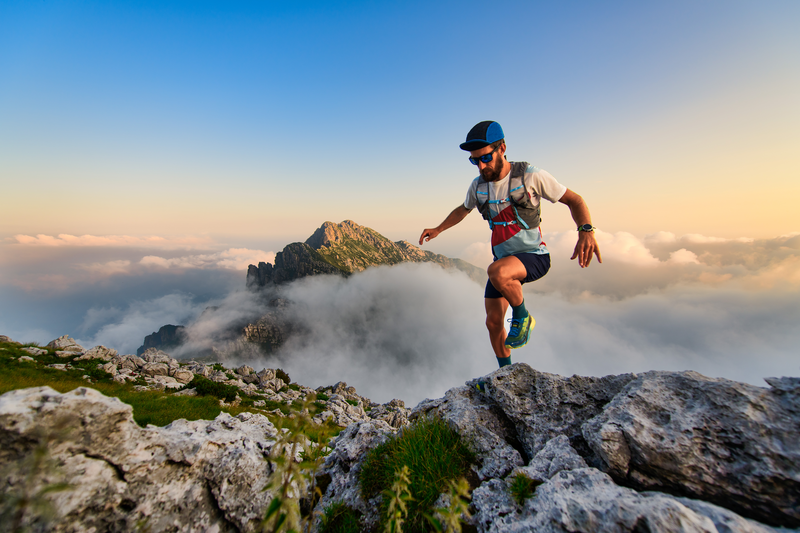
94% of researchers rate our articles as excellent or good
Learn more about the work of our research integrity team to safeguard the quality of each article we publish.
Find out more
ORIGINAL RESEARCH article
Front. Nutr. , 29 July 2022
Sec. Nutrition and Microbes
Volume 9 - 2022 | https://doi.org/10.3389/fnut.2022.934621
This article is part of the Research Topic Next-Generation Prebiotics and Probiotics: Current Status and Future Development View all 7 articles
Polydextrose is a nutrient supplement, which is widely applied in the food industry. The use of polydextrose in combination with prebiotics and probiotics has recently increased, whereas the fermentation properties of its blend have not yet been fully revealed. We evaluated the metabolic profile of polydextrose, inulin, and their blends by a batch in vitro fermentation of fifteen human fecal inocula. After 24 h of fermentation, polydextrose increased the production of gas, ammonia, and several short chain fatty acids, including propionate and butyrate, when compared to its blends, inulin, and fructo-oligosaccharides. Furthermore, polydextrose had the slowest degradation rate of all the carbohydrates tested, consistent with its partial fermentation in the distal colon. The 16S rRNA gene sequencing analysis of the gut microbiome exhibited significantly increased relative abundance of Clostridium_XVIII, Megamonas, Mitsuokella, and Erysipelotrichaceae_incertae_sedis in polydextrose compared to other carbohydrates. On the other hand, the blends of polydextrose and inulin (1:1 or 2:1) showed reduced gas production and similar bifidogenicity to inulin alone. The blends not only had similar alpha-diversity and PCoA to inulin but also had a similar abundance of beneficial bacteria, such as Faecalibacterium and Roseburia, suggesting potential health benefits. Also their low gas production was likely due to the abundance of Faecalibacterium and Anaerostipes, which were negatively correlated with gas production. Additionally, our in vitro fermentation model shows advantages in the large-scale assessment of fermentation performance.
Polydextrose (PDX) is a highly branched glucose polymer with complex random bonds. It was approved 30 years ago as a direct food additive by FDA for use as a nutrient supplement, texturizer, stabilizer or thickener, formulation acid, and humectant (1). Today, it is widely utilized in the food industry as a sucrose substitute (2), a fat replacer (3), or is recognized as a dietary fiber used in healthy foods (4, 5) in a large number of countries.
Early studies have shown PDX offers a variety of health benefits due to the partial fermentation of PDX in the distal colon, such as increasing fecal bulk, softening stools, and reducing fecal pH (6). Recent trials in animals and humans have revealed additional health benefits, such as inducing satiety (7), relief of constipation, improving postprandial serum glucose, protecting against offspring obesity in mouse (8), absorption of minerals from the colon (9), and reducing the risk of developing cancer (10). A clinical trial demonstrated that a combination of PDX with probiotic species Bifidobacterium animalis spp. lactis 420 reduced waist circumference and food intake (11).
Given the key role of gut microbiota in human health, the effect of human gut microbiota has become one of the important properties of dietary fiber and prebiotics. The microbiota contributes to homeostatic regulation in different tissues in our body (12). Diet is an important regulator of the gut microbiota. Western-style diet, which is low in microbiota-accessible carbohydrates, may irreversibly reduce microbial diversity and lead to the disappearance of specific bacterial species in the digestive system (13). These alterations may result in dysfunctions, contributing to the increase in the development of chronic inflammatory diseases (14). Also, adjustment of the human diet with dietary fiber is recognized to at least in part prevent these diseases (15). As a dietary fiber, the impact of PDX on human gut microbiota remains inconsistent, even on Bifidobacteria spp. The stimulatory effect of Bifidobacteria spp. of PDX was not only reported in the continuous in vitro fermentation study (16) but also in the human clinical trial (17). However, the level of Bifidobacterium and Ruminococcus species was reported to decline after PDX supplementation by 16S rRNA gene sequencing (18). The changes in the composition of gut microbiota resulting from PDX intervention vary from study to study. Costabile and colleagues reported a significant increase in the butyrate producer Ruminococcus intestinalis, Clostridium histolyticum (clusters I and II) group, and Clostridium leptum (cluster IV) for PDX in comparison to the placebo by qPCR, which failed to detect by fluorescence in situ hybridization (19). A human study of 20 healthy adult men using 454 sequencings exhibited the relative abundance of Faecalibacterium, Phascolarctobacterium, Dialister, Clostridium, and Akkermansia, which was increased upon PDX consumption, as well as increased abundance of fecal Clostridiaceae and Veillonellaceae, and decreased abundance of Eubacteriaceae (18). In contrast to these dramatic changes in the composition of the healthy gut microbiota, the results of two other human intervention trials with PDX were relatively monotonic but different. PDX consumption increased the relative abundance of Bacteroidetes in the fecal microbiota of healthy men (20) while increasing Christensenellaceae in overweight or obese individuals (21). Obviously, these disputed results of the effects on gut microbiota do not meet the requirements for the application of PDX as a dietary fiber.
