- 1State Key Laboratory for Conservation and Utilization of Subtropical Agro-bioresources, College of Animal Science and Technology, Guangxi University, Nanning, China
- 2Animal Nutritional Genome and Germplasm Innovation Research Center, College of Animal Science and Technology, Hunan Agricultural University, Changsha, China
The purpose of this experiment was to investigate the effects of different protein levels on lipid metabolism and gut microbes in mice of different genders. A total of 60 mice (30 female and 30 male) were randomly assigned to six groups and fed female mice with low protein diet (FLP), basal protein diet (FBD), and high protein diet (FHP). Similarly, the male mice fed with low protein diet (MLP), basal protein diet (MBD), and high protein diet (MHP). The low protein diet contained 14% CP, the basal diet contained 20% CP, and the high protein diet contained 26% CP. The results of the study showed that both basal and high protein diets significantly reduced the perirenal adipose tissues (PEAT) index in male mice compared to low protein diet (p < 0.05). For the gut, the FHP significantly increased the relative gut weight compared to the FBD and FLP (p < 0.05). At the same time, the FHP also significantly increased the relative gut length compared with the FBD and FLP (p < 0.05). The MHP significantly increased TC concentration compared with the MLP (p < 0.05), and the MBD tended to increase TC concentration compared with the MLP in serum (p = 0.084). The histomorphology result of the jejunum and ileum showed that a low protein diet was beneficial to the digestion and absorption of nutrients in the small intestine of mice. While different protein levels had no effect on the total number of fecal microbial species in mice, different protein levels had a significant effect on certain fecal microbes in mice, the absolute abundance of Verrucomicrobia in the feces of male mice was significantly higher in both high and basal protein diets than in the low protein diet (p < 0.05). The high protein diet significantly reduced the absolute abundance of Patescibacteria in the feces of female mice compared to both the basal and low protein diets (p < 0.05). The absolute abundance of Patescibacteria in male feces was not affected by dietary protein levels (p > 0.05). Taken together, our results suggest that a low protein diet can alter fat deposition and lipid metabolism in mice, and that it benefited small intestinal epithelial structure and microbes.
Introduction
Nutrients are essential elements for maintaining health in all living organisms. Various nutrient regulatory systems exist between different organisms in order to correctly sense the nutrient environment in which they live (1). Normally, when a shortage/excess of each nutrient is detected in body tissues, some specific signaling pathways are activated, and then responsive metabolic responses are triggered (1). Protein is one of the most important nutrients, as nearly half of the dry weight of the mammalian body is made up of proteins with an incredible variety of biological functions (2). Obesity has become a major public health problem in China. In the most recent national survey, more than half of Chinese adults were overweight or obese, according to criteria for the Chinese population (3). Overweight and obesity were responsible for 11.1% of non-communicable disease (NCDs) related deaths in 2019, an increase of 5.7% from 1990 (4). These circumstances also lead to substantial health expenditures by countries to manage NCDs (5). While overweight and obesity are rapidly increasing, China has also undergone social, economic and environmental transformations. Lifestyle factors such as eating habits and physical activity have changed dramatically (6, 7). Weight loss is a major goal of reducing the risk of diabetes in humans (8). One study suggested a low-fat diet and energy restriction for obesity prevention and weight loss, but a high-protein diet is a popular alternative (9). Traditional weight loss strategies typically advise overweight and obese individuals to reduce fat intake to reduce energy intake by 500–750 calories (10). However, a high protein diet can firstly increase the body’s satiety and also increase energy expenditure, so it has become an alternative to energy restriction for weight loss (11–13).
It should be noted that the long-term effects of high protein diet, especially when combined with high fat diet, remain controversial (14). The results of systematic reviews and meta-analyses suggest that the long-term efficiency of high protein diet to induce weight loss in humans is uncertain unless dietary intervention is combined with energy restriction (15–17). While human trials have examined the ability of the high protein diet to induce weight loss in obese patients, the effects of the high protein diet in rodents were primarily conducted in preventing the development of obesity. In order to study the complex relationship between host and microbes in general, it is crucial to better understand the interactions between host and gut microbes. This can be achieved by measuring the molecules that contribute to this interaction, especially the metabolites formed by the microbiota that are available for uptake by the host. Gut microbes play key roles in animal health, including digestion of food, metabolism, regulation of immunity, and defense against invading pathogens (18–20). Many animal experiments have reported that high fat/high protein diet can effectively reduce or even prevent the development of obesity (21–26), but the ability of high protein diet to reverse obesity in rodents and its effects on fat metabolism and changes in gut microbes are far from clear. The current global obesity epidemic requires effective weight loss strategies in addition to effective methods to prevent weight gain.
The low protein diet is critical for addressing environmental concerns and conserving protein resources, but their impact on the gut microenvironment is not fully understood. Given the popularity of high protein diet, we here aimed to investigate whether diets with different protein levels can affect lipid metabolism in mice. Furthermore, we explored whether the improvement of lipid metabolism at different protein levels also affected gut microbes. Using mice as a model, it is possible to systematically study the importance of different protein levels on fat metabolism in mice, and to evaluate the possible harmful or beneficial effects of diets with different protein levels on intestinal tissue structure.
Materials and Methods
Animals and Dietary Treatments
The studies were approved by the Laboratory Animal Welfare and Animal Experimental Ethical Inspection Committee at the Guangxi University (Nanning, China).
Four-week-old male C57 mice (specific pathogen-free) were purchased from SLAC Laboratory Animal Central (Changsha, China). After a 1-week adaptation period, the mice were housed in a controlled environment (temperature: 25 ± 2°C, relative humidity: 45–60%, and a 12-h light–dark cycle), with free access to food and drinking water during the experiment. The diet of mice was mainly composed of corn, soybean meal, beer yeast, casein and lard. The low protein diet contained 14% CP, the basal diet contained 20% CP, and the high protein diet contained 26% CP. A total of 30 male mice of similar weight were randomly grouped into the low protein diet (MLP), basic diet (MBD), and high protein diet (MHP). Similarly, 30 female mice of similar weight were randomly grouped into the low protein diet (FLP), basic diet (FBD), and high protein diet (FHP). Throughout the experiment, the body weight of mice was measured weekly for 4 weeks. Fecal samples were collected and stored at –80°C until further analysis. At the end of the experiment, all mice were fasted overnight and killed by cervical dislocation with sodium pentobarbital anesthesia, and all efforts were made to minimize suffering. After killing, blood, subcutaneous adipose tissues (SAT), abdominal adipose tissues (AAT), perirenal adipose tissues (PEAT), liver, jejunum, ileum, and fecal contents were collected for further analyses.
Analysis of Biochemical Parameters in Blood Samples
Serum samples were separated after centrifugation at 3,000 rpm for 10 min at 4°C. An automatic biochemistry analyzer was used to test serum biochemical parameters (27), including total cholesterol (TC, 2021061K), high density lipoprotein (HDL, 2021061K), low density lipoprotein (LDL, 2021061K) and triglycerides (TG, 2021051K).
Histology Analysis
The jejunal and ileal tissues were removed and fixed in 4% formaldehyde solution, after which the fixed tissues were paraffin-embedded and the jejunal and ileal tissues blocks were cut into 5 μm sections, and stained with hematoxylin and eosin. In the present study, we used the pre-defined method which was reported to define the lesion (28). In each section, villus height (VH) and crypt depth (CD) were measured using a light microscope with a computer-assisted morphometric system. VH was defined as the distance from the villus tip to the crypt mouth, and CD from the crypt mouth to the base.
Microbiota Analysis
Total genome DNA from fecal samples was extracted for amplification using a specific primer with a barcode (16S V3–V4). Sequencing libraries were generated and analyzed according to our previous study (29).
Statistical Analyses
Statistical analyses between the means of each group were analyzed by using one-way ANOVA (one-way analysis of variance) followed by Duncan comparison range tests through SPSS 22.0. Statistical significance was set at p < 0.05.
