- 1College of Life Sciences and Agronomy, Zhoukou Normal University, Zhoukou, China
- 2College of Food Science and Technology, Henan University of Technology, Zhengzhou, China
- 3School of Biological Engineering, Henan University of Technology, Zhengzhou, China
- 4College of Life Sciences, Chongqing Normal University, Chongqing, China
Researchers have investigated the role of polysaccharides in disease treatment via gut microbiota regulation but ignore their function in disease prevention and physique enhancement. In this work, a Gynostemma pentaphyllum polysaccharide (GPP) was tested by methyl thiazolyl tetrazolium (MTT) assay and proved to be safe to Caco-2 cells. Animal experiments showed that the administration of GPP for 3 weeks decreased the body weight gain of mice from 15.4 ± 1.7 to 12.2 ± 1.8 g in a concentration-dependent manner. Analysis of short-chain fatty acids (SCFAs) indicated that GPP increased the levels of acetate, propionate, butyrate, and total SCFAs in the cecum contents of normal mice. Furthermore, GPP improved the species richness and abundance in the gut microbiota but reduced the Firmicutes/Bacteroidetes ratio from 0.8021 to 0.3873. This work provides a basis for incorporating GPP into diet to prevent or mitigate the occurrence of obesity via gut microbiota regulation.
Introduction
With lifestyle changes and living standard improvement, unreasonable diet has become an important factor that directly influences human health. Three high-metabolic syndromes, namely, hyperglycemia, hyperlipidemia, and hypertension caused by high sugar and high fat diet, have become a serious threat to physical health, quality of life, and national economy development. Therefore, the irreplaceable diet in people's daily life could be used as an entry point by modifying functional properties to improve health and prevent the occurrence and development of diseases. A daily intake of dietary fiber could reduce the harmful effects of stroke, coronary disease, type 2 diabetes, and colon cancer (1). Active substances in diet have good prevention and treatment effects against nutritional diseases and may include functional polysaccharides from natural medicinal and edible plants (2). Functional polysaccharides derived from edible plants can be used as food supplements that can improve people's diet by scavenging oxygen free radicals and inhibiting lipid peroxidation, α-glucosidase activity, α-amylase activity, promoting insulin secretion, and regulating the metabolism of glucose and lipids (3).
Gut microbiota, a community of microorganisms in the gut, plays an important role as a bridge among food nutrition and health. The gut microbiota not only participates in nutrition, metabolism, and immune regulation but also in the occurrence and development of nutritional, metabolic, and immune diseases. Cani (4) found that depending on the source of amino acids, gut microbiota can mitigate metabolic diseases via improving or altering metabolism of body and the corresponding metabolites. Zhao et al. (5) suggested that dietary fibers could promote gut microbiota to produce diversity and abundance of short-chain fatty acids (SCFAs) and diminish production of metabolically detrimental compounds such as indole and hydrogen sulfide, thus alleviating type 2 diabetes via improving hemoglobin A1c levels and increasing glucagon-like peptide-1 production. Mardinoglu et al. (6) reported that low-carbohydrate diet alleviated non-alcoholic fatty liver might via increasing the folate-producing Streptococcus in gut microbiota and serum folate concentrations, thus down-regulating of the fatty acid synthesis pathway and up-regulating of folate-mediated one-carbon metabolism and fatty acid oxidation pathways. Furthermore, certain effects have been achieved by gut microbiota transplantation, in which the feces of a healthy donor are transplanted into patients to reconstruct a new gut microbiota and regulate health (7). Gut microbiota has gradually developed into a biomarker of human health, disease prevention, and immune regulation.
Polysaccharides can affect nutrition and health by regulating the gut microbiota. For instance, Sun et al. (8) demonstrated that polysaccharides extracted from plants could alleviate circadian rhythm disorders and related psychiatric disorders by regulating the gut microbiota. Liu et al. (9) verified that mannan-oligosaccharide could alleviate the cognitive and behavioral disorders of mice with 5xFAD Alzheimer's disease by regulating the gut microbiota–brain axis. Mo et al. (10) found that insoluble yeast β-glucan could alleviate the high-fat diet-induced obesity of mice by regulating the gut microbiota and its metabolites. Li et al. (11) suggested that alginate oligosaccharides can protect against fumonisin B1-induced intestinal damage by promoting gut microbiota homeostasis. In our previous work, we found that a Gynostemma pentaphyllum polysaccharide (GPP) could adjust the high blood sugar level of diabetic mice to the normal level (12). When the absorption mechanisms of a Ganoderma lucidum polysaccharide (GLP) were investigated in vivo and in vitro, we found that GLP entered the body and reached the posterior part of the intestinal tract to participate in gut microbiota metabolism (13). However, the role of GPP is improving body health through gut microbiota regulation remains unclear. Therefore, the toxicity of GPP was first investigated; after which, the effect of GPP on body weight and short-chain fatty acids (SCFAs) in the intestine and gut microbiota of mice was analyzed.
