- 1Guangdong Provincial Key Laboratory of Food Quality and Safety, College of Food Science, South China Agricultural University, Guangzhou, China
- 2School of Biotechnology and Health Sciences, Wuyi University, Jiangmen, China
- 3Guangdong Laboratory for Lingnan Modern Agriculture, Guangzhou, China
Rosa roxburghii Tratt (R. roxburghii) tea is a traditional Chinese beverage. This study aims to investigate and compare the phenolics in free and bound forms of two cultivars of R. roxburghii leaves, and their bioactivities. The total phenolic content of free and bound fractions was 72.71 and 17.75 mg GAE/g DW in Gui Nong No. 5 (GNN5) and 94.28 and 11.19 mg GAE/g DW in Seedless Cili (SC). A total of 37 phenolic compounds were characterized and quantified by UPLC-Q-Exactive Orbitrap/MS with ellagic acid, quercitrin, isoquercitrin, and quininic acid in free fraction, while gallic acid, ellagic acid, and hyperoside were main compounds in bound fraction. The free fraction with higher phenolic contents also showed excellent performances on antioxidant activities and α-glucosidase inhibitory potency than bound phenolics. Therefore, the results highlight that R. roxburghii leaves are a promising source enriched in phenolic constituents for functional beverages and nutritional foods.
Introduction
Rosa roxburghii Tratt (R. roxburghii), a member of the Rosaceae family, is widely planted in the mountainous and central hilly areas of the southwest and central south regions of China. Currently, Gui Nong No. 5 (GNN5) and Seedless Cili (SC) are the largest two cultivars (1). According to the records of “Encyclopedia of Guizhou” and “A Supplement to the Compendium of Materia Medica,” the fruits, leaves, and roots of R. roxburghii are widely used to treat many diseases, such as invigorating spleen and dissolving heat stroke. As a medicinal food homoeologous plant, R. roxburghii has recently attracted increasing attention, as it is rich in nutritional and functional constituents. The tea made from R. roxburghii leaves not only has a unique tea aroma, but also possesses various health benefits, including antioxidant (2), anti-inflammatory, and lowering blood sugar activities (3). It has been historically used as an edible and medicinal resource for the treatment of diabetes in southwestern China. Based on these advantages, R. roxburghii tea has been selected into “the national famous, special and excellent new agricultural products catalog.” These healthcare benefits have been mainly ascribed to high contents of phenolics. Studies on R. roxburghii tea have focused on process optimization and functional activities, but there are a few reports on the polyphenol species and contents in them.
As the most abundant secondary metabolites of plants, polyphenols are often widely present in plant and plant-derived products in the daily diet, which have various biological functions, such as antioxidant, anti-inflammatory, and antidiabetic activities (4). In particular, polyphenols strongly inhibit α-glucosidase, which is located on the brush border of the small intestine villi and is a key carbohydrate-hydrolyzing enzyme, mostly the terminal α-bonds of oligosaccharides, form glucose, which is the final stage of carbohydrate digestion (5). Therefore, inhibition of α-glucosidase is an effective method for alleviating postprandial hyperglycemia without diarrhea and other intestinal disturbances caused by current drugs, such as acarbose and miglitol (6). Exploration of effective natural polyphenolic α-glucosidase inhibitors with minimal side effects is required and is considered important for the management of diabetes mellitus.
Polyphenols exist in plants in two main forms, namely, those in the free form are often found in the vacuole of plant cells, and those in the bound form are linked to other natural chemical products through ester linkages, ether linkages, and glycosidic linkages (7, 8). Currently, most studies mainly concentrate on the composition and functional activities of free phenolics than that of bound phenolics. In fact, bound phenolics is the more prevalent form of phenolics in foods. For instance, about 50–95% of phenolic contents were contributed in bound form in vegetables (9), fruits, and legume/seeds (10). The significant amounts of bound phenolics released slowly and continuously by the digestion and colonic fermentation may allow improving the bioaccessibility, bioavailability, and bioactivity of phenolics for a comparatively long time. As a result, both free and bound phenolics should be considered to fully estimate the bioactive values of foods, which is conducive to the rational development and utilization of natural chemical resources. However, there are few studies on thorough polyphenols identification based on mass spectrometry and evaluation of antioxidant and α-glucosidase enzyme inhibition activity of R. roxburghii leaves. Hence, the characterization of phenolic metabolites of R. roxburghii leaves and assessment of their potential biological activities are of great interest in the food and pharmaceutical industries.
Thus, the aim of this study was to characterize the phenolic profile (free and bound) of the largest two cultivars of R. roxburghii leaves using ultra-performance liquid chromatography along with Q-Exactive Orbitrap tandem mass spectrometry (UPLC-Q-Exactive Orbitrap/MS). Furthermore, the antioxidant capacities of each phenolic extract were determined by different antioxidant methods and hypoglycemic function was evaluated by the inhibition of α-glucosidase.
Experiment
Chemicals
The standard reference materials were purchased from Sigma Aldrich (Shanghai, China). The 2,2’-azinobis [3-ethylbenzothiazoline-6-sulfonic acid ammonium salt (ABTS)], 2,2-diphenyl-1-picrylhydrazyl (DPPH), fluorescein, 6-hydroxy-2,5,7,8-tetramethylchroman-2-carboxylic acid (Trolox), 3’,6’-dihydroxyspiro [isobenzofuran-1 (3 H),9’-(9 H) xanthene]-3-one (FL)2,2’-azobis (2-amidinopropane)dihydrochloride (AAPH), chromato-graphic-grade methanol and Folin-Ciocalteu phenol reagent (2 M), p-nitrophenyl-β-D-galactopyranoside (p-NPG), α-glucosidase were obtained from Sigma Aldrich (Shanghai, China). Other analytical grade materials were purchased from Tianjin Kemiou Chemical Reagent Co., Ltd. (Tianjin, China).
Leaf Sample
Leaves of R. roxburghii (GNN5 and SC) were harvested from mountainous areas in Guizhou Province (103° 36′ to 109° 35′ E and 24° 37′ to 29° 13′ N) in late December 2020 and precooled and stored at −80°C for 12 h. Using a vacuum lyophilizer (Christ, Osterode am Harz, Germany) and grinder, a fine powder was made and stored at −20°C.