Results
Body, Adipose Tissue, and Gut Weight or Length
As shown in Figure 1, the three different ratios of protein had no significant effect on the final body weight of male mice (p > 0.05). At the same time, the different levels of protein had no significant effect on body weight of female mice (p > 0.05). Although different ratios of protein had no significant effect on SAT and AAT indexes in female and male mice, both MBD and MHP significantly reduced the PEAT index compared to MLP (p < 0.05). There was no significant effect on liver index of female and male mice by protein ratios (p > 0.05). For the gut, the high protein diet significantly increased the relative gut weight of female mice compared to the basal and low protein diets (p < 0.05). At the same time, the high protein diet also significantly increased the relative gut length of female mice compared with the basal and low protein diets (p < 0.05). However, different levels of protein concentration diet had no significant effect on the relative weight and relative length of gut to male mice. Furthermore, there was no significant difference in gut length between female and male mice between diets with different protein levels (p > 0.05).
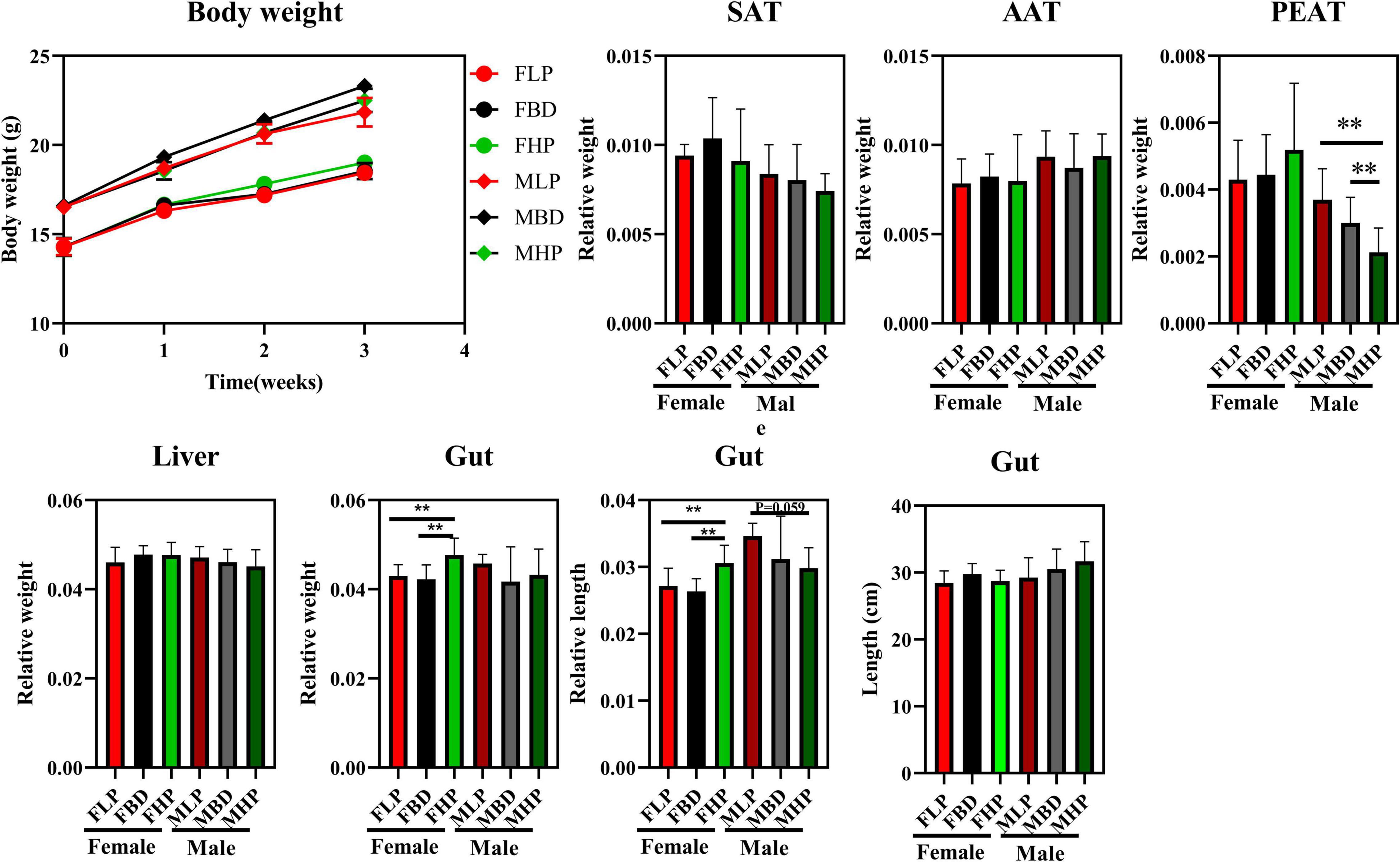
Figure 1. Effects of diets with different protein levels on body weight, lipid accumulation, liver weight and gut appearance in mice.
Lipid Metabolism Index in Serum
As shown in Figure 2, the FHP tended to increase serum TC concentration compared with the FLP (p = 0.083). However, the MHP significantly increased TC concentration compared with the MLP (p < 0.05), and the MBD tended to increase TC concentration compared with the MLP in serum (p = 0.084). Although diets with different protein levels in female mice had no significant effect on serum TG concentrations (p > 0.05), however, the MBD significantly increased the content of TG in serum compared with the MLP (p < 0.05). The FLP resulted in significantly higher serum HDL than the FBD and FHP (p < 0.05), whereas both the FHP and FBD significantly increased serum LDL level in female mice (p < 0.05). Different levels of protein diets did not significantly alter serum HDL and LDL levels in male mice (p > 0.05).
Histomorphological Analysis of Small Intestine
The jejunal tissue morphology under three different dietary treatments to female and male mice was shown in Figure 3. According to our study, jejunal villus length decreased with increasing dietary protein levels in both male and female mice, and a high protein diet resulted in a significantly lower jejunal villus length than a low protein diet (p < 0.05). In female mice, both the basal diet and the high protein diet significantly increased CD in the jejunum (p < 0.05), while the three dietary treatments had no effect on CD in male mice (p > 0.05). With the increase of dietary protein level, the ratio of jejunal villus length to CD (L/D) decreased in female mice, and the L/D ratio of basal diet and high protein diet was significantly lower than that of low protein diet (p < 0.05). The results for male mice showed that increased protein levels led to a decrease in jejunal L/D, but the difference was not significant (p > 0.05). The jejunal villus width of the FLP was significantly lower than that of the FBD (p < 0.001) and the FBD was significantly higher than that of the FHP (p < 0.05), and the protein concentration had no effect on the villus width of the male mice (p > 0.05). The number of jejunal goblet cells on the FBD was significantly lower than that on the FLP and FHP (p < 0.05), and the number of jejunal goblet cells in male mice also tended to be higher on the low protein diet than on the basal diet (p = 0.082). The high protein diet resulted in significantly lower jejunal villus area in female mice than on the basal diet (p < 0.05), while the high protein diet in male mice resulted in significantly lower jejunal villus area than the low protein diet (p < 0.05).
