- 1Sino-Norway Joint Lab on Fish Gut Microbiota, Institute of Feed Research, Chinese Academy of Agricultural Sciences, Beijing, China
- 2Norway-China Joint Lab on Fish Gastrointestinal Microbiota, Institute of Biology, Norwegian University of Science and Technology, Trondheim, Norway
- 3Faculty of Biosciences, Fisheries, and Economics, Norwegian College of Fisheries Science, UiT The Arctic University of Norway, Tromsø, Norway
- 4Key Laboratory for Feed Biotechnology of the Ministry of Agriculture and Rural Affairs, Institute of Feed Research, Chinese Academy of Agricultural Sciences, Beijing, China
High-fat diets (HFD) are harmful to fish health. Probiotics are commonly utilized to improve fish nutrition metabolism, immune response, and health. Nucleic acids of the probiotic bacterium can be hydrolyzed by nuclease to generate nucleotides. The present study aimed to evaluate the effects of stabilized fermentation product of nuclease-treated Cetobacterium somerae XMX-1 [XMX-1 (N)] on growth, non-specific immunity, and liver health of zebrafish (Danio rerio). Compared to the HFD group, 100 g/kg XMX-1 (N) significantly increased weight gain and decreased feed conversion ratio (FCR). However, 5 or 10 g/kg XMX-1 (N) had no influence on zebrafish growth. In addition, supplementation of 100 g/kg XMX-1 (N) significantly increased lysozyme activity and total antioxidant capacity in skin mucus, and the expression of inflammation related genes interleukin 1 beta (IL-1β), interleukin 10 (IL-10), and interleukin 6 (IL-6) in the gut as well as fatty acid oxidation related genes uncoupling protein 2 (UCP2) and proliferator-activated receptor γ coactivator 1α (PGC1α) in the liver, while decreased the content of hepatic triacylglycerol (TAG) in zebrafish. The gene sequencing, 16S rRNA, showed that 100 g/kg XMX-1 (N) enhanced the relative abundance of Firmicutes while lowered Proteobacteria and Actinobacteria. 10 g/kg XMX-1 (N) significantly increased lysozyme activity and complement component 4 (C4) in skin mucus, and intestinal expression of inflammation-related genes. In the 5 g/kg XMX-1 (N) group, however, only an increase in C4 level in skin mucus was observed. Together, these results reveal that dietary supplementation with nuclease-treated C. somerae XMX-1 (N) has a dose-dependent beneficial effect on fish health.
Introduction
With the widespread adoption of large-scale and intense fish culture practices, the phenomena of excessive body fat deposition in farmed fish have become quite frequent, resulting in fatty liver, which may affect fish health and reduce harvest yields (1–4). Excessive fat accumulation has been reported to cause hepatic and intestinal oxidative stress (5, 6), and affect normal gut microbiota leading to dysbiosis (7, 8). Therefore, it is significant to seek strategies to mitigate the negative consequences of high-fat diets. The previous studies have shown that probiotics can maintain gut microbiota homeostasis and ameliorate fatty liver, as well as improve liver function of fish (6, 9, 10). In addition, probiotics have been reported to improve growth, intestinal health, the immune system, and prevent diseases (11–14).
Cetobacterium is an indigenous dominant bacterium in the gut microbiota of fish (15, 16). Cetobacterium and its metabolite acetate improved glucose homeostasis through parasympathetic activation in zebrafish (17). In our previous studies, dietary supplemented fermentation product of Cetobacterium somerae can reverse the negative effect associated with higher plant proteins addition by improving gut and liver health, as well as reducing liver lipid deposition of common carp (Cyprinus carpio) (18). In zebrafish, the addition of stabilized fermentation product of C. somerae can improve gut and liver health and antiviral immunity, suggesting C. somerae can be used as a potential probiotic to improve fish health (19). Although the beneficial effects of C. somerae have been characterized, the associated effector molecules of C. somerae are largely unknown, and it is practical to improve the function of C. somerae fermentation product by a treatment targeting potential beneficial components.
Nucleic acids of the probiotic bacterium can be hydrolyzed by nuclease to produce nucleotides and other metabolites (20–23). Although nucleotides are low-molecular-weight compounds, they are extremely important in organisms and many biological activities rely on nucleotides and their associated metabolites (24, 25). Consistent with the results in mammals (26, 27), supplementation of nucleotides in fish and shrimp feed can stimulate growth, improve intestinal health, and boost anti-stress as well as immune responses (28). A recent study also showed that dietary nucleotides reduced hepatic steatosis in zebrafish (29).
The aim of the study is to further improve the availability of nucleotides in the fermentation product of C. somerae by nuclease treatment, and assess the effects of nuclease-treated C. somerae fermentation product on zebrafish health. To our knowledge, this is the first study about the beneficial role of the commensal bacteria C. somerae treated with nuclease in fish. Overall, the findings might help to promote the utilization of nuclease-treated C. somerae fermentation product as a feed supplement.
Materials and Methods
Bacteria Culture and Nuclease Treatment
Cetobacterium somerae XMX-1, with the preservation number CGMCC No. 18908 in the China General Microbiological Culture Collection Center, was anaerobically cultivated in an anaerobic incubator (Electrotek, England) growing in Glfu Anaerobic Medium (GAM) Broth medium (Haibo, China) at 28°C for 18 h to reach the concentration of 108 CFU/ml. The protocols for obtaining stabilized fermentation product of C. somerae were conducted as previously described (19).
The stabilized fermentation product of C. somerae was treated with 3% nuclease (Nanning Pang Bo Bioengineering Co., Ltd., Guangxi, China) with the marker of 50,000 U/g in a 60°C incubator for 48 h. The concentration of nucleotides was measured at 48 h after treatment of ultrasonic crushing, which was shown as 58.2 μg/g. In contrast, the concentration of nucleotides was 44.2 μg/g without the treatment of nuclease. Proximate composition analysis of the fermentation product of C. somerae and nuclease-treated fermentation product of C. somerae was listed in Table 1. The moisture content was measured using the drying method (GB/T 6435-2014); crude ash was determined according to the ashing method (GB/T 6438-2007); crude fat was determined by the Soxhlet extraction method (GB/T 6433-2006); crude protein was measured by the Kjeldahl method (GB/T 6432-1994); crude fiber was measured using filter bag technology (GB/T 6434-2006). Finally, stabilized fermentation product of C. somerae and the nuclease-treated stabilized fermentation product of C. somerae were added to the diet, respectively.

Table 1. Proximate composition analysis of the fermentation product of C. somerae and nuclease-treated fermentation product of C. somerae (dry matter, g/kg)a.
Zebrafish Husbandry and Experimental Diets
In Experiment 1, zebrafish with 0.06 ± 0.01 g average weight were randomly allocated into 6 groups. In each group, there were 3 replicate tanks and 15 fish in each tank. Zebrafish in the control group were fed with a high-fat diet and the XMX-1 groups were fed with a high-fat diet supplemented with a 5 or 10 g XMX-1/kg diet. In the XMX-1 (N) groups, zebrafish were fed with a high-fat diet supplemented with a 5 or 10 g XMX-1 (N)/kg diet. The basal dietary formulation and proximate composition analysis were given in Table 2.