Extraction of Polyphenols
Free and bound phenolics were extracted according to the method reported by Li et al. (11). For free phenolics, the frozen leaves sample (0.5 g) was mixed with 15 ml of chilled 80% methanol (1% HCl). The mixture was ultrasonicated for 20 min and then centrifuged (4,000 rpm, 20 min, 4°C) to collect the supernatant (three times). The combined supernatants were evaporated using a rotary vacuum evaporator (BUCHI, China) at 35°C, and then dissolved in methanol (final volume, 10 ml) to obtain a free phenolic fraction. After the free phenolic extraction, obtained residues were used to extract bound phenolic compounds by a two-step sequential solvent extraction with base hydrolysis and acid hydrolysis. First, the residue was added to 15 ml NaOH (3 M), after hydrolyzing for 4 h at 30°C under a stream of N2, acidified with HCl to pH 2 (6 M), and then centrifuged at 10,000 rpm for 20 min. The supernatant was extracted by equal volumes of ethyl acetate three times. The upper organic phase was collected and evaporated using a rotary evaporator (BUCHI, China). After base hydrolysis, the remaining residue was hydrolyzed with 15 ml of HCl (3 M) for 1 h at 85°C. Once the hydrolysis was completed, this mixture was acidified to pH 2 with NaOH (6 M) and then immediately centrifuged and extracted by the similar procedures described above. The two parts of extracts obtained by alkaline and acid hydrolysis were combined, evaporated, and resolubilized with 10 ml of methanol, which was considered bound phenolics.
Determination of Total Phenolics Content and Total Flavonoid Content
The total phenolics content was determined by the Folin-Ciocalteu colorimetric method (12). Briefly, 25 μl of sample solution or standard solution and 125 μl of Folin-Ciocalteu reagent were reacted for 10 min at room temperature, and then 125 μl Na2CO3 was added. The reaction was continued for 30 min after shaking by an oscillator. The absorbance was measured at 765 nm using a spectrophotometer (Molecular Devices, United States). The gallic acid was used as a standard (y = 0.0052x + 0.3651, R2 = 0.9982) and expressed as mg GAE (gallic acid equivalents)/g DW (dry weight) of leaves.
The total flavonoid content of the extracts was determined based on the aluminum chloride colorimetric method as previously described (13). Briefly, 25 μl of sample solution or standard was mixed with 110 μl of NaNO2 solution (0.066 M) for 5 min. The mixture was allowed to stand at room temperature for 5 min, and then 15 μl of AlCl3 solution (0.75 M) was added and incubated for 6 min. Then, 100 μl of 0.5 M NaOH solution was added. The absorbance was measured immediately at 510 nm by a spectrophotometer (Molecular Devices, United States). The catechin was used as a standard (y = 0.002x + 0.0341, R2 = 0.9973) and expressed as mg CAE (catechin equivalents)/g DW (dry weight) of leaves.
UPLC–Q–Exactive Orbitrap/MS Analyses
The phenolic compounds of R. roxburghii leaf extracts were characterized and quantified using a UPLC system connected to a Q-Exactive Orbitrap MS (Thermofisher Scientific, China) equipped with an electrospray ionization (ESI) source according to the method from our previous study (14). Separation of phenolics was carried out with an ACQUITY UPLC BEH C18 column (2.1 mm × 100 mm, particle size 1.7 μm, Waters, Milford, MA, United States), eluting with solvent A (acetonitrile) and solvent B (0.1% formic acid in Milli-Q grade water) gradient elution as the mobile phase. The gradient was formed as follows: 0–3 min 95–85% B, 3–11 min 85–70% B, 11–15 min 70–50% B, 15–21 min 50–10% B, 21–22 min 10–95% B. The flow rate was 150 μl/min with a sample injection volume of 2 μl, and the column temperature was equilibrated to 20°C. MS experiments work with the following conditions: auxiliary gas (N2), 10 arb; sheath gas (N2), 35 arb; capillary voltage, 3,200 V; capillary temperature, 320°C; scan range, 100–1,500 m/z.
The total ion current chromatogram was presented in negative ionization mode because of the presence of hydroxyl, glycoside, and/or carboxylic acid groups. Phenolics compounds were identified by comparison of their spectra and retention times with those of externally injected standards such as quininic acid, gallic acid, (-)-gallocatechin, protocatechuic acid, neochlorogenic acid, chlorogenic acid, catechin, cryptochlorogenic acid, p-hydroxybenzoic acid, 6,7-dihydroxycoumarin, caffeic acid, benzoic acid, vanillin, rutin, p-hydroxy-cinnamic acid, p-coumaric acid, ellagic acid, hyperoside, isoquercitrin, ferulic acid, kaempferol -3-o-rutinoside, proanthocyanidins, isoferulic acid, astragaline, quercitrin, phlorizin, quercetin, naringenin, kaempferol, and isorhamnetin. For compounds with no available standards, identification was made using the precise mass of the parent ion [(M–H)] and typical MS fragmentation pattern compared with references and OTCML database. The quantification of the characterized phenolic compounds was carried out by the external calibration curve of each standard. When the standard was not available, the compound quantification was expressed as equivalent to the structurally closest phenolic compound.
Evaluation of Antioxidant Activity
Assay of DPPH Radical Scavenging Activity
The DPPH assay was performed by the reported method (15). In dark conditions, a 100 μl sample or blank solution was mixed with 100 μl DPPH (0.35 M) and incubated for 30 min. Its absorbance was measured at 517 nm using a spectrophotometer (Molecular Devices, United States). The result was calculated as mg Trolox equivalents per gram of dry weight (mg TE/g DW) (Trolox standard curve, y = 0.0204x − 0.0393, R2 = 0.9951).
Assay of ABTS Radical Scavenging Activity
The ABTS assay was performed as previously described (16). A total of 5 ml 3-ethylbenzothiazoline-6-sulfonic acid diammonium salt (ABTS) (7.0 mM) and 88 μl potassium persulphate (140 mM) were allowed to react for 12 h in dark in order to prepare the fresh stock solution. The ABTS working solution was freshly prepared by diluting 80% methanol to get an absorbance of 0.70 ± 0.02 units at 734 nm. A total of 20 μl sample or blank solution was mixed with 200 μl working solution for 6 min. Its absorbance was measured at 734 nm. The results were calculated as mg Trolox equivalents per gram of dry weight (mg TE/g DW) by comparing with the Trolox standard curve (y = 0.0015 x − 0.0492, R2 = 0.9989).
Oxygen Radical Absorbance Capacity Assay
The oxygen radical absorbance capacity (ORAC) assay was performed by the reported method (17). PBS buffer (pH 7.4) was used as the solvent for reagents. A total of 100 μl fluorescein sodium solution (8 mM) and 25 μl sample solution or blank solution were mixed well in a black 96-well plate, and the plate was equilibrated at 37°C for 15 min; then, 75 μl AAPH (119.4 mM) solution was added and recording of fluorescence intensity was started by scheduled recording function of a fluorometer with excitation at 485 nm and emission at 530 nm every 2 min for 2 h. The results were expressed as μmol Trolox equivalents per gram of dry weight (μmol TE/g DW) (Trolox standard curve, y = 303934 x − 647020, R2 = 0.9966).
