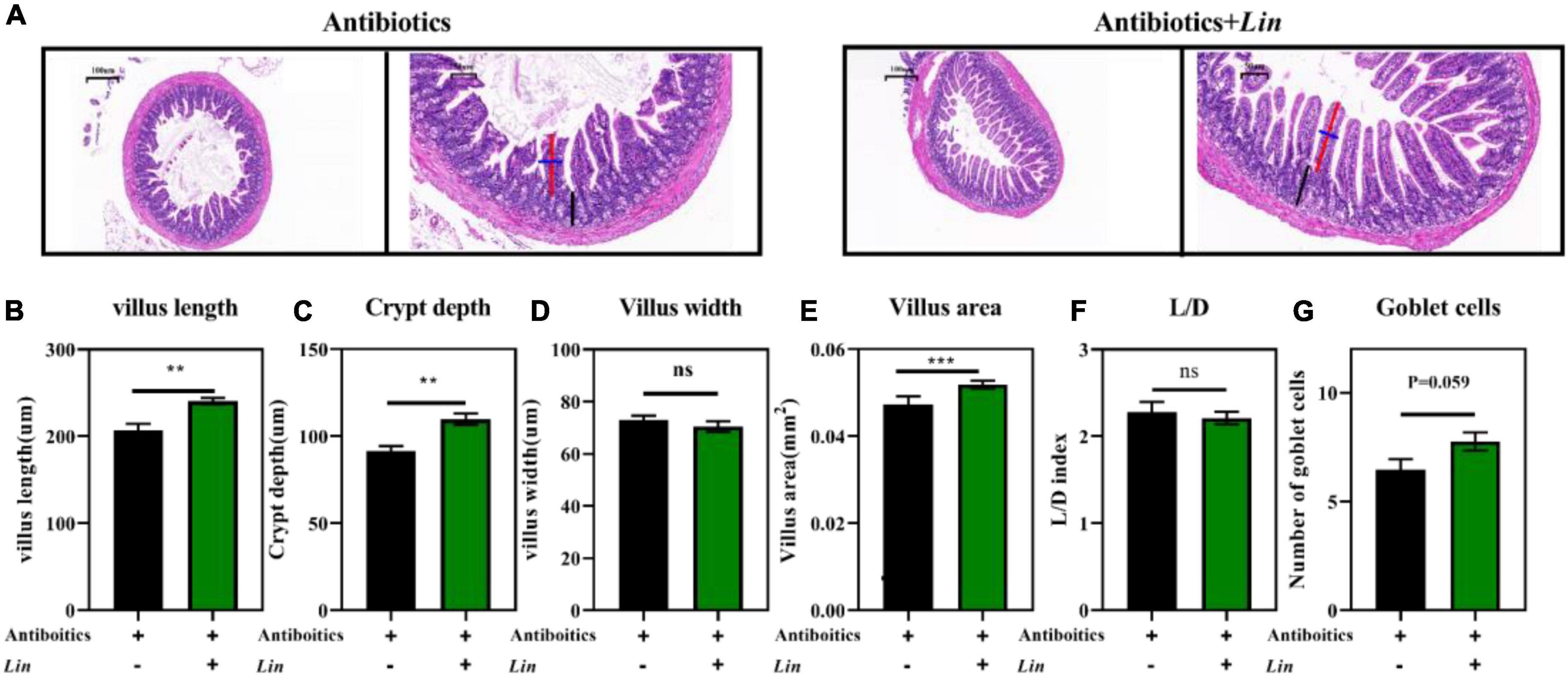
Figure 7. Lactobacillus intestinalis colonization improved intestinal development in mice. HE staining the ileum (A), villus length in the ileum (B), villus width in the ileum (C), villus areas in the ileum (D), crypt depth in the ileum (E), L/D in the ileum (F), and the number of goblet cells (G) (n = 10). Differences were assessed by t-test and denoted as follows: **P < 0.01, ***P < 0.001.