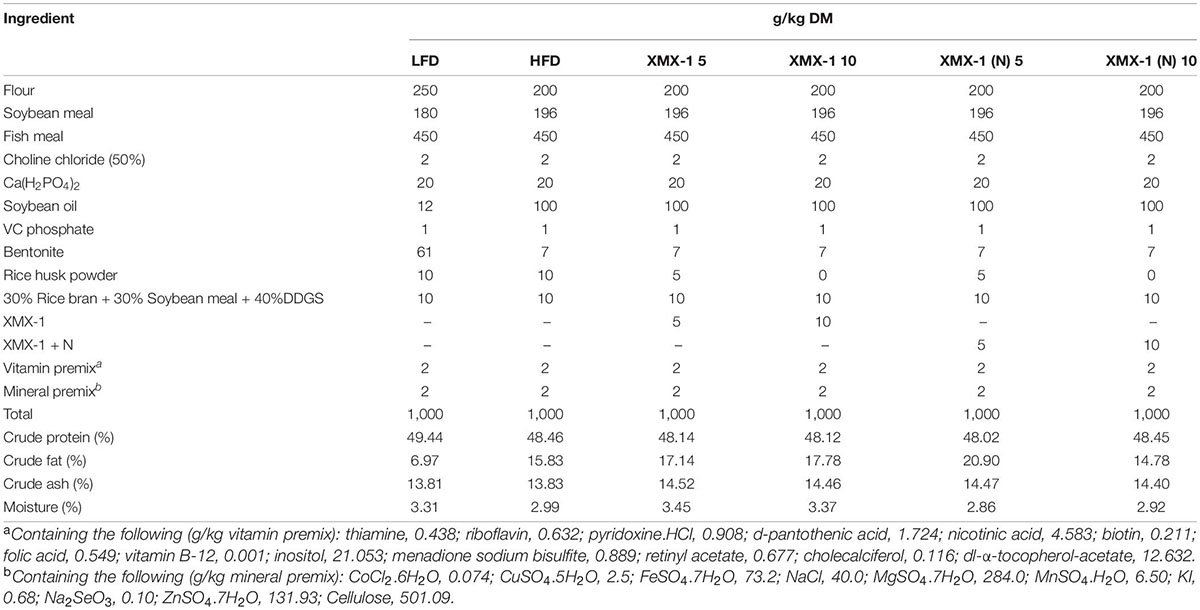
Table 2. Feed formulation and proximate composition analysis of diets for zebrafish (dry matter, g/kg).
In Experiment 2, 360 zebrafish (average initial mass 0.026 ± 0.0 g) were randomly divided into 12 tanks, each having three randomly assigned replicate tanks per treatment and 15 fish in each tank. In the XMX-1 and XMX-1 (N) groups, zebrafish were fed with a high-fat diet supplemented with a 100 g XMX-1/kg diet and 100 g XMX-1 (N)/kg diet, respectively. The basal dietary formulation and proximate composition analysis were given in Table 3.
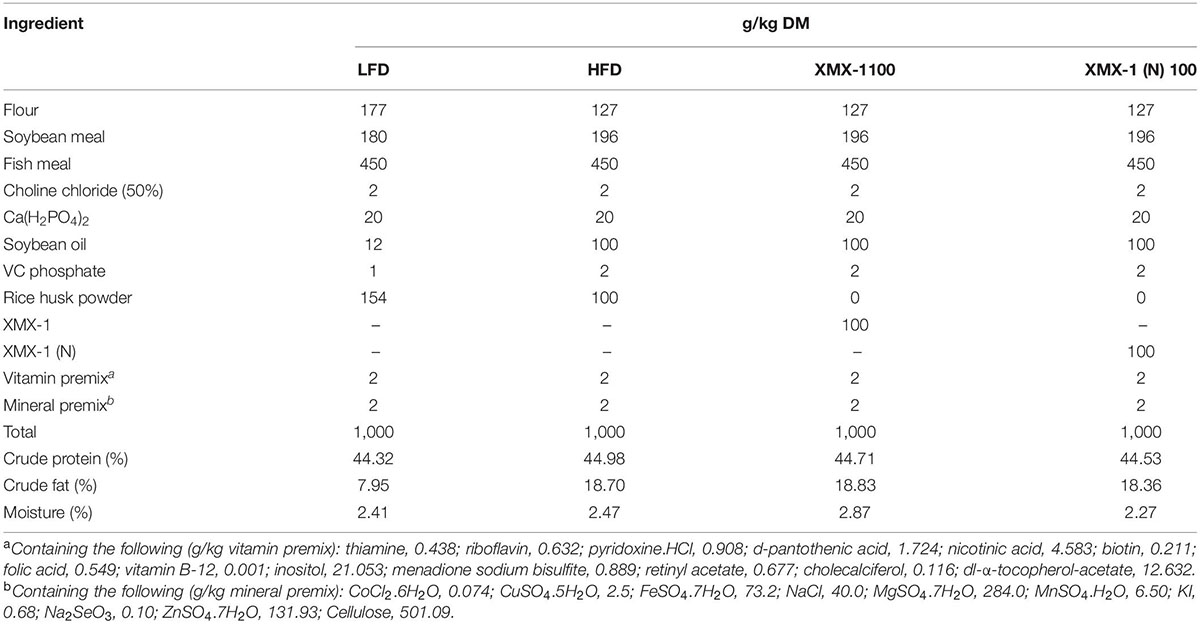
Table 3. Feed formulation and proximate composition analysis of diets for zebrafish (dry matter, g/kg).
In both experiments, zebrafish were fed with diets at a ratio of 6% of body weight at 09:00 and 16:00 every day in a recirculating system with water temperature 28–28.5°C, pH 7.0–7.5, dissolved oxygen ≥ 6 mg/L and a 12 h/12 h light/dark cycle.
Growth Measurements
Zebrafish were weighed at the start of the experiment. After a 3-week feeding, the fish was weighed after 24 h fasting. The survival rate, weight gain, and feed conversion rate of fish were evaluated as previously described (18).
Determination of Total Antioxidant Capacity (T-AOC), Lysozyme, and Complement Component 4 in Skin Mucus
Skin mucus was collected from 24 fish in each group with 6 replicates. The T-AOC content was determined according to the manufacturer’s instructions using a kit (Cominbio, Suzhou, China). Assay kits were used to determine the levels of lysozyme and C4 (Jiancheng Bioengineering Ins., China).
Determination of Liver Triacylglycerol Content
The liver samples of zebrafish were weighed and homogenized in phosphate buffered saline (PBS). The TAG was extracted according to the protocol described previously (30). To quantify TAG, free glucose reagent (Sigma–Aldrich, Shanghai) and triglyceride reagent (Sigma–Aldrich, Shanghai) were utilized. The concentrations of TAG are shown as μg/mg liver.
Total RNA Extraction, Reverse Transcription, and qRT-PCR Analysis
Trizol (Invitrogen, Carlsbad, CA, United States) was used to extract total RNA from zebrafish liver and intestine. The cDNA synthesis and the quantitative real-time PCR response were conducted using the previously published procedures (30). Table 4 contains a list of primer sequences. The 2–ΔΔCTmethod was used to analyze the data. The reference gene was ribosomal protein S11 (RPS11). GraphPad Prism 8.0 software was used to analyze the data.
The 16S rRNA Gene Sequencing
Six hours following the last feeding, the gut content was taken from each treatment group. The gut microbiota was studied using 16S rRNA gene sequencing. The protocols for DNA extraction, sequencing, and data analysis were carried out as described previously (30). Microbiota sequencing data in this study are available from the National Center for Biotechnology Information (NCBI) under accession numbers: PRJNA 825074 (Experiment 1) and PRJNA 824236 (Experiment 2).
Statistical Analysis
The data were presented as mean ± SEMs. GraphPad Prism 8.0 and Microsoft Office Excel 2010 software were used to generate all statistics from at least three different tests. The Student’s t-test was used to compare the differences between the two groups. The difference with p-value less than 0.05, was considered significant.
Results
Effects of XMX-1 and XMX-1 (N) Diets on Growth of Zebrafish
Feeding zebrafish which have similar initial body weight (Figure 1A), a high-fat diet led to a higher weight gain and lower FCR compared with the control group (Figures 1B,C, p < 0.05). The addition of 5 or 10 g/kg XMX-1 and 5 g/kg or 10 g/kg XMX-1 (N) had no effect on zebrafish growth (Figures 1B,C, p > 0.05). Interestingly, 100 g/kg XMX-1 or 100 g/kg XMX-1 (N) supplementation significantly increased weight gain and decreased FCR of zebrafish with similar initial body weight (Figure 1E) compared with the HFD group (Figures 1F,G, p < 0.05). The survival rate of fish in all treatments has no significant differences compared to the HFD group (Figures 1D,H, p > 0.05).