Polydextrose has also been studied as a member of the prebiotics combination in in vitro studies (22, 23), animal experiments (24), and human trials (25), the studies of most of which focused on the gas production and Bifidobacteria spp. count. Gas is one of the major end products of fermentation by gut microbiota. Also gas production is also one cause of the major adverse effects after dietary fiber and prebiotics intake, such as bloating and abdominal pain (26). PDX is tolerant of microbial fermentation and is, in turn, believed to release less gas (10). However, the results were controversial. Ghoddusin and colleagues exhibited high gas generation in the mixture of PDX with other prebiotics (22), whereas Vester Boler and colleagues reported lower gas production, butyrate, and total SCFAs production in the mixture of PDX with other carbohydrates (23). Although both of the above studies suggested the low bifidogenic capability of the PDX blends, a double-blind, randomized study demonstrated that infant formula with the blend of PDX with GOS showed a bifidogenic effect closer to breast milk than formula without the blend (25). Recently, the Panel of European Food Safety Authority concluded that there is no need for numerical acceptable daily intake for polydextrose after its re-evaluation as a food additive (27). Therefore, further studies on the impact of PDX and its blends on human gut microbiota are needed to meet their increasing application.
In this study, we assessed the health benefits and adverse effects of PDX, inulin, and their blends and their correlation with the gut microbiota by a modified gut model in which the fermentation system is reduced to accommodate large-scale assays. PDX was blended with inulin in two ratios: 1:1(Mix1) and 2:1 (Mix2). Based on the comparative analysis of fermentation metabolites, PDX exhibited a remarkably unique fermentation performance when compared with its blends. The analysis of 16S rRNA gene sequencing revealed the difference in microbial selection and fermentation associated with the metabolites. Our results demonstrated that the potential health benefits of the blends were due to the similarity in both diversity and composition of fermentation microbiota to inulin. Also, the significantly increased relative abundance of Faecalibacterium and Anaerostipes, which were negatively associated with the gas production, was responsible for the reduced adverse effect of the blends compared to PDX.
Fifteen healthy volunteers aged 25–60 years were recruited for the study. All volunteers consumed traditional Chinese food and no one claimed to be vegetarian. The donors had received neither antibiotics, nor pro- or prebiotic treatments for at least 3 months prior to sample collection. The study protocol was approved by West China Hospital Ethics Committee and registered with the Clinical Trials 2018 No. (286). Fresh fecal samples weighing greater than 3 g were collected into sterilized containers and shipped to the laboratory within 4 h for further analysis.
Polydextrose was provided by Henan Tailijie Biotech Co., Ltd., Mengzhou, China). The degree of polymerization (DP) distribution was as following: 8.1% of DP < 5, 19.9% of DP 5-10, 65.4% of DP 10-20, and 6.6% of DP > 20. Its molecular weight is approximately 2,130 Da. Fructo-oligosaccharide (VILOF® -NanoFOS P95) and inulin (VILOF® -NanoIN) were provided by Fengning Ping An High-Tech, Chengde, China, of which the percentages of DP 2 to 8 were 93.2 and 45%, respectively. The blends, Mix1 and Mix2, were mixed by PDX1 and inulin in ratios 1:1 and 2:1, respectively. Resistant Dextrin (RD), Fibersol® -2 with 90% of total fiber, was provided by Shanghai Toong Yeuan Food Tech Co., Ltd., Shanghai, China.
The basal medium was based on the composition of YCFA described by Duncan (28) and contained the following: 2.5 g/L yeast extract; 10 g/L peptone; 0.8 g/L L-cysteine hydrochloride; 0.05 g/L hemin; 0.9 g/L NaCl; 0.09 g/L MgSO4.7H2O; 0.09 g/L CaCl2.6H2O; 0.45 g/L KH2 PO4, 0.45 g/L K2H PO4; a mixture of micro vitamins and 0.1 mg/L Resazurin which is the indicator of anaerobic condition. The sole carbon sources (8.0 g/L) were added to YCFA to make the respective growth media. The anaerobic media were prepared by flushing N2 and dispensed into 5 to 10 mL serum bottles and autoclaved.
Batch culture fermentation was performed similar to the previous study (29). Briefly, fresh fecal samples (0.8 g) were homogenized with 0.1 M phosphate-buffered saline (pH 7.0) using an automatic fecal homogenizer (Halo Biotechnology Co. Ltd., Suzhou, China) to make a 10% (w/v) slurry. Then 0.5 mL of the fecal slurry was inoculated to 5 mL growth media and subject to anaerobic fermentation at 37°C.
After 24 h of fermentation, the air pressure difference of the serum bottle was measured. Subsequently, the broth was aliquoted and centrifuged. The supernatant and precipitation of the broth were stored at −30°C for further metabolic analysis and DNA extraction for qPCR.
The gas production capacity was determined by the air pressure difference of the serum bottle using a digital manometer (HT1895, Dongguan Xintai Instrument Co., Ltd., Dongguan, China).
The concentrations of SCFAs in each broth and its initial feces were determined by gas chromatography (GC) (Shimadzu, GC-2010 Plus, Japan) equipped with a DB-FFAP column (0.32 mm × 30 m × 0.5 μm) (Agilent Technologies, United States) using H2 flame ionization detector. The amounts of acetic, propionic, butyric, isobutyric, valeric, and isovaleric acids in the filtrates of the broth and the 10% fecal slurry were measured by the internal standard method with crotonic acid (trans-2-butenoic acid) as the internal standard (30).
Ammonia in feces and its fermentation were determined by colorimetry using the enzymatic method described by Barsotti (31).
Degradation of oligosaccharides, such as PDX and inulin, was detected by the thin layer chromatography (TLC) analysis described in the previous study (29). The densities of the oligosaccharide spots in the TLC images were quantified by Quantity One (Bio-Rad, United States). The consumed amounts of oligosaccharides by bacteria were calculated based on the difference in the densities between the medium and the broth. The degradation rate of oligosaccharide was the percentage of the amount consumed to its original amount in the medium.
The bacterial genomic DNA of both the feces and the precipitations of fermentation broths were extracted by TIANamp Stool DNA Kit according to the manufacturer’s instructions (TIANGEN BIOTECH, Beijing, China) and quantified by Nanodrop ND-2000 (NanoDrop Technologies, United States).
