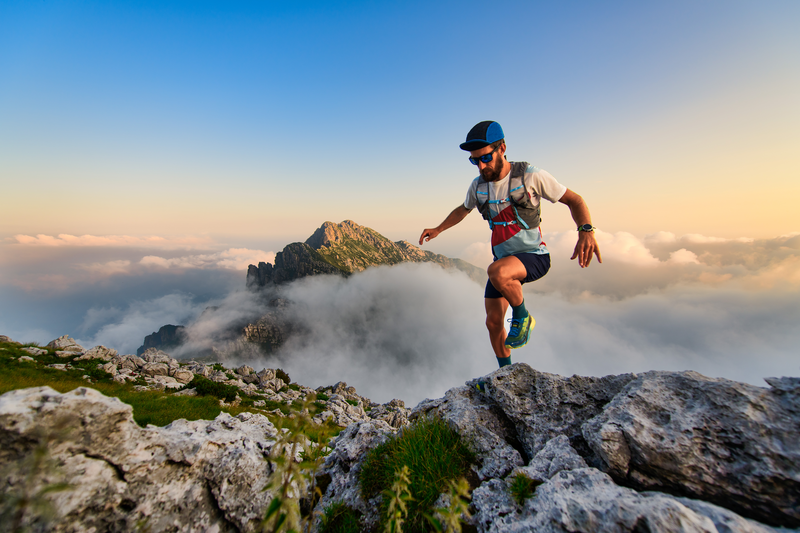
94% of researchers rate our articles as excellent or good
Learn more about the work of our research integrity team to safeguard the quality of each article we publish.
Find out more
ORIGINAL RESEARCH article
Front. Nutr. , 27 April 2022
Sec. Sport and Exercise Nutrition
Volume 9 - 2022 | https://doi.org/10.3389/fnut.2022.896503
This article is part of the Research Topic Nutrition to Support Gut Health and the Microbiome in Athletes View all 5 articles
Increasing numbers of researchers are investigating the benefits of probiotics in enhancing exercise performance and verifying the role of the gut–muscle axis. In our previous study, Lactobacillus plantarum PL-02 improved exercise performance and muscle mass. Therefore, the purpose of this study was to investigate whether supplementation with PL-02 combined with resistance training has a synergistic effect on exercise performance and muscle mass. All the animals were assigned into four groups (n = 8/group): a sedentary control with normal distilled water group (vehicle, n = 8); PL-02 supplementation group (PL-02, 2.05 × 109 CFU, n = 8); resistance training group (RT, n = 8); PL-02 supplementation combined with resistance training group (PL-02 + RT, 2.05 × 109 CFU, n = 8). Supplementation with PL-02 for four consecutive weeks combined with resistance exercise training significantly improved the grip strength and the maximum number of crawls; increased the time of exhaustive exercise; significantly reduced the time required for a single climb; and reduced the lactate, blood ammonia, creatine kinase, and blood urea nitrogen produced after exercise (p < 0.05). In addition, it produced substantial benefits for increasing muscle mass without causing any physical damage. In summary, our findings confirmed that PL-02 or RT supplementation alone is effective in improving muscle mass and exercise performance and in reducing exercise fatigue, but the combination of the two can achieve increased benefits.
Skeletal muscle is one of the most important tissues in the human body, accounting for approximately 40% of body weight, so maintaining skeletal muscle mass and strength is critical. Resistance training is an effective anabolic stimulus that increases muscle mass and strength in men and women of all ages (1). Regular resistance training (RT) can also improve exercise performance and health (2). Appropriate RT prescriptions and strategies can effectively prevent and improve cardiovascular disease (3), diabetes (4), and arthritis (5), and can improve and delay the risk of sarcopenia and frailty-related diseases in the elderly (6). For athletes, improving muscle strength and mass through RT can help to develop increased explosive power and power output, and can improve muscle endurance to maintain exercise performance during competition (7). Therefore, formulating different training modes, times, intensities, and frequencies for different needs is an important method of optimizing resistance training (8). In recent years, sports nutrition supplements, when combined with exercise training intervention, more effectively repairs muscles, increases muscle protein synthesis (MPS) rate, reduces exercise injuries, and improves exercise performance (9, 10). In addition to the common high-protein creatine diet, a growing body of evidence supports the idea of a gut–muscle axis, implying a link between muscle mass and strength performance and gut microbes (11). Probiotics are a safe dietary supplement that improves the richness and abundance of gut microorganisms (12).
Although the mechanisms underlying the relationship between gut microbes and muscle have not been fully defined, researchers have found that exercise training can alter the composition of the gut microbiota, possibly due to energy demand and use (13). Gut microbes may also stimulate insulin-like growth factor-1 (IGF-1) to increase skeletal muscle mitochondrial levels, promote skeletal muscle cell synthesis (14), or maintain or improve muscle mass by increasing glycogen storage using short-chain fatty acids (SCFA) (15). Many factors can cause changes in the gut microbiome, such as diet and exercise. Diet and exercise can positively change the gut microbiota (16). In addition to exercise training, supplementation with probiotic is another method to affect the gut microbiota. Probiotics are defined as live microorganisms that, when administered in sufficient doses, confer a health benefit to the host (17). Currently, lactic acid bacteria (LAB) and bifidobacteria are the main probiotic species. The health benefits of probiotics include the modulation of immune responses, maintenance of the gut barrier, and the antagonism of pathogen adhesion to host tissues (18–20). However, different strains have different levels of efficacy, and probiotics vary in their ability to colonize the gastrointestinal (GI) tract, clinical efficacy, and the type and extent of health benefits they provide to different hosts (21). In recent years, increasing numbers of studies have shown that probiotic supplementation can effectively improve exercise endurance performance, increase glycogen storage, and delay fatigue. Among them, Lactobacillus plantarum, Bifidobacterium longum subsp. longum, and Lactobacillus salivarius subsp. salicinius significantly improve muscle mass and muscle strength. In our previous research, L. plantarum TWK10, Bifidobacterium longum subsp. longum OLP-01, and Lactobacillus salivarius subsp. salicinius SA-03 supplementation was found to be effective not only in increasing muscle mass and grip strength in animals (22, 23), but also in improving body composition and exercise performance in healthy adults (24–26).