α-Glucosidase Activity in vitro
The α-glucosidase inhibitory activity in vitro was estimated by the method previously described (18). At 37°C, 20 μl different concentrations of sample or control solution were incubated with 45 μl α-glucosidase (1 U/ml) in 0.1 M pH = 6.8 phosphate buffer solution for 10 min, and the reaction was continued for 20 min after 45 μl p-NPG (2.5 mM) in 0.1 M. Phosphate buffer solution with pH = 6.8 was added. After the addition of 100 μl sodium carbonate (0.2 M), its absorbance was measured at 405 nm. In the control group, PBS buffer was used instead of the sample. Acarbose (0.625–20 mg/ml) was used as the positive control. The α-glucosidase inhibiting activity of the extracts was repressed as a half-inhibition concentration (IC50) value. The α-glucosidase inhibiting activity was calculated according to the following formula:
Statistical Analysis
All assays were conducted in triplicate and repeated three times, with the data reported as mean ± standard deviation (SD). Data were analyzed by one-way analysis of variance (one-way ANOVA) with Tukey’s HSD test to determine the differences between means. Principal component analysis (PCA) and hierarchical cluster analysis (HCA) were performed and images were generated with Origin 2021b (OriginLab, Northampton, MA, United States). Correlation analyses between phenolics and bioactivities were performed using standard Pearson correlation. All statistical analyses were carried out at a significance level of 5% (p ≤ 0.05) using IBM SPSS.
Results and Discussion
Total Phenolic Content and Total Flavonoid Content
Rosa roxburghii leaves rich in phenolics are traditionally processed into healthy beverages, such as tea. However, the qualitative and quantitative analysis of polyphenols in R. roxburghii leaves is limited. Figure 1 shows the phenolic content (free, bound, and total phenolics, and the total phenolic content is the sum of free and bound fractions) of two cultivars of R. roxburghii leaves. The free phenolic content of SC was 94.28 ± 4.01 mg GAE/g DW, which was significantly higher than that of GNN5 with 72.71 ± 5.67 mg GAE/g DW, while the bound phenolic content of SC (11.19 ± 1.66 mg GAE/g DW) was lower than that of GNN5 (17.75 ± 0.29 mg GAE/g DW). A similar trend was observed for flavonoids, which was 27.14 ± 1.76 and 17.38 ± 1.90 mg CAE/g DW (free fraction) and 4.42 ± 0.06 and 5.71 ± 0.06 mg CAE/g DW (bound fraction) for SC and GNN5, respectively (Figure 1).
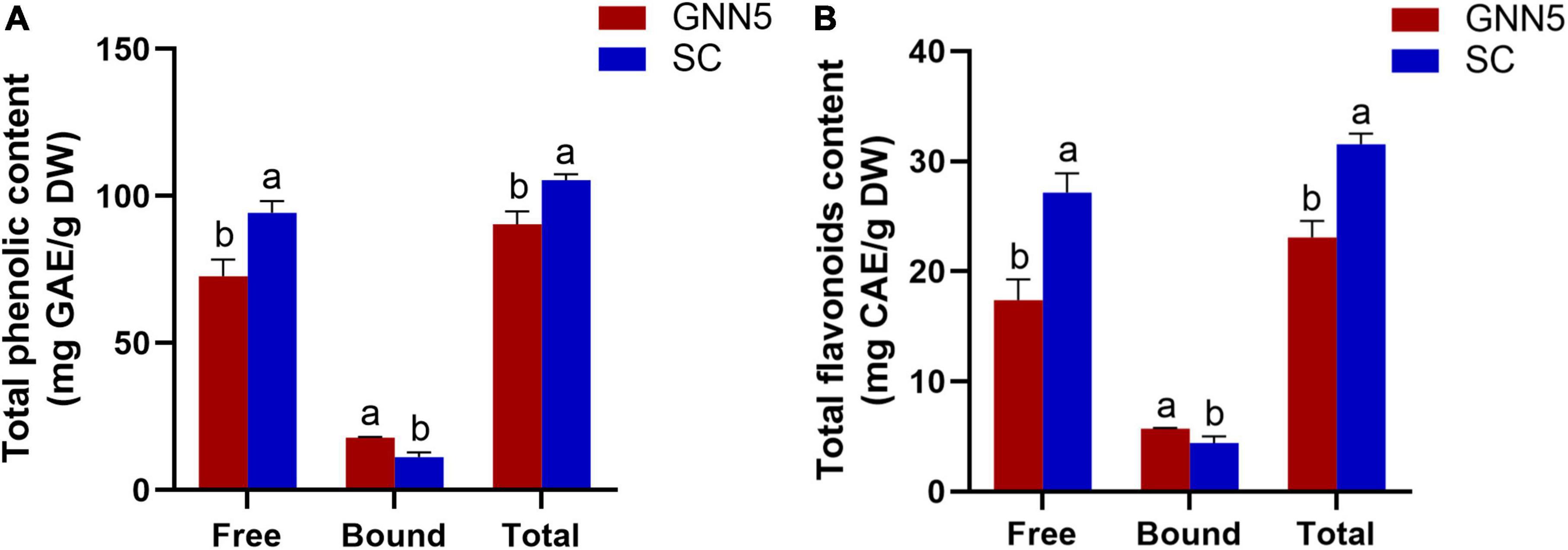
Figure 1. Total phenolic contents (A) and total flavonoid contents (B) of free and bound phenolic fractions extracted from two R. roxburghii leaves. Data represent the mean values ± SD (n = 3), and different letters indicate significant difference (p < 0.05) between two cultivars. Total, the sum of free and bound fractions.
The bound phenolics contributed to 10.61% and 19.62% of TPC in SC and GNN5, resepctively, indicating that there also existed a considerable amount of bound phenolics in addition to the free phenolics in R. roxburghii leaves. Importantly, the average sum of TPC (free + bound) of these two cultivars was 97.97 mg GAE/g DW, which suggested that R. roxburghii leaves are indeed a valuable source of phenolic compounds compared with other foods. For example, these values are greater than that of 14.16–14.54 mg GAE/g DW in mint (19) and comparable to that of 34.78–40.33 and 87.59–88.27 mg GAE/g DW in green tea (20) and raw black tea leaves (21), which were the well-known natural good source of phenolic compounds.
