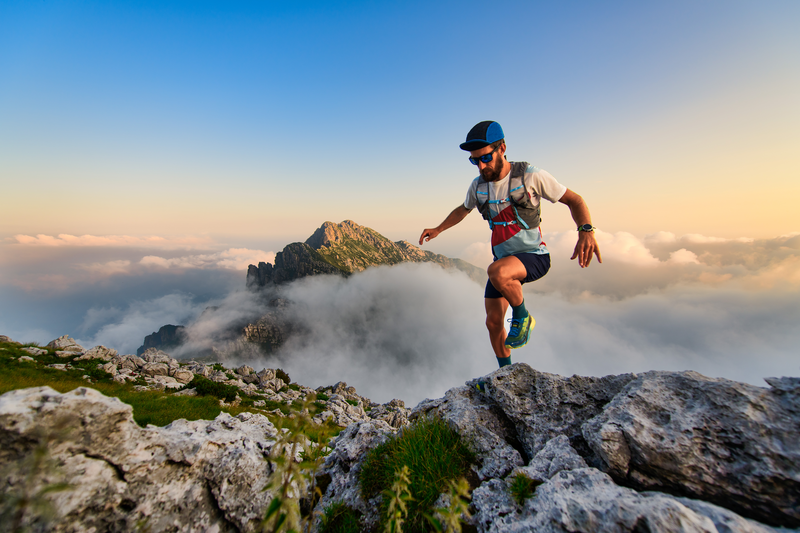
94% of researchers rate our articles as excellent or good
Learn more about the work of our research integrity team to safeguard the quality of each article we publish.
Find out more
REVIEW article
Front. Nutr. , 25 May 2022
Sec. Nutrition and Food Science Technology
Volume 9 - 2022 | https://doi.org/10.3389/fnut.2022.890066
This article is part of the Research Topic Advances in Alcoholic Beverages Technology View all 4 articles
There is ample evidence regarding the health benefits of red wine consumption due to its content of phenolic compounds, as an alternative to improve the state of health and prevent various diseases, being the implementation of procedures that allow a greater extraction and stability of phenolic compounds during the elaboration a key aspect. The first part of this review summarizes some studies, mostly at the preclinical level, on the mechanisms by which phenolic compounds act in the human organism, taking advantage of their antioxidant, anti-inflammatory, antitumor, antithrombotic, antiatherogenic, antimicrobial, antiviral, and other activities. Although the migration of grape components into the must/wine occurs during the winemaking process, the application of new technologies may contribute to increasing the content of phenolic compounds in the finished wine. Some of these technologies have been evaluated on an industrial scale, and in some cases, they have been included in the International Code of Oenological Practice by the International Organization of Vine and Wine (OIV). In this sense, the second part of this review deals with the use of these novel technologies that can increase, or at least maintain, the polyphenol content. For example, in the pre-fermentative stage, phenolic extraction can be increased by treating the berries or must with high pressures, pulsed electric fields (PEF), ultrasound (US), e-beam radiation or ozone. At fermentative level, yeasts with high production of pyranoanthocyanins and/or their precursor molecules, low polyphenol absorption, and low anthocyanin-β-glucosidase activity can be used. Whereas, at the post-fermentative level, aging-on-lees (AOL) can contribute to maintaining polyphenol levels, and therefore transmitting health benefits to the consumer.
In recent years, the consumption of foods rich in bioactive compounds, whose intake has been related to protection against certain diseases, has gained relevance. Red wine is one of the foods that has aroused greater interest, and around which several studies have been developed to elucidate the mechanisms of action and its potential benefit to the consumer’s health. Most of the benefits are attributable to phenolic compounds, which are generally classified into flavonoids (anthocyanins, flavanols, flavonols, among others) and non-flavonoids (stilbenes, phenolic acids, among others) (1, 2). These compounds are attributed with antioxidant, anti-inflammatory, antitumor, antithrombotic, antiatherogenic, antimicrobial, and antiviral activity, among other benefits (1–4).
Antioxidant and anti-inflammatory activities are the basis of most of the mechanisms of action involved in the protection of phenolic compounds. Antioxidant activity, observed, for example, in non-alcoholic red wine, by increasing the activity of the catalase, superoxide dismutase (SOD), and glutathione reductase (GR) enzymes (5), or increasing the production of nitric oxide (NO), with a consequent lower cardiovascular risk (6), is particularly interesting.
Quantitatively, the most abundant polyphenols are anthocyanins, which are mainly located in the grape skin and account for 50–60% of the phenolic fraction (7, 8) and flavanols, which are mainly found in the seeds and stalk (8).
Another group of interest, from a qualitative point of view, are the stilbenes, mainly resveratrol (trans-3,4′,5-trihydroxystilbene) and its glycoside, polydatin (9), to which a large part of the protective effects against various diseases are attributed (4, 6, 8, 9).
Phenolic compounds may prevent oxidative damage caused by free radicals that may cause damage to proteins, carbohydrates, lipids, or nucleic acids (10, 11). Polyphenols can generate less reactive species (12) by capturing unpaired electrons present in free radicals, in addition to chelating Fe and Cu (13), preventing the consequent production of new free radicals.
Other mechanisms of action include the interruption of autooxidation chain reactions, deactivation of singlet oxygen, mitigation of nitrosative stress, activation of antioxidant enzymes, or inhibition of oxidative enzymes (10). The synergy between antioxidant compounds also contributes, e.g., between tannins (14) or between tannins and compounds such as quercetin and resveratrol (15).
