- 1College of Food Science and Technology, Henan University of Technology, Zhengzhou, China
- 2Kemen Noodle Manufacturing Co., Ltd., Changsha, China
- 3Angel Yeast Co., Ltd., Yichang, China
Wheat germ, a byproduct of wheat industrial processing, contains 30% protein and is a comprehensive source of plant-based protein. But a large amount of wheat germs are disposed of as waste every year. Wheat germ protein can be hydrolyzed into polypeptides with antioxidant, antihypertensive, anti-tumor, bacteriostatic and other activities. At present, researches on the hydrolysis of wheat germ protein and the preparation of bioactive peptides from wheat germ protein have attracted increasing attentions. However, the traditional protein hydrolysis method, protease hydrolysis, can no longer meet the market's needs for efficient production. Various auxiliary means, such as ultrasound, microwave and membrane separation, were applied to boost the yield and biological activity of wheat germ peptides by enzymatic hydrolysis. Under ultrasound and microwave, the protein structure may expand to increase the binding sites between enzyme and substrate and promote hydrolysis efficiency. Membrane separation is applied to separate products from enzymatic hydrolysate to reduce the inhibitory effect of the product on the hydrolysis reaction. The paper reviewed the hydrolysis methods of wheat germ protein and summarized the biological activity of wheat germ peptides to provide references for further study of wheat germ peptides.
Introduction
Wheat (Triticum aestivum L.) is a kind of important grain cereals, and wheat seeds consist of endosperm, bran and germ. Wheat germ, accounting for 2%-3% of the total grain weight, is the part that breeds new life, and is rich in protein, fat, unsaturated fatty acids, dietary fiber, minerals, and vitamins (1). Wheat germ and bran are the most important by-products of the dry milling industry, and these by-products are not well utilized. At present, wheat germ is mainly used to produce wheat germ oil, and wheat germ protein is the most important by-product in the production of wheat germ oil. Defatted wheat germ contained 30% protein, which meet the requirements of FAO/WHO for high-quality protein (2–4). Wheat germ protein contained 18.9% globulin, 0.30%-0.37% glutenin, 30.0% soluble albumin, 14.0% malt soluble protein, and various essential amino acids such as lysine, threonine and methionine (5–7). It is one of the most abundant sources of plant protein (8), and it is easier to obtain higher nutritional value than animal protein sources (9, 10). Wheat germ protein might affect immune regulation, aging and inhibit tumors. It could be directly used to treat cholesterol reduction (11–13), but its allergens also need to be alerted (14).
Wheat germ proteins have many amino acid sequences with biological action and can be used to prepared bioactive peptides. The production of wheat germ peptides not only can increase the source of bioactive peptides, but also improve the utilization rate of wheat germ (15). Wheat germ powder was defatted and hydrolyzed to obtain wheat germ protein hydrolysates (WGPHs) (16). WGPHs contained free amino acids and polypeptides with different molecular weights, which could be purified by ultrafiltration, anion exchange chromatography, gel filtration chromatography, reversed-phase high performance liquid chromatography and other methods (16). According to molecular weight, peptides could be divided into 11,563–1512 Da (17.78%), 1512–842 Da (17.50%), 842–372 Da (27.38%) and 372–76 Da (30.65%) (17). The traditional enzymatic hydrolysis method was protease hydrolysis, such as alcalase alkaline protease, flavourzyme, papain, neutrase and protamex (18). However, the traditional method has the disadvantage of low hydrolysis efficiency. To overcome this shortcoming, several technologies, including ultrasonic (19), microwave (20) and membrane separation technology (21), were adopted to maximize the hydrolysis rate of protein and the utilization rate of enzyme.
More and more researchers have begun to study bioactive peptides to replace drugs to treat cancer (22), hypertension and other diseases. Bioactive peptides could be extracted from corn protein, soybean powder, tea seed powder, and so on (23–26). Wheat germ could release bioactive peptides under the action of protease, such as an anti-fatigue peptide, antihypertensive peptide, antioxidant peptide and antibacterial peptide (2). Wheat germ polypeptide had immune activity in treating celiac disease (27), and could be used as a natural antioxidant to destroy reactive oxygen species (28). Antioxidant peptides in wheat germ could inhibit ultraviolet-induced oxidative damage by promoting the activity of superoxide dismutase, glutathione peroxidase, catalase and ascorbate peroxidase (29). Electron beam irradiation (EBI) could destroy the secondary structure of protein, reduce the surface hydrophobicity of protein, and at the same time improve the antioxidant capacity of WGPHs (30, 31). However, heat and malondialdehyde treatment could reduce the free radical scavenging ability of wheat germ polypeptides (32). Therefore, the focuses of this paper were as follows: (1) The principles, advantages and disadvantages of preparation methods of wheat germ polypeptide; (2) The biological activity of wheat germ polypeptide and its current application direction.
The preparation methods of wheat germ polypeptides
The preparation of wheat germ protein and selection of proteases
There are many impurities in hydrolysate (such as carbohydrate, pigment, etc), which are difficult to remove when the defatted wheat germ used as substrate (33). Therefore, the extraction of defatted wheat germ protein is more beneficial to the preparation of high purity polypeptide products. The preparation of wheat germ protein usually comprised the following steps (18): heating wheat germ 105 °C for 15 min to inactivate the endogenous enzymes of wheat germ, defatting with supercritical carbon dioxide to obtain defatted wheat germ (DWG) powder, extracting defatted wheat germ protein (DWGP) with alkaline solution (pH 9.5), adjusting pH to the isoelectric point of the wheat germ protein to precipitate the protein (34), and freeze drying to obtain the wheat germ protein powder. DWGP could also be extracted directly by reverse micellar extraction (35).
The peptide bond of protein was broken under the action of protease, and peptides with different molecular weight are generated, which were more easily absorbed by the human body. The degree of hydrolysis (DH) was used to indicate the degree of protein hydrolysis. If the DH value was higher, it means that the more peptide bonds were fractured and the proteins were hydrolyzed more thoroughly. With the extension of hydrolysis time, the degree of hydrolysis of protein gradually increased, while the molecular weight of peptide gradually decreased. However, peptides had the optimal molecular size or chain length (mainly dipeptide and tripeptide) and better functional characteristics (36–38). Therefore, it was necessary to find the suitable conditions for enzymatic hydrolysis. Proteases commonly used in enzymatic hydrolysis of wheat germ protein include flavor protease (18), Alcalase alkaline protease (19), papain (39), and so on. The enzymatic hydrolysis conditions of protease were different, and the properties of hydrolysate were different, as shown in Table 1.