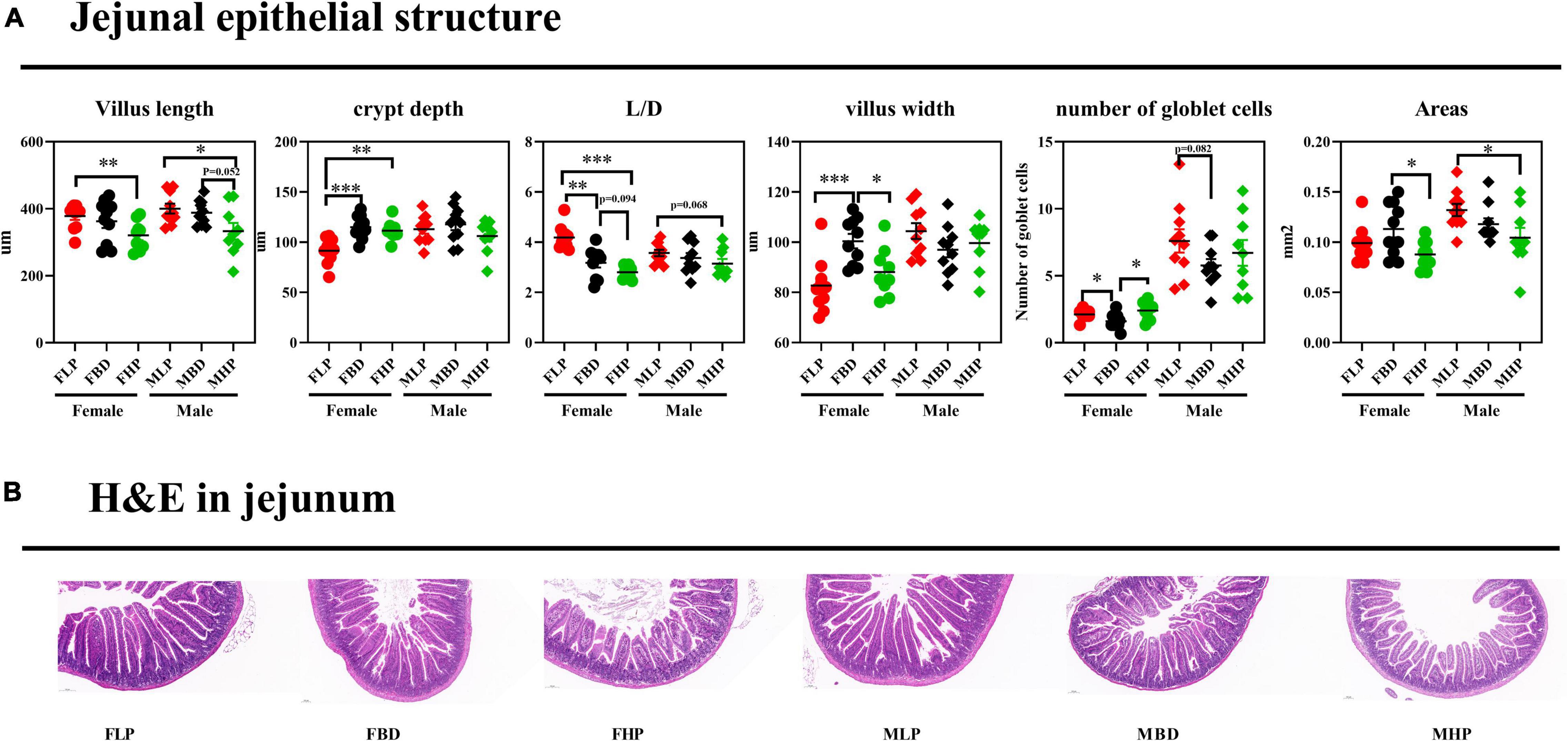
Figure 3. Effects of diets with different protein levels on the morphology of jejunal epithelial tissue in mice. (A) The structure of the jejunal epithelial tissue. (B) Light microscopy cross-section of jejunal tissue.
The ileal tissue morphology under three different dietary treatments to female and male mice was shown in Figure 4. In this study, the ileal villus length significantly decreased in high protein diet than low protein diet for male mice (p < 0.05), and three protein diets had no significantly difference on villus length of female mice (p > 0.05). In female mice, the FBD significantly increased CD in the ileum compared with the FLP (p < 0.05), while the MBD tended to decreased CD than MLP in the ileum of male mice (p = 0.079). With the increase of dietary protein level, the ratio of ileal L/D decreased in female mice, and the L/D ratio of basal diet and high protein diet was significantly lower than that of low protein diet (p < 0.05). The results for male mice showed that various protein level diets had no significant difference on ileal L/D (p > 0.05). The ileal villus width of the MBD was significantly higher than that of the MLP (p < 0.05), and the MBD tended to increase the ileal villus width than that of the MHP in male mice (p = 0.054). The protein concentration had no effect on the ileal villus width of the female mice (p > 0.05). The number of ileal goblet cells on the FBD was significantly higher than that on the FLP (p < 0.05), and the number of ileal goblet cells in male mice had no significant difference between the low and high protein diet in female mice (p > 0.05). Meanwhile, different levels of protein diet had no effect on the number of goblet cells in the ileum of male mice (p > 0.05). Finally, different levels of protein diet had no significant effect on ileal villus area in both female and male mice (p > 0.05).
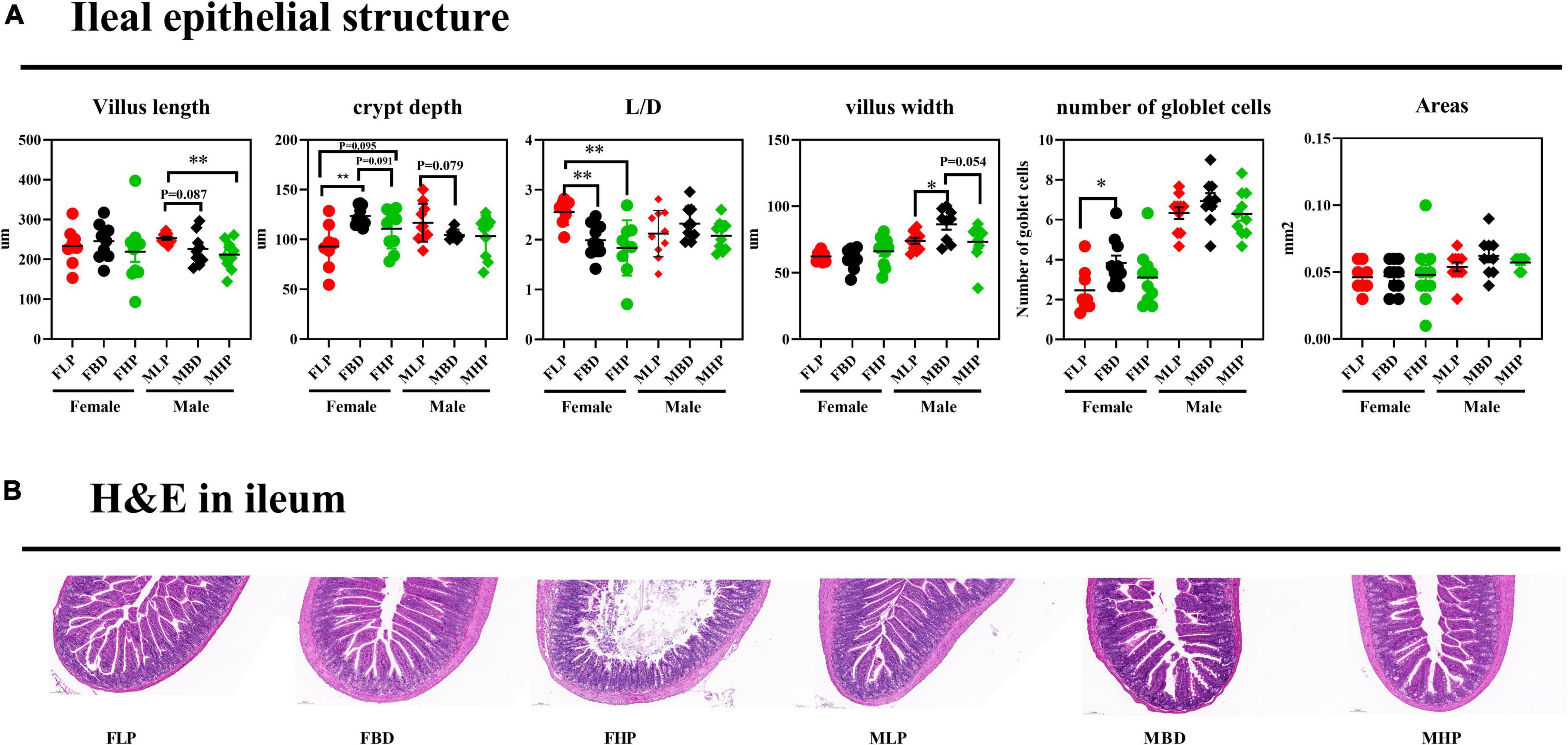
Figure 4. Effects of diets with different protein levels on the morphology of ileal epithelial tissue in mice. (A) The structure of the ileal epithelial tissue. (B) Light microscopy cross-section of ileal tissue.