Characterization of Phenolic Compounds
Considering the presence of some possible interfering compounds that react with Folin-Ciocalteu reagent, such as sugars, aromatic amines, and organic acids (22), UPLC-Q-Exactive Orbitrap/MS analysis was conducted to further identify and quantify the compositions of phenolic compounds. A total of 37 individual phenolics were identified in all samples of R. roxburghii leaves (Supplementary Table 1 and Supplementary Figure 1). According to the reference standards (retention times, the accurate mass, and the secondary fragment), 30 individual phenolics were directly identified. They included quininic acid, gallic acid, (-)-gallocatechin, protocatechuic acid, neochlorogenic acid, chlorogenic acid, catechin, cryptochlorogenic acid, p-hydroxybenzoic acid, 6,7-dihydroxycoumarin, caffeic acid, benzoic acid, vanillin, rutin, p-hydroxy-cinnamic acid, p-coumaric acid, ellagic acid, hyperoside, isoquercitrin, ferulic acid, kaempferol -3-o-rutinoside, proanthocyanidins, isoferulic acid, astragaline, quercitrin, phlorizin, quercetin, naringenin, kaempferol, and isorhamnetin. The other seven peaks were tentatively identified by comparing their MS data with those reported in the literature. Peak 7 was characterized as brevifolin carboxylic acid by an [M-H]– ion at m/z 291.0148 and fragment ions at m/z 247.0246 due to the loss of carboxyl moieties (23). Peak 11 (m/z 951.0756) was identified as geranium from its fragment ions at m/z 933.0653 and m/z 300.9991 (24), based on the similar fragmentation pattern reported previously. Peak 12 was preliminarily authenticated as corilagin with parent ion m/z 633.0742 (M–H)– and the fragment ions at m/z 300.9991 (C14H5O8–) and m/z 275.0198 (C13H7O7–) associated with hexahydroxydiphenoyl group of corilagin (25). Peak 16 (m/z 933.065) may be associated with castalagin, with the ions at m/z 915.0599 indicating fragmentation of these core molecules after the release of one water molecule. Peak 26 (m/z 505.0993) was tentatively characterized as quercetin 3-O-6″-acetylglucoside because of its fragment ions at m/z 301.0348, corresponding to the quercetin moiety (26). Peak 29 and peak 32 were identified as kaemperol-3-O-glucuronide and kaempferol-pentoside, respectively, at their [M-H]– ion and the fragment ions at m/z 285.0389 (27). Among the abundant individual phenolics, protocatechuic acid, neochlorogenic acid, brevifolin carboxylic acid, catechin, benzoic acid, ellagic acid, hyperoside, ferulic acid, isoferulic acid, astragaline, quercitrin, quercetin, naringenin, and kaempferol derivative were detected in the free and bound phenolic extracts. (-)-Gallocatechin, chlorogenic acid, corilagin, 6,7-dihydroxycoumarin, castalagin, rutin, quercetin 3-O-6″-acetylglucoside, and phlorizin derivative were found only in the extracts of free phenolics. In addition, caffeic acid, p-hydroxy-cinnamic acid, and p-coumaric acid derivatives were found only in the extracts of bound phenolics (Table 1). Interestingly, many individual phenolics detected in R. roxburghii leaves such as gallic acid, catechin, rutin, p-coumaric acid, hyperoside, isoquercitrin, and kaempferol also abundantly exist in green tea (20).
Quantitative Analysis of Individual Phenolic Compounds in Various Extracts
The quantitative analysis of individual phenolic compounds from R. roxburghii leaves was performed with the corresponding relative standards (Supplementary Table 2). As shown in Table 1, abundant individual phenolics of SC and GNN5 in free form were ellagic acid, quercitrin, quininic acid, isoquercitrin, hyperoside, brevifolin carboxylic acid, and catechin. Compared with those abundant in free phenolics, those abundant phenolics in bound fractions were gallic acid, ellagic acid, and hyperoside for SC and GNN5, respectively. The bound phenolics contributed to 29.50% and 43.67% of total phenolics content in SC and GNN5, respectively. Acosta-Estrada et al. (9) reported that 16.7–76.3% of phenolics compounds exist fundamentally in the bound form in fruits. Considering the effect of bound phenolics on gut health after liberation by microbiota in the colon, exploring bound compounds of R. roxburghii leaves is significant to estimating the scientific support for healthcare. In addition, the total concentration of phenolics detected by UPLC-ESI-MS/MS in R. roxburghii leaves was up to 1,831.55 mg/100 g DW (GNN5) and 1,833.04 mg/100 g DW (SC), which suggested R. roxburghii leaves as a good source of phenolic compounds. The high phenolics (free + bound forms) clearly indicated that R. roxburghii leaves have a great potential application in the development of functional and value-added foods. In addition, the abundant individual phenolics of green tea, including catechins, gallic acid, and ellagic acid (28), were also found in R. roxburghii leaves.
Antioxidant Capacity
The healthy function of polyphenols has been mainly ascribed to their antioxidant activity, which can be measured by scavenging or reducing radical species (e.g., DPPH, ABTS, and ORAC assays) (29). Therefore, DPPH, ABTS, and ORAC assays were employed to assess the antioxidant potential of the different phenolic extracts from R. roxburghii leaves. As summarized in Table 2, the DPPH values of free phenolics in SC (311.80 ± 3.53 mg TE/g DW) and GNN5 (310.15 ± 4.55 mg TE/g DW) were significantly higher than that of their bound phenolics (28.35 ± 0.52 mg TE/g DW and 50.53 ± 1.43 mg TE/g DW) (p < 0.05). However, no significant difference was observed between the two cultivars for free phenolic extracts (p > 0.05). With similar results found in ABTS, ABTS values of free phenolics from SC (1,336.87 ± 70.49 mg TE/g DW) and GNN5 (1,355.48 ± 182.13 mg TE/g DW) exhibited 9.03 and 5.41 times that of their bound phenolics, respectively. Moreover, free phenolics of SC exhibited the highest ORAC value (2,017.38 ± 20.95 μmol TE/g DW), followed by free phenolics of GNN5 (1,730.52 ± 8.57 μmol TE/g DW), bound phenolics of GNN5 (839.58 ± 34.95 μmol TE/g DW), and SC (610.25 ± 23.05 μmol TE/g DW). These values are greater than that of 777.00–1,173.00 μmol TE/g in black tea (30) and comparable to that of 934.00–1,302.60 and 1,060.10–1,125.00 μmol TE/g in oolong tea and green tea (31), respectively, which were normally well-known natural antioxidant products. Overproduction of free radicals relates to oxidative stress and plays a significant role in the development of chronic diseases, such as cardiovascular diseases and diabetes, and dietary antioxidants may block the occurrence and progression of these diseases (32). The results indicated that phenolic extracts of R. roxburghii leaves may be consumed as natural dietary antioxidants.

Table 2. Antioxidant capacity of free and bound phenolic compounds extracted from two R. roxburghii leaves.