Protease hydrolysis
Traditional protease hydrolysis
The traditional enzymatic hydrolysis method was through protease hydrolysis without any auxiliary methods. Zhu et al. (40) hydrolysated DWGP by flavourzyme, alcalase alkaline protease and papain. They found that alcalase alkaline protease had the highest DH value and metal chelation activity. After 180 min, the rate of enzymolysis slowed down, but the metal chelation activity of hydrolysate reached the maximum of 69.62±0.96% at 200 min, and then decreased with the progress of hydrolysis reaction (40). Wang et al. (16) found that the maximum calcium binding capacity of WGPHs was 48.26 ± 0.53% after hydrolyzing DWGP for 240 min. When the hydrolysis time exceeded 240 min, the calcium binding capacity decreased rapidly. The amino acid sequence FVDVT (PHE-ASP-Val-THR) of WGPHs was obtained after purification, and its calcium binding capacity reached 89.94 ± 0.75%, which was 86.37% higher than that of WGPHs (16). Protease hydrolysis had many advantages, such as high biosafety, no toxic and harmful by-products, controllable enzymatic hydrolysis conditions, economy and high efficiency. However, the conventional enzymatic hydrolysis method takes too long, and as time goes on, the activity of enzyme will decrease. It is necessary to look for auxiliary methods to solve these problems.
Ultrasonic assisted enzymatic hydrolysis
There were two main methods of ultrasound-assisted enzymatic hydrolysis: sonication during enzymatic hydrolysis and ultrasound pretreatment of DWGP and preparation of enzymatic hydrolysates. At present, most of the research is about ultrasonic pretreatment of wheat germ protein. The effects of ultrasonic on protein enzymatic hydrolysis mainly included the change of protein structure, enzyme activity and enzymatic hydrolysis process. The porous protein structure was obtained by ultrasonic treatment to break the hydrogen bonds and other secondary bonds between protein molecules (49). After ultrasonic treatment, the β-turns and β-sheets of DWGP transformed, and the hydrophobic groups of proteins were exposed, which made them more susceptible to alkaline protease, while the contents of hydrophobic amino acids related to angiotensin converting enzyme (ACE) inhibitory activity increased (41, 50, 51). Liu et al. (51) found that ACE inhibitory activity of protein enzymatic hydrolysate was increased by 23.96% after 10 min of ultrasonic pretreatment with 600 W. Huang et al. (42) confirmed that after DWGP was pretreated with 600 W ultrasonic for 10 min, the ACE inhibitory activity could increase by 32.14% compared with the control group. Ultrasonic wave could change the enzyme activity by changing the structure of the enzyme. When the ultrasonic wave was strong, the enzyme would be destroyed and its activity would decrease or disappear (52). Ultrasonic technology could be divided into probe ultrasonic instrument and ultrasonic cleaning instrument. Both ultrasonic and temperature could affect the enzymatic hydrolysis effect, especially at low temperature. DWGP solution was pretreated by ultrasonic cleaning instrument at 20 °C for 30 min at 1200 W power, and pretreated by ultrasonic instrument with 600 W probe at 20 °C for 10 min. Under the same conditions, the concentration of peptide obtained by enzymatic hydrolysis was 231.019 μg/mL or 231.320 μg/mL (53). Ultrasonic wave could generate mechanical heat and accelerate the hydrolysis reaction of enzyme and substrate, which not only shortened the reaction time, but also improved the utilization rate of enzyme and the conversion rate of the substrate (19). However, in the specific experimental process, attention should be paid to the temperature change during ultrasonic treatment, because long-term ultrasonic treatment will cause the temperature to rise sharply, adversely affecting the heat-sensitive materials. In addition, the application of ultrasonic wave in enzymatic hydrolysis process of DWGP should be lucubrated.
Microwave-assisted enzymatic hydrolysis
The microwave radiation region are between infrared radiation and radio wave, and the frequencies of microwaves is between 0.3–300 GHz. The matrix must contain dipole or ionic material to react and generate energy (54). Microwave technology was mainly used for stabilization of wheat germ, because microwave treatment can inactivate lipase of wheat germ, reduce water content and prolong the storage period of wheat germ. However, too high microwave power might have adverse effects (20, 55). The heating of proteins by microwave energy was achieved by the rotational absorption of microwave energy by bipolar water molecules and the translation of ionic components into proteins. Microwave could also change the flexibility of the enzyme, increase the activity of the protease, and accelerate the rate of enzyme hydrolysis (56). Microwave treatments improved the solubility of protein, the recovery rate of protein, degree of hydrolysis and the scavenging activity of 2,2'-azino-bis (3-ethylbenzthiazoline-6-sulphonic acid) (ABTs) free redical (57). Compared with untreated proteins, the hydrolysates treated by microwave showed higher ACE inhibitory activity and lower immunological activity, and the hydrolysate also contained a large amount of antioxidant peptides (58, 59). Tian et la. (39) hydrolyzed wheat germ protein under microwave power of 600 W and finally obtained the polypeptide with solubility >96%. In order to accelerate the enzymatic hydrolysis reaction under the microwave environment, the protease could be immobilized on the recyclable magnetite beads (immobilized enzyme). Magnetite beads absorbed microwave radiation, and the immobilized enzymes hydrolyzed protein faster than free enzymes, therefore the immobilized enzymes could be easily separated and reused (60). Microwave-assisted enzymatic hydrolysis can overcome the shortcoming of conventional enzymatic hydrolysis time-consuming and reduce the immune response of polypeptide. However, the application of microwave in wheat germ protein hydrolysis is not extensive, so it is necessary to study the changes of polypeptide of wheat germ protein after microwave treatment.
The coupling of enzymatic hydrolysis and membrane separation
In the process of enzymatic hydrolysis, the accumulation of hydrolysis products inhibited the reaction rate. The reaction model was shown in Figure 1. The low concentration of substrate would make the model reach a stable state, while the high substrate concentration would lead to an increase in the number of peptide bonds. At that time, the system of continuous coupling of enzymatic hydrolysis and membrane separation (CEH-MS) was unstable, which aggravated the accumulation of unhydrolyzed protein (21). In order to ensure the smooth progress of enzymatic hydrolysis, it is necessary to strictly control the substrate concentration and clean the model on time. Membrane separation technology could be used to separate and purify peptides from enzymatic hydrolysate, as shown in Figure 2. The wheat germ protein solution was enzymatically hydrolyzed in a constant temperature-controlled reactor. The enzymatic hydrolysis products pass through the ultrafiltration membrane under the pressure of the pump, the target peptide was collected on the membrane module, and the macromolecules return to the reactor. The reactor combined protease solution and polypeptide extraction, and the reaction efficiency was significantly improved (43).
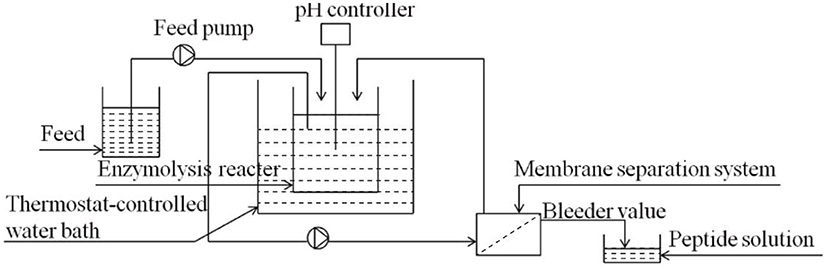
Figure 1. Continuous coupling of enzymatic hydrolysis and membrane separation (CEH-MS) set-up (21).