In the current study, we used L. plantarum PL-02 in combination with resistance training to evaluate the effect on improving exercise performance and increasing muscle mass in mice. This strain is a human-derived probiotic, which was screened from the gut microbiota of a women’s weightlifting Olympic gold medalist. In our previous study, we confirmed that supplementation with PL-02 without exercise training effectively improved muscle strength, exercise endurance performance, glycogen storage, and muscle mass in mice, and delayed and reduced the fatigue index after exercise (27). Therefore, we aimed to explore the effects of supplementary PL-02 and resistance exercise training on improving muscle mass, strength, exercise performance, and physical fitness.
We screened L. plantarum (PL-02) from the feces of the Olympic women’s 48 kg weightlifting gold medalist Wei-ling Chen and collected fecal specimens using a fecal preservation kit. We analyzed the specimens after 1–4 days at 37°C using glucose-molasses medium (GMM) under three different oxygen conditions (aerobic, facultative anaerobic, and obligate anaerobic). The cultured intestinal flora was extracted using 16S rDNA sequencing, and the Food Industry Research and Development Institute (Hsinchu, Taiwan) confirmed that the isolate was L. plantarum. The probiotics supplementation (PL-02) was provided by Bioflag Biotech Co., Ltd. (Tainan, Taiwan), and the PL-02 was preserved in Food Industry Research and Development Institute (Hsinchu, Taiwan) and China General Microbiological Culture Collection Center (Beijing, China), the deposition number of PL-02 were BCRC-911012 and CGMCC-20485, respectively. The viable cell number of PL-02 cells was 1.07 × 1011 CFU/g. We dissolved the strain powder in phosphate-buffered saline (PBS, pH. 7.2) prior to supplementation. The Institutional Animal Care and Use Committee (IACUC) of the National Taiwan Sport University approved the animal experimentation and procedure (IACUC no. 11008). All mice were housed in the Animal Facility of the Institute of Sports Science, National Taiwan Sports University, and maintained under stable photoperiod, temperature, and humidity conditions (12 h light/12 h dark cycle, 22 ± 2°C, and 60–70%, respectively). A standard laboratory diet (No. 5,001; PMI Nutrition International, Brentwood, MO, United States) and distilled water were provided ad libitum for the mice. Thirty-two male ICR mice (6 weeks old) were purchased from BioLASCO (Yi-Lan, Taiwan). After a 2-week acclimation period, all the mice were randomly divided into four groups (8 mice/group) for PL-02 supplementation and/or resistance training (RT) as follows: (1) sedentary control with vehicle (SC), (2) sedentary control with PL-02 supplementation (PL-02, 2.05 × 109 CFU/kg mouse/day), (3) resistance training with vehicle (RT), and (4) resistance training with PL-02 supplementation (PL-02 + RT, 2.05 × 109 CFU/kg mouse/day). Each group was administered the same volume of distilled water or ISP by oral gavage. We recorded water consumption, food intake, and animal weights twice per week.
The resistance training protocol was performed 5 days/week for 4 weeks, and the indicated intensity load was adjusted by individual animal weight using the protocol. The weight-loading started at 5% of body weight and increased by 25% of body weight weekly until the final weight was 100% body weight, as we previously described. In resistance training, the climbing routines involved 4 repetitions/set and 3 sets/day with 1 min rest between sets (28). The equipment was set under 5 cm of water to provide negative stimulation and increase climbing motivation. The muscular power was evaluated in terms of shortest time to climb, and the maximum number of climbs to exhaustion was used to evaluate anaerobic performance.
Grip strength was measured through a pull rod (2 mm in diameter, 7.5 cm in length) on a low-force testing system (Model-RX-5, Aikoh Engineering, Nagoya, Japan). We gently held the top of the mouse tail to allow it to swing naturally, until the two front limbs of the mouse held the lever. Then, with the mouse’s body parallel to the device, we slightly pulled in the opposite direction, repeating 10 times, and we recorded the maximum value, as previously described (26).
We used a motor-driven treadmill for rodents (model MK-680, Muromachi Kikai, Tokyo, Japan) was used to assess aerobic endurance performance, and a shock grid to increase test motivation through veterinary monitoring. All mice were initially fit to run on a motorized treadmill at a 10 m/min, 5% incline for 5 min per day for 1 week prior to the exhaustive exercise test. In the formal test, we set a fixed 15° inclination angle and an initial speed of 15 m/min for the mouse to run on the treadmill, and we increased the speed by 3 m/min every 2 min. When the mouse fell into the impact zone multiple times and stayed there for more than 5 s, we defined it as exhausted (29).
Referring to our previous study, we performed a fatigue biochemical assessment in this trial to explore the benefits of PL-02 supplementation in combination with resistance training in reducing post-exercise fatigue. We collected blood from the submandibular hemorrhage of the mice immediately after swimming in water in a non-weight-bearing fashion for 15 min at a water temperature of 30°C. The blood samples were centrifuged at 1,000 × g and 4°C for 15 min after complete clotting for serum separation and analyzed using an automatic analyzer (Hitachi 7,060, Hitachi, Tokyo, Japan) to measure glucose, lactate, blood urine nitrogen (BUN), creatine kinase (CK), and ammonia levels.
At the end of the experiment, we euthanized all the mice with 95% CO2 and collected blood samples via the heart. The serum was collected after centrifugation and assessed by using an automatic analyzer (Hitachi 717, Hitachi, Tokyo, Japan) for the levels of alanine aminotransferase (ALT), aspartate aminotransferase (AST), albumin (ALB), total cholesterol (TC), triacylglycerol (TG), BUN, creatinine (CREA), uric acid (UA), total protein (TP), CK, lactate dehydrogenase (LDH), and glucose.
After the mice were euthanized, the liver, kidney, heart, lung, muscle (gastrocnemius), quadriceps, epididymal fat pad (EFP), and brown adipocyte tissue (BAT) were accurately excised and weighed. Of these, 100 μg of liver and muscle tissue was homogenized in 500 μL of cold perchloric acid, then centrifuged at 15,000 × g for 15 min at 4°C, the supernatant was collected, and the glycogen concentration was determined. We determined the glycogen content (mg/g) in the liver and muscle using a commercial assay kit (Sigma-Aldrich, St. Louis, MO, United States) according to the manufacturer’s instructions.