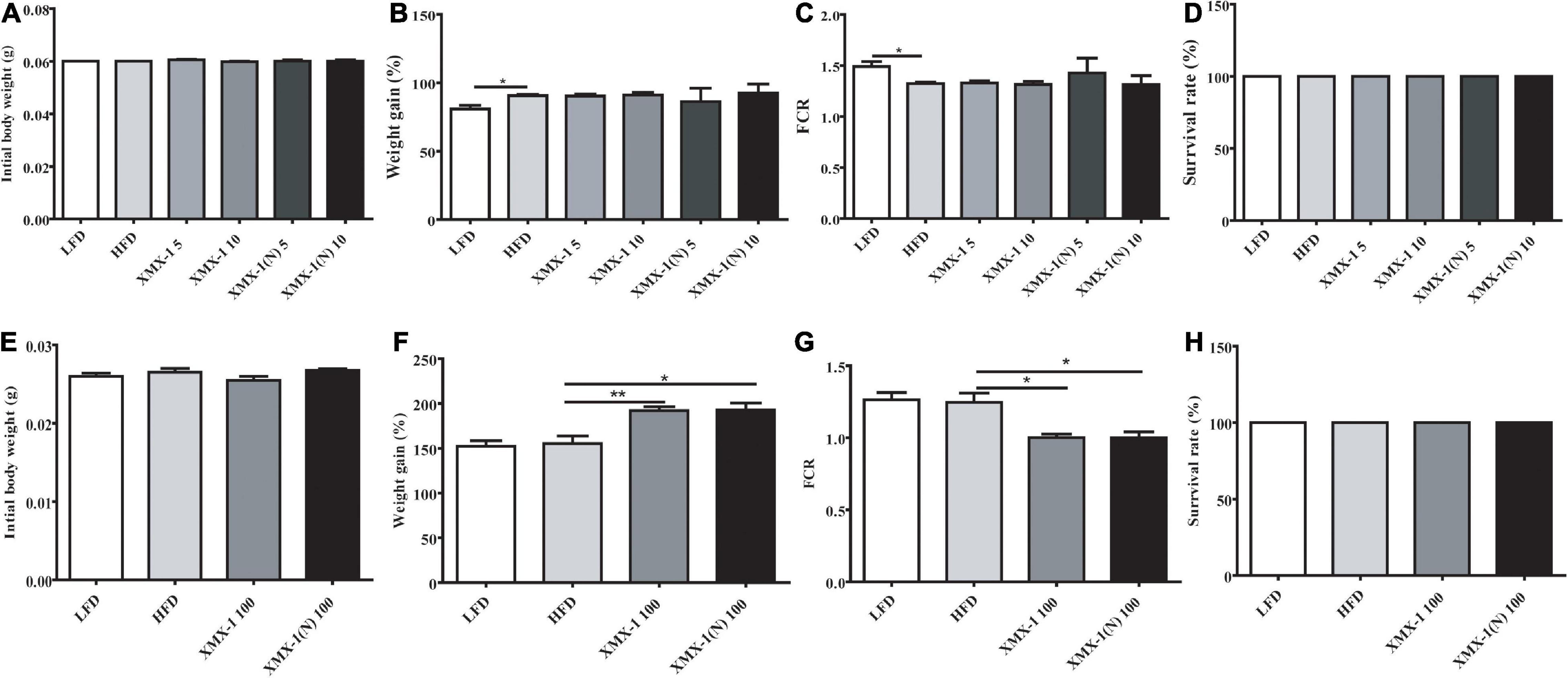
Figure 1. Effects of XMX-1 and XMX-1 (N) diets on zebrafish survival, weight gain (%), and feed conversion ratio. (A) Initial body weight (g), (B) weight gain (percentage), (C) feed conversion ratio (FCR), and (D) survival rate with low dosage addition. (E) Initial body weight (g), (F) weight gain (percent), (G) feed conversion ratio (FCR), and (H) survival rate with high dosage addition. The means (SEMs) (n = 3) were used to represent the data. *p < 0.05 and **p < 0.01 comparison to the control group.
Effects of XMX-1 and XMX-1 (N) Diets on the Non-specific Immunity of Zebrafish
Dietary 5 or 10 g/kg XMX-1, and 5 g/kg XMX-1 (N) had no effect on skin mucus lysozyme activity compared with the HFD group (Figure 2A, p > 0.05). Lysozyme activity increased significantly in the 10 g/kg XMX-1 (N) group as compared to the HFD group (Figure 2A, p < 0.05). Furthermore, the supplementation with 5 g/kg or 10 g/kg XMX-1 and 5 g/kg or 10 g/kg XMX-1 (N) significantly enhanced the level of C4 in zebrafish skin mucus (Figure 2B, p < 0.05). The fish fed with a 100 g/kg XMX-1 diet had no effect on lysozyme activity and the total antioxidant capacity (Figures 2C,D, p > 0.05). In contrast, the dietary XMX-1 (N) at 100 g/kg significantly increased lysozyme activity and total antioxidant capacity compared with the HFD group (Figures 2C,D, p < 0.05).

Figure 2. Effects of XMX-1 and XMX-1 (N) diets on zebrafish body surface mucus immunity. (A) Lysozyme activity and (B) complement component 4 with low dosage addition. (C) Lysozyme activity and (D) total antioxidant capacity with high dosage addition. The means (SEMs) (n = 6) were used to represent the data. *p < 0.05 and ***p < 0.001 comparisons to the control group.
The expression of inflammation-related genes such as tumor necrosis factor alpha (TNF-α), interleukin 1 beta (IL-1β), interleukin 10 (IL-10), and transforming growth factor beta (TGF-β) was significantly higher in the 10 g/kg XMX-1 (N) group (Figures 3A–D, p < 0.05). Moreover, feeding 100 g/kg XMX-1, didn’t affect TNF-α expression (Figure 3E, p > 0.05) but significantly increased the expression of IL-10 (Figure 3H, p < 0.05), while the expression of IL-1β, IL-10, and interleukin 6 (IL-6) was significantly increased in the 100 g/kg XMX-1 (N) group compared with the HFD group (Figures 3F–H, p < 0.01).
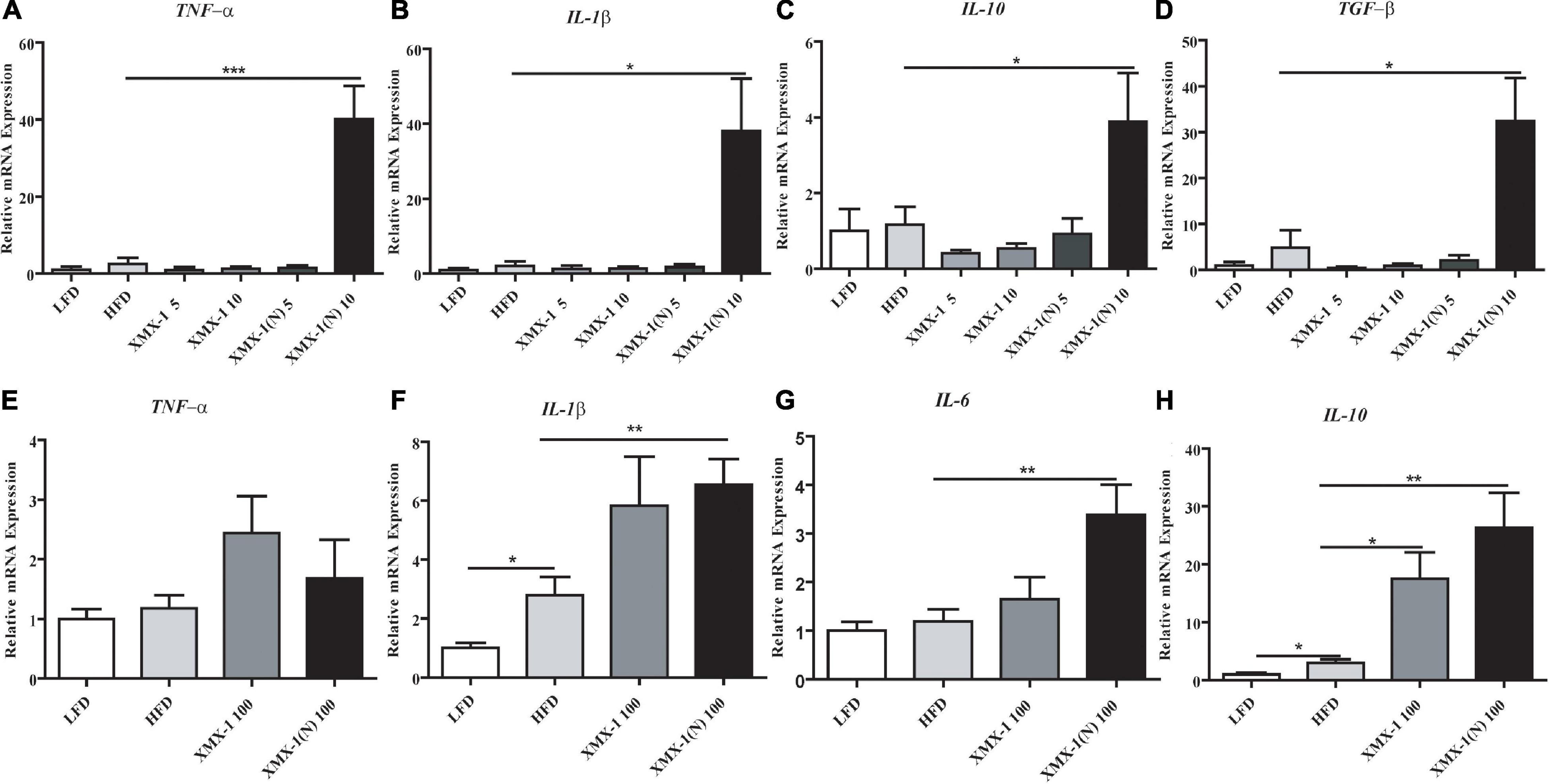
Figure 3. Effects of XMX-1 and XMX-1 (N) diets on the expression of intestinal inflammation-related genes of zebrafish. (A) TNF-α, (B) IL-1β, (C) IL-10, and (D) TGF-β with low dosage addition. (E) TNF-α, (F) IL-1β, (G) IL-6, and (H) IL-10 with high dosage addition. The means (SEMs) (n = 6) were used to represent the data. *p < 0.05, **p < 0.01, and ***p < 0.001 comparison to the control group.