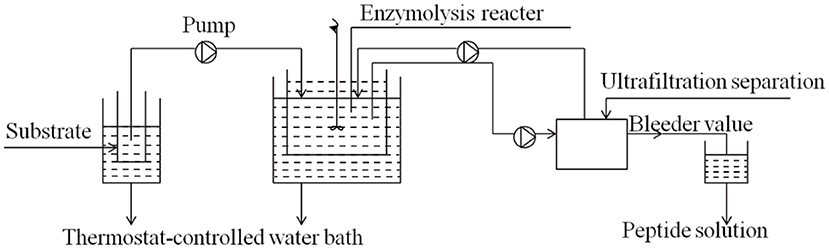
Figure 2. A schematic of enzymatic membrane reactor system for wheat germ protein isolates peptides preparation (44).
Fermentative method
Wheat germ protein could be hydrolyzed by protease, and wheat germ polypeptide could also be directly prepared by microbial fermentation. Bacillus subtilis B1 hydrolyzed DWGP under the conditions of inoculation amount of 8%, fermentation temperature of 31 °C and fermentation time of 48 h. The yield of wheat germ peptide was 8.69 mg/mL, which showed high antioxidant activity (44). WGPHs achieved the purpose of antioxidation by scavenging free radicals, increasing glutathione level and inhibiting the production of nitric oxide (45). Glutathione consisted of glutamic acid, cysteine and glycine, which could be obtained by gene cloning (61), and its molecular formula was shown in Figure 3.
The biological activity of wheat germ peptides
Antioxidant activity
During the normal aerobic metabolism, organisms can produce free radicals, which can cause cell damage and even lead to atherosclerosis, arthritis, diabetes and cancer. Wheat germ polypeptide could be used as a natural antioxidant, because it had high free radical scavenging activity and strong lipid oxidation inhibition (62, 63). The ability of polypeptide to reduce and scavenging free radicals could be used to achieve the purpose of antioxidation (64). Zhang et al. (65) found that the wheat germ polypeptides had the activity of scavenging 1,1-Diphenyl-2-Picrylhydrazyl (DPPH) free radicals, and the peptides of WG-P-4 (Gly-Pro-Phe, GLy-Pro-Glu, and PHE-Gly-Glu were the major peptides of WG-P-4) with the highest antioxidant activity. The IC50 values for DPPH were found to be 1.17 mg/mL. Karami et al. (66) hydrolyzed wheat germ protein with pepsin and obtained the amino acid sequence of the antioxidant peptide with the highest clearance rate of 2,2'-azinobis-(3-ethyl-benzothiazoline-6-onate) (ABTs) as KELPPSDADW.
Yin et al. (67) tested non-steroidal anti-inflammatory drugs (NSAIDS) on rats, and they found that the malondialdehyde (MDA) value of gastric mucosa, which caused damage and oxidative stress in small intestine of rats, was increased. Wheat peptides could enhance the activity of glutathione peroxidase (GSH) and reduce oxidative stress. Wheat peptides prevented gastric damage caused by NSAIDS by reducing oxidative stress and nitric oxide levels. Low concentration of wheat polypeptide solution had high activity and could protect gastric mucosa after oral administration (67, 68). At the same time, wheat peptides significantly decreased the activities of superoxide dismutase (SOD) and glutathione peroxidase (GSH) (69). In most organisms, superoxide anion radical can be easily converted to hydrogen peroxide by superoxide dismutase. In the absence of transition metal ions, hydrogen peroxide is quite stable, but it will produce hydroxyl radicals, which will attack and destroy every molecule in a living cell in the presence of metal ions such as iron or copper. WGPHs could scavenge superoxide anion, and the scavenging effect was enhanced with the increase of concentration (22). Ultraviolet radiation caused the generation of reactive oxygen species, such as superoxide anion radical, hydroxyl radical, and hydrogen peroxide. Scavenging reactive oxygen species could protect skin cells from the damage of ultraviolet radiation (70). In addition, reactive oxygen species was also the main cause of food spoilage (71). The antioxidant activity of wheat germ peptides could prevent their harm (72).
Antihypertensive
Hypertension is an important risk factor of cardiovascular disease. Angiotensin converting enzyme (ACE) inhibitors, angiotensin II receptor blockers (ARBs), calcium channel blockers (CCBs), beta blockers and diuretics were all antihypertensive drugs (73). Angiotensin converting enzyme (ACE) was a dipeptide carboxypeptidase, which catalyzed the hydrolysis of angiotensin I to angiotensin II in the renin-angiotensin system. In the case of abnormal metabolism, ACE would catalyze degradation and inactivation of bradykinin, a vasodilator, which lead to trigger excessive levels of angiotensin II and lead to hypertension (46, 74). The antihypertensive activity of wheat germ was due to the ACE inhibitory peptide produced after hydrolysis of protein (75), and the dominant peptide of ACE inhibitory peptide was Ile-Val-Tyr (Ivy) (76). The activity of ACE obtained by different proteolytic methods was various (77, 78). The activity of ACE inhibitory peptide in wheat germ enzymatic hydrolysate obtained by Qu et al. (19) was 65.9%, while the activity of ACE inhibitory peptide in wheat germ enzymatic hydrolysate pretreated by Jia et al. (41) ultrasonic increased by 21.0-40.7%. Phenolic compounds and dipeptides with large side chains and hydrophobic residues could effectively inhibit ACE activity, and tripeptide with the same C-terminal as ACE inhibitory peptide could also inhibit ACE activity (79). ACE inhibitory peptide has remarkable effects in alternative drugs for treating hypertension, but it is usually necessary to take antihypertensive drugs for life, and further clinical trials are needed to determine whether ACE inhibitory peptide will increase the risk of cancer.
Anticancer
In the past, the activity of glucose metabolism enzymes such as hexokinase, glucose-6-P dehydrogenase (G6PD) and transketoolase were controlled to inhibit the formation of cancer cells. The new effective natural and synthetic anticancer drugs mainly inhibited glucose-ribose conversion rather than the synthesis of nucleic acid purines and pyrimidines (80). Lunatic, which had the same sequence as soybean and barley, could be extracted from wheat. Lunasin was a unique 43-amino-acid cancer prevention peptide, which binded closely to deacetylated histone, destroying the kinetics of histone acetylation-deacetylation and leading to cell death, but this only occurred in transforming cells and does not affect normal cells. The polypeptide extracted from transgenic wheat had higher anticancer activity (81–83).
A fermented wheat germ extract (MSC) had immune activity on cancer cell system and could inhibit the synthesis and transfer of nucleic acid RNA of cancer cells (84). Methoxy-ρ-benzoquinone (MBQ) and 2,6-dimethoxy-ρ-benzoquinone (DMBQ) were two potential anticancer compounds. During the fermentation of wheat germ, the β-glycosidic bond of hydroquinone glycoside in wheat germ was cleaved by β-glucosidase of saccharomyces cerevisiae, and MBQ and DMBQ were produced (85). MSC could be used as a safe dietary supplement (86), and the addition of highly active β-glucosidase and peroxidase in fermentation broth could improve the yield of MBQ and DMBQ (87).