Fecal Bacterial α and β Diversity
The fecal bacterial α diversity in male and female mice with different levels of protein diets was shown in Figure 5A. The results showed that the FBD tended to reduce observed species compared to the FLP (p = 0.068), while no significant difference was found between the high protein diet and the other two groups (p > 0.05). There were no significant differences in fecal observed species in male mice fed the diets of the three protein levels (p > 0.05). The low protein diet significantly increased the Shannon index compared with the basal diet in female mice (p < 0.05), while the high protein diet did not produce significant differences with the other two groups on Shannon index in female mice (p > 0.05). In the fecal bacteria of male mice, the MLP significantly increased the Shannon index of bacteria compared to the MBD (p < 0.05), while the MHP showed a trend to increase the Shannon index compared to the MBD (p = 0.055). The high protein diet significantly improved Simpson index over basal diet in female mice (p < 0.05), whereas high protein diets over basal diets tended to increase Simpson index in male mice (p = 0.051). At the same time, the low protein diet also significantly improved the Simpson index in male mice compared to the basal diet (p < 0.05). There were no significant differences in the fecal bacterial chao1 index and PD_whole_tree between the three diets in either female or male mice (p > 0.05). As shown in Figure 5B, the principal components of female mice were similar between the different protein levels and did not produce significant separation. In male mice, however, there was a distinctly different cluster of microbiomes between the basal and high protein diets.
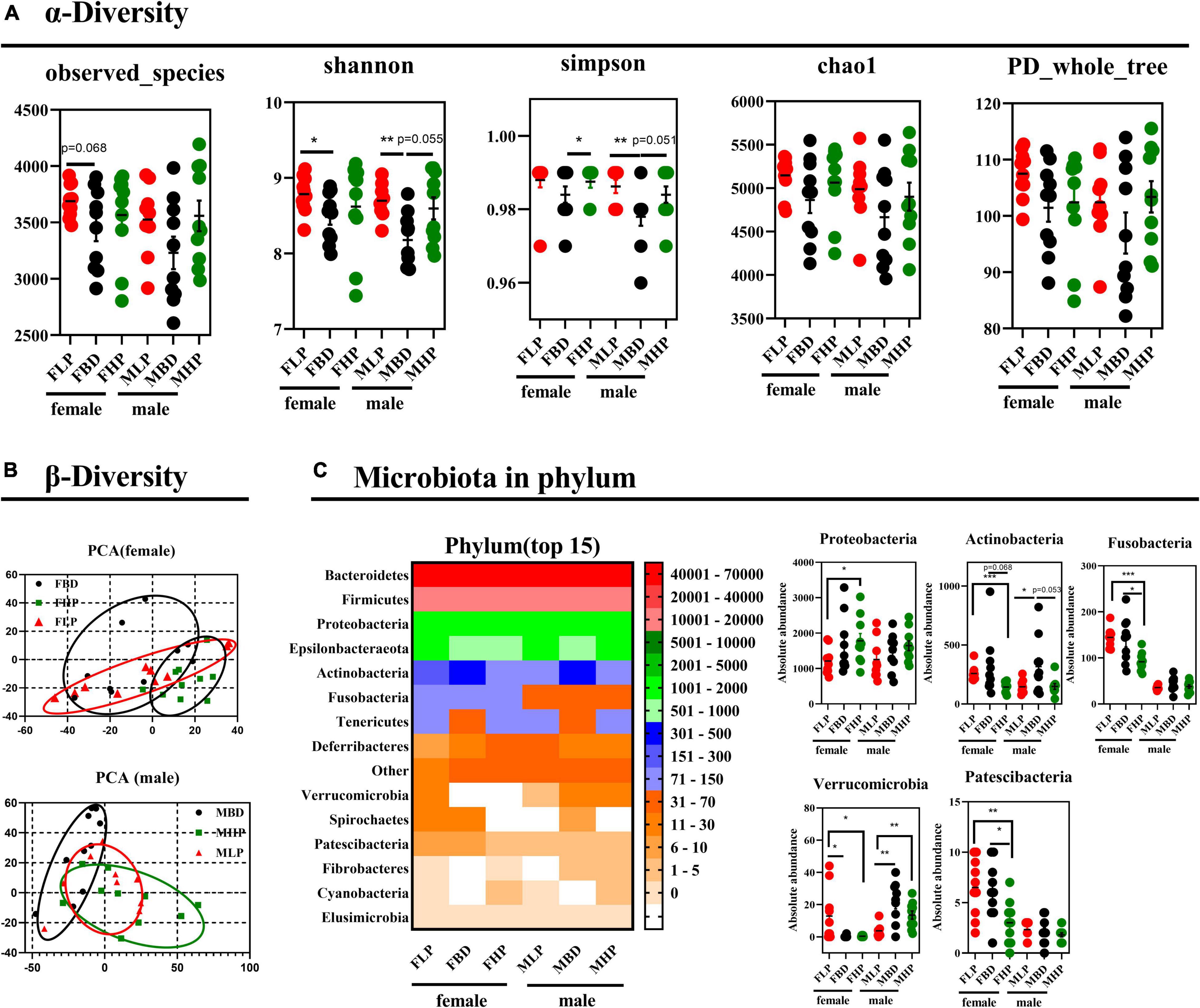
Figure 5. Effects of diets with different protein levels on (A) α diversity, (B) β diversity, and (C) phylum-level bacteria in the fecal microbiota of mice.
Diets With Different Protein Levels Affect Fecal Microbiota Composition
The bacterial effects of different protein levels on the top 15 bacteria at the phylum level of the fecal microbiota of male and female mice were shown in Figure 5C. The high protein diet significantly increased the absolute abundance of Proteobacteria in the feces of female mice compared to the low protein diet (p < 0.05). The abundance of Proteobacteria did not make a difference in male feces (p > 0.05). In contrast, the high protein diet significantly reduced the absolute abundance of Actinobacteria in the feces of female mice compared to the low protein diet (p < 0.05). The abundance of Actinobacteria in the feces of female mice tended to decrease in the high protein diet compared with the basal protein diet (p = 0.068). Furthermore, the high protein diet significantly reduced the absolute abundance of Fusobacteria in the feces of female mice compared to the low and basal protein diet (p < 0.05). The absolute abundance of Fusobacteria in male mice was not affected by dietary protein levels (p > 0.05). In addition, both high protein and basal protein diets significantly reduced the absolute abundance of Verrucomicrobia in the feces of female mice compared to low protein diets (p < 0.05). Conversely, the absolute abundance of Verrucomicrobia in the feces of male mice was significantly higher in both high and basal protein diets than in the low protein diet (p < 0.05). The high protein diet significantly reduced the absolute abundance of Patescibacteria in the feces of female mice compared to both the basal and low protein diets (p < 0.05). The absolute abundance of Patescibacteria in male feces was not affected by dietary protein levels (p > 0.05).
Downward to species level (Figure 6), we found that 17 out of 30 species were markedly changed throughout the entire stage. The high protein diet of female mice significantly increased the absolute abundances of Burkholderiales_bacterium_YL45 and Culturomica_massiliensis (p < 0.05), but significantly decreased the absolute abundances of Lachnospiraceae_bacterium_28-4, Clostridiales_bacterium_CIEAF_020, Streptococcus_pneumoniae and Campylobacter_showae_CC57C compared with the basal diet (p < 0.05). At the same time, the abundance of Azospirillum_sp._47_25 has an upward trend in the high protein diet compared with the basal diet in the feces of female mice (p = 0.069). However, the abundances of Lactobacillus_murinus, Bacteroides_massiliensis_B84634_, Clostridium_sp._ASF502, Lactobacillus_plantarum and Brochothrix_thermosphacta in female mice feces were not affected by protein levels (p > 0.05). In the fecal microbiota of male mice, a high protein diet significantly increased the absolute abundances of Lactobacillus_plantarum and Brochothrix_thermosphacta (p < 0.05), but significantly decreased the absolute abundances of Clostridium_sp._ASF502 and Clostridium_sp._Culture_Jar-19 compared with a low protein diet (p < 0.05). In addition, the high protein diet tended to increase Clostridium_sp._Culture_Jar-19 abundance in male mice feces compared to basal protein diet (p = 0.064). And in male mice fecal microbiota, the absolute abundances of Burkholderiales_bacterium_ YL45, Lachnospiraceae_bacterium_28-4, Lactobacillus_reuteri, Bacteroides_thetaiotaomicron, Clostridiales_bacterium_CIEAF_ 020, Culturomica_massiliensis, Lactobacillus_iners_AB-1, Azospirillum_sp._47_25, Streptococcus_pneumoniae, Campy-lobacter_ showae_CC57C, and Bacteroides_ovatus_V975 were not affected by dietary protein levels (p > 0.05).