α-Glucosidase Inhibition Activity
Located in the small intestine, the role of α-glucosidase is to hydrolyze glycosidic bonds in oligosaccharides and disaccharides and release D-glucose. Glucosidase inhibitors interfere with the role of α-glucosidase, delay the absorption of D-glucose, and reduce postprandial blood glucose levels (18). So far, the widely used drug product is acarbose; for this experiment, it was used as a positive control. As shown in Figure 2, the polyphenolic extracts of R. roxburghii leaves were superior to the positive control in terms of their inhibitory effects. The IC50 values of phenolic extracts of R. roxburghii leaves were significantly lower than that of the positive control, among which free phenolic extracts of SC (39.72 ± 0.59 μg/ml) exhibited the lowest (p < 0.05) IC50 value, which was significantly lower by 99.34% compared with the positive control acarbose (IC50 = 5.98 mg/ml). The α-glucosidase inhibition activity of free phenolics of GNN5 (IC50 = 49.00 ± 1.71 μg/ml) was also significantly lower than that of acarbose, but higher than that of free phenolics of SC. Meanwhile, bound phenolics of SC and GNN5 also possessed higher α-glucosidase inhibitory activity (IC50 = 3.711 ± 0.21 and 4.524 ± 0.30 mg/ml), which were also significantly lower than the positive control acarbose. In line with the previous research (33, 34), phenolics from Rosa roxburghii leaves also exhibited a strong inhibition against the α-glucosidase activity. Previous studies reported that gallic acid, quercitrin, isoquercitrin, and catechin inhibited the α-glucosidase activity and significantly decreased the hydrolysis rates of different starches (35). Ellagic acid (180 mg/day for 8 weeks), as a strong α-glucosidase inhibitor (36), significantly reduced the blood glucose level and insulin resistance of patients with type 2 diabetes (37). Therefore, the α-glucosidase inhibiting activity of R. roxburghii leaf extracts can be mainly attributed to the presence of phenolics compounds. As the raw material of R. roxburghii tea, the good inhibitory effect of R. roxburghii leaves on glucosidase provided strong evidence for the validation of its functional activity.
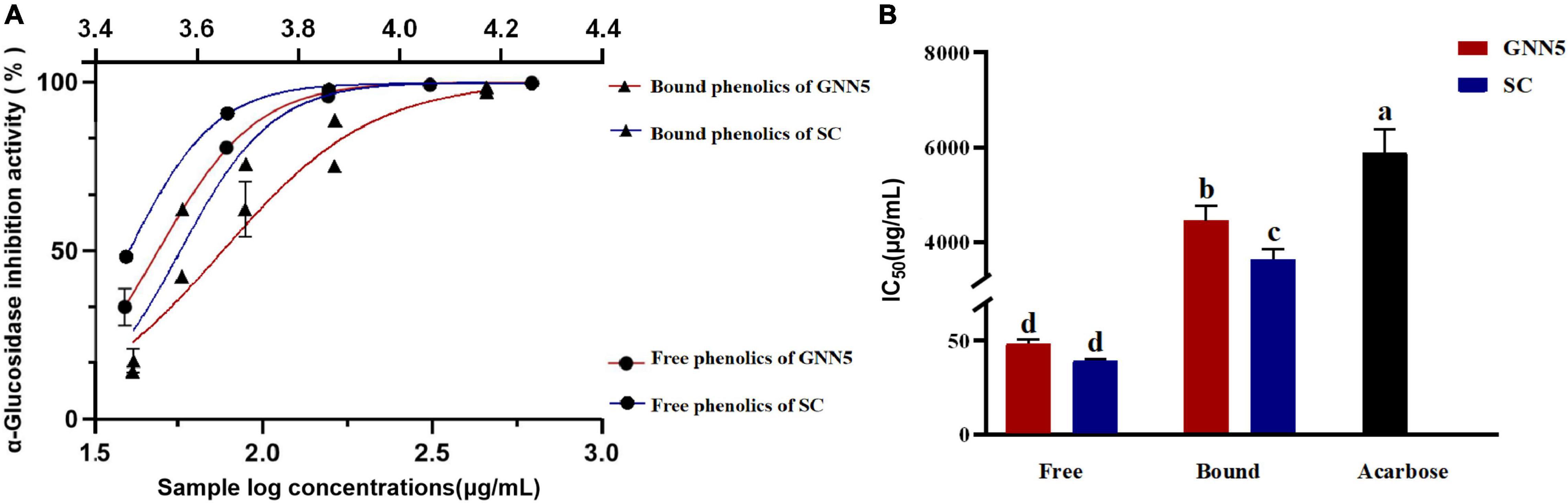
Figure 2. The α-glucosidase inhibitory potency of free and bound phenolic compounds extracted from two R. roxburghii leaves (A). Panel (B) represents the corresponding IC50 values of the samples. Different letters denote statistically significant differences at p < 0.05.
Principal Component Analysis and Hierarchical Cluster Analysis
Principal component analysis, which provided direct visualization of the distribution of individual phenolic compounds and bioactivity of different samples, was performed and the maps were generated (Figures 3A,B). The loading plot of PCA showed that PC1 86.5% and PC2 8.4% accounted for 94.9% of the total variances, which indicated that these two principal components could load maximum information from the original data. The free phenolics obtained from GNN5 and SC were classified into cluster 1, while the bound phenolics extracted from these two cultivars were classified into cluster 2. Two clusters from the same phenolic fraction of different cultivars with intersections indicated that their compositions were similar. With respect to the score plot of PCA, the cosine values may indicate the relationship between two variables (Figure 3B). The results confirmed that the bioactivities of phenolic extracts from R. roxburghii leaves were evidently influenced by TPC and TFC. Among them, quininic acid (1), neochlorogenic acid (5), brevifolin carboxylic acid (7), ellagic acid (21), and hyperoside (22) were significantly correlated with ABTS and DPPH scavenging capacity and ORAC (the antioxidant activities). In addition, quininic acid (1), brevifolin carboxylic acid (7), and catechin (8) were significantly correlated with the α-glucosidase inhibiting activity. This study highlighted the significant effect of phenolics on the biological activities of R. roxburghii leaf extracts.