Arbutin promotes the growth of Lactobacillus intestinalis in vitro
In order to verify the previous results, we co-cultured arbutin and L. intestinalis to investigate the growth of L. intestinalis in vitro. The results showed that arbutin significantly promotes the growth of L. intestinalis (P < 0.05) (Figure 8).
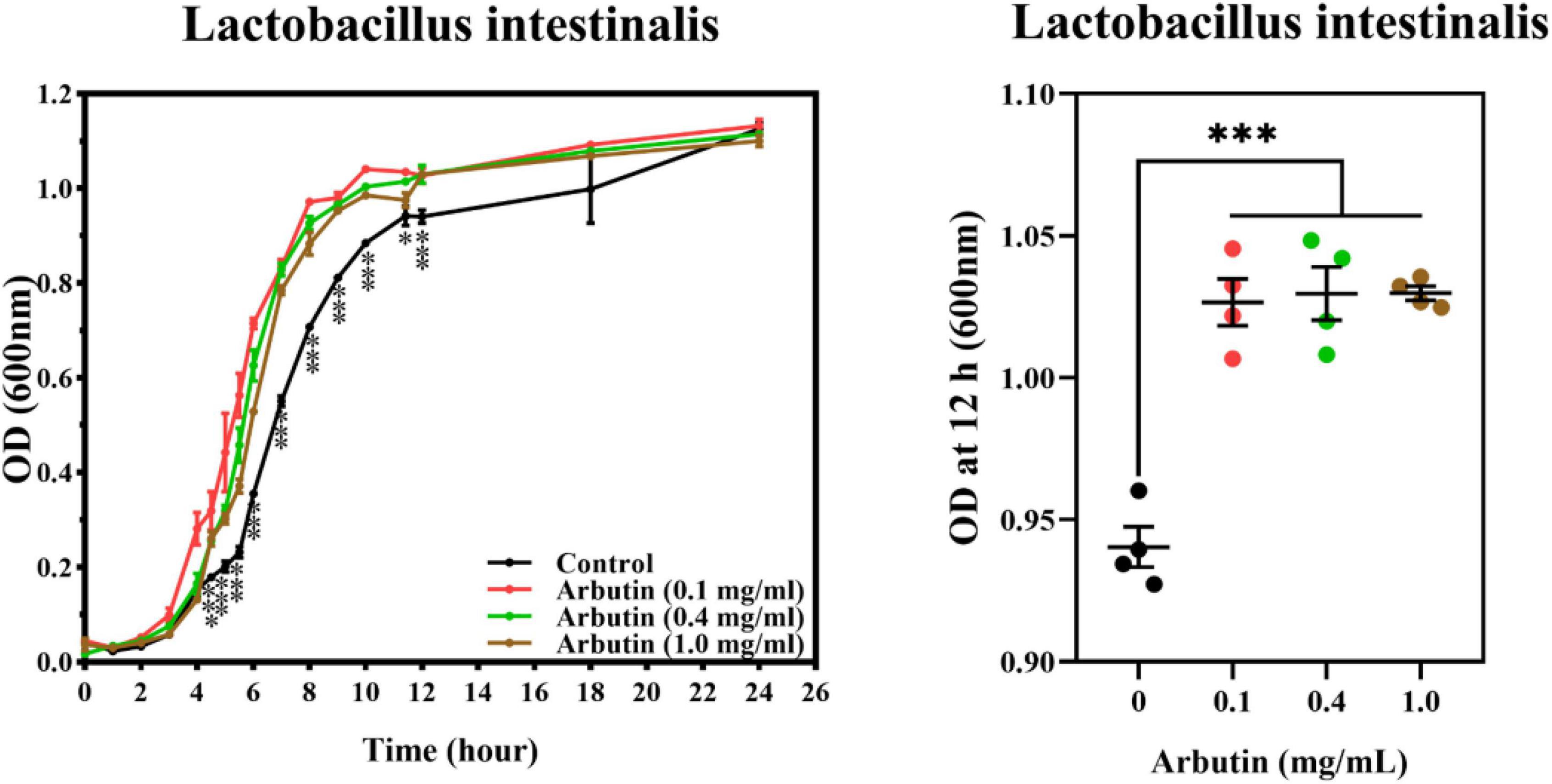
Figure 8. Arbutin promotes the growth of Lactobacillus intestinalis in vitro. Arbutin and L. intestinalis were co-cultured and determined the OD value at 0–24 h. Differences were assessed by t-test and denoted as follows: *P < 0.05, ***P < 0.001.