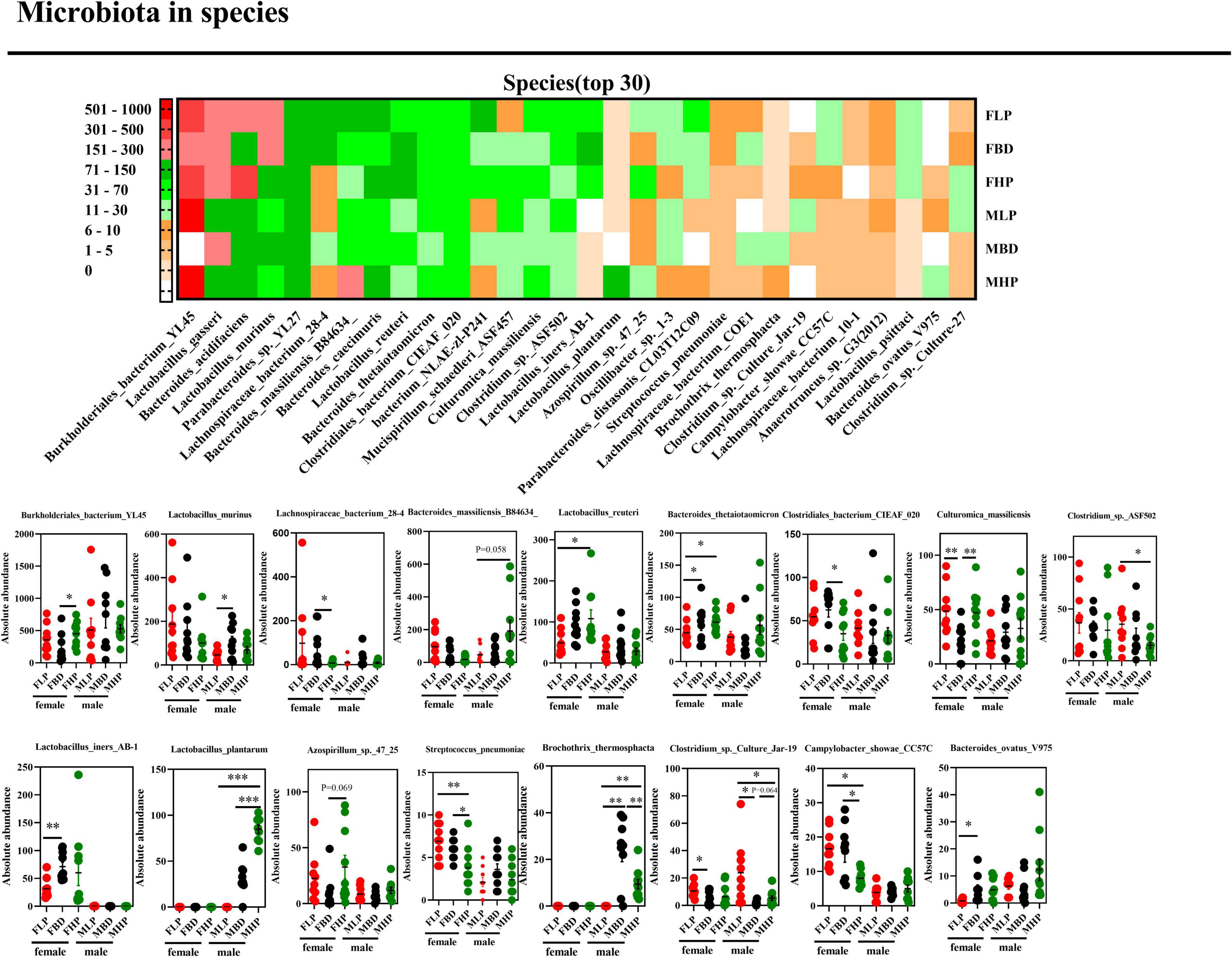
Figure 6. Effects of diets with different protein levels on species-level bacteria in the fecal microbiota of mice.
Discussion
In this study, female and male mice were used as experimental models to investigate the effects of different levels of protein diets on fat deposition, fecal microbiota, and small intestinal tissue structure in mice. Studies have shown that dietary protein played a role in regulating lipid biological synthesis. In some rodents’ research, genes participating in new fat production and lipid storage such as PPARγ (21), ACC (21, 30), FASN (21, 30), SCD1 (31), and SREBP1c (31, 32) were reduced by intake of HP diet. Compared with LP diet, the HP diet fat intake and lipid biological synthetic genes were lower. The HP diet reduces liver fat more effectively than the LP diet (33). For example, in mice and human research, the HP diet has proven to increase energy consumption, reduce blood glucose levels, promote fat oxidation, thereby supporting weight loss, and reducing liver fat (34–37). The HP diet may inhibit liver fat production at the molecular level to reduce liver fat (21, 22, 38). The relatively lower carbohydrate content in the HP diet may also be part of the cause of new fat production and reduced intrahepatic fat. In addition, compared with fat-generating genes, in several rats’ research, dietary protein intake has not changed the expression of genes related to lipid oxidation or substrate oxidation (30, 32). Our results showed that the HP diet is significantly reduced in the perirenal fat more than the LP and the basic diet. The reason may be that the fat intake and lipid biological synthesis may be inhibited.
The biochemical indicators of the body’s blood can not only feedback the health of the host and the strength of immune function, but also reveal the biological characteristics of different hosts (39). When the level of TC in the host’s blood rises, hypertrophymia will occur. Compared with LDL, HDL level may lead to this situation (40). Some researchers have compared the influence of LP and HP diets on obesity and their related diseases. One of the main discoveries in the system summary was that the HP solution has a favorable impact on TG and HDL (16). This was consistent with the results of the HP diet in our research reduced the HDL in the female blood. The HP diet reduced TC and HDL, but LDL has increased, indicating that the lipid metabolism in the blood has changed. These data are very important for preventing hyperlipidemia and heart and liver disease (41).
Different parts of the digestive tract assume different responsibilities. The small intestine was the key place for the host to absorb nutrients. The height of villi and the depth of crypts in the small intestine were important indicators to evaluate its digestion and absorption function. The CD reflects the speed of cell formation, while shallower crypts indicate that the cell maturity was accelerated and the secretion function is enhanced. The VH and CD could fully reflect the functional state of the small intestine (42). In the present study, jejunal villus length decreased and CD increased after mice were fed the HP diet, suggesting that the HP diet may have a potential adverse effect on jejunal function. Since the small intestine surface area (represented by the tight villus packing and long villus protrusions) showed the maximum nutrient absorption allowed (43), the reduced ratio of VH to CD in the jejunum and ileum of the HP group diet indicated nutrient absorption reduce. Crypts were where ISCs were synthesized and amplifying cells were transported, and CD could also reflect the proliferation of intestinal epithelial cells. In the present study, an increase in jejunal CD with increasing protein levels in the diet showed the possibility of intestinal epithelial proliferation, whereas an increase in epithelial proliferation with increasing protein levels in the ileum occurred only in the FLP and FBD groups. The intestinal epithelial barrier in animals was initiated by the ISC niche, which develops differentiated cell types, including Paneth cells and goblet cells (44). The increase in goblet cells in the FBD group indicated the possibility of ISC proliferation in the ileum. Thus, the microbiota in the FBD group than in the FLP group may have stimulated ISCs, improved ileal barrier function, and was supported by an increase in Lactobacillus, which has been reported to stimulate intestinal epithelial cell proliferation through Nox-mediated reactive oxygen species production (45).