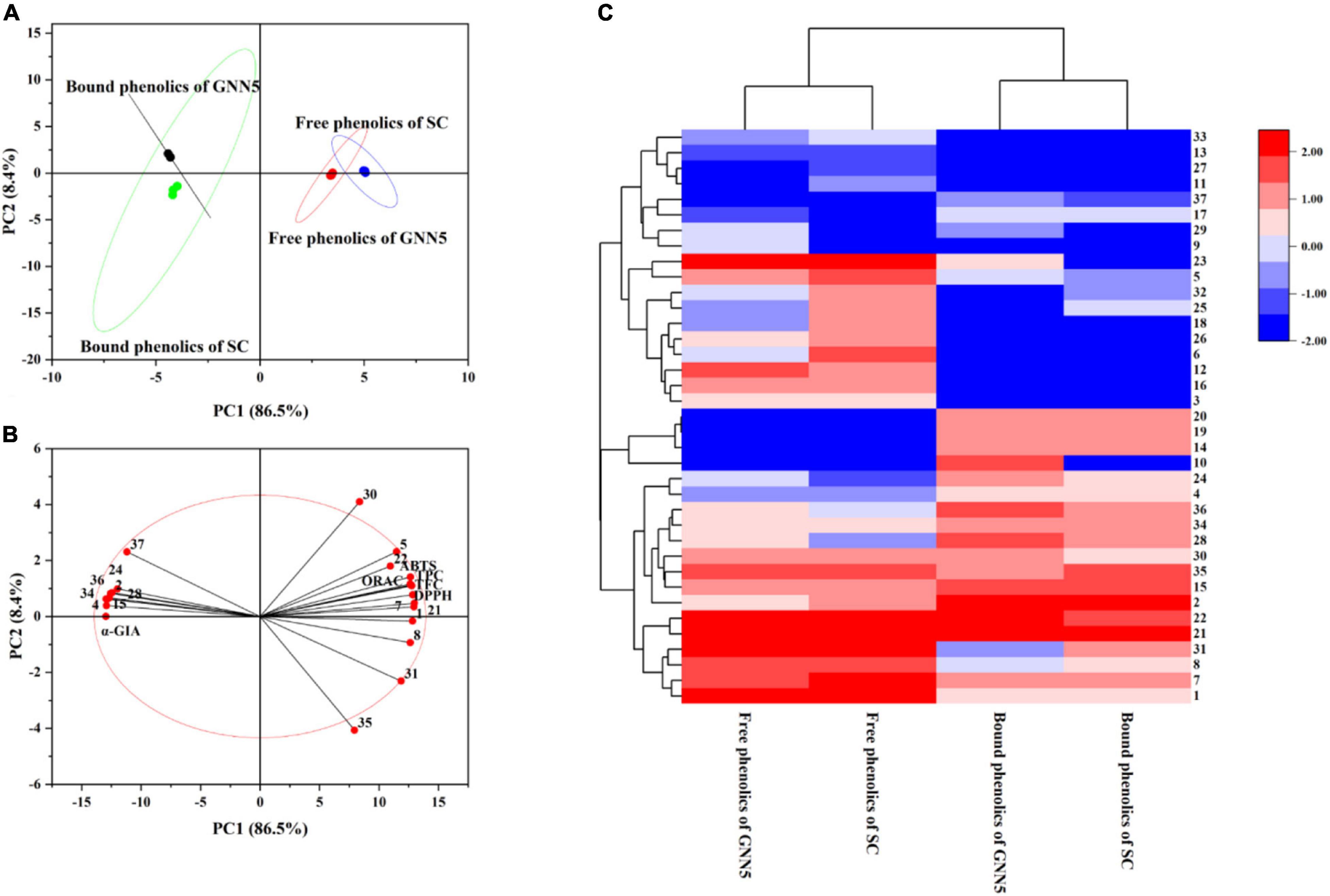
Figure 3. Multivariate analysis on the datasets of two R. roxburghii leaf extracts. (A) The PCA loading plot; (B) the PCA score plot; (C) Hierarchically clustered heat (HCA) map. The component numbers are in one-to-one correspondence with Supplementary Table 1.
In the model of HCA, the data of thirty-seven phenolic compounds in four extractions (Table 1) through standardization were used to conduct analysis, and a hierarchically clustered heat map was obtained as the output (Figure 3C). On the one hand, four extracts were clearly divided into two groups, and the difference between free phenolics and bound phenolics was more significant than that of cultivars, which is consistent with the result of PCA. On the other hand, thirty-seven phenolic compounds were also classified into three groups. For group 1, they were abundant in all samples. Phenolic compounds, including p-hydroxybenzoic acid, caffeic acid, p-hydroxy-cinnamic acid, and p-coumaric acid, from group 2 were only found in bound phenolics, and phenolics of group 3 were almost present in free form. The result of PCA and HCA demonstrated that the bound fraction was indispensable for the evaluation and utilization of phenolic resources in R. roxburghii leaves.
Correlation of Bioactivity With Different Extracts
Table 3 summarizes the correlations between phenolic compounds and bioactivities of leaf extracts from GNN5 and SC. The results showed that the antioxidant activities (DPPH, ABTS, and ORAC) were correlated significantly (p < 0.01) with total phenolics (Folin-Ciocalteu) (r = 0.958–1.000). Positive high linear correlations (p < 0.01) were also observed between the antioxidant activities and total phenolic compounds by UPLC-MS (r = 0.972–0.999). IC50 was used to express α-glucosidase inhibition, and a smaller IC50 value corresponds to stronger α-glucosidase inhibition. Consequently, the negative relationships between IC50 value and total phenolics (Folin-Ciocalteu) (r = −0.988 to −0.998), and total phenolic compounds (UPLC-MS) (r = −0.982 to −0.995) were also observed. The correlations between these bioactivities and phenolic compounds content in R. roxburghii leaf extracts were in line with the previous studies (14). The significant and positive correlations between the total phenolic compounds (UPLC-MS) and total phenolics (Folin-Ciocalteu) from SC and GNN5 implied that the method used to extract the phenolic compounds was effective to remove the non-phenolic interfering substances that could react with Folin-Ciocalteu reagent (38). Moreover, the correlation analysis was conducted to evaluate the major phenolic compound contributors to the bioactivities of R. roxburghii leaves. The major phenolics of SC and GNN5, quininic acid, brevifolin carboxylic acid, ellagic acid, hyperoside, and isoquercitrin correlated significantly (p < 0.01) with the bioactivities (DPPH, ABTS, ORAC, and α-glucosidase inhibition activity) (rDPPH = 0.948–1.000, rABTS = 0.941–0.996, rORAC = 0.937–1.000, rIC50 = −0.935 to −0.997). The results revealed that R. roxburghii leaves can be sources of natural phenolics for utilization in the development of functional foods and nutraceuticals.
Conclusion
For the first time, phenolics fractioned into free and bound forms from R. roxburghii leaves of two cultivars were studied and their antioxidant and α-glucosidase inhibition activities were evaluated. The profiles of free and bound phenolics and their bioactivities were quite contrasting. The content of free and bound phenolics in GNN5 was 72.71 and 17.75 mg GAE/g DW and in SC was 94.28 and 11.19 mg GAE/g DW, respectively. Flavonoids comprised the maximum phenolics from the free fraction, whereas phenolic acids made a similar contribution for free and bound phenolics. A total of 37 phenolic compounds were identified and quantified by UPLC-Q-Exactive Orbitrap/MS in which ellagic acid, quercitrin, isoquercitrin, and quininic acid were abundant in the free phenolic fraction, while the bound phenolic fraction contained more gallic acid, ellagic acid, and hyperoside. In addition, multivariate analysis revealed that a strong correlation existed between the TPC/individual phenolic compounds and bioactivities of the R. roxburghii extracts. Therefore, the characterization and quantification of phenolic compounds in R. roxburghii leaves provide a scientific basis for the traditional practices of the leaves in treating different ailments. The data presented in the phenolic profile of R. roxburghii leaves clearly reveal that this leaf provides good sources of natural antioxidants for the development of functional foods, nutraceuticals, cosmetics, medicine, or high value-added products.