Others
Numerous proteins with anti-microbial activity can protect plants against pathogens, for example, lectins, pathogenesis-related proteins, hydroxyproline-rich glycoproteins, cyclophilin-like proteins, ribosome-inactivating proteins, and protease inhibitors (88, 89). On the other hand, wheat had anti-microbial peptides (AMPs) which showed higher resistance to microbial contamination (90). The main characteristic of AMPs was cationic, which enabled them to interact with microbial membranes directly or through a receptor-mediated mechanism, thus increasing the permeability of plasma membranes. Wheat peptides were resistant to most pathogens, such as brown rust, stem rust, and root rot (91, 92). In addition, it could also inhibit the adhesion of helicobacter pylori to gastric epithelial cells and prevent helicobacter pylori infection (93)).
Wheat peptide could protect rats from ethanol-induced gastric mucosal injury by inhibiting inflammation response, improving gastric mucosal blood flow, inducing endogenous molecules for cytoprotective, and maintaining the integrity of the gastric mucosal barrier (94). Wheat auxin-releasing peptides could effectively stimulate the secretion of auxin and improve gastric digestion (47). In addition, the combination of wheat peptides with fucoidan (WPF) would speed up the repair of gastric mucosa, which was due to WPF-nduced phosphorylation of a transmembrane receptor tyrosine kinase (EGFR) to protect gastric epithelial cells (95, 96). The process of preventing ethanol-induced gastric mucosal damage would not produce side effects (97). WPF could also improve the distribution of intestinal microorganisms and promote the production of short–chain fatty acids (SCFAs), thus alleviating chronic gastritis (98).
Arginine-valine-phenylalanine (RVF) derived from wheat germ peptide could prevent or delay the progression of neurodegenerative diseases (99). Wheat protein was rich in glutamine and proline residues, which could be separated and purified into opioid active peptides for treating neurological disorders (100). Wu et al. (101) found that glutelin (Gll) of wheat germ could enhance immune function by promoting lymphocyte proliferation. Wu et al. (48) hydrolyzed defatted wheat germ globulin (DWGG), and found that alcalase hydrolysate (AH) had the strongest immunomodulatory activity on lymphocyte proliferation, phagocytosis of neutral red and secretion of pro-inflammatory cytokines. The activities of polypeptide are shown in Table 1.
Conclusion
So far, the reprocessing and utilization of industrial by-products is a way to reduce waste of resources and protect the environment. As a byproduct of dry milling industry, the nutritional value of wheat germ is often underestimated. Wheat germ protein is a kind of high quality protein. Wheat germ polypeptide has various biological activities, and is widely used in the fields of medicine, beauty and food. Bioactive peptides can be produced by enzymatic hydrolysis of wheat germ protein. The common proteases include alcalase alkaline protease, flavourzyme, papain, neutrase, and protamex. The enzymatic hydrolysis method using only protease is simple but inefficient. Microwave or ultrasound-assisted hydrolysis can improve hydrolysis efficiency, and different assisted methods enhance the bioactivity of peptides to various degrees. Although assisted enzymatic hydrolysis can shorten the time, microwave or ultrasound-assisted hydrolysis will produce noise, and need to find the best parameters when using these methods.
Wheat germ polypeptide has antioxidant, antihypertensive, anticancer activities, and can be used as natural medicine. Antioxidant peptide can also be used as a food additive. However, the research and mechanism of wheat germ polypeptide in drug production is still in the stage of animal experiment, and no side effects of wheat germ polypeptide have been found. Therefore, the researches on the extraction and activity of wheat germ polypeptide still need further study. Because the chain length of wheat germ polypeptide will affect its biological activity, it is necessary to separate and purify WGPHs to obtain the polypeptide with the highest activity. The cost of separation and purification is high, and bioactive peptide can not be fully absorbed and utilized. Then, the future research directions are as follows: (1) Researchers need to deeply understand whether wheat germ polypeptide has negative effects as a drug; (2) In the future, researchers will find a more effective and economical method for separation and purification; (3) Researchers need to find a new method to make more effective use of wheat germ polypeptide; (4) In-depth analysis of amino acid sequences of bioactive peptides.
Author contributions
KD: conceptualization, software, and writing-original draft preparation. ST: funding acquisition, writing-reviewing and editing. HC: visualization and investigation. SG: supervision and project administration. XD: funding acquisition and supervision. FY: writing-reviewing, modify and editing. All authors contributed to the article and approved the submitted version.
Conflict of interest
HC and SG were employed by Kemen Noodle Manufacturing Co., Ltd., and XD was employed by Angel Yeast Co., Ltd.
The remaining authors declare that the research was conducted in the absence of any commercial or financial relationships that could be construed as a potential conflict of interest.
Publisher's note
All claims expressed in this article are solely those of the authors and do not necessarily represent those of their affili-ated organizations, or those of the publisher, the editors and the reviewers. Any product that may be evaluated in this article, or claim that may be made by its manufacturer, is not guaranteed or endorsed by the publisher.
Acknowledgments
The authors would like to acknowledge NSFC for financial assistance under NSFC Research Contract no. 31701636. This research was also supported by Cultivation Programme for Young Backbone Teachers in Henan Province (2021GGJS059) and Henan University of Technology and Natural Science Innovation Fund Support Program from Henan University of Technology (2021ZKCJ12).