Dietary protein was an essential nutrient for animals and it was necessary for the physiological functions of organs. The structural integrity of the gut and the homeostasis of the gut microbiota ensure the chemical induction and digestive functions of the gut, which were prerequisites for nutrient absorption, metabolism, and deposition. The gut microbiota was considered a key factor in maintaining gut function (46, 47), it was a complex ecosystem with nearly 100 trillion microorganisms, most of which were bacteria (48). Many factors influenced gut microbiota composition and activity, including diet, environment, and age; of these, diet was the most important (49–51). Diet played a crucial role in shaping the composition of animal gut microbiota and the state of immune responses mediated by gut microbiota (52). We explored the effect of diets with different protein levels on fecal microbial diversity in mice. In the present study, it can be concluded that the fecal community diversity of mice on a low protein diet was higher than that on a basal diet and a high protein diet, but it was observed that different protein levels had no effect on the total fecal microbial population in mice. The microbiome consists of trillions of microbial cells with high inter- and intra-species variability, so it was difficult to define a healthy gut microbiome based on the species in the gut (53, 54). However, the variability of microbial functional genes and metabolites may be low (54, 55). An increased ratio of Firmicutes to Bacteroides has been reported in obese animals (56) and was associated with host energy intake (57, 58). Some researchers have shown that the composition of different microbial communities in the digestive tract of animals was different, and the diversity and density of microbial communities gradually increased from the stomach to the hindgut (59). For the ileal microbiota, the reduced Enterobacteriaceae within the Proteobacteria phylum were thought to contain many pathogenic bacteria when dietary protein decreased by 3 percentage points (60), suggesting the potential for pathogen suppression by moderate dietary protein restriction. The Streptococcus and Escherichia-Shigella can cause a variety of infections and diseases, such as scarlet fever and bacillary dysentery (61, 62). In this study, different protein level diets had no difference in Escherichia-Shigella of female and male mice. Research by NEIS et al. showed that when the concentration of diet protein is reduced by 3 percentage points, the number of Streptococcus and Escherichia-Shigella declined in the ileum, and the Streptococcus participated in the use of amino acids (63). Therefore, the decline of link bacteria such as Streptococcus_pneumoniae in feces of FHP diet, it can be explained as insufficient protein substrates required for fermentation, which was consistent with previous research (64, 65). The Lactobacillus bacteria in the intestine could ferment the carbohydrates in the diet into lactic acid and improved the intestinal environment (66, 67). The elevation of Lactobacillus_plantarum and Lactobacillus_reuteri in the high protein diet in this study fitted this situation. An increasing on Lactobacillus_murinus and Lactobacillus_iners_AB-1 in the basal diet compared to the low protein diet may be because the carbohydrates in the basal diet were higher than the low protein diet, but the two bacteria were decreased in the high protein diet. It may be that the high protein diet weakened the intestinal barrier function. Chen et al. used pigs as an experimental model to show that the abundance of Lachnospiraceae, which was saccharolytic and can degrade cellulose, was increased in low protein diet (68). This was consistent with our findings that the high protein diet reduced Lachnospiraceae_bacterium_28-4 in the feces of female mice compared to the basal diet. Therefore, according to the results of this study, a low protein diet was more beneficial to the gut microbiome.
Conclusion
In conclusion, this study demonstrated that diets with different protein levels can affect lipid deposition and lipid metabolism in mice. At the same time, a high protein diet weakened the small intestinal barrier structure, which weakened the host’s ability to digest and absorb nutrients. Although different protein levels had no effect on the total number of fecal microbial species in mice, different protein levels had significant effects on the structure of some fecal microbiota in mice. The reduction of Lactobacillus_murinus and Lactobacillus_iners_AB-1 on the low protein diet due to higher carbohydrates in the basal diet than the low protein diet. Besides, the high protein diet reduced Lachnospiraceae_bacterium_28-4 in the feces of female mice compared to the basal diet. Our study provided a more comprehensive understanding of lipid metabolism, gut barrier and fecal microbial response to diets at different protein levels in female and male mice.
Data Availability Statement
The original contributions presented in the study are included in the article/supplementary material, further inquiries can be directed to the corresponding author.
Ethics Statement
The studies were approved by the Laboratory Animal Welfare and Animal Experimental Ethical Inspection Committee at the Guangxi University (Nanning, China).
Author Contributions
KW and HS designed the experiment. KW conducted the experiment and wrote the manuscript. KW, XP, AY, YH, YT, YQ, and FL collected and analyzed the data. HS revised the manuscript. All authors contributed to the article and approved the submitted version.
Funding
This research was supported by a grant from the Science and Technology Major Project of Guangxi (China) (No. AA17204057) and the Key Research and Development Plan of Guangxi (China) (No. AB19245037).
Conflict of Interest
The authors declare that the research was conducted in the absence of any commercial or financial relationships that could be construed as a potential conflict of interest.
Publisher’s Note
All claims expressed in this article are solely those of the authors and do not necessarily represent those of their affiliated organizations, or those of the publisher, the editors and the reviewers. Any product that may be evaluated in this article, or claim that may be made by its manufacturer, is not guaranteed or endorsed by the publisher.
References
1. Chantranupong L, Wolfson RL, Sabatini DM. Nutrient-sensing mechanisms across evolution. Cell. (2015) 161:67–83. doi: 10.1016/j.cell.2015.02.041
2. Forbes RM, Cooper AR, Mitchell HH. The composition of the adult human body as determined by chemical analysis. J Biol Chem. (1953) 203:359–66.
3. The State Council Information Office of the People’s Republic of China.Press briefing for the Report on Chinese Residents’ Chronic Diseases and Nutrition 2020. (2020). Available online at: http://www.gov.cn/xinwen/2020-12/24/content_5572983.htm (accessed December 26, 2020).
4. Institute for Health Metrics and Evaluation.Global Health Data Exchange. GBD Results Tool. (2021). Available online at: http://ghdx.healthdata.org/gbd-results-tool (accessed January 10, 2021).