Data Availability Statement
The original contributions presented in this study are included in the article/Supplementary Material, further inquiries can be directed to the corresponding author.
Author Contributions
YY: formal analysis, investigation, data curation, and writing – original draft. WX: visualization. WL: conceptualization and supervision. WH: resources and funding acquisition. RY: writing – review and editing and project administration. All authors contributed to the article and approved the submitted version.
Funding
This study was financially supported by the National Natural Science Foundation of China (31871800) and Lingnan Modern Agricultural Science and Technology experiment project of Guangdong Province (LNSYSZX001): Implementation plan of all natural ingredients determination and functional analysis in Roxburgh rose fruit and honeylocust fruit in the whole growth cycle.
Conflict of Interest
The authors declare that the research was conducted in the absence of any commercial or financial relationships that could be construed as a potential conflict of interest.
Publisher’s Note
All claims expressed in this article are solely those of the authors and do not necessarily represent those of their affiliated organizations, or those of the publisher, the editors and the reviewers. Any product that may be evaluated in this article, or claim that may be made by its manufacturer, is not guaranteed or endorsed by the publisher.
Supplementary Material
The Supplementary Material for this article can be found online at: https://www.frontiersin.org/articles/10.3389/fnut.2022.922496/full#supplementary-material
References
1. Xu J, Vidyarthi SK, Bai W, Pan Z. Nutritional constituents, health benefits and processing of Rosa Roxburghii: a review. J Funct Foods. (2019) 60:103456. doi: 10.1016/j.jff.2019.103456
2. Wu H, Li M, Yang X, Wei Q, Sun L, Zhao J, et al. Extraction optimization, physicochemical properties and antioxidant and hypoglycemic activities of polysaccharides from roxburgh rose (Rosa roxburghii Tratt.) leaves. Int J Biol Macromol. (2020) 165:517–29. doi: 10.1016/j.ijbiomac.2020.09.198
3. Zhou Y. The Study on the Hypoglycemic and Hypolipidemic and Antioxidant Activity Effect from the Tea of Rosa Roxbugrhill Tratt. Guiyang: Guizhou University of China (2017).
4. Wang T, Li Q, Bi K. Bioactive flavonoids in medicinal plants: structure, activity and biological fate. Asian J Pharm Sci. (2018) 13:12–23. doi: 10.1016/j.ajps.2017.08.004
5. Peng X, Zhang G, Liao Y, Gong D. Inhibitory kinetics and mechanism of kaempferol on alpha-glucosidase. Food Chem. (2016) 190:207–15. doi: 10.1016/j.foodchem.2015.05.088
6. Dhameja M, Gupta P. Synthetic heterocyclic candidates as promising alpha-glucosidase inhibitors: an overview. Eur J Med Chem. (2019) 176:343–77. doi: 10.1016/j.ejmech.2019.04.025
7. Shahidi F, Yeo J. Insoluble-bound phenolics in food. Molecules. (2016) 21:1216. doi: 10.3390/molecules21091216
8. Saura-Calixto F. Concept and health-related properties of nonextractable polyphenols: the missing dietary polyphenols. J Agric Food Chem. (2012) 60:11195–200. doi: 10.1021/jf303758j
9. Acosta-Estrada BA, Gutiérrez-Uribe JA, Serna-Saldívar SO. Bound phenolics in foods, a review. Food Chem. (2014) 152:46–55. doi: 10.1016/j.foodchem.2013.11.093
10. Nayak B, Liu RH, Tang J. Effect of processing on phenolic antioxidants of fruits, vegetables, and grains-a review. Crit Rev Food Sci. (2015) 55:887–918. doi: 10.1080/10408398.2011.654142
11. Li W, Yang R, Ying D, Yu J, Sanguansri L, Augustin MA. Analysis of polyphenols in apple pomace: a comparative study of different extraction and hydrolysis procedures. Ind Crop Prod. (2020) 147:112250. doi: 10.1016/j.indcrop.2020.112250
12. You B, Yang S, Yu J, Xian W, Deng Y, Huang W, et al. Effect of thermal and dry salt-curing processing on free and bound phenolics and antioxidant activity in Prunus mume fruits together with the phenolic bioaccessibility. LWT. (2021) 145:111355. doi: 10.1016/j.lwt.2021.111355
13. Zhishen J, Mengcheng T, Jianming W. The determination of flavonoid contents in mulberry and their scavenging effects on superoxide radicals. Food Chem. (1999) 64:555–9. doi: 10.1016/S0308-8146(98)00102-2
14. Yu J, Li W, You B, Yang S, Xian W, Deng Y, et al. Phenolic profiles, bioaccessibility and antioxidant activity of plum (Prunus salicina Lindl). Food Res Int. (2021) 143:110300. doi: 10.1016/j.foodres.2021.110300
15. Brand-Williams W, Cuvelier ME, Berset C. Use of a free radical method to evaluate antioxidant activity. Lebensm Wiss Technol. (1995) 28:25–30. doi: 10.1016/S0023-6438(95)80008-5
16. He Z, Tao Y, Zeng M, Zhang S, Tao G, Qin F, et al. High pressure homogenization processing, thermal treatment and milk matrix affect in vitro bioaccessibility of phenolics in apple, grape and orange juice to different extents. Food Chem. (2016) 200:107–16. doi: 10.1016/j.foodchem.2016.01.045
17. White BL, Howard LR, Prior RL. Polyphenolic composition and antioxidant capacity of extruded cranberry pomace. J Agr Food Chem. (2010) 58:4037–42. doi: 10.1021/jf902838b
18. Kim M, Jo S, Jang H, Lee MS, Kwon Y. Antioxidant activity and alpha-glucosidase inhibitory potential of onion (Allium cepa L.) extracts. Food Sci Biotechnol. (2010) 19:159–64. doi: 10.1007/s10068-010-0022-1
19. Chou O, Ali A, Subbiah V, Barrow CJ, Dunshea FR, Suleria HAR. LC-ESI-QTOF-MS/MS characterisation of phenolics in herbal tea infusion and their antioxidant potential. Fermentation Basel. (2021) 7:73. doi: 10.3390/fermentation7020073
20. Das PR, Islam MT, Lee S, Lee M, Kim J, Eun J. UPLC-DAD-QToF/MS analysis of green tea phenolic metabolites in their free, esterified, glycosylated, and cell wall-bound forms by ultra-sonication, agitation, and conventional extraction techniques. LWT Food Sci Technol. (2020) 127:109440. doi: 10.1016/j.lwt.2020.109440