The chemical structure has also been shown to influence antioxidant activity. For example, the presence of a greater number of hydroxyl (−OH) groups in epicatechin gallate and epigallocatechin gallate, concerning the non-galloyl tannins (catechin and epicatechin) (14), as well as the presence of −OH and methoxyl (−OCH3) groups in the B-ring of anthocyanins (16).
One of the most studied compounds is resveratrol, which has been shown capacity to inhibit the so-called “oxidative burst” (production of O2– and H2O2), to inhibit the expression of NADPH oxidase, to inhibit the uncoupling of the endothelial nitric oxide synthase (eNOS) (17–19), to regulate catalase, superoxide dismutase (SOD), glutathione peroxidase (GPx), glutathione reductase (GR), glutathione-S-transferase (GST) and NAD (P) H: quinone oxidoreductase 1 (NQO1) activities (20), as well as to induce endogenous antioxidant defenses such as the nuclear factor (erythroid-derived 2)-like 2 (Nrf2) pathway (21). The Nrf2 pathway regulates the expression of inflammatory biomarkers inducible NO synthase, interleukin 6 (IL-6), tumor necrosis factor-alpha (TNF-α) (22), as well as the microglial function and neuroinflammation as in cases of Parkinson’s disease (23).
Free radicals such as reactive oxygen species (ROS) can trigger the production of inflammatory mediators such as the cytosine TNF-α, which in turn can lead to increased oxidative stress, in a cycle that contributes to the genesis of pathologies such as cancer, atherosclerosis, neurodegenerative disorders, among others (4, 22, 24). Figure 1 summarizes the main antioxidant and anti-inflammatory activities of red wine polyphenols reported in previous studies.
Figure 1. Main involved mechanisms in the antioxidant and anti-inflammatory activities of the phenolic compounds of red wine.
The following sections summarize the most important mechanisms through which polyphenols exert their protective action against the development of various diseases.
It has been observed that red wine consumption can decrease the level of inflammatory biomarkers related to cardiovascular disease (CVD) such as intercellular adhesion molecule-1 (ICAM-1), IL-6 (25, 26), interleukin 1α (IL-1α) (27) or C-reactive protein (CRP) (26, 28), besides decreasing plasma insulin levels and insulin resistance in patients with high cardiovascular risk and diabetes (29, 30). The latter effects were also observed in non-alcoholic red wine (31). Table 1 summarizes some of the beneficial effects of red wine consumption on biomarkers of cardiovascular risk in various clinical studies.
Table 1. Some clinical studies on the effect of red wine on biomarkers of cardiovascular risk and related pathologies.
Red wine has also been shown to affect plasma lipoproteins. Proposed mechanisms include decreasing levels of low-density lipoprotein (LDL) or “bad” cholesterol (32), responsible for transporting and depositing cholesterol in tissues (initiation of atherosclerotic plaque formation), and increased levels of high-density lipoprotein (HDL) or “good” cholesterol (33, 34), responsible for removing cholesterol from tissues (35). These benefits have been observed in patients who have previously suffered a myocardial infarction (36) and in patients with carotid atherosclerosis (37).
It is considered that oxidized LDL is responsible for the atherogenic process so that the function of phenolic compounds would be to protect them against oxidation, showing greater activity quercetin and resveratrol, which bind to LDL through glycosidic bonds, protecting them against free radicals and reducing oxidation induced by metal ions (13, 38). Anthocyanins have also been shown to reduce LDL levels, for example, in patients with dyslipidemia (39).
Another mechanism includes the effect on nitrous oxide (NO), an important vasodilator, with potential benefits in smokers. Quercetin, tannic acid, malvidin, and resveratrol may contribute to improving endothelial NO production, reducing oxidative stress, vascular inflammation, and platelet aggregation (Figure 2) (40).
Among the main benefits of red wine consumption are the prevention of memory loss, thus attenuating cell death and the development of diseases such as Alzheimer’s disease. The involved mechanisms include the ability of quercetin against cell aging, by activating the proteasome complex (41). Resveratrol has been used to suppress the activation of nuclear transcription factor-kappa B (NF-kB) and the production of prostaglandins (42), reducing the expression of interleukin 1 beta (IL-1β) and TNF-α factor (4, 43), increase the activity of the telomerase enzyme, involved in the prevention of cellular senescence and delay of cognitive decline (44), or promote the activity of sirtuins and peroxisome proliferator-activated receptor-γ co-activator 1-α (PGC-1α) (4, 45). In addition to activating anti-apoptotic Bcl-2 proteins and inactivating pro-apoptotic Bax proteins in the hippocampus (46). Other mechanisms reported in the literature are summarized in Figure 2.
Also, moderate consumption of red wine has been associated with a reduction of up to 50% in the risk of death from stroke (Figure 2) (47) due to the increase in cerebral blood flow, which would be related to the action of resveratrol (48). Resveratrol can also regulate the function of estrogen receptors α and β, reducing cholesterol levels (49) and the formation of atherosclerotic plaque, and thus the risk of ictus due to circulatory failure (50).
Another benefit of red wine consumption is related to its antidepressive effect. Resveratrol, for example, can regulate the monoaminergic system, increasing the levels of serotonin, noradrenaline, and dopamine (51). Likewise, resveratrol, quercetin, ferulic acid, ellagic acid, and proanthocyanidins can modulate the activity of the hypothalamic-pituitary-adrenal axis (HPA axis), as well as serotonergic neurotransmission (52, 53), mechanisms that play an important role against anxiety and depression.
The main involved mechanisms in the anticancer activity of phenolic compounds are described below.