Effects of XMX-1 and XMX-1 (N) Diets on the Gut Microbiota of Zebrafish
Figure 4A shows that supplementation with 5 and 10 g/kg XMX-1 increased the relative abundance of Actinobacteria compared with the HFD group. Dietary XMX-1 at 10 g/kg reduced the relative abundance of Proteobacteria while increased the relative abundance of Firmicutes. Proteobacteria, Actinobacteria, and Firmicutes were found to be more abundant in the 5 g/kg XMX-1 (N) treatment. A 10 g/kg XMX-1 (N) increased the relative abundance of Proteobacteria and Fusobacteria. Furthermore, there were significant differences among groups based on PCoA analysis, indicating that the XMX-1 and XMX-1 (N) diets had substantial effects on the autochthonous microbiota (Figure 4B, p < 0.05).
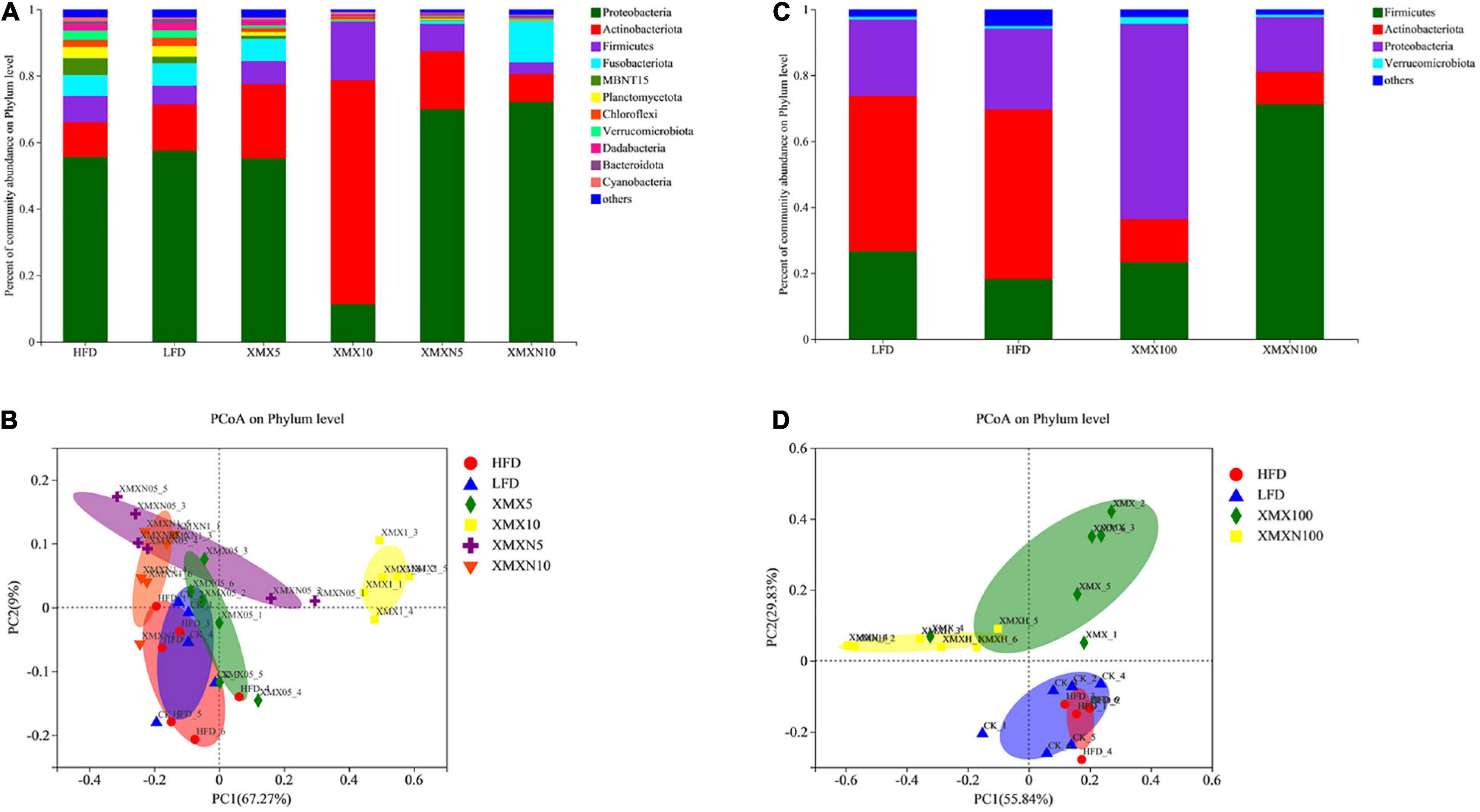
Figure 4. Effects of XMX-1 and XMX-1 (N) diets on the gut microbiota of zebrafish. (A) Relative abundance; (B) principal coordinates analysis (PCoA) at phylum level of the gut microbiota with low dosage addition. (C) Relative abundance; (D) PCoA at phylum level of the gut microbiota with high dosage addition.
Dietary 100 g/kg XMX-1 enhanced the relative abundance of Firmicutes and Proteobacteria while decreased the relative abundance of Actinobacteria (Figure 4C). The supplementation 100 g/kg XMX-1 (N) enhanced the relative abundance of Firmicutes while decreased the relative abundance of Proteobacteria and Actinobacteria (Figure 4C). Furthermore, PCoA analysis revealed substantial differences among groups, indicating that 100 g/kg XMX-1 and 100 g/kg XMX-1 (N) diets had significant effects on the autochthonous microbiota compared with HFD control (Figure 4D, p < 0.05).
Effects of XMX-1 and XMX-1 (N) Diets on the Liver Health of Zebrafish
As can be seen in Figure 5, dietary 5 or 10 g/kg XMX-1 and 5 or 10 g/kg XMX-1 (N) reduced the expression of inflammation-related genes in zebrafish liver compared with the HFD group (Figures 5A–D, p < 0.05). In contrast, the fish fed with a 100 g/kg XMX-1 diet increased IL-10 expression (Figure 5H, p < 0.01). Furthermore, IL-6 and IL-10 expressions were considerably elevated in the 100 g/kg XMX-1 (N) diet group (Figures 5F,H, p < 0.01).