21. Rumaisa N, Hanim MRN, Hii CL. Superheated steam drying of black tea and quality improvement. Int J Food Eng. (2018) 14:20180185. doi: 10.1515/ijfe-2018-0185
22. Milena V, Tatjana M, Gokhan Z, Ivana B, Aleksandra C, Mohammad MF, et al. Advantages of contemporary extraction techniques for the extraction of bioactive constituents from black elderberry (Sambucus nigra L.) flowers. Ind Crop Prod. (2019) 136:93–101. doi: 10.1016/j.indcrop.2019.04.058
23. Fischer UA, Carle R, Kammerer DR. Identification and quantification of phenolic compounds from pomegranate (Punica granatum L.) peel, mesocarp, aril and differently produced juices by HPLC-DAD-ESI/MSn. Food Chem. (2011) 127:807–21. doi: 10.1016/j.foodchem.2010.12.156
24. Hernandez C, Ascacio-Valdes J, De la Garza H, Wong-Paz J, Noe Aguilar C, Cristian Martinez-Avila G, et al. Polyphenolic content, in vitro antioxidant activity and chemical composition of extract from Nephelium lappaceum L. (Mexican rambutan) husk. Asian Pac J Trop Med. (2017) 10:1201–5. doi: 10.1016/j.apjtm.2017.10.030
25. Yisimayili Z, Guo X, Liu H, Xu Z, Abdulla R, Aisa HA, et al. Metabolic profiling analysis of corilagin in vivo and in vitro using high-performance liquid chromatography quadrupole time-of-flight mass spectrometry. J Pharmaceut Biomed. (2019) 165:251–60. doi: 10.1016/j.jpba.2018.12.013
26. Liu P, Lindstedt A, Markkinen N, Sinkkonen J, Suomela J, Yang B. Characterization of metabolite profiles of leaves of bilberry (Vaccinium myrtillus L.) and lingonberry (Vaccinium vitis-idaea L.). J Agr Food Chem. (2014) 62:12015–26. doi: 10.1021/jf503521m
27. Wang R, He R, Li Z, Lin X, Wang L. HPLC-Q-Orbitrap-MS/MS phenolic profiles and biological activities of extracts from roxburgh rose (Rosa roxburghii Tratt.) leaves. Arab J Chem. (2021) 14:103257. doi: 10.1016/j.arabjc.2021.103257
28. Zhang C, Suen CL, Yang C, Quek SY. Antioxidant capacity and major polyphenol composition of teas as affected by geographical location, plantation elevation and leaf grade. Food Chem. (2018) 244:109–19. doi: 10.1016/j.foodchem.2017.09.126
29. Shahidi F, Ambigaipalan P. Phenolics and polyphenolics in foods, beverages and spices: antioxidant activity and health effects - a review. J Funct Foods. (2015) 18:820–97. doi: 10.1016/j.jff.2015.06.018
30. Serpen A, Pelvan E, Alasalvar C, Mogol BA, Yavuz HT, Gökmen V, et al. Nutritional and functional characteristics of seven grades of black tea produced in turkey. J Agric Food Chem. (2012) 60:7682–9. doi: 10.1021/jf302058d
31. Moldoveanu SC, Oden R. Antioxidant character and levels of polyphenols in several tea samples. ACS Omega. (2021) 6:9982–8. doi: 10.1021/acsomega.0c05818
32. Valko M, Leibfritz D, Moncol J, Cronin MTD, Mazur M, Telser J. Free radicals and antioxidants in normal physiological functions and human disease. Int J Biochem Cell B. (2007) 39:44–84. doi: 10.1016/j.biocel.2006.07.001
33. Giuberti G, Rocchetti G, Lucini L. Interactions between phenolic compounds, amylolytic enzymes and starch: an updated overview. Curr Opin Food Sci. (2020) 31:102–13. doi: 10.1016/j.cofs.2020.04.003
34. Nina N, Theoduloz C, Gimenez A, Schmeda-Hirschmann G. Phenolics from the Bolivian highlands food plant Ombrophytum subterraneum (Aspl.) B. Hansen (Balanophoraceae): antioxidant and alpha-glucosidase inhibitory activity. Food Res Int. (2020) 137:109382. doi: 10.1016/j.foodres.2020.109382
35. Zhou B, Wang F, Jang H. Enhanced antioxidant and antidiabetic activities of barley and wheat after soaking with tea catechin. Food Sci Biotechnol. (2013) 22:1753–61. doi: 10.1007/s10068-013-0277-4
36. Marella S, Hema K, Shameer S, Prasad T. Nano-ellagic acid: inhibitory actions on aldose reductase and alpha-glucosidase in secondary complications of diabetes, strengthened by in silico docking studies. 3 Biotech. (2020) 10:439. doi: 10.1007/s13205-020-02411-1
37. Ghadimi M, Foroughi F, Hashemipour S, Rashidi Nooshabadi M, Ahmadi MH, Ahadi Nezhad B, et al. Randomized double-blind clinical trial examining the Ellagic acid effects on glycemic status, insulin resistance, antioxidant, and inflammatory factors in patients with type 2 diabetes. Phytother Res. (2021) 35:1023–32. doi: 10.1002/ptr.6867
38. Arruda HS, Pereira GA, de Morais DR, Eberlin MN, Pastore GM. Determination of free, esterified, glycosylated and insoluble-bound phenolics composition in the edible part of araticum fruit (Annona crassiflora Mart.) and its by-products by HPLC-ESI-MS/MS. Food Chem. (2018) 245:738–49. doi: 10.1016/j.foodchem.2017.11.120
Keywords: phenolic profiles, antioxidant activity, alpha-glucosidase, UPLC-Q-Exactive Orbitrap/MS, Rosa roxburghii Tratt leaves
Citation: Yang Y, Li W, Xian W, Huang W and Yang R (2022) Free and Bound Phenolic Profiles of Rosa roxburghii Tratt Leaves and Their Antioxidant and Inhibitory Effects on α-Glucosidase. Front. Nutr. 9:922496. doi: 10.3389/fnut.2022.922496
Received: 18 April 2022; Accepted: 03 June 2022;
Published: 28 June 2022.
Edited by:
Michael Rychlik, Technical University of Munich, GermanyReviewed by:
Yun Ping Neo, Taylor’s University, MalaysiaRadjassegarin Arumugam, A. V. C. College, India
Copyright © 2022 Yang, Li, Xian, Huang and Yang. This is an open-access article distributed under the terms of the Creative Commons Attribution License (CC BY). The use, distribution or reproduction in other forums is permitted, provided the original author(s) and the copyright owner(s) are credited and that the original publication in this journal is cited, in accordance with accepted academic practice. No use, distribution or reproduction is permitted which does not comply with these terms.
*Correspondence: Ruili Yang, cmx5YW5nNzdAc2NhdS5lZHUuY24=
†These authors have contributed equally to this work