Anthocyanins and tannins have shown protection against UV-B radiation-induced damage by acting on the free radicals produced (54), besides suppressing the activity of cyclooxygenase-2 (COX-2) induced by this radiation (55). Quercetin has shown activity against the myeloperoxidase (MPO) enzyme induced by UV-B radiation (56), contributing to preventing the development of skin cancer.
On the other hand, during lipid peroxidation, unstable intermediate compounds are generated, which in turn may act as “new toxic messengers” in successive reactions (57). For example, malondialdehyde, that at high plasma concentrations may be considered a prognostic factor in ovarian cancer (58). Red wine consumption may regulate plasma levels of malondialdehyde (30).
Resveratrol has shown activity in key stages of carcinogenesis: as an antioxidant and antimutagen in the initiation phase, and as an anti-inflammatory and inhibitor of COX and hydroperoxidase in tumor cells in the promotion phase, besides inducing cell differentiation in the progression phase (59). Resveratrol has also shown activity against the enzyme tank-binding kinase 1 (TBK1), related to carcinogenic inflammatory processes (60), besides reducing the protein methylation related to breast cancer (61) and the ornithine decarboxylase (ODC) activity responsible for the synthesis of polyamines linked to colorectal carcinogenesis (62).
At a clinical level, it has been possible to reduce the proliferation of tumor cells in patients with colon cancer by 5% with doses of 1 g of resveratrol, and it has also been possible to detect resveratrol-3-O-glucuronide, resveratrol-4′-O-glucuronide, resveratrol-3-O-sulfate, resveratrol-4′-O-sulfate, resveratrol sulfate glucuronide, and resveratrol disulfate, which would indicate a joint anticancer action between resveratrol and its derivatives (63).
Proanthocyanidins have been shown to reduce DNA methylation levels and inhibit DNA-methyltransferase and histone deacetylase (HDAC) activities, promoting the re-expression of tumor suppressor genes in skin cancer cells (64). While quercetin has been shown to inhibit the growth of melanoma cells by blocking the transducer protein and inhibiting the activation of signal transducer and activator of transcription 3 (STAT3) proteins (65).
The induction of cancer cell apoptosis involves mechanisms such as cell cycle stop, blockade of c-Jun N-terminal kinase (JNK), NF-kB factor inhibition, kinase C suppression (66), deregulation of fatty acid synthase (FAS), activation of DAPK2 and BNIP3 genes that encode proapoptotic enzymes (67), among others.
Resveratrol at low doses can be useful for maintaining health, while at high doses it can be useful for inducing cell apoptosis (68), and therefore a therapeutic alternative against cancer.
For its part, the ellagic acid has been shown capacity to stimulate apoptosis in prostate carcinoma (PC3) cells by activating the caspase enzyme, besides a decrease in the levels of antiapoptotic protein Bcl-2 and an increase in the proapoptotic protein Bax (69). Delphinidin has shown apoptotic activity in colon cancer cells (70), in addition to retarding growth of liver cancer cells (71) and PC3 cells (72).
Proanthocyanidins can alter migration and invasion processes in pancreatic cancer cells (73) by inhibiting phosphorylation of extracellular signal-regulated kinase 1/2 (ERK1/2) and the inactivation of NF- kB factor. While delphinidin, cyanidin, and resveratrol have shown anti-metastatic activity in human colon cancer cells (74, 75).
The use of resveratrol in synergy with zinc and other compounds for the treatment of prostate cancer has also been suggested (76), which requires further studies to elucidate the action mechanisms, as well as the conditions under which the treatment is clinically effective.
Phenolic compounds in red wine have shown effectiveness against microorganisms involved in the development of caries and periodontitis. Proanthocyanidins act on Streptococcus mutans, responsible for the formation of dental plaque, by inhibiting the enzyme glucosyltransferase (GTF), which catalyzes the conversion of sucrose into glucans (base of dental plaque) (77), besides inhibiting the enzyme F-ATPase, responsible for protecting S. mutans from acidic environmental stress generated in the environment of dental plaque (78). For their part, Fusobacterium nucleatum, Streptococcus oralis, and Actinomyces oris have shown sensitivity to red wine, non-alcoholic red wine, and red wine solutions enriched with grape seed extracts, an effect attributed to catechin and procyanidin B2 from the seeds (79).
Regarding quercetin, it has shown effects against alveolar bone loss in rodents affected by periodontitis, by reducing the production of inflammatory mediators such as IL-1β, IL-17, TNF-α, of the receptor activator of NF-κB ligand (RANKL) and ICAM in gingival tissue (80). Resveratrol has shown a similar effect against oxidative stress and the progression of periodontitis through the activation of sirtuins and the Nrf2 pathway, besides helping to improve alveolar bone resorption (81).
An increase in the bacteria Enterococcus, Bacteroides, Bifidobacterium, Eggerthella tarda, Blautia coccoides, among others, has been observed in the intestinal microflora after the consumption of red wine and non-alcoholic red wine (28). Similar results were subsequently obtained with Enterococcus, Bifidobacterium, and E. lenta, proposing the interaction of anthocyanins with these bacteria, especially with Bifidobacterium, whose growth was positively correlated with the presence of syringic and 4-hydroxybenzoic acids, both anthocyanin derivatives (82). Thus, the prebiotic effect of red wine would be related to the catabolism of phenolic compounds.
The action mechanisms include the antioxidant capacity of phenolic compounds, as well as their capacity to inhibit essential viral enzymes, activate self-defense mechanisms, and prevent the attachment and penetration of the virus into the host cell (83). The latter is of vital importance to inhibiting the synthesis of viral DNA. Quercetin and myricetin have been shown to inhibit DNA and RNA polymerase activity (84), involved in the process of DNA replication and RNA synthesis in viruses (85).