Materials and Methods
Materials
Gynostemma pentaphyllum (Thumb) Makino herb was bought from a local drugstore of Zhengzhou (China). Caco-2 cells (HTB037) were acquired from the American Type Culture Collection and stored in our laboratory (13). Specific pathogen-free Kunming male mice (SCXK 2017-0002, 18–22 g) were bought from the Experimental Animal Center of Henan province (China). Dimethyl sulfoximine (DMSO), Dulbecco's modified Eagle's medium (DMEM), and 3-(4,5-Dimethylthiazol-2-yl)-2,5-bromo diphenyltetrazolium were purchased from Sigma-Aldrich (Shanghai, China). Fetal bovine serum, penicillin, and streptomycin were bought from Beyotime Biotechnology Co., Ltd., (Shanghai, China). Ethyl alcohol, sodium chloride, ethyl ether, sulfuricacid, calcium chloride, acetate, propionate, butyrate, and other chemical reagents were bought from Sinopharm Chemical Reagent Co., Ltd., (Beijing, China).
Preparation of GPP
Extraction, collection, and purification of the Gynostemma pentaphyllum polysaccharide (GPP) were conducted based on previous methods (12), no protein or nucleic acid were detected in the obtained GPP. Then, the freeze-dried GPP powder was used in following experiments.
Toxicity Analysis of GPP
Toxicity of GPP was determined using methyl thiazolyl tetrazolium (MTT) method against Caco-2 cells by using a previously reported method with some modifications (14). The freeze-dried GPP powder was dissolved and stirred in DMEM to concentrations of 50, 100, 200, 400, and 800 μg/ml, respectively. Meanwhile, DMEM solution without dissolving GPP was used as blank control. Caco-2 cells were cultured in DMEM with fetal bovine serum (10%, v/v), penicillin (100 U/ml), and streptomycin (100 μg/ml) in a humidified 5% CO2 incubator (Series 8000 WJ, Thermo Fisher Scientific, Waltham, MA, United States) at 37°C. During cultivation, Caco-2 cells were digested and counted intermittently. Upon reaching 2 × 104 cells/ml, Caco-2 cells were transferred into 96-well plates and incubated in the CO2 incubator for 24 h at 37°C. Each well was added with 100 μl of GPP solution with different concentrations by using a pipette (Eppendorf, Germany) and cultured for another 24 h. The solution was then added with 20 μl of 3-(4,5-dimethylthiazol-2-yl)-2,5-bromo diphenyltetrazolium (5 mg/ml) and incubated for 4 h. The cell supernatant was discarded, and the insoluble crystals in Caco-2 cells were dissolved by adding 150 μl of DMSO. Absorbance was recorded using a microplate reader (BIO-RAD, Hercules, CA, United States) at 490 nm.
Experimental Design and Sample Collection
Thirty mice (20 ± 1.0 g) were acquired from the Experimental Animal Center of Henan province (China) and fed in a specific-free pathogen environment, with temperature of 22–26°C, humidity of 60 ± 5%, and light: dark cycle of 12: 12 h. All animal procedures were performed in accordance with the guidelines for care and use of laboratory animals of Henan University of Technology. Experiments were approved by the Animal Ethics Committee of Henan University of Technology. Mice were fed according to routine feeding procedure and given food and drink freely for the first 7 days to acclimatize. After which, the mice ate (components of mice food are as follows: water 95 g/kg, crude protein 195.5 g/kg, crude fat 47.3 g/kg, coarse fiber 21.4 g/kg, crude ash 54.6 g/kg, calcium 13.6 g/kg, and total phosphorus 8.09 g/kg) and drank (tap water is boiled and cooled for use) freely throughout the whole experiment except for administration process. Then, twenty-four robust mice were selected and randomly divided into four groups, with six mice each (n = 6): control group (NC), administered with 0.4 ml of distilled water orally once a day; based on the preliminary experiment, experimental groups, orally administered with 100 μg/ml (2 g/kg) GPP (low-dose group, LOW), 400 μg/ml (8 g/kg) GPP (middle-dose group, MID), and 800 μg/ml (16 g/kg) GPP (high-dose group, HIG). If a single administration of 0.4 ml was difficult, then two consecutive doses of 0.2 ml were given. The night before administration, mice were fasted but drank freely. Mouse experiments lasted for 21 days, and the mice weight was measured weekly. The experimental mice were then fasted for 12 h and sacrificed by cervical dislocation. The contents in cecum were collected and stored at −80°C for further analysis.
Detection of Short-Chain Fatty Acids
In brief, 0.2 g of cecum contents were dissolved in 1 ml of deionized water, vibrated for 3 min, and processed with ultrasonic wave for 30 min. The solution was added with 0.2 ml of 50% H2SO4 and 2 ml of diethyl ether and mixed acutely for 30 min. The mixture was placed in a water bath shaker at room temperature at 250 r/min for 30 min and centrifuged at 8,000 × g and 4°C for 10 min. The organic supernatant was collected and mixed with 1.0 g of anhydrous calciumchloride for 10 min to remove water. The supernatant was filtered against a 0.22 μm organic-based filter membrane, and SCFAs in organic layer were analyzed according to previously reported methods (14).