5. Qin X, Pan J. The medical cost attributable to obesity and overweight in China: estimation based on longitudinal surveys. Health Econom. (2016) 25:1291–311. doi: 10.1002/hec.3217
6. Popkin BM. Synthesis and implications: China’s nutrition transition in the context of changes across other low- and middle-income countries. Obes Rev. (2014) 15:60–7. doi: 10.1111/obr.12120
7. Du SF, Wang HJ, Zhang B, Zhai FY, Popkin BM. China in the period of transition from scarcity and extensive undernutrition to emerging nutrition-related non-communicable diseases, 1949-1992. Obes Rev. (2014) 15:8–15. doi: 10.1111/obr.12122
8. Hamman RF, Wing RR, Edelstein SL, Lachin JM, Bray GA, Delahanty L, et al. Effect of weight loss with lifestyle intervention on risk of diabetes. Diab Care. (2006) 29:2102–7. doi: 10.2337/dc06-0560
9. Myrmel LS, Fauske KR, Fjære E, Bernhard A, Liisberg U, Hasselberg AE, et al. The impact of different animal-derived protein sources on adiposity and glucose homeostasis during ad libitum feeding and energy restriction in already obese mice. Nutrients. (2019) 11:11051153. doi: 10.3390/nu11051153
10. Koliaki C, Spinos T, Spinou M, Brinia M E, Mitsopoulou D, Katsilambros N. Defining the optimal dietary approach for safe, effective and sustainable weight loss in overweight and obese adults. Healthcare. (2018) 6:73. doi: 10.3390/healthcare6030073
11. Madsen L, Myrmel LS, Fjære E, Øyen J, Kristiansen K. Dietary proteins, brown fat, and adiposity. Front Physiol. (2018) 9:1792. doi: 10.3389/fphys.2018.01792
12. Pesta DH, Samuel VT. A high-protein diet for reducing body fat: mechanisms and possible caveats. Nutrit Metabol. (2014) 11:53. doi: 10.1186/1743-7075-11-53
13. Westerterp-Plantenga MS. Protein intake and energy balance. Regulat Peptides. (2008) 149:67–9. doi: 10.1016/j.regpep.2007.08.026
14. Cuenca-Sánchez M, Navas-Carrillo D, Orenes-Piñero E. Controversies surrounding high-protein diet intake: satiating effect and kidney and bone health. Adv Nutrit. (2015) 6:260–6. doi: 10.3945/an.114.007716
15. Lepe M, Bacardí Gascón M, Jiménez Cruz A. Long-term efficacy of high-protein diets: a systematic review. Nutric Hospital. (2011) 26:1256–9. doi: 10.1590/s0212-16112011000600010
16. Santesso N, Akl EA, Bianchi M, Mente A, Mustafa R, Heels-Ansdell D, et al. Effects of higher-versus lower-protein diets on health outcomes: a systematic review and meta-analysis. Eur J Clin Nutrit. (2012) 66:780–8. doi: 10.1038/ejcn.2012.37
17. Wycherley TP, Moran LJ, Clifton PM, Noakes M, Brinkworth GD. Effects of energy-restricted high-protein, low-fat compared with standard-protein, low-fat diets: a meta-analysis of randomized controlled trials. Am J Clin Nutrit. (2012) 96:1281–98. doi: 10.3945/ajcn.112.044321
18. Wang K, Peng X, Lv F, Zheng M, Long D, Mao H, et al. Microbiome-metabolites analysis reveals unhealthy alterations in the gut microbiota but improved meat quality with a high-rice diet challenge in a small ruminant model. Animals. (2021) 11:2306. doi: 10.3390/ani11082306
19. Sun J, Wang K, Xu B, Peng X, Chai B, Nong S, et al. Use of hydrolyzed Chinese gallnut tannic acid in weaned piglets as an alternative to zinc oxide: overview on the gut microbiota. Animals. (2021) 11:2000. doi: 10.3390/ani11072000
20. Cani PD, Delzenne NM. The role of the gut microbiota in energy metabolism and metabolic disease. Curr Pharmaceut Design. (2009) 15:1546–58. doi: 10.2174/138161209788168164
21. Freudenberg A, Petzke KJ, Klaus S. Comparison of high-protein diets and leucine supplementation in the prevention of metabolic syndrome and related disorders in mice. J Nutrit Biochem. (2012) 23:1524–30. doi: 10.1016/j.jnutbio.2011.10.005
22. Freudenberg A, Petzke KJ, Klaus S. Dietary l-leucine and l-alanine supplementation have similar acute effects in the prevention of high-fat diet-induced obesity. Amino Acids. (2013) 44:519–28. doi: 10.1007/s00726-012-1363-2
23. Kiilerich P, Myrmel LS, Fjære E, Hao Q, Hugenholtz F, Sonne SB, et al. Effect of a long-term high-protein diet on survival, obesity development, and gut microbiota in mice. Am J Physiol Endocrinol Metab. (2016) 310:E886–99. doi: 10.1152/ajpendo.00363.2015
24. Marsset-Baglieri A, Fromentin G, Tomé D, Bensaid A, Makkarios L, Even PC. Increasing the protein content in a carbohydrate-free diet enhances fat loss during 35% but not 75% energy restriction in rats. J Nutrit. (2004) 134:2646–52. doi: 10.1093/jn/134.10.2646
25. McAllan L, Skuse P, Cotter PD, O’Connor P, Cryan JF, Ross RP, et al. Protein quality and the protein to carbohydrate ratio within a high fat diet influences energy balance and the gut microbiota in C57BL/6J mice. PLoS One. (2014) 9:e88904. doi: 10.1371/journal.pone.0088904
26. Pichon L, Huneau JF, Fromentin G, Tomé D. A high-protein, high-fat, carbohydrate-free diet reduces energy intake, hepatic lipogenesis, and adiposity in rats. J Nutrit. (2006) 136:1256–60. doi: 10.1093/jn/136.5.1256
27. Gao J, Ma L, Yin J, Liu G, Ma J, Xia S, et al. Camellia (Camellia oleifera bel.) seed oil reprograms gut microbiota and alleviates lipid accumulation in high fat-fed mice through the mTOR pathway. Food Funct. (2022) 13:4977–92. doi: 10.1039/d1fo04075h
28. Wang KJ, Yan QX, Ren A, Zheng ML, Zhang PH, Tan ZL, et al. Novel linkages between bacterial composition of hindgut and host metabolic responses to sara induced by high-paddy diet in young goats. Front Vet Sci. (2022) 8:791482. doi: 10.3389/fvets.2021.791482
29. Yin J, Han H, Li Y, Liu Z, Zhao Y, Fang R, et al. Lysine restriction affects feed intake and amino acid metabolism via gut microbiome in piglets. Cell Physiol Biochem. (2017) 44:1749–61. doi: 10.1159/000485782
30. Stepien M, Gaudichon C, Fromentin G, Even P, Tomé D, Azzout-Marniche D. Increasing protein at the expense of carbohydrate in the diet down-regulates glucose utilization as glucose sparing effect in rats. PLoS One. (2011) 6:e14664. doi: 10.1371/journal.pone.0014664
31. Noguchi Y, Nishikata N, Shikata N, Kimura Y, Aleman JO, Young JD, et al. Ketogenic essential amino acids modulate lipid synthetic pathways and prevent hepatic steatosis in mice. PLoS One. (2010) 5:e12057. doi: 10.1371/journal.pone.0012057
32. Chaumontet C, Even PC, Schwarz J, Simonin-Foucault A, Piedcoq J, Fromentin G, et al. High dietary protein decreases fat deposition induced by high-fat and high-sucrose diet in rats. Br J Nutrit. (2015) 114:1132–42. doi: 10.1017/s000711451500238x
33. Xu C, Markova M, Seebeck N, Loft A, Hornemann S, Gantert T, et al. High-protein diet more effectively reduces hepatic fat than low-protein diet despite lower autophagy and FGF21 levels. Liver Int. (2020) 40:2982–97. doi: 10.1111/liv.14596
34. Markova M, Pivovarova O, Hornemann S, Sucher S, Frahnow T, Wegner K, et al. Isocaloric diets high in animal or plant protein reduce liver fat and inflammation in individuals with type 2 diabetes. Gastroenterology. (2017) 152:571.e–85.e. doi: 10.1053/j.gastro.2016.10.007
35. Benjaminov O, Beglaibter N, Gindy L, Spivak H, Singer P, Wienberg M, et al. The effect of a low-carbohydrate diet on the nonalcoholic fatty liver in morbidly obese patients before bariatric surgery. Surg Endosc. (2007) 21:1423–7. doi: 10.1007/s00464-006-9182-8
36. Bortolotti M, Maiolo E, Corazza M, Van Dijke E, Schneiter P, Boss A, et al. Effects of a whey protein supplementation on intrahepatocellular lipids in obese female patients. Clin Nutrit. (2011) 30:494–8. doi: 10.1016/j.clnu.2011.01.006
37. Arslanow A, Teutsch M, Walle H, Grünhage F, Lammert F, Stokes CS. Short-term hypocaloric high-fiber and high-protein diet improves hepatic steatosis assessed by controlled attenuation parameter. Clin Transl Gastroenterol. (2016) 7:e176. doi: 10.1038/ctg.2016.28