Of special relevance are the recent publications about effect of phenolic compounds against severe acute respiratory syndrome coronavirus 2 (SARS-CoV-2), which causes coronavirus disease 2019 (COVID-19). The tannic acid has shown capacity to inhibit the activity of two proteases that play a key role in cellular entry and replicating of SARS-CoV-2: transmembrane protease serine 2 (TMPRSS2, in the host cell) and 3-chemotrypsin-like protease (Mpro or 3CLpro, in the virus) (86). At clinical level, quercetin has shown positive effects in the treatment of COVID-19 patients by decreasing the serum levels of alkaline phosphatase (ALP), quantitative C-reactive protein (q-CRP), and lactate dehydrogenase (LDH), as critical markers involved in COVID-19 severity. Besides, earlier discharge time was observed in the patients who were taking quercetin. In general, those patients showed better clinical improvements in terms of the COVID-19 symptoms (87).
The synergistic effect of phenolic compounds with other substances can also be used. Resveratrol can improve the action of decitabine in treatments against human immunodeficiency virus (HIV), by inhibiting the enzyme ribonucleotide reductase, thereby blocking viral DNA synthesis and subsequent virus replication (88). For its part, since cytokine storm and sepsis are major causes of death in severe COVID-19, in patients who had received a combined treatment of resveratrol and copper (preprint: observational study), the number of deaths in resveratrol-Cu group was 1.9-fold lower respect to patients with standard care. Although the mechanism of action is unclear, it could be related to the generation of free radicals, which can inactivate or degrade cell-free chromatin released from dying cells and contributing to the sepsis cascade. However, according to the authors, although these results are promising, these need to be confirmed in a randomized clinical trial (89).
However, the antimicrobial and antiviral activity shown by red wine and/or its constituents cannot be comparable to that shown by antibiotics and antivirals, so it should not be used for this purpose.
Various investigations have focused in recent years on increasing the content of phenolic compounds in red wine. Although most of them are aimed at improving the physicochemical stability and the sensory profile, they can also be used to improve the bioactive profile.
It is estimated that around 50% of polyphenols are extracted into the must/wine during vinification (90), that is, almost a half remain in the grape pomace. These polyphenols are not always used, for example, for the development of new foods and other applications (91).
Traditional practices such as the use of pre-fermentative enzymes and cold maceration can help improve the extraction of anthocyanins and tannins (92). On the other hand, the contact time between the skins and the must/wine is an important aspect during the transfer of polyphenols, as in the case of resveratrol, which in some cases reaches its maximum extraction after 10 days (93).
However, the use of non-conventional technologies applicable in the different stages of winemaking process may increase the extraction of polyphenols. These technologies include high pressures, ultrasound (US), pulsed electric fields (PEF), e-beam irradiation, and ozone, all of them applicable in the pre-fermentative stage (in grape berries or grape must), in addition to biotechnological strategies at fermentative level and during the aging of red wine (Figure 3).
Figure 3. Technological strategies to improve the content of phenolic compounds during red winemaking.
The so-called emerging technologies have been studied mainly for the control of microbial load in food. However, they may also be useful to improve the extraction of phenolic compounds, with the advantage of reducing maceration times, not generating thermal damage to the treated product, inactivating oxidative enzymes, and reducing SO2 doses (94). SO2 is an additive that has been related to health problems in the consumer (95) and to generate aromatic defects related to its excessive use (96).
Emerging technologies can also be used to improve extraction in grapes with low phenolic content, as a complementary treatment, or as an alternative to the traditional use of pectolytic enzymes or the “blended” with grape varieties with higher phenolic content, allowing, for example, the elaboration of wines with higher levels of resveratrol (up to 16 mg/L in Pinot noir wines) (97) and quercetin (up to 13 mg/L in Shiraz-grape wines) (90).
High pressures are one of the promising alternatives for the treatment of grapes and/or musts, highlighting high hydrostatic pressure (HHP) and ultra-high pressure homogenization (UHPH), which have been implemented in the development of equipment for industrial applications [more details in Morata et al. (98)].
HHP is a discontinuous flow technique. Besides reducing the microbial load and preserving aroma, HHPs can improve the extraction of phenolic compounds into the must/wine, as well as protect them against oxidation by partially inhibiting the PPO enzyme at pressures greater than 600 MPa (99), which makes it possible to keep the antioxidant properties of wine and reduce the necessary doses of SO2.
Another advantage of HHP is that once applied it allows to keep the integrity of the berry (100), thus facilitating the handling of the grape, without loss of raw material or risk of microbial contamination. On the contrary, the diffusion of anthocyanins to the pulp and the external surface of the seeds is facilitated, because of the rupture of the cell walls of the skin (Table 2) (101).
Table 2. Applications of high-pressure technologies to improve the extraction of phenolic compounds during red winemaking.
HHP improves the selective extraction of phenolic compounds, for example, acylated anthocyanins, which would be related to a better solubility of acylated anthocyanins (less polar than the non-acylated ones), due to the effect of HHP on must polarity (decrease in the dielectric constant of water), and the decrease in pH (molecular deprotonation at high pressures), improving the content of p-coumaroylated anthocyanins, color intensity (CI), and total polyphenol index (TPI) (101).