Gut Microbiota Analysis
Gut microbiota was extracted and detected according to method reported previously with some modifications (14). Briefly, the total microbial DNA in the bacteria of collected cecum contents was extracted by using DNA extraction kit. V3-V4 hypervariable region of 16S rRNA was used as primer, and the primer sequences were 338F (5′-ACTCC TACGG GAGGC AGCAG-3′) and 806R (5′-GGACT ACHVG GGTWT CTAAT-3′). The amplification process was repeated three times for each sample, and the PCR products were recovered by using 2% (w/v) agarose gel electrophoresis. Meanwhile, AxyPrep DNA Gel Extraction Kit was used to purify the PCR products. After which, the PCR products were sent to Majorbio Co., Ltd., China (Shanghai, China) for gut microbiota analysis using Illumina MiSeq platforms according to the operation manual.
Statistical Analysis
All data were expressed as mean of three parallel experiments. Analysis of variance (ANOVA) was performed using Origin software (Origin Pro 8.5).
Results and Discussion
Toxicity Analysis of GPP
Previously, a polysaccharide of GPP was successfully extracted from Gynostemma pentaphyllum herb, the monosaccharide composition of which was rhamnose, arabinose, galactose, glucose, xylose, mannose, galacturonic acid and glucuronic acid with molar ratio of 4.11: 7.34: 13.31: 20.99: 1.07: 0.91: 4.75: 0.36. Meanwhile, weight-average molecular weight (Mw) and number-average molecular weight (Mn) of GPP were 4.070 × 104 and 3.924× 104 Da, and its polydispersity (Mw/Mn) was 1.037 (12). However, due to geography and climate difference, monosaccharide composition and molecular weight of GPP were different from polysaccharides extracted from different G. pentaphyllum herbs reported by other researchers (15, 16).
Meanwhile, GPP could reduce the blood sugar level of diabetic mice to normal via α-glucosidase inhibition and anti-inflammatory effects (3, 12), but the safety of GPP has been neglected. The toxicity of GPP to Caco-2 cells was detected by MTT assay. Figure 1 shows that none of the detected GPP concentration affected the viability of Caco-2 cells, indicating that GPP was safe to Caco-2 cells. Similarly, Li et al. (17) reported that G. pentaphyllum polysaccharide had no specific cytotoxicity to Hs-68 cells. Li et al. (18) also found that Eucommia ulmoides polysaccharide was non-toxic to Raw 264.7 macrophages. In our previous works, we found that Ganoderma lucidum polysaccharide and Chaetomium globosum CGMCC 6882 exo-polysaccharide were not toxic to Caco-2 cells (13, 14). In the present work, experimental mice showed no symptoms of death or disease, suggesting the safety of GPP to mice, which was in consistent with the safe result of GPP to Caco-2 cells. Furthermore, He et al. (19) reported that polysaccharide produced by Streptomyces Virginia H03 was non-toxic and did not kill mice even at 500 mg/kg/day.
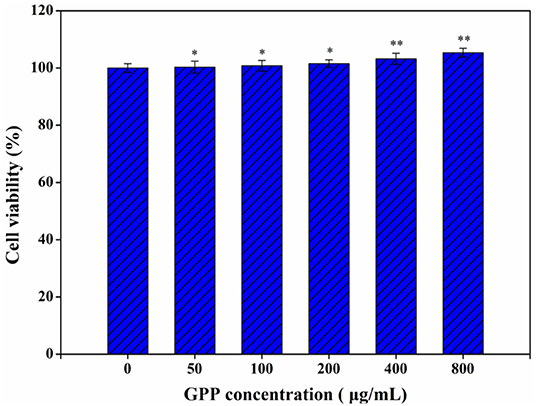
Figure 1. Toxicity of GPP to Caco-2 cells by MTT assay. Data are expressed as mean ± SD (n = 3). Significance was determined through ANOVA, *P < 0.05, **P < 0.01.
Influence of GPP on Mouse Body Weight
With improvement of living standards, changes in lifestyle, and reduction in physical activity, an increasing number of people suffer from obesity. Except for proper exercise and diet control, reasonable diet structure is an effective means to prevent and alleviate obesity (20). As shown in Table 1, the weights of mice in the control and experimental groups increased gradually with extension of feeding time. However, the body weight gain of experimental mice was negatively correlated with GPP concentration, that is, it decreased from 15.4 ± 1.7 g (CON) to 14.3 ± 1.9 g (LOW), 13.7 ± 1.6 g (MID), and 12.2 ± 1.8 g (HIG). Hence, GPP could be added in food or diet to prevent and mitigate obesity. Similarly, Chen et al. (21) reported that Pueraria lobata polysaccharide had similar body weight regulation effects. In contrast to the present study, Zhao et al. (22) found that Auricularia auricular polysaccharide did not affect the body weight. Furthermore, Yin et al. (23) found that non-polysaccharide substance of resveratrol could regulate the loss of body weight. GPP regulates body weight possibly by three mechanisms: inhibiting enzyme activity and nutrient absorption rate (24); adjusting the composition and proportion of gut microbiota, especially Firmicutes/Bacteroidetes (F/B) ratio (25); and degradation and conversion of GPP into SCFAs (26). The exact mechanism of GPP in regulating body weight is still being investigated.