The number of Bifidobacteria spp. and Lactobacilli spp. in the feces and the broth were determined by qPCR using CFX96™ Real-time PCR Detection System (Bio-Rad, United States). Total Bifidobacteria species were measured using specific primers Bifi601F: 5’-GGGTGGTAATGCCGGATG and Bifi601R: 5’-TAAGCCATGGACTTTCACACC-3’ (32). Total Lactobacilli species were measured using specific primers Lac-F: 5’-CACCGCTACACATGGAG-3’ and Lac-R: 5’-AGCAGTAGGGAATCTTCCA-3’ (33). The qPCR condition was based on the previous study (34). Bacterial quantification was performed using standard curves constructed from the known concentrations of a plasmid DNA containing the respective amplicons for each set of primers. The copy number of Bifidobacterium amplicons was 5.81 × 107 at 0.2 ng/μL and 5.99 × 109 at 20 ng/μL for Lactobacilli.
The V4-V5 variable region of the 16S rRNA gene was amplified using universal primers 515F (5’-GTGCCAGCMGCCGCGG-3’) and 907-R (5’- CCGTCAATTCMTTTRAGTTT-3’). High-throughput sequencing was performed using the Ion Torrent S5 platform at Zhejiang University, China. Operational Units (OTUs) were clustered with a 97% similarity cutoff using USEARCH UPARSE, and the chimeric sequences were removed in the UPARSE pipeline. The phylogenetic affiliation of each 16S rRNA gene sequence was analyzed by USEARCH SINTAX algorithm against the RDP training set (version 18) 16S rRNA database using a confidence threshold of 0.8.
The alpha diversity indices (Chao1, richness, Simpson and Shannon index) and beta-diversity index (Bray-Curtis, Unifrac, Jacaard, and Unifrac-binary distance) were calculated using USEARCH alpha_div and beta_div, respectively. Principal coordinate analysis (PCoA) based on UniFrac-binary distance matrix was plotted by Vegan 2.4.2 and the p value was obtained by permutational multivariate analysis of variance (PERMANOVA). The raw reads count tables at different taxonomic levels (phylum, order, class, family, and genus) were used for differential abundance analysis with DEseq2. Spearman’s rank correlation coefficient was performed to reveal the correlations with the metabolites and the top 50 genera.
The statistical analysis was performed using GraphPad Prism 7.00 software. Significance (p < 0.05) was performed by non-parametric tests, of which Friedman test was used for metabolic parameters and Wilcoxon test for alpha-diversity indices and relative abundance.
In this study, fecal slurries from 15 volunteers were inoculated for the batch in vitro fermentation to assess the health effects of PDX, inulin, and their blends: Mix1 and Mix2. Along with the metabolic profiles of the fermentation broths (gas production, SCFAs, ammonia, and degradation rates of carbohydrates), the contents of Bifidobacteria spp. and Lactobacillus spp. were also analyzed. For comparison, we also tested the well-studied prebiotics, FOS and inulin as well as a potential prebiotic RD with similar DP to PDX.
Based on the analysis of the broth after 24 h of anaerobic fermentation, PDX exhibited different metabolic profiles from inulin and FOS, as well as the blends. As shown in Figure 1, PDX produced the most gas, ammonia, and total SCFAs while showing the lowest degradation rate, followed by RD. They are significantly different from the fructans and the blends except for the total SCFAs. PDX produced more gas than FOS, inulin, and the two blends. The ammonia concentration of PDX was higher than the fructans and Mix1 which contained 50% of inulin. The degradation rates of PDX were significantly lower than that of FOS and the two blends. Interestingly, the blends’ degradation rates were higher than both PDX and inulin.
Figure 1. Comparison of metabolic parameters after 24h in vitro fermentation. (A) The gas productions of different carbohydrate sources were determined by the air pressure difference of the vial. (B) The concentrations of the total SCFAs were determined by GC with the internal standard method. (C) The concentrations of ammonia in the fermentation broths were determined by colorimetry. (D) The degradations of each carbohydrate were determined by TLC. The data of fifteen volunteers are shown in scatter dot plot, the line is at medium. Only the significant differences between inulin (INU) and PDX are shown. *p value < 0.05, **p value < 0.01, ***p value < 0.001, ****p value < 0.0001.
Despite no significant difference in the total SCFAs (Figure 1B), PDX showed the highest production of individual SCFAs among all carbohydrates except for acetate (Figure 2). The amount of PDX in propionate was significantly higher than those of FOS, inulin, and the blends (Figure 2B). As for butyrate, PDX showed significantly stronger production capacity than FOS and Mix2 (Figure 2C). Polydextrose also exhibited significantly higher isovalerate content than FOS, inulin, and Mix1 (Figure 2F). However, the production of acetate in PDX was trending lower compared with FOS, inulin, and the blends but without significance (Figure 2A).
Figure 2. Comparison of individual SCFAs after 24h in vitro fermentation. The concentrations of individual SCFAs, including acetate (A), propionate (B), butyrate (C), isobutyrate (D), valerate (E), and isovalerate (F) were determined by GC with the internal standard method. The data of fifteen volunteers were shown in a scatter dot plot, line is at mean. Only the significant differences between inulin (INU) and PDX were shown. *p value < 0.05, **p value < 0.01, ***p value < 0.001.
To understand the influence of the carbon sources on the growth of Bifidobacteria spp and Lactobacilli spp, qPCR was performed for the broths after 24 h fermentation. Consistent with the previous report (35), there was no significant difference in lactobacilli growth among carbohydrates (Figure 3B). FOS, inulin, Mix1, and Mix2 stimulated the growth of Bifidobacteria spp at a high level, whereas PDX and RD performed at a low level (Figure 3A). The Bifidobacteria spp amount in PDX was significantly lower than that in FOS, inulin, and Mix2. These results suggested that the blends of inulin and PDX are bifidogenic.
Figure 3. Comparison of Bifidobacteria spp and Lactobacilli spp after 24h in vitro fermentation. The amounts of Bifidobacteria spp. (A) and Lactobacilli spp. (B) in the fermented broth of each carbohydrate were determined by qPCR. The data of fifteen volunteers were shown in scatter dot plot, line is at the mean. Only the significant differences between inulin (INU) and PDX were shown. **p value < 0.01, ***p value < 0.001.