A better extraction of polyphenols from red grape residues at 600 MPa has also been observed (Table 2), increasing the extraction of acylated anthocyanins, besides increasing the extraction of phenolic compounds with HHP, US (35 KHz), and PEF (3 kV cm–1), and improving the antioxidant capacity with HHP and PEF, regarding the control. In the latter case, it may be related to the inactivation of oxidative enzymes (102).
On the other hand, HHPs can favor the formation of pyranoanthocyanins, mainly derivatives of vitisin A at 600 MPa and 70°C (103), while at 500 MPa (5 min at 20°C) levels of pyranoanthocyanins, prodelphinidins, and polymerized tannins similar to those found in samples obtained with micro-oxygenation and in contact with wood, have been observed (104). However, the content of anthocyanins such as cyanidin may decrease, as occurs with other techniques such as e-beam irradiation (101, 105), thus being able to affect the antioxidant activity.
HHP has also been applied on wines to maintain stability and reduce the necessary doses of SO2. However, a decrease in the content of aromatic compounds, phenolic compounds, and antioxidant capacity has been reported in red wines stored for 12 months and treated with HHP at 350 MPa, in which it is necessary to keep a minimum SO2 concentration of 60 mg/L (Table 2) (106). So that, HHP can be applied as a complementary treatment to reduce the necessary doses of SO2.
UHPH is a technology that is arousing interest in winemaking, mainly for microbial inactivation and inactivation of oxidative enzymes in white musts (107–109), acceleration of yeast autolysis, and production of yeast derivatives for oenological use (108). However, few publications have addressed their applicability in red winemaking processes.
UHPH is a continuous flow technique applicable to liquid samples, for example, musts, at pressures between 200 and 600 MPa, followed by instantaneous depressurization to atmospheric pressure with the consequent generation of 100–300 nm nanofragmentations in the treated sample (94). It is characterized by having a total process time of 0.2 s, with the advantage of not generating damage at the level of vitamins, aromatic compounds, and pigments, even at temperatures close to 80°C (94, 107, 110). Another advantage of UHPH is a greater release of nitrogenous compounds for the nutrition of the yeast in the treated musts, besides allowing the synthesis of fermentative esters with a positive impact on the aroma of the wine compared to the conventional treatment with SO2 (109).
The application of UHPH allows an almost complete inactivation of the enzyme polyphenoloxidase (PPO) (> 90%) (107, 109), with the consequent protection of phenolic compounds, besides maintaining the antioxidant capacity of the must, and color stability, even when exposed to air (Table 2) (110), being greater its capacity to inactivate oxidative enzymes concerning high hydrostatic pressure (HHP).
Although it has not been reported in the literature the effect of UHPH on other grape enzymes with a direct impact on wine quality, such as β-glucosidase, β-lyase, protease, pectinase, hydroxycinnamate decarboxylase (HCDC), cellulase, among others (111, 112), the effect of UHPH at the level of quaternary structure denaturation in proteins should be considered (113). However, the use of exogenous enzymes can help counteract the effect of UHPH on these grape enzymes.
Unlike HHP, which works under static conditions, high-pressure homogenization is a dynamic technology, and its effect, besides high pressure, depends on phenomena such as cavitation, turbulence, and shearing (108). Initially, UHPH would not be applicable for the processing of red grape mash due to the presence of peels and seeds, which can clog the homogenizer valves, considering that particle sizes smaller than 500 μm have been suggested (94). Thus, the application of UHPH would be limited only to musts free of peels, seeds, and other solids. Previously, the application of high-pressure homogenization, at 200 MPa at a flow rate of 120 L h–1, to Trepat red grape must has been reported, in order to reduce the microbial load and evaluate the impact on the sensory profile. However, the article does not mention whether the red must was treated together with seeds and peels (grape mash), or whether these solids were previously separated (114).
Considering that one of the main advantages of UHPH is its inhibitory effect on PPO (107, 109, 110), an interesting alternative has recently been reported in Cabernet Sauvignon red must (110), which, after a maceration process at 0°C for 15 days, was separated from peels and seeds, and subsequently treated with UHPH at 300 MPa (Table 2). Among the most important results, the maintenance of anthocyanin levels in the wine produced stands out.
Another alternative could include the application of technologies that allow greater extraction of phenolic compounds during maceration from the grape mash, such as ultrasound, pulsed electric fields, irradiation, or ozone, following the methodology developed by Vaquero et al. (110), with the subsequent draining of the must to separate seeds and peels, followed by the application of UHPH to reduce the microbial load and inactivate the PPO, protecting the polyphenols against oxidation.
Through Resolution OIV-OENO 634-2020, the International Organization of Vine and Wine (OIV) included the use of pulsed electric fields (PEF) for treatment of red grapes destemmed and crushed in order to facilitate and increase the extraction of polyphenols and other valuable substances located inside the grape cells and reduce maceration time. The text has been included in the International Code of Oenological Practices.
Several works have reported the application of pulsed electric fields (PEF) to reduce the microbial load in winemaking processes, in which their efficacy for the extraction of polyphenols is also highlighted (Table 3). Among the advantages of PEFs, the reduction of SO2 doses during must sterilization stands out (115), besides improving the extraction of phenolic compounds and preserving color at doses of up to 41 kV cm–1 (116).
Table 3. Applications of pulsed electric fields to improve the extraction of phenolic compounds during red winemaking.
This technology has shown high efficiency in the extraction of phenolic compounds, at rates higher than 50% (102), due to its action on the cell walls of the skin, producing fragmentation at the nanometric scale, besides reducing maceration time up to 50% at doses of 5–10 kV cm–1 (117) and 75% at doses of 33–41 kV cm–1 (116), being the efficacy variable depending on the intensity of the PEF, duration of the pulse or number of pulses (118), as well as the variety of treated grape (117).