Effect of GPP on SCFAs
Non-starch polysaccharides are resistant to saliva, succus gastricus, and succus entericus, which will be digested by the gut microbiota into SCFAs to exert biological activities (26). As shown in Figure 2A, after administration of different concentrations of GPP for 3 weeks, the acetate concentration in the cecum contents increased from 21.03 ± 0.75 μmol/g (CON) to 23.55 ± 0.58 μmol/g (LOW), 26.75 ± 1.03 μmol/g (MID), and 32.88 ± 0.82 μmol/g (HIG). Figure 2B shows that the propionate concentration increased from 8.95 ± 0.41 μmol/g (CON) to 10.29 ± 0.53 μmol/g (LOW), 12.55 ± 0.37 μmol/g (MID), and 17.36 ± 0.48 μmol/g (HIG). Figure 2C indicates that the butyrate concentration increased from 6.53 ± 18 μmol/g (CON) to 8.17 ± 0.25 μmol/g (LOW), 9.26 ± 0.21 μmol/g (MID), and 11.85 ± 0.15 μmol/g (HIG). Figure 2D illustrates that the level of total SCFAs increased from 36.51 ± 1.15 μmol/g (CON) to 42.01 ± 96 μmol/g (LOW), 48.56 ± 1.24 μmol/g (MID), and 62.09 ± 1.53 μmol/g (HIG). Furthermore, the increase in SCFAs was positively correlated with GPP concentration, with acetate having the highest increment.
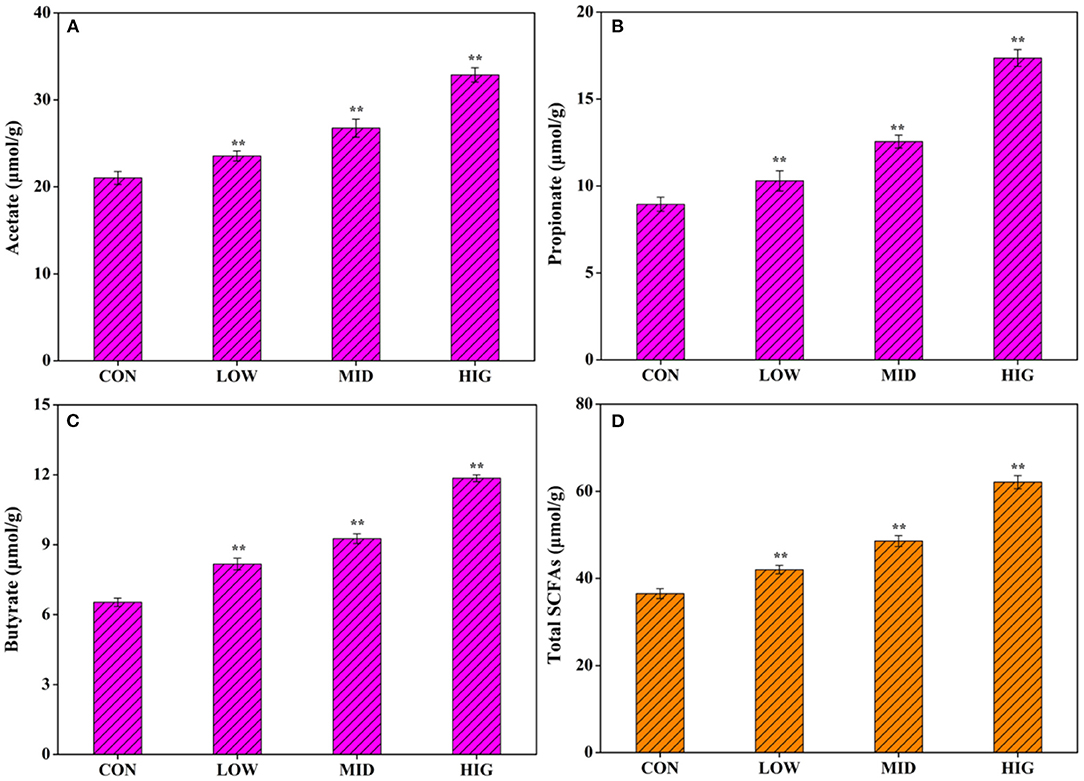
Figure 2. Influence of GPP on acetate, propionate, butyrate, and total SCFAs. (A) Acetate, (B) propionate, (C) butyrate, and (D) total SCFAs. CON: control group, LOW: 100 μg/ml GPP, MID: 400 μg/ml GPP, HIG: 800 μg/ml GPP. Data are expressed as mean ± SD (n = 6). Significance was determined through ANOVA, *P < 0.05, **P < 0.01.