To reveal the microbial difference responsible for the significantly different metabolites among PDX, inulin, and their blends, their broths were subjected to 16S rRNA genes sequencing. As shown in Figure 4A, the alpha-diversity indices of the blends of PDX and inulin were higher than both PDX and inulin, but there was no significant difference between PDX and inulin. PcoA plot displayed the overlap of PDX and the blends, which was less than that between inulin and the blends (Figure 4B). Based on the pairwise difference analysis of PCoA1 dimension, the differences between PDX and Mix1 or Mix2 are greater than that between inulin and the blends. This is the same result we see for PCoA2. These results suggest that the microbial community structures of the two blends were close to inulin. As for composition in the genus level, the blends were also similar to inulin, but not PDX (Figure 4C).
Figure 4. Microbiota comparison of the fermentation broths of inulin, PDX, and their blends. (A) The alpha-diversity of the microbiota of the fermentation broths. (B) PCoA plot of the fermentation broths with Unifrac-binary distance and the p value was calculated by PERMANOVA. (C) The microbial composition of the fermentation broths at the genus level. *p value < 0.05, **p value < 0.01, ***p value < 0.001.
The differential abundance analysis revealed that eight genera were significantly different among PDX, inulin, and their blends, with more than 2-fold changes in relative abundance. Roseburia, Anaerostipes, and Clostridium_XVlb (Figure 5A) were significantly decreased in PDX compared to inulin or the blends. Clostridum_XVIII and Erysipelotrichaceae_incertae_sedis (Figure 5B) significantly increased in PDX compared to the other media. Mitsuokella and Megamonas (Figure 5B) were significantly lower in the blends than those in PDX or inulin, while there was no significant difference between PDX and inulin. Dorea (Figure 5C) was the differential genus between inulin and the blends and the only genus with similar relative abundance between PDX and Mix1 or Mix2. Additionally, Faecalibacterium significantly decreased in PDX with the comparison of inulin or the blends (Figure 5A). On the other hand, the relative abundance of Bifidobacterium was significantly lower in PDX than in inulin or Mix2. There was no significant difference in Lactobacillus abundance among the 4 carbohydrates, despite low amount in PDX. These data confirmed the results obtained by qPCR (Figure 3).
Figure 5. Differential genera between PDX and inulin or their blends and their contribution to metabolites. (A) The genera in PDX with 2-fold lower relative abundance than in inulin (INU) or their blends. (B) The genera in PDX with 2-fold higher relative abundance than in INU or their blends. (C) The genera with a less than 2-fold change abundance between PDX and INU or their blends. (D) Heatmap of the correlations with the top 50 genera and the metabolites revealed by Spearman’s rank coefficient correlation analysis. Three genera in panel (A) are shown in blue and in panel (B) are in red. The metabolites with significant differences between PDX and INU or their blends were in purple. TA, total SCFAs, AA, acetate, DeR, degradation rate, VA, valerate, iBA, isobutyrate, iVA, isovalerate, PA, propionate, GasP, gas production, BA, butyrate, AMM, ammonia. *p value < 0.05, **p value < 0.01, ***p value < 0.001, ****p value < 0.0001.
Spearman’s rank correlation coefficient analysis was performed to reveal the correlation between genera and metabolites (Figure 5D). The genera enriched in PDX (Figure 5B), Erysipelotrichaceae_incertae_sedis, Megamonas, Mitsuokella, and Clostridium_XVIII, were positively correlated with propionate, ammonia, butyrate, and gas production while negatively associated with degradation rate, which was significant except for degradation rate. In addition, Clostridium_XVIII also had a significant positive correlation with isovalerate. The genera reduced in PDX (Figure 5A), Faecalibacterium, Roseburia, Anaerostipes, and Clostridium_XVlb, were negatively correlated with gas production, butyrate, propionate, and ammonia. Additionally, Clostridium_XVlb showed a significant negative correlation with isovalerate. These results suggest oppositive effects on metabolic parameters between the enriched and reduced genera in PDX, leading to a significant difference in the metabolites between PDX and inulin or their blends (Figures 1, 2).
Polydextrose has been regarded as a food supplement since 1987. Many studies including human trials, animal experiments, and in vitro studies, have demonstrated that the ingestion of PDX confers benefits to hosts. In this study, 15 human fecal samples were collected and subjected to batch fermentation with the media containing Mix1, Mix2, PDX, inulin, FOS, and RD as the sole carbohydrate source. PDX exhibited distinct fermentation properties compared to other carbohydrates except for RD. The metabolic and microbial parameters of Mix1 and Mix2, the blends of PDX and inulin, were close to those of inulin but not PDX.
Based on the data from fifteen individuals, PDXs produced a greater increase in propionate (p < 0.01) and butyrate (p < 0.05) than an equal dose of FOS, inulin, and its blends, which was supported by the recent in vitro studies. Romero Marcia and colleagues observed that PDX produced higher levels of butyrate and propionate compared to other soluble resistant glucans in batch fermentation (36). Increased propionate in PDX fermentation was observed in a 3-stage continuous gut model (17) and semicontinuous human colon simulator (37). Propionate and butyrate were reported to regulate energy homeostasis and appetite since they are the most potent agonists for G protein-coupled receptor 41 (GPR41/FFAR3). GPR41 is strongly expressed in the L cell, which is responsible for the release of peptide tyrosine (PYY) and glucagon-like peptide-1 (GLP-1), which is the biomarker or potential biomarker of satiety (38). In addition, GLP-1 has long been recognized as a potent stimulator of insulin secretion (39). Therefore, the significantly high propionate and butyrate observed in this study likely provide an explanation of the health benefits of PDX, such as improving postprandial glycemia and satiety (10, 40).