38. Petzke KJ, Freudenberg A, Klaus S. Beyond the role of dietary protein and amino acids in the prevention of diet-induced obesity. Int J Mol Sci. (2014) 15:1374–91. doi: 10.3390/ijms15011374
39. Chen C, Yang B, Zeng Z, Yang H, Liu C, Ren J, et al. Genetic dissection of blood lipid traits by integrating genome-wide association study and gene expression profiling in a porcine model. BMC Genom. (2013) 14:848. doi: 10.1186/1471-2164-14-848
40. Abd El-Gawad IA, El-Sayed EM, Hafez SA, El-Zeini HM, Saleh FA. The hypocholesterolaemic effect of milk yoghurt and soy-yoghurt containing bifidobacteria in rats fed on a cholesterol-enriched diet. Int Dairy J. (2005) 15:37–44. doi: 10.1016/j.idairyj.2004.06.001
41. Abdel-Maksoud M, Sazonov V, Gutkin SW, Hokanson JE. Effects of modifying triglycerides and triglyceride-rich lipoproteins on cardiovascular outcomes. J Cardiovasc Pharmacol. (2008) 51:331–51. doi: 10.1097/FJC.0b013e318165e2e7
42. Rieger J, Janczyk P, Hünigen H, Neumann K, Plendl J. Intraepithelial lymphocyte numbers and histomorphological parameters in the porcine gut after Enterococcus faecium NCIMB 10415 feeding in a Salmonella Typhimurium challenge. Vet Immunol Immunopathol. (2015) 164:40–50. doi: 10.1016/j.vetimm.2014.12.013
43. Shyer AE, Huycke TR, Lee CH, Mahadevan L, Tabin CJ. Bending gradients: how the intestinal stem cell gets its home. Cell. (2015) 161:569–80. doi: 10.1016/j.cell.2015.03.041
44. Peterson LW, Artis D. Intestinal epithelial cells: regulators of barrier function and immune homeostasis. Nat Rev Immunol. (2014) 14:141–53. doi: 10.1038/nri3608
45. Jones RM, Luo L, Ardita CS, Richardson AN, Kwon YM, Mercante JW, et al. Symbiotic lactobacilli stimulate gut epithelial proliferation via Nox-mediated generation of reactive oxygen species. Embo J. (2013) 32:3017–28. doi: 10.1038/emboj.2013.224
46. Sczesnak A, Segata N, Qin X, Gevers D, Petrosino JF, Huttenhower C, et al. The genome of Th17 cell-inducing segmented filamentous bacteria reveals extensive auxotrophy and adaptations to the intestinal environment. Cell Host Microbe. (2011) 10:260–72. doi: 10.1016/j.chom.2011.08.005
47. Chen J, Li Y, Tian Y, Huang C, Li D, Zhong Q, et al. interaction between microbes and host intestinal health: modulation by dietary nutrients and gut-brain-endocrine-immune axis. Curr Protein Pept Sci. (2015) 16:592–603. doi: 10.2174/1389203716666150630135720
48. Collins SM, Surette M, Bercik P. The interplay between the intestinal microbiota and the brain. Nat Rev Microbiol. (2012) 10:735–42. doi: 10.1038/nrmicro2876
49. Fan P, Li L, Rezaei A, Eslamfam S, Che D, Ma X. Metabolites of dietary protein and peptides by intestinal microbes and their impacts on gut. Curr Protein Pept Sci. (2015) 16:646–54. doi: 10.2174/1389203716666150630133657
50. Sonnenburg ED, Smits SA, Tikhonov M, Higginbottom SK, Wingreen NS, Sonnenburg JL. Diet-induced extinctions in the gut microbiota compound over generations. Nature. (2016) 529:212–5. doi: 10.1038/nature16504
51. Ma N, Tian Y, Wu Y, Ma X. Contributions of the interaction between dietary protein and gut microbiota to intestinal health. Curr Protein Pept Sci. (2017) 18:795–808. doi: 10.2174/1389203718666170216153505
52. Saresella M, Mendozzi L, Rossi V, Mazzali F, Piancone F, LaRosa F, et al. Immunological and clinical effect of diet modulation of the gut microbiome in multiple sclerosis patients: a pilot study. Front Immunol. (2017) 8:1391. doi: 10.3389/fimmu.2017.01391
53. Nguyen TL, Vieira-Silva S, Liston A, Raes J. How informative is the mouse for human gut microbiota research? Dis Models Mechanisms. (2015) 8:1–16. doi: 10.1242/dmm.017400
54. Sender R, Fuchs S, Milo R. Revised estimates for the number of human and bacteria cells in the body. PLoS Biol. (2016) 14:e1002533. doi: 10.1371/journal.pbio.1002533
55. McCoy KD, Geuking MB, Ronchi F. Gut microbiome standardization in control and experimental mice. Curr Protoc Immunol. (2017) 117:21–3. doi: 10.1002/cpim.25
56. Bäckhed F, Ding H, Wang T, Lora VH, Gou YK, Nagy A, et al. The gut microbiota as an environmental factor that regulates fat storage. PNAS. (2004) 101:15718–23. doi: 10.1073/pnas.0407076101
57. Cottenie K. Integrating environmental and spatial processes in ecological community dynamics. Ecol Lett. (2005) 8:1175–82. doi: 10.1111/j.1461-0248.2005.00820.x
58. Ley RE, Turnbaugh PJ, Lein SK, Gordon JI. Human gut microbes associated with obesity. Nature. (2006) 444:1022–3. doi: 10.1038/4441022a
59. Hooper LV, Wong MH, Thelin A, Hansson L, Falk PG, Gordon JI. Molecular analysis of commensal host-microbial relationships in the intestine. Science. (2001) 291:881–4. doi: 10.1126/science.291.5505.881
60. Lv LX, Fang DQ, Shi D, Chen DY, Yan R, Zhu YX, et al. Alterations and correlations of the gut microbiome, metabolism and immunity in patients with primary biliary cirrhosis. Environ Microbiol. (2016) 18:2272–86. doi: 10.1111/1462-2920.13401
61. Davies MR, Holden MT, Coupland P, Chen JHK, Venturini C, Barnett TC, et al. Emergence of scarlet fever Streptococcus pyogenes emm12 clones in Hong Kong is associated with toxin acquisition and multidrug resistance. Nat Genet. (2015) 47:84–7. doi: 10.1038/ng.3147
62. Pop M, Walker AW, Paulson J, Lindsay B, Antonio M, Hossain MA, et al. Diarrhea in young children from low-income countries leads to large-scale alterations in intestinal microbiota composition. Genome Biol. (2014) 15:R76. doi: 10.1186/gb-2014-15-6-r76
63. Neis E, Dejong C, Rensen S. The role of microbial amino acid metabolism in host metabolism. Nutrients. (2015) 7:7042930. doi: 10.3390/nu7042930
64. Zhou LP, Fang LD, Sun Y, Su Y, Zhu WY. Effects of the dietary protein level on the microbial composition and metabolomic profile in the hindgut of the pig. Anaerobe. (2016) 38:61–9. doi: 10.1016/j.anaerobe.2015.12.009
65. Fan PX, Liu P, Song PX, Chen XY, Ma X. Moderate dietary protein restriction alters the composition of gut microbiota and improves ileal barrier function in adult pig model. Sci Rep. (2017) 7:43412. doi: 10.1038/srep43412
66. Kleerebezem M, Boekhorst J, van Kranenburg R, Molenaar D, Oscar PK, Leer R, et al. Complete genome sequence of Lactobacillus plantarum WCFS1. PNAS. (2003) 100:1990–5. doi: 10.1073/pnas.0337704100
67. Fukuda S, Toh H, Hase K, Oshima K, Nakanishi Y, Yoshimura K, et al. Bifidobacteria can protect from enteropathogenic infection through production of acetate. Nature. (2011) 469:543–7. doi: 10.1038/nature09646
Keywords: protein, lipid, gut, bacterial community, microbiota
Citation: Wang K, Peng X, Yang A, Huang Y, Tan Y, Qian Y, Lv F and Si H (2022) Effects of Diets With Different Protein Levels on Lipid Metabolism and Gut Microbes in the Host of Different Genders. Front. Nutr. 9:940217. doi: 10.3389/fnut.2022.940217
Received: 10 May 2022; Accepted: 30 May 2022;
Published: 15 June 2022.
Edited by:
Hui Han, Chinese Academy of Sciences (CAS), ChinaReviewed by:
Yuying Li, Institute of Subtropical Agriculture (CAS), ChinaJing Gao, Institute of Subtropical Agriculture (CAS), China
Copyright © 2022 Wang, Peng, Yang, Huang, Tan, Qian, Lv and Si. This is an open-access article distributed under the terms of the Creative Commons Attribution License (CC BY). The use, distribution or reproduction in other forums is permitted, provided the original author(s) and the copyright owner(s) are credited and that the original publication in this journal is cited, in accordance with accepted academic practice. No use, distribution or reproduction is permitted which does not comply with these terms.
*Correspondence: Hongbin Si, shb2009@gxu.edu.cn