Previously, Gao et al. (27) verified that when gellan gum was degraded to gellan oligosaccharide with molecular weight of 72,903 Da, the production of acetic acid and propionic acid by gellan oligosaccharide was increased in a bionic intestinal reactor in vitro, indicating that relatively low molecular weight is a key factor for polysaccharide to regulate gut microbiota for SCFAs production. Molecular weight of GPP used in present work was 40,700 Da (12), which might be a factor affecting its probiotic activity on increasing SCFAs production. SCFAs not only are important in energy supply and gut microbiota health but also might affect the metabolism or function of peripheral tissues via entering systemic circulation (28), such as body weight regulation. For instance, Canfora et al. (29) found that SCFAs could prevent and counteract obesity by promoting satiety. Other researchers reported that acetate in SCFAs plays an important role in body weight regulation (30). GPP reduced body weight possibly via increasing the level of SCFAs, especially acetate.
Effect of GPP on Gut Microbiota
Influence of Gut Microbiota Diversity by GPP
Many statistical indices are used to assess the abundance and diversity of microbial communities. Ace and Chao1 indices are used to assess the number of operational taxonomic units (OTUs) in the gut microbiota; Simpson and Shannon indices reflect the species diversity of the gut microbiota (31). As shown in Table 2, the Ace and Chao1 indices of the gut microbiota in the experimental group increased compared with that in the control group in a concentration-dependent manner. Hence, the intake of GPP increased the species richness of the gut microbiota. The Shannon index of the gut microbiota showed an increasing trend, whereas the Simpson index had a downward trend. This finding suggested that the increase of species diversity of the gut microbiota was induced by administration of GPP (22). When Deng et al. (32) studied different molecular weights konjac glucomannans (KGM) on the hypoglycemic effects of type 2 diabetic rats, they found that medium molecular weights KGMs could increase gut microbiota diversity. Therefore, molecular weight (40,700 Da) might be one of the factors affecting gut microbiota diversity by GPP (12).
The effect of GPP on the species richness of the gut microbiota was detected by number of OTUs, and the results are shown as Venn diagram in Figure 3. The total numbers of OTUs in CON, LOW, MID, and HIG were 434, 455, 462, and 499, respectively. Among all OTUs, 318 were shared by four groups and 42 were only shared by three experimental groups. Meanwhile, the numbers of OTUs separately shared between control group and experimental groups were 369 (CON and LOW), 374 (CON and MID), and 399 (CON and HIG). In addition, each group had its own unique OTUs: 7 for CON, 8 for LOW, 11 for MID, and 33 for HIG. Hence, GPP improved the species richness and diversity of the gut microbiota.
Gut Microbiota Analysis at Phylum Level
The regulatory effect of GPP on the gut microbiota is shown in Figure 4. Among the four groups, the main bacteria (relative abundance > 1%) at the phylum level are Bacteroidetes, Firmicutes, Spirochaete, Proteobacteria, Verrucomicrobia, and Cyanobacteria, accounting for more than 95% of the sequences. Bacteroidetes and Firmicutes are the main bacteria that utilize undigested non-starch polysaccharides (33) and are involved in maintaining the balance of energy metabolism (25). In experimental mice, the relative abundance levels of Bacteroidetes were 47.58% (CON), 59.04% (LOW), 61.13% (MID) and 67.21% (HIG), and those of Firmicutes were 38.16, 36.58, 27.20, and 26.03%, respectively. The F/B ratios in the four groups were 0.8021, 0.6196, 0.4450, and 0.3873, indicating a decreasing trend. Bacteroidetes possess genes for encoding succinate pathway and are the primary polysaccharide-degrading and propionate-producing bacteria in gut (34), this might be the reason of SCFAs increase in gut induced by F/B ratio decrease and Bacteroidetes increase.
Many structural characteristics could affect the probiotic activity of polysaccharides. For instance, Yu et al. (34) reported that the monosaccharide order of sugar beet pulp polysaccharide used by gut microbiota might arabinose, glucose, fucose and galacturonic acid. Zhao et al. (35) found that glycosidic bond type could not only affect the body weight of mice, but also the composition and proportion of mice gut microbiota. Deng et al. (32) suggested that molecular weight of konjac glucomannan might affect its effect on gut microbiota diversity. Gong et al. (36) demonstrated that uronic acid could affect the biological activity of polysaccharides. GPP was composed of rhamnose, arabinose, galactose, glucose, xylose, mannose, galacturonic acid and glucuronic acid in a molar ratio of 4.11: 7.34: 13.31: 20.99: 1.07: 0.91: 4.75: 0.36, and its weight-average molecular weight was 4.070 × 104 Da (12). Arabinose, glucose and uronic acid in its monosaccharide composition and moderate molecular weight (4.070 × 104 Da) might be the reason for probiotic activity of GPP.