Besides propionate and butyrate, PDX was found to increase gas production after 24 h fermentation when compared to FOS, inulin, and the blends. This was supported by the results of the 21-day human trial of PDX supplementation, in which subjects taking PDX had the greatest amount of flatulence compared to placebo and soluble maize fiber (23). In fact, the gas production of PDX seems to increase with fermentation time. It has been reported to be low in 12-h fermentation and high after 32 h (22). Lower gas production compared to FOS and inulin in the early time of in vitro fermentation was also observed at 4, 8, and 12 h after inoculation (23). The partial fermentation of PDX in the distal colon has been confirmed by both a multiple-stage colon simulator study (41) and a human trial (19), leading to a high mean laxative threshold of approximately 90 g/d (42). Our data indicated that the degradation rate of PDX was observed to be the lowest among all carbohydrates, also suggesting the tolerance of PDX to fermentation. Therefore, the increased gas production after 24 h fermentation indicated the beginning and growing of a delayed fermentation of PDX, which is consistent with the concomitantly detected high amounts of SCFAs. As a result, our in vitro study supports that PDX is resistant to microbial utilization in the early stages of fermentation, while produces large amounts of SCFAs, especially butyrate and propionate, in the later stages of fermentation. According to Spearman’s correlation analysis, Clostridium_XVIII, Megamonas, Mitsuokella, and Erysipelotrichaceae_incertae_sedis were positively correlated with both propionate and butyrate. Clostridium was previously reported to increase after PDX intake compared to the placebo group by both 16S rRNA sequencing (18) and qPCR (19). Clostridial species are producers of propionate (43) and butyrate, in particular, Clostridium cluster IV and XIVa, such as Faecalibacterium prausnitzii and Eubacterium rectale, respectively (44). Also Clostridium_XVIII was reported to induce homeostatic T-reg responses, as well as Clostridium cluster IV and XIVa (45), which have been considered the next-generation probiotics (46). Thus, our results implied that Clostridium_XVIII was also a producer of propionate and butyrate, at least following the fermentation of PDX. Besides Clostridium_XVIII, our data indicated the significantly elevated Erysipelotrichaceae_incertae_sedis in PDX compared to both inulin and the blends, which was significantly positively correlated with propionate. Lamichhane and colleagues used the isotopic technique to find that the abundance of Erysipelotrichaceae was positively correlated with the yield of SCFAs derived from PDX, especially of butyrate and propionate (47). The recent in vitro study revealed the increased Erysipelotrichaceae in PDX compared to other resistant glucans (36). Erysipelotrichaceae family is reported as SCFAs producers, and some species within this family are capable of producing butyrate (48, 49). It is reported that Mitsuokella is a potential genus responsible for the presence of butyric acid in exhaled breath volatile metabolites after chitin-glucan consumption (50). Megamonas is regarded as a producer of SCFAs. It produced propionate and acetate in the poultry intestine (51) and positively correlated with fecal lactate in dogs (52) and butyrate in human feces (53). Hence, the significantly increased propionate and butyrate after PDX fermentation in this study is supported by the previous literature. Coinciding with the previous study that several species belonging to Clostridium were ammonia producers (54), Clostridium_XVIII exhibited a significantly positive association with ammonia production in this study, leading to the great amount of ammonia after PDX fermentation.
Unfortunately, we did not find the bacteria responsible for gas production, in agreement with the opinion of the previous study that it was difficult to establish the bacteria is responsible for gas production (22). As an alternative, a strongly negative correlation with gas production was found in the predominant genera of inulin compared to PDX, including Roseburia, Anaerostipes, and Clostridium_XVlb. Our data suggested that the inhibition activity could also be responsible for the significant metabolic difference.
In this study, two blends of PDX with inulin were prepared. Although the ratio of PDX in Mix2 (2:1) was higher than that of Mix1 (1:1), both blends produced a lower amount of gas than PDX, which is similar to that of inulin. These results were not in agreement with the prior data that inulin blended with PDX produced the highest amount of gas compared to inulin and PDX alone (22). This might be assigned to the difference in the measurement method and sample size. Besides gas production, other metabolic parameters of the blends were also at the same level as those of inulin but not PDX, such as ammonia, degradation rate, individual SCFAs except for acetate. Furthermore, the analysis of 16S sequencing revealed that not only the microbial community structures but also the compositions of the blends were closer to inulin than to PDX. Among the 8 different genera, only genus Dorea showed a significantly different abundance between the blends and inulin. Dorea was reported to be positively associated with intestinal permeability (55). Hence, our result suggested the role of inulin in improving colonic barrier function. Mix2 has an increased difference of Dorea abundance from inulin than Mix1, suggesting increasing the proportion of inulin in the blend helps reduce intestinal permeability. Both Mix1 and Mix2 increased the relative abundance of Faecalibacterium, Roseburia, Anaerostipes, and Clostridium_XVlb, of which the level was comparable to inulin. Faecalibacterium is a highly abundant gut microbiota with anti-inflammatory and immunoregulatory properties in healthy human individuals (56). Roseburia is an anaerobic bacterium belonging to Clostridium_XIVa and plays an important role in maintaining the intestinal barrier function and immune defense (57). Consistent with our result, Anaerostipes was reported to increase with Bifidobacterium after inulin supplementation (58). Despite the lowest abundance of Bifidobacterium in PDX, both Mix1 and Mix2 showed high selectivity toward Bifidobacterium, which was comparable to inulin. This was consistent with the previous result that the blends of inulin can augment or maintain their bifidogenic capacity (22). As a result, our results suggested the potential health benefits of the blends of PDX and inulin.
A limitation of this study is the small sample size. However, our results show that the combination of PDX and inulin has the advantage of high bifidogenic capacity and low gas yield. The topic of gas production from the dietary fiber is important because dietary fiber supplementation has emerged as a solution to reduce the health threat posed by Westernization of the diet, while gas production can cause gastrointestinal discomfort and affect the application of dietary fiber. Furthermore, our previous study elucidates increased gas production following inulin fermentation in middle-aged people compared with younger subjects (59). Therefore, further studies are planned in larger cohorts with different age groups, especially the elderly population.
The fermentation performance of PDX and its blends with inulin were evaluated by the in vitro fermentation of the inocula from 15 volunteers. PDX was resistant to being degraded by the gut microbiota to an extent at the beginning of fermentation and enhanced the production of butyrate and propionate later in distal colon, which was likely due to the high abundance of SCFAs producers, Clostridium_XVIII, Megamonas, Mitsuokella and Erysipelotrichaceae_incertae_sedis. Also the highest gas production among all carbon sources seemed to result from the significantly reduced abundance of Faecalibacterium and Anaerostipes, which significantly suppressed the gas production. Despite high gas production and low bifidogenicity of PDX, the blends of PDX with inulin showed reduced gas production and increased bifidogenicity due to their microbiota similar to inulin. Our results suggested the potential health benefits of the blends and the advantages of our fermentation model in characterizing the fermentation properties of prebiotics, especially for large-scale assessment.