Discussion
In recent years, arbutin has often been extensively studied that it inhibited tyrosinase activity to reduce melanin deposition in the cosmetic industry (32). Meanwhile, arbutin is often used to treat various diseases, such as types of cancers, central nervous system disorders, osteoporosis, diabetes, and so on (20). Arbutin, as a phytochemical active substance, is low bioavailability in the gut, and incompletely played a beneficial role (33). Further, they are degraded by microbes to increase their biological activity (34). However, the potential of arbutin has rarely been reported about promoting intestinal health. Thus, we explored the effects of arbutin on gut health in the common condition, oral antibiotic cocktails, fecal microflora transplantation, and Lin monocolonization.
We investigated the effects of different concentrations of arbutin on gut health and serum lipids in mice in normal conditions. We found that low concentrations of arbutin reduced serum lipids, whereas reversed at high concentrations. Previous studies have shown that arbutin significantly reduced adipocyte differentiation and promoted fatty acid uptake in 3T3-L1 adipocytes (35). The polyjuice decoction containing arbutin decreased the total cholesterol, triglyceride, VLDL, and LDL in diabetic rats (36). Importantly, we found that arbutin plays an important role in promoting intestinal development. In this trial, 0.4 and 1.0 mg/kg arbutin markedly enhanced the villus length, villus areas, and L/D compared to control. Villus index was highly associated with nutrient absorption and gut health (37); thus, the increased villus length, villus areas, and L/D indicated a positive role of arbutin in gut nutrient absorption. The gut microbiota may be an important reason for this result. Arbutin, as a natural phytochemical, is a β-glucoside derived from hydroquinone (2, 3) whose bioactivity and bioavailability can be modified by glycoside hydrolase activity of gut microbiota through the release of acylglycines (38). Microorganisms are associated with the absorption and metabolism of arbutin, a novel Janthinobacterium strain (SNU WT3), isolated from the kidney of rainbow trout showed that different biochemical details such as arbutin compared to its close relatives identified (39). Further, Bifidobacterium was proved to degrade arbutin (containing glycosides) to elevate bioavailability by secreting β-glucosidase (38). We found that L. intestinalis was significantly increased by arbutin, which played an important role in gut health and metabolic disorders (26, 29, 40). However, the abundance of another 21 species of bacteria (such as Bifidobacterium animalis, Bacillus velezensis, Lachnospiraceae bacterium_M18-1, Eubacterium sp_14-2, and Helicobacter ganmani) was significantly reduced. Interestingly, arbutin was reported to reduce colitis symptoms and inhibit lipopolysaccharide-induced inflammation (41), and there were significant negative correlations between arbutin contents and the enriched gut microbiota (e.g., Eubacterium and Ruminococcus) (42), suggesting that there was bactericidal ability about arbutin.