Although Gao et al. (37) found that there was no direct relationship between F/B ratio and body weight regulation, many researchers suggested that an increase in the F/B ratio induced obesity and prevented body weight loss. Zhao et al. (22) reported that the body weight reduction effect of Auricularia auricular polysaccharide might be related to decrease in the F/B ratio. Ley et al. (33) demonstrated that an increase in Firmicutes and a decrease in Bacteroidetes are likely to induce obesity. Chang et al. (38) and Koliada et al. (39) suggested that the reduction in the F/B ratio could lead to weight loss and improved body health. Therefore, the decreased F/B ratio may lead to decrease in body weight gain caused by GPP.
Conclusion
In present work, a polysaccharide fraction of GPP extracted from Gynostemma pentaphyllum was used to detect its effect on the body weight and gut microbiota of normal mice. With administration of GPP once a day for 3 weeks, body weight gain of normal mice reduced but SCFAs levels in gut increased. Meanwhile, GPP increased gut microbiota diversity but decreased Firmicutes/Bacteroidetes ratio in the gut of normal mice. However, the mechanism through which the polysaccharide affects the gut microbiota and the type of bacteria that participate in the degradation of polysaccharides remain unclear.
Data Availability Statement
The original contributions presented in the study are included in the article/supplementary material, further inquiries can be directed to the corresponding author/s.
Ethics Statement
The animal study was reviewed and approved by the Animal Ethics Committee of Henan University of Technology.
Author Contributions
SL contributed to conception, design, and funding of the study. YW, WD, and WH organized the database. TN wrote the first draft of the manuscript. QS and ZW contributed to writing—review and editing. All authors contributed to the article and approved the submitted version.
Funding
This work was supported by the Science and Technology Research Project of Henan Province (182102310687), Henan Provincial Education Department Project (19B550010), the Natural Science Foundation of Henan Province (212300410131), and the Natural Science Foundation of Chongqing (cstc2019jcyj-msxmX0459).
Conflict of Interest
The authors declare that the research was conducted in the absence of any commercial or financial relationships that could be construed as a potential conflict of interest.
Publisher's Note
All claims expressed in this article are solely those of the authors and do not necessarily represent those of their affiliated organizations, or those of the publisher, the editors and the reviewers. Any product that may be evaluated in this article, or claim that may be made by its manufacturer, is not guaranteed or endorsed by the publisher.
References
1. Reynolds A, Mann J, Cummings J, Winter N, Mete E, Te Morenga L. Carbohydrate quality and human health: a series of systematic reviews and meta-analyses. Lancet. (2019) 393:434–45. doi: 10.1016/S0140-6736(18)31809-9
2. Ji X, Hou C, Shi M, Yan Y, Liu Y. An insight into the research concerning Panax ginseng C. A. meyer polysaccharides: a review. Food Rev Int. (2020) 1–17. doi: 10.1080/87559129.2020.1771363
3. Wang Z, Wang Z, Huang W, Suo J, Chen X, Ding K, et al. Antioxidant and anti-inflammatory activities of an anti-diabetic polysaccharide extracted from Gynostemma pentaphyllum herb. Int J Biol Macromol. (2020) 145:484–91. doi: 10.1016/j.ijbiomac.2019.12.213
4. Cani PD. Microbiota and metabolites in metabolic diseases. Nat Rev Endocrinol. (2019) 15:69–70. doi: 10.1038/s41574-018-0143-9
5. Zhao L, Zhang F, Ding X, Wu G, Lam YY, Wang X, et al. Gut bacteria selectively promoted by dietary fibers alleviate type 2 diabetes. Science. (2018) 359:1151–6. doi: 10.1126/science.aao5774
6. Mardinoglu A, Wu H, Bjornson E, Zhang C, Hakkarainen A, Räsänen SM, et al. An integrated understanding of the rapid metabolic benefits of a carbohydrate-restricted diet on hepatic steatosis in humans. Cell Metab. (2018) 27:559–71.e555. doi: 10.1016/j.cmet.2018.01.005
7. Wu Z, Huang S, Li T, Li N, Han D, Zhang B, et al. Gut microbiota from green tea polyphenol-dosed mice improves intestinal epithelial homeostasis and ameliorates experimental colitis. Microbiome. (2021) 9:184. doi: 10.1186/s40168-021-01115-9