The datasets presented in this study can be found in online repositories. The names of the repository/repositories and accession number(s) can be found below: https://nmdc.cn/, NMDC40017470.
The studies involving human participants were reviewed and approved by West China Hospital Ethics Committee and registered with the Clinical Trials 2018 No. (286). The patients/participants provided their written informed consent to participate in this study.
ZG did the experiments. FG, XC, and XQ conceived the idea for the study. WJ and XiangL recruited volunteers. XiaoL and JL analyzed the data. LZ and XW designed the experiments. LZ wrote the manuscript. All authors reviewed the manuscript.
This study was supported by the National Key R & D Program of China (project number: 2017YFD0400302) and Sichuan Key R&D Program (No. 2018SZ0112).
FG and ZG were employed by Henan Tailijie Biotech Co., Ltd. XC and XQ were employed by Fengning Pingan High-Tech Industrial Co., Ltd. XiangL was employed by Suzhou Hailu Biotech Co., Ltd.
The remaining authors declare that the research was conducted in the absence of any commercial or financial relationships that could be construed as a potential conflict of interest.
All claims expressed in this article are solely those of the authors and do not necessarily represent those of their affiliated organizations, or those of the publisher, the editors and the reviewers. Any product that may be evaluated in this article, or claim that may be made by its manufacturer, is not guaranteed or endorsed by the publisher.
1. Shimada M, Nagano N, Goto S, Ito K, Tsutsui T, Ando T, et al. Effect of polydextrose intake on constipation in Japanese dialysis patients: a triple-blind, randomized, controlled trial. J Nutr Sci Vitaminol. (2015) 61:345–53. doi: 10.3177/jnsv.61.345
2. Gałkowska D, Południak M, Witczak M, Juszczak L. Effect of prebiotic polysaccharides on the rheological properties of reduced sugar potato starch based desserts. Polymers. (2020) 12:2224. doi: 10.3390/polym12102224
3. Hamdy SM, Abdelmontaleb HS, Mabrouk AM, Abbas KA. Physicochemical, viability, microstructure, and sensory properties of whole and skimmed buffalo set-yogurts containing different levels of polydextrose during refrigerated storage. J Food Process Preserv. (2021) 45:e15643.
4. Canbulat Z, Ozcan T. Effects of short-chain and long-chain inulin on the quality of probiotic yogurt containing Lactobacillus rhamnosus. J Food Process Preserv. (2014) 39:1251–60.
5. Bisar G, El-Saadany K, Khattab A, El-Kholy W. Implementing maltodextrin, polydextrose and inulin in making a synbiotic fermented dairy product. Br Microbiol Res J. (2015) 8:585–603.
6. Endo K, Kumemura M, Nakamura K, Fujisawa T, Suzuki K, Benno Y, et al. Effects of high cholesteroal diet and polydextorse supplementation on the microflora, bascteria enzyme activity, putrefactive products, volatile fatty acid profile, weight, and pH of the feces in healthy volunteers. Bifidobacteria Microflora. (1991) 10:53–64.
7. Ibarra A, Astbury NM, Olli K, Alhoniemi E, Tiihonen K. Effect of polydextrose on subjective feelings of appetite during the satiation and satiety periods: a systematic review and meta-analysis. Nutrients. (2016) 8:45. doi: 10.3390/nu8010045
8. Maragkoudaki X, Naylor M, Papacleovoulou G, Stolarczyk E, Rees D, Pombo JM, et al. Supplementation with a prebiotic (polydextrose) in obese mouse pregnancy improves maternal glucose homeostasis and protects against offspring obesity. Int J Obesity. (2020) 44:2382–93. doi: 10.1038/s41366-020-00682-5
9. do Carmo MM, Walker JC, Novello D, Caselato VM, Sgarbieri VC, Ouwehand AC, et al. Polydextrose: physiological function, and effects on health. Nutrients. (2016) 8:553.
10. Roytio H, Ouwehand AC. The fermentation of polydextrose in the large intestine and its beneficial effects. Benef Microbes. (2014) 5:305–13.
11. Stenman LK, Lehtinen MJ, Meland N, Christensen JE, Yeung N, Saarinen MT, et al. Probiotic with or without fiber controls body fat mass, associated with serum zonulin, in overweight and obese adults-randomized controlled trial. EBioMedicine. (2016) 13:190–200.
12. Schroeder BO, Bäckhed F. Signals from the gut microbiota to distant organs in physiology and disease. Nat. Med. (2016) 22:1079–89.
13. Sonnenburg ED, Smits SA, Tikhonov M, Higginbottom SK, Wingreen NS, Sonnenburg JL. Diet-induced extinctions in the gut microbiota compound over generations. Nature. (2016) 529:212–5. doi: 10.1038/nature16504
14. Makki K, Deehan EC, Walter J, Bäckhed F. The impact of dietary fiber on gut microbiota in host health and disease. Cell Host Microbe. (2018) 23:705–15.
15. Deehan EC, Walter J. The fiber gap and the disappearing gut microbiome: implications for human nutrition. Trends Endocrinol Metab. (2016) 27:239–42. doi: 10.1016/j.tem.2016.03.001
16. Probert HM, Apajalahti JH, Rautonen N, Stowell J, Gibson GR. Polydextrose, lactitol, and fructo-oligosaccharide fermentation by colonic bacteria in a three-stage continuous culture system. Appl Environ Microbiol. (2004) 70:4505–11. doi: 10.1128/AEM.70.8.4505-4511.2004
17. Zhong J, Luo B, Xiang M, Liu H, Zhai Z, Wang T, et al. Studies on the effects of polydextrose intake on physiologic functions in Chinese people. Am J Clin Nutr. (2000) 72:1503–9. doi: 10.1093/ajcn/72.6.1503
18. Hooda S, Boler BM, Serao MC, Brulc JM, Staeger MA, Boileau TW, et al. 454 pyrosequencing reveals a shift in fecal microbiota of healthy adult men consuming polydextrose or soluble corn fiber. J Nutr. (2012) 142:1259–65.