The application of PEF (0.8 kV cm–1, 100 ms, 42 kJ kg–1 and 5 kV cm–1, 1 ms, 53 kJ kg–1, respectively) in Cabernet Franc musts (119) has allowed obtain higher yields of: anthocyanins (46 and 62%, respectively, concerning the control) and tannins (50 and 59%, respectively), besides increasing the antioxidant capacity (51 and 52%, respectively) at the end of fermentation. In a similar study with Cabernet Franc and Cabernet Sauvignon musts (120), the application of PEF (5 kV cm–1, 1 ms) during cold maceration improved the extraction of quercetin 3-β-D-glucoside (100 and 74%, respectively, concerning the control), anthocyanins (98 and 60%, respectively) and proanthocyanidins by more than 35% in both varieties, in addition to increasing antioxidant activity by more than 100%.
Subsequently, the application of PEF (0.8 kV cm–1, 100 ms and 5 kV cm–1, 1 ms) during cold maceration and alcoholic fermentation in Cabernet Sauvignon musts was studied, obtaining a better extraction of anthocyanins and proanthocyanidins in the maceration stage with 0.8 kV cm–1 (121).
Furthermore, the application of PEF (7.4 kV cm–1) to Tempranillo, Graciano, and Grenache musts (122) improved anthocyanin extraction by up to 49, 87, and 163%, respectively, concerning the control. However, the most remarkable feature of this study was the improvement of total stilbene content, up to 60, 200, and 50%, respectively, mainly the trans- and cis-piceido fractions (glycosidic forms of resveratrol). No significant effect was observed in the extraction of trans-resveratrol, which would be related to the absence of ethanol in the maceration stage (123).
Regarding the antioxidant capacity, improvements of more than 25% were obtained in the viability of Caco-2 cells incubated in digested grape must, against H2O2-induced stress. The digested grape must was obtained by digestion of Pinot Noir must treated with PEF (1.5 kV cm–1) and macerated for 8 days (Table 3) (124).
PEFs can also increase the selective extraction of acylated anthocyanins by more than 7 times at a dose of 3 kV cm–1 in comparison to the control (Table 3) (102), besides contributing to the depolymerization of the skin tannins, improving the permeability and diffusion through the cell walls fragmented by the PEF (4 kV cm–1, 1 ms) (125). Although from a sensory point of view smaller tannins can increase the sensation of astringency and bitterness in the wine (125, 126), the application of processes such as aging-on-lees may help reduce their impact.
Respect to aging of wine, a higher content of flavanols, flavonols, anthocyanins, and hydroxycinnamic acids has also been reported after 12 and 24 months of aging in Cabernet Sauvignon and Grenache wines (respectively) made from grapes treated with PEF at doses of 5 and 4.3 kV cm–1, respectively (Table 3) (127, 128). Intensities lower than 4 kV cm–1 followed by macerations for 6 days allowed higher anthocyanin and tannin extractions than treatments at intensities > 5.5 kV cm–1 with 4-day macerations (Table 3) (118), conditions that could be applied to reduce maceration time and improve phenolic content and other parameters of red wine at the end of the fermentation and aging process.
PEFs also improve the extraction of anthocyanins and total phenols in grape varieties considered to have a low red color, such as Rondinella, being possible to increase anthocyanin contents by more than 50% in comparison to wines made with untreated must, and by more than 30% in comparison to wines made with musts treated with pectolytic enzymes (129). An alternative to improve the extraction of polyphenols is the combined application of PEF and pre-fermentative maceration (immediately after destemming and crushing the grapes), in order to obtain a higher content of polyphenols and a greater antioxidant capacity (130).
Ultrasound (US) is a technology that can be used for the extraction of phenolic compounds thanks to the mechanical action that produces the successive compression and expansion of the bubbles formed by the ultrasonic waves, which, when collapsing, release energy, reaching localized temperatures of up to 5,000 K and pressures up to 200 MPa (131).
Through Resolution OIV-OENO 616-2019, the International Organization of Vine and Wine (OIV) included the use of US during pre-fermentative maceration, after destemming and crushing, to improve the extraction of phenolic compounds, besides shortening the maceration time and limiting the excessive extraction of tannins in grapes with deficient phenolic maturation. The text has been included in the International Code of Oenological Practices.
The treatment of red musts with US is an effective alternative to increase the extraction of tannins and total phenols by up to 58 and 27%, respectively (132), and to maintain anthocyanin levels by up to 97% (133), without affecting its chemical stability. The treatment with US may also generate a greater increase in the content of polymeric anthocyanins in wines stored for 12 months (132) compared to the increase observed in the same fresh fermented wines (at the end of alcoholic fermentation) (Table 4).
Table 4. Applications of ultrasound and irradiation to improve the extraction of phenolic compounds during red winemaking.
It is also possible to take advantage of the application of US together with other treatments (Table 4), for example, in sequential applications with pectolytic enzymes (134). Although the decrease in monomeric anthocyanins and the increase in polymeric forms is a normal phenomenon during red wine aging, the treatment of musts with US has made it possible to obtain red wines (after 3 months of bottle storage) with a higher content of polymeric anthocyanins and tannins compared to the control wine produced through the traditional process (134). The increase in these phenolic compounds was even greater when the musts were treated sequentially with pectolytic enzymes and US. Although in both cases a decrease in the monomeric anthocyanin content was reported, the application of US to the must reduced the loss of anthocyanins in the elaborated wine.