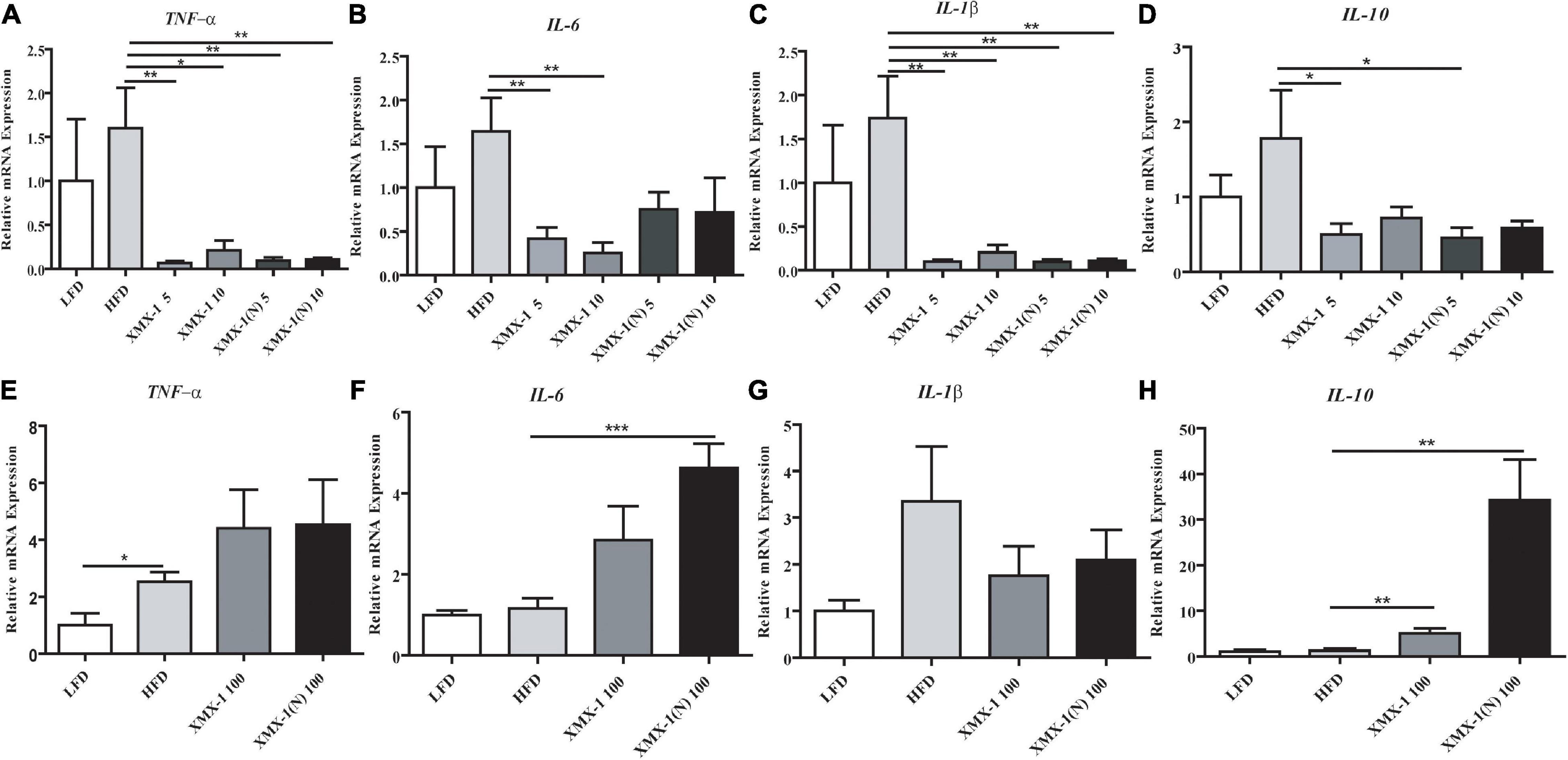
Figure 5. Effects of XMX-1 and XMX-1 (N) diets on the expression of liver inflammation-related genes of zebrafish. (A) TNF-α, (B) IL-6, (C) IL-1β, and (D) IL-10 with low dosage addition. (E) TNF-α, (F) IL-6, (G) IL-1β, and (H) IL-10 with high dosage addition. The means (SEMs) (n = 6) were used to represent the data. *p < 0.05, **p < 0.01, and ***p < 0.001 comparison to the control group.
The TAG content of zebrafish liver was significantly reduced in the 5 or 10 g/kg XMX-1 groups and the 5 or 10 g/kg XMX-1 (N) groups compared with the HFD group (Figure 6A, p < 0.05). In addition, we detected the expression of genes involved in lipid metabolism. In comparison to the HFD group, the addition of 5 or 10 g/kg XMX-1 and 5 or 10 g/kg XMX-1 (N) significantly increased the expression of genes such as fatty acid synthase (FAS), acetyl-CoA carboxylase (ACC), peroxisome proliferator-activated receptor gamma (PPARγ), uncoupling protein 2 (UCP2), carnitine palmitoyl transferase 1 (CPT1), and peroxisome proliferator-activated receptor alpha (PPARα) (Figures 6B–G, p < 0.05).
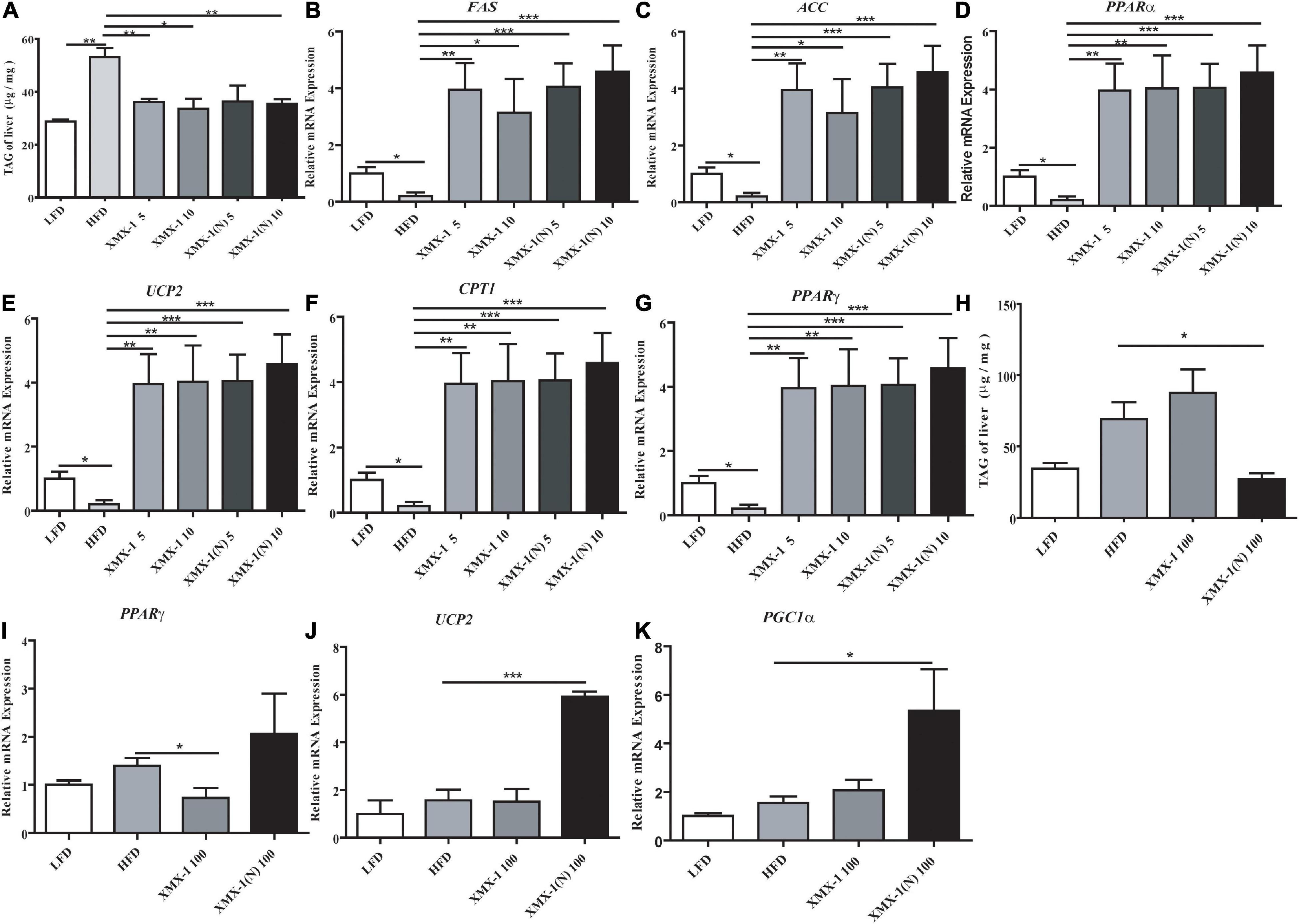
Figure 6. Effects of XMX-1 and XMX-1 (N) diets on the expression of the content of TAG and hepatic lipid metabolism related genes of zebrafish liver. (A) TAG, (B) FAS, (C) ACC, (D) PPARα, (E) UCP2, (F) CPT1, and (G) PPARγ with low dosage addition. (H) TAG, (I) PPARγ, (J) UCP2, and (K) PGC1α with high dosage addition. The means (SEMs) (n = 6) were used to represent the data. *p < 0.05, **p < 0.01, and ***p < 0.001 comparison to the control group.