8. Sun Q, Ho CT, Zhang X, Liu Y, Zhang R, Wu Z. Strategies for circadian rhythm disturbances and related psychiatric disorders: a new cue based on plant polysaccharides and intestinal microbiota. Food Funct. (2022) 13:1048–61. doi: 10.1039/D1FO02716F
9. Liu Q, Xi Y, Wang Q, Liu J, Li P, Meng X, et al. Mannan oligosaccharide attenuates cognitive and behavioral disorders in the 5xFAD Alzheimer's disease mouse model via regulating the gut microbiota-brain axis. Brain Behav Immun. (2021) 95:330–43. doi: 10.1016/j.bbi.2021.04.005
10. Mo X, Sun Y, Liang X, Li L, Hu S, Xu Z, et al. Insoluble yeast β-glucan attenuates high-fat diet-induced obesity by regulating gut microbiota and its metabolites. Carbohydr Polym. (2022) 281:119046. doi: 10.1016/j.carbpol.2021.119046
11. Li T, Huang S, Wang J, Yin P, Liu H, Sun C. Alginate oligosaccharides protect against fumonisin B1-induced intestinal damage via promoting gut microbiota homeostasis. Food Res Int. (2022) 152:110927. doi: 10.1016/j.foodres.2021.110927
12. Wang Z, Zhao X, Liu X, Lu W, Jia S, Hong T, et al. Anti-diabetic activity evaluation of a polysaccharide extracted from Gynostemma pentaphyllum. Int J Biol Macromol. (2019) 126:209–14. doi: 10.1016/j.ijbiomac.2018.12.231
13. Wang Z, Zhang H, Shen Y, Zhao X, Wang X, Wang J, et al. Characterization of a novel polysaccharide from Ganoderma lucidum and its absorption mechanism in Caco-2 cells and mice model. Int J Biol Macromol. (2018) 118:320–6. doi: 10.1016/j.ijbiomac.2018.06.078
14. Sun X, Wang Z, Hu X, Zhao C, Zhang X, Zhang H. Effect of an antibacterial polysaccharide produced by Chaetomium globosum CGMCC 6882 on the gut microbiota of mice. Foods. (2021) 10:1084. doi: 10.3390/foods10051084
15. Li B, Zhang X, Wang M. Jiao L. Characterization and antioxidant activities of acidic polysaccharides from Gynostemma pentaphyllum (Thunb) Markino. Carbohydr Polym. (2015) 127:209–14. doi: 10.1016/j.carbpol.2015.03.069
16. Liu J, Zhang L, Ren Y, Gao Y, Kang L, Qiao Q. Anticancer and immunoregulatory activity of Gynostemma pentaphyllum polysaccharides in H22 tumor-bearing mice. Int J Biol Macromol. (2014) 69:1–4. doi: 10.1016/j.ijbiomac.2014.05.014
17. Li XL, Wang ZH, Zhao YX, Luo SJ, Zhang DW, Xiao SX, et al. Purification of a polysaccharide from Gynostemma pentaphyllum Makino and its therapeutic advantages for psoriasis. Carbohydr Polym. (2012) 89:1232–7. doi: 10.1016/j.carbpol.2012.04.001
18. Li Q, Feng Y, He W, Wang L, Wang R, Dong L, et al. Post-screening characterisation and in vivo evaluation of an anti-inflammatory polysaccharide fraction from Eucommia ulmoides. Carbohydr Polym. (2017) 169:304–14. doi: 10.1016/j.carbpol.2017.04.034
19. He F, Yang Y, Yang G, Yu L. Studies on antibacterial activity and antibacterial mechanism of a novel polysaccharide from Streptomyces virginia H03. Food Control. (2010) 21:1257–62. doi: 10.1016/j.foodcont.2010.02.013
20. Cao SY, Zhao CN, Xu XY, Tang GY, Corke H, Gan RY, et al. Dietary plants, gut microbiota, and obesity: effects and mechanisms. Trends Food Sci Tech. (2019) 92:194–204. doi: 10.1016/j.tifs.2019.08.004
21. Chen R, Liu B, Wang X, Chen K, Zhang K, Zhang L, et al. Effects of polysaccharide from Pueraria lobata on gut microbiota in mice. Int J Biol Macromol. (2020) 158:740–9. doi: 10.1016/j.ijbiomac.2020.04.201
22. Zhao R, Cheng N, Nakata PA, Zhao L, Hu Q. Consumption of polysaccharides from Auricularia auricular modulates the intestinal microbiota in mice. Food Res Int. (2019) 123:383–92. doi: 10.1016/j.foodres.2019.04.070
23. Yin X, Liao W, Li Q, Zhang H, Liu Z, Zheng X, et al. Interactions between resveratrol and gut microbiota affect the development of hepatic steatosis: a fecal microbiota transplantation study in high-fat diet mice. J Funct Foods. (2020) 67:103883. doi: 10.1016/j.jff.2020.103883
24. Yuan Q, He Y, Xiang PY, Wang SP, Cao ZW, Gou T, et al. Effects of simulated saliva-gastrointestinal digestion on the physicochemical properties and bioactivities of okra polysaccharides. Carbohydr Polym. (2020) 238:116183. doi: 10.1016/j.carbpol.2020.116183
25. Goodrich Julia K, Waters Jillian L, Poole Angela C, Sutter Jessica L, Koren O, Blekhman R, et al. Human genetics shape the gut microbiome. Cell. (2014) 159:789–99. doi: 10.1016/j.cell.2014.09.053
26. Chen G, Xie M, Wan P, Chen D, Ye H, Chen L, et al. Digestion under saliva, simulated gastric and small intestinal conditions and fermentation in vitro by human intestinal microbiota of polysaccharides from Fuzhuan brick tea. Food Chem. (2018) 244:331–9. doi: 10.1016/j.foodchem.2017.10.074
27. Gao M, Li H, Yang T, Li Z, Hu X, Wang Z, et al. Production of prebiotic gellan oligosaccharides based on the irradiation treatment and acid hydrolysis of gellan gum. Carbohydr Polym. (2022) 279:119007. doi: 10.1016/j.carbpol.2021.119007
28. Liu L, Li M, Yu M, Shen M, Wang Q, Yu Y, et al. Natural polysaccharides exhibit anti-tumor activity by targeting gut microbiota. Int J Biol Macromol. (2019) 121:743–51. doi: 10.1016/j.ijbiomac.2018.10.083
29. Canfora EE, Jocken JW, Blaak EE. Short-chain fatty acids in control of body weight and insulin sensitivity. Nat Rev Endocrinol. (2015) 11:577–91. doi: 10.1038/nrendo.2015.128
30. Tian B, Zhao J, An W, Zhang J, Cao X, Mi J, et al. Lycium ruthenicum diet alters the gut microbiota and partially enhances gut barrier function in male C57BL/6 mice. J Funct Foods. (2019) 52:516–28. doi: 10.1016/j.jff.2018.11.034
31. Wu T, Shen M, Guo X, Huang L, Yang J, Yu Q, et al. Cyclocarya paliurus polysaccharide alleviates liver inflammation in mice via beneficial regulation of gut microbiota and TLR4/MAPK signaling pathways. Int J Biol Macromol. (2020) 160:164–74. doi: 10.1016/j.ijbiomac.2020.05.187
32. Deng J, Zhong J, Long J, Zou X, Wang D, Song Y, et al. Hypoglycemic effects and mechanism of different molecular weights of konjac glucomannans in type 2 diabetic rats. Int J Biol Macromol. (2020) 165:2231–43. doi: 10.1016/j.ijbiomac.2020.10.021
33. Ley RE, Bäckhed F, Turnbaugh P, Lozupone CA, Knight RD, Gordon JI. Obesity alters gut microbial ecology. P Nat A Sci. (2005) 102:11070–5. doi: 10.1073/pnas.0504978102
34. Yu C, Ahmadi S, Shen S, Wu D, Xiao H, Ding T, et al. Structure and fermentation characteristics of five polysaccharides sequentially extracted from sugar beet pulp by different methods. Food Hydrocolloid. (2022) 126:107462. doi: 10.1016/j.foodhyd.2021.107462
35. Zhao D, Dai W, Tao H, Zhuang W, Qu M. Chang YN. Polysaccharide isolated from Auricularia auricular-judae (Bull) prevents dextran sulfate sodium-induced colitis in mice through modulating the composition of the gut microbiota. J Food Sci. (2020) 85:2943–51. doi: 10.1111/1750-3841.15319
36. Gong Y, Zhang J, Gao F, Zhou J, Xiang Z, Zhou C, et al. Structure features and in vitro hypoglycemic activities of polysaccharides from different species of Maidong. Carbohydr Polym. (2017) 173:215–22. doi: 10.1016/j.carbpol.2017.05.076
37. Gao R, Zhu C, Li H, Yin M, Pan C, Huang L, et al. Dysbiosis signatures of gut microbiota along the sequence from healthy, young patients to those with overweight and obesity. Obesity. (2018) 26:351–61. doi: 10.1002/oby.22088
38. Chang CJ, Lin CS, Lu CC, Martel J, Ko YF, Ojcius DM, et al. Ganoderma lucidum reduces obesity in mice by modulating the composition of the gut microbiota. Nat Commun. (2015) 6:7489. doi: 10.1038/ncomms8489
Keywords: Gynostemma pentaphyllum, polysaccharide, mice, body weight, gut microbiota
Citation: Li S, Wang Y, Dun W, Han W, Ning T, Sun Q and Wang Z (2022) Effect of Polysaccharide Extracted From Gynostemma Pentaphyllum on the Body Weight and Gut Microbiota of Mice. Front. Nutr. 9:916425. doi: 10.3389/fnut.2022.916425
Received: 09 April 2022; Accepted: 05 May 2022;
Published: 26 May 2022.
Edited by:
Xin Wang, Northwest A&F University, ChinaReviewed by:
Lin Peng, Taizhou University, ChinaBaoming Tian, Zhejiang University of Technology, China
Copyright © 2022 Li, Wang, Dun, Han, Ning, Sun and Wang. This is an open-access article distributed under the terms of the Creative Commons Attribution License (CC BY). The use, distribution or reproduction in other forums is permitted, provided the original author(s) and the copyright owner(s) are credited and that the original publication in this journal is cited, in accordance with accepted academic practice. No use, distribution or reproduction is permitted which does not comply with these terms.
*Correspondence: Qi Sun, c3VucWkyMDE3JiN4MDAwNDA7Y3FudS5lZHUuY24=; Zichao Wang, emN3YW5nJiN4MDAwNDA7aGF1dC5lZHUuY24=