Like other technologies, the efficiency in the extraction of phenolic compounds depends on the intensity of US. For example, an optimal range between 12 and 37.5 kHz has been reported to increase anthocyanin extraction by up to 18%. Higher doses can alter the content of anthocyanins (135).
Electron beam irradiation (e-beam) is characterized by the use of high-energy accelerated electrons and can be applied continuously on an industrial scale without increasing the temperature of the treated product, even at doses of 10 kGy (100), with the advantage of reducing the necessary doses of SO2 as a preservative and antioxidant.
Doses of 10 kGy may improve the extraction of anthocyanins by more than 70% (Table 4) (136), also improving the fruity smell of the produced wine. Doses of less than 10 kGy affect the pectin structure, allowing a greater diffusion of phenolic compounds into the must/wine, besides reducing the necessary doses of exogenous pectins (98). Lower doses (0.5–3 kGy) have also shown improvements in the extraction of anthocyanins and phenolic compounds in general (137, 138), and doses of 2 kGy have allowed obtaining better antioxidant capacity in wines made from irradiated grapes (137).
A recent study has reported the decrease in the content of flavonols, flavanols, anthocyanins, and phenolic acids in red wines treated directly with e-beam radiation, at doses between 1 and 10 kGy, as an alternative to the use of SO2 (139). However, another study reported a higher content of flavonols, flavanols, anthocyanins, total phenols, and higher antioxidant capacity in wines made from grapes irradiated at a dose of 1.5 kGy, compared to wines made from non-irradiated grapes (137).
Furthermore, although the content of phenolic compounds, mainly anthocyanins, decreases during aging, it has been reported that after 4 months in bottle, red wines made from irradiated grapes maintain anthocyanins, total phenols, and antioxidant capacity at higher levels than the respective wines made from non-irradiated grapes (Table 4) (137). Likewise, greater stability of vinylphenolic pyranoanthocyanins and vitisins has been reported in wines made from irradiated grapes at doses of 10 kGy (136). Which indicates that the advantage of this technology would be focused on its applicability on the raw material (grape berry).
It has been possible to increase the extraction of anthocyanins and tannins by up to 19 and 16%, respectively, in Petit Verdot grapes treated with ozone, besides shortening the fermentation time in large-scale vinifications (Table 4) (140). On the other hand, has been showed higher extractability of flavanols in musts from Nebbiolo grapes treated with ozone (short exposures: < 72 h, 30 μL L–1) during maceration (141).
One aspect to optimize is the treatment time, considering that prolonged exposure, even at low concentrations, can induce a decrease in the total phenolic content due to oxidation caused by ozone activity (141, 142). However, short treatments may increase the levels of anthocyanins and tannins in the berry, as a response to mild to moderate ozone-induced stress, activating the protective antioxidant response through the synthesis of phenolic substances (140, 141).
Moreover, the prolonged exposure can harden the skin of the berries (143), which would be related to a reduction of the disassembly phenomena of the pectic structure of the cell wall (less solubilization of pectins, less depolymerization of polyuronides, and less pectin methylesterase activity) (144), generating a slow extraction of polyphenols, for example, of high-molecular-weight flavanols, whose affinity with cell walls increases when the berry is treated with ozone (141). On the other hand, in shorter ozone treatments, higher polygalacturonase and pectin methylesterase activity has been observed (142), whose action contributes to degrading the pectins of cell walls, facilitating the diffusion of polyphenols into the must/wine. The effect of ozone on the hardening of the cell wall of treated berries, and, therefore, on the extractability of polyphenols, also depends on the stage of grape ripeness, ozone concentration (140), and grape variety (Table 4) (141).
During maceration, di-substituted anthocyanins (cyanidin-3-glucoside and peonidin-3-glucoside) are the first ones to migrate into the must, followed by tri-substituted anthocyanins (malvidin-3-glucoside, delphinidin-3-glucoside, petunidin-3-glucoside) (145). It has been proposed that the hardening of ozone-treated berries may result in slower extraction of di-substituted anthocyanins, which are also more easily lost due to oxidation during the early stages of winemaking when oxidative enzymes are more active and dissolved oxygen levels are higher. That is, the slow extraction would help to maintain the content of these anthocyanins at the end of maceration, especially peonidin-3-glucoside (141). In this regard, higher contents of peonidin-3-glucoside (di-substituted) and malvidin-3-glucoside (tri-substituted) have been reported at the end of maceration of Barbera and Nebbiolo grape musts treated with ozone (141). In other words, the application of ozone would make it possible to maintain the final levels of anthocyanins (143).
Finally, the application of ozone would be recommended only on the raw material, that is, in the grape before crushing and destemming, considering that the direct application to red wine at a dose of 3.5 g/h, as an alternative to the use of SO2, may lead to the loss of flavonols, flavanols, anthocyanins and phenolic acids (139), thus affecting the bioactive profile of the wine.
During alcoholic fermentation, one aspect to consider is the use of yeasts with low expression of anthocyanin-β-glucosidase (anthocyanase) activity, which causes anthocyanin hydrolysis (146), although this enzyme can contribute to improving the varietal aroma of the wine through the release of aromatic compounds from non-aromatic precursors of the grape (112). The use of yeasts with low adsorption capacity for phenolic compounds, such as anthocyanins (126) and resveratrol (147), should also be taken into account, due to their adsorption by the cell walls of yeasts, which due to their non-polar character, have shown greater adsorption capacity for acylated anthocyanins (from higher to lower adsorption: coumarilated > caffeinated > acetylated) compared to the non-acylated ones (148, 149), indicating an inverse relationship between the polarity of anthocyanins and their adsorption. This would also explain the adsorption of resveratrol (with low hydrophilic character) (123, 150), by the cell walls of yeasts.