The liver, kidney, muscle, quadriceps, heart, lung, EFP, and BAT were fixed in 10% formalin, embedded in paraffin, and cut into 4 μm-thick sections for morphological and pathological evaluation. The tissue sections were stained with hematoxylin and eosin (H&E) and examined by a clinical pathologist for light microscopy using a CCD camera (BX-51, Olympus, Tokyo, Japan).
The statistical analyses were performed using SAS v9.0 (SAS, Cary, NC, United States). Two-way ANOVA was used to assess the effect of the RT and PL-02 supplementation on all the experimental data. The data are expressed as mean ± SD, and p < 0.05 was considered statistically significant.
As shown in Table 1, we found no significant differences in diet, water intake, or body weight in the 4 weeks of PL-02 supplementation combined with RT. In terms of body composition, both in absolute and relative weight, liver, kidney, heart, lung, EFP, and BAT showed no significant differences between the groups. However, the absolute and relative muscle weights in the PL-02 + RT group was significantly higher than in the SC group [1.16-fold (p = 0.0017) and 1.13-fold (p = 0.0030), respectively]. The main effect of PL-02 supplementation was a significant change in the absolute (p = 0.0046) and relative (p = 0.0117) muscle weights. We obtained a similar result for the quadriceps: the PL-02 + RT group showed a significant increase compared to the SC group [1.23-fold (p = 0.0337) and 1.10-fold (p = 0.0137), respectively]. The main effect of PL-02 supplementation was a significant change in the absolute (p = 0.0470) and relative (p = 0.0284) quadriceps weights.
As shown in Figure 1A, the SC, PL-02, RT, and PL-02 + RT groups were 155 ± 24, 164 ± 13, 172 ± 17 and 191 ± 1 g, respectively. The PL-02 + RT group was significantly heavier than the SC, PL-02 and RT groups [1.23-fold (p = 0.0002), 1.17-fold (p = 0.0033), and 1.11-fold (p = 0.0353), respectively]. The main effect of RT (p = 0.0010) and PL-02 (p = 0.0305) was a significant increase in grip strength, but we found no significant interaction effect. We calculated the relative grip strength (%) normalized to body weight, and the RT and PL-02 + RT groups scored significantly higher than the SC group [1.18-fold (p = 0.0086) and 1.23-fold (p = 0.0010), respectively], with only significant RT main effects (p = 0.0005) (Figure 1B).
Figure 1. Effect of PL-02 supplementation combined with RT on (A) absolute forelimb grip strength and (B) forelimb grip strength (%) relative to body weight. Data are expressed as mean ± SD and different superscript letters (a,b,c) indicate significant difference at p < 0.05.
Speed and muscular endurance were used as measures of anaerobic exercise performance, as shown in Figure 2A. The speed performance in the SC, PL-02, RTm and PL-02 + RT groups were 14.46 ± 4.80, 10.61 ± 0.86, 10.05 ± 1.69 and 7.69 ± 1.91 s, respectively. The PL-02, RT and PL-02 + RT groups scored significantly lower than the SC group by 26.62% (p = 0.0092), 30.47% (p = 0.0034), and 46.80% (p < 0.0001), respectively. The main effect of RT (p = 0.0008) and PL-02 (p = 0.0035) was a significant increase in climbing speed, but we found no significant interaction effect. The repetition maximum (RM) of the SC, ISP, RT, and ISP + RT groups was 11.6 ± 1.3, 13.6 ± 1.1, 48.9 ± 4.4, and 57.0 ± 5.7 times, respectively. The RMs of the RT and ISP + RT groups were significantly higher than those of the SC group [4.20-fold (P = 0.0027) and 4.90-fold (P < 0.0001), respectively]. The main effect of RT (p < 0.0001) and PL-02 (p = 0.0006) was a significantly increased muscular endurance performance, and they had a significant interaction effect (p = 0.0257) (Figure 2B).
Figure 2. Effect of PL-02 supplementation combined with RT on (A) time for each climbing and (B) exhaustion times for climbing. Data are expressed as mean ± SD and different superscript letters (a,b,c) indicate significant difference at p < 0.05.
As shown in Figure 3, the treadmill running times to exhaustion in the SC, PL-02, RT, and PL-02 + RT groups were 11.27 ± 2.87, 16.69 ± 3.66, 18.44 ± 1.87, and 19.21 ± 1.47 (min), respectively. Compared to the SC group, the PL-02, RT and PL-02 + RT groups scored significantly higher [1.48-fold (p = 0.0008), 1.64-fold (p = 0.0002), and 1.70-fold (p < 0.0001), respectively]. The main effect of RT (p < 0.0001) and PL-02 (p = 0.0023) was a significant increase in muscular endurance performance, and we found a significant interaction effect (p = 0.0179).
Figure 3. Effect of PL-02 supplementation combined with RT on endurance exercise performance. Data are expressed as mean SD and different superscript letters (a,b) indicate significant difference at p < 0.05.
After 4 weeks’ PL-02 supplementation combined with RT, we conducted 15 min of acute exercise with each mouse to explore the effects on the biochemical markers of fatigue. The lactate levels (Figure 4A) of the SC, PL-02, RT, and PL-02 + RT groups were 6.53 ± 0.68, 5.00 ± 0.25, 4.65 ± 0.50, and 4.55 ± 0.26 (mmol/L), respectively. Compared to the SC group, the lactate levels of the PL-02, RT and PL-02 + RT groups showed significant decreases of 23.39% (p < 0.0001), 28.86% (p < 0.0001), and 30.37% (p < 0.0001), respectively. As shown in Figure 4B, the NH3 levels in the SC, PL-02, RT, and PL-02 + RT groups were 169 ± 8, 147 ± 11, 157 ± 8, and 142 ± 11 (μmol/L), respectively, with the levels in the RT and PL-02 + RT groups showing significant decreases of 15.85% (p = 0.0025) and 31.63% (p < 0.0001) compared to the SC group, respectively. The BUN levels in the SC, PL-02, RT, and PL-02 + RT groups were 31.8 ± 1.6, 29.7 ± 1.1, 29.3 ± 1.5, and 28.8 ± 1.2 (mg/dL), respectively. Compared to the SC group, the BUN levels in the PL-02, RT and PL-02 + RT groups showed significantly decreases of 6.60% (p = 0.0044), 7.97% (p = 0.0008), and 9.70% (p < 0.0001), respectively (Figure 4C). As for CK activity, the SC, PL-02, RT and PL-02 + RT groups scored 794 ± 66, 740 ± 98, 668 ± 76, and 543 ± 57 (U/L), respectively. The RT and PL-02 + RT groups showed significant decreases in the CK activity of 15.85% (p = 0.0025) and 31.63% (p < 0.0001), respectively (Figure 4D). The main effect of RT and PL-02 was a significant decrease in lactate, NH3, BUN, and CK levels (p < 0.05), but only the decrease in the lactate level had a significant interaction effect (p < 0.0001).