Compared with the HFD group, 100 g/kg XMX-1 (N) significantly decreased the content of TAG of zebrafish (Figure 6H, p < 0.05). Moreover, the dietary 100 g/kg XMX-1(N) didn’t affect PPARγ expression (Figure 6I, p > 0.05) but significantly increased the expression of lipolysis genes such as UCP2 and proliferator-activated receptor γ coactivator 1α (PGC1α) (Figures 6J,K, p < 0.05).
Discussion
In the present work, feeding C. somerae XMX-1 improved the non-specific immunity and liver health, as well as reduced lipid deposition in HFD-diet zebrafish. In addition, the effect of XMX-1 supplementation on fish growth was dose dependent. These favorable effects of XMX-1, along with the previous results (18, 19) indicate that the fermentation product of C. somerae has a great potential for improving fish health when used as feed additives. To further improve the function of the fermentation product of C. somerae, nuclease was used to treat the fermentation product and produce nucleotides. Compared to XMX-1, the concentration of nucleotides was elevated by 31.67% after the treatment of nuclease in XMX-1 (N). It has been reported that the dietary nucleotides can enhance growth, increase stress and disease resistance, modulate immune functions, and improve intestinal morphology and gut microbiota of fish (28). In particular, nucleotides have been reported to enhance growth in zebrafish (29), grouper (Epinephelus malabaricus) (31), red drum (Sciaenops ocellatus) (32), amberjack (Seriola dumerili) (33) and Nile tilapia (Oreochromis niloticus) (34). Interestingly, dietary 100 g/kg XMX-1 (N) significantly increased weight gain and decreased FCR compared to the HFD diet, while supplementation with low levels of C. somerae XMX-1 (N) at 5 or 10 g/kg had no influence on the growth of zebrafish. The dose effect of XMX-1 (N) on growth performance is correlated with the dose response of dietary nucleotides on growth of fish. For instance, Tahmasebi-Kohyani et al. added different levels of supplemented nucleotides (0.5, 1, 1.5, and 2 g/kg) to the diet of rainbow trout (Oncorhynchus mykiss) and found that the percentage of body weight gain and feed efficiency of fish was better when the fish were fed with 1.5–2 g/kg diets (35). Notably, the nucleotide levels in 5–100 g/kg XMX-1 (N) are in a similar range as the doses used in the studies investigating dietary nucleotides (33–35). Therefore, the nucleotides may contribute to the beneficial effects of nuclease-treated C. somerae. There are some explanations for how nucleotides work to improve fish growth, such as being feed attractants (36), supporting high rate of cell replication of fish (28), higher hepatic insulin-like growth factor 1 (IGF-1) gene expression (37), and reduced standard metabolic rate (38).
Fish live in an aqueous environment that is rich in pathogens, and lysozyme is critical in the defense against infection (39–41). Complement activity can trigger cellular defense and affect fish phagocytosis (42). In the current study, higher lysozyme activity and C4 were observed in the fish fed with an XMX-1 (N) diet than in fish on the HFD diet, and dietary 100 g/kg XMX-1 (N) significantly enhanced the antioxidant capacity of zebrafish. Dietary nucleotides have been reported to increase the lysozyme and complement the activities in common carp (43). Cheng et al. found that red drum fed with 5 g/kg of nucleotides had higher lysozyme activity (44). In addition, nucleotides supplementation can improve the oxidative stress resistance of fish (33, 37). Xu et al. showed that juvenile hybrid tilapia (Oreochromis niloticus♀ × O. aureus♂) fed with 6 g/kg nucleotides had higher superoxide dismutase activity and lower malondialdehyde levels than the control fish, indicating an improvement in the antioxidant status (45). Moreover, the current study also demonstrated that the expression of inflammation-related genes was significantly higher in the XMX-1 (N) diet group compared to the HFD group. In line with our results, Reda et al. discovered that the addition of nucleotides (0.5, 1.5, and 2.5 g/kg) increased the intestinal cytokine TNF-α, IL-1β, IL-10, and TGF-β and had a dosage effect compared to the control (34). Notably, the dose effect of XMX-1 (N) on lysozyme activity, antioxidant capacity, and cytokines expression is consistent with the dose responses of dietary nucleotides reported in the previous studies (44–46). These findings suggest that the dietary supplementation with XMX-1 (N) can promote a non-specific immunity in zebrafish and the nucleotides generated by the nuclease treatment of C. somerae fermentation product may play a role in this process.
Gut microbiota plays a key role in regulating gene expression of the digestive tract, promoting nutrition metabolism, and innate immune response (47). In the present work, PCoA analysis revealed substantial differences across groups, indicating that XMX-1 (N) diets had significant effects on the autochthonous microbiota. Specifically, 100 g/kg XMX-1 (N) supplementation enhanced the relative abundance of Firmicutes while decreased the relative abundance of Proteobacteria and Actinobacteria. Guo et al. reported that the nucleotide-fed zebrafish exhibited a higher abundance of Fusobacteria and a lower abundance of Proteobacteria than the control group (38). Furthermore, Pedicoccus acidilactici has been reported to successfully colonize the digestive tract of goldfish (Carassius auratus) fed with 5 g/kg nucleotides supplemented diets (48). These findings suggest that the nucleotides may contribute to the gut microbiota modulation effect of XMX-1 (N), which awaits further investigation.
Notably, the three doses of XMX-1 (N) supplementation all lowered the level of TAG in zebrafish liver. The previous studies have shown that the dietary nucleotides improved hepatic function and reduced hepatic lipid when compared to a control group, indicating that the dietary nucleotides influenced hepatic lipid deposition (49–52). Consistent with these results in mammals, the improvement in body lipid contents and hepatosomatic index can be observed in red sea bream (Pagrus major) fed with nucleotides diets (37). Interestingly, Ran et al. found that 1 g/kg nucleotides supplementation lowered the level of TAG in the liver and decreased the expression of genes involved in lipid synthesis while increased the expression of genes involved in fatty acid oxidation in zebrafish (29). In the present work, the nucleotides levels in the three XMX-1 (N) groups (0.29, 0.58, 5.8 g/kg, respectively) are in a similar range as previous studies (29, 38), suggesting the relative contribution of nucleotides to the hepatic lipid-lowering effect.
Conclusion
The current findings suggest that the dietary XMX-1 (N) at 100 g/kg improved zebrafish growth. Three doses of XMX-1 (N) supplementation enhanced non-specific immunity. In addition, 100 g/kg XMX-1 (N) supplementation improved zebrafish gut microbiota and reduced hepatic lipid deposition. These findings indicate that the nuclease-treated C. somerae XMX-1 fermentation product improves fish growth and health, implying that it might be used as a functional feed additive in fish culture.
Data Availability Statement
The datasets presented in this study can be found in online repositories. The names of the repository/repositories and accession number(s) can be found below: https://www.ncbi.nlm.nih.gov/, PRJNA 825074; https://www.ncbi.nlm.nih.gov/, PRJNA 824236.