19. Costabile A, Fava F, Roytio H, Forssten SD, Olli K, Klievink J, et al. Impact of polydextrose on the faecal microbiota: a double-blind, crossover, placebo-controlled feeding study in healthy human subjects. Br J Nutr. (2012) 108:471–81. doi: 10.1017/S0007114511005782
20. Holscher HD, Caporaso JG, Hooda S, Brulc JM, Fahey GC Jr., Swanson KS. Fiber supplementation influences phylogenetic structure and functional capacity of the human intestinal microbiome: follow-up of a randomized controlled trial. Am J Clin Nutr. (2014) 101:55–64. doi: 10.3945/ajcn.114.092064
21. Hibberd AA, Yde CC, Ziegler ML, Honoré AH, Saarinen MT, Lahtinen S, et al. Probiotic or synbiotic alters the gut microbiota and metabolism in a randomised controlled trial of weight management in overweight adults. Benefic Microbes. (2019) 10:121–35.
22. Ghoddusi HB, Grandison MA, Grandison AS, Tuohy KM. In vitro study on gas generation and prebiotic effects of some carbohydrates and their mixtures. Anaerobe. (2007) 13:193–9. doi: 10.1016/j.anaerobe.2007.06.002
23. Vester Boler BM, Hernot DC, Boileau TW, Bauer LL, Middelbos IS, Murphy MR, et al. Carbohydrates blended with polydextrose lower gas production and short-chain fatty acid production in an in vitro system. Nutr. Res. (2009) 29:631–9. doi: 10.1016/j.nutres.2009.09.003
24. Peuranen S, Tiihonen K, Apajalahti J, Kettunen A, Saarinen M, Rautonen N. Combination of polydextrose and lactitol affects microbial ecosystem and immune responses in rat gastrointestinal tract. Br J Nutr. (2004) 91:905–14. doi: 10.1079/BJN20041114
25. Scalabrin DM, Mitmesser SH, Welling GW, Harris CL, Marunycz JD, Walker DC, et al. New prebiotic blend of polydextrose and galacto-oligosaccharides has a bifidogenic effect in young infants. J Pediatr Gastroenterol Nutr. (2012) 54:343–52. doi: 10.1097/MPG.0b013e318237ed95
26. Probert HM, Gibson GR. Investigating the prebiotic and gas-generating effects of selected carbohydrates on the human colonic microflora. Lett Appl Microbiol. (2002) 35:473–80. doi: 10.1046/j.1472-765x.2002.01223.x
27. Younes M, Aquilina G, Castle L, Engel KH, Fowler P, Fürst P, et al. Re-evaluation of polydextrose (E 1200) as a food additive. EFSA J Eur Food Safety Author. (2021) 19:e06363. doi: 10.2903/j.efsa.2021.6363
28. Duncan SH, Louis P, Thomson JM, Flint HJ. The role of pH in determining the species composition of the human colonic microbiota. Environ Microbiol. (2009) 11:2112–22.
29. Wu Q, Pi X, Liu W, Chen H, Yin Y, Yu HD, et al. Fermentation properties of isomaltooligosaccharides are affected by human fecal enterotypes. Anaerobe. (2017) 48:206–14. doi: 10.1016/j.anaerobe.2017.08.016
30. Mao S, Huo W, Zhu W. Use of pyrosequencing to characterize the microbiota in the ileum of goats fed with increasing proportion of dietary grain. Curr Microbiol. (2013) 67:341–50. doi: 10.1007/s00284-013-0371-0
32. Bartosch S, Fite A, Macfarlane GT, Mcmurdo MET. Characterization of bacterial communities in feces from healthy elderly volunteers and hospitalized elderly patients by using real-time PCR and effects of antibiotic treatment on the fecal microbiota. Appl Environ Microbiol. (2004) 70:3575–81. doi: 10.1128/AEM.70.6.3575-3581.2004
33. Yang D, Yu X, Wu Y, Chen X, Wei H, Shah NP, et al. Enhancing flora balance in the gastrointestinal tract of mice by lactic acid bacteria from Chinese sourdough and enzyme activities metabolism of protein, fat, and carbohydrate by the flora. J Dairy Sci. (2016) 99:7809–20. doi: 10.3168/jds.2016-11467
34. Yin Y, Wang Y, Zhu L, Liu W, Liao N, Jiang M, et al. Comparative analysis of the distribution of segmented filamentous bacteria in humans, mice and chickens. Isme J. (2013) 7:615–21. doi: 10.1038/ismej.2012.128
35. Hernot DC, Boileau TW, Bauer LL, Middelbos IS, Murphy MR, Swanson KS, et al. In vitro fermentation profiles, gas production rates, and microbiota modulation as affected by certain fructans, galactooligosaccharides, and polydextrose. J Agric Food Chem. (2009) 57:1354–61. doi: 10.1021/jf802484j
36. Romero Marcia AD, Yao T, Chen M-H, Oles RE, Lindemann SR. Fine carbohydrate structure of dietary resistant glucans governs the structure and function of human gut microbiota. Nutrients. (2021) 13:2924. doi: 10.3390/nu13092924
37. Mäkeläinen HS, Mäkivuokko HA, Salminen SJ, Rautonen NE, Ouwehand AC. The effects of polydextrose and xylitol on microbial community and activity in a 4-stage colon simulator. J Food Sci. (2007) 72:M153–9. doi: 10.1111/j.1750-3841.2007.00350.x
38. de Graaf C, Blom WA, Smeets PA, Stafleu A, Hendriks HF. Biomarkers of satiation and satiety. Am J Clin Nutr. (2004) 79:946–61.
39. Smith NK, Hackett TA, Galli A, Flynn CR. GLP-1: molecular mechanisms and outcomes of a complex signaling system. Neurochem Int. (2019) 128:94–105.
40. Olli K, Salli K, Alhoniemi E, Saarinen M, Ibarra A, Vasankari T, et al. Postprandial effects of polydextrose on satiety hormone responses and subjective feelings of appetite in obese participants. Nutr J. (2015) 14:2. doi: 10.1186/1475-2891-14-2
41. Mäkivuokko H, Nurmi J, Nurminen P, Stowell J, Rautonen N. In vitro effects on polydextrose by colonic bacteria and caco-2 cell cyclooxygenase gene expression. Nutr Cancer. (2005) 52:94–104. doi: 10.1207/s15327914nc5201_12
42. Flood MT, Auerbach MH, Craig SA. A review of the clinical toleration studies of polydextrose in food. Food Chem Toxicol. (2004) 42:1531–42. doi: 10.1016/j.fct.2004.04.015
43. Macfabe DF. Short-chain fatty acid fermentation products of the gut microbiome: implications in autism spectrum disorders. Microb Ecol Health Dis. (2012) 24:23.
44. Rivière A, Selak M, Lantin D, Leroy F, De Vuyst L. Bifidobacteria and butyrate-producing colon bacteria: importance and strategies for their stimulation in the human gut. Front Microbiol. (2016) 7:979. doi: 10.3389/fmicb.2016.00979
45. Atarashi K, Tanoue T, Oshima K, Suda W, Nagano Y, Nishikawa H, et al. Treg induction by a rationally selected mixture of Clostridia strains from the human microbiota. Nature. (2013) 500:232–6. doi: 10.1038/nature12331
46. El Hage R, Hernandez-Sanabria E, Van de Wiele T. Emerging trends in “Smart Probiotics”: functional consideration for the development of novel health and industrial applications. Front Microbiol. (2017) 8:1889. doi: 10.3389/fmicb.2017.01889
47. Lamichhane S, Yde CC, Jensen HM, Morovic W, Hibberd AA, Ouwehand AC, et al. Metabolic fate of (13)C-labeled polydextrose and impact on the gut microbiome: a triple-phase study in a colon simulator. J Proteome Res. (2018) 17:1041–53. doi: 10.1021/acs.jproteome.7b00683
48. Vital M, Howe AC, Tiedje JM. Revealing the bacterial butyrate synthesis pathways by analyzing (meta)genomic data. mBio. (2014) 5:e00889. doi: 10.1128/mBio.00889-14
49. Pozuelo M, Panda S, Santiago A, Mendez S, Accarino A, Santos J, et al. Reduction of butyrate- and methane-producing microorganisms in patients with Irritable Bowel Syndrome. Sci Rep. (2015) 5:12693. doi: 10.1038/srep12693
50. Neyrinck AM, Rodriguez J, Zhang Z, Seethaler B, Mailleux F, Vercammen J, et al. Noninvasive monitoring of fibre fermentation in healthy volunteers by analyzing breath volatile metabolites: lessons from the FiberTAG intervention study. Gut Microbes. (2021) 13:1–16. doi: 10.1080/19490976.2020.1862028
51. Scupham AJ, Patton TG, Bent E, Bayles DO. Comparison of the cecal microbiota of domestic and wild turkeys. Microbial Ecol. (2008) 56:322–31. doi: 10.1007/s00248-007-9349-4
52. Sandri M, Dal Monego S, Conte G, Sgorlon S, Stefanon B. Raw meat based diet influences faecal microbiome and end products of fermentation in healthy dogs. BMC Vet Res. (2017) 13:65. doi: 10.1186/s12917-017-0981-z
53. Shi J, Zhao D, Zhao F, Wang C, Zamaratskaia G, Li C. Chicken-eaters and pork-eaters have different gut microbiota and tryptophan metabolites. Sci Rep. (2021) 11:11934. doi: 10.1038/s41598-021-91429-3
54. Richardson AJ, McKain N, Wallace RJ. Ammonia production by human faecal bacteria, and the enumeration, isolation and characterization of bacteria capable of growth on peptides and amino acids. BMC Microbiol. (2013) 13:6. doi: 10.1186/1471-2180-13-6
55. Haro C, Villatoro M, Vaquero L, Pastor J, Giménez MJ, Ozuna CV, et al. The dietary intervention of transgenic low-gliadin wheat bread in patients with Non-Celiac Gluten Sensitivity (NCGS) showed no differences with Gluten Free Diet (GFD) but provides better gut microbiota profile. Nutrients. (2018) 10:1964. doi: 10.3390/nu10121964
56. Laursen MF, Laursen RP, Larnkjær A, Mølgaard C, Michaelsen KF, Frøkiær H, et al. Faecalibacterium gut colonization is accelerated by presence of older siblings. mSphere. (2017) 2:e00448–17. doi: 10.1128/mSphere.00448-17
57. Kasahara K, Krautkramer KA, Org E, Romano KA, Kerby RL, Vivas EI, et al. Interactions between Roseburia intestinalis and diet modulate atherogenesis in a murine model. Nat Microbiol. (2018) 3:1461–71. doi: 10.1038/s41564-018-0272-x
58. Vandeputte D, Falony G, Vieira-Silva S, Wang J, Sailer M, Theis S, et al. Prebiotic inulin-type fructans induce specific changes in the human gut microbiota. Gut. (2017) 66:1968–74.
Keywords: polydextrose, in vitro study, short chain fatty acids, gas production, 16S rRNA gene sequencing, blends of PDX and inulin, bifidogenic effect
Citation: Zhu L, Guo F, Guo Z, Chen X, Qian X, Li X, Li X, Li J, Wang X and Jia W (2022) Potential health benefits of lowering gas production and bifidogenic effect of the blends of polydextrose with inulin in a human gut model. Front. Nutr. 9:934621. doi: 10.3389/fnut.2022.934621
Received: 02 May 2022; Accepted: 28 June 2022;
Published: 29 July 2022.
Edited by:
Satya Prakash, McGill University, CanadaReviewed by:
Lucille Marie Yanckello, University of Kentucky, United StatesCopyright © 2022 Zhu, Guo, Guo, Chen, Qian, Li, Li, Li, Wang and Jia. This is an open-access article distributed under the terms of the Creative Commons Attribution License (CC BY). The use, distribution or reproduction in other forums is permitted, provided the original author(s) and the copyright owner(s) are credited and that the original publication in this journal is cited, in accordance with accepted academic practice. No use, distribution or reproduction is permitted which does not comply with these terms.
*Correspondence: Liying Zhu, emh1bGl5aW5nQGhvdG1haWwuY28uanA=; Weiguo Jia, amlhd2dAdmlwLjE2My5jb20=
Disclaimer: All claims expressed in this article are solely those of the authors and do not necessarily represent those of their affiliated organizations, or those of the publisher, the editors and the reviewers. Any product that may be evaluated in this article or claim that may be made by its manufacturer is not guaranteed or endorsed by the publisher.
Research integrity at Frontiers
Learn more about the work of our research integrity team to safeguard the quality of each article we publish.