Gut damage is often associated with drugs, environmental stress, and lifestyle (43). Especially, antibiotics are considered only beneficial, but also potentially harmful drugs, as their abuse appears to play a role in the pathogenesis of several disorders associated with microbiota impairment (44). In this trial, we demonstrated the beneficial effects of arbutin in improving gut health with antibiotics cocktail and fecal microflora transplantation. The result was attributed to arbutin administration altering the gut microflora, such as L. intestinalis. Fecal microflora transplantation is a common technique for the treatment of host metabolic disorders and diseases (45, 46). The gut microbiota development of cesarean section infants was rapidly restored by orally derived fecal microflora transplantation (47). Fecal microflora transplantation played beneficial effects on gastrointestinal transport and intestinal barrier dysfunction (48), which were related to intestinal permeability and pathology (49), such as villus length, villus areas, and L/D. Furthermore, the monocolonization technique improves the gut microbiota structure and metabolic process of the host (50, 51) and is also one of the measures to investigate bacterial function. For example, probiotic colonization improved intestinal barrier function and intestinal health, newly identified health-associated bacteria, such as Faecalibacterium prausnitzii, Akkermansia muciniphila, Ruminococcus bromii, and Roseburia species (52, 53). Our results showed that L. intestinalis monocolonization reduced intestinal damage after an antibiotics cocktail, such as villus length, crypt depth, villus areas, and the number of goblet cells.
To prove the effect of arbutin on L. intestinalis growth, we used the co-culture method of arbutin and L. intestinalis. Previous studies have found that Bifidobacterium degraded β-glucosidase to enhance the activity of glycoside by secreting β-glucosidase (38). Liu et al. identified a glycoside hydrolase, which is very important for the growth of type I rhamnogalacturonan acid by commensal bacteroides (54). We found that arbutin significantly promoted the proliferation of L. intestinalis, suggesting the potential of arbutin on L. intestinalis proliferation and utilizing arbutin to increase biological activity.
Conclusion
Arbutin, as a phytochemical, has been widely studied in whitening, anti-inflammatory, and antioxidant, while the interaction between arbutin and intestinal microbes has been rarely studied. In this trial, we focused on the effects of arbutin on intestinal development and microbes. Predictably, arbutin played a positive role in the gut, such as improving the pathological state of the jejunum and ileum and altering the intestinal microbial structure. In addition, we demonstrated the beneficial effects of arbutin on intestinal development through fecal microflora transplantation and L. intestinalis monocolonization by antibiotic cocktail therapy. However, the specific mechanisms of L. intestinalis in intestinal development need to be further explored.
Data availability statement
The datasets presented in this study can be found in online repositories. The names of the repository/repositories and accession number(s) can be found in the article/supplementary material. The data presented in the study are deposited in the NCBI (https://dataview.ncbi.nlm.nih.gov/object/PRJNA839245) repository, accession number: PRJNA839245.
Ethics statement
The animal model and experimental procedures used in this experiment were approved by the Hunan Agricultural University Institutional Animal Care and Use Committee (202005).
Author contributions
JM was the primary investigator in this study. SC participated in the animal experiments. YL performed the statistical data analysis. XW participated in the sample analysis. ZS examined the manuscript. All authors contributed to the article and approved the submitted version.
Funding
This study was supported by the National Natural Science Foundation of China (Grant No: 31902171).
Acknowledgments
We thank the College of Animal Science and Technology, Hunan Agricultural University, Animal Nutrition Genome, and Germplasm Innovation Research Center for their support.
Conflict of interest
The authors declare that the research was conducted in the absence of any commercial or financial relationships that could be construed as a potential conflict of interest.
Publisher’s note
All claims expressed in this article are solely those of the authors and do not necessarily represent those of their affiliated organizations, or those of the publisher, the editors and the reviewers. Any product that may be evaluated in this article, or claim that may be made by its manufacturer, is not guaranteed or endorsed by the publisher.
