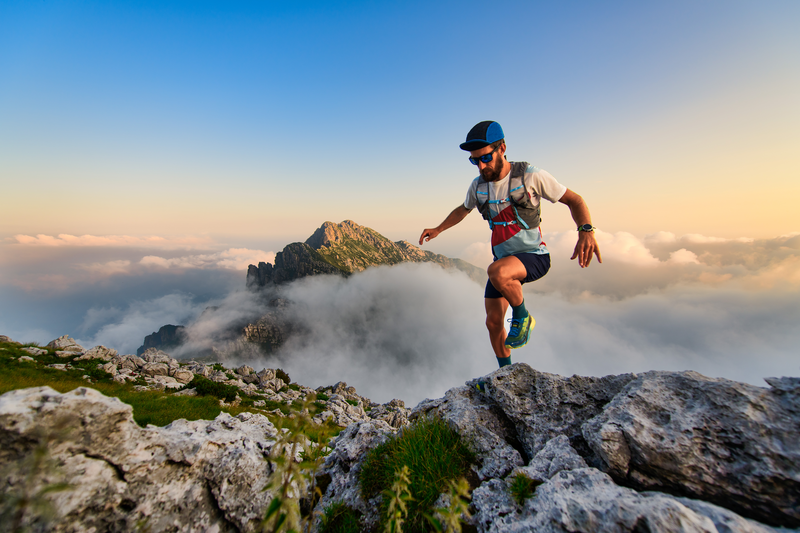
95% of researchers rate our articles as excellent or good
Learn more about the work of our research integrity team to safeguard the quality of each article we publish.
Find out more
ORIGINAL RESEARCH article
Front. Nutr. , 18 May 2022
Sec. Nutrition and Food Science Technology
Volume 9 - 2022 | https://doi.org/10.3389/fnut.2022.913106
This article is part of the Research Topic Innovative Non-Thermal Technologies for The Extraction and Modification of Bioactive Compounds from Food Processing By-Products View all 8 articles
In this work, the investigation mainly focused on ultrasonic-assisted dual-alkali pretreatment and enzymatic hydrolysis of sugarcane bagasse followed by Candida tropicalis fermentation to produce xylitol. The results showed that the combination of NaOH and ammonia water had the best effect by comparing the effects of the four single-alkali (NaOH, KOH, ammonia water, Ca(OH)2) and their mixed double-alkali pretreatments on xylose content. Then, the optimal conditions for ultrasonic-assisted pretreatment and enzymatic hydrolysis of sugarcane bagasse were obtained by response surface methodology. When the ratio of NaOH and ammonia water was 2:1, the mixed alkali concentration (v/v) was 17%, the ultrasonic temperature was 45°C, the ultrasonic power was 300 W, and the ultrasonic time was 40 min, the content of xylose reached a maximum of 2.431 g/L. Scanning electron microscopy showed that sugarcane bagasse by ultrasonic-assisted alkali pretreatment aggravated with more folds and furrows. Moreover, the fermentation results showed that the concentration ratio of enzymatic hydrolysate of sugarcane bagasse affected the xylitol yield, and when concentrated three times, the highest yield of xylitol (54.42%) was obtained.
As one of the main by-products of sugarcane processing to produce sugar, sugarcane bagasse is usually burned or treated as soil fertilizer, resulting in low added value due to its unreasonable disposal (1). The major components of sugarcane bagasse are cellulose, hemicellulose, and lignin, of which hemicellulose is a good source of xylose and can be further hydrolyzed into xylitol by microbial fermentation and enzymatic conversion (2, 3). Xylitol is a natural sweetener extracted from birch, oak, corncob, sugarcane bagasse, and other plant raw materials, which are also widely used in the food industry as food additives (4). Xylitol can be produced using microbial fermentation. The Candida tropicalis has a strong xylose conversion ability and high tolerance to inhibitors such as furfural, acetate, and phenolics in xylose mother liquor (5).
Pretreatment of lignocellulosic biomass is a critical step in xylitol production. The common methods of pretreatment of sugarcane bagasse are physical methods, chemical methods, biological methods, and physical-chemical combination methods. Common physical methods such as mechanical activation (6), microwave treatment (7), and ultrasonic treatment (8) mainly increase the surface area of the raw material by changing the physical structure of the lignocellulosic raw materials, while chemical methods mainly use chemical reagents such as acid, alkali, and ionic liquid to make raw materials swell, thereby disrupting the tightly bound structure of hemicellulose, lignin, and cellulose, reducing the crystallinity of cellulose (9). Alkaline pretreatments, including NaOH, Ca(OH)2, and ammonia water pretreatment, can be used to remove lignin from sugarcane bagasse, which generally swells the sugarcane bagasse and reduces the crystallinity of cellulose (10–12). Besides, biological pretreatment is performed by enzymes or fungi during the raw material storage, and hemicellulose and lignin are partially removed (13). However, the biological pretreatment is still in the theoretical stage and cannot be put into practical production due to its time-consuming and high requirements for reaction conditions.
As a non-thermal food processing technique, ultrasound has been widely applied in various fields of food processing such as crystallization (14), hydrolysis (15), extraction (16), and microbial inactivation. Compared with the conventional thermal techniques, the ultrasound technique presents many advantages such as no chemical residues, minimal thermal effects, and less influence on food quality (17). The effect of ultrasonic on lignocellulosic is mainly derived from mechanical action and cavitation, while ultrasound pretreatment can disrupt cell wall structure to increase the available surface area of biomass. Previous studies have shown that ultrasonic pretreatment combined with chemical methods can improve reaction efficiency and achieve efficient lignin removal (18, 19). Therefore, ultrasonic-assisted alkali pretreatment could be a novel technique to promote the enzymatic hydrolysis of sugarcane bagasse.
The objective of this study was to investigate the effects of ultrasonic-assisted four single-alkali (NaOH, KOH, ammonia water, Ca(OH)2) and their mixed double-alkali pretreatments on the enzymatic hydrolysis of sugarcane bagasse, and to further evaluate the effect of the concentration ratio of the obtained enzymatic hydrolysate on the production of xylitol by Candida tropicalis fermentation.
Sugarcane bagasse was obtained from Guangxi Baiguitang Food Science and Technology Co., Ltd. (Guigang, China) and passed through the 80-mesh screen to obtain the powder for further use. Sodium hydroxide, potassium hydroxide, calcium hydroxide, and ammonia water were from Damao Chemical Reagent Factory (Tianjin, China); xylanase was sourced from Sigma-Aldrich (San Francisco, USA); Candida tropicalis 31949 was purchased from China Industrial Microbiology collection management center (Beijing, China). All reagents were of analytical grade unless otherwise mentioned.
The numeric control ultrasonic cleaning machine (KQ-500DE, Kunshan ultrasonic instruments Co. Ltd., Kunshan, China) was applied in this work. One gram sugarcane bagasse powder and 20 mL NaOH solution (10%) were mixed in a 100 mL conical flask. The treated temperature of the treatment was 60°C and the power of the ultrasonic was 300 W for 50 min. After the ultrasonic treatment, the pH of the mixture solution was adjusted to 5.5, and 0.05 g xylanase was added to the constant temperature water bath oscillator to obtain xylose. The xylose content was measured using the phloroglucinol method with xylose as the reference with a slight modification (20).
Among them, the alkali solution conditions were changed as follows: 20 mL 10% NaOH solution (A); 20 mL 10% KOH solution (B); 20 mL 10% ammonia water (C); 20 mL 10% Ca (OH)2 solution (D); 10 mL 10% NaOH solution and 10 mL 10% KOH solution (AB and BA); 10 mL 10% NaOH solution and 10 mL 10% ammonia water (AC and CA); 10 mL 10% NaOH solution and 10 mL 10% Ca (OH)2 solution (AD and DA); 10 mL 10% KOH solution and 10 mL 10% ammonia water (BC and CB); 10 mL 10% KOH solution and 10 mL 10% Ca (OH)2 solution (BD and DB); 10 mL 10% ammonia water and 10 mL 10% Ca (OH)2 solution (CD and DC).
Scanning electron micrographs (SEM) were taken by a scanning electron microscope (F16502, Phenom, Netherlands). All samples were subsequently coated with a thin gold layer before examining under a scanning electron microscope.
To optimize the preparation conditions and obtain the maximum xylose content, the Box–Behnken experimental design (BBD) of the response surface methodology (RSM) was carried out with five variables at each of the three levels. The most important key independent variables and their optimization ranges, such as the ratio of NaOH and ammonia water (A: 1:1, 2:1, 3:1); mixed alkali concentration (B: 10, 15, 20%, v/v); ultrasonic temperature (C: 30, 40, 50°C); ultrasonic power (D: 200, 300, 400 W) and ultrasonic time (E: 20, 30, 40 min). Forty-six combinations were produced by the BBD as shown in Table 1. The design was analyzed using Design Expert 8.0 for the experiments. All determinations were conducted in triplicate.
After hydrolysis under the above optimal conditions, the hydrolysate was filtered and the obtained partial volume was concentrated under vacuum at 70°C to increase the xylose concentration 2–5-fold. The pH of the original and concentrated hydrolysates was adjusted to 5.5 with CaO and then filtered to use as culture media. The fermentation media containing hydrolysates obtained as a function of different treatments, yeast extract (3 g/L), peptone (3 g/L), (NH4)2SO4 (10 g/L), KH2PO4 (2.4 g/L), MgSO4 (0.2 g/L), and CaCl2 (0.3 g/L) were autoclaved at 115°C for 15 min. Then, pre-cultured Candida tropicalis was inoculated in a sterile flask containing 50 mL of fermentation medium under agitation with 200 rpm at 30°C until the end of fermentation. After incubation, the fermentation media was centrifuged and the supernatant was carefully collected. The xylitol content in the supernatant solutions was measured using the colorimetric assay method with a slight modification (21). The xylitol yield was calculated by the following Equation (1):
The xylose content produced by ultrasound-assisted mixed alkali pretreatment was higher than which obtained in NaOH single alkali pretreatment, and the xylose content was AC >AB > AD (Figure 1A). The xylose content was 1.928 g/L for mixed alkaline solution treatment at AC, which was significantly (P < 0.05) increased by 45% compared to the NaOH single alkali pretreatment. Several factors have been identified to affect the enzymatic hydrolysis of sugarcane bagasse, which include accessible surface area, crystallinity, and polymerization degree of lignocellulose (22). Pretreatment is the process to alter indirect factors and improve direct factors, thus enhancing the accessibility of cellulose. The results on the NaOH and its mixed alkaline treatment of sugarcane bagasse proved that the treatment modifies the composition, structure, and properties of the fibers. Hemicellulose and lignin content decrease, while cellulose crystals disintegrate and dissolve. The sodium ions treatment can loosen up the structure of lignocellulose (23). Lignocellulose structural degradation induced by ultrasonic treatment is attributed to cavitation effects, and reduces its surface area, thus enhancing the subsequent enzymatic hydrolysis rate.
Figure 1. Effects of ultrasonic-assisted (A) NaOH, (B) KOH, (C) ammonia water, and (D) Ca(OH)2 and its mixed alkaline solution on xylose content from sugarcane bagasse enzymatic hydrolysate. Error bars are the standard deviation of three replications and different lowercase letters (a–c) on top of the bars indicate a significant difference (P < 0.05). Different letters are significantly different (P < 0.05).
In addition, the ammonia water pretreatment can remove lignin and inhibit acetic acid produced by fermentation, providing favorable conditions for microbial fermentation of xylitol (24). An example of this is the study carried out by Tizazu et al. (25) in which a xylitol yield of 0.66 g/g was obtained in ultrasound-assisted from sugarcane bagasse using immobilized Candida tropicalis. Another example by Chen et al. (26) was the xylose recovery of 55.54% and ethanol fermentation efficiency of 93.37% from sugarcane bagasse by two-stage ultrasonic-assisted dilute acid.
The xylose content produced by ultrasound-assisted mixed alkali pretreatment was higher than that of KOH single alkali pretreatment, and the xylose content was BC > BA > BD (Figure 1B). It is worth stressing that the xylose content of BC was significantly increased by 19% compared to B (P < 0.05), but the xylose content was not significantly different between the KOH mixed alkali treatments (P > 0.05). Alkaline is an effective reagent for delignification from plants, and alkali pretreatments can increase cellulose digestibility, and they are impressive for lignin solubilization, exhibiting minor cellulose and hemicellulose solubilization. KOH is a strong alkali solution, and hydroxyl ion can destroy the tight structure formed between hemicellulose, lignin, and cellulose, increasing the accessibility of xylanase to hemicellulose (27). Compared to NaOH mixed alkali pretreatment, the growth rate of xylose content with KOH pretreatment did not increase significantly, and the market price of KOH was more expensive, which was not favorable for further fermentation to produce xylitol. In a similar case, Safirzadeh (28) compared the pretreatment of sugarcane bagasse with different concentrations of NaOH and KOH. They found that NaOH was more effective than KOH in reducing the lignin and hemicellulose contents.
The xylose content produced by ultrasound-assisted mixed alkali pretreatment was higher than that of ammonia water single alkali pretreatment, but there was no significant difference in xylose content between the mixed ammonia water pretreatments (P > 0.05) (Figure 1C). Ammonia water pretreatment is an alkali pretreatment method and has been widely used in hemicellulase research (29). The pretreatment of ammonia water fiber expansion resulted in cellulose decrystallization, partial hemicellulose depolymerization, lignin C–O–C bond cleavage, and increased accessible surface area due to structural disruption (30). In addition, ammonia water is cheap, volatile, and can be recycled and reused, so it is an ideal method for pretreatment of sugarcane bagasse enzymatic. It can be seen that ultrasonic-assisted NaOH and ammonia water mixed alkali pretreatment resulted in higher xylose content. Wang et al. (31) obtained a total reducing sugar concentration of 16.23 g/L through NaOH and ammonia water pretreatment of enzymatic hydrolysis from sugarcane bagasse.
The xylose content produced by ultrasound-assisted mixed alkali pretreatment was higher than that of Ca(OH)2 single alkali pretreatment, and the xylose content was DC > DB > DA (Figure 1D). Notably, the xylose content was significantly increased by 18% in the Ca(OH)2 mixed alkaline solution treatment compared with the Ca(OH)2 single alkaline solution treatment (P < 0.05). NaOH pretreatment particularly cleaves the ester bonds in lignin-carbohydrate complexes, and the carbon-to-carbon bonds in lignin molecules, while Ca(OH)2 pretreatment mainly removes acetyl groups (32). Additionally, Ca(OH)2 belongs to a binary strong base and the hydroxyl ion can destroy the tight structure formed between hemicellulose. Ca(OH)2 was slightly soluble in water, which made the solid-liquid separation of enzymatic hydrolysate difficult and unfavorable for further microbial fermentation of xylitol. NaOH has a moderate cost in comparison with KOH and Ca(OH)2. Similarly, Chang et al. (33) reported that NaOH pretreatment was more suitable for treating sugarcane bagasse than Ca(OH)2 pretreatment due to its advantage of more significant enzymatic hydrolysis efficiency and easier lignin recovery.
The surface morphology of the sugarcane bagasse was demonstrated by the SEM micrographs (Figure 2). The untreated sugarcane bagasse had a smooth and continuous surface, which was not conducive to the enzymatic hydrolysis process (Figure 2A). Rough surface (Figure 2B) and even larger holes (Figure 2C) could be observed after the treatment with solutions of single and mixed alkali, and the fibers lost their integrity after the treatment with enzymatic hydrolysis (Figure 2D). These observations demonstrated that ultrasonic-assisted mixed alkali treatment had a significant effect on the structure of sugarcane bagasse, and cellulose became more accessible to enzymes. Similar structural changes were reported earlier for sugarcane bagasse pretreated with short duration microwave (34) and rice straw pretreated with ammonia water soaking pretreatment (35).
Figure 2. The SEM images of sugarcane bagasse with different pretreatments. (A) Without pretreatment, (B) with ultrasonic-assisted single alkali pretreatment, (C) with ultrasonic-assisted mixed alkali pretreatment, (D) with ultrasonic–assisted mixed alkali pretreatment and enzymatic hydrolysis.
From the experimental conditions and corresponding response values shown in Table 1, the experimental data were analyzed by multiple regression. The xylose content was predicted by the second-order polynomial Equation (2), as follows:
where Y is the xylose content; A, B, C, D, and E represent the ratio of NaOH and ammonia water, mixed alkali concentration (%), ultrasonic temperature (°C), ultrasonic power (W), and ultrasonic time (min), respectively.
The analysis of variance (ANOVA) for the response surface quadratic model was summarized in Table 2. The ideal regression equation and the high model significance were confirmed by its high F value (11.0712) and low P-value (P < 0.0001). The goodness-of-fit and adequacy were testified by the determination coefficient (R2 = 0.8985) and adjusted determination coefficient ( = 0.8174), respectively. There was no significance in the lack of fit (P > 0.05) in the model (P-value 0.2351), which indicates the accurate prediction of the model response. As summarized in Table 2, the reaction factors of B (P = 0.0136 < 0.05), C (P < 0.0001), E (P = 0.0006 < 0.01), AB (P = 0.0493 < 0.05), CD (P = 0.0114 < 0.05), DE (P = 0.0002 < 0.01), A2, B2, C2, and D2 (P < 0.0001) were significant in this model.
The 3D response plot shown in Figure 3 were graphical representations of the regression equation. The comprehensive effects of the ratio of NaOH and ammonia water (A), mixed alkali concentration (B), ultrasonic temperature (C) ultrasonic power (D), and ultrasonic time (E) on xylose content were discussed and the optimal condition for xylose content was proposed as well. Figure 3A shows the combined effect of mixed alkali concentration (B) and the ratio of NaOH and ammonia water (A) on xylose content. It indicated that the xylose content decreased at first and then increased with the increasing mixed alkali concentration and the ratio of NaOH and ammonia water. Quadratic effects of ultrasonic temperature (C) and ultrasonic power (D) were shown in Figure 3B. The xylose content increased when ultrasonic power increased from 200 to 300 W. Even though higher ultrasonic power yielded higher xylose content, more thermal energy was generated resulting in the increasing reaction temperature from 30 to 40°C. Therefore, the ultrasonic power in this study was controlled at a moderate degree of 250–350 W to avoid cellulose structure regrouping and improve the xylose content as well. Figure 3C showed that the ultrasonic power (D) and ultrasonic time (E) had a quadratic effect on the xylose content. The xylose content decreased with increasing reaction time.
Figure 3. The effects of (A) the ratio of NaOH and ammonia water; (B) mixed alkali concentration (%); (C) ultrasonic temperature (°C); (D) ultrasonic power (W) and (E) ultrasonic time (min) on xylose content of three-dimensional (3D) response surface curves.
In summary, when the ratio of NaOH and ammonia water was 2:1, the mixed alkali concentration (v/v) was 17%, the ultrasonic temperature was 45°C, the ultrasonic power was 300 W, and the ultrasonic time was 40 min, the maximum content of xylose in the actual experiment (2.431 g/L) was obtained, and this value was consistent with the theoretically predicted value (2.462 g/L).
The production of xylitol by fermentation of Candida tropicalis based on the sugarcane bagasse hydrolysate obtained by the above method was investigated. As shown in Figure 4, the content of xylitol obtained in the original hydrolysate was 0.84 g/L. With the increase in the concentration ratio of the hydrolysate, the obtained xylitol content gradually increased and the maximum value reached 5.56 g/L. However, this value decreased when the concentration ratio exceeded 4 times. By contrast, a similar tendency was obtained for xylitol yields. The yield of xylitol obtained in the original hydrolysate was 34.15%. As the concentration ratio of the hydrolysate increased, the obtained xylitol yield gradually increased and the maximum value reached 54.42%. However, this value decreased when the concentration ratio exceeded 3 times. These results are in agreement with the previous study that the concentration ratio of the sugarcane bagasse hydrolysate significantly affected the xylose reductase and xylitol dehydrogenase activity of yeast which may lead to differences in xylitol yields (36). Moreover, previous studies reported that fermentation inhibitors such as acetic acid and furfural were present in the bagasse hydrolysate (36, 37). In this case, the xylitol fermentation was severely inhibited, resulting in a low yield of xylitol. With the increase of the concentration ratio, the yield of xylitol increased gradually due to the reduction of volatile harmful substances in the hydrolysate. In addition, when the xylose concentration was low, Candida tropicalis mainly use xylose for its growth, which may also lead to low xylitol yield. When the hydrolysate was concentrated to a certain multiple, the xylose concentration gradually approached the most favorable value for fermentation, which increased the yield of xylitol. However, when the concentration ratio exceeded 3 times, the yield of xylitol decreased due to the loss of xylose, the formation of fermentation inhibitors and the accumulation of non-volatile fermentation inhibitors in the hydrolysate. Therefore, the sugarcane bagasse hydrolysate used for xylitol fermentation should be reasonably concentrated and further detoxified.
Figure 4. Influence of the concentration ratio of the enzymatic hydrolysate of sugarcane bagasse on xylitol obtained by fermentation with Candida tropicalis.
In this study, xylitol production from sugarcane bagasse through ultrasonic-assisted dual-alkali pretreatment and enzymatic hydrolysis followed by Candida tropicalis fermentation were investigated. First, the effects of ultrasonic-assisted NaOH, KOH, ammonia water, Ca(OH)2 and their mixed double-alkali solution pretreatment on the enzymatic hydrolysis of sugarcane bagasse were compared. The results showed that the highest xylose content (1.928 g/L) was obtained under the condition of ultrasonic-assisted NaOH and ammonia water mixed alkali pretreatment. Then, the processing parameters were optimized by RSM coupled with BBD. When the ratio of NaOH and ammonia water was 2:1, the mixed alkali concentration (v/v) was 17%, the ultrasonic temperature was 45°C, the ultrasonic power was 300 W, and the ultrasonic time was 40 min, the content of xylose reached a maximum of 2.431 g/L. The sugarcane bagasse pretreated with ultrasonic-assisted alkaline pretreatment had more folds and furrows in external appearance. Moreover, the results of Candida tropicalis fermentation showed that the yield of xylitol increased (maximum 54.42%) with the increase of the concentration ratio of the enzymatic hydrolysate, but this value decreased when the concentration ratio exceeded 3 times. Overall, this study provides a high-value utilization of sugarcane bagasse for xylitol production.
The raw data supporting the conclusions of this article will be made available by the authors, without undue reservation.
LG: writing—original draft, software, formal analysis, and visualization. E-FR: conceptualization, data curation, and writing—review and editing. WT: investigation, resources, and writing—original draft. DN: funding acquisition, supervision, writing—review and editing, resources, and validation. WS: investigation, supervision, writing—review and editing, and project administration. FH and KL: writing—review and editing. All authors contributed to the article and approved the submitted version.
This work was supported by the National Natural Science Foundation of China (32102133) and the Natural Science Foundation of Guangxi Province (2021JJA130374).
The authors declare that the research was conducted in the absence of any commercial or financial relationships that could be construed as a potential conflict of interest.
All claims expressed in this article are solely those of the authors and do not necessarily represent those of their affiliated organizations, or those of the publisher, the editors and the reviewers. Any product that may be evaluated in this article, or claim that may be made by its manufacturer, is not guaranteed or endorsed by the publisher.
1. Faria KCP, Gurgel RF, Holanda JNF. Recycling of sugarcane bagasse ash waste in the production of clay bricks. J Environ Manage. (2012) 101:7–12. doi: 10.1016/j.jenvman.2012.01.032
2. Wang Y, Song H, Hou J-P, Jia C-M, Yao S. Systematic isolation and utilization of lignocellulosic components from sugarcane bagasse. Sep Sci Technol. (2013) 48:2217–24. doi: 10.1080/01496395.2013.791855
3. Wan L, Gao Z, Wu B, Cao F, Jiang M, Wei P, et al. Hydrolysis of corncob hemicellulose by solid acid sulfated zirconia and its evaluation in xylitol production. Appl Biochem Biotechnol. (2021) 193:205–17. doi: 10.1007/s12010-020-03412-9
4. Yoon J, Choi H, An G. Roles of lignin biosynthesis and regulatory genes in plant development. J Integr Plant Biol. (2015) 57:902–12. doi: 10.1111/jipb.12422
5. Rao RS, Jyothi CP, Prakasham RS, Sarma PN, Rao LV. Xylitol production from corn fiber and sugarcane bagasse hydrolysates by Candida tropicalis. Bioresour Technol. (2006) 97:1974–8. doi: 10.1016/j.biortech.2005.08.015
6. Barakat A, Mayer-Laigle C, Solhy A, Arancon RAD, de Vries H, Luque R. Mechanical pretreatments of lignocellulosic biomass: towards facile and environmentally sound technologies for biofuels production. RSC Adv. (2014) 4:48109–27. doi: 10.1039/C4RA07568D
7. Chen W-H, Tu Y-J, Sheen H-K. Disruption of sugarcane bagasse lignocellulosic structure by means of dilute sulfuric acid pretreatment with microwave-assisted heating. Appl Energy. (2011) 88:2726–34. doi: 10.1016/j.apenergy.2011.02.027
8. Liu C-F, Ren J-L, Xu F, Liu J-J, Sun J-X, Sun R-C. Isolation and characterization of cellulose obtained from ultrasonic irradiated sugarcane bagasse. J Agric Food Chem. (2006) 54:5742–8. doi: 10.1021/jf060929o
9. Dionísio SR, Santoro DCJ, Bonan CIDG, Soares LB, Biazi LE, Rabelo SC, et al. Second-generation ethanol process for integral use of hemicellulosic and cellulosic hydrolysates from diluted sulfuric acid pretreatment of sugarcane bagasse. Fuel. (2021) 304:121290. doi: 10.1016/j.fuel.2021.121290
10. Alokika Anu Kumar A, Kumar V, Singh B. Cellulosic and hemicellulosic fractions of sugarcane bagasse: potential, challenges and future perspective. Int J Biol Macromol. (2021) 169:564–82. doi: 10.1016/j.ijbiomac.2020.12.175
11. Su T-C, Fang Z. One-pot microwave-assisted hydrolysis of cellulose and hemicellulose in selected tropical plant wastes by naoh-freeze pretreatment. ACS Sustain Chem Eng. (2017) 5:5166–74. doi: 10.1021/acssuschemeng.7b00509
12. Yu N, Tan L, Sun Z-Y, Nishimura H, Takei S, Tang Y-Q, et al. Bioethanol from sugarcane bagasse: focused on optimum of lignin content and reduction of enzyme addition. Waste Manag. (2018) 76:404–13. doi: 10.1016/j.wasman.2018.03.047
13. Machado A da S, Ferraz A. Biological pretreatment of sugarcane bagasse with basidiomycetes producing varied patterns of biodegradation. Bioresour Technol. (2017) 225:17–22. doi: 10.1016/j.biortech.2016.11.053
14. Ma X, Yang D, Qiu W, Mei J, Xie J. Influence of multifrequency ultrasound-assisted freezing on the flavour attributes and myofibrillar protein characteristics of cultured large yellow croaker (Larimichthys crocea). Front Nutr. (2021) 8:779546. doi: 10.3389/fnut.2021.779546
15. Duangjai A, Saokaew S, Goh B-H, Phisalprapa P. Shifting of physicochemical and biological characteristics of coffee roasting under ultrasound-assisted extraction. Front Nutr. (2021) 8:724591. doi: 10.3389/fnut.2021.724591
16. Niu D, Ren E-F, Li J, Zeng X-A, Li S-L. Effects of pulsed electric field-assisted treatment on the extraction, antioxidant activity and structure of naringin. Sep Purif Technol. (2021) 265:118480. doi: 10.1016/j.seppur.2021.118480
17. Niu D, Zeng X-A, Ren E-F, Xu F-Y, Li J, Wang M-S, et al. Review of the application of pulsed electric fields (PEF) technology for food processing in China. Food Res Int. (2020) 137:109715. doi: 10.1016/j.foodres.2020.109715
18. Sharma V, Nargotra P, Sharma S, Bajaj BK. Efficacy and functional mechanisms of a novel combinatorial pretreatment approach based on deep eutectic solvent and ultrasonic waves for bioconversion of sugarcane bagasse. Renew Energy. (2021) 163:1910–22. doi: 10.1016/j.renene.2020.10.101
19. Ye X, Chen Y. Kinetics study of enzymatic hydrolysis of Paulownia by dilute acid, alkali, and ultrasonic-assisted alkali pretreatments. Biotechnol Bioprocess Eng. (2015) 20:242–8. doi: 10.1007/s12257-014-0490-x
20. Marconi O, Tomasi I, Sileoni V, Bonciarelli U, Guiducci M, Maranghi S, et al. Effects of growth conditions and cultivar on the content and physiochemical properties of arabinoxylan in barley. J Agric Food Chem. (2020) 68:1064–70. doi: 10.1021/acs.jafc.9b05488
21. Scarano S, Pascale E, Minunni M. The early nucleation stage of gold nanoparticles formation in solution as powerful tool for the colorimetric determination of reducing agents: the case of xylitol and total polyols in oral fluid. Anal Chim Acta. (2017) 993:71–8. doi: 10.1016/j.aca.2017.09.020
22. Wang C, Qi W, Liang C, Wang Q, Wang W, Wang Z, et al. Impact of alkaline pretreatment condition on enzymatic hydrolysis of sugarcane bagasse and pretreatment cost. Appl Biochem Biotechnol. (2021) 193:2087–97. doi: 10.1007/s12010-021-03530-y
23. Bartos A, Anggono J, Farkas ÁE, Kun D, Soetaredjo FE, Móczó J, et al. Alkali treatment of lignocellulosic fibers extracted from sugarcane bagasse: composition, structure, properties. Polym Test. (2020) 88:106549. doi: 10.1016/j.polymertesting.2020.106549
24. Zhao C, Qiao X, Shao Q, Hassan M, Ma Z, Yao L. Synergistic effect of hydrogen peroxide and ammonia on lignin. Ind Crops Prod. (2020) 146:112177. doi: 10.1016/j.indcrop.2020.112177
25. Tizazu BZ, Roy K, Moholkar VS. Ultrasonic enhancement of xylitol production from sugarcane bagasse using immobilized Candida tropicalis MTCC 184. Bioresour Technol. (2018) 268:247–58. doi: 10.1016/j.biortech.2018.07.141
26. Chen S-J, Chen X, Zhu M-J. Xylose recovery and bioethanol production from sugarcane bagasse pretreated by mild two-stage ultrasonic assisted dilute acid. Bioresour Technol. (2022) 345:126463. doi: 10.1016/j.biortech.2021.126463
27. Huang G-L, Shi JX, Langrish TAG. Environmentally friendly bagasse pulping with NH4OH–KOH–AQ. J Clean Prod. (2008) 16:1287–93. doi: 10.1016/j.jclepro.2007.06.011
28. Safirzadeh S, Chorom M, Karimi R, Ariz A, Behravan HR, Fadami M. Effects of alkaline pretreatments on chemical composition of sugarcane bagasse for easy degradation in soil. Sugar Tech. (2017) 19:89–94. doi: 10.1007/s12355-016-0435-0
29. Hans M, Garg S, Pellegrini VOA, Filgueiras JG, de Azevedo ER, Guimaraes FEC, et al. Liquid ammonia pretreatment optimization for improved release of fermentable sugars from sugarcane bagasse. J Clean Prod. (2021) 281:123922. doi: 10.1016/j.jclepro.2020.123922
30. Tsutsui S, Sakuragi K, Igarashi K, Samejima M, Kaneko S. Evaluation of ammonia pretreatment for enzymatic hydrolysis of sugarcane bagasse to recover xylooligosaccharides. J Appl Glycosci. (2020) 67:17–22. doi: 10.5458/jag.jag.JAG-2019_0017
31. Wang J, Xu Y, Tang B, Zeng M, Liang Z, Jiang C, et al. Enhanced saccharification of sugarcane bagasse by the optimization of low concentration of NaOH and ammonia pretreatment. Ind Crops Prod. (2021) 172:114016. doi: 10.1016/j.indcrop.2021.114016
32. Kim J, Sunagawa M, Kobayashi S, Shin T, Takayama C. Developmental localization of calcitonin gene-related peptide in dorsal sensory axons and ventral motor neurons of mouse cervical spinal cord. Neurosci Res. (2016) 105:42–48. doi: 10.1016/j.neures.2015.09.003
33. Chang M, Li D, Wang W, Chen D, Zhang Y, Hu H, et al. Comparison of sodium hydroxide and calcium hydroxide pretreatments on the enzymatic hydrolysis and lignin recovery of sugarcane bagasse. Bioresour Technol. (2017) 244:1055–58. doi: 10.1016/j.biortech.2017.08.101
34. Binod P, Satyanagalakshmi K, Sindhu R, Janu KU, Sukumaran RK, Pandey A. Short duration microwave assisted pretreatment enhances the enzymatic saccharification and fermentable sugar yield from sugarcane bagasse. Renew Energy. (2012) 37:109–16. doi: 10.1016/j.renene.2011.06.007
35. Ko JK, Bak JS, Jung MW, Lee HJ, Choi I-G, Kim TH, et al. Ethanol production from rice straw using optimized aqueous-ammonia soaking pretreatment and simultaneous saccharification and fermentation processes. Bioresour Technol. (2009) 100:4374–80. doi: 10.1016/j.biortech.2009.04.026
36. Alves L A, Vitolo M, Felipe M D G A, De Almeida E Silva J B. Xylose reductase and xylitol dehydrogenase activities of Candida guilliermondii as a function of different treatments of sugarcane bagasse hemicellulosic hydrolysate employing experimental design. Appl Biochem Biotech. (2002) 98:403–13. doi: 10.1007/978-1-4612-0119-9_34
Keywords: ultrasonic, enzymatic hydrolysis, sugarcane bagasse, xylitol, pretreatment
Citation: Gai L, Ren E-F, Tian W, Niu D, Sun W, Hang F and Li K (2022) Ultrasonic-Assisted Dual-Alkali Pretreatment and Enzymatic Hydrolysis of Sugarcane Bagasse Followed by Candida tropicalis Fermentation to Produce Xylitol. Front. Nutr. 9:913106. doi: 10.3389/fnut.2022.913106
Received: 05 April 2022; Accepted: 26 April 2022;
Published: 18 May 2022.
Edited by:
Zhi-Hong Zhang, Jiangsu University, ChinaReviewed by:
Daorui Pang, Guangdong Academy of Agricultural Sciences, ChinaCopyright © 2022 Gai, Ren, Tian, Niu, Sun, Hang and Li. This is an open-access article distributed under the terms of the Creative Commons Attribution License (CC BY). The use, distribution or reproduction in other forums is permitted, provided the original author(s) and the copyright owner(s) are credited and that the original publication in this journal is cited, in accordance with accepted academic practice. No use, distribution or reproduction is permitted which does not comply with these terms.
*Correspondence: Debao Niu, aGFwcHluZGJAZ3h1LmVkdS5jbg==
†These authors have contributed equally to this work
Disclaimer: All claims expressed in this article are solely those of the authors and do not necessarily represent those of their affiliated organizations, or those of the publisher, the editors and the reviewers. Any product that may be evaluated in this article or claim that may be made by its manufacturer is not guaranteed or endorsed by the publisher.
Research integrity at Frontiers
Learn more about the work of our research integrity team to safeguard the quality of each article we publish.