Ethics Statement
The animal study was reviewed and approved by the Institute of Feed Research of Chinese Academy of Agricultural Sciences, which was chaired by the China Council for Animal Care (Assurance No. 2018-AF-FRI-CAAS-001).
Author Contributions
MX and QH: formal analysis, data curation, writing original draft preparation, and revising the draft. RX: methodology and investigation. RO: resources and methodology. ER: analysis and methodology. YY and ZZ: analysis and resources. CR: data curation, project administration, and funding acquisition. ZGZ: project administration, supervision, and funding acquisition. All authors contributed to the article and approved the submitted version.
Funding
This study was funded by the National Key R&D Program of China (2018YFD0900400) and the National Natural Science Foundation of China (31925038 and 31972807).
Conflict of Interest
The authors declare that the research was conducted in the absence of any commercial or financial relationships that could be construed as a potential conflict of interest.
Publisher’s Note
All claims expressed in this article are solely those of the authors and do not necessarily represent those of their affiliated organizations, or those of the publisher, the editors and the reviewers. Any product that may be evaluated in this article, or claim that may be made by its manufacturer, is not guaranteed or endorsed by the publisher.
References
1. Henriksson PJG, Troell M, Banks LK, Belton B, Beveridge MCM, Klinger DH, et al. Interventions for improving the productivity and environmental performance of global aquaculture for future food security. One Earth. (2021) 4:1220–32. doi: 10.3390/s21072499
2. Naylor RL, Goldburg RJ, Primavera JH, Kautsky N, Beveridge M, Clay J, et al. Effect of aquaculture on world fish supplies. Nature. (2000) 405:1017–24. doi: 10.1038/35016500
3. Tocher DR. Metabolism and functions of lipids and fatty acids in teleost fish. Rev Fish Sci. (2003) 11:107–84. doi: 10.1080/713610925
4. Catacutan MR, Pagador GE, Teshima S. Effect of dietary protein and lipid levels and protein to energy ratios on growth, survival and body composition of the mangrove red snapper, Lutjanus argentimaculatus (Forsskal 1775). Aquac Res. (2001) 32:811–8. doi: 10.1046/j.1365-2109.2001.00618.x
5. Ding Q, Zhang Z, Li Y, Liu H, Hao Q, Yang Y, et al. Propionate induces intestinal oxidative stress via Sod2 propionylation in zebrafish. iScience. (2021) 24:102515. doi: 10.1016/j.isci.2021.102515
6. Zhao H, Luo Y, Zhang Y, Chen X, Wu Z. Effects of Bacillus subtilis on hepatic lipid metabolism and oxidative stress response in grass carp (Ctenopharyngodon idellus) fed with a high-fat diet. Mar Life Sci Technol. (2020) 2:50–9. doi: 10.1007/s42995-019-00005-2
7. Al-Muzafar HM, Amin KA. Probiotic mixture improves fatty liver disease by virtue of its action on lipid profiles, leptin, and inflammatory biomarkers. BMC Complement Altern Med. (2017) 17:43. doi: 10.1186/s12906-016-1540-z
8. Tian Y, Wang H, Yuan F, Li N, Huang Q, He L, et al. Perilla oil has similar protective effects of fish oil on high-fat diet-induced nonalcoholic fatty liver disease and gut dysbiosis. Biomed Res Int. (2016) 2016:9462571. doi: 10.1155/2016/9462571
9. Falcinelli S, Rodiles A, Hatef A, Picchietti S, Cossignani L, Merrifield DL, et al. Dietary lipid content reorganizes gut microbiota and probiotic L. rhamnosus attenuates obesity and enhances catabolic hormonal milieu in zebrafish. Sci Rep. (2017) 7:5512. doi: 10.1038/s41598-017-05147-w
10. Zhang F, Yang Y, Zhang Z, Yao Y, Xia R, Gao C, et al. Surface-displayed Amuc_1100 from Akkermansia muciniphila on Lactococcus lactis ZHY1 improves hepatic steatosis and intestinal health in high-fat-fed zebrafish. Front Nutr. (2021) 8:726108. doi: 10.3389/fnut.2021.726108
11. Feèkaninová A, Košèová J, Mudroòová D, Popelka P, Toropilova J. The use of probiotic bacteria against Aeromonas infections in salmonid aquaculture. Aquaculture. (2017) 469:1–8. doi: 10.1016/j.aquaculture.2016.11.042
12. Panigrahi A, Kiron V, Kobayashi T, Puangkaew J, Satoh S, Sugita H. Immune responses in rainbow trout Oncorhynchus mykiss induced by a potential probiotic bacteria Lactobacillus rhamnosus JCM 1136. Vet Immunol Immunopathol. (2004) 102:379–88. doi: 10.1016/j.vetimm.2004.08.006
13. Vaughan E, Vries MD, Zoetendal EG, Ben-Amor K, Akkermans AD, Vos WD. The intestinal LABs. Antonie Van Leeuwenhoek. (2002) 82:341.
14. Martínez Cruz P, Ibáñez AL, Monroy Hermosillo OA, Ramírez Saad HC. Use of probiotics in aquaculture. Isrn Microbiol. (2012) 2012:916845.
15. Ray C, Bujan N, Tarnecki A, Davis AD, Browdy C, Arias C. Analysis of the gut microbiome of Nile tilapia Oreochromis niloticus L. fed diets supplemented with Previda§and Saponin. J Fish Sci. (2017) 11:36.
16. van Kessel MA, Dutilh BE, Neveling K, Kwint MP, Veltman JA, Flik G, et al. Pyrosequencing of 16S rRNA gene amplicons to study the microbiota in the gastrointestinal tract of carp (Cyprinus carpio L.). AMB Express. (2011) 1:41. doi: 10.1186/2191-0855-1-41
17. Wang A, Zhang Z, Ding Q, Yang Y, Bindelle J, Ran C, et al. Intestinal Cetobacterium and acetate modify glucose homeostasis via parasympathetic activation in zebrafish. Gut Microbes. (2021) 13:1–15.
18. Xie M, Zhou W, Xie Y, Li Y, Zhang Z, Yang Y, et al. Effects of Cetobacterium somerae fermentation product on gut and liver health of common carp (Cyprinus carpio) fed diet supplemented with ultra-micro ground mixed plant proteins. Aquaculture. (2021) 543:736943. doi: 10.1016/j.aquaculture.2021.736943
19. Xie M, Xie Y, Li Y, Zhou W, Zhang Z, Yang Y, et al. Stabilized fermentation product of Cetobacterium somerae improves gut and liver health and antiviral immunity of zebrafish. Fish Shellfish Immunol. (2021) 120:56–66.
20. Gite S, Reddy G, Shankar V. Active-site characterization of S1 nuclease I. Affinity purification and influence of amino-group modification. Biochem J. (1992) 285:489–94. doi: 10.1042/bj2850489
21. Koval T, Dohnálek J. Characteristics and application of S1–P1 nucleases in biotechnology and medicine. Biotechnol Adv. (2018) 36:603–12.
22. Papayannopoulos V. Infection: microbial nucleases turn immune cells against each other. Curr Biol. (2014) 24:R123–5. doi: 10.1016/j.cub.2013.12.027
23. Sharma P, Garg N, Sharma A, Capalash N, Singh R. Nucleases of bacterial pathogens as virulence factors, therapeutic targets and diagnostic markers. Int J Med Microbiol. (2019) 309:151354. doi: 10.1016/j.ijmm.2019.151354
24. Carver JD, Walker WA. The role of nucleotides in human nutrition. J Nutr Biochem. (1995) 6:58–72. doi: 10.1016/0955-2863(94)00019-I
25. Gil A. Modulation of the immune response mediated by dietary nucleotides. Eur J Clin Nutr. (2002) 56:S1–4. doi: 10.1038/sj.ejcn.1601475
26. Xu M, Zhao M, Yang R, Zhang Z, Li Y, Wang J. Effect of dietary nucleotides on immune function in Balb/C mice. Int Immunopharmacol. (2013) 17:50–6.