References
1. Shang Y, Wei W, Zhang P, Ye BC. Engineering Yarrowia lipolytica for enhanced production of arbutin. J Agric Food Chem. (2020) 68:1364–72. doi: 10.1021/acs.jafc.9b07151
2. Zhou H, Zhao J, Li A, Reetz MT. Chemical and biocatalytic routes to arbutin (dagger). Molecules. (2019) 24:3303. doi: 10.3390/molecules24183303
3. Zhu X, Tian Y, Zhang W, Zhang T, Guang C, Mu W. Recent progress on biological production of alpha-arbutin. Appl Microbiol Biotechnol. (2018) 102:8145–52. doi: 10.1007/s00253-018-9241-9
4. Park JJ, Hwang SJ, Kang YS, Jung J, Park S, Hong JE, et al. Synthesis of arbutin-gold nanoparticle complexes and their enhanced performance for whitening. Arch Pharm Res. (2019) 42:977–89. doi: 10.1007/s12272-019-01164-7
5. Zaid AN, Al Ramahi R. Depigmentation and anti-aging treatment by natural molecules. Curr Pharm Des. (2019) 25:2292–312. doi: 10.2174/1381612825666190703153730
6. Bonifacio MA, Cerqueni G, Cometa S, Licini C, Sabbatini L, Mattioli-Belmonte M, et al. Insights into arbutin effects on bone cells: Towards the development of antioxidant titanium implants. Antioxidants. (2020) 9:579. doi: 10.3390/antiox9070579
7. Boo YC. Arbutin as a skin depigmenting agent with antimelanogenic and antioxidant properties. Antioxidants. (2021) 10:1129. doi: 10.3390/antiox10071129
8. Hazman O, Sariova A, Bozkurt MF, Cigerci IH. The anticarcinogen activity of beta-arbutin on MCF-7 cells: Stimulation of apoptosis through estrogen receptor-alpha signal pathway, inflammation and genotoxicity. Mol Cell Biochem. (2021) 476:349–60. doi: 10.1007/s11010-020-03911-7
9. Ahmadian SR, Ghasemi-Kasman M, Pouramir M, Sadeghi F. Arbutin attenuates cognitive impairment and inflammatory response in pentylenetetrazol-induced kindling model of epilepsy. Neuropharmacology. (2019) 146:117–27. doi: 10.1016/j.neuropharm.2018.11.038
10. Ebrahim-Tabar F, Nazari A, Pouramir M, Ashrafpour M, Pourabdolhossein F. Arbutin improves functional recovery and attenuates glial activation in lysolecethin-induced demyelination model in rat optic chiasm. Mol Neurobiol. (2020) 57:3228–42. doi: 10.1007/s12035-020-01962-x
11. Ding Y, Kong D, Zhou T, Yang ND, Xin C, Xu J, et al. alpha-arbutin protects against Parkinson’s disease-associated mitochondrial dysfunction in vitro and in vivo. Neuromolecular Med. (2020) 22:56–67. doi: 10.1007/s12017-019-08562-6
12. Bernier-Latmani J, Mauri C, Marcone R, Renevey F, Durot S, He L, et al. ADAMTS18(+) villus tip telocytes maintain a polarized VEGFA signaling domain and fenestrations in nutrient-absorbing intestinal blood vessels. Nat Commun. (2022) 13:3983. doi: 10.1038/s41467-022-31571-2
13. Short K, Derrickson EM. Compensatory changes in villus morphology of lactating Mus musculus in response to insufficient dietary protein. J Exp Biol. (2020) 223:jeb210823. doi: 10.1242/jeb.210823
14. Sumigray KD, Terwilliger M, Lechler T. Morphogenesis and compartmentalization of the intestinal crypt. Dev cell. (2018) 45:183.e–97.e. doi: 10.1016/j.devcel.2018.03.024
15. Cai R, Cheng C, Chen J, Xu X, Ding C, Gu B. Interactions of commensal and pathogenic microorganisms with the mucus layer in the colon. Gut Microbes. (2020) 11:680–90. doi: 10.1080/19490976.2020.1735606
