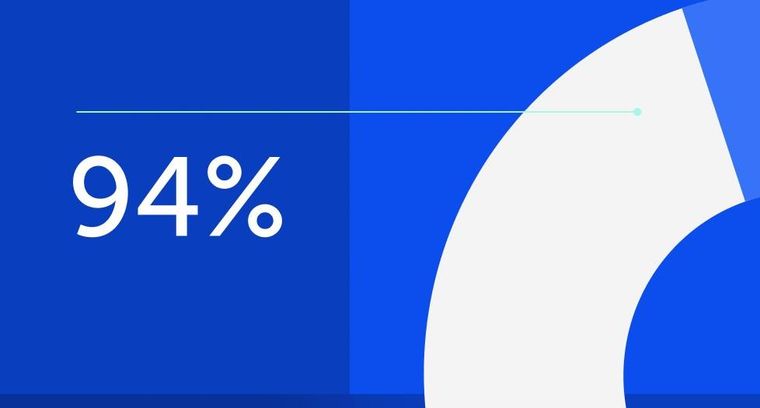
94% of researchers rate our articles as excellent or good
Learn more about the work of our research integrity team to safeguard the quality of each article we publish.
Find out more
ORIGINAL RESEARCH article
Front. Nutr., 17 May 2022
Sec. Food Chemistry
Volume 9 - 2022 | https://doi.org/10.3389/fnut.2022.900138
This article is part of the Research TopicRising Stars in Food ChemistryView all 28 articles
In this study, the fungal community structure, metabolites, antioxidant ability, and taste characteristics of five Fu brick tea (FBT) from different regions of China were determined and compared. A total of 69 operational taxonomic units (OTUs) were identified and assigned into 5 phyla and 27 genera, with Eurotium as the predominant genus in all samples. Hunan (HN) sample had the strongest fungal diversity and richness, followed by Guangxi (GX) sample, and Zhejiang (ZJ) sample had the lowest. GX sample had higher amounts of gallic acid (GA), total catechins, gallocatechin (GC), and epicatechin gallate (ECG) as well as antioxidant activity than the other samples. The levels of total phenolics, total flavonoids, epigallocatechin (EGC), catechin, epicatechin (EC), thearubigins (TRs), and theaflavins (TFs) were the highest in the ZJ sample. Guizhou (GZ) and Shaanxi (SX) samples contained the highest contents of epigallocatechin gallate (EGCG) and gallocatechin gallate (GCG), respectively. Total phenolics, GA, EC, CG, and TFs were positively associated with most of fungal genera. Total phenolic content (TPC), total flavonoid content (TFC), and most of catechins contributed to the antioxidant activities of FBT. HN sample had the strongest sourness and sweetness, ZJ sample had the strongest saltiness, SX sample had the strongest umami, and GZ sample had the strongest astringency, which was ascribed to the varied metabolites. This work reveals that FBT in different regions vary greatly in fungal community, metabolites, antioxidant activity, and taste characteristics, and provides new insight into the quality characteristics formation of FBT in different regions.
Conventional tea can be traditionally categorized into six types according to oxidation and fermentation degree: non-oxidized green tea, slightly oxidized white tea, lightly oxidized yellow tea, partially oxidized oolong tea, fully oxidized black tea, and post-fermented dark tea. As one of the important commercial dark tea varieties, Fu brick tea (FBT) is a special post-fermented dark tea manufactured from raw matured leaves of Camellia sinensis var. sinensis through the following steps: steaming, piling, tea brick pressing, fungal fermentation, and drying (1), in which fungal fermentation played a critical role in the formation of its quality characteristics. FBT has attracted people’s interest throughout China, Korea, and Japan due to its unique sensory attributes and health-promoting effects, including anti-obesity, anti-hyperlipidemic, anti-proliferative, and antidysenteric effects (2–4). The main production regions of FBT are Shaanxi (SX) province, Hunan (HN) province, Guizhou (GZ) province, Zhejiang (ZJ) province, and Guangxi Zhuang Autonomous Region (GX) in China (Figure 1), especially in Hunan and Shaanxi provinces.
The special taste and health benefits of FBT are closely associated with the formation of metabolites during fungal fermentation. Fungal community and its relationship to metabolites formation have been previously investigated (5–8). In the past few years, the biotransformation of chemical constituents related to FBT quality during microbial fermentation has been identified; these constituents include free amino acids, polyphenolic compounds (phenolic acids, catechins and their derivatives, flavonoids, and tea pigments, etc.), purine alkaloids, and terpenoids (9). They are formed due to a sequence of biochemical reactions including oxidation, structural modification, methylation, degradation, condensation, and glycosylation catalyzed by extracellular enzymes from functional core microorganisms (10). Previous studies have revealed that Eurotium, Aspergillus, and Penicillium are the dominant genera during FBT manufacturing (11). The raw materials, processing and cultivation conditions of diverse FBT during manufacturing are different, which might greatly influence their microbial composition and caused the different quality characteristics. It was previously reported that the fungal community composition of various FBT vary greatly (12), which might lead to the great differences of chemical components in FBT. Different filamentous have significant effects on transformation ability of phenolic acid, catechines, flavonoids, and anthocyanins. For instance, both A. niger and E. cristatum reduces galloyl catechins and increase theabrownins (TBs), while E. cristatum contribute to the accumulation of B-ring fission derivatives of degalloyl catechins (13) and A. niger contribute to the accumulation of A-ring fission derivatives of degalloyl catechins in the fermentation (9). Therefore, it is of significant importance for analyzing the fungal community to enhance our understanding of the mechanisms on quality characteristics formation of FBT in different regions. High-throughput sequencing is a rapid, sensitive, and comprehensive technique that provides thousands of reads in a run and accurately describes microbial diversity in food matrices, which received great attention in the last years and become the main method to analysis of microbial composition. However, systematic study on the fungal community by using this approach, and analyze its influence on the metabolites formation of FBT in different regions of China is still lacking.
Numerous literatures have concentrated on the in vitro antioxidant activities of FBT and its association with chemical metabolites (14–16). It is suggested that the antioxidant capacity is recently attributed to flavonols and flavones, tea pigments, caffeine, alkaloids, terpenoids, polysaccharides as well as catechins and their derivatives (3, 14–16). For instance, tea catechins, especially epigallocatechin gallate (EGCG), have been extensively investigated for their excellent antioxidant activity. Likewise, both kaempferol-3-O-glucoside in flavonoids and TBs in tea pigments have significant impact on antioxidant capacity (14). Moreover, it is of great importance to mention that the antioxidant activity in FBT has been reported to be superior compared with other teas (16). FBT in different regions might have diverse antioxidant capacity due to their different metabolites, which have not yet been clearly studied. In addition, previous studies reported that FBT shows strong smoothness, sweetness, and mellowness attributes but with low levels of bitter and astringent. The distinctive taste phenotype of FBT is caused by differences in the contents of various metabolites (5, 8, 12). For instance, catechins, flavonols, and flavones and caffeine are key contributors to the bitterness and astringency of FBT infusion, which greatly influences tea quality (8, 12). The taste profile was mainly detected by an E-tongue sensor system, which simulates human tongue and exhibits good reproducibility, high sensitivity, and low detection limits of tastes compared with the human sense of taste (17). As an emerging technique, E-tongue has been increasingly used to describe the taste characteristics of dry-cured pork, fruit juice, and tea infusion (18, 19). To date, detailed and clear data on the diversity of antioxidant activity and taste quality of FBT produced from different regions of China is rare studied, and their association with bioactive metabolites is not available.
Therefore, the present work aims to investigate the structure and diversity of fungal communities by using Illumina Miseq sequencing of ITS2 region and illustrate the function of microorganisms in the conversion of chemical constituents. Network correlation analysis was used to evaluate the effect of metabolites on the antioxidant activity in vitro. The E-tongue system was also employed to detect the taste attributes of five FBT and clarify the key chemical substances contributing to variations in the taste profile. This study is of significant importance for providing valuable information to enhance our understanding of the mechanisms on quality characteristics formation of FBT in different regions of China.
Catechin, epicatechin gallate (ECG), EC epicatechin (EC), epigallocatechin (EGC), gallocatechin (GC), catechin gallate (CG), EGCG, and gallocatechin gallate (GCG) were acquired from Sigma-Aldrich Co. (St. Louis, MO, United States). High-Performance Liquid Chromatography (HPLC)-grade methanol was obtained from Merck (Darmstadt, Germany). All other chemicals and reagents were of analytical grade. FBT is mainly consumed in Shaanxi province, Hunan province, Guizhou province, Zhejiang province, and Guangxi Zhuang Autonomous Region in China. From these main producing areas, five FBT samples were collected from large factories and named as SX (Shaanxi Xianxi Lamu Tea Co., Ltd.), HN (Anhua Yuntiange Tea Industry Co., Ltd.), GZ (Guizhou Fanjin Tea Industry Co., Ltd.), ZJ (Zhejiang Wuyi Luotuo Jiulong Brick Tea Co., Ltd.), and GX (Guangxi Jinhua Tea Co., Ltd.). All tea samples used in this study were produced in 2018.
Microbial genomic DNA was extracted from FBT samples by using E.Z.N.A. Stool DNA Kit (D4015, Omega, Inc., United States) based on the manufacturer’s instructions. Nuclear-free water was used for blank and elution buffer (50 μL) was used to elute the total DNA. The ITS2 gene was amplified by PCR with forward primers fITS7 (5′-GTGARTCATCGAATCTTTG-3′) and ITS4 (5′-TCCTCCGCTTATTGATATGC-3′). The amplification process was conducted in a reaction mixture (25 μL) with 2.5 μL of each primer, 12.5 μL PCR Premix, 25 ng of template DNA, and PCR-grade water to adjust the volume. The PCR conditions were as follows: initial denaturation for 30 s at 98°C; 35 cycles of denaturation for 10 s at 98°C, annealing for 30 s at 54°C/52°C, and an extension for 45 s at 72°C; and final extension for 10 min at 72°C. The PCR products were extracted from 2% agarose gel, purified by AMPure XT beads (Beckman Coulter Genomics, Danvers, MA, United States), and quantified by Qubit (Invitrogen, United States). After sequencing with the amplicon pools, the size and quantity of the amplicon library were estimated on Agilent 2100 Bioanalyzer (Agilent, United States) and with the Library Quantification Kit for Illumina (Kapa Biosciences, Woburn, MA, United States), respectively. Combined PhiX Control library (v3) (Illumina) and amplicon library (expected at 30%) together. The libraries were sequenced in 250PE MiSeq runs, and one library was sequenced using standard Illumina sequencing primers by both protocols without the need for a third (or fourth) index read.
Total phenolic content in tea samples was determined using Folin-Ciocalteu method in accordance with literature (20). The content was calculated based on standard curve with gallic acid (GA) and expressed as milligram of GA equivalent per gram of tea sample (mg GAE/g d.w.). Total flavonoid content was evaluated using aluminum nitrate colorimetry in accordance with the method of Xiao et al. (21). The result was calculated based on standard curve with rutin and expressed as grams of rutin equivalent per gram of dry tea sample (g RE/g d.w.). The contents of TFs, TRs, and TBs in the tea samples were systematically measured using the method in literature (22).
FBT samples were freeze-dried and crushed using an electric grinder (FW 100, Beijing Yongguangming Medical Instrument Co., Ltd., Beijing, China). About 1 g of tea sample was placed in an extraction bottle and added with deionized water [1:40 (w/v)]. The sample was subjected to thermal extraction at 95°C for 30 min, with shaking every 5 min. The blend was centrifuged for 15 min at 10,000 × g after cooling, and the supernatant was collected. After the volume was adjusted to 50 mL, the sample was stored in darkness for future use.
The contents of catechin, GA, GCG, EGC, GC, EC, EGCG, ECG, and CG in the tea samples were analyzed using HPLC (23). A 0.45 μm PVDF membrane was used to filter the sample extracts. The filtrate (10 μL) was added into the Agilent 1260 Infinity II LC system (Agilent, Santa Clara, United States) for analysis of GA and catechins. An Agilent 5 TC-C18 (2) reverse-phase column (Eclipse Plus, Agilent Technologies, United States) (5 μm particle size; 4.6 mm × 250 mm) was also used. Water containing 0.1% (v/v) formic acid (mobile phase A) and acetonitrile (mobile phase B) were used for chromatographic elution as follows: 0–40 min, 10–35% B; 40–42 min, 35–10% B. The flow rate was kept at 0.8 mL/min for a total running time of 42 min at 25°C. The sample was monitored at 210 nm and subjected to a photodiode array detector (Agilent G7114A variable wavelength detector; Agilent, Santa Clara, United States). The identification and quantification of catechin, GA, GCG, EGC, GC, EC, EGCG, ECG, and CG were performed by comparing their UV spectra and retention times with their authentic standards. The contents were calculated as mg/100 g of dry weight tea samples. Total catechins were the summation of GCG, catechin, EGC, GC, EC, EGCG, ECG, and CG contents.
Several assays with different mechanisms were conducted to assess the antioxidant activities of the FBT samples. ABTS radical cation scavenging activity (ABTS), DPPH radical scavenging activity (DPPH), reducing power (RP), and hydroxyl radical scavenging ability (HAS) were performed according to our previous study (24). An array of vitamin C concentration were carried out to plot the calibration curve, and the results were expressed as milligram of vitamin C per gram dry weight FBT flour (mg VCE/g d.w.). Ferric reducing antioxidant power (FRAP) was conducted using the method reported by Xiao et al. (20). Various concentrations of FeSO4 solution were used to plot the calibration curve. The FRAP values were presented as millimole Fe (II) equivalents per gram dry weight sample.
The electronic tongue analysis of FBT samples from different regions in China was performed using TS-sa402b (INSENT Inc., Japan), which has imitated lipid membrane sensors with a wide area of selection. Every sample was infused with 200 mL of distilled boiled water (40°C) to homogenize (10,000 r/min) for 1 min. The tea infusion was then centrifuged at 3,000 rpm for 10 min at 4°C, and the supernatant was filtered through three layers of gauze. The determination process contained three steps: 30 s of sample detection, 30 s of aftertaste detection and 120 s of cleaning. Each FBT infusion was detected four times, and the average value of the last three cycles was determined, which were analyzed using TS-sa402b Library search software (INSENT, Japan) to characterize the taste quality of FBT samples.
All measurements were conducted in triplicate, and data were presented as mean value ± standard deviation (SD) and analyzed by ANOVA. The significance of differences (p < 0.05) between the average values was determined by Duncan’s multiple range test using SPSS version 16.0 (SPSS Inc., Chicago, United States). Data graphs were plotted by OriginPro 8.1 statistical software (OriginLab Co., United States). With regard to the ITS2 sequencing data, mixed sample amplicons were paired-end sequenced on an Illumina MiSeq platform according to the manufacturer’s protocol from LC-Bio. Raw tags were quality-controlled filtered under specific conditions using fqtrim (V 0.94). Chimeric sequences were filtered using Vsearch software (v2.3.4). The representative sequences of operational taxonomic units (OTUs) were obtained by clustering ≥97% similarity using Vsearch (v2.3.4). Alpha diversity was determined by Chao1, Observed species, Goods coverage, Shannon, and Simpson indices using QIIME (Version 1.8.0). Beta diversity was estimated using (PCoA) and clustered using QIIME software (Version 1.8.0).
A total of 1,667,155 effective reads were generated from the five FBT samples in quadruplicate analysis after filtering quality and removing chimera, with a mean sequence number of 83,358 for each sample (20 samples; 79,869–86,727 reads per sample). On the basis of the 97% similarity threshold, these high-quality sequences were clustered into 69 OTUs for all samples, from the range of 4–19 OTUs per sample. The rarefaction curves for Shannon diversity reached saturation (Supplementary Figure 1), suggesting that the sequencing dataset was adequate to distinguish the species richness in the five regional FBT samples. The diversity and richness of the fungal communities was measured by alpha diversity analysis using ACE, Chao1 (community richness), goods coverage (sequencing depth), Shannon (evenness), and Simpson (richness) indices (Supplementary Table 1). The goods coverage of all the samples was >99.99%, confirming that the sequencing depth was sufficient to saturate the fungal diversity and most fungal microbes had already been captured, consistent with the rarefaction curves. As shown in Supplementary Table 1, no significant differences were observed in the Shannon and Simpson indices (the diversity of detected fungal community) among SX, GZ, and ZJ samples and between HN and GX samples (p > 0.05). The fungal diversity of HN and GX samples was higher than that of SX, GZ, and ZJ samples. In addition, the ACE and Chao1 indexes of the HN sample was higher than those of the other samples, consistent with the differences in the observed OTUs and indicating HN sample shows the highest fungal richness. The results implied that the fungal diversity and richness of the HN sample was the best, followed by GX, and the ZJ sample was the lowest. Rank-abundance curves revealed that a large proportion of the sequences were classified as rare microorganisms represented by only a few sequences, especially for ZJ sample (Supplementary Figure 2). The beta diversity results of NMDS analysis were analyzed based on unweighted uniFrac to estimate the distribution of fungal communities in the five FBT samples. As shown in Supplementary Figure 3, the fungal community structure of the GX sample had the highest level of similarity, while the GZ sample had the lowest. Venn diagram analysis was used to present the number of features that are common and unique to each sample/group of different FBT samples. Only 4 of the identified OTUs were shared in all groups. Eight of 36 OTUs, 6 of 49 OTUs, 6 of 37 OTUs, and 12 of 42 OTUs were shared between the HN sample and the SX, GX, ZJ, and GZ samples, respectively. Six of 34 OTUs, 7 of 22 OTUs, and 5 of 35 OTUs were shared between the SX sample and GX, ZJ, and GZ samples, respectively. Seven of 33 OTUs and 7 of 44 OTUs were shared between the GX sample and ZJ and GZ samples, respectively. Seven of 32 OTUs were shared between ZJ and GZ samples (Figure 2A). These results clearly demonstrated that fungal communities of FBT produced in different regions are greatly different.
Figure 2. The Venn diagram analysis of fungal community compositions of five Fu brick teas in different regions (A). The average relative abundance of fungal communities (B) at phylum level; (C) at genus level; (D) genus level with the highest abundance group removed] of FBT samples.
Ribosomal Database Project (RDP) and unite databases were used for species classification and subsequent analysis to ensure the complete and accurate annotation results at the 70% threshold to investigate the taxonomic composition of microbial community and diversity among the five FBT samples. The detected fungal communities were subdivided into 5 phyla, 15 classes, 20 orders, 23 families, 27 genus, and 33 species. As the taxonomical granularity increased, the percentages of unclassified fungal ITS rRNA gene sequences increased to 1.86 at the species level. As shown in Figure 2B, Ascomycota was the only predominant phylum in all sequences, making up 99.97–100% of the total relative abundance. Basidiomycota, Chytridiomycota, and Zygomycota, even if they were also detected, accounted for a low percentage of the total effective sequences. At the genus level (Figure 2C), Eurotium and Aspergillus had more than 99.97% of all valid reads. Eurotium was predominant in all samples and represented the largest fraction, with average relative abundance of up to 88.84%, consistent with previous reports (24, 25). Eurotium is widely regarded as the main fungus in the fermentation of FBT (26), which has been reported as the key contributor in the formation of the special chemical components of FBT (5). E. cristatum could secrete many extracellular enzymes that have the great potential to transform chemical compounds in tea leaves into other components. For instance, previous studies reported that E. cristatum played an important role in the significant increase of TBs, soluble carbohydrates and three purine alkaloids (27). In addition, E. cristatum transformed phenolic compounds that leading into stronger red and yellow color of tea infusion (28). When the fungal composition was analyzed at the genus level with the dominant group removed (Figure 2D), Aspergillus was overwhelmingly dominant in all samples (SX 98.55%, HN 99.81%, GZ 98.59%, ZJ 94.17%, and GX 99.91%, respectively). Aspergillus is a genus of filamentous and saprophytic fungi that are widely distributed in nature and contains more than 250 species (29). Numerous previous studies have indicated that the quality formation of FBT was also closely related to the metabolism of Aspergillus genus. For instance, Zhao et al. reported that Aspergillus produced a variety of enzymes (pectinesterase, endo-1,4-β-xylanase, catalases, catalase-peroxidases, alkaline protease, and peroxiredoxins) that contributed to the oxidation of tea polyphenols and degradation of plant cell wall (30). Furthermore, the genus Aspergillus make great contribution to the enhancement of taste by secreting enzymes like proteases, α-amylases glucoamylases, xylanases, and other hemicellulose to decompose complex carbohydrates, proteins and lipid into small molecules in FBT and produce unique flavor (31, 32). Besides, it was found that Saccharomycopsis and Debaryomyces were more abundant in the ZJ sample than that in other regions, and Monascus and Wallemia were the two genera that only existed in the ZJ sample. Among them, the genus Debaryomyces has been considered to be an essential candidate microorganism in a fermentation starter for artificial fermentation of FBT, as it has a distinguished influence on the metabolites biotransformation during the manufacturing process. Their enzyme activities enhance the quality of FBT by producing the sweet substance xylitol, vitamins, and other organic acids, contributing to richer taste and flavor (33). Some species of Monascus genus have been widely used in tea fermentation owing to their abilities to decrease hydrogen peroxide and to bio-convert tea polyphenols into TFs and TRs during the fermentation process, exhibiting a strong antioxidant activity by preventing oxidative stress-induced diseases (34). The genus Wallemia was also identified as the core functional microorganisms that associated with the metabolic variations of volatile components during the pile-fermentation of FBT, and may play an important role in contributing the stale and betelnut aroma in dark tea (35). To better understand differences and similarities among different samples, we carried out Z-value transformation on the basis of the heat map and did cluster analysis on fungal genera (Figure 3). Among the 27 detected genera, eight were found in the GZ sample but not in the other samples. These unique genera were Marasmius, Alternaria, Penicillium, Saccharomycetales Incertae sedis unclassified, Mortierella, Sporobolomyces, Eurotiomycetes unclassified, and Monosporascus. Only one unique genus was found in the SX sample, namely, Chaetothyriales unclassified, and it also had the least number of genus-level community composition. LEfSe analysis was carried out to determine the specific fungal taxa that can distinguish FBT samples from different regions. LDA emphasized that about 11 fungal clades, whose abundance in a given regional variety differed from those in the other samples, exhibited statistically significant differences (Figure 4A). LEfSe was used to illustrate differentiating taxa on the phylogenetic tree of the fungal community in the FBT samples from the domain to the species level (Figure 4B). No distinguishable fungal taxa were detected in GZ and SX samples. However, three residual samples appeared as differentially abundant fungi belong to one class (Saccharomycetes), one order (Saccharomycetales), 3 genera (Eurotium, Aspergillus, Thermoascus), and 6 species (Eurotium amstelodami, Aspergillus vitricola, A. penicillioides, A. sp. FLS 2010, Thermoascus aurantiacus, and Eurotium unclassified). The GX sample had the largest number of discriminate taxa, involving class Saccharomycetes, order Saccharomycetales, and genera Aspergillus, Eurotium, and Thermoascus, which were all statistically different and distinctive. Eurotium and Aspergillus were significantly enriched in HN and ZJ samples, respectively.
Figure 4. Cladogram (A) and linear discriminant analysis (LDA) score (B) showed the significant abundance differences of fungal taxa by LEfSe analysis. The node size represents the difference in relative abundance.
The TPC and TFC of the five FBT samples from different regions were evaluated (Figures 5A,B). The TPC and TFC in the ZJ sample were the highest (57.64 mg GAE/g d.w. and 76.24 g RE/g d.w., respectively). The GZ sample had the lowest TPC and TFC, which were 1.80 and 1.99 times lower than those in the ZJ sample. The HN sample contained higher TFC (55.28 g RE/g d.w.) and lower TPC (32.54 mg GAE/g d.w.) than the SX sample. The different results of FBT samples were partly due to their diverse fungal community composition. In this study, network correlation analysis revealed that TPC and TFC were significantly positively associated with Monascus, Debaryomyces, Wallemia, and Quambalaria. TFC was also found to be strongly correlated with Monascus, Wallemia, Thermomyces, and Quambalaria (Figure 6). Previous studies have also reported that the TPC was highly positively related to Cyberlindnera, Wallemia, Penicillium, Ascomycota unclassified, and Eucasphaeria, while negatively correlated with Aspergillus during manufacturing process of FBT (36). The TFC were significantly positively (p < 0.05) associated with Candida, Cyberlindnera, Debaryomyces, and Penicillium, while were negatively correlated with Chlamydomonas during the pile-fermentation period (37). Changes of phenolics and flavonoids are connected with the action of extracellular microbial enzymes, such as glucansucrase and cellulase, secreted by microorganisms including Chlamydomonas, Penicillium, and Aspergillus (9). Moreover, A. pallidofulvus and A. sesamicola make contribution to the accumulation of several flavonoids during fermentation (38, 39). It has been illustrated that the genus Debaryomyces increase both TPC and TFC during pile-fermentation probably attributed to the tannase secreted by the microbe, which could hydrolyze the ester and phenol condensation bonds in gallic tannin to produce simple catechins and GA, and also virtually hydrolyze the acetal bonds of EGCG to produce GA and EGC (40). The Thermomyces genus was reported to be one of the high-temperature resistant genera and occur when the humidity and temperature sharply increased during the early and mid-periods of pile-fermentation. Three non-volatile compounds (L-Tyrosine, L-Phenylalanine and GA) and six volatile compounds (2-ethylhexanol, rose oxide, safranal, α-ionone, β-Ionone, and ionone) were found to be significantly or highly significantly positively correlated with Thermomyces, contributing to the unique taste and flavor of dark tea (37, 41). However, the TFC in FBT declined significantly during manufacturing (36), which could be caused by moisture and heat reaction apart from the influence of microorganisms. The quality of FBT was mainly affected by raw materials and processing technology. Nevertheless, the degradation of TPC, TFC, and flavonoid glycosides during microbial fermentation might contribute to the taste characteristics and special aroma of dark tea (42).
Figure 5. Contents of (A) total polyphenols, (B) total flavonoids, (C) theaflavins, (D) thearubigins and (E) theabrownins of five Fu brick teas in different regions.
Figure 6. Network visualized the relationship between main metabolites and fungal community, as well as antioxidant activity and main metabolites of five Fu brick teas in different regions.
As the main component of tea polyphenols (accounting for approximately 60–80%), catechins are divided into ester and non-ester catechins, and the molecular structure of the former has 1–2 more galloyl groups than the latter, and their content has a certain influence on the characteristic flavor and bioactivities of teas (9). The galloyl moiety of catechins is an essential donor to strong bitterness and astringency, while non-gallated catechins are not astringent (43). Therefore, GA, EGC, GC, EC, catechin, GCG, EGCG, CG, and ECG were identified and quantified by HPLC analysis to better understand the difference in the GA and catechins content of FBT produced from different regions (Table 1). The level of GA in the GX sample was significantly higher than that in the other samples (p < 0.05). GA and its derivatives are abundant in dark tea and exhibit anti-inflammatory, anti-mutagenic, and antioxidant activities as well as cardiovascular protective effects (44). In the present research, GA was positively associated with Monascus, Debaryomyces, Wallemia, and Quambalaria (Figure 6). Previous studies reported that the formation of GA probably resulted from moisture and heat reaction in the early stage and microbial metabolism is the main contributor at the later stage (33) during FBT manufacturing. In the early stage, the galloylated catechins underwent a gradual decomposition through hydrolysis by the effect of moisture and heat treatment, then corresponding degalloyl catechins along with GA were produced (28). Among them, EGCG is one of the most abundant and active galloylated catechins and was reported to be the main pathway for GA biosynthesis (45). In addition, the microbial tannase contributed to the formation of GA through the degradation of gallotannins like 3-O-galloylquinic acid or 1-galloylglucose and 1,2-digalloylglucose (6). Zhao et al. found that the content of EGCG and ECG decreased from 47.3 ± 2.42 and 39.94 ± 2.59 mg/g to 0.08 ± 0.15 and 0.48 ± 0.3 2 mg/g, respectively, after fermentation (30). By contrast, they found that the level of GA increased more than 11 times. Except for GA, the contents of total catechins, GC, and ECG were the highest in the GX sample. Tea samples produced from GZ and SX had the highest contents of EGCG (690.3 ± 0.89 mg/100 g) and GCG (82.48 ± 2.68 mg/100 g), respectively. The ZJ sample had higher amounts of non-galloylated catechins, including EGC, catechin, and EC, which could be attributed to the hydrolysis of galloylated EGCG, CG, and ECG; the lower bitterness and astringency in the ZJ sample could be explained at the same time (46). Interestingly, our results showed that GA, EGC, GC, EC, catechin, CG ECG, and total catechins were closely correlated with Quambalaria (Figure 6). As shown in Figure 6, catechin was positively related to the genus Debaryomyces, Monascus, Wallemia and Quambalaria. EGCG was significantly positively correlated with Alternaria, Penicillium, Marasmius, Udeniomyces, etc. ECG was positively associated with Thermoascus, Saccharomycopsis, Saccharomyces, Thermomyces, Quambalaria, etc. This result was comparable to the results from previous studies. For instance, it has been reported that the catechin was positively associated with Candida, Cyberlindnera, Debaryomyces, and Penicillium (37). ECG was significantly positively correlated with Candida, Wallemia, Penicillium, and Psychrobacter, while negatively correlated with Carnobacterium and Aspergillus (36). EGCG was significantly associated with Penicillium and Alternaria, while negatively associated with Burkholderia-Caballeronia-Paraburkholderia and Aspergillus (12, 36, 47). GCG was significantly correlated with Wallemia, Penicillium, Cyberlindnera, and Candida (36). During microbial fermentation process, the formation of GA and non-gallyolated catechins are resulted from the gradual hydrolysis of gallyolated catechins and polymeric catechins in FBT. At the same time, non-galloylated catechins are further degraded into phenolic acids or converted into complex phenolic tea pigments under the chemical reactions, such as oxidation and polymerization, leading to their decrease (48). For this reason, several catechin derivatives were newly formed in FBT, involving 2S, 3R-6-methoxycar-bonylgallocatechin (MCGE) and 2R, 3R-6-methox-ycarbonylgallocatechin-3-O-gallate (EGCGD) (4). Besides, due to the strong microbial action and hydrolysis during the fermentation of FBT, chemical reactions, such as oxidation and isomerization of catechins, occur, and a large amount of ester catechins are degraded into simple catechins. Larger amount of non-ester catechins to ester catechins leads to less astringent and more mellow taste (49).
Tea pigments such as TBs, TFs, and TRs are the water-soluble oxidation products of polyphenols in tea infusion and significantly contribute to the characteristic taste and color of FBT (46). TFs are primary transitional metabolites, which have been presumed to be produced initially by the oxidative polymerization of tea polyphenols, especially catechins, and were further polymerized into TBs with other compounds (such as polysaccharides, proteins, and caffeine) during pile fermentation (50). The level of TFs was the highest in the ZJ sample (1.36 ± 0.01 mg/g) and was almost equivalent to that in SX sample (1.34 ± 0.03 mg/g). Nevertheless, no statistically significant differences were found in the level of TFs among HN, GZ, and GX samples (Figure 5C). A significant positive correlation (p < 0.05) existed between the levels of TFs and the genera of Eurotium, Monascus, Debaryomyces, Chaetothyriales unclassified, Wallemia, and Spizellomycetaceae unclassified (Figure 6). Lee et al. used Monascus as the strain to ferment tea for thirty days, the content of TFs in tea increased almost nine times compared with unfermented tea (34). It has also been reported that tea fermentation with inoculated Debaryomyces hansenii improved TFs (51). In addition, studies indicated there were other microorganisms including A. pallidofulvus, A. sesamicola, and Penicillium manginii also had significantly (p < 0.05) positive influence on TFs (10). Although the content of TFs in tea leaves is lower than that of TRs and TBs, it is an important factor that affects the brightness, taste intensity, and freshness of tea infusion. The ZJ sample also contained the highest level of TRs (35.84 ± 0.11 mg/g), which was 1.44, 1.95, and 10.28 mg/g higher in SX, GZ, and GX samples, respectively. The TRs content (13.60 ± 0.29 mg/g) was the lowest in the HN sample (Figure 5D). The level of TRs was positively associated with Eurotium and Debaryomyces but negatively correlated with Aspergillus, Udeniomyces, Nectriaceae unclassified, Agaricomycetes unclassified, and Fungi unclassified (Figure 6). A. pallidofulvus and A. sesamicola were also reported to have significant (p < 0.05) negative effects on TRs (10). TRs are one of the main substances that contribute to the red color and taste intensity of tea infusion. Importantly, TRs play a role similar to insulin or insulin-like growth factor in human cells and can become a new alternative for the treatment of overweight and obesity; it functions by activating lipolysis via mitochondrial uncoupling capacity and inactivating phosphoenolpyruvate carboxykinase (PEPCK) promoter as the major enzyme of gluconeogenesis through Forkhead box protein O1a (FOXO1a) phosphorylation (52). With the increasing oxidation degree of tea polyphenols, TRs and TFs are further oxidized and polymerized to TBs under the synergistic action of polyphenol oxidase (PPO), peroxidase (POD), and laccase produced by microorganisms, such as the genera Aspergillus, Pseudomonas, Eurotium, Debaryomyces, Lichtheimia, Blastobotrys, Rosellinia, Geotrichum, Lichtheimia, and Rasamsonia (9). The content of TBs was the highest in the SX sample (59.96 ± 0.29 mg/g) and was 1.04–1.44 folds higher than that in the four other samples, followed by ZJ sample (Figure 5E). This result was comparable to the results from Wang et al. (53), who determined that the levels of TBs, TFs, and TRs in the fermented tea were 98.67 ± 6.55, 0.98 ± 0.13, and 25.39 ± 10.56, respectively. The TBs content had a strong positive correlation with Eurotium but was extremely (p < 0.01) negatively correlated with Thermoascus, Chytridiomycota unclassified, Thermomyces, and Malassezia. Diverse strains isolated from fermentation of dark tea have been applied for producing TBs involving E. cristatum, A. niger, A. tubingensis, A. marvanovae, A. fumigatus, Rhizomucor pusillus, and R. tauricus (21, 53, 54). A. sesamicola was also thought to had highly significant (p < 0.01) negative effects on TBs (53). TBs are characteristic constituents in microbial-fermented tea that can regulate fatty acid metabolism and attenuate hypercholesterolemic capacities (55, 56). Previous research confirmed the gradual decrease in tea polyphenols and the accumulation of TBs when catechins were finally converted into TBs during the pile-fermentation process (50). This finding is consistent with the present results that GX sample had the highest TBs content and the lowest total catechin content. In addition, TFs, TRs, and TBs were significantly (p < 0.05) negatively associated with Aspergillus, with correlation coefficients (r) of −0.597, −0.660, and −0.715, respectively, which might be caused by few enzymes discharged from Aspergillus; these enzymes include proteases, hemicellulases, α-amylases, and cellulases, which could catalyze tea polyphenols, especially catechins and tea pigments.
FBT from different regions had diverse in vitro antioxidant activities. Five regular antioxidant capacity methods with disparate working mechanisms were used to assess the antioxidant activity of the five FBT samples (Figure 7). The GX sample had relatively stronger antioxidant capacity measured using ABTS (84.50 mg VCE/g d.w.), DPPH (210.14 mg VCE/g d.w.), RP (76.85 mg VCE/g d.w.), and FRAP (1.13 mmol Fe(II)/g d.w.) assays compared with tea samples from the other regions. Despite the lower HSA of GX sample than the ZJ sample, no statistically significant differences were detected among the samples. FBT produced in SX exhibited significant (p < 0.05) higher antioxidant activity measured using the five methods than HN and GZ samples. DPPH had no significant (p > 0.05) difference between SX and HN samples. The HN sample demonstrated the lowest value in ABTS (38.06 mg VCE/g d.w.), RP (39.24 mg VCE/g d.w.), FRAP (0.56 mmol Fe(II)/g d.w.), and HSA (52.80 mg VCE/g d.w.). Principal component analysis (PCA) was further carried out to detect cluster formation and reveal relationships among tea samples, bioactive composition, and antioxidant properties. As shown in Figure 8A, the PCA score plot indicated that tea samples from different regions were clearly distinguished from one another. The ZJ sample in the upper right part of Figure 8A was positively related to PC1 and PC2. This sample can be considered as having relatively higher antioxidant activity and higher content of polyphenolic metabolites. Loading plots (Figure 8B) were constructed to distinguish the most significant contributors. ABTS, DPPH, RP, and FRAP were found to be in a similar manner loaded on PC1, indicating that the four properties were connected with antioxidant activity. Moreover, most polyphenolic compounds had high loading on PC1 except for EGCG and TBs, which indicated that polyphenols were good antioxidants. According to literature, the reason behind the discrepancy in antioxidant capacity might be based on the presence of polyphenolic constituents, such as tea polyphenols, flavonoids, catechins, TFs, TRs, etc. in different FBT samples (57). Network correlation analysis was conducted to confirm the relationship between the antioxidant activity and polyphenolic components of tea products (Figure 6). The ABTS, DPPH, RP, and FRAP showed highly significant positive correlations with TPC and TFC (| r| > 0.805 and p < 0.01), while no significant (p > 0.05) correlation was found between HSA and TFC. Numerous studies have linked the increase in the antioxidant activity in tea with phenolic compounds (58, 59). The antioxidant activity of phenolic constituents mainly depends on the quantity of OH-groups and their position. The OH-groups on the 3′−, 4′−, and 5′− position of the B-ring of flavonols and flavones enhance the antioxidant capacity of these substances compared with phenolics with one hydroxy group (12). The glycosylation of flavonoids by microbial enzymatic biotransformation during fermentation contributes to the antioxidant activity of FBT. GA and its derivatives have great antioxidant and antimicrobial capacity, which might be due to the methylization and glycosylation of GA by microbial enzymatic activity (60). As predicted, an extremely high correlation was observed between the results obtained of the five antioxidant activity assays involving ABTS, DPPH, RP, FRAP, and HSA and the GA content, with correlation coefficients of r = 0.952, r = 0.947, r = 0.965, r = 0.979, r = 0.778, respectively (p < 0.01). In vitro antioxidant activity in microbial enzymatically fermented teas including oolong tea, black tea, dark tea, and white tea was positively related to the high level of catechins (15, 61). All the five antioxidant activity methods exhibited the highly significant (p < 0.01) positive correlation with total catechins. Additionally, the antioxidant effects displayed the highest correlation with EGC among all types of catechins (r > 0.780). This remarkable antioxidant capacity of EGC can be ascribed to the higher extent of hydroxylation of the B ring and/or hydroxyl group at the C-3 position of the basic catechin structure, thereby improving the radical scavenging ability (62). Hence, catechins are potent antioxidants and play a protective role of dark tea in disease states related to reactive oxygen species (ROS). HSA showed significant positive correlation with TFs and TRs; no significant (p > 0.05) correlation was found between the four other in vitro antioxidant capacity assays and the two tea pigments. However, FRAP, ABTS, DPPH, and HSA had significant (p < 0.05) or highly significant (p < 0.01) negative correlation with TFs in dark tea in previous study (63). TFs was reported that can decrease the concentration of malondialdehyde and the activity of lactate acid dehydrogenase to improve superoxide dismutase capacity; as such, they are beneficial to increase the antioxidant activity (64). Moreover, colorless flavanols can be partially biotransformed into TFs and TRs under enzymatic oxidation by endogenous polyphenol oxidases and peroxidases, which contribute to the aroma and color of tea infusion (65). No significant (p > 0.05) correlation was observed between in vitro antioxidant activity and TBs (Figure 6). However, other reports noted that dark tea with higher TBs content exhibited stronger superoxide radical scavenging activity (54). Tea pigments are non-enzymatic scavengers that have effective free radical scavenging capacities and kinetics and are similar to polyphenols (66). Hence, TPC, TFC, GA, GC, EGC, catechin, EC, ECG, CG, and tea pigments contributed to the antioxidant capacities of FBT in this study.
Figure 7. Antioxidant activity of five Fu brick teas in different regions. ABTS radical cation scavenging activity (A), DPPH radical scavenging activity (B), reducing power (C), OH radical scavenging ability (D) and ferric reducing antioxidant power (E). Data were recorded as the mean value ± standard deviation of three replicates. Mean values marked by the different letters among the samples denoted significant difference (p < 0.05).
Figure 8. Principal component analysis score (A) and loading plot (B) assessing the metabolites and antioxidant activity of five Fu brick teas in different regions.
Phenolic compounds, particularly gallic acid and catechins, exhibited greatly positive relationship with antioxidant activities in microbial fermented dark teas (e.g., Pu-erh tea, Fu brick tea, Liubao tea) (3, 14). Fungal communities influenced the metabolites of FBT during fermentation, thus leading to the variant antioxidant activity of FBT. Previous studies reported that TPC, TFC, and TFs are the main contributors to the strong antioxidant activity of dark tea (14). The TPC, TFC, and TFs in the ZJ sample is higher compared with SX, HN, and GZ samples might possibly be due to the higher abundance of Monascus, Debaryomyces, Wallemia, and Quambalaria (Figure 6), thus, ZJ sample was noted with stronger antioxidant activities than the SX, HN, and GZ samples. Furthermore, previous studies reported that GA has been considered to be one of the key antioxidants in dark tea (67), similar result was also found in this study. For instance, it was found that GA and all antioxidant assays were highly positively correlated (Figure 6), higher GA in ZJ and GX samples is one of an important reason for their higher antioxidant activity compared with the other three FBT. Besides, the characteristics of gallic acid, catechins, and tea pigments, which can act synergistically, additively or antagonistically should also be considered for the observed antioxidant activity of different FBT.
Differences in the taste quality attributes of dark tea were investigated by E-tongue and presented in the spider plot (Figure 9A). The HN sample had the strongest sourness and sweetness; the ZJ sample had the strongest saltiness; the SX sample had the strongest umami; and the GZ sample had the strongest astringency among the FBT samples from different regions. No statistically significant difference (p > 0.05) in bitterness and richness was found among the FBT samples. The taste attributes of the FBT samples were clustered into three groups: HN, SX-ZJ, and GZ-GX (Figure 9B). The cluster of SX-ZJ samples demonstrated close taste characteristics. The GZ sample indicated similar taste attributes to the GX sample. The distinctive taste phenotype of FBT could be due to differences in the levels of various metabolites. Hence, network correlation analysis was performed to better understand the association between taste profiles and main constituents of dark tea (Figure 10). The bitterness of the FBT samples was positively related to EGCG (| r| = 0.576, p < 0.05) but negatively correlated with TFs (| r| = 0.616, p < 0.05), indicating that EGCG is the main contributor to the bitter taste of FBT. EGCG belongs to ester-type catechins and is thought to be the main source of bitterness in tea. The acceptance of green tea is related to bitterness genes, and that bitter taste is mainly linked with EGCG content (68), consistent with our research. Caffeine and several amino acids (arginine, alanine, etc.) also result in the bitter taste of tea infusion (8). Furthermore, the astringency of FBT had the most significant correlation with EGCG (| r| = 0.526 and p < 0.05). The amount of catechins in tea infusion greatly contributes to the bitterness and astringency of tea. However, previous studies reported that the level of catechins was sharply reduced under the action of enzymes during microbial fermentation (69). This finding may explain the decrease in bitterness and astringency and the increase in the mellow taste of FBT compared with green tea (8, 9, 25). The sweetness in tea was significantly (p < 0.05) negatively associated with GCG and tea pigments (TBs, TRs, and TFs). Sweet taste was related to soluble sugars including monosaccharides (most commonly glucose, galactose, fructose), polysaccharides, oligosaccharides, and a small number of other sugars (35). Sweetness is not the main taste of tea leaves, but it can harmonize the bitterness and astringency in tea soup. From our results, a negative relationship was found between sourness and the contents of GC and TRs. Tea contains a variety of organic acids (up to 3% of dry matter) mainly including GA, citric acid, ascorbic acid, malic acid, fatty acid, oxalic acid, etc. Most of the acid substances in tea are esterified with other compounds, such as alcohols, through processing, and the remaining part enters the tea soup to play a flavoring role (34). For example, soluble fruit acid is viscous and can increase the concentration of tea soup, making the tea taste richer and have better sense of hierarchy. Moreover, the main compounds responsible for the umami taste in tea infusion are amino acids such as theanine, glutamic acid, glutamine, aspartic acid, asparagine, etc., of which theanine and glutamate have the most remarkable effect (40). Interestingly, though the saltiness in FBT almost exhibited significant (p < 0.05) or highly significant (p < 0.01) positive correlations to all metabolites measured, the salty taste is mainly the result of the action of monovalent ions, such as potassium and sodium, and the corresponding negative ions, which are very low in content and are often masked by other tastes. Despite the lack of significant (p > 0.05) difference between richness and the 15 metabolites in our result, tea polyphenols and tea polysaccharides in tea infusion mainly contributed to richness (17). In fact, the taste characteristics of different FBT samples are not only depended by main metabolites catechins, tea polyphenols and tea pigments investigated in this study, some of other metabolites such as amino acids, soluble proteins, peptides, fatty acids, polysaccharides, etc. might also contributed to the taste attributes of FBT. These metabolites can act synergistically, additively or antagonistically with catechins to affect the taste attributes of FBT. Further research is essential to perform on identification of these compounds and their relationship with fungal community, as well as clarify their contribution to the taste characteristics of FBT.
Figure 9. Spider plot (A) and dendrogram plot (B) of the taste characteristics of five Fu brick teas in different regions evaluated by E-tongue. *p < 0.05, **p < 0.01, ***p < 0.001
This study systematically characterized the diversity of fungal communities, chemical composition, antioxidant activity, and taste quality of FBT samples from five different regions of China. The relationship between bioactive metabolites and fungal communities and the key chemical substances contributing to the variations in antioxidant activity and taste profile were assessed. Results showed a total of 69 OTUs, which assigned into 5 phyla, 15 classes, 20 orders, 23 families, 27 genus, and 33 species, with Eurotium as predominant genus. The HN sample had the strongest fungal diversity and richness, followed by GX, and the ZJ sample had the lowest. Additionally, 15 metabolites (including total phenolics, total flavonoids, GA, 8 catechins, total catechins and 3 tea pigments) were evaluated. Higher contents of total phenolics, total flavonoids, TFs, TRs, catechin, EC, and CG were found in the ZJ sample. Higher levels of TBs and GCG were found in the SX sample. Higher contents of GA, GC, EGC, ECG, and total catechins were found in the GX sample. TPC, GA, EC, CG, and TFs were positively associated with most genera, while TFC, catechins, and TBs were negatively correlated. The antioxidant activities of FBT exhibited significant (p < 0.05) differences in the ABTS, DPPH, RP, FRAP, and HSA of the FBT samples from different regions. The GX sample had relatively stronger antioxidant capacity. The network correlation analysis uncovered that TPC, TFC, and most catechins contributed to the antioxidant activities in FBT. The taste phenotypes of FBT were classified into HN, SX-ZJ, and GZ-GX. The HN sample had the strongest sourness and sweetness; the ZJ sample showed the strongest saltiness; the SX sample showed the strongest umami; and the GZ sample showed the strongest astringency. This study provides insights into the fungal community in FBT and demonstrates the potential role of fungal community in metabolite formation to improve the quality characteristics of FBT products.
The raw data supporting the conclusions of this article will be made available by the authors, without undue reservation.
YC: methodology, writing—original draft, and investigation. JC: writing—original draft, data curation, and investigation. RC: methodology and investigation. XW, LH, and LX: investigation. ZL and YW: resources. ZL: conceptualization. MZ: supervision, methodology, and writing—review and editing. YX: project administration, supervision, funding acquisition, conceptualization, investigation, data curation, validation, formal analysis, methodology, writing—original draft, writing—review, and editing. All authors have read and agreed to the published version of the manuscript.
This work was financially supported by Natural Science Foundation of Hunan Province (No. 2020JJ5243), the Science and Technology Innovation Program of Hunan Province (Nos. 2020RC2055 and 2021NK4260), Natural Science Foundation of China (Nos. 32002095 and 32172217), and Excellent Youth Foundation of Education Department of Hunan Province (No. 19B269).
The authors declare that the research was conducted in the absence of any commercial or financial relationships that could be construed as a potential conflict of interest.
All claims expressed in this article are solely those of the authors and do not necessarily represent those of their affiliated organizations, or those of the publisher, the editors and the reviewers. Any product that may be evaluated in this article, or claim that may be made by its manufacturer, is not guaranteed or endorsed by the publisher.
We thank the Key Technologies Research and Development Program of Hunan Province (Nos. 2020NK2030 and 2020WK2017), the Hunan Provincial Natural Science Foundation of China (No. 2019JJ50238), and the Changsha City Outstanding Innovative Youth Training Program (No. kq2107015).
The Supplementary Material for this article can be found online at: https://www.frontiersin.org/articles/10.3389/fnut.2022.900138/full#supplementary-material
1. Chen GJ, Peng YJ, Xie MH, Xu WQ, Chen CX, Zeng XX, et al. A critical review of Fuzhuan brick tea: processing, chemical constituents, health benefits and potential risk. Crit Rev Food Sci Nutr. (2021) 1–18. doi: 10.1080/10408398.2021.2020718 [Epub ahead of print].
2. Du Y, Yang WR, Yang CC, Yang XB. A comprehensive review on microbiome, aromas and flavors, chemical composition, nutrition and future prospects of Fuzhuan brick tea. Trends Food Sci Technol. (2021) 119:452–66. doi: 10.1016/j.tifs.2021.12.024
3. Ma BS, Wang JC, Xu CC, Wang ZY, Yin DF, Zhou BX, et al. Interrelation analysis between phenolic compounds and in vitro antioxidant activities in Pu-erh tea. LWT Food Sci Technol. (2022) 158:113117. doi: 10.1016/j.lwt.2022.113117
4. Lin FJ, Wei XL, Liu HY, Li H, Xia Y, Wu DT, et al. State-of-the-art review of dark tea: from chemistry to health benefits. Trends Food Sci Technol. (2021) 109:126–38. doi: 10.1016/j.tifs.2021.01.030
5. Zhang C, Guo J, Zhang Z, Tian S, Liu Z, Shen C. Biochemical components and fungal community dynamics during the flowering process of Moringa-Fu brick tea, a novel microbially fermented blended tea. LWT Food Sci Technol. (2021) 140:110822. doi: 10.1016/j.lwt.2020.110822
6. Xu J, Hu FL, Wang W, Wan XC, Bao GH. Investigation on biochemical compositional changes during the microbial fermentation process of Fu brick tea by LC-MS based metabolomics. Food Chem. (2015) 186:176–84. doi: 10.1016/j.foodchem.2014.12.045
7. Li Q, Li Y, Luo Y, Zhang Y, Chen Y, Lin H, et al. Shifts in diversity and function of the bacterial community during the manufacture of Fu brick tea. Food Microbiol. (2019) 80:70–6. doi: 10.1016/j.fm.2019.01.001
8. Li Q, Jin Y, Jiang R, Xu Y, Zhang Y, Luo Y, et al. Dynamic changes in the metabolite profile and taste characteristics of Fu brick tea during the manufacturing process. Food Chem. (2021) 344:128576. doi: 10.1016/j.foodchem.2020.128576
9. Zhu MZ, Li N, Zhou F, Ouyang J, Lu DM, Xu W, et al. Microbial bioconversion of the chemical components in dark tea. Food Chem. (2020) 312:126043. doi: 10.1016/j.foodchem.2019.126043
10. Zhou BX, Ma CQ, Ren XY, Xia T, Li XHLC-MS. /MS-based metabolomic analysis of caffeine-degrading fungus Aspergillus sydowii during tea fermentation. J Food Sci. (2020) 85:477–85. doi: 10.1111/1750-3841.15015
11. Zhao XX, Wang YR, Cai WC, Yang MJ, Zhong XD, Guo Z, et al. High-throughput sequencing-based analysis of microbial diversity in rice Wine Koji from different areas. Curr Microbiol. (2020) 77:882–9. doi: 10.1007/s00284-020-01877-9
12. Li MY, Xiao Y, Zhong K, Gao H. Study on taste characteristics and microbial communities in Pingwu Fuzhuan brick tea and the correlation between microbiota composition and chemical metabolites. J Food Sci Technol. (2022) 59:34–45. doi: 10.1007/s13197-021-04976-y
13. Xie HF, Kong YS, Li RZ, Nothias L-F, Melnik A, Zhang H, et al. Feature-based molecular networking analysis of the metabolites produced by in vitro solid-state fermentation reveals pathways for the bioconversion of epigallocatechin gallate. J Agric Food Chem. (2020) 68:7995–8007. doi: 10.1021/acs.jafc.0c02983
14. Lv HP, Zhang Y, Shi J, Lin Z. Phytochemical profiles and antioxidant activities of Chinese dark teas obtained by different processing technologies. Food Res Int. (2017) 100:486–93. doi: 10.1016/j.foodres.2016.10.024
15. Tang GY, Zhao CN, Xu XY, Gan RY, Cao SY, Shang A, et al. Phytochemical composition and antioxidant capacity of 30 Chinese teas. Antioxidants. (2019) 8:180. doi: 10.3390/antiox8060180
16. Cao SY, Li BY, Gan RY, Mao QQ, Wang YF, Shang A, et al. The in vivo antioxidant and hepatoprotective actions of selected Chinese teas. Foods. (2020) 9:262. doi: 10.3390/foods9030262
17. Cheng LZ, Wang YF, Zhang JR, Xu LR, Zhou H, Wei K, et al. Integration of non-targeted metabolomics and E-tongue evaluation reveals the chemical variation and taste characteristics of five typical dark teas. LWT Food Sci Technol. (2021) 150:111875. doi: 10.1016/j.lwt.2021.111875
18. Tian X, Li ZJ, Chao YZ, Wu ZQ, Zhou MX, Xiao ST, et al. Evaluation by electronic tongue and headspace-GC-IMS analyses of the flavor compounds in dry-cured pork with different salt content. Food Res Int. (2020) 137:109456. doi: 10.1016/j.foodres.2020.109456
19. Vitalis F, Tjandra Nugraha D, Aouadi B, Aguinaga Bósquez JP, Bodor Z, Zaukuu J-LZ, et al. Detection of monilia contamination in plum and plum juice with NIR spectroscopy and electronic tongue. Chemosensors. (2021) 9:355. doi: 10.3390/chemosensors9120355
20. Xiao Y, Wu X, Yao XS, Chen YL, Ho CT, He C, et al. Metabolite profiling, antioxidant and α-glucosidase inhibitory activities of buckwheat processed by solid-state fermentation with Eurotium cristatum YL-1. Food Res Int. (2021) 143:110262. doi: 10.1016/j.foodres.2021.110262
21. Xiao Y, He C, Chen YL, Ho CT, Wu X, Huang YX, et al. UPLC–QQQ–MS/MS-based widely targeted metabolomic analysis reveals the effect of solid-state fermentation with Eurotium cristatum on the dynamic changes in the metabolite profile of dark tea. Food Chem. (2022) 378:131999. doi: 10.1016/j.foodchem.2021.131999
22. Jiang YW, Hua JJ, Wang B, Yuan HB, Ma HL. Effects of variety, season, and region on theaflavins content of fermented Chinese Congou black tea. J Food Q. (2018) 2018:5427302. doi: 10.1155/2018/5427302
23. Li YJ, Zhang S, Sun YM. Measurement of catechin and gallic acid in tea wine with HPLC. Saudi J Biol Sci. (2020) 27:214–21. doi: 10.1016/j.sjbs.2019.08.011
24. Chen YL, Wang YL, Chen JX, Tang H, Wang CH, Li ZJ, et al. Bioprocessing of soybeans (Glycine max L.) by solid-state fermentation with Eurotium cristatum YL-1 improves total phenolic content, isoflavone aglycones, and antioxidant activity. RSC Adv. (2020) 10:16928–41. doi: 10.1039/C9RA10344A
25. Mao Y, Wei BY, Teng JW, Huang L, Xia N. Analyses of fungal community by Illumina MiSeq platforms and characterization of Eurotium species on Liupao tea, a distinctive post fermented tea from China. Food Res Int. (2017) 99:641–9. doi: 10.1016/j.foodres.2017.06.032
26. Mo HZ, Zhang H, Li YQ, Zhu Y. Antimicrobial activity of the indigenously microbial fermented Fuzhuan brick-tea. J Biotechnol. (2008) 136:S722–722. doi: 10.1016/j.jbiotec.2008.07.1719
27. Xiao Y, Zhong K, Bai JR, Wu YP, Gao H. Insight into effects of isolated Eurotium cristatum from Pingwu Fuzhuan brick tea on the fermentation process and quality characteristics of Fuzhuan brick tea. J Sci Food Agric. (2020) 100:3598–607. doi: 10.1002/jsfa.10353
28. Zhu YF, Chen JJ, Ji XM, Hu X, Ling TJ, Zhang ZZ, et al. Changes of major tea polyphenols and production of four new B-ring fission metabolites of catechins from post-fermented Jing-Wei Fu brick tea. Food Chem. (2015) 170:110–7. doi: 10.1016/j.foodchem.2014.08.075
29. Samson RA, Visagie CM, Houbraken J, Hong S-B, Hubka V, Klaassen CHW, et al. Phylogeny, identification and nomenclature of the genus Aspergillus. Stud Mycol. (2014) 78:141–73. doi: 10.1016/j.simyco.2014.07.004
30. Zhao M, Su XQ, Nian B, Chen LJ, Zhang DL, Duan SM, et al. Integrated meta-omics approaches to understand the microbiome of spontaneous fermentation of traditional Chinese pu-erh tea. Msystems. (2019) 4:e00680–19. doi: 10.1128/mSystems.00680-19
31. Ward OP, Qin WM, Dhanjoon J, Ye J, Singh A. Physiology and biotechnology of Aspergillus. Adv Appl Microbiol. (2005) 58:1–75. doi: 10.1016/S0065-2164(05)58001-8
32. Li Z, Huang L, Xia N, Teng J, Wei B, Peng D. Amount of Eurotium sp. in Chinese Liupao tea and its relationship with tea quality. J Appl Microbiol. (2020) 128:1658–68. doi: 10.1111/jam.14589
33. Xu A, Wang Y, Wen J, Liu P, Liu Z, Li Z. Fungal community associated with fermentation and storage of Fuzhuan brick-tea. Int J Food Microbiol. (2011) 146:14–22. doi: 10.1016/j.ijfoodmicro.2011.01.024
34. Lee YK, Lee SI, Kim JS, Yang SH, Lee I, Kim SD, et al. Antioxidant activity of green tea fermented with Monascus pilosus. J Appl Biol Chem. (2012) 55:19–25. doi: 10.3839/jabc.2011.054
35. Li Q, Hong X, Zheng XX, Xu YQ, Lai XM, Teng CQ, et al. Characterization of key aroma compounds and core functional microorganisms in different aroma types of Liupao tea. Food Res Int. (2022) 152:110925. doi: 10.1016/j.foodres.2021.110925
36. Li Q, Huang JN, Li YD, Zhang YY, Luo Y, Chen Y, et al. Fungal community succession and major components change during manufacturing process of Fu brick tea. Sci Rep. (2017) 7:6947. doi: 10.1038/s41598-017-07098-8
37. Hu S, He C, Li YC, Yu Z, Chen YQ, Wang YM, et al. Changes of fungal community and non-volatile metabolites during pile-fermentation of dark green tea. Food Res Int. (2021) 147:110472. doi: 10.1016/j.foodres.2021.110472
38. Wang ZH, Zheng CQ, Ma CQ, Ma BS, Wang JC, Zhou BX, et al. Comparative analysis of chemical constituents and antioxidant activity in tea-leaves microbial fermentation of seven tea-derived fungi from ripened Pu-erh tea. LWT Food Sci Technol. (2021) 142:111006. doi: 10.1016/j.lwt.2021.111006
39. Ma Y, Ling TJ, Su XQ, Jiang B, Nian B, Chen LJ, et al. Integrated proteomics and metabolomics analysis of tea leaves fermented by Aspergillus niger, Aspergillus tamarii and Aspergillus fumigatus. Food Chem. (2021) 334:127560. doi: 10.1016/j.foodchem.2020.127560
40. Bajpai B, Patil S. Induction of tannin acyl hydrolase (EC 3.1.1.20) activity in some members of fungi imperfecti. Enzyme Microb Technol. (1997) 20:612–4. doi: 10.1016/s0141-0229(96)00206-2
41. Hu S, He C, Li YC, Yu Z, Chen YQ, Wang YM, et al. The formation of aroma quality of dark tea during pile-fermentation based on multi-omics. LWT Food Sci Technol. (2021) 147:111491. doi: 10.1016/j.lwt.2021.111491
42. Castiglioni S, Damiani E, Astolfi P, Carloni P. Influence of steeping conditions (time, temperature, and particle size) on antioxidant properties and sensory attributes of some white and green teas. Int J Food Sci Nutr. (2015) 66:491–7. doi: 10.3109/09637486.2015.1042842
43. Obanda M, Owuor PO, Mang’oka R. Changes in the chemical and sensory quality parameters of black tea due to variations of fermentation time and temperature. Food Chem. (2001) 75:395–404. doi: 10.1016/S0308-8146(01)00223-0
44. Li HS, Fang QY, Nie QX, Hu JL, Yang C, Huang T, et al. Hypoglycemic and hypolipidemic mechanism of tea polysaccharides on type 2 diabetic rats via gut microbiota and metabolism alteration. J Agric Food Chem. (2020) 68:10015–28. doi: 10.1021/acs.jafc.0c01968
45. Tanaka T, Umeki H, Nagai S, Shii T, Matsuo Y, Kouno I. Transformation of tea catechins and flavonoid glycosides by treatment with Japanese post-fermented tea acetone powder. Food Chem. (2012) 134:276–81. doi: 10.1016/j.foodchem.2012.02.136
46. Zheng WJ, Wan XC, Bao GH. Brick dark tea: a review of the manufacture, chemical constituents and bioconversion of the major chemical components during fermentation. Phytochem Rev. (2015) 14:499–523. doi: 10.1007/s11101-015-9402-8
47. Zeng Q, Lv SH, Li X, Hu X, Liang Y, Fan C, et al. Bioactive ingredients and microbial diversity of Fuzhuan tea produced from different raw materials. Food Sci. (2020) 41:69–77. doi: 10.7506/spkx1002-6630-20190927-333
48. Ge YH, Bian XQ, Sun BQ, Zhao M, Ma Y, Tang YP, et al. Dynamic profiling of phenolic acids during Pu-erh tea fermentation using derivatization liquid chromatography-mass spectrometry approach. J Agric Food Chem. (2019) 67:4568–77. doi: 10.1021/acs.jafc.9b00789
49. Guo X, Ho CT, Schwab W, Wen Z, Zhu H, Liu Q, et al. Qualitative and quantitative changes of non-volatile compounds and taste profiles in Wuyi rock tea during the manufacturing processes. J Food Bioact. (2021) 14:14270. doi: 10.31665/JFB.2021.14270
50. Wang QP, Gong JS, Chisti Y, Sirisansaneeyakul S. Production of theabrownins using a crude fungal enzyme concentrate. J Biotechnol. (2016) 231:250–9.
51. Sansone C, Rita Massardo D, Pontieri P, Maddaluno L, De Stefano M, Maurizio Tredici S, et al. Isolation of a psychrotolerant Debaryomyces hansenii strain fromfermented tea plant (Camellia sinensis) leaves. J Plant Interact. (2007) 2:169–74. doi: 10.1080/17429140701585169
52. Susanto H, Kharisma VD, Listyorini D, Taufiq A, Sunaryono, Aulanni’am A. Effectivity of black tea polyphenol in adipogenesis related IGF-1 and its receptor pathway through in silico based study. J Phys. (2018) 1093:012037. doi: 10.1088/1742-6596/1093/1/012037
53. Wang QP, Gong JS, Chisti Y, Sirisansaneeyakul S. Fungal isolates from a pu-erh type tea fermentation and their ability to convert tea polyphenols to theabrownins. J Food Sci. (2015) 80:M809–17. doi: 10.1111/1750-3841.12831
54. Wang YW, Zhang MY, Zhang ZZ, Lu HQ, Gao XL, Yue PX. High-theabrownins instant dark tea product by Aspergillus niger via submerged fermentation: α-glucosidase and pancreatic lipase inhibition and antioxidant activity. J Sci Food Agric. (2017) 97:5100–6. doi: 10.1002/jsfa.8387
55. Huang FJ, Zheng XJ, Ma XH, Jiang RQ, Zhou WY, Zhou SP, et al. Theabrownin from Pu-erh tea attenuates hypercholesterolemia via modulation of gut microbiota and bile acid metabolism. Nat Commun. (2019) 10:4971–87. doi: 10.1038/s41467-019-12896-x
56. Yue SJ, Zhao D, Peng CX, Tan C, Wang QP, Gong JS. Effects of theabrownin on serum metabolites and gut microbiome in rats with a high-sugar diet. Food Funct. (2019) 10:7063–80. doi: 10.1039/c9fo01334b
57. Dai WD, Qi DD, Yang T, Lv HP, Guo L, Zhang Y, et al. Non-targeted analysis using ultraperformance liquid chromatography-quadrupole time-of-flight mass spectrometry uncovers the effects of harvest season on the metabolites and taste quality of tea (Camellia sinensis L.). J Agric Food Chem. (2015) 63:9869–78. doi: 10.1021/acs.jafc.5b03967
58. Shannon E, Jaiswal AK, Abu-Ghannam N. Polyphenolic content and antioxidant capacity of white, green, black, and herbal teas: a kinetic study. Food Res. (2018) 2:1–11. doi: 10.26656/fr.2017.2(1).117
59. Rice-evans CA, Miller NJ, Bolwell PG, Bramley PM, Pridham JB. The relative antioxidant activities of plant-derived polyphenolic flavonoids. Free Radic Res. (1995) 22:375–83. doi: 10.3109/10715769509145649
60. Anissi J, Hassouni ME, Ouardaoui A, Sendide K. A comparative study of the antioxidant scavenging activity of green tea, black tea and coffee extracts: a kinetic approach. Food Chem. (2014) 150:438–47. doi: 10.1016/j.foodchem.2013.11.009
61. Seyoum A, Asres K, El-Fiky FK. Structure-radical scavenging activity relationships of flavonoids. Phytochem. (2006) 67:2058–70. doi: 10.1016/j.phytochem.2006.07.002
62. Dufresne CJ, Farnworth ER. A review of latest research findings on the health promotion properties of tea. J Nutr Biochem. (2001) 12:404–21. doi: 10.1016/S0955-2863(01)00155-3
63. Zhou BX, Ma BS, Ma CQ, Xu CC, Wang JC, Wang ZY, et al. Classification of Pu-erh ripened teas and their differences in chemical constituents and antioxidant capacity. LWT Food Sci Technol. (2022) 153:112370. doi: 10.1016/j.lwt.2021.112370
64. Zhang L, Ho CT, Zhou J, Santos JS, Armstrong L, Granato D. Chemistry and biological activities of processed Camellia sinensis teas: a comprehensive review. Compr Rev Food Sci Food Saf. (2019) 18:1474–95. doi: 10.1111/1541-4337.12479
65. Obanda M, Owuor PO, Mang’oka R, Kavoi M. Changes in thearubigen fractions and theaflavins levels due to variations in processing conditions and their effects on black tea liquor brightness and total colour. Food Chem. (2004) 85:163–73. doi: 10.1016/S0308-8146(02)00183-8
66. Zhou BX, Ma CQ, Ren XY, Xia T, Zheng CQ, Liu XH. Correlation analysis between filamentous fungi and chemical compositions in a type pu-erh tea after a long-term storage. Food Sci Nutr. (2020) 8:2501–11. doi: 10.1002/fsn3.1543
67. Yao YN, Wu MY, Huang YJ, Li CK, Pan X, Zhu W, et al. Appropriately raising fermentation temperature beneficial to the increase of antioxidant activity and gallic acid content in Eurotium cristatum-fermented loose tea. LWT Food Sci Technol. (2017) 82:248–54. doi: 10.1016/j.lwt.2017.04.032
68. Yu P, Yeo ASL, Low MY, Zhou WB. Identifying key non-volatile compounds in ready-to-drink green tea and their impact on taste profile. Food Chem. (2014) 155:9–16. doi: 10.1016/j.foodchem.2014.01.046
Keywords: Fu brick tea, fungal community, chemical compositions, antioxidant activity, taste characteristics
Citation: Chen Y, Chen J, Chen R, Xiao L, Wu X, Hu L, Li Z, Wang Y, Zhu M, Liu Z and Xiao Y (2022) Comparison of the Fungal Community, Chemical Composition, Antioxidant Activity, and Taste Characteristics of Fu Brick Tea in Different Regions of China. Front. Nutr. 9:900138. doi: 10.3389/fnut.2022.900138
Received: 20 March 2022; Accepted: 26 April 2022;
Published: 17 May 2022.
Edited by:
Biao Yuan, China Pharmaceutical University, ChinaReviewed by:
Xin Zhang, Ningbo University, ChinaCopyright © 2022 Chen, Chen, Chen, Xiao, Wu, Hu, Li, Wang, Zhu, Liu and Xiao. This is an open-access article distributed under the terms of the Creative Commons Attribution License (CC BY). The use, distribution or reproduction in other forums is permitted, provided the original author(s) and the copyright owner(s) are credited and that the original publication in this journal is cited, in accordance with accepted academic practice. No use, distribution or reproduction is permitted which does not comply with these terms.
*Correspondence: Mingzhi Zhu, bXp6aHVjbkBob3RtYWlsLmNvbQ==; Yu Xiao, eGlhb3l1QGh1bmF1LmVkdS5jbg==, eXV4aWFvXzg5QDE2My5jb20=
†These authors have contributed equally to this work
Disclaimer: All claims expressed in this article are solely those of the authors and do not necessarily represent those of their affiliated organizations, or those of the publisher, the editors and the reviewers. Any product that may be evaluated in this article or claim that may be made by its manufacturer is not guaranteed or endorsed by the publisher.
Research integrity at Frontiers
Learn more about the work of our research integrity team to safeguard the quality of each article we publish.