Figure 4. Effect of PL-02 supplementation combined with RT on (A) lactate, (B) NH3, (C) BUN and (D) CK after 15 min swimming. Data are expressed as mean ± SD and different superscript letters (a,b,c) indicate significant difference at p < 0.05.
Glycogen is mainly stored in the liver and skeletal muscle. The liver glycogen levels in the SC, PL-02, RT, and PL-02 + RT groups were 15.30 ± 1.71, 20.39 ± 1.82, 18.78 ± 1.82, and 23.02 ± 1.83 (mg/g), respectively. The levels in the PL-02, RT, and PL-02 + RT groups showed significant increases compared to the SC group [1.33-fold (p < 0.0001), 1.23-fold (p = 0.0006), and 1.50-fold (p < 0.0001), respectively; Figure 5A]. As shown in Figure 5B, the muscle glycogen levels in the SC, PL-02, RT, and PL-02 + RT groups were 0.95 ± 0.07, 1.33 ± 0.10, 1.34 ± 0.09, and 1.67 ± 0.12 (mg/g), respectively. The muscle glycogen levels in the PL-02, RT, and PL-02 + RT groups were significantly increased compared to that of the SC group [1.41-fold (p < 0.0001), 1.41-fold (p < 0.0001), and 1.76-fold (p < 0.0001), respectively]. The main effect of RT and PL-02 was a significant increase in liver and muscle glycogen (p < 0.0001), but neither of them showed a significant interaction effect.
Figure 5. Effect of PL-02 supplementation combined with RT on (A) liver glycogen and (B) muscle glycogen. Data are expressed as mean SD and different superscript letters (a,b,c) indicate significant difference at p < 0.05.
At the end of the experiment, a clinical biochemical analysis was performed on the serum, as shown in Table 2. We found no significant differences in ALB, TC, TG, CREA, UA, TP, CK, LDH, or glucose levels. However, the AST and ALT activity levels in the RT group were significantly lower than in the sedentary groups, being the main effect of exercise training (p < 0.0001).
Table 2. The effect of PL-02 supplementation combined with RT on biochemical assessments of serum at the end of the experiment.
Histological examinations of the liver, quadriceps, muscle, heart, kidney, lung, EFP, and BAT were performed at the end of the study, and no abnormalities were observed in any group (Figure 6). Intrahepatic sinusoidal and hepatic cord arrangement did not change. In addition, no Zenker degeneration or hyperplasia was observed in cardiomyocytes, and we found no differences in tubular or glomerular structure between treatment groups.
Figure 6. Effect of PL-02 supplementation combined with RT on (A) liver, (B) quadriceps, (C) muscles, (D) heart, (E) kidney, (F) lung, (G) EFP, and (H) BAT tissue in mice. (H&E stain, magnification: 200×; bar, 40 μm; BAT magnification: 100×; bar, 80 μm). EFP, epididymal fat pad; BAT, brown adipose tissue.
In recent years, a growing body of researchers has explored the role of probiotics in improving athletic performance. However, literature on the use of probiotics as a sports nutrition supplement in combination with exercise training is scarce. In the current study, we found that L. plantarum PL-02 supplementation was effective in improving muscle mass, muscle strength, and exercise endurance performance, and in increasing glycogen content, consistent with previous findings (27). In addition, the effect when combined with RT intervention was more significant.
RT is the primary form of exercise for building muscle health regardless of diet and nutrition. Regular resistance exercise can enhance the phosphorylation of p70S6 kinase and MPS, promoting significant increases in muscle strength and hypertrophy (30). This works by causing microscopic damage or tearing to muscle cells, which in turn are quickly repaired by the body to help the muscle regenerate and become stronger (31). Furthermore, higher proportions of SCFA bacteria involved in carbohydrate and amino acid metabolism in the gut of athletes lead to higher concentrations of acetate, butyrate, and propionate in feces (32). Among them, acetate enhances glucose uptake and fatty acid metabolism by activating activated protein kinase (AMPK) and increasing the expression of glucose transporter type 4 (GLUT4) and myoglobin. Myocyte enhancer factor 2A (MEF2A), a metabolic pathway thought to be important for increasing muscle mass, is involved in the expression of myoglobin and GLUT4 genes (33). Butyrate may increase ATP and the metabolic efficiency of muscle fibers by activating pathways such as UCP2-AMPK-ACC and PGC1-α (34). In addition, it prevents apoptosis and prevents muscle protein catabolism by inhibiting histone deacetylases (35). In addition to increasing the production of SCFA in the gut, probiotic supplementation is effective in increasing the alpha diversity in the gut and may affect muscle mass, function, and energy metabolism by altering the microbiota. In our previous study, mice administered TWK10 or PL-02 for 6 or 4 weeks, respectively, showed increased muscle mass without exercise training (22, 27). In this study, after 4 weeks of PL-02 supplementation with resistance exercise training, muscle mass significantly increased (Table 1). Increased muscle mass positively correlates with improved muscle strength, which increases with muscle mass (36). We found similar trend results in this study. Supplementation with PL-02 in combination with RT significantly improved muscle strength and relative muscle strength (Figures 1A,B). Increases in muscle strength generally increase explosive power, and explosive strength training can help improve muscular endurance and endurance performance (37). In this study, we measured the shortest crawl time (explosive power) and the maximum number of crawls (muscular endurance). The results showed that PL-02 supplementation in combination with RT had a significant effect not only on improved muscle mass and strength, but also on explosive power and muscular endurance performance (Figures 2A,B).