16. Martel J, Ojcius DM, Ko YF, Young JD. Phytochemicals as prebiotics and biological stress inducers. Trends Biochem Sci. (2020) 45:462–71. doi: 10.1016/j.tibs.2020.02.008
17. Dey P. Gut microbiota in phytopharmacology: A comprehensive overview of concepts, reciprocal interactions, biotransformations and mode of actions. Pharmacol Res. (2019) 147:104367. doi: 10.1016/j.phrs.2019.104367
18. Fan Y, Pedersen O. Gut microbiota in human metabolic health and disease. Nat Rev Microbiol. (2021) 19:55–71. doi: 10.1038/s41579-020-0433-9
19. Morais LH, Schreiber HLt, Mazmanian SK. The gut microbiota-brain axis in behaviour and brain disorders. Nat Rev Microbiol. (2021) 19:241–55. doi: 10.1038/s41579-020-00460-0
20. Saeedi M, Khezri K, Seyed Zakaryaei A, Mohammadamini H. A comprehensive review of the therapeutic potential of alpha-arbutin. Phytother Res. (2021) 35:4136–54. doi: 10.1002/ptr.7076
21. Yin J, Li Y, Han H, Chen S, Gao J, Liu G, et al. Melatonin reprogramming of gut microbiota improves lipid dysmetabolism in high-fat diet-fed mice. J Pineal Res. (2018) 65:e12524. doi: 10.1111/jpi.12524
22. Yin J, Li Y, Han H, Ma J, Liu G, Wu X, et al. Administration of Exogenous Melatonin Improves the Diurnal Rhythms of the Gut Microbiota in Mice Fed a High-Fat Diet. mSystems. (2020) 5:e2–20. doi: 10.1128/mSystems.00002-20
23. Tsou AM, Olesen SW, Alm EJ, Snapper SB. 16S rRNA sequencing analysis: The devil is in the details. Gut Microbes. (2020) 11:1139–42. doi: 10.1080/19490976.2020.1747336
24. Church DL, Cerutti L, Gurtler A, Griener T, Zelazny A, Emler S. Performance and application of 16S rRNA gene cycle sequencing for routine identification of bacteria in the clinical microbiology laboratory. Clin Microbiol Rev. (2020) 33:e53–19. doi: 10.1128/CMR.00053-19
25. Larabi A, Barnich N, Nguyen HTT. New insights into the interplay between autophagy, gut microbiota and inflammatory responses in IBD. Autophagy. (2020) 16:38–51. doi: 10.1080/15548627.2019.1635384
26. Wang S, Ishima T, Zhang J, Qu Y, Chang L, Pu Y, et al. Ingestion of Lactobacillus intestinalis and Lactobacillus reuteri causes depression- and anhedonia-like phenotypes in antibiotic-treated mice via the vagus nerve. J Neuroinflammation. (2020) 17:241. doi: 10.1186/s12974-020-01916-z
27. Lim EY, Song EJ, Kim JG, Jung SY, Lee SY, Shin HS, et al. Lactobacillus intestinalis YT2 restores the gut microbiota and improves menopausal symptoms in ovariectomized rats. Benef Microbes. (2021) 12:503–16. doi: 10.3920/BM2020.0217
28. Wang N, Wu T, Du D, Mei J, Luo H, Liu Z, et al. Transcriptome and gut microbiota profiling revealed the protective effect of tibetan tea on ulcerative colitis in mice. Front Microbiol. (2021) 12:748594. doi: 10.3389/fmicb.2021.748594
29. Wang Y, Ouyang M, Gao X, Wang S, Fu C, Zeng J, et al. Phocea, pseudoflavonifractor and Lactobacillus intestinalis: Three potential biomarkers of gut microbiota that affect progression and complications of obesity-induced type 2 diabetes mellitus. Diabetes Metab Syndr Obes. (2020) 13:835–50. doi: 10.2147/DMSO.S240728
30. Lu X, Jing Y, Li Y, Zhang N, Zhang W, Cao Y. The differential modulatory effects of Eurotium cristatum on the gut microbiota of obese dogs and mice are associated with improvements in metabolic disturbances. Food Funct. (2021) 12:12812–25. doi: 10.1039/d1fo02886c