References
1. Boukid F, Folloni S, Ranieri R, Vittadini E. A compendium of wheat germ: separation, stabilization and food applications. Trends Food Sci Technol. (2018) 78:120–33. doi: 10.1016/j.tifs.2018.06.001
2. Areado R, Arigoni E. Nutritive and functional properties of wheat germ. Int Food Ingredients. (1992) 4:30–4. Available online at: https://www.researchgate.net/publication/264747431_Nutritive_and_functional_properties_of_wheat_germ
3. Sun XH, Zhu KX, Zhou HM. Protein extraction from defatted wheat germ by reverse micelles: optimization of the forward extraction. J. Cereal Sci. (2008) 48:6. doi: 10.1016/j.jcs.2008.06.006
4. Arshad MU, Anjum FM, Zahoor T. Nutritional assessment of cookies supplemented with defatted wheat germ. Food Chem. (2007) 102:123–8. doi: 10.1016/j.foodchem.2006.04.040
5. Rizzello CG, Nionelli L, Coda R, Angelis MD, Gobbetti M. Effect of sourdough fermentation on stabilisation, and chemical and nutritional characteristics of wheat germ. Food Chem. (2009) 199:1079–89. doi: 10.1016/j.foodchem.2009.08.016
6. Grewe E, Leclerc JA. Commercial wheat germ, its composition. Cereal Chem. (1943) 20:423–34. Available online at: http://spis.hnlat.com/scholar/detail/54a63ff7e69d4e6ce6cb8ad3eb3a32d3
7. Zhu K, Zhou H, Qian H. Antioxidant and free radical-scavenging activities of wheat germ protein hydrolysates (WGPH) prepared with alcalase. Process Biochem. (2006) 41:1296–302. doi: 10.1016/j.procbio.2005.12.029
8. Pinckaers PJM, Kouw IWK, Hendriks FK, van Kranenburg JMX, de Groot LCPGM, Verdijk LB, et al. No differences in muscle protein synthesis rates following ingestion of wheat protein, milk protein, and their protein blend in healthy, young males. Br J Nutr. (2021) 126:1832–42. doi: 10.1017/S0007114521000635
9. van Vliet S, Burd NA, van Loon LJC. The skeletal muscle anabolic response to plant- versus animal-based protein consumption. J Nutr. (2015) 145:1981–91. doi: 10.3945/jn.114.204305
10. David P, Marcia P. Sustainability of meat-based and plant-based diets and the environment. Am J Clin Nutr. (2003) 78:660–3., 11. doi: 10.1093/ajcn/78.3.660S
11. Zhang YZ, Guan X, Guo JX, Zhang J, Chen W. Lowing effect of wheat germ protein (WGP) on cholesterol level in hypercholesterolemic HepG2 cell. J Biobased Mater Bioenergy. (2019) 13:269–73. doi: 10.1166/jbmb.2019.184
12. Yun LY, Wang S, Wu T, Li Q, Zhang M. Structural characterization of a novel glycoprotein in wheat germ and its physicochemical properties. Int J Biol Macromol. (2018) 117:1058–65. doi: 10.1016/j.ijbiomac.2018.05.169
13. Zhu K, Zhou H. Purification and characterization of a novel glycoprotein from wheat germ water-soluble extracts. Process Biochem. (2005) 40:1469–74. doi: 10.1016/j.procbio.2004.06.030
14. Zhao JL, Li ZX, Khan MU, Gao X, Yu M, Gao HY, et al. Extraction of total wheat (Triticum aestivum) protein fractions and cross-reactivity of wheat allergens with other cereals. Food Chem. (2021) 347:129064. doi: 10.1016/j.foodchem.2021.129064
15. Liu F, Chen Z, Shao J, Wang C, Chen Z. Effect of fermentation on the peptide content, phenolics and antioxidant activity of defatted wheat germ. Food Biosci. (2017) 20:141–8., 00 doi: 10.1016/j.fbio.2017.10.002
16. Wang L, Ding YY, Zhang XX, Li YF, Wang R, Luo XH, et al. Isolation of a novel calcium-binding peptide from wheat germ protein hydrolysates and the prediction for its mechanism of combination. Food Chem. (2018) 239:416–26., 090 doi: 10.1016/j.foodchem.2017.06.090
17. Cheng YH, Wang Z, Xu SY. Antioxidant properties of wheat germ protein hydrolysates evaluated in vitro. J Cent South Univ Technol. (2006) 13:160–5. doi: 10.1007/s11771-006-0149-7
18. Claver IP, Zhou H. Enzymatic hydrolysis of defatted wheat germ by proteases and the effect on the functional properties of resulting protein hydrolysates. J Food Biochem. (2005) 29:13–26. doi: 10.1111/j.1745-4514.2005.00004.x
19. Qu WJ, Ma HL, Jia JQ, He RH, Luo L, Pan ZL, et al. Enzymolysis kinetics and activities of ACE inhibitory peptides from wheat germ protein prepared with SFP ultrasound-assisted processing. Ultrason Sonochem. (2012) 19:1021–6. doi: 10.1016/j.ultsonch.2012.02.006
20. Zhang YK, Tang N, Shi L, Miao YX, Liu X, Ge XH, et al. Characterization and comparison of predominant aroma compounds in microwave-treated wheat germ and evaluation of microwave radiation on stability. J Cereal Sci. (2020) 93:102942. doi: 10.1016/j.jcs.2020.102942
21. Qu WJ, Ma HL, Zhao WR, Pan ZL. ACE-inhibitory peptides production from defatted wheat germ protein by continuous coupling of enzymatic hydrolysis and membrane separation: modeling and experimental studies. Chem Eng J. (2013) 226:139–45. doi: 10.1016/j.cej.2013.04.030
22. Sun YS, Ma CJ, Hua YY, Wei TT, Zhang LF. Multifunctional nanoparticles of paclitaxel and cyclodextrin–polypeptide conjugates with in vitro anticancer activity. Pharm Dev Technol. (2020) 25:1071–80. doi: 10.1080/10837450.2020.1787441
23. Wang YC, Li YL, Ruan SY, Lu F, Tian WM, Ma HL, et al. Antihypertensive effect of rapeseed peptides and their potential in improving the effectiveness of captopril. J Sci Food Agric. (2020) 101:3049–55. doi: 10.1002/jsfa.10939
24. Karami Z, Peighambardoust SH, Hesari J, Akbari-Adergani B, Andreu D. Identification and synthesis of multifunctional peptides from wheat germ hydrolysate fractions obtained by proteinase K digestion. J Food Biochem. (2019) 43:12800. doi: 10.1111/jfbc.12800
25. Jin DX, Liu XL, Zheng XQ, Wang XJ, He JF. Preparation of antioxidative corn protein hydrolysates, purification and evaluation of three novel corn antioxidant peptides. Food Chem. (2016) 204:427–36. doi: 10.1016/j.foodchem.2016.02.119
26. Mäkinen S, Streng T, Larsen LB, Laine A, Pihlanto A. Angiotensin-I-converting enzyme inhibitory and antihypertensive properties of potato and rapeseed protein-derived peptides. J Funct Foods. (2016) 25:160–73. doi: 10.1016/j.jff.2016.05.016
27. Vojdani A. The characterization of the repertoire of wheat antigens and peptides involved in the humoral immune responses in patients with gluten sensitivity and Crohn's disease. ISRN Allergy. (2011) 2011:29–40. doi: 10.5402/2011/950104
28. Zhang QX, Ling YF, Sun Z, Zhang L, Yu HX, Kamau SM, et al. Protective effect of whey protein hydrolysates against hydrogen peroxide-induced oxidative stress on PC12 cells. Biotechnol Lett. (2012) 34:2001–6. doi: 10.1007/s10529-012-1017-1
29. Han YT, Han ZW, Yu GY, Wang YJ, Cui RY, Wang CB, et al. Inhibitory effect of polypeptide from Chlamys farreri on ultraviolet A-induced oxidative damage on human skin fibroblasts in vitro. Pharmacol Res. (2004) 49:265–74., 009 doi: 10.1016/j.phrs.2003.09.009
30. Wang L, Li T, Sun DL, Tang MQ, Sun Z, Chen LF, et al. Effect of electron beam irradiation on the functional properties and antioxidant activity of wheat germ protein hydrolysates. Innovative Food Sci Emerging Technol. (2019) 54:192–9., 003 doi: 10.1016/j.ifset.2018.09.003
31. Wang L, Ding YY, Zhang XX, Li YF, Wang R, Luo XH, et al. Effect of electron beam on the functional properties and structure of defatted wheat germ proteins. J Food Eng. (2017) 202:9–17. doi: 10.1016/j.jfoodeng.2017.01.024
32. Tang X, Wu QP, Le GW, Wang J, Yin KJ, Shi YH, et al. Structural and antioxidant modification of wheat peptides modified by the heat and lipid peroxidation product malondialdehyde. J Food Sci. (2012) 77:16–22. doi: 10.1111/j.1750-3841.2011.02500.x
33. Matsui T, Li CH, Osajima Y. Preparation and characterization of novel bioactive peptides responsible for angiotensin I-converting enzyme inhibition from wheat germ. J Pept Sci. (1999) 5:289–97. doi: 10.1002/(SICI)1099-1387(199907)5:7<289::AID-PSC196>3.0.CO;2-6
34. Ge Y, Sun A, Ni Y, Cai TY. Some nutritional and functional properties of defatted wheat germ protein. J Agric Food Chem. (2000) 48:6215–8. doi: 10.1021/jf000478m
35. Zhu KX, Sun XH, Chen ZC, Peng W, Qian HF, Zhou HM, et al. Comparison of functional properties and secondary structures of defatted wheat germ proteins separated by reverse micelles and alkaline extraction and isoelectric precipitation. Food Chem. (2010) 123:1163–9. doi: 10.1016/j.foodchem.2010.05.081
36. Tardioli PW, Sousa RJr, Giordano RC, Giordano RLC. Kinetic model of the hydrolysis of polypeptides catalyzed by alcalase, immobilized on 10% glyoxylagarose. Enzyme Microb. Technol. (2005) 36:555–564. doi: 10.1016/j.enzmictec.2004.12.002
37. Clemente A. Enzymatic protein hydrolysates in human nutrition. Trends Food Sci Technol. (2000) 11:254–62. doi: 10.1016/S0924-2244(01)00007-3
38. Miedzianka J, Peksa A, Pokora M, Rytel E, Tajner-Czopek A, Kita A, et al. Improving the properties of fodder potato protein concentrate by enzymatic hydrolysis. Food Chem. (2014) 159:512–8., 054 doi: 10.1016/j.foodchem.2014.03.054
39. Tian SQ, Du K, Yan F, Li YH. Microwave-assisted enzymatic hydrolysis of wheat germ albumin to prepare polypeptides and influence on physical and chemical properties. Food Chem. (2022) 374:131707. doi: 10.1016/j.foodchem.2021.131707
40. Zhu KX, Wang XP, Guo XN. Isolation and characterization of zinc-chelating peptides from wheat germ protein hydrolysates. J Funct Foods. (2015) 12:23–32. doi: 10.1016/j.jff.2014.10.030
41. Jia JQ, Ma HL, Zhao WR, Wang ZB, Tian WM, Luo L, et al. The use of ultrasound for enzymatic preparation of ACE-inhibitory peptides from wheat germ protein. Food Chem. (2010) 119:336–42. doi: 10.1016/j.foodchem.2009.06.036
42. Huang L, Liu B, Ma H, Zhang X. Combined effect of ultrasound and enzymatic treatments on production of ACE inhibitory peptides from wheat germ protein. J Food Process Preserv. (2014) 38:1632–40. doi: 10.1111/jfpp.12125
43. He RH, Xing H, Wang ZP, Ding WH, Zhu PP, Liu B, et al. Establishment of an enzymatic membrane reactor for angiotensin-converting enzyme inhibitory peptides preparation from wheat germ protein isolates. J Food Process Eng. (2016) 39:296–305. doi: 10.1111/jfpe.12224
44. Niu LY, Jiang ST, Pan LJ. Preparation and evaluation of antioxidant activities of peptides obtained from defatted wheat germ by fermentation. J Food Sci Technol. (2013) 50:53–61. doi: 10.1007/s13197-011-0318-z
45. Cruz-Chamorro I, Álvarez-Sanchez N, Santos-Sanchez G, Pedroche J, Fernández-Pachón MS, Millán F, et al. Immunomodulatory and antioxidant properties of wheat gluten protein hydrolysates in human peripheral blood mononuclear cells. Nutrients. (2020) 12:1673. doi: 10.3390/nu12061673
46. Zhang P, Chang C, Liu HJ, Li B, Yan QJ, Jiang ZQ, et al. Identification of novel angiotensin I-converting enzyme (ACE) inhibitory peptides from wheat gluten hydrolysate by the protease of Pseudomonas aeruginosa. J Funct Foods. (2020) 65:103751. doi: 10.1016/j.jff.2019.103751
47. Tanikawa K, Kaneko K, Abe S, Nakato J, Tokuyama Y, Odaka S, et al. Wheat-ghretropins: novel ghrelin-releasing peptides derived from wheat protein. FEBS Open Bio. (2021) 11:1144–52. doi: 10.1002/2211-5463.13124
48. Wu WJ, Zhang MM, Sun CZ, Brennan M, Li HX, Wang G, et al. Enzymatic preparation of immunomodulatory hydrolysates from defatted wheat germ (Triticum Vulgare) globulin. Int J Food Sci Technol. (2016) 51:2556–66. doi: 10.1111/ijfs.13238
49. Zhang J, Luo DL, Xiang JL, Xu W, Xu BC, Li PY, et al. Structural variations of wheat proteins under ultrasound treatment. J Cereal Sci. (2021) 99:103219. doi: 10.1016/j.jcs.2021.103219
50. Yang X, Li YL, Li SY, Oladejo AO, Wang YC, Huang SF, et al. Effects of multi-frequency ultrasound pretreatment under low power density on the enzymolysis and the structure characterization of defatted wheat germ protein. Ultrason Sonochem. (2017) 38:410. doi: 10.1016/j.ultsonch.2017.03.001
51. Liu B, Ma HL, Li SJ, Tian WM, Wu BG. Study on the structure of defatted wheat germ protein isolate under ultrasonic treatment. Spectrosc Spectral Anal. (2011) 31:2220–5.
52. Knorr D, Zenker M, Heinz V, Lee DU. Applications and potential of ultrasonics in food processing. Trends Food Sci Technol. (2004) 15:261–6., 001 doi: 10.1016/j.tifs.2003.12.001
53. Qu WJ, Ma HL, Liu B, He RH, Pan ZL, Abano EE, et al. Enzymolysis reaction kinetics and thermodynamics of defatted wheat germ protein with ultrasonic pretreatment. Ultrason Sonochem. (2013) 20:1408–13. doi: 10.1016/j.ultsonch.2013.04.012
54. Wathey B, Tierney J, Lidström P, Westman J. The impact of microwave-assisted organic chemistry on drug discovery. Drug Discovery Today. (2002) 7:373–80. doi: 10.1016/S1359-6446(02)02178-5
55. Chen ZW, Li YL, Wang LK, Liu SY, Wang KK, Sun J, et al. Evaluation of the possible non-thermal effect of microwave radiation on the inactivation of wheat germ lipase. J Food Process Eng. (2017) 40:1–11. doi: 10.1111/jfpe.12506
56. Nguyen E, Jones O, Kim YHB, Martin-Gonzalez FS, Liceaga AM. Impact of microwave-assisted enzymatic hydrolysis on functional and antioxidant properties of rainbow trout oncorhynchus mykiss by-products. Fish. Sci. (2017) 83:317–31. doi: 10.1007/s12562-017-1067-3
57. Ketnawa S, Liceaga AM. Effect of microwave treatments on antioxidant activity and antigenicity of fish frame protein hydrolysates, Food Bioprocess Technol. (2017) 10:582–91. doi: 10.1007/s11947-016-1841-8
58. Hall F, Liceaga A. Effect of microwave-assisted enzymatic hydrolysis of cricket (Gryllodes sigillatus) protein on ACE and DPP-IV inhibition and tropomyosin-IgG binding. J Funct Foods. (2020) 64:103634. doi: 10.1016/j.jff.2019.103634
59. Urbizo-Reyes U, Martin-Gonzalez MFS, Garcia-Bravo J, Vigilc ALM, Liceagaa AM. Physicochemical characteristics of chia seed (Salvia hispanica) protein hydrolysates produced using ultrasonication followed by microwave-assisted hydrolysis. Food Hydrocoll. (2019) 97:105187. doi: 10.1016/j.foodhyd.2019.105187
60. Lin S, Yao GP, Qi DW, Li Y, Deng CH, Yang PY, et al. Fast and Efficient proteolysis by microwave-assisted protein digestion using trypsin-immobilized magnetic silica microspheres. Anal Chem. (2008) 80:3655–65. doi: 10.1021/ac800023r
61. Chen DF, Kawarasaki Y, Nakano H, Yamane T. Clbning and in vitro and in vivo expression of plant glutathione s-Transferase zeta class genes. J Biosci Bioeng. (2003) 95:594–600. doi: 10.1016/S1389-1723(03)80168-8
62. Halliwell B. Free radicals, antioxidants, and human disease: curiosity, cause, or consequence. Lancet. (1994) 344:721–4. doi: 10.1016/S0140-6736(94)92211-X
63. Rajapakse N, Mendis E, Jung WK, Je JY, Kim SK. Purification of a radical scavenging peptide from fermented mussel sauce and its antioxidant properties. Food Res Int. (2005) 38:175–82. doi: 10.1016/j.foodres.2004.10.002
64. Zeng WC, Zhang WC, Zhang WH, Shi B. Antioxidant activity and characterization of bioactive polypeptides from bovine hair. React Funct Polym. (2013) 73:573–8. doi: 10.1016/j.reactfunctpolym.2012.12.013
65. Zhang JX, Wen CT, Li XZ, Duan YQ, Zhang HH, Ma HL, et al. Antioxidant peptide fractions isolated from wheat germ protein with subcritical water extraction and its transport across Caco-2 cells. J Food Sci. (2019) 84:2139–46. doi: 10.1111/1750-3841.14720
66. Karami Z, Peighambardoust SH, Hesari J, Akbari-Adergani B, Andreu D. Antioxidant, anticancer and ACE-inhibitory activities of bioactive peptides from wheat germ protein hydrolysates. Food Biosci. (2019) 32:100450. doi: 10.1016/j.fbio.2019.100450
67. Yin H, Cai HZ, Wang SK, Yang LG, Sun GJ. Wheat peptides reduce oxidative stress and inhibit NO production through modulating μ-opioid receptor in a rat NSAID-induced stomach damage model. Chin J Nat Med. (2015) 13:22–9. doi: 10.1016/S1875-5364(15)60003-6
68. Yin H, Pan XC, Wang SK, Yang LG, Sun GJ. Protective effect of wheat peptides against small intestinal damage induced by non-steroidal anti-inflammatory drugs in rats. J Integr Agric. (2014) 13:2019–27. doi: 10.1016/S2095-3119(13)60619-X
69. Yin H, Pan XC, Song ZX, Wang SK, Yang LG, Sun GJ, et al. Protective effect of wheat peptides against indomethacin-induced oxidative stress in IEC-6 cells. Nutrients. (2014) 6:564–74. doi: 10.3390/nu6020564
70. Hou H, Li BF, Zhang ZH, Xue CH, Yu GL, Wang JF, et al. Moisture absorption and retention properties, and activity in alleviating skin photodamage of collagen polypeptide from marine fish skin. Food Chem. (2012) 135:1432–9., 009. doi: 10.1016/j.foodchem.2012.06.009
71. Mei DJ, Yu GP, Sun AM. Preparation, purification and identification of antioxidant peptides with bienzyme hydrolysis from rice bran protein. Adv Mater Res 610-613. (2013) 72–80. doi: 10.4028/www.scientific.net/AMR.610-613.72
72. Qiao HR, Bi XJ, Zhang YY, Liu MR, Zu SC, Jia N, et al. Enzymic polypeptide antioxidant activity and inhibitory activity on α-glucosidase and α-amylase from Paeonia ostii cake. Ind Crops Prod. (2020) 146:112158. doi: 10.1016/j.indcrop.2020.112158
73. Sanidas E, Velliou M, Papadopoulos D, Fotsali A, Iliopoulos D, Mantzourani M, et al. Antihypertensive drugs and risk of cancer: between scylla and charybdis. Am J Hypertens. (2020) 33:1049–58. doi: 10.1093/ajh/hpaa098
74. Daliri EBM, Lee BH, Oh DH. Current trends and perspectives of bioactive peptides. Crit Rev Food Sci Nutr. (2017) 58:2273–84. doi: 10.1080/10408398.2017.1319795
75. Yoshikawa M, Fujita H, Matoba N, Takenaka Y, Yamamoto T, Yamauchi R, et al. Bioactive peptides derived from food proteins preventing lifestyle-related diseases. Biofactors. (2000) 12:143–6. doi: 10.1002/biof.5520120122
76. Matsui T, Li CH, Tanaka T, Maki T, Osajima Y, Matsumoto K, et al. Depressor effect of wheat germ hydrolysate and its novel angiotensin I-converting enzyme inhibitory peptide, Ile-Val-Tyr, and the metabolism in rat and human plasma. Biol Pharm Bull. (2000) 23:427–31. doi: 10.1248/bpb.23.427
77. Chirinos R, Cerna E, Pedreschi R, Calsin M, Aguilar-Galvez A, Campos D, et al. Multifunctional in vitro bioactive properties: Antioxidant, antidiabetic, and antihypertensive of protein hydrolyzates from tarwi (Lupinus mutabilis Sweet) obtained by enzymatic biotransformation. Cereal Chem. (2020) 98:423–33. doi: 10.1002/cche.10382
78. Ra JE, Woo SY, Hui J, Mi JL, Seo WD. Evaluation of antihypertensive polyphenols of barley (Hordeum vulgare L.) seedlings via their effects on angiotensin-converting enzyme (ACE) inhibition. Appl Biol Chem. (2020) 63:441–444. doi: 10.1186/s13765-020-00519-9
79. Daliri EBM, Tyagi A, Ofosu FK, Chelliah R, Kim JH, Kim JR, et al. A discovery-based metabolomic approach using UHPLC Q-TOF MS/MS unveils a plethora of prospective antihypertensive compounds in Korean fermented soybeans. LWT. (2021) 137:110399. doi: 10.1016/j.lwt.2020.110399
80. Boros LG, Cascante M, Lee WNP. Metabolic profiling of cell growth and death in cancer: applications in drug discovery. Drug Discov Today. (2002) 7:364–72. doi: 10.1016/S1359-6446(02)02179-7
81. Jeong HJ, Lam Y, Lumen BO. Barley lunasin suppresses ras-induced colony formation and inhibits core histone acetylation in mammalian cells. J Agric Food Chem. (2002) 50:7901–06. doi: 10.1021/jf0256945
82. Jeong HJ, Jeong JB, Kim DS, Park JH, Lee JB, Kweon DH, et al. The cancer preventive peptide lunasin from wheat inhibits core histone acetylation. Cancer Lett. (2007) 255:42–8., 022 doi: 10.1016/j.canlet.2007.03.022
83. Fan X, Qin PY, Hao YQ, Guo HM, Blecker C, Everaert N, et al. Overexpression of soybean-derived lunasin in wheat and assessment of its anti-proliferative activity in colorectal cancer HT-29 Cells. Int J Mol Sci. (2020) 21:9594. doi: 10.3390/ijms21249594
84. Jakab F, Shoenfeld Y, Balogh A, Nichelatti M, Hoffmann A, Kaha'n Zs, et al. A medical nutriment has supportive value in the treatment of colorectal cancer. Br J Cancer. (2003) 89:465–69. doi: 10.1038/sj.bjc.6601153
85. Zheng ZY, Guo XN, Zhu KX, Peng W, Zhou HM. Artificial neural network–genetic algorithm to optimize wheat germ fermentation condition: application to the production of two anti-tumor benzoquinones. Food Chem. (2017) 227:264. doi: 10.1016/j.foodchem.2017.01.077
86. Fajka-Boja R, Hidvegi M, Shoenfeld Y, Ion G, Demydenko D, Tomoskozi-Farkas R, et al. Fermented wheat germ extract induces apoptosis and downregulation of major histocompatibility complex class I proteins in tumor T and B cell lines. Int J Oncol. (2002) 20:563–70. doi: 10.3892/ijo.20.3.563
87. Rizzello CG, Mueller T, Coda R, Reipsch F, Gobbetti M. Synthesis of 2-methoxy benzoquinone and 2,6-dimethoxybenzoquinone by selected lactic acid bacteria during sourdough fermentation of wheat germ. Microb Cell Fact. (2013) 12:105. doi: 10.1186/1475-2859-12-105
88. Siemion IZ, Wilusz T, Polanowski A. On the genetic and structural similarities between the squash seeds polypeptide trypsin inhibitors and wheat germ agglutinin. Mol Cell Biochem60. (1984) 159–61. doi: 10.1007/BF00222486
89. Selitrennikoff CP. Antifungal proteins. Appl Environ Microbiol. (2001) 67:2883–94. doi: 10.1128/AEM.67.7.2883-2894.2001
90. Egorov TA, Odintsova TI, Pukhalsky VA, Grishin EV. Diversity of wheat anti-microbial peptides. Peptides. (2005) 26:2064–73. doi: 10.1016/j.peptides.2005.03.007
91. Andreu D, Rivas L. Animal antimicrobial peptides: an overview. Biopolymers. (1998) 47:415–33. doi: 10.1002/(SICI)1097-0282(1998)47:6<415::AID-BIP2>3.0.CO;2-D
92. Bertrand B, Munoz-Garay C. Marine antimicrobial peptides: a promising source of new generation antibiotics and other bio-active molecules. Int J Pept Res Ther. (2019) 25:1441–50. doi: 10.1007/s10989-018-9789-3
93. Sun XH, Zhang SY, Udenigwe CC, Ren J, Li SN, Wang H, et al. Wheat germ-derived peptides exert anti-adhesive activity against Helicobacter pylori: insights into structural characteristics of identified peptides. J Agric Food Chem. (2020) 68:11954–74. doi: 10.1021/acs.jafc.0c04367
94. Yu LL, Li RJ, Liu W, Zhou YL, Li Y, Qin Y, et al. Protective effects of wheat peptides against ethanol-induced gastric mucosal lesions in rats: vasodilation and anti-inflammation. Nutrients. (2020) 12:2355. doi: 10.3390/nu12082355
95. Jorissen RN, Walker F, Pouliot N, Garrett TPJ, Ward CW, Burgess AW, et al. Epidermal growth factor receptor: mechanisms of activation and signaling. Exp Cell Res. (2003) 284:31–53. doi: 10.1016/S0014-4827(02)00098-8
96. Zwick E, Hackel PO, Prenzel N, Ullrich A. The EGF receptor as central transducer of heterologous signalling systems. Trends Pharmacol Sci. (1999) 20:408–12. doi: 10.1016/S0165-6147(99)01373-5
97. Kan JT, Hood M, Burns C, Scholten J, Chuang J, Tian F, et al. A novel combination of wheat peptides and fucoidan attenuates ethanol-induced gastric mucosal damage through anti-oxidant, anti-inflammatory, and pro-survival mechanisms. Nutrients. (2017) 9:978. doi: 10.3390/nu9090978
98. Kan JT, Cheng JR, Xu LM, Hood M, Zhong DF, Cheng MJ, et al. The combination of wheat peptides and fucoidan protects against chronic superficial gastritis and alters gut microbiota: a double-blinded, placebo-controlled study. Eur J Nutr. (2020) 59:1655–66. doi: 10.1007/s00394-019-02020-6
99. Cheng YH, Zhang L, Sun W, Tang JQ, Lv ZL, Xu Z, et al. Protective effects of a wheat germ peptide (RVF) against H2O2-induced oxidative stress in human neuroblastoma cells. Biotechnol Lett. (2014) 36:1615–22. doi: 10.1007/s10529-014-1521-6
100. Huebner FR, Lieberman KW, Rubino RP, Wall JS. Demonstration of high opioid-like activity in isolated peptides from wheat gluten hydrolysates. Peptides. (1984) 5:1139–47. doi: 10.1016/0196-9781(84)90180-3
Keywords: wheat germ protein, enzymatic hydrolysis, peptides, biological activity, proteases
Citation: Du K, Tian S, Chen H, Gao S, Dong X and Yan F (2022) Application of enzymes in the preparation of wheat germ polypeptides and their biological activities. Front. Nutr. 9:943950. doi: 10.3389/fnut.2022.943950
Received: 14 May 2022; Accepted: 27 June 2022;
Published: 18 July 2022.
Edited by:
Miguel Angel Prieto Lage, University of Vigo, SpainReviewed by:
Jose Zapata Montoya, University of Antioquia, ColombiaPauline Donn, University of Yaounde I, Cameroon
Sepidar Seyyedimansour, Islamic Azad University of Tabriz, Iran
Copyright © 2022 Du, Tian, Chen, Gao, Dong and Yan. This is an open-access article distributed under the terms of the Creative Commons Attribution License (CC BY). The use, distribution or reproduction in other forums is permitted, provided the original author(s) and the copyright owner(s) are credited and that the original publication in this journal is cited, in accordance with accepted academic practice. No use, distribution or reproduction is permitted which does not comply with these terms.
*Correspondence: Shuangqi Tian, tianshuangqi2021@163.com