Muscle mass and quantity, as well as mitochondrial mass, affect glycogen content (38). Glycogen is a glucose polymer that produces adenosine triphosphate (ATP) through glycogen catabolism and provides the main source of fuel for muscles during exercise (39). If glycogen stores are reduced, bioenergy metabolism is impaired, resulting in insufficient energy supply and decreased muscle strength and function (40). Previous study had shown that, when the gut microbiota is dysregulated, skeletal muscle glucose availability may be reduced, resulting in reduced glycogen storage (13). Germ-free mice showed significantly lower glycogen stores compared to mice with a normal gut microbiota (41). The strains in the gut may produce SCFA, in which butyrate can maintain blood glucose homeostasis and promote glycogen metabolism through the GPR43–AKT–GSK3 signaling pathway (42), whereas propionate can promote gluconeogenesis in hepatocytes (43). Supplementation with probiotics can increase the production of SCFAs in the gut. Lactobacillus acidophilus modulates glycogen-synthesis-related genes (GSK-3β and Akt) and glycogen content in tissues (44). In our previous study, we also found that Bifidobacterium longum OLP-01 supplementation for four consecutive weeks significantly increased glycogen levels in the muscle and liver of mice and improved exercise endurance performance (23). In this study, either RT or PL-02 supplementation significantly increased liver or muscle glycogen content, but the effect of RT combined with PL-02 intervention was more significant (Figures 5A,B). Optimizing and increasing glycogen storage can effectively improve exercise endurance performance, thereby delaying post-exercise fatigue and accelerating recovery (45). In addition, the modulation of SCFA production by the gut microbiota affects energy metabolism during exercise, thereby contributing to exercise-induced adaptation and serving as an energy source for liver and muscle cells, enhancing endurance performance using the long-term maintenance of blood glucose (46). Our previous findings showed that supplementation with PL-02 can significantly increase the A. muciniphila in the gut (27), which is thought to have a higher proportion in the gut microbiota of athletes. Maintaining gut barrier function and glucose homeostasis can help sustain or enhance endurance performance (47). In the current study, RT or PL-02 supplementation had a significant effect on improving exercise endurance, and we observed an interaction effect (Figure 3).
During prolonged or strenuous exercise, the body is unable to provide or maintain the required energy load, resulting in decreased exercise performance and an increased production of fatigue byproducts (47). Among them, exercise-related indicators such as lactate, NH3, BUN, and CK are widely used to evaluate the physiological state of exercise. Lactate, a product of carbohydrate glycolysis under anaerobic conditions, is positively correlated with exercise duration and intensity. During vigorous exercise, glucose is broken down into pyruvate. Under anaerobic conditions, a part of pyruvate is reduced to lactic acid by lactate dehydrogenase (LDH) and hydrogen ions are released, resulting in a decrease in the pH of blood and muscle tissue and the inhibition of glycolysis, which in turn interferes with normal cell function (48, 49). In order to maintain the ATP/ADP ratio, two molecules of ADP can be converted into one molecule of ATP and one molecule of AMP when the ATP supply is insufficient to cope with exercise consumption. Among them, AMP is degraded into IMP and ammonia by AMP deaminase. Ammonia is converted to BUN through the urea cycle, thereby increasing in blood (50). Long-term exercise training can significantly increase the intestinal level of Veillonella. Veillonella species metabolize lactate to SCFA acetate and propionate via the methylmalonyl–CoA pathway (51). In addition, the intestinal colonization of Veillonella may enhance the Cori cycle by providing an alternative lactate disposal method that converts systemic lactate into SCFAs that re-enter the circulation (52). Probiotic supplementation increases SCFAs in the gut and converts lactic acid to propionate, which provides energy for muscles during exercise (53). The mechanism of these synergistic effects may involve the increased availability of glycogen or glucose fuel (54). Therefore, these results were validated in our research. In our study, RT and PL-02 supplementation had a significant effect on reduced post-exercise lactate, NH3, BUN, and CK (Figures 4A–D).
Resistance training has been shown to improve gut microbiota diversity and composition and to help increase the richness of SCFA-producing gut microbiota and reduce the relative abundance of pro-inflammatory-inducing species, including Pseudomonas, Serratia, Comamonas, etc. (55, 56). A past study showed that elite rugby players had higher gut microbial diversity compared to sedentary people, and with a significantly higher percentage of Akkermansia (32). A. muciniphila, a gut microbe known for mucin degradation and SCFA production, is thought to be positively correlated with exercise performance in its abundance (57), in addition to maintaining gut barrier function and glucose homeostasis (46). In our previous study also confirmed that supplementation of PL-02 alone without any exercise training also significantly increased the proportion of Akkermansia in the mouse gut and improved muscle mass and exercise performance (27). In the current study, designed to investigate the benefits of PL-02 in combination with resistance training to improve athletic performance and muscle mass, we confirmed this result. However, whether it has a synergistic effect on gut microbial composition and changes remains to be further confirmed in the future.
We found that supplementation with PL-02 combined with RT for four consecutive weeks effectively improved muscle strength, muscle mass, endurance, and glycogen storage in mice, and significantly reduced the biochemical values of fatigue after exercise. In addition, according to the serum biochemical analysis and histopathological results, no abnormality or injury was observed even after repeated exercise challenges. However, no synergistic effect was observed between RT and PL-02 supplementation for muscle strength, muscle mass, biochemical markers of fatigue, and glycogen stores. We think that the intervention period needs to be further expanded or the training intensity or dose of probiotics needs to be increased. Therefore, we must further explore the optimal cycle and strategy of probiotics combined with exercise training intervention. In addition, in animal experiments, the administration of probiotics effectively increased muscle mass, improved exercise performance, and reduced fatigue after exercise without exercise intervention; its applicability and efficacy were also successfully demonstrated in humans (18, 19). Therefore, we infer that similar result can be achieved in human trials in the future. However, further research is needed to clarify the mechanism of human-related effects.
In conclusion, we found that L. plantarum PL-02 supplementation combined with resistance exercise training over 4 weeks significantly improved forelimb grip, endurance exercise performance, and glycogen storage, and increased muscle mass. In addition, PL-02 + RT significantly reduced the levels of fatigue indicators such as lactate, BUN, ammonia, and CK. Furthermore, PL-02 + RT did not cause harm to physiological performance or histopathology. Our research confirmed that PL-02 or RT supplementation alone are effective in reducing exercise fatigue and in improving muscle mass and exercise performance, but the combination of the two can achieve increased benefits.
The raw data supporting the conclusions of this article will be made available by the authors, without undue reservation.
The animal study was reviewed and approved by the Institutional Animal Care and Use Committee (IACUC) of the National Taiwan Sport University approved the animal experimentation and procedure (IACUC no. 11008).
W-LY, Y-JH, and C-CH designed the study. Y-JH, C-SH, and M-CL carried out the experiments. W-LY, C-SH, C-CH, and M-CL analyzed the data. H-HH, W-YL, Y-WK, S-YT, and C-CH provided reagents and other lab supplies. W-LY, Y-JH, C-CH, and M-CL prepared the figures and wrote the manuscript. W-LY, C-CH, and M-CL revised the manuscript. All authors contributed to the article and approved the submitted version.
This research was supported by the Lo-Hsu Medical Foundation, Inc., Lotung Poh-Ai Hospital (Number: E169) and partially supported by institutional fund from NTSU.
H-HH, Y-WK, and S-YT were employed by Bioflag Biotech Co., Ltd.
The remaining authors declare that the research was conducted in the absence of any commercial or financial relationships that could be construed as a potential conflict of interest.
All claims expressed in this article are solely those of the authors and do not necessarily represent those of their affiliated organizations, or those of the publisher, the editors and the reviewers. Any product that may be evaluated in this article, or claim that may be made by its manufacturer, is not guaranteed or endorsed by the publisher.
We thank C-CC for technical assistance in the histological examinations. The probiotic supplementation was generated by Bioflag Biotech Co., Ltd. (Tainan, Taiwan).
1. Viecelli C, Aguayo D. May the force and mass be with you-evidence-based contribution of mechano-biological descriptors of resistance exercise. Front Physiol. (2022) 12:686119. doi: 10.3389/fphys.2021.686119
2. El-Kotob R, Ponzano M, Chaput JP, Janssen I, Kho ME, Poitras VJ, et al. Resistance training and health in adults: an overview of systematic reviews. Appl Physiol Nutr Metab. (2020) 45:S165–79. doi: 10.1139/apnm-2020-0245
3. Baffour-Awuah B, Pearson MJ, Smart NA, Dieberg G. Safety, efficacy and delivery of isometric resistance training as an adjunct therapy for blood pressure control: a modified Delphi study. Hypertens Res. (2022) 12:1–13. doi: 10.1038/s41440-021-00839-3
4. Bronczek GA, Soares GM, de Barros JF, Vettorazzi JF, Kurauti MA, Marconato-Júnior E, et al. Resistance exercise training improves glucose homeostasis by enhancing insulin secretion in C57BL/6 mice. Sci Rep. (2021) 11:8574. doi: 10.1038/s41598-021-88105-x
5. Wang HN, Chen Y, Cheng L, Wang ST, Hu DX, Wang LN, et al. Effect of low-load resistance training with different degrees of blood flow restriction in patients with knee osteoarthritis: study protocol for a randomized trial. Trials. (2022) 23:6. doi: 10.1186/s13063-021-05946-7
6. Rodrigues F, Domingos C, Monteiro D, Morouço P. A review on aging, sarcopenia, Falls, and resistance training in community-dwelling older adults. Int J Environ Res Public Health. (2022) 19:874. doi: 10.3390/ijerph19020874
7. Behm DG, Young JD, Whitten JHD, Reid JC, Quigley PJ, Low J, et al. Effectiveness of traditional strength vs. power training on muscle strength, power and speed with youth: a systematic review and meta-analysis. Front Physiol. (2017) 8:423. doi: 10.3389/fphys.2017.00423
8. American College of Sports Medicine. American college of sports medicine position stand. progression models in resistance training for healthy adults. Med Sci Sports Exerc. (2009) 41:687–708. doi: 10.1249/MSS.0b013e3181915670
9. Dias KA, da Conceição AR, Oliveira LA, Pereira SMS, Paes SDS, Monte LF, et al. Effects of curcumin supplementation on inflammatory markers, muscle damage, and sports performance during acute physical exercise in sedentary individuals. Oxid Med Cell Longev. (2021) 2021:9264639. doi: 10.1155/2021/9264639
10. Oikawa SY, Brisbois TD, van Loon LJC, Rollo I. Eat like an athlete: insights of sports nutrition science to support active aging in healthy older adults. Geroscience. (2021) 43:2485–95. doi: 10.1007/s11357-021-00419-w
11. Grosicki GJ, Fielding RA, Lustgarten MS. Gut microbiota contribute to age-related changes in skeletal muscle size, composition, and function: biological basis for a gut-muscle axis. Calcif Tissue Int. (2018) 102:433–42. doi: 10.1007/s00223-017-0345-5
12. Didari T, Solki S, Mozaffari S, Nikfar S, Abdollahi M. A systematic review of the safety of probiotics. Expert Opin Drug Saf. (2014) 13:227–39. doi: 10.1517/14740338.2014.872627
13. Przewłócka K, Folwarski M, Kaźmierczak-Siedlecka K, Skonieczna-Żydecka K, Kaczor JJ. Gut-muscle axisexists and may affect skeletal muscle adaptation to training. Nutrients. (2020) 12:1451. doi: 10.3390/nu12051451
14. Prokopidis K, Chambers E, Ni Lochlainn M, Witard OC. Mechanisms linking the gut-muscle axis with muscle protein metabolism and anabolic resistance: implications for older adults at risk of sarcopenia. Front Physiol. (2021) 12:770455. doi: 10.3389/fphys.2021.770455
15. Lahiri S, Kim H, Garcia-Perez I, Reza MM, Martin KA, Kundu P, et al. The gut microbiota influences skeletal muscle mass and function in mice. Sci Transl Med. (2019) 11:eaan5662. doi: 10.1126/scitranslmed.aan5662
16. Monda V, Villano I, Messina A, Valenzano A, Esposito T, Moscatelli F, et al. Exercise modifies the gut microbiota with positive health effects. Oxid Med Cell Longev. (2017) 2017:3831972. doi: 10.1155/2017/3831972
17. Hill C, Guarner F, Reid G, Gibson GR, Merenstein DJ, Pot B, et al. Expert consensus document. The international scientific association for probiotics and prebiotics consensus statement on the scope and appropriate use of the term probiotic. Nat Rev Gastroenterol Hepatol. (2014) 11:506–14. doi: 10.1038/nrgastro.2014.66
18. Duranti S, Ferrario C, van Sinderen D, Ventura M, Turroni F. Obesity and microbiota: an example of an intricate relationship. Genes Nutr. (2017) 12:18. doi: 10.1186/s12263-017-0566-2
19. Lin CW, Chen YT, Ho HH, Kuo YW, Lin WY, Chen JF, et al. Impact of the food grade heat-killed probiotic and postbiotic oral lozenges in oral hygiene. Aging (Albany NY). (2022) 14:2221–38. doi: 10.18632/aging.203923
20. Liao CA, Huang CH, Ho HH, Chen JF, Kuo YW, Lin JH, et al. A combined supplement of probiotic strains AP-32, bv-77, and CP-9 increased Akkermansia mucinphila and reduced non-esterified fatty acids and energy metabolism in HFD-induced obese rats. Nutrients. (2022) 14:527. doi: 10.3390/nu14030527
21. Pyne DB, West NP, Cox AJ, Cripps AW. Probiotics supplementation for athletes – clinical and physiological effects. Eur J Sport Sci. (2015) 15:63–72. doi: 10.1080/17461391.2014.971879
22. Chen YM, Wei L, Chiu YS, Hsu YJ, Tsai TY, Wang MF, et al. Lactobacillus plantarum TWK10 supplementation improves exercise performance and increases muscle mass in mice. Nutrients. (2016) 8:205. doi: 10.3390/nu8040205
23. Lee MC, Hsu YJ, Chuang HL, Hsieh PS, Ho HH, Chen WL, et al. In vivo ergogenic properties of the Bifidobacterium longum OLP-01 isolated from a weightlifting gold medalist. Nutrients. (2019) 11:2003. doi: 10.3390/nu11092003
24. Huang WC, Lee MC, Lee CC, Ng KS, Hsu YJ, Tsai TY, et al. Effect of Lactobacillus plantarum TWK10 on exercise physiological adaptation, performance, and body composition in healthy humans. Nutrients. (2019) 11:2836. doi: 10.3390/nu11112836
25. Lin CL, Hsu YJ, Ho HH, Chang YC, Kuo YW, Yeh YT, et al. Bifidobacterium longum subsp. Longum OLP-01 supplementation during endurance running training improves exercise performance in middle– and long-distance runners: a double-blind controlled trial. Nutrients. (2020) 12:1972. doi: 10.3390/nu12071972
26. Lee MC, Hsu YJ, Ho HH, Hsieh SH, Kuo YW, Sung HC, et al. Lactobacillus salivarius subspecies salicinius SA-03 is a new probiotic capable of enhancing exercise performance and decreasing fatigue. Microorganisms. (2020) 8:545. doi: 10.3390/microorganisms8040545
27. Lee MC, Hsu YJ, Ho HH, Kuo YW, Lin WY, Tsai SY, et al. Effectiveness of human-origin Lactobacillus plantarum PL-02 in improving muscle mass, exercise performance and anti-fatigue. Sci Rep. (2021) 11:19469. doi: 10.1038/s41598-021-98958-x
28. Lee MC, Hsu YJ, Yang LH, Huang CC, Ho CS. Ergogenic effects of green tea combined with isolated soy protein on increasing muscle mass and exercise performance in resistance-trained mice. Nutrients. (2021) 13:4547. doi: 10.3390/nu13124547
29. Kan NW, Lee MC, Tung YT, Chiu CC, Huang CC, Huang WC. The synergistic effects of resveratrol combined with resistant training on exercise performance and physiological adaption. Nutrients. (2018) 10:1360. doi: 10.3390/nu10101360
30. Baar K, Esser K. Phosphorylation of p70(S6k) correlates with increased skeletal muscle mass following resistance exercise. Am J Physiol. (1999) 276:C120–7. doi: 10.1152/ajpcell.1999.276.1.C120
31. Ambrosio F, Kadi F, Lexell J, Fitzgerald GK, Boninger ML, Huard J. The effect of muscle loading on skeletal muscle regenerative potential: an update of current research findings relating to aging and neuromuscular pathology. Am J Phys Med Rehabil. (2009) 88:145–55. doi: 10.1097/PHM.0b013e3181951fc5
32. Clarke SF, Murphy EF, O’Sullivan O, Lucey AJ, Humphreys M, Hogan A, et al. Exercise and associated dietary extremes impact on gut microbial diversity. Gut. (2014) 63:1913–20. doi: 10.1136/gutjnl-2013-306541
33. Maruta H, Yoshimura Y, Araki A, Kimoto M, Takahashi Y, Yamashita H. Activation of AMP-activated protein kinase and stimulation of energy metabolism by acetic acid in l6 myotube cells. PLoS One. (2016) 11:e0158055. doi: 10.1371/journal.pone.0158055
34. den Besten G, Gerding A, van Dijk TH, Ciapaite J, Bleeker A, van Eunen K, et al. Protection against the metabolic syndrome by guar gum-derived short-chain fatty acids depends on peroxisome proliferator-activated receptor γ and glucagon-like peptide-1. PLoS One. (2015) 10:e0136364. doi: 10.1371/journal.pone.0136364
35. Walsh ME, Bhattacharya A, Sataranatarajan K, Qaisar R, Sloane L, Rahman MM, et al. The histone deacetylase inhibitor butyrate improves metabolism and reduces muscle atrophy during aging. Aging Cell. (2015) 14:957–70. doi: 10.1111/acel.12387
36. Chen L, Nelson DR, Zhao Y, Cui Z, Johnston JA. Relationship between muscle mass and muscle strength, and the impact of comorbidities: a population-based, cross-sectional study of older adults in the United States. BMC Geriatr. (2013) 13:74. doi: 10.1186/1471-2318-13-74
37. Paavolainen L, Häkkinen K, Hämäläinen I, Nummela A, Rusko H. Explosive-strength training improves 5-km running time by improving running economy and muscle power. J Appl Physiol (1985). (1999) 86:1527–33. doi: 10.1152/jappl.1999.86.5.1527
38. Jensen J, Rustad PI, Kolnes AJ, Lai YC. The role of skeletal muscle glycogen breakdown for regulation of insulin sensitivity by exercise. Front Physiol. (2011) 2:112. doi: 10.3389/fphys.2011.00112
39. Murray B, Rosenbloom C. Fundamentals of glycogen metabolism for coaches and athletes. Nutr Rev. (2018) 76:243–59. doi: 10.1093/nutrit/nuy001
40. Philp A, Hargreaves M, Baar K. More than a store: regulatory roles for glycogen in skeletal muscle adaptation to exercise. Am J Physiol Endocrinol Metab. (2012) 302:E1343–51. doi: 10.1152/ajpendo.00004.2012
41. Nay K, Jollet M, Goustard B, Baati N, Vernus B, Pontones M, et al. Gut bacteria are critical for optimal muscle function: a potential link with glucose homeostasis. Am J Physiol Endocrinol Metab. (2019) 317:E158–71. doi: 10.1152/ajpendo.00521.2018
42. Zhang WQ, Zhao TT, Gui DK, Gao CL, Gu JL, Gan WJ, et al. Sodium butyrate improves liver glycogen metabolism in type 2 diabetes mellitus. J Agric Food Chem. (2019) 67:7694–705. doi: 10.1021/acs.jafc.9b02083
43. Samuel BS, Shaito A, Motoike T, Rey FE, Backhed F, Manchester JK, et al. Effects of the gut microbiota on host adiposity are modulated by the short-chain fatty-acid binding G protein-coupled receptor, Gpr41. Proc Natl Acad Sci USA. (2008) 105:16767–72. doi: 10.1073/pnas.0808567105
44. Yan F, Li N, Shi J, Li H, Yue Y, Jiao W, et al. Lactobacillus acidophilus alleviates type 2 diabetes by regulating hepatic glucose, lipid metabolism and gut microbiota in mice. Food Funct. (2019) 10:5804–15. doi: 10.1039/c9fo01062a
45. Lawler TP, Cialdella-Kam L. Non-carbohydrate dietary factors and their influence on post-exercise glycogen storage: a review. Curr Nutr Rep. (2020) 9:394–404. doi: 10.1007/s13668-020-00335-z
46. Barton W, Penney NC, Cronin O, Garcia-Perez I, Molloy MG, Holmes E, et al. The microbiome of professional athletes differs from that of more sedentary subjects in composition and particularly at the functional metabolic level. Gut. (2018) 67:625–33. doi: 10.1136/gutjnl-2016-313627
47. Halson SL, Bridge MW, Meeusen R, Busschaert B, Gleeson M, Jones DA, et al. Time course of performance changes and fatigue markers during intensified training in trained cyclists. J Appl Physiol. (2002) 93:947–56. doi: 10.1152/japplphysiol.01164.2001
48. Cairns SP. Lactic acid and exercise performance: culprit or friend Sports Med. (2006) 36:279–91. doi: 10.2165/00007256-200636040-00001
49. Proia P, Di Liegro CM, Schiera G, Fricano A, Di Liegro I. Lactate as a metabolite and a regulator in the central nervous system. Int J Mol Sci. (2016) 17:1450. doi: 10.3390/ijms17091450
50. Wan JJ, Qin Z, Wang PY, Sun Y, Liu X. Muscle fatigue: general understanding and treatment. Exp Mol Med. (2017) 49:e384. doi: 10.1038/emm.2017.194
51. Ng SK, Hamilton IR. Carbon dioxide fixation by Veillonella parvula M 4 and its relation to propionic acid formation. Can J Microbiol. (1973) 19:715–23. doi: 10.1139/m73-116
52. Mohr AE, Jäger R, Carpenter KC, Kerksick CM, Purpura M, Townsend JR, et al. The athletic gut microbiota. J Int Soc Sports Nutr. (2020) 17:24. doi: 10.1186/s12970-020-00353-w
53. Scheiman J, Luber JM, Chavkin TA, MacDonald T, Tung A, Pham LD, et al. Meta-omics analysis of elite athletes identifies a performance-enhancing microbe that functions via lactate metabolism. Nat Med. (2019) 25:1104–9. doi: 10.1038/s41591-019-0485-4
54. Hawley JA. Microbiota and muscle highway – two way traffic. Nat Rev Endocrinol. (2020) 16:71–2. doi: 10.1038/s41574-019-0291-6
55. Chen H, Shen L, Liu Y, Ma X, Long L, Ma X, et al. Strength exercise confers protection in central nervous system autoimmunity by altering the gut microbiota. Front Immunol. (2021) 12:628629. doi: 10.3389/fimmu.2021.628629
56. Bycura D, Santos AC, Shiffer A, Kyman S, Winfree K, Sutliffe J, et al. Impact of different exercise modalities on the human gut microbiome. Sports (Basel). (2021) 9:14. doi: 10.3390/sports9020014
57. de la Cuesta-Zuluaga J, Mueller NT, Corrales-Agudelo V, Velásquez-Mejía EP, Carmona JA, Abad JM, et al. Metformin is associated with higher relative abundance of mucin-degrading Akkermansia muciniphila and Several short-chain fatty acid-producing microbiota in the gut. Diabetes Care. (2017) 40:54–62. doi: 10.2337/dc16-1324
Keywords: Lactobacillus plantarum PL-02, probiotic, muscle mass, exercise, training
Citation: Yeh W-L, Hsu Y-L, Ho C-S, Ho H-H, Kuo Y-W, Tsai S-Y, Huang C-C and Lee M-C (2022) Lactobacillus plantarum PL-02 Supplementation Combined With Resistance Training Improved Muscle Mass, Force, and Exercise Performance in Mice. Front. Nutr. 9:896503. doi: 10.3389/fnut.2022.896503
Received: 15 March 2022; Accepted: 07 April 2022;
Published: 27 April 2022.
Edited by:
Alev Kural, University of Health Sciences, TurkeyReviewed by:
Raees Khan, National University of Medical Sciences (NUMS), PakistanCopyright © 2022 Yeh, Hsu, Ho, Ho, Kuo, Tsai, Huang and Lee. This is an open-access article distributed under the terms of the Creative Commons Attribution License (CC BY). The use, distribution or reproduction in other forums is permitted, provided the original author(s) and the copyright owner(s) are credited and that the original publication in this journal is cited, in accordance with accepted academic practice. No use, distribution or reproduction is permitted which does not comply with these terms.
*Correspondence: Chi-Chang Huang, am9objU1MjNAbnRzdS5lZHUudHc=; Mon-Chien Lee, MTA2MTMwNEBudHN1LmVkdS50dw==
Disclaimer: All claims expressed in this article are solely those of the authors and do not necessarily represent those of their affiliated organizations, or those of the publisher, the editors and the reviewers. Any product that may be evaluated in this article or claim that may be made by its manufacturer is not guaranteed or endorsed by the publisher.
Research integrity at Frontiers
Learn more about the work of our research integrity team to safeguard the quality of each article we publish.