31. Ang QY, Alexander M, Newman JC, Tian Y, Cai J, Upadhyay V, et al. Ketogenic diets alter the gut microbiome resulting in decreased intestinal Th17 cells. Cell. (2020) 181:1263.e–75.e. doi: 10.1016/j.cell.2020.04.027
32. Jin YH, Jeon AR, Mah JH. Tyrosinase inhibitory activity of soybeans fermented with Bacillus subtilis capable of producing a phenolic glycoside, arbutin. Antioxidants. (2020) 9:1301. doi: 10.3390/antiox9121301
33. Wan MLY, Co VA, El-Nezami H. Dietary polyphenol impact on gut health and microbiota. Crit Rev Food Sci Nutr. (2021) 61:690–711. doi: 10.1080/10408398.2020.1744512
34. Luca SV, Macovei I, Bujor A, Miron A, Skalicka-Wozniak K, Aprotosoaie AC, et al. Bioactivity of dietary polyphenols: The role of metabolites. Crit Rev Food Sci Nutr. (2020) 60:626–59. doi: 10.1080/10408398.2018.1546669
35. Bedi O, Aggarwal S, Trehanpati N, Ramakrishna G, Grewal AS, Krishan P. In vitro targeted screening and molecular docking of stilbene, quinones, and flavonoid on 3T3-L1 pre-adipocytes for anti-adipogenic actions. Naunyn Schmiedebergs Arch Pharmacol. (2020) 393:2093–106. doi: 10.1007/s00210-020-01919-w
36. Madic V, Petrovic A, Juskovic M, Jugovic D, Djordjevic L, Stojanovic G, et al. Polyherbal mixture ameliorates hyperglycemia, hyperlipidemia and histopathological changes of pancreas, kidney and liver in a rat model of type 1 diabetes. J Ethnopharmacol. (2021) 265:113210. doi: 10.1016/j.jep.2020.113210
37. Moor AE, Harnik Y, Ben-Moshe S, Massasa EE, Rozenberg M, Eilam R, et al. Spatial reconstruction of single enterocytes uncovers broad zonation along the intestinal villus axis. Cell. (2018) 175:1156.e–67.e. doi: 10.1016/j.cell.2018.08.063
38. Modrackova N, Vlkova E, Tejnecky V, Schwab C, Neuzil-Bunesova V. Bifidobacterium beta-glucosidase activity and fermentation of dietary plant glucosides is species and strain specific. Microorganisms. (2020) 8:839. doi: 10.3390/microorganisms8060839
39. Jung WJ, Kim SW, Giri SS, Kim HJ, Kim SG, Kang JW, et al. Janthinobacterium tructae sp. nov., isolated from kidney of rainbow trout (Oncorhynchus mykiss). Pathogens. (2021) 10:229. doi: 10.3390/pathogens10020229
40. Lan J, Wang K, Chen G, Cao G, Yang C. Effects of inulin and isomalto-oligosaccharide on diphenoxylate-induced constipation, gastrointestinal motility-related hormones, short-chain fatty acids, and the intestinal flora in rats. Food Funct. (2020) 11:9216–25. doi: 10.1039/d0fo00865f
41. Wang L, Feng Y, Wang J, Luo T, Wang X, Wu M, et al. Arbutin ameliorates murine colitis by inhibiting JAK2 signaling pathway. Front Pharmacol. (2021) 12:683818. doi: 10.3389/fphar.2021.683818
42. Fang X, Wu H, Wang X, Lian F, Li M, Miao R, et al. Modulation of gut microbiota and metabolites by berberine in treating mice with disturbances in glucose and lipid metabolism. Front Pharmacol. (2022) 13:870407. doi: 10.3389/fphar.2022.870407
43. Fassarella M, Blaak EE, Penders J, Nauta A, Smidt H, Zoetendal EG. Gut microbiome stability and resilience: Elucidating the response to perturbations in order to modulate gut health. Gut. (2021) 70:595–605. doi: 10.1136/gutjnl-2020-321747
44. Ianiro G, Tilg H, Gasbarrini A. Antibiotics as deep modulators of gut microbiota: Between good and evil. Gut. (2016) 65:1906–15. doi: 10.1136/gutjnl-2016-312297
45. Weingarden AR, Vaughn BP. Intestinal microbiota, fecal microbiota transplantation, and inflammatory bowel disease. Gut Microbes. (2017) 8:238–52. doi: 10.1080/19490976.2017.1290757
46. de Groot PF, Frissen MN, de Clercq NC, Nieuwdorp M. Fecal microbiota transplantation in metabolic syndrome: History, present and future. Gut Microbes. (2017) 8:253–67. doi: 10.1080/19490976.2017.1293224
47. Korpela K, Helve O, Kolho KL, Saisto T, Skogberg K, Dikareva E, et al. Maternal fecal microbiota transplantation in cesarean-born infants rapidly restores normal gut microbial development: A proof-of-concept study. Cell. (2020) 183:324.e–34.e. doi: 10.1016/j.cell.2020.08.047
48. Palma GD, Lynch MDJ, Lu J, Dang VT, Deng Y, Jury J, et al. Transplantation of fecal microbiota from patients with irritable bowel syndrome alters gut function and behavior in recipient mice. Sci Transl Med. (2017) 9:eaaf6397. doi: 10.1126/scitranslmed.aaf6397
49. Craven L, Rahman A, Nair Parvathy S, Beaton M, Silverman J, Qumosani K, et al. Allogenic fecal microbiota transplantation in patients with nonalcoholic fatty liver disease improves abnormal small intestinal permeability: A randomized control trial. Am J Gastroenterol. (2020) 115:1055–65. doi: 10.14309/ajg.0000000000000661
50. Koch BEV, Yang S, Lamers G, Stougaard J, Spaink HP. Intestinal microbiome adjusts the innate immune setpoint during colonization through negative regulation of MyD88. Nat Commun. (2018) 9:4099. doi: 10.1038/s41467-018-06658-4
51. Schretter CE, Vielmetter J, Bartos I, Marka Z, Marka S, Argade S, et al. A gut microbial factor modulates locomotor behaviour in Drosophila. Nature. (2018) 563:402–6. doi: 10.1038/s41586-018-0634-9
52. Lordan C, Thapa D, Ross RP, Cotter PD. Potential for enriching next-generation health-promoting gut bacteria through prebiotics and other dietary components. Gut Microbes. (2020) 11:1–20. doi: 10.1080/19490976.2019.1613124
53. Sanders ME, Merenstein DJ, Reid G, Gibson GR, Rastall RA. Probiotics and prebiotics in intestinal health and disease: From biology to the clinic. Nat Rev Gastroenterol Hepatol. (2019) 16:605–16. doi: 10.1038/s41575-019-0173-3
Keywords: arbutin, gut development, gut microbiota, fecal microflora transplantation, Lactobacillus intestinalis
Citation: Ma J, Chen S, Li Y, Wu X and Song Z (2022) Arbutin improves gut development and serum lipids via Lactobacillus intestinalis. Front. Nutr. 9:948573. doi: 10.3389/fnut.2022.948573
Received: 20 May 2022; Accepted: 02 August 2022;
Published: 09 September 2022.
Edited by:
Yong Su, Nanjing Agricultural University, ChinaReviewed by:
Hao Zhong, Zhejiang University of Technology, ChinaShunfen Zhang, State Key Laboratory of Animal Nutrition, Institute of Animal Sciences (CAAS), China
Copyright © 2022 Ma, Chen, Li, Wu and Song. This is an open-access article distributed under the terms of the Creative Commons Attribution License (CC BY). The use, distribution or reproduction in other forums is permitted, provided the original author(s) and the copyright owner(s) are credited and that the original publication in this journal is cited, in accordance with accepted academic practice. No use, distribution or reproduction is permitted which does not comply with these terms.
*Correspondence: Zehe Song, emVoZXNvbmcxMTFAMTYzLmNvbQ==