27. Yau KIT, Huang CB, Chen W, Chen SJ, Chou YH, Huang FY, et al. Effect of nucleotides on diarrhea and immune responses in healthy term infants in Taiwan. J Pediatr Gastroenterol Nutr. (2003) 36:37–43. doi: 10.1097/00005176-200301000-00009
28. Hossain MS, Koshio S, Kestemont P. Recent advances of nucleotide nutrition research in aquaculture: a review. Rev Aquacult. (2020) 12:1028–53. doi: 10.1111/raq.12370
29. Ran C, Xie M, Li J, Xie Y, Ding Q, Li Y, et al. Dietary nucleotides alleviate hepatic lipid deposition via exogenous AMP-Mediated AMPK activation in zebrafish. J Nutr. (2021) 151:2986–96. doi: 10.1093/jn/nxab232
30. Zhang Z, Ran C, Ding Q, Liu H, Xie M, Yang Y, et al. Ability of prebiotic polysaccharides to activate a HIF1α-antimicrobial peptide axis determines liver injury risk in zebrafish. Commun Biol. (2019) 2:274. doi: 10.1038/s42003-019-0526-z
31. Lin YH, Wang H, Shiau SY. Dietary nucleotide supplementation enhances growth and immune responses of grouper, Epinephelus malabaricus. Aquacult Nutr. (2009) 15:117–22. doi: 10.1111/j.1365-2095.2007.00561.x
32. Li P, Gatlin IIIDM, Neill WH. Dietary supplementation of a purified nucleotide mixture transiently enhanced growth and feed utilization of juvenile red drum, Sciaenops ocellatus. J World Aquacult Soc. (2007) 38:281–6.
33. Hossain MS, Koshio S, Ishikawa M, Yokoyama S, Sony NM, Kader MA, et al. Effects of dietary administration of inosine on growth, immune response, oxidative stress and gut morphology of juvenile amberjack, Seriola dumerili. Aquaculture. (2017) 468:534–44. doi: 10.1016/j.aquaculture.2016.11.020
34. Reda RM, Selim KM, Mahmoud R, El-Araby IE. Effect of dietary yeast nucleotide on antioxidant activity, non-specific immunity, intestinal cytokines, and disease resistance in Nile Tilapia. Fish Shellfish Immunol. (2018) 80:281–90. doi: 10.1016/j.fsi.2018.06.016
35. Tahmasebi-Kohyani A, Keyvanshokooh S, Nematollahi A, Mahmoudi N, Pasha-Zanoosi H. Effects of dietary nucleotides supplementation on rainbow trout (Oncorhynchus mykiss) performance and acute stress response. Fish Physiol Biochem. (2012) 38:431–40. doi: 10.1007/s10695-011-9524-x
36. Coman GJ, Sarac HZ, Fielder D, Thorne M. Evaluation of crystalline amino acids, betaine and AMP as food attractants of the giant tiger prawn (Penaeus monodon). Comp Biochem Physiol A Physiol. (1996) 113:247–53. doi: 10.1016/0300-9629(96)00031-X
37. Hossain MS, Koshio S, Ishikawa M, Yokoyama S, Sony NM, Dawood MA, et al. Efficacy of nucleotide related products on growth, blood chemistry, oxidative stress and growth factor gene expression of juvenile red sea bream, Pagrus major. Aquaculture. (2016) 464:8–16. doi: 10.1016/j.aquaculture.2016.06.004
38. Guo X, Ran C, Zhang Z, He S, Jin M, Zhou Z. The growth-promoting effect of dietary nucleotides in fish is associated with an intestinal microbiota-mediated reduction in energy expenditure. J Nutr. (2017) 147:781–8. doi: 10.3945/jn.116.245506
39. Jollès P, Jollès J. What’s new in lysozyme research? Always a model system, today as yesterday. Mol Cell Biochem. (1984) 63:165–89. doi: 10.1007/BF00285225
40. Saurabh S, Sahoo PK. Lysozyme: an important defence molecule of fish innate immune system. Aquac Res. (2008) 39:223–39. doi: 10.1111/j.1365-2109.2007.01883.x
41. Nielsen ME, Esteve-Gassent MD. The eel immune system: present knowledge and the need for research. J Fish Dis. (2006) 29:65–78. doi: 10.1111/j.1365-2761.2006.00695.x
42. Holland M, Lambris JD. The complement system in teleosts. Fish Shellfish Immunol. (2002) 12:399–420. doi: 10.1006/fsim.2001.0408
43. Sakai M, Taniguchi K, Mamoto K, Ogawa H, Tabata M. Immunostimulant effects of nucleotide isolated from yeast RNA on carp, Cyprinus carpio L. J Fish Dis. (2001) 24:433–8. doi: 10.1046/j.1365-2761.2001.00314.x
44. Cheng Z, Buentello A, Gatlin IIIDM. Dietary nucleotides influence immune responses and intestinal morphology of red drum Sciaenops ocellatus. Fish Shellfish Immunol. (2011) 30:143–7. doi: 10.1016/j.fsi.2010.09.019
45. Xu L, Ran C, He S, Zhang J, Hu J, Yang Y, et al. Effects of dietary yeast nucleotides on growth, non-specific immunity, intestine growth and intestinal microbiota of juvenile hybrid tilapia Oreochromis niloticus?× Oreochromis aureus? Anim Nutr. (2015) 1:244–51. doi: 10.1016/j.aninu.2015.08.006
46. Low C, Wadsworth S, Burrells C, Secombes CJ. Expression of immune genes in turbot (Scophthalmus maximus) fed a nucleotide-supplemented diet. Aquaculture. (2003) 221:23–40. doi: 10.1016/S0044-8486(03)00022-X
47. Rawls JF, Samuel BS, Gordon JI. Gnotobiotic zebrafish reveal evolutionarily conserved responses to the gut microbiota. Proc Natl Acad Sci USA. (2004) 101:4596–601. doi: 10.1073/pnas.0400706101
48. Mehdinejad N, Imanpour MR, Jafari V. Combined or individual effects of dietary probiotic Pedicoccus acidilactici and nucleotide on growth performance, intestinal microbiota, hemato-biochemical parameters, and innate immune response in goldfish (Carassius auratus). Probiotics Antimicrob Proteins. (2018) 10:558–65. doi: 10.1007/s12602-017-9297-3
49. Pérez MJ, Suárez A, Gómez-Capilla JA, Sánchez-Medina F, Gil A. Dietary nucleotide supplementation reduces thioacetamide-induced liver fibrosis in rats. J Nutr. (2002) 132:652–7. doi: 10.1093/jn/132.4.652
50. Pérez MJ, Sánchez-Medina F, Torres M, Gil A, Suárez A. Dietary nucleotides enhance the liver redox state and protein synthesis in cirrhotic rats. J Nutr. (2004) 134:2504–8. doi: 10.1093/jn/134.10.2504
51. Cai X, Bao L, Wang N, Ren J, Chen Q, Xu M, et al. Dietary nucleotides protect against alcoholic liver injury by attenuating inflammation and regulating gut microbiota in rats. Food Funct. (2016) 7:2898–908. doi: 10.1039/c5fo01580d
Keywords: Cetobacterium somerae, nuclease, growth, non-specific immunity, liver health
Citation: Xie M, Hao Q, Xia R, Olsen RE, Ringø E, Yang Y, Zhang Z, Ran C and Zhou Z (2022) Nuclease-Treated Stabilized Fermentation Product of Cetobacterium somerae Improves Growth, Non-specific Immunity, and Liver Health of Zebrafish (Danio rerio). Front. Nutr. 9:918327. doi: 10.3389/fnut.2022.918327
Received: 12 April 2022; Accepted: 08 June 2022;
Published: 06 July 2022.
Edited by:
Haohao Wu, Ocean University of China, ChinaCopyright © 2022 Xie, Hao, Xia, Olsen, Ringø, Yang, Zhang, Ran and Zhou. This is an open-access article distributed under the terms of the Creative Commons Attribution License (CC BY). The use, distribution or reproduction in other forums is permitted, provided the original author(s) and the copyright owner(s) are credited and that the original publication in this journal is cited, in accordance with accepted academic practice. No use, distribution or reproduction is permitted which does not comply with these terms.
*Correspondence: Chao Ran, cmFuY2hhb0BjYWFzLmNu; Zhigang Zhou, emhvdXpoaWdhbmcwM0BjYWFzLmNu
†These authors have contributed equally to this work and share first authorship