The adsorption capacity has also been related to the degree of methoxylation and hydroxylation of the B-ring of anthocyanins. Malvidin and peonidin, with a higher degree of methoxylation (more non-polar), are adsorbed to a greater extent than delphinidin and petunidin, with a higher degree of hydroxylation (more polar) (148). On the contrary, a lower adsorption capacity has been observed with vitisins A and B (149).
It is estimated that some Saccharomyces strains can adsorb up to 5.8% of anthocyanins (149), while the use of certain non-Saccharomyces yeast strains, such as Metschnikowia pulcherrima and Lachancea thermotolerans can contribute to retaining a higher amount of anthocyanins in wine (151). Therefore, the selection of yeast strains with low anthocyanin adsorption and low anthocyanin-β-glucosidase activity is of utmost importance, in addition to other characteristics such as high production of pyranoanthocyanins.
Although many of the technologies applicable to the grapes, must, or even wine, may lead to losses in the content of anthocyanins, at the fermentation level the content of more stable polyphenols may be increased (Table 5). Vinylphenolic pyranoanthocyanins are formed by the condensation of vinylphenols and anthocyanins. The use of yeasts with hydroxycinnamate decarboxylase (HCDC +) activity is an interesting strategy to increase vinylphenolic pyranoanthocyanins in wine since they can release precursor vinylphenols from grape hydroxynamic acids (152). For their part, vitisins A and B come from the condensation of malvidin-3-O-glucoside (M3G) with pyruvic acid and acetaldehyde, respectively, during the fermentation process or aging (153). These pyranoanthocyanins have shown high chemical stability due to the presence of the fourth heteroaromatic ring in their structure, formed from the integration of a non-anthocyanin molecule (vinylphenols, pyruvate, or acetaldehyde) in the structure of the precursor anthocyanin (152), resulting in greater resistance to oxidation.
Table 5. Strategies applied at the fermentative level and during the aging of red wines to improve and/or maintain the content of phenolic compounds.
The synthesis of vinylphenolic pyranoanthocyanidins also contributes to improving anthocyanin protection against degradation by microorganisms such as Dekkera/Brettanomyces (154, 155), since this yeast cannot degrade these pyranoanthocyanins (156), allowing their content to be maintained in the wine.
Pyranoanthocyanins have shown antioxidant and anti-inflammatory activity against prooxidant (H2O2) and pro-inflammatory (TNF-α) molecules, besides neutralizing the secretion of inflammatory biomarkers such as interleukin 8 (IL-8) in human colon adenocarcinoma cell cultures (24). Vitisin A has shown a protective effect against the secretion of the biomarker monocytic chemoattractant protein-1 (MCP-1) induced by the TNF-α factor in human endothelial cell cultures (157). Furthermore, vitisin A has shown good stability under simulated gastrointestinal conditions (in vitro) (158).
An increase in the content of vinylphenolic pyranoanthocyanins has been reported in mixed fermentation of S. cerevisiae with Pichia guillermondii (159), and the synthesis of vitisins in mixed fermentations of S. cerevisiae with Schizosaccharomyces pombe and with Torulaspora delbruecki (Table 5) (160, 161). Species with high acetaldehyde production such as Saccharomycodes ludwigii (162, 163) can also be used to increase the synthesis of vitisin B.
Another alternative could be the use of metabolic inhibitors during alcoholic fermentation, to increase the production of acetaldehyde by S. cerevisiae (164, 165), because of the inhibitory effect on the enzyme alcohol dehydrogenase (ADH), responsible for converting the aldehyde into ethanol (166), which could increase the substrate available for the synthesis of vitisin B.
During aging, a series of physicochemical processes occur that, individually or together, may modify the content of bioactive compounds, because of isomerization, hydrolysis, polymerization, condensation, or even degradation reactions that may have effects on the bioavailability and bioactivity of polyphenols. In general, the content of anthocyanins, resveratrol, and flavonols tends to decrease (7, 126, 167), as a consequence of their transformation into other molecules, so that greater health benefits from consuming young red wines would be expected.
On the contrary, the content of monomeric flavanols may increase due to the hydrolysis of tannins (7). These monomers have shown high antioxidant capacity (13, 14), as well as antimicrobial activity (79). Although from a sensory point of view monomeric tannins can increase bitterness and astringency (126), the application of processes such as aging-on-lees may help reduce their impact.
The synthesis of vitisins may occur during alcoholic fermentation by the action of yeasts, although their synthesis mostly occurs during wine aging. Vitisins have shown a lower capacity to neutralize free radicals, such as the O2– anion, than their precursor anthocyanins (16), although the pyruvic adduct of delphinidin-3-O-glucoside has shown a greater capacity to neutralize OH– and O2– anions in comparison to other pyranoanthocyanins, but to a lesser extent than its precursor delphinidin-3-O-glucoside.
During the synthesis of vitisin A, pyruvic acid is incorporated in positions 4 and 5 of the A ring of the precursor anthocyanin, which may reduce its antioxidant capacity. This phenomenon would be related to the loss of the −OH group of carbons 5 and 7 of the anthocyanin, which contributes to its antioxidant activity (168). Thus, greater health benefits would be expected from the consumption of young red wines, where the anthocyanin content is higher.
However, these condensations, according to traditional vinification, are necessary to confer physicochemical and microbiological stability to the wine (152, 156). Although the increase in the content of pyