- 1Department of Pharmacy, School of Medicine and Health Sciences, Atma Jaya Catholic University of Indonesia, Jakarta, Indonesia
- 2Research Division for Natural Products Technology (BPTBA), National Research and Innovation Agency (BRIN), Yogyakarta, Indonesia
- 3Department of Pharmacology and Toxicology, Faculty of Pharmacy, Zagazig University, Zagazig, Egypt
- 4Institute of Pharmacy and Molecular Biotechnology (IPMB), Heidelberg University, Heidelberg, Germany
- 5AgroBioSciences Department, Mohammed VI Polytechnic University, Ben-Guerir, Morocco
The metabolic syndrome (MS) is a multifactorial syndrome associated with a significant economic burden and healthcare costs. MS management often requires multiple treatments (polydrug) to ameliorate conditions such as diabetes mellitus, insulin resistance, obesity, cardiovascular diseases, hypertension, and non-alcoholic fatty liver disease (NAFLD). However, various therapeutics and possible drug-drug interactions may also increase the risk of MS by altering lipid and glucose metabolism and promoting weight gain. In addition, the medications cause side effects such as nausea, flatulence, bloating, insomnia, restlessness, asthenia, palpitations, cardiac arrhythmias, dizziness, and blurred vision. Therefore, is important to identify and develop new safe and effective agents based on a multi-target approach to treat and manage MS. Natural products, such as curcumin, have multi-modalities to simultaneously target several factors involved in the development of MS. This review discusses the recent preclinical and clinical findings, and up-to-date meta-analysis from Randomized Controlled Trials regarding the effects of curcumin on MS, as well as the metabonomics and a pharma-metabolomics outlook considering curcumin metabolites, the gut microbiome, and environment for a complementary personalized prevention and treatment for MS management.
Introduction
The metabolic syndrome (MS) is a complex health disorder and causes a substantial economic burden (1) as it involves numerous health conditions including obesity, type 2 diabetes, insulin resistance, atherosclerotic cardiovascular disease, elevated blood pressure, and dyslipidemia (2, 3). Polydrug approaches are often utilized in MS management but such therapeutics often exhibit adverse side effects, such as nausea, flatulence, and diarrhea as common side effects of metformin (4, 5). Insomnia, anxiety, asthenia, palpitations, vertigo, and blurred vision are caused by phentermine and diethylpropion (6), as well as hypoglycemia, pain on injection site, weight gain, and nausea, are frequent side effects of Neutral Protamine Hagedorn (NPH), liraglutide, and exenatide (7, 8).
Natural products offer broad pharmacological activities acting via multiple mechanisms on MS pathophysiological signaling (9–11). However, the lack of understanding of MS pathophysiology at the cellular and molecular level (12, 13) means that clinical reports on natural products, for instance as preventive agents, often disagree with the observed preclinical findings. Furthermore, only a few natural products and food items have been translated into clinical trials, for example, resveratrol, orange, grape, walnut, soy, and mushroom improve insulin secretion and sensitivity, modulating several risk factors such as obesity, dyslipidemia, lipid peroxidation, coronary artery abnormalities, and Non-Alcoholic Fatty Liver Disease (NAFD) (14, 15).
Curcumin, a substantial active compound of turmeric (Curcuma longa), has multiple pharmacological effects and is safe for food-based biotechnology and medical applications (Figure 1) (16, 17). Curcumin and derivatives can be classified as phenolic secondary metabolite which possess at least two phenolic hydroxyl groups, which can dissociate to negative charged phenolate ions under physiological conditions. Curcumin can thus interact with proteins by forming hydrogen bridges and ionic bonds, which in consequence can alter the properties of a protein (18). Furthermore, as a phenolic phytoconstituent, curcumin has antioxidative properties and can thus counteract the negative consequences of a high concentration of reactive oxygen species (ROS) (19). However, its application is limited by poor bioavailability (20, 21), thus there have been attempts to develop curcumin delivery systems, such as nanoparticles and liposomes, along with some evidence on its efficacy and safety (22–25).
A systematic review published in 2016 reviewed the effects of curcumin on chronic diseases including cancer, inflammatory diseases, metabolic diseases, cardiovascular diseases, neurological disease, and skin diseases (26). More recent clinical studies and systematic review/meta-analysis of curcumin, especially regarding MS, have been published but there is heterogeneity regarding curcumin's effects in MS, mainly based on the phenotypic causes. Tabrizi et al. reported that curcumin reduced fasting glucose, HbA1c, triglycerides, and total cholesterol in MS patients, although no changes in low density lipoprotein (LDL) and high density lipoprotein (HDL) levels were observed (27). In the other hand, Azhadri et al. found that curcumin improved fasting blood glucose, triglycerides, HDL, and diastolic blood pressure without affecting systolic blood pressure or waist circumference (28). Curcumin supplementation can increase circulating adiponectin in patients with MS, reducing the risk of cardiovascular diseases (29) and promote lipid metabolism and glycemic control in polycystic ovary syndrome (PCOS) patients with no significant adverse effects (30).
The rationale for the current review is as follows:
• To identify new possible molecular and cellular targets of curcumin
• To provide a comprehensive updated understanding and meta-analysis of the clinical effects of curcumin on MS and its components
• To understand that the response to curcumin depends on the metabolic profile and phenotypes involved in the disease progression
• To provide information about the effects of “environmental factors” (metabolizing enzymes, gut microbiome, nutrient) on curcumin metabolism and biotransformation that appear strongly correlated with its efficacy and bioavailability and represents a necessary knowledge to move forward personalized interventions and prescriptions
Molecular Targets of Curcumin
Fundamental Molecular Targets
Curcumin interacts with macrophages, muscle cells, adipocytes, hepatic stellate, and pancreatic cells via targeting multiple molecular components including transcription factors, inflammatory mediators, enzymes, such as protein kinases (31, 32), histone acetyltransferase (33, 34), DNA methyltransferases, histone deacetylases, and modulation of micro RNAs (Figure 2) (35). Furthermore, many of the protein targets are components of multiple cell signaling pathways involved in the development of metabolic disorders, such as Peroxisome proliferator-activated receptor gamma (PPARγ), nuclear factor κB (NF-κB)/nuclear factor-erythroid 2 related factor 2 (NRF2), Wnt/β-catenin, NRF2/Kelch-like ECH-associated protein 1 (KEAP1), FOXO, and phosphatidylinositol-3-kinase(PI-3-K)/Akt/tumor necrosis factor-α (TNF-α) (Figure 3) (36).
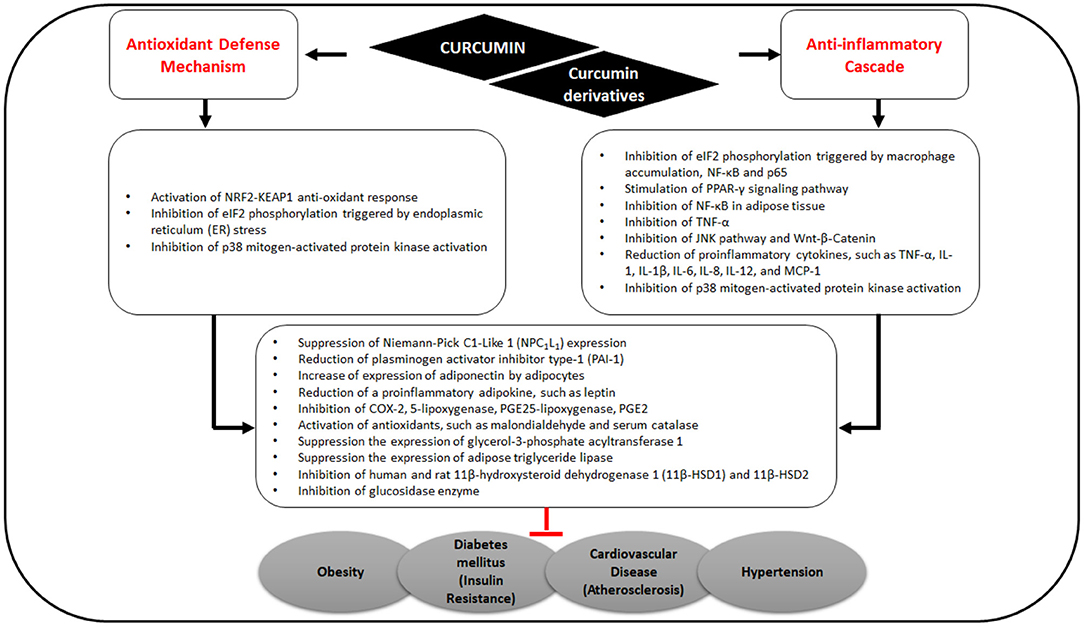
Figure 3. Effects of curcumin on antioxidant defense mechanisms and anti-inflammatory cascade related to MS.
By stimulating PPARγ and NRF2 signaling pathways, curcumin consequently up-regulates adiponectin and other gene products, leading to the downregulation of adipokines including resistin, leptin, TNF, interleukin (IL)-6, and monocyte chemotactic protein-1 (37). In a recent in silico study, Shakour et al. reported that curcumin and its derivatives such as bisdemethoxycurcumin, cyclocurcumin, and demethoxy curcumin have an important regulatory function in oxidative and inflammatory processes involved in many diseases (38).
Curcumin in Inflammation, Obesity, and Diabetes
Obesity is a chronic disease associated with inflammation; it has a main role in MS development as well as increasing the risk of atherosclerosis. Alteration of adipose tissue reduces lipid metabolism, causing an imbalance in the energy storage in fat tissue, thus affecting endocrine system and immune response. These alterations are primarily marked by the secretion of leptin, adipocyte-specific hormone resistin, and plasminogen activator inhibitor type-1 (PAI-1). In addition, inflammatory cytokines are also released such as TNF, IL-1, IL-6, and monocyte chemotactic protein (MCP)-1. These cytokines and chemokines significantly promote insulin resistance and mediate chronic inflammation.
Curcumin in turmeric is notable for its antioxidant and anti-inflammatory effects (Figure 3) (39, 40) and for ameliorating obesity risk factors (41) via the inhibition of NF-κB in adipose tissue, thereby reducing the expression of TNF-α, IL-6, MCP-1, PAI-1, and increasing adiponectin expression (40).
Some molecular pathways of inflammation and obesity are cross-talked with insulin resistance and diabetes (42, 43). Oxidative stress induced by elevated levels of circulating free fatty acids, endoplasmic reticulum (ER) stress, and proinflammatory signaling pathways also contribute to the induction of insulin resistance (43–45). Curcumin acts mainly as a signaling modulator that activates endogenous antioxidant responses such as the NRF2-KEAP1 (45) and has been demonstrated to reduce ER stress in a high-fat diet mice model, in vitro in primary adipocytes (46), and in palmitate-induced insulin resistance in human umbilical vein endothelial cells (HUVECs) (47). Lipolysis decreases and insulin resistance improves with decreased ER stress (47) but the relationship between ER stress, mitochondrial stress, and inflammation is complex (43). Thus, the curcumin effects require further investigation to elucidate the mechanism and links to other metabolic processes.
Curcumin in Cardiovascular Diseases
Cardiovascular diseases (CVDs) are the notable cause of death globally. Curcumin has been shown to prevent and treat CVDs via modulation of multiple signaling pathways related to cellular proliferation, growth, survival, oxidative stress, and inflammation. Curcumin prevents cardiovascular dysfunction and its primary risk factors (atherosclerosis, aortic aneurysm, myocardial infarction, and stroke) (48, 49) by activating endogenous antioxidant, anti-inflammatory, and anti-apoptotic responses, thereby improving cardiovascular complications, heart failure, and cardiotoxicity (50–52).
Preclinical Studies
Preclinical studies are a fundamental stage in drug development in which essential pharmacologic features of drug candidates are revealed before translating into clinical studies (53, 54). Investigations of pharmacodynamics and pharmacokinetics determine how the body responds to the drug also elucidate potential toxicity (55). It is vital to consider the disease origin and pathology to select an appropriate model, either cells or animals; however developing animal models to mimic MS is challenging (56, 57) as this multifactorial disease involves various enzymes and biomolecules in several organs. Also, interconnected organs can potentially result in a complication, thus optimizing appropriate models to understand the effect of curcumin on specific sites, therapeutic dosage, and administration route is critical. Table 1 presents a summary of the preclinical models used to study the effect of curcumin on MS, with dose administration noted to be a critical aspect resulting in different therapeutic effects.
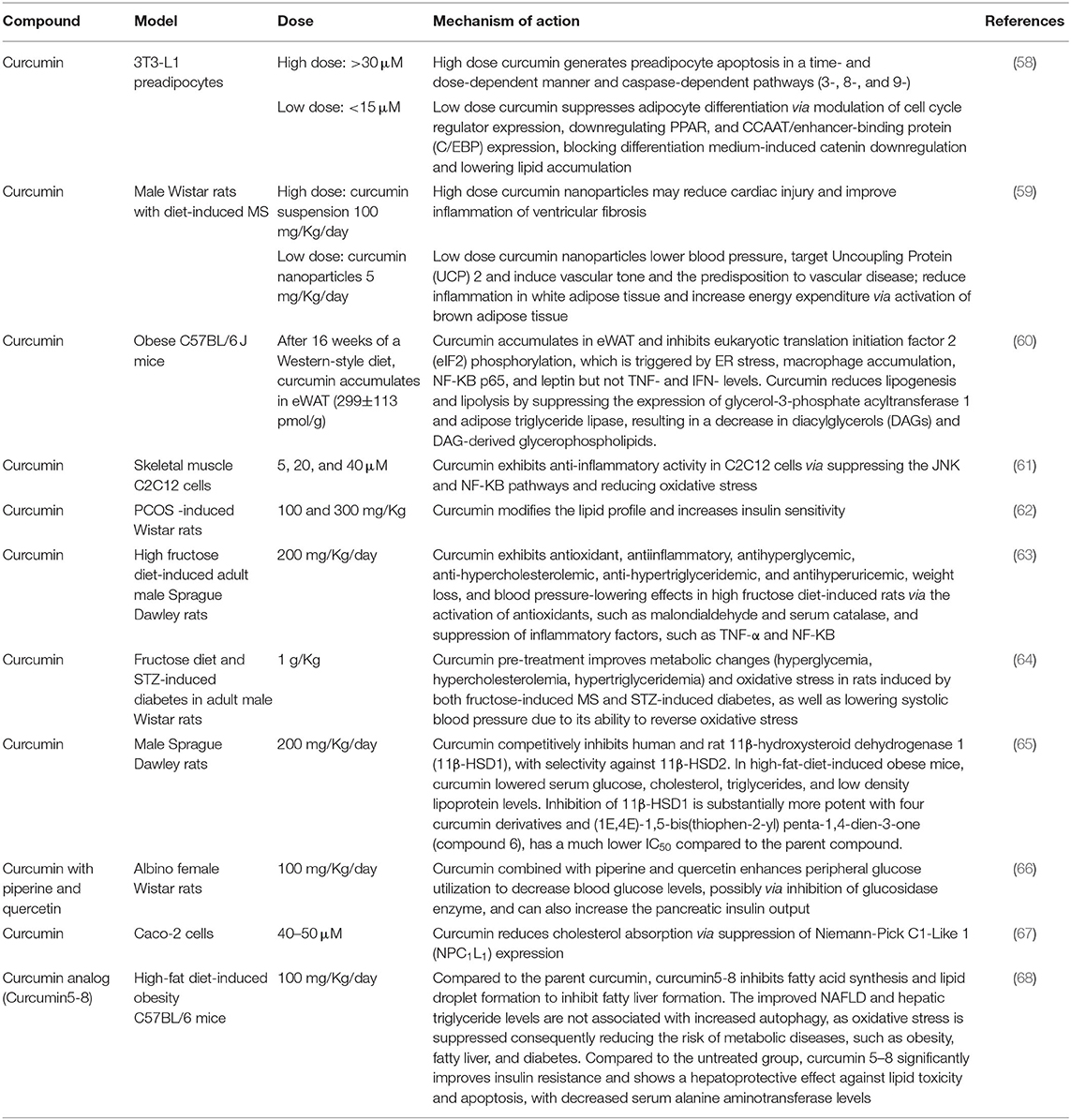
Table 1. Summary of in vitro or animal models studies on the effect of curcumin and its analogs on the individual parameter of MS.
Meta-Analysis of Curcumin in Metabolic Syndrome
Due to the divergence of clinical evidence of curcumin in improving MS, we included up-to-date meta-analyses in the current review to precisely estimate the effect size. Heterogeneity value in meta-analyses is our main concern to analyze and synthesize rigorous conclusions. We specify the heterogeneity data based on several MS parameters to provide parameter-specific effectiveness of curcumin. Eighteen meta-analyses from Randomized Controlled Trials (RCT) were evaluated (Table 2) showing high heterogeneity on some parameters (I2 > 50%, P <0.05), indicating that curcumin may partially reduce MS through a specific mechanism.
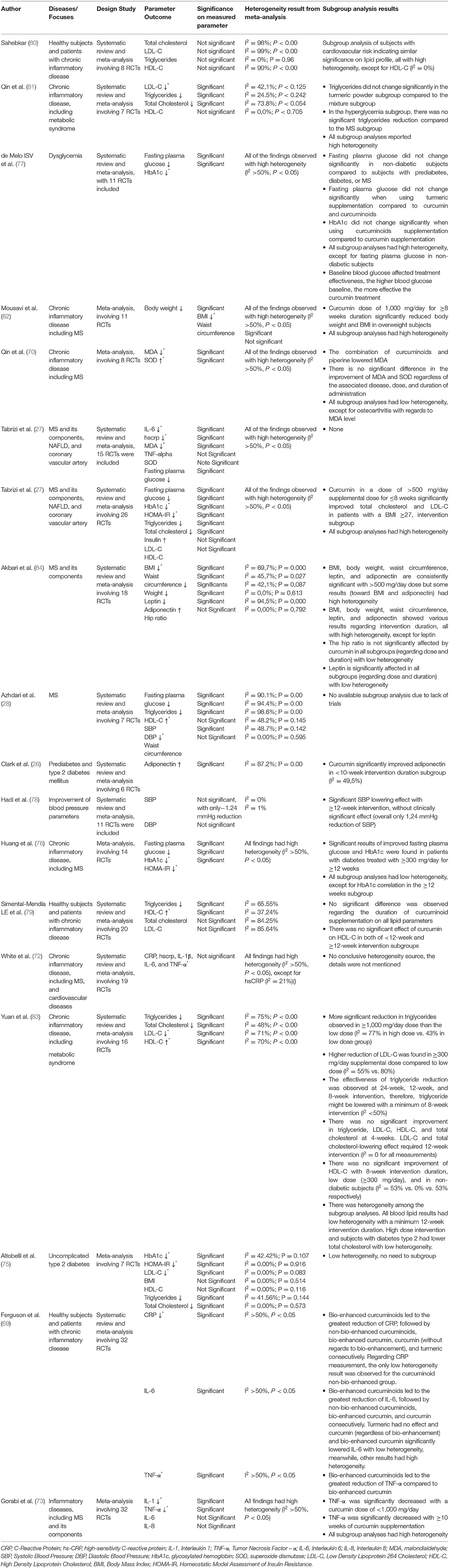
Table 2. Meta-analysis of RCT of curcumin, curcuminoids, or turmeric in patients with triggering components in the origin of the MS (27, 28, 69–83).
Curcumin Effects on Inflammation
Curcumin is a popular traditional medicine for inflammatory conditions (39). Inflammation is involved in MS as elevated visceral adipose tissue modulates adipokine secretion leading to decreasing adiponectin levels, increasing leptin and proinflammatory cytokines (i.e. TNF-α and IL-6), thereby promoting endothelial cell dysfunction and atherosclerosis (85). Non-bio-enhanced curcuminoid can lower C-Reactive Protein (CRP), while other forms of curcumin, such as bio-enhanced curcuminoids, bio-enhanced curcumin, curcumin without bio-enhancement, and turmeric had no effect, showing the tendency of curcumin to lower IL-6 regardless of bio-enhancement (69). Qin et al. (70) also showed that malondialdehyde (MDA), a stress oxidative marker, is lowered by curcumin regardless of intervention dose, duration, and underlying disease, except for osteoarthritis. Moreover, the combination of curcuminoids and piperine is more effective than curcumin alone to reduce MDA levels (70) but does not affect TNF-α, leptin, or adiponectin (27, 69, 71–74, 84). Consumption of nanoparticles of curcumin for 6 weeks, along with regular exercise in 44 older women with MS, decreased IL-6, increased IL-10, and increased brain-derived neurotrophic (BDNF) level (86).
Curcumin Effects on Obesity
Curcumin has been reported to activate AMP-activated protein kinase (AMPK) to inhibit adipocyte differentiation. Stimulation of AMPK down-regulated PPAR-γ in 3T3-L1 adipocytes to prevent insulin resistance and obesity (87). Di Piero et al. reported that curcumin has a beneficial effect on weight management in 44 overweight subjects by improving weight loss, reducing body fat, increasing waistline reduction, improving hip circumference reduction, and enhancing reduction of BMI (p <0.01 for all comparisons) without significant statistical effect on phosphatidylserine (88). Akbari et al. (84) reported that curcumin in a dose of 70–2,400 mg/day for 4–36 weeks can significantly reduce BMI and body weight compared to placebo. The effect of curcumin based on dose and duration of intervention, however, showed high heterogeneity among clinical studies. Waist circumference was also significantly reduced but there was no clinical data regarding a clinical significance in the hip ratio (84). Azhdari et al. and Altobelli et al. also reported the insignificant effect of curcumin in reducing BMI and waist circumference (28, 75), thus the effectiveness of curcumin in improving obesity is unclear.
Curcumin Effects on Diabetes Mellitus and Insulin Resistance
Diabetes mellitus (DM) is a metabolic disorder with hyperglycemia as the main clinical feature. The association between insulin and its producing organ, the pancreas, forms the fundamental understanding of the diseases. Over the last 100 years, clear evidence has distinct two types of diabetes: “insulin-dependent” (Type 1–T1DM) in which the pancreas completely fails to produce insulin and “non-insulin-dependent” (Type 2–T2DM). In T2DM, insulin hypersecretion by β-cell (hyperinsulinemia) causes the inability of the pancreas to cope with required insulin and later can experience insulin resistance, resulting in elevated blood glucose levels (hyperglycaemic hyperinsulinemia). Insulin resistance, as an onset of hyperinsulinemia, can cause the inability of β-cell to secrete insulin, apparently due to the manifestation of hyperglycemia (hyperglycaemic hypoinsulinemia). In addition, the liver contributes to systemic insulin resistance in which gluconeogenesis is undertaken, and hepatic insulin resistance can occur during the hyperglycemia state. Muscle insulin resistance can also occur when fat accumulation disturbs glucose uptake and affects insulin signaling. Fat accumulation in pancreatic cells causes β-cell dysfunction, increases plasma glucose, and reduces insulin response. Understanding the complex pathologies of DM promotes the evidence that DM is associated with greater risk factors related to cardiovascular diseases, such as coronary heart diseases and stroke, and non-vascular diseases, including cancer, neuro disorder, and liver diseases (89).
Nine-month curcumin consumption improves β-cell function by increasing Homeostatic Model Assessment (HOMA)-β, lowering Homeostatic Model Assessment of Insulin Resistance (HOMA-IR) and C-peptide (90). In the meta-analysis by Huang et al. (76), curcumin significantly improved fasting plasma glucose, HOMA-IR, and glycated hemoglobin (HbA1c). Subgroup analysis confirmed the significant effects of curcumin (≥300 mg/day dose and ≥12-week intervention) in improving plasma fasting glucose and HbA1c in the diabetes subgroup, with no significant effects in the non-diabetic subgroup (76). Melo et al. also reported that curcumin treatment was more effective in the higher blood glucose baseline (77). Hodaei et al. confirmed the findings reporting daily administration of 1,500 mg curcumin has a beneficial influence in decreasing weight and fasting blood glucose in subjects with type 2 diabetes (91).
Curcumin Effects on Hypertension
Hypertension is a common component of MS and there are two meta-analyses of curcumin in hypertension. Hadi et al. reported no significant effect of curcumin on systolic blood pressure (SBP) and diastolic blood pressure (DBP), although SBP was reduced (average reduction of 1.24 mmHg) but not significantly at ≥ 12-week (78). Azhdari et al. confirmed that curcumin has no significant effect on SBP but significantly improved DBP (heterogeneity <50%) (28).
Curcumin Effects on Dyslipidemia
Dyslipidemia is a clinical condition described as elevated total or LDL-cholesterol (LDL-C) or low HDL cholesterol (HDL-C) levels and is considered a serious risk factor for coronary heart disease and stroke (92). Yuan et al. (83) reported that curcumin might reduce total cholesterol when administrated to diabetic patients for a minimum of 12 weeks (>300 mg/day), reduce LDL-C after a minimum 12-week intervention, and triglycerides after a minimum 8-week intervention. More prolonged administration may be required to improve the efficacy of curcumin on dyslipidemia. However, Simental-Mendía et al. found that curcumin did not affect HDL-C regardless of intervention duration (<12-week and ≥12-week) (79). Another meta-analysis also confirmed the non-significant effect of curcumin in improving HDL-C with low heterogeneity (75, 80, 81).
Other Clinical Studies
Apart from the meta-analysis included in this review, other studies were conducted on subjects not specifically on the MS.
Curcumin Effects on Non-alcoholic Fatty Liver Disease (NAFLD)
NAFLD and MS are two different disorders with overlapping clinical and pathophysiological characteristics. NALFD indicates mere liver steatosis to overt liver cirrhosis (93). Oxidative stress and inflammation play major roles in the progression of NAFLD. As an anti-inflammatory modulator, curcumin might improve health parameters related to NAFLD (94). Some RCT reported the effectiveness of curcumin and curcuminoids in improving various health parameters in NAFLD patients, i.e., serum levels of pro-inflammatory cytokines (IL-1α, IL-1β, IL-2, IL-4, IL-6, IL-8, IL-10, TNF-α, monocyte chemoattractant protein-1, interferon γ, vascular endothelial growth factor, and epidermal growth factor, fasting plasma insulin (FPI), HOMA-IR, liver transaminases, fatty liver index, serum cortisol, ultrasound morphological of the liver, and other parameters included in MS (p <0.05) (94–112). The findings were consistent regarding the beneficial effects on improving NAFLD risk factors (113), with only one study having conflicting results, whereby curcumin was reported to have no impact on liver transaminases, metabolic and inflammatory parameters. This inconsistency is probably due to the low curcumin dose of only 35 mg curcumin per intake, thus reducing its bioavailability and effectiveness (114). The combination of curcumin with other substances, such as ursodeoxycholic and piperine, has shown a positive outcome in improving NAFLD (115–117). Dose and term administration should be critically appraised.
Curcumin Effect on Sleep Duration
Sleep duration might be linked to MS and a short sleep duration has been associated with obesity. Sleep deprivation could increase oxidative stress mediators in the brain and since curcumin may relieve oxidative stress, it may be beneficial in suppressing stress and prolonging sleep duration. However, according to a trial by Saberi-Karimian et al. curcumin did not have any impact on sleep duration in patients with MS (118).
Curcumin Effect on Oxidative Stress and Heat Shock Proteins
Serum copper and zinc and the ratio of Zn/Cu are often used to identify oxidative stress and inflammation (119, 120). Furthermore, protein chaperones, such as heat shock proteins (HSP) are highly expressed during oxidative stress (121). Some recent studies reported the effects of curcumin and its derivatives on some oxidative stress markers in MS patients (122), for instance, serum copper, zinc, and trace elements are elevated in patients with MS compared to healthy subjects (120). A double-blind clinical trial of 120 subjects with MS reported increased serum zinc and Zn/Cu ratio after administration of curcumin and phospholipid curcumin complex at a dose of 1 g/day for 6 weeks (123). However, Mohammadi et al. reported no significant difference in the alteration of HSP 27 serum concentrations among the study groups (group received curcumin, phospholipid -curcumin complex, or a placebo for 6 weeks) (124). Oxidative stress occurs as a result of an imbalance between pro-oxidant and antioxidant defense systems, resulting in cellular damage and associated diseases including MS. The redox stress index indicates the pro-oxidant/antioxidant balance in human serum due to oxidation and reduction reactions that coincide (125). Ghazimoradi et al. (126) conducted a double-blind, randomized, placebo-controlled trial over 6 weeks of 120 individuals with MS and three groups of subjects received curcumin, phospholipid curcumin, and placebo. Serum PAB was elevated significantly in the curcumin group. Still, the study did not assess different doses of curcumin and did not consider the impact of other adjuvants and its possible interaction that may affect the efficacy of curcumin (126). No significant effect was observed on the serum antioxidant vitamin E level after administration of 1 g curcumin supplement per day for 6 weeks, indicating that the reported antioxidant effect of curcumin is mainly via the modulatory action on the intracellular antioxidant system rather than serum vitamin E (127).
Clinical Studies of Nano Curcumin
One of the limitations of using phytochemicals often is their poor bioavailability in the human body, thus nano formulations have been employed to improve the delivery of phytochemicals such as curcumin, berberine, naringenin, quercetin, emodin, myricitrin, stevioside, and resveratrol to the target organ and cells (128). Nano-based formulations for the MS that are biodegradable, biocompatible, nontoxic, and stable after oral administration should be developed focusing on the multifactorial effects rather than a single factor (128). A recent study by Bateni et al. reported that nano-micelle curcumin supplement at a dose of 80 mg/day significantly improved serum triglycerides in patients with MS, although no significant difference was observed in anthropometric measurements, blood pressure, and several biochemical factors (129). Nano curcumin supplementation (80 mg/day for 6 weeks) along with regular exercise significantly reduced inflammation in an elderly woman with MS, as well as decreased the percentage of fat and IL-6, and increased IL-10 and BDNF (86).
Biosafety of Curcumin
Curcumin had been approved as a safe agent under the Generally Recognized as Safe (GRAS) category by the FDA (130). Nevertheless, the European Medicine Agency (EMA) released a revised version of the guideline for using C. longa with special warnings and precautions for patients with obstruction of the bile duct, cholangitis, liver disease, gallstones, and any other forms other biliary diseases. Due to a lack of data on children, adolescents under 18 years, pregnancy, and lactation, the use of C. longa is not recommended to those groups of the population (131). Mild undesirable side effects such as dry mouth, flatulence, and gastric irritation should also be critically appraised (131). Panahi et al. reported curcumin is well tolerated with the possibility of minor side effects, including diarrhea, constipation, headache, skin rash (132). A clinical study also reported nausea and colds in the curcumin group (20).
Acceptable Daily Intake (ADI) for curcumin as food additive defined by the European Food Safety Agency (EFSA) is 3 mg/kg BW/day based on No Observed Adverse Effect Level (NOAEL) of 250–320 mg/kg BW/day from the toxicity study (133). Some studies reported curcumin is considered safe even at high doses of up to 4–8 g/day (40, 134). Rigorous studies to understand curcumin's safety as a single modality and/or combination with other substances are warranted, including human trials, to repulse possible hepatotoxicity and genotoxicity disputes (135–137).
Ongoing Trials
There are currently six ongoing trials examining the efficacy of curcumin in improving MS parameters, including five trials assessing blood glucose, four trials evaluating serum lipid profile, three trials measuring blood pressure, two trials examining body weight, and three trials evaluating oxidative stress and inflammation markers. Due to the fast metabolism and low gut absorption of curcumin, three of these trials are also examining specific curcumin formulations for enhanced bioavailability, e.g., micellar curcumin, nano curcumin, and nano micelles. Another trial is also investigating the effects of curcumin in combination with other agents including inulin, resveratrol, and omega-3 fatty acids to enhance its effectiveness. One study in Thailand is recruiting many participants (n = 200) with a long intervention duration (12 months) of regular curcumin that anticipates that the long-term supplementation with this new curcumin formulation may result in a better outcome (138).
Metabolomics and Personalized Treatment
Personalized medicine or treatment offers a potential prospect for better patient care by tailoring therapy based on the genotype and phenotype profiles of the patient and diseases progression with greater precision (139, 140). The approach includes earlier diagnoses, risk assessments, and choosing optimum treatment (139). Analysis of metabolic phenotype was introduced more than a decade ago to distinguish health and diseases (141, 142) but its application in intensive care only recently began following the emergence of personalized treatment (143). The application of metabolomics is particularly promising for MS, a syndrome characterized by complex human body metabolites, environmental and genetic factors. Some exploratory experiments recently reported the metabolic profile of MS in which some biomarkers have been elucidated for pathogenesis diagnostic, disease progression, and better management (targeted therapy) (144–147).
Since MS is multifaceted, identifying metabolomics and lipidomics is crucial as a comprehensive strategy to manage the significant heterogeneity of metabolites in biofluids. However, this will require appropriate sample preparation, the complexity of data analysis, and new biomarker discovery. The identification of MS biomarkers in adults and the elderly are also worthy of investigation as currently many limitations exist (146). Using this approach, sub-typing pathophysiology of MS offers stratified management, including the prevention and co-treatment using a natural product such as curcumin.
Besides elucidation of phenotype and sub-phenotype of diseases, pharmacometabolomics can provide information regarding how an individual will respond to a drug. Worth noting that the genome, the gut microbiome, sex, nutrition, age, stress, health status, and environment influence the individual response to pharmaceutical compounds, including herbal and phytomedicines. Real-time metabolomic profiling during the treatment could discern the drug mechanism of action, variation in the pharmacokinetic response and determine better-targeted therapy. Pharmacometabolomics in phytomedical clinical research has been considered to aid clinical translation and inform utilization of phytomedicines, most importantly, to be a personalized treatment (148, 149).
Pharmacometabolomics apprise the advance of pharmacogenomics (150), so metabolic profiling can be employed synergistically with genomic analyses since systems biology integrates both genetic and environmental factors. Conversely, employing genetic information only (pharmacogenomics) may not develop into a comprehensive disease phenotyping and therapeutic response, hence both approaches should be implemented simultaneously.
Metabolomics and pharmacometabolomics are particularly necessary to advance the utilization of natural products, such as curcumin (106), as natural products can undergo biotransformation during absorption and metabolism in the body, for instance, the presence of the metabolizing enzyme can alter the activity of the natural products. The intestine microbiome also plays a vital role in the body's response to any drug or nutrition.
Biotransformation of Curcumin
Curcumin is unstable with low bioavailability (20, 21). There is much evidence that the pharmacological effects of curcumin are mainly due to its metabolites (151). Holder et al. suggest that curcumin elimination will mainly undergoes through bile: The primary biliary metabolites of curcumin consist of the two glucuronide conjugates [tetrahydrocurcumin (THC) and hexahydrocurcumin (HHC)], whereas the minor metabolites consist of ferulic acid and dihydro ferulic acid (152). Glucuronide and sulfate derivatives are the main detectable metabolites in urine and after five days, about 40% are excreted in an unchanged form. Another in vivo study indicated that less than half of the curcumin remains in the lower part of the gut, attributed to the biotransformation of curcumin to other components in the gut (153). Kunnumakkara et al. reported that curcumin is undetectable in the heart, blood, and at a very low concentration in the liver, kidney, and portal blood (154).
The intravenous administration of curcumin in rats was associated with a low concentration of THC and dihydrocurcumin (DHC) in plasma. Curcumin was detected in the kidney, liver, and brain. In contrast, THC and DHC were detected in only the kidney and liver, indicating that curcumin crosses the blood-brain barrier, converting a prodrug molecule and possibly targeting neuron dysfunction and disorder (155).
Some investigations in human and rat intestinal tissues showed that curcumin is metabolized in the human intestinal tissue. The extent of curcumin conjugation in the human intestine is much higher than in the rat intestine, however, there is less curcumin conjugation in the human liver compared to rat liver, indicating that curcumin is biotransformed into THC and DHC, thereafter converted into glucuronide conjugates (156).
Enzymes involved in sulphation and glucuronidation are also found in the kidney, liver, and intestinal mucosa. In the intestine, curcuminoids, including curcumin, are conjugated to glucuronide, then form a glucuronide conjugates in the portal vein and subsequently conjugate into glucuronide/sulfate in the liver. Unlike oral administration, curcuminoids administrated intravenously in rats enter the blood circulation and dispose of in the liver as a free form (157).
To date, there are two known phases of curcumin metabolism, phase I and phase II. Phase I metabolism involves saturating double bonds to form several curcumin metabolites such as di, tetra, hexa, and octahydrocurcumin, whereas, in phase II metabolism, glucuronide or sulfate is conjugated to curcumin as well as its metabolites (154). Curcumin and its metabolites can form conjugation to any of their phenol-OH groups (21). Additionally, curcumin metabolism may occur in the intestine by microorganisms such as Escherichia coli whereby the NADPH-dependent enzyme converts curcumin into di- and tetrahydrocurcumin (158).
Curcumin degradation products including its metabolites may involve in the observed therapeutic effects. A few curcumin metabolites have been investigated in preclinical studies, for example, DHC exhibited lipid biosynthesis and increased lipid oxidation in HepG2 and LO2 cells. A molecular docking study revealed that DHC showed more affinity for binding to the phospholipase A2 active site than curcumin, indicating that DHC possesses anti-inflammatory activity (159), decreasing oxidative stress by upregulating NRF2, decreasing insulin resistance by increasing glucose uptake, and modulating the PI3K/AKT pathway (160).
Based on the chemical structure, THC is more stable and soluble in an aqueous medium than curcumin, as the former lacks α, β dienes, so it is unable to form Michael adducts with intracellular proteins. Nevertheless, the therapeutic activities of THC have been reported such as anti-aging, anti-neurodegeneration, and anticancer effects with significant antioxidant activity, so it can prevent human diseases related to oxidative stress (36). Hexahydrocurcumin (HHC), a hydrogenated metabolite of curcumin, is not well studied but it has been documented to have anticancer, antioxidant, antiinflammatory, and cardioprotective activities. Some of which have been reported to be more potent than the parent compound, curcumin, such as free radical scavenging activities (161). Octahydrocurcumin (OHC) exhibits several activities similar to the parent compound, curcumin, while its antitumor and anti-inflammatory activities were more potent than curcumin (162–164).
Metabolism of Curcumin by Metabolizing Enzymes
Drug metabolism is categorized into phase I and phase II metabolism. Cytochrome P450 (CYP) enzymes, a large superfamily of heme proteins, play a major role in modulating phase I metabolism by catalyzing oxidative biotransformation of drugs and xenobiotics (165, 166). At least 57 CYP genes have been identified in humans. Of 18 families and 44 subfamilies human CYPs, only families 1–3 are most active in hepatic metabolism with five of them (CYP 1A2, 2C9, 2C19, 2D6, and 3A4) contributing to metabolism of 75% of drugs (165, 167). Drug therapy can induce or inhibit CYP enzymes resulting in drug-drug interaction. Induction or inhibition of CYP may decrease or increase plasma drug levels causing adverse drug reactions or toxicity (168). After CYP enzyme metabolism, most drugs are metabolized by conjugation reaction in phase II metabolism. Phase II enzymes contribute significantly to metabolic inactivation of drug compounds and biotransformation of xenobiotics to make them easier to excrete from the body. Phase II enzymes are mainly transferases, including glutathione S-transferases (GSTs), thiopurine-S-methyltransferase (TPMT), sulfotransferases (SULTs), uridine-diphosphate glucuronosyltransferases (UGTs), N-acetyltransferases (NATs), and NADH quinone oxidases (169).
Curcumin can modulate the activity of drug-metabolizing enzymes, both phase I and phase II metabolic enzymes (170). Curcumin is reported to significantly inhibit CYP2D6 activity in in vitro as well as in vivo. In vitro studies using human liver microsomes showed that 100 μg/mL of tumeric extract inhibited about 70% dextrorphan (metabolites of dextromethorphan mediated by CYP2D6) formation. Meanwhile, in vivo model exhibited a significant increase in human urinary metabolic ratio of dextromethorphan/dextrorphan after administration of tumeric extract (171). Similarly, CYP inhibitory activity assay using human recombinant CYP enzymes demonstrated that curcumin inhibited CYP2D6 by 60% at concentration of 120 μM and effectively inhibited CYP3A4 by almost eliminating the enzyme function at concentration ≥ 60 μM (172). In contrast, other in vivo and ex-vivo studies found that curcumin significantly activated CYP3A4. The study showed that 50 and 100 mg/Kg of curcumin markedly reduced the AUC0−540 of everolimus (a probe drug of P-glycoprotein/CYP3A4) by 70.6 and 71.5%, respectively (173). Meanwhile, Mach et al. reported that curcumin in liposomal formulation did not inhibit CYP2D6 and CYP3A4 activity, but liposomal curcumin at concentration of 58.3 μM inhibited CYP2C9 (10.5%) and CYP2C8 (22.5%) by in vitro assay (174).
Chen et al. investigated that 14 days of treatment with curcumin after caffeine administration significantly repressed CYP1A2 activity by 28.6% and significantly induced CYP2A6 activity by 48.9% in human subjects, but had no significant effect on N-acetyltransferase (NAT2) and xanthine oxidase (XO) (175). Volak et al. (176) reported that by in vitro assay using human liver microsomes, curcuminoid extract exhibits moderate to potent inhibitory activity (IC50<50 μM) toward various CYPs in the following ascending order CYP2C19 > CYP2B6 > CYP2C9 > CYP3A. In contrast, curcuminoid extract exhibits low inhibitory activity of CYP2D6, CYP1A2, and CYP2E1 (IC50 > 60 μM). Meanwhile, pure synthetic curcuminoids (curcumin, demethoxycurcumin, and bisdemethoxycurcumin) show similar inhibition to the CYP3A and CYP2C9 activities (176).
Several studies reported that curcumin modulates phase II metabolism enzymes. Curcuminoids can inhibit acetaminophen glucuronidation (by UGT enzyme) and acetaminophen sulfonation (by SULT enzyme) in LS180 cells. Curcumin is a more significant potent inhibitor of acetaminophen glucuronidation than demethoxycurcumin and bisdemethoxycurcumin, but compared to other curcuminoids, curcumin is the least potent inhibitor of acetaminophen sulfonation (176). In addition, curcumin is a potent inhibitor of phenol sulfotransferase (SULT1A1) in human liver, kidney, colon, duodenum, and lung (177). However, in a clinical study, short-term use (2 days) of curcuminoid/piperine combination is unlikely to affect significantly the pharmacokinetic disposition of drugs involving UGT and SULT (178).
In addition, curcumin was reported to modulate GST activity. Liu et al. (179) investigated the therapeutic potential of curcumin and resveratrol in mice bearing lung carcinoma induced by benzo(a)pyrene. The result showed that curcumin and a combination of curcumin-resveratrol treatment for 22 weeks significantly increased GST level by 14 and 18%, respectively, compared to benzo(a)pyrene treatment (179). Similarly, a study reported that 2 weeks of curcumin oral administration (400 mg/kg) in mice increased GST activity by 20% (180).
Curcumin and the Intestinal Microbiome
Gut microbiota has recently gained great attention due to their role in understanding the pathobiology of diseases, efficacy of treatment, as well as diet and nutrition that affect human health (181). There is increasing evidence supporting the concept that the gut microbiota is responsible for the regulation of metabolic disorder including obesity, weight gain, and insulin sensitivity (162–164). Phyla Firmicutes and Bacteroidetes are associated with genetic obesity in rodents (182, 183), but efficacy may differ among specific genera and species. Bacteroides, for instance, are linked to a high fat/low-fiber intake, while Prevotella are associated with complex carbohydrate intake (184). In-depth knowledge of diet-microbiota interactions is also important to identify the risk factors of metabolic disorders closely related to diet and nutrition. The gut microbiome can be modified and regulated by probiotics and/or prebiotics, such as non-digestible carbohydrates (163, 185), that are considered as part of therapeutic strategies (186).
A preclinical study of obese mice showed that dietary supplementation of prebiotic oligofructose decreased Streptococcus intermedius, a cytolysin-producing microorganism that normally disturbs intestinal wall permeability. The human intestinal mucin-degrading bacterium, Akkermansia muciniphila, was also increased after the administration of prebiotics and correlated with elevated levels of Glucagon-like peptide-1 (GLP-1) and Glucagon-like peptide-2 (GLP-2) in L-cells (187). This organism is associated with metabolic-regulating proteins (188). In addition, the elevated concentration of these GLPs can improve gut barrier function and metabolism via the metabolism of fatty acids to short-chain fatty acids (189). Oral intake of curcumin enhanced the intestinal barrier function, improved glucose tolerance, and lowered circulating LPS levels in Western diet-fed LDL receptor knockout (LDLR) mice (190). Curcumin can act as a prebiotic to influence the microbiome, consequently, influence metabolic imbalance such as obesity (191). A double-blind, randomized, placebo-controlled pilot study revealed turmeric and curcumin alter gut microbiota, including the increases in most Clostridium spp., Bacteroides spp., Citrobacter spp., Cronobacter spp., Enterobacter spp., Enterococcus spp., Klebsiella spp., Parabacteroides spp., and Pseudomonas spp. and the reduction of several Blautia spp. and most Ruminococcus spp. (192). Although clinical trials are challenging, especially introducing prebiotics and probiotics to modulate the gut microbiome, constant and in-depth studies must be performed as there are several differences in metabolic profiles determined by gut microbiota (193). The microbiota response to treatment is highly personalized. The breakdown of curcumin and the biotransformed products must also be elucidated, as curcumin degradation products and/or metabolites possibly access the systemic circulation and metabolic system, albeit at great variability. Thus, the results from clinical trials must be facilitated by concomitant biofluids marker assessments to investigate curcumin administration and the correlated clinical outcomes.
Conclusion and Future Perspectives
Preclinical findings of promising natural products frequently encounter challenges in clinical trials. Moreover, the diversity of clinical results is due to the divergence of metabolic phenotypes and physiological processes that affect disease progression in individuals/populations. Differing individual pharmacokinetic and dynamic profiles also affect the individual's response to natural products. Since MS is multifactorial, assessment of a single disease parameter after treatment is not sufficient, therefore large-scale studies and efforts are required to minimize bias. Some key points for further research and development include:
1. Oxidative stress and chronic inflammation are key factors in the pathogenesis of MS (194), with higher levels of oxidative stress markers observed in subjects with MS (195). Chronic hyperglycemia leads to oxidative stress leading to complications in patients with diabetes (194). In addition, fat accumulation in obese patients correlates with systemic oxidative stress in humans and mice, increased NADPH oxidase, and decreased endogenous antioxidant enzymes (196). Excess reactive oxygen species, chronic inflammation, increased fat accumulation, and insulin resistance leads to oxidative stress, whereby the antioxidant capacity of the human body is exceeded. Administration of curcumin, an antioxidant, sometimes failed to eliminate the excess free radicals, therefore an increased dose, delivery formulation, and duration of administration require further investigation. Elucidation of the effects of curcumin in healthy subjects before the excess oxidative stress occurs and can no longer be anticipated by single/mono phytochemical intervention should also be evaluated by measuring the ratio of pro-oxidants to antioxidants.
2. There is evidence that curcumin is degraded upon release into the physiological system or media regardless of the delivery mechanism. The combination of curcumin with various nutraceuticals may improve delivery, efficacy, and reduce toxicity, offering pleiotropic effects or modulation of multiple targets. Also, the use of crude turmeric extract might have more beneficial effects than the individual active compound, curcumin. When individual curcumin is explored, structural modification of curcumin is required to improve the bioavailability as well as the rate of metabolism. Investigations of the effects of curcumin in combination with other agents are ongoing, as well as a few clinical studies. Nonetheless, more, larger well-controlled clinical trials are necessary to evaluate the efficacy of each formulation compared to the parental compound focusing on specific disease targets.
3. The development of analogs and delivery systems are also ongoing to overcome the low bioavailability of curcumin, such as lipid vesicles, nanoparticles, and nanofibers. Besides, alternative routes of curcumin administration may enhance the therapeutic effect. One consideration that may require attention is the narrow therapeutic window leading to off-target toxicity.
4. Improved preclinical models are also required as animal model physiology is frequently different from human biology, accounting for the challenge in translating preclinical findings to the clinic. The stability and/or reactivity of a compound in assay buffer, media, and chemicals must be characterized for a rigorous and valid method. Also, the selection of the molecular target and pharmacology network should be identified, including the potency of off-target effects.
5. Degradation products or curcumin metabolites, including those produced by the gut microbiome, are of interest. Numerous curcumin studies have overlooked the investigation of the degradation products or curcumin metabolites, whether they share similar targets and responses in in vivo studies and clinical trials. There is much scope to investigate the role of gut microbiota on the pharmacological activity of curcumin regardless of the low systemic circulation.
6. Based on clinical studies and meta-analysis, curcumin/curcuminoid/turmeric might effectively lower fasting plasma glucose and HbAic, triglyceride, total cholesterol, and LDL, but no effect on patients with no diabetic history. The compound(s) show no effect on improving SBP and HDL-C. Metabolomics and pharmacometabolomics studies are particularly necessary to advance the precise and personalized utilization of curcumin, particularly in the case of MS. Metabolic profiling should be employed synergistically with genomic analyses (i.e. genes that encode metabolizing enzymes and drug transporters), as employing genetic information only (pharmacogenomics) may not lead to comprehensive disease phenotyping and translate into a therapeutic response.
Author Contributions
AN reviewed, drafted and designed, and conceived the manuscript. FC, MPW, and MM reviewed and drafted the manuscript. MW revised the manuscript. MS revised, designed, and conceived the manuscript. All authors contributed to the article and approved the submitted version.
Funding
APC was paid by UM6P.
Conflict of Interest
The authors declare that the research was conducted in the absence of any commercial or financial relationships that could be construed as a potential conflict of interest.
Publisher's Note
All claims expressed in this article are solely those of the authors and do not necessarily represent those of their affiliated organizations, or those of the publisher, the editors and the reviewers. Any product that may be evaluated in this article, or claim that may be made by its manufacturer, is not guaranteed or endorsed by the publisher.
Acknowledgments
The authors are grateful to Proofreading Service UK (PSUK) Communication LTD for notable manuscript proofreading.
References
1. Scholze J, Alegria E, Ferri C, Langham S, Stevens W, Jeffries D, Uhl-Hochgraeber K. Epidemiological and economic burden of metabolic syndrome and its consequences in patients with hypertension in Germany, Spain and Italy; a prevalence-based model. BMC Public Health. (2010) 10. doi: 10.1186/1471-2458-10-529
2. Rochlani Y, Pothineni NV, Kovelamudi S, Mehta JL. Metabolic syndrome: pathophysiology, management, and modulation by natural compounds. Ther Adv Cardiovasc Dis. (2017) 11:215. doi: 10.1177/1753944717711379
3. Cornier M-A, Dabelea D, Hernandez TL, Lindstrom RC, Steig AJ, Stob NR, et al. The metabolic syndrome. Endocr Rev. (2008) 29:777. doi: 10.1210/ER.2008-0024
4. Siavash M, Tabbakhian M, Sabzghabaee AM, Razavi N. Severity of gastrointestinal side effects of metformin tablet compared to metformin capsule in Type 2 Diabetes Mellitus patients. J Res Pharm Pract. (2017) 6:73. doi: 10.4103/JRPP.JRPP_17_2
5. Foss MT, Clement KD. Metformin as a cause of late-onset chronic diarrhea. Pharmacotherapy. (2001) 21:1422–4. doi: 10.1592/PHCO.21.17.1422.34430
6. Sherafat-Kazemzadeh R, Yanovski SZ, Yanovski JA. Pharmacotherapy for childhood obesity: present and future prospects. Int J Obes (Lond). (2013) 37:1. doi: 10.1038/IJO.2012.144
7. Chaudhury A, Duvoor C, Dendi VSR, Kraleti S, Chada A, Ravilla R, et al. Clinical review of antidiabetic drugs: Implications for Type 2 Diabetes Mellitus management. Front Endocrinol (Lausanne). (2017) 8:6. doi: 10.3389/FENDO.2017.00006
8. Riddle MC, Forst T, Aronson R, Sauque-Reyna L, Souhami E, Silvestre L, et al. Adding once-daily lixisenatide for type 2 diabetes inadequately controlled with newly initiated and continuously titrated basal insulin glargine: A 24-week, randomized, placebo-controlled study (GetGoal-Duo 1). Diabetes Care. (2013) 36:2497–503. doi: 10.2337/DC12-2462/-/DC1
9. Tabatabaei-Malazy O, Larijani B, Abdollahi M. Targeting metabolic disorders by natural products. J Diabetes Metab Disord. (2015) 14:1–21. doi: 10.1186/s40200-015-0184-8
10. Karou SD, Tchacondo T, Djikpo Tchibozo MA, Abdoul-Rahaman S, Anani K, Koudouvo K, et al. Ethnobotanical study of medicinal plants used in the management of diabetes mellitus and hypertension in the Central Region of Togo. Pharm Biol. (2011) 49:1286–97. doi: 10.3109/13880209.2011.621959
11. Chukwuma CI, Matsabisa MG, Ibrahim MA, Erukainure OL, Chabalala MH, Islam MS. Medicinal plants with concomitant anti-diabetic and anti-hypertensive effects as potential sources of dual acting therapies against diabetes and hypertension: a review. J Ethnopharmacol. (2019) 235:329–60. doi: 10.1016/j.jep.2019.02.024
12. Guo S. Insulin signaling, resistance, and the metabolic syndrome: insights from mouse models to disease mechanisms. J Endocrinol. (2014) 220:T1. doi: 10.1530/JOE-13-0327
13. Garg R, Kumariya S, Katekar R, Verma S, Goand UK, Gayen JR, et al. Signaling pathway in metabolic disorders: an emerging therapeutic target. Eur J Pharmacol. (2021) 901:174079. doi: 10.1016/J.EJPHAR.2021.174079
14. Gaggini M, Morelli M, Buzzigoli E, DeFronzo RA, Bugianesi E, Gastaldelli A. Non-Alcoholic Fatty Liver Disease (NAFLD) and its connection with insulin resistance, dyslipidemia, atherosclerosis and coronary heart disease. Nutrients. (2013) 5:1544. doi: 10.3390/NU5051544
15. Patti AM, Al-Rasadi K, Giglio RV, Nikolic D, Mannina C, Castellino G, et al. Natural approaches in metabolic syndrome management. Arch Med Sci. (2018) 14:422. doi: 10.5114/AOMS.2017.68717
16. Sharifi-Rad J, Rayess Y, El Rizk AA, Sadaka C, Zgheib R, Zam W, et al. Turmeric and its major compound curcumin on health: Bioactive effects and safety profiles for food, pharmaceutical, biotechnological and medicinal applications. Front Pharmacol. (2020) 11:1021. doi: 10.3389/FPHAR.2020.01021
17. Singh L, Sharma S, Xu S, Tewari D, Fang J. Curcumin as a natural remedy for atherosclerosis: a pharmacological review. Molecules. (2021) 26:4036. doi: 10.3390/MOLECULES26134036
18. Wink M. Modes of action of herbal medicines and plant secondary metabolites. Medicines. (2015) 2:251. doi: 10.3390/MEDICINES2030251
19. Wink M. Current understanding of mode of action of multicomponent bioactive phytochemicals: potential for nutraceuticals and antimicrobials. Annu Rev Food Sci Technol. (2022) 13:6.1–6.23. doi: 10.1146/annurev-food-052720-100326
20. Lopresti AL. The problem of curcumin and its bioavailability: Could its gastrointestinal influence contribute to its overall health-enhancing effects? Adv Nutr. (2018) 9:41–50. doi: 10.1093/ADVANCES/NMX011
21. Cas MD, Ghidoni R. Dietary Curcumin: Correlation between bioavailability and health potential. Nutrients. (2019) 11:2147. doi: 10.3390/NU11092147
22. Prasad S, Tyagi AK, Aggarwal BB. Recent developments in delivery, bioavailability, absorption and metabolism of curcumin: the golden pigment from golden spice. Cancer Res Treat. (2014) 46:2. doi: 10.4143/CRT.2014.46.1.2
23. Jäger R, Lowery RP, Calvanese AV, Joy JM, Purpura M, Wilson JM. Comparative absorption of curcumin formulations. Nutr J. (2014) 13:1–8. doi: 10.1186/1475-2891-13-11
24. Schiborr C, Kocher A, Behnam D, Jandasek J, Toelstede S, Frank J. The oral bioavailability of curcumin from micronized powder and liquid micelles is significantly increased in healthy humans and differs between sexes. Mol Nutr Food Res. (2014) 58:516–27. doi: 10.1002/MNFR.201300724
25. Paolino D, Vero A, Cosco D, Pecora TMG, Cianciolo S, Fresta M, et al. Improvement of oral bioavailability of curcumin upon microencapsulation with methacrylic copolymers. Front Pharmacol. (2016) 0:485. doi: 10.3389/FPHAR.2016.00485
26. Kunnumakkara AB, Bordoloi D, Padmavathi G, Monisha J, Roy NK, Prasad S, et al. Curcumin, the golden nutraceutical: multitargeting for multiple chronic diseases. Br J Pharmacol. (2017) 174:1325–48. doi: 10.1111/BPH.13621
27. Tabrizi R, Vakili S, Lankarani KB, Akbari M, Mirhosseini N, Ghayour-Mobarhan M, et al. The effects of curcumin on glycemic control and lipid profiles among patients with metabolic syndrome and related disorders: a Systematic Review and Metaanalysis of Randomized Controlled Trials. Curr Pharm Des. (2018) 24:3184–99. doi: 10.2174/1381612824666180828162053
28. Azhdari M, Karandish M, Mansoori A. Metabolic benefits of curcumin supplementation in patients with metabolic syndrome: a systematic review and meta-analysis of randomized controlled trials. Phyther Res. (2019) 33:1289–301. doi: 10.1002/PTR.6323
29. Salahshooh MM, Parizadeh SMR, Pasdar A, Saberi Karimian M, Safarian H, Javandoost A, et al. The effect of curcumin (Curcuma longa L) on circulating levels of adiponectin in patients with metabolic syndrome. Comp Clin Path. (2017) 26:17–23. doi: 10.1007/s00580-016-2339-5
30. Chien Y-J, Chang C-Y, Wu M-Y, Chen C-H, Horng Y-S, Wu H-C. Effects of curcumin on glycemic control and lipid profile in polycystic ovary syndrome: Systematic review with meta-analysis and trial sequential analysis. Nutrients. (2021) 13:1–14. doi: 10.3390/NU13020684
31. Zhou H, Beevers CS, Huang S. Targets of curcumin. Curr Drug Targets. (2011) 12:332. doi: 10.2174/138945011794815356
32. Xu X-Y, Meng X, Li S, Ga R-Y, Li Y, Li H-B. Bioactivity, health benefits, and related molecular mechanisms of curcumin: current progress, challenges, and perspectives. Nutrients. (2018) 10:1553. doi: 10.3390/NU10101553
33. Hassan F, Rehman MS, Khan MS, Ali MA, Javed A, Nawaz A, et al. Curcumin as an alternative epigenetic modulator: mechanism of action and potential effects. Front Genet. (2019) 10. doi: 10.3389/FGENE.2019.00514
34. Yun J-M, Jialal I, Devaraj S. Epigenetic regulation of high glucose-induced proinflammatory cytokine productionin monocytes by curcumin. J Nutr Biochem. (2011) 22:450. doi: 10.1016/J.JNUTBIO.2010.03.014
35. Ramzan F, Vickers MH, Mithen RF. Epigenetics, microRNA and metabolic syndrome: a comprehensive review. Int J Mol Sci. (2021) 22:5047. doi: 10.3390/IJMS22095047
36. Agarwai BB, Deb L, Prasad S. Curcumin differs from tetrahydrocurcumin for molecular targets, signaling pathways and cellular responses. Molecules. (2014) 20:185–205. doi: 10.3390/MOLECULES20010185
37. Aggarwal BB. Targeting inflammation-induced obesity and metabolic diseases by curcumin and other nutraceuticals. Annu Rev Nutr. (2010) 30:173–99. doi: 10.1146/ANNUREV.NUTR.012809.104755
38. Shakour N, Cabezas R, Santos J, Barreto G, Jamialahmadi T, Hadizadeh F, et al. Curcumin can bind and interact with CRP: an in silico study. Adv Exp Med Biol. (2021) 1308:91–100. doi: 10.1007/978-3-030-64872-5_7
39. Jurenka JS. Anti-inflammatory properties of curcumin, a major constituent of Curcuma longa: a review of preclinical and clinical research. Altern Med Rev A J Clin Ther. (2009) 14:141–53.
40. Soleimani V, Sahebkar A, Hosseinzadeh H. Turmeric (Curcuma longa) and its major constituent (curcumin) as nontoxic and safe substances: review. Phyther Res. (2018) 32:985–95. doi: 10.1002/PTR.6054
42. Bastard J-P, Maachi M, Lagathu C, Kim MJ, Caron M, Vidal H, Capeau J, Feve B. Recent advances in the relationship between obesity, inflammation, and insulin resistance. Euro Cytokine Netw. (2006) 17:4–12.
43. Hotamisligil GS. Inflammation, metaflammation and immunometabolic disorders. Nat Med. (2017) 542:177–85. doi: 10.1038/nature21363
44. Arruda AP, Pers BM, Parlakgül G, Güney E, Inouye K, Hotamisligil GS. Chronic enrichment of hepatic endoplasmic reticulum–mitochondria contact leads to mitochondrial dysfunction in obesity. Nat Med. (2014) 20:1427–35. doi: 10.1038/nm.3735
45. Montgomery MK, Turner N. Mitochondrial dysfunction and insulin resistance: an update. Endocr Connect. (2015) 4:R1. doi: 10.1530/EC-14-0092
46. Wang L, Zhang B, Huang F, Liu B, Xie Y. Curcumin inhibits lipolysis via suppression of ER stress in adipose tissue and prevents hepatic insulin resistance. J Lipid Res. (2016) 57:1243. doi: 10.1194/JLR.M067397
47. Ye M, Qiu H, Cao Y, Zhang M, Mi Y, Yu J, et al. Curcumin improves palmitate-induced insulin resistance in human umbilical vein endothelial cells by maintaining proteostasis in endoplasmic reticulum. Front Pharmacol. (2017) 8:148. doi: 10.3389/FPHAR.2017.00148
48. Campbell MS, Fleenor BS. The emerging role of curcumin for improving vascular dysfunction: a review. Crit Rev Food Sci Nutr. (2017) 58:2790–9. doi: 10.1080/10408398.2017.1341865
49. Li W, Suwanwela NC, Patumraj S. Curcumin prevents reperfusion injury following ischemic stroke in rats via inhibition of NF-κB, ICAM-1, MMP-9 and caspase-3 expression. Mol Med Rep. (2017) 16:4710–20. doi: 10.3892/MMR.2017.7205
50. Bai X-J, Hao J-T, Wang J, Zhang W-F, Yan C-P, Zhao J-H, et al. Curcumin inhibits cardiac hypertrophy and improves cardiovascular function via enhanced Na +/Ca 2+ exchanger expression after transverse abdominal aortic constriction in rats. Pharmacol Reports. (2018) 70:60–8. doi: 10.1016/J.PHAREP.2017.07.014
51. Chung C-C, Kao Y-H, Liou J-P, Chen Y-J. Curcumin suppress cardiac fibroblasts activities by regulating proliferation, migration, and the extracellular matrix. Acta Cardiol Sin. (2014) 30:474–82.
52. Karuppagounder V, Arumugam S, Giridharan V V, Sreedhar R, Bose RJC, Vanama J, et al. Tiny molecule, big power: multi-target approach for curcumin in diabetic cardiomyopathy. Nutrition. (2017) 34:47–54. doi: 10.1016/J.NUT.2016.09.005
53. Pan SY, Zhou SF, Gao SH, Yu ZL, Zhang SF, Tang MK, et al. New perspectives on how to discover drugs from herbal medicines: CAM'S outstanding contribution to modern therapeutics. Evidence-based complement. Altern Med. (2013) 2013. doi: 10.1155/2013/627375
54. Polson AG, Fuji RN. The successes and limitations of preclinical studies in predicting the pharmacodynamics and safety of cell-surface-targeted biological agents in patients. Br J Pharmacol. (2012) 166:1600. doi: 10.1111/J.1476-5381.2012.01916.X
55. Higgins J, Cartwright ME, Templeton AC. Progressing preclinical drug candidates: strategies on preclinical safety studies and the quest for adequate exposure. Drug Discov Today. (2012) 17:828–36. doi: 10.1016/J.DRUDIS.2012.03.016
56. Lai M, Chandrasekera PC, Barnard ND. You are what you eat, or are you? The challenges of translating high-fat-fed rodents to human obesity and diabetes. Nutr Diabetes. (2014) 4. doi: 10.1038/NUTD.2014.30
57. Wong SK, Chin KY, Suhaimi FH, Fairus A, Ima-Nirwana S. Animal models of metabolic syndrome: a review. Nutr Metab (Lond). (2016) 13:1–12. doi: 10.1186/S12986-016-0123-9
58. Wu L-Y, Chen C-W, Chen L-K, Chou H-Y, Chang C-L, Juan C-C. Curcumin attenuates adipogenesis by inducing preadipocyte apoptosis and inhibiting adipocyte differentiation. Nutrients. (2019) 11. doi: 10.3390/NU11102307
59. Preez R du, Pahl J, Arora M, Kumar MR, Brown L, Panchal SK. Low-dose curcumin nanoparticles normalise lood pressure in male wistar rats with diet-induced metabolic syndrome. Nutrients. (2019) 11. doi: 10.3390/NU11071542
60. Kobori M, Takahashi Y, Takeda H, Takahashi M, Izumi Y, Akimoto Y, et al. Dietary intake of curcumin improves eIF2 signaling and reduces lipid levels in the white adipose tissue of obese mice. Sci Rep. (2018) 8:1–13. doi: 10.1038/s41598-018-27105-w
61. Sadeghi A, Rostamirad A, Seyyedebrahimi S, Meshkani R. Curcumin ameliorates palmitate-induced inflammation in skeletal muscle cells by regulating JNK/NF-kB pathway and ROS production. Inflammopharmacology. (2018) 26:1265–72. doi: 10.1007/S10787-018-0466-0
62. Mohammadi S, Bardei LK, Hojati V, Ghorbani A, Nabiuni M. Anti-inflammatory effects of curcumin on insulin resistance index, levels of interleukin-6, C-Reactive Protein, and liver histology in Polycystic Ovary Syndrome-Induced rats. Cell J. (2017) 19:425–33. doi: 10.22074/CELLJ.2017.4415
63. Kelany ME, Hakami TM, Omar AH. Curcumin improves the metabolic syndrome in high-fructose-diet-fed rats: role of TNF-α, NF-κB, and oxidative stress. Can J Physiol Pharmacol. (2017) 95:140–50. doi: 10.1139/CJPP-2016-0152
64. Bulboaca A, Bolboaca SD, Suci S. Protective effect of curcumin in fructose-induced metabolic syndrome and in streptozotocin-induced diabetes in rats. Iran J Basic Med Sci. (2016) 19:585–93.
65. Hu G-X, Lin H, Lian Q-Q, Zhou S-H, Guo J, Zhou H-Y, Chu Y, Ge R-S. Curcumin as a potent and selective inhibitor of 11β-hydroxysteroid dehydrogenase 1: improving lipid profiles in high-fat-diet-treated rats. PLoS ONE. (2013) 8. doi: 10.1371/JOURNAL.PONE.0049976
66. Kaur G, Meena C. Amelioration of obesity, glucose intolerance, and oxidative stress in high-fat diet and low-dose streptozotocin-induced diabetic rats by combination consisting of “curcumin with piperine and quercetin”. ISRN Pharmacol. (2012) 2012:1–7. doi: 10.5402/2012/957283
67. Feng D, Ohlsson L, Duan R-D. Curcumin inhibits cholesterol uptake in Caco-2 cells by down-regulation of NPC1L1 expression. Lipids Health Dis. (2010) 9:40. doi: 10.1186/1476-511X-9-40
68. Lee ES, Kwon M-H, Kim HM, Woo HB, Ahn CM, Chung CH. Curcumin analog CUR5-8 ameliorates nonalcoholic fatty liver disease in mice with high-fat diet-induced obesity. Metabolism. (2020) 103. doi: 10.1016/J.METABOL.2019.154015
69. Ferguson JJA, Abbott KA, Garg ML. Anti-inflammatory effects of oral supplementation with curcumin: a systematic review and meta-analysis of randomized controlled trials. Nutr Rev. (2021) 79:1043–66. doi: 10.1093/NUTRIT/NUAA114
70. Qin S, Huang L, Gong J, Shen S, Huang J, Tang Y, et al. Meta-analysis of randomized controlled trials of 4 weeks or longer suggest that curcumin may afford some protection against oxidative stress. Nutr Res. (2018) 60:1–12. doi: 10.1016/J.NUTRES.2018.08.003
71. Tabrizi R, Vakili S, Akbari M, Mirhosseini N, Lankarani KB, Rahimi M, et al. The effects of curcumin-containing supplements on biomarkers of inflammation and oxidative stress: a systematic review and meta-analysis of randomized controlled trials. Phytother Res. (2019) 33:253–62. doi: 10.1002/PTR.6226
72. White CM, Pasupuleti V, Roman YM Li Y, Hernandez A V. Oral turmeric/curcumin effects on inflammatory markers in chronic inflammatory diseases: a systematic review and meta-analysis of randomized controlled trials. Pharmacol Res. (2019) 146:104280. doi: 10.1016/j.phrs.2019.104280
73. Gorabi AM, Razi B, Aslani S, Abbasifard M, Imani D, Sathyapalan T, et al. Effect of curcumin on proinflammatory cytokines: a meta-analysis of randomized controlled trials. Cytokine. (2021) 143. doi: 10.1016/J.CYTO.2021.155541
74. Clark CC, Ghaedi E, Arab A, Pourmasoumi M, Hadi A. The effect of curcumin supplementation on circulating adiponectin: a systematic review and meta-analysis of randomized controlled trials. Diabetes Metab Syndr Clin Res Rev. (2019) 13:2819–25. doi: 10.1016/J.DSX.2019.07.045
75. Altobelli E, Angeletti PM, Marziliano C, Mastrodomenico M, Giuliani AR, Petrocelli R. Potential therapeutic effects of curcumin on glycemic and lipid profile in uncomplicated Type 2 Diabetes-A meta-analysis of Randomized Controlled Trial. Nutrients. (2021) 13:1–13. doi: 10.3390/NU13020404
76. Huang J, Qin S, Huang L, Tang Y, Ren H, Hu H. Efficacy and safety of Rhizoma curcumea longae with respect to improving the glucose metabolism of patients at risk for cardiovascular disease: a meta-analysis of randomised controlled trials. J Hum Nutr Diet. (2019) 32:591–606. doi: 10.1111/JHN.12648
77. de Melo ISV, Santos AF, dos Bueno NB. Curcumin or combined curcuminoids are effective in lowering the fasting blood glucose concentrations of individuals with dysglycemia: Systematic review and meta-analysis of randomized controlled trials. Pharmacol Res. (2018) 128:137–44. doi: 10.1016/J.PHRS.2017.09.010
78. Hadi A, Pourmasoumi M, Ghaedi E, Sahebkar A. The effect of Curcumin/Turmeric on blood pressure modulation: a systematic review and meta-analysis. Pharmacol Res. (2019) 150. doi: 10.1016/J.PHRS.2019.104505
79. Simental-Mendía LE, Pirro M, Gotto AM, Banach M, Atkin SL, Majeed M, et al. Lipid-modifying activity of curcuminoids: a systematic review and meta-analysis of randomized controlled trials. Crit Rev Food Sci Nutr. (2019) 59:1178–87. doi: 10.1080/10408398.2017.1396201
80. Sahebkar A. A systematic review and meta-analysis of randomized controlled trials investigating the effects of curcumin on blood lipid levels. Clin Nutr. (2014) 33:406–14. doi: 10.1016/J.CLNU.2013.09.012
81. Qin S, Huang L, Gong J, Shen S, Huang J, Ren H, et al. Efficacy and safety of turmeric and curcumin in lowering blood lipid levels in patients with cardiovascular risk factors: a meta-analysis of randomized controlled trials. Nutr J. (2017) 16. doi: 10.1186/S12937-017-0293-Y
82. Mousavi SM, Milajerdi A, Varkaneh HK, Gorjipour MM, Esmaillzadeh A. The effects of curcumin supplementation on body weight, body mass index and waist circumference: a systematic review and dose-response meta-analysis of randomized controlled trials. Crit Rev Food Sci Nutr. (2020) 60:171–80. doi: 10.1080/10408398.2018.1517724
83. Yuan F, Dong H, Gong J, Wang D, Hu M, Huang W, et al. A systematic review and meta-analysis of Randomized Controlled Trials on the effects of turmeric and curcuminoids on blood lipids in adults with metabolic diseases. Adv Nutr. (2019) 10:791–802. doi: 10.1093/ADVANCES/NMZ021
84. Akbari M, Lankarani KB, Tabrizi R, Ghayour-Mobarhan M, Peymani P, Ferns G, et al. The effects of curcumin on weight loss among patients with metabolic syndrome and related disorders: a systematic review and meta-analysis of Randomized Controlled Trials. Front Pharmacol. (2019) 10:649. doi: 10.3389/FPHAR.2019.00649
85. López-Jaramillo P, Gómez-Arbeláez D, López-López J, López-López C, Martínez-Ortega J, Gómez-Rodríguez A, et al. The role of leptin/adiponectin ratio in metabolic syndrome and diabetes. Horm Mol Biol Clin Investig. (2014) 18:37–45. doi: 10.1515/hmbci-2013-0053
86. A O. Aerobic exercise and nano-curcumin supplementation improve inflammation in elderly females with metabolic syndrome. Diabetol Metab Syndr. (2020) 12. doi: 10.1186/S13098-020-00532-4
87. Lee YK, Lee WS, Hwang JT, Kwon DY, Surh YJ, Park OJ. Curcumin exerts antidifferentiation effect through AMPKalpha-PPAR-gamma in 3T3-L1 adipocytes and antiproliferatory effect through AMPKalpha-COX-2 in cancer cells. J Agric Food Chem. (2009) 57:305–10. doi: 10.1021/JF802737Z
88. Di Pierro F, Bressan A, Ranaldi D, Rapacioli G, Giacomelli L, Bertuccioli A. Potential role of bioavailable curcumin in weight loss and omental adipose tissue decrease: preliminary data of a randomized, controlled trial in overweight people with metabolic syndrome. Preliminary study. Eur Rev Med Pharmacol Sci. (2015) 19:4195–202.
89. Zaccardi F, Webb DR, Yates T, Davies MJ. Pathophysiology of type 1 and type 2 diabetes mellitus: a 90-year perspective. Postgrad Med J. (2016) 92:63–9. doi: 10.1136/POSTGRADMEDJ-2015-133281
90. Chuengsamarn S, Rattanamongkolgul S, Luechapudiporn R, Phisalaphong C, Jirawatnotai S. Curcumin extract for prevention of type 2 diabetes. Diabetes Care. (2012) 35:2121–7. doi: 10.2337/DC12-0116
91. Hodaei H, Adibian M, Nikpayam O, Hedayati M, Sohrab G. The effect of curcumin supplementation on anthropometric indices, insulin resistance and oxidative stress in patients with type 2 diabetes: a randomized, double-blind clinical trial. Diabetol Metab Syndr. (2019) 11. doi: 10.1186/S13098-019-0437-7
92. Cicero AF, Colletti A. Combinations of phytomedicines with different lipid lowering activity for dyslipidemia management: the available clinical data. Phytomedicine. (2016) 23:1113–8. doi: 10.1016/J.PHYMED.2015.10.011
93. Rinaldi L, Pafundi PC, Galiero R, Caturano A, Morone MV, Silvestri C, et al. Mechanisms of non-alcoholic fatty liver disease in the metabolic syndrome. A narrative review. Antioxidants. (2021) 10:1–25. doi: 10.3390/ANTIOX10020270
94. Saberi-Karimian M, Keshvari M, Ghayour-Mobarhan M, Salehizadeh L, Rahmani S, Behnam B, et al. Effects of curcuminoids on inflammatory status in patients with non-alcoholic fatty liver disease: A randomized controlled trial. Complement Ther Med. (2020) 49. doi: 10.1016/J.CTIM.2020.102322
95. Cicero AF, Sahebkar A, Fogacci F, Bove M, Giovannini M, Borghi C. Effects of phytosomal curcumin on anthropometric parameters, insulin resistance, cortisolemia and non-alcoholic fatty liver disease indices: a double-blind, placebo-controlled clinical trial. Eur J Nutr. (2020) 59:477–83. doi: 10.1007/S00394-019-01916-7
96. Moradi B, Rahmati-Ahmadabad S, Farzanegi P, Helalizadeh M, Azarbayjani MA. Effects of non-linear resistance training and curcumin supplementation on the liver biochemical markers levels and structure in older women with non-alcoholic fatty liver disease. J Bodyw Mov Ther. (2020) 24:154–60. doi: 10.1016/J.JBMT.2020.02.021
97. Mirhafez SR, Azimi-Nezhad M, Dehabeh M, Hariri M, Naderan RD, Movahedi A, et al. The effect of curcumin phytosome on the treatment of patients with non-alcoholic fatty liver disease: a Double-Blind, Randomized, Placebo-Controlled Trial. Adv Exp Med Biol. (2021) 1308:25–35. doi: 10.1007/978-3-030-64872-5_3
98. Cicero AFG, Colletti A, Bellentani S. Nutraceutical approach to Non-Alcoholic Fatty Liver Disease (NAFLD): the available clinical evidence. Nutrients. (2018) 10. doi: 10.3390/NU10091153
99. Saadati S, Sadeghi A, Mansour A, Yari Z, Poustchi H, Hedayati M, et al. Curcumin and inflammation in non-alcoholic fatty liver disease: a randomized, placebo controlled clinical trial. BMC Gastroenterol. (2019) 19. doi: 10.1186/S12876-019-1055-4
100. Mirhafez SR, Farimani AR, Dehhabe M, Bidkhori M, Hariri M, Motlagh Ghouchani BFN, et al. Effect of phytosomal curcumin on circulating levels of adiponectin and leptin in patients with Non-Alcoholic Fatty Liver Disease: A randomized, double-blind, placebo-controlled clinical trial. J Gastrointestin Liver Dis. (2019) 28:183–9. doi: 10.15403/JGLD-179
101. Saadati S, Hatami B, Yari Z, Shahrbaf MA, Eghtesad S, Mansour A, et al. The effects of curcumin supplementation on liver enzymes, lipid profile, glucose homeostasis, and hepatic steatosis and fibrosis in patients with non-alcoholic fatty liver disease. Eur J Clin Nutr. (2019) 73:441–9. doi: 10.1038/S41430-018-0382-9
102. Panahi Y, Kianpour P, Mohtashami R, Jafari R, Simental-Mendía LE, Sahebkar A. Efficacy and safety of phytosomal curcumin in Non-Alcoholic Fatty Liver Disease: A Randomized Controlled Trial. Drug Res (Stuttg). (2017) 67:244–51. doi: 10.1055/S-0043-100019
103. Jazayeri-Tehrani SA, Rezayat SM, Mansouri S, Qorbani M, Alavian SM, Daneshi-Maskooni M, et al. Efficacy of nanocurcumin supplementation on insulin resistance, lipids, inflammatory factors and nesfatin among obese patients with non-alcoholic fatty liver disease (NAFLD): a trial protocol. BMJ Open. (2017) 7. doi: 10.1136/BMJOPEN-2017-016914
104. Panahi Y, Kianpour P, Mohtashami R, Jafari R, Simental-Mendiá LE, Sahebkar A. Curcumin lowers serum lipids and uric acid in subjects with Nonalcoholic Fatty Liver Disease: a Randomized Controlled Trial. J Cardiovasc Pharmacol. (2016) 68:223–9. doi: 10.1097/FJC.0000000000000406
105. Selmanovic S, Beganlic A, Salihefendic N, Ljuca F, Softic A, Smajic E. Therapeutic effects of curcumin on ultrasonic morphological characteristics of liver in patients with metabolic syndrome. Acta Inform Medica. (2017) 25:169–74. doi: 10.5455/AIM.2017.25.169-174
106. Chashmniam S, Mirhafez SR, Dehabeh M, Hariri M, Azimi Nezhad M, Nobakht M, et al. A pilot study of the effect of phospholipid curcumin on serum metabolomic profile in patients with non-alcoholic fatty liver disease: a randomized, double-blind, placebo-controlled trial. Eur J Clin Nutr. (2019) 73:1224–35. doi: 10.1038/S41430-018-0386-5
107. Jarhahzadeh M, Alavinejad P, Farsi F, Husain D, Rezazadeh A. The effect of turmeric on lipid profile, malondialdehyde, liver echogenicity and enzymes among patients with nonalcoholic fatty liver disease: a randomized double blind clinical trial. Diabetol Metab Syndr. (2021) 13:1–9. doi: 10.1186/S13098-021-00731-7/TABLES/4
108. Rahmani S, Asgary S, Askari G, Keshvari M, Hatamipour M, Feizi A, et al. Treatment of non-alcoholic fatty liver disease with curcumin: a randomized placebo-controlled trial. Phytother Res. (2016) 30:1540–48. doi: 10.1002/PTR.5659
109. Baziar N, Parohan M. The effects of curcumin supplementation on body mass index, body weight, and waist circumference in patients with nonalcoholic fatty liver disease: a systematic review and dose-response meta-analysis of randomized controlled trials. Phytother Res. (2020) 34:464–74. doi: 10.1002/PTR.6542
110. Mirhafez SR, Rezai A, Dehabeh M, Nobakht M, Gh BF, Bidkhori M, et al. Efficacy of phytosomal curcumin among patients with non-alcoholic fatty liver disease. Int J Vitam Nutr Res. (2021) 91:278–86. doi: 10.1024/0300-9831/A000629
111. Hariri M, Gholami A, Mirhafez SR, Bidkhori M, Sahebkar A. A pilot study of the effect of curcumin on epigenetic changes and DNA damage among patients with non-alcoholic fatty liver disease: a randomized, double-blind, placebo-controlled, clinical trial. Complement Ther Med. (2020) 51. doi: 10.1016/J.CTIM.2020.102447
112. Rózański G, Kujawski S, Newton JL, Zalewski P, Słomko J. Curcumin and biochemical parameters in Metabolic-Associated Fatty Liver Disease (MAFLD)-A Review. Nutrients. (2021) 13. doi: 10.3390/NU13082654
113. Jalali M, Mahmoodi M, Mosallanezhad Z, Jalali R, Imanieh MH, Moosavian SP. The effects of curcumin supplementation on liver function, metabolic profile and body composition in patients with non-alcoholic fatty liver disease: a systematic review and meta-analysis of randomized controlled trials. Complement Ther Med. (2020) 48. doi: 10.1016/J.CTIM.2019.102283
114. Cerletti C, Colucci M, Storto M, Semeraro F, Ammollo CT, Incampo F, et al. Randomised trial of chronic supplementation with a nutraceutical mixture in subjects with non-alcoholic fatty liver disease. Br J Nutr. (2020) 123:190–7. doi: 10.1017/S0007114519002484
115. Gheibi S, Gouvarchin Ghaleh HE, Motlagh BM, Azarbayjani AF, zarei L. Therapeutic effects of curcumin and ursodexycholic acid on non-alcoholic fatty liver disease. Biomed Pharmacother. (2019) 115. doi: 10.1016/J.BIOPHA.2019.108938
116. Mirhafez SR, Dehabeh M, Hariri M, Farimani AR, Movahedi A, Naderan RD, et al. Curcumin and piperine combination for the treatment of patients with Non-Alcoholic Fatty Liver Disease: a double-blind randomized placebo-controlled trial. Adv Exp Med Biol. (2021) 1328:11–9. doi: 10.1007/978-3-030-73234-9_2
117. Panahi Y, Valizadegan G, Ahamdi N, Ganjali S, Majeed M, Sahebkar A. Curcuminoids plus piperine improve nonalcoholic fatty liver disease: a clinical trial. J Cell Biochem. (2019) 120:15989–96. doi: 10.1002/JCB.28877
118. Saberi-Karimin M, Ghazizadeh H, Mohammadzadeh E, Ferns GA, Ghayour-Mobarhan M, Sahebkar A. Does curcumin have an effect on sleep duration in metabolic syndrome patients? Avicenna J Phytomed. (2021) 11:190–8.
119. Olechnowicz J, Tinkov A, Skalny A, Suliburska J. Zinc status is associated with inflammation, oxidative stress, lipid, and glucose metabolism. J Physiol Sci. (2017) 68:19–31. doi: 10.1007/S12576-017-0571-7
120. Lu C-W, Lee Y-C, Kuo C-S, Chiang C-H, Chang H-H, Huang K-C. Association of serum levels of zinc, copper, and iron with risk of metabolic syndrome. Nutrients. (2021) 13:1–9. doi: 10.3390/NU13020548
121. Kalmar B, Greensmith L. Induction of heat shock proteins for protection against oxidative stress. Adv Drug Deliv Rev. (2009) 61:310–8. doi: 10.1016/J.ADDR.2009.02.003
122. Armutcu F, Ataymen M, Atmaca H, Gurel A. Oxidative stress markers, C-reactive protein and heat shock protein 70 levels in subjects with metabolic syndrome. Clin Chem Lab Med. (2008) 46:785–90. doi: 10.1515/CCLM.2008.166
123. Safarian H, Parizadeh SMR, Saberi-Karimain M, Darroudi S, Javandoost A, Mohammadi F, et al. The effect of curcumin on serum copper and zinc and zn/cu ratio in individuals with metabolic syndrome: a double-blind clinical trial. (2018) 16:625–634. doi: 10.1080/19390211.2018.1472711
124. Mohammadi F, Ghazi-Moradi M, Ghayour-Mobarhan M, Esmaeili H, Moohebati M, Saberi-Karimian M, et al. The effects of curcumin on serum heat shock protein 27 antibody titers in patients with metabolic syndrome. J Diet Suppl. (2019) 16:592–601. doi: 10.1080/19390211.2018.1472710
125. Alamdari DH, Ghayour-Mobarhan M, Tavallaie S, Parizadeh MR, Moohebati M, Ghafoori F, et al. Prooxidant-antioxidant balance as a new risk factor in patients with angiographically defined coronary artery disease. Clin Biochem. (2008) 41:375–80. doi: 10.1016/J.CLINBIOCHEM.2007.12.008
126. Ghazimoradi M, Saberi-Karimian M, Mohammadi F, Sahebkar A, Tavallaie S, Safarian H, et al. The effects of curcumin and curcumin-phospholipid complex on the serum pro-oxidant-antioxidant balance in subjects with metabolic syndrome. Phyther Res. (2017) 31:1715–21. doi: 10.1002/PTR.5899
127. Mohammadi A, Sadeghnia HR, Saberi-Karimian M, Safarian H, Ferns GA, Ghayour-Mobarhan M, et al. Effects of curcumin on serum vitamin E concentrations in individuals with metabolic syndrome. Phyther Res. (2017) 31:657–62. doi: 10.1002/PTR.5779
128. Taghipour YD, Hajialyani M, Naseri R, Hesari M, Mohammadi P, Stefanucci A, et al. Nanoformulations of natural products for management of metabolic syndrome. Int J Nanomedicine. (2019) 14:5303–21. doi: 10.2147/IJN.S213831
129. Bateni Z, Rahimi HR, Hedayati M, Afsharian S, Goudarzi R, Sohrab G. The effects of nano-curcumin supplementation on glycemic control, blood pressure, lipid profile, and insulin resistance in patients with the metabolic syndrome: A randomized, double-blind clinical trial. Phyther Res. (2021) 35:3945–53. doi: 10.1002/PTR.7109
130. Burgos-Morón E, Calderón-Montaño JM, Salvador J, Robles A, López-Lázaro M. The dark side of curcumin. Int J Cancer. (2010) 126:1771–5. doi: 10.1002/IJC.24967
131. Medicines Agency E. European Union herbal monograph on Curcuma longa L., rhizoma Final Initial assessment Discussion in Working Party on European Union monographs and European Union list (MLWP). London: European Medicines Agency. (2018)
132. Panahi Y, Khalili N, Hosseini MS, Abbasinazari M, Sahebkar A. Lipid-modifying effects of adjunctive therapy with curcuminoids-piperine combination in patients with metabolic syndrome: results of a randomized controlled trial. Complement Ther Med. (2014) 22:851–7. doi: 10.1016/J.CTIM.2014.07.006
133. (ANS) EP on FA and NS added to F. Scientific opinion on the re-evaluation of curcumin (E 100) as a food additive. EFSA J. (2010) 8. doi: 10.2903/J.EFSA.2010.1679
134. Basnet P, Skalko-Basnet N. Curcumin: an anti-inflammatory molecule from a curry spice on the path to cancer treatment. Molecules. (2011) 16:4567–98. doi: 10.3390/molecules16064567
135. Stati G, Rossi F, Sancilio S, Basile M, Di Pietro R. Curcuma longa hepatotoxicity: a baseless accusation. Cases assessed for causality using RUCAM method. Front Pharmacol. (2021) 12:3048. doi: 10.3389/FPHAR.2021.780330/BIBTEX
136. Sohal A, Alhankawi D, Sandhu S, Chintanaboina J. Turmeric-induced hepatotoxicity: report of 2 cases. Int Med Case Rep J. (2021) 14:849–52. doi: 10.2147/IMCRJ.S333342
137. Luber RP, Rentsch C, Lontos S, Pope JD, Aung AK, Schneider HG, et al. Turmeric induced liver injury: a report of two cases. Case Reports Hepatol. (2019) 2019:1–4. doi: 10.1155/2019/6741213
138. clinicaltrials.gov. Available online at: https://clinicaltrials.gov/ct2/results?term=curcumin&cond=Metabolic+Syndrome&age_v=&gndr=&type=Intr&rslt=&Search=Apply
139. Vogenberg FR, Barash CI, Pursel M. Personalized medicine: Part 1: evolution and development into theranostics. Pharm Ther. (2010) 35:560.
140. Redekop WK, Mladsi D. The faces of personalized medicine: a framework for understanding its meaning and scope. Value Health. (2013) 16:S4. doi: 10.1016/J.JVAL.2013.06.005
141. Holmes E, Wilson ID, Nicholson JK. Metabolic phenotyping in health and disease. Cell. (2008) 134:714–7. doi: 10.1016/J.CELL.2008.08.026
142. Nicholson JK, Lindon JC. Systems biology: metabonomics. Nature. (2008) 455:1054–6. doi: 10.1038/4551054A
143. Antcliffe D, Gordon AC. Metabonomics and intensive care. Crit Care. (2016) 20: doi: 10.1186/S13054-016-1222-8
144. Shim K, Gulhar R, Jialal I. Exploratory metabolomics of nascent metabolic syndrome. J Diabetes Complications. (2019) 33:212–6. doi: 10.1016/J.JDIACOMP.2018.12.002
145. Lent-Schochet D, McLaughlin M, Ramakrishnan N, Jialal I. Exploratory metabolomics of metabolic syndrome: a status report. World J Diabetes. (2019) 10:23. doi: 10.4239/WJD.V10.I1.23
146. Monnerie S, Comte B, Ziegler D, Morais JA, Pujos-Guillot E, Gaudreau P. Metabolomic and lipidomic signatures of metabolic syndrome and its physiological components in adults: a systematic review. Sci Rep. (2020) 10:1–13. doi: 10.1038/s41598-019-56909-7
147. Pujos-Guillot E, Brandolini M, Pétéra M, Grissa D, Joly C, Lyan B, et al. Systems metabolomics for prediction of metabolic syndrome. J Proteome Res. (2017) 16:2262–72. doi: 10.1021/ACS.JPROTEOME.7B00116
148. Khoomrung S, Wanichthanarak K, Nookaew I, Thamsermsang O, Seubnooch P, Laohapand T, et al. Metabolomics and integrative omics for the development of Thai traditional medicine. Front Pharmacol. (2017) 8:474. doi: 10.3389/FPHAR.2017.00474/BIBTEX
149. Yang M, Lao L. Emerging applications of metabolomics in traditional chinese medicine treating hypertension: biomarkers, pathways and more. Front Pharmacol. (2019) 10:158. doi: 10.3389/FPHAR.2019.00158
150. Neavin D, Kaddurah-Daouk R, Weinshilboum R. Pharmacometabolomics informs pharmacogenomics. Metabolomics. (2016) 12. doi: 10.1007/S11306-016-1066-X
151. Pandey A, Chaturvedi M, Mishra S, Kumar P, Somvanshi P, Chaturvedi R. Reductive metabolites of curcumin and their therapeutic effects. Heliyon. (2020) 6:e05469. doi: 10.1016/J.HELIYON.2020.E05469
152. Holder GM, Plummer JL, Ryan AJ. The metabolism and excretion of curcumin (1,7-Bis-(4-hydroxy-3-methoxyphenyl)-1,6-heptadiene-3,5-dione) in the rat. (2008) 8:761–8. doi: 10.3109/00498257809069589
153. Anand P, Kunnumakkara AB, Newman RA, Aggarwal BB. Bioavailability of curcumin: problems and promises. Mol Pharm. (2007) 4:807–18. doi: 10.1021/MP700113R
154. Goel A, Kunnumakkara AB, Aggarwal BB. Curcumin as “Curecumin”: from kitchen to clinic. Biochem Pharmacol. (2008) 75:787–809. doi: 10.1016/J.BCP.2007.08.016
155. Wang J, Yu X, Zhang L, Wang L, Peng Z, Chen Y. The pharmacokinetics and tissue distribution of curcumin and its metabolites in mice. Biomed Chromatogr. (2018) 32: doi: 10.1002/BMC.4267
156. Pan M-H, Huang T-M, Lin J-K. Biotransformation of curcumin through reduction and glucuronidation in mice. Drug Metab Dispos. (1999) 27.
157. Asai A, Miyazawa T. Occurrence of orally administered curcuminoid as glucuronide and glucuronide/sulfate conjugates in rat plasma. Life Sci. (2000) 67:2785–93. doi: 10.1016/S0024-3205(00)00868-7
158. Hassaninasab A, Hashimoto Y, Tomita-Yokotani K, Kobayashi M. Discovery of the curcumin metabolic pathway involving a unique enzyme in an intestinal microorganism. Proc Natl Acad Sci U S A. (2011) 108:6615–20. doi: 10.1073/PNAS.1016217108/-/DCSUPPLEMENTAL
159. Dileep K, Tintu I, Sadasivan C. Molecular docking studies of curcumin analogs with phospholipase A2. Interdiscip Sci. (2011) 3:189–97. doi: 10.1007/S12539-011-0090-9
160. Yu Q, Liu Y, Wu Y, Chen Y. Dihydrocurcumin ameliorates the lipid accumulation, oxidative stress and insulin resistance in oleic acid-induced L02 and HepG2 cells. Biomed Pharmacother. (2018) 103:1327–36. doi: 10.1016/J.BIOPHA.2018.04.143
161. Yoysungnoen B, Bhattarakosol P, Changtam C, Patumraj S. Effects of tetrahydrocurcumin on tumor growth and cellular signaling in cervical cancer xenografts in nude mice. Biomed Res Int. (2016) 2016. doi: 10.1155/2016/1781208
162. Bäckhed F, Ding H, Wang T, Hooper L V, Koh GY, Nagy A, et al. The gut microbiota as an environmental factor that regulates fat storage. Proc Natl Acad Sci. (2004) 101:15718–23. doi: 10.1073/PNAS.0407076101
163. Delzenne N, Neyrinck A, Bäckhed F, Cani P. Targeting gut microbiota in obesity: effects of prebiotics and probiotics. Nat Rev Endocrinol. (2011) 7:639–46. doi: 10.1038/NRENDO.2011.126
164. Round JL, Mazmanian SK. The gut microbiota shapes intestinal immune responses during health and disease. Nat Rev Immunol. (2009) 9:313–23. doi: 10.1038/NRI2515
165. Valikhani D, Bolivar JM, Pelletier JN. An overview of cytochrome P450 immobilization strategies for drug metabolism studies, biosensing, and biocatalytic applications: challenges and opportunities. ACS Catal. (2021) 11:9418–34. doi: 10.1021/ACSCATAL.1C02017
166. Zanger UM, Schwab M. Cytochrome P450 enzymes in drug metabolism: regulation of gene expression, enzyme activities, and impact of genetic variation. Pharmacol Ther. (2013) 138:103–41. doi: 10.1016/J.PHARMTHERA.2012.12.007
167. Manikandan P, Nagini S. Cytochrome P450 structure, function and clinical significance: a Review. Curr Drug Targets. (2018) 19. doi: 10.2174/1389450118666170125144557
168. Hakkola J, Hukkanen J, Turpeinen M, Pelkonen O. Inhibition and induction of CYP enzymes in humans: an update. Arch Toxicol. (2020) 94:3671–722. doi: 10.1007/S00204-020-02936-7
169. Ch Yiannakopoulou E. Pharmacogenomics of phase II metabolizing enzymes and drug transporters: clinical implications. Pharmacogenomics J. (2012) 13:105–9. doi: 10.1038/tpj.2012.42
170. Rosengren R, Chen S. Modulation of hepatic drug metabolizing enzymes by the natural polyphenol curcumin. Intern Med Rev. (2017) 3. doi: 10.18103/IMR.V3I11.631
171. Al-Jenoobi FI, Al-Thukair AA, Alam MA, Abbas FA, Al-Mohizea AM, Alkharfy KM, et al. Effect of Curcuma longa on CYP2D6- and CYP3A4-mediated metabolism of dextromethorphan in human liver microsomes and healthy human subjects. Eur J Drug Metab Pharmacokinet. (2015) 40:61–6. doi: 10.1007/S13318-014-0180-2
172. Shamsi S, Tran H, Tan RSJ, Tan ZJ, Lim LY. Curcumin, piperine, and capsaicin: a comparative study of spice-mediated inhibition of human cytochrome P450 isozyme activities. Drug Metab Dispos. (2017) 45:49–55. doi: 10.1124/DMD.116.073213
173. Hsieh YW, Huang CY, Yang SY, Peng YH Yu CP, Chao PDL, Hou YC. Oral intake of curcumin markedly activated CYP 3A4: in vivo and ex-vivo studies. Sci Rep. (2014) 4:1–7. doi: 10.1038/srep06587
174. Mach CM, Chen JH, Mosley SA, Kurzrock R, Smith JA. Evaluation of liposomal curcumin cytochrome P450 metabolism. Anticancer Res. (2010) 30:811–4.
175. Chen Y, Liu WH, Chen BL, Fan L, Han Y, Wang G, et al. Plant polyphenol curcumin significantly affects CYP1A2 and CYP2A6 activity in healthy, male Chinese volunteers. Ann Pharmacother. (2010) 44:1038–45. doi: 10.1345/APH.1M533
176. Volak LP, Ghirmai S, Cashman JR, Court MH. Curcuminoids inhibit multiple human cytochromes P450, UDP-glucuronosyltransferase, and sulfotransferase enzymes, whereas piperine is a relatively selective CYP3A4 inhibitor. Drug Metab Dispos. (2008) 36:1594–605. doi: 10.1124/DMD.108.020552
177. Vietri M, Pietrabissa A, Mosca F, Spisni R, Pacifici GM. Curcumin is a potent inhibitor of phenol sulfotransferase (SULT1A1) in human liver and extrahepatic tissues. Xenobiotica. (2003) 33:357–63. doi: 10.1080/0049825031000065197
178. Volak LP, Hanley MJ, Masse G, Hazarika S, Harmatz JS, Badmaev V, et al. Effect of a herbal extract containing curcumin and piperine on midazolam, flurbiprofen and paracetamol (acetaminophen) pharmacokinetics in healthy volunteers. Br J Clin Pharmacol. (2013) 75:450–62. doi: 10.1111/J.1365-2125.2012.04364.X
179. Liu Y, Wu YM Yu Y, Cao CS, Zhang JH Li K, Zhang PY. Curcumin and resveratrol in combination modulate drug-metabolizing enzymes as well as antioxidant indices during lung carcinogenesis in mice. Hum Exp Toxicol. (2015) 34:620–7. doi: 10.1177/0960327114551396
180. Valentine SP, Le Nedelec MJ, Menzies AR, Scandlyn MJ, Goodin MG, Rosengren RJ. Curcumin modulates drug metabolizing enzymes in the female Swiss Webster mouse. Life Sci. (2006) 78:2391–8. doi: 10.1016/J.LFS.2005.09.017
181. Hills RD, Pontefract BA, Mishcon HR, Black CA, Sutton SC, Theberge CR. Gut Microbiome: profound implications for diet and disease. Nutrients. (2019) 11: doi: 10.3390/NU11071613
182. Hildebrandt MA, Hoffman C, Sherrill-Mix SA, Keilbaugh SA, Hamady M, Chen YY, et al. High-fat diet determines the composition of the murine gut microbiome independently of obesity. Gastroenterology. (2009) 137. doi: 10.1053/J.GASTRO.2009.08.042
183. Ley RE, Bäckhed F, Turnbaugh P, Lozupone CA, Knight RD, Gordon JI. Obesity alters gut microbial ecology. Proc Natl Acad Sci U S A. (2005) 102:11070–5. doi: 10.1073/PNAS.0504978102
184. Wu GD, Chen J, Hoffmann C, Bittinger K, Chen Y-Y, Keilbaugh SA, et al. Linking long-term dietary patterns with gut microbial enterotypes. Science. (2011) 334:105. doi: 10.1126/SCIENCE.1208344
185. Bindels LB, Delzenne NM, Cani PD, Walter J. Towards a more comprehensive concept for prebiotics. Nat Rev Gastroenterol Hepatol. (2015) 12:303–10. doi: 10.1038/NRGASTRO.2015.47
186. Cunningham M, Azcarate-Peril MA, Barnard A, Benoit V, Grimaldi R, Guyonnet D, et al. Shaping the future of probiotics and prebiotics. Trends Microbiol. (2021) 29:667–85. doi: 10.1016/J.TIM.2021.01.003
187. Everard A, Belzer C, Geurts L, Ouwerkerk JP, Druart C, Bindels LB, et al. Cross-talk between Akkermansia muciniphila and intestinal epithelium controls diet-induced obesity. Proc Natl Acad Sci. (2013) 110:9066–71. doi: 10.1073/PNAS.1219451110
188. Plovier H, Everard A, Druart C, Depommier C, Van Hul M, Geurts L, et al. A purified membrane protein from Akkermansia muciniphila or the pasteurized bacterium improves metabolism in obese and diabetic mice. Nat Med. (2016) 23:107–13. doi: 10.1038/nm.4236
189. Cani PD, Everard A, Duparc T. Gut microbiota, enteroendocrine functions and metabolism. Curr Opin Pharmacol. (2013) 13:935–40. doi: 10.1016/J.COPH.2013.09.008
190. Ghosh SS, Bie J, Wang J, Ghosh S. Oral supplementation with non-absorbable antibiotics or curcumin attenuates western diet-induced atherosclerosis and glucose intolerance in LDLR-/- mice–role of intestinal permeability and macrophage activation. PLoS ONE. (2014) 9. doi: 10.1371/JOURNAL.PONE.0108577
191. Anhê FF, Varin T V, Barz M, Le Desjardins Y, Levy E, Roy D, et al. Gut microbiota dysbiosis in obesity-linked metabolic diseases and prebiotic potential of polyphenol-rich extracts. Curr Obes Rep. (2015) 4:389–400. doi: 10.1007/S13679-015-0172-9
192. Peterson CT, Vaughn AR, Sharma V, Chopra D, Mills PJ, Peterson SN, et al. Effects of turmeric and curcumin dietary supplementation on human gut microbiota: a double-blind, randomized, placebo-controlled pilot study. J Evidence-Based Integr Med. (2018) 23:1–8. doi: 10.1177/2515690X18790725
193. Silberberg M, Morand C, Mathevon T, Besson C, Manach C, Scalbert A, et al. The bioavailability of polyphenols is highly governed by the capacity of the intestine and of the liver to secrete conjugated metabolites. Eur J Nutr. (2006) 45:88–96. doi: 10.1007/S00394-005-0568-5
194. Mahjoub S, Masrour-Roudsari J. Role of oxidative stress in pathogenesis of metabolic syndrome. Casp J Intern Med. (2012) 3:386.
195. Yubero-Serrano EM, Delgado-Lista J, Peña-Orihuela P, Perez-Martinez P, Fuentes F, Marin C, et al. Oxidative stress is associated with the number of components of metabolic syndrome: LIPGENE study. Exp Mol Med. (2013) 45:e28–e28. doi: 10.1038/emm.2013.53
Keywords: metabolic syndrome, curcumin, curcuminoid, turmeric, personalized treatment, gut microbiome, meta-analysis, randomized controlled trial
Citation: Nurcahyanti ADR, Cokro F, Wulanjati MP, Mahmoud MF, Wink M and Sobeh M (2022) Curcuminoids for Metabolic Syndrome: Meta-Analysis Evidences Toward Personalized Prevention and Treatment Management. Front. Nutr. 9:891339. doi: 10.3389/fnut.2022.891339
Received: 07 March 2022; Accepted: 21 April 2022;
Published: 09 June 2022.
Edited by:
Annalisa Terranegra, Sidra Medicine, QatarReviewed by:
Azita Hekmatdoost, National Nutrition and Food Technology Research Institute, IranRoberta Masella, National Institute of Health (ISS), Italy
Tannaz Jamialahamdi, Mashhad University of Medical Sciences, Iran
Copyright © 2022 Nurcahyanti, Cokro, Wulanjati, Mahmoud, Wink and Sobeh. This is an open-access article distributed under the terms of the Creative Commons Attribution License (CC BY). The use, distribution or reproduction in other forums is permitted, provided the original author(s) and the copyright owner(s) are credited and that the original publication in this journal is cited, in accordance with accepted academic practice. No use, distribution or reproduction is permitted which does not comply with these terms.
*Correspondence: Mansour Sobeh, mansour.sobeh@um6p.ma
Agustina Dwi Retno Nurcahyanti, adr.nurcahyanti@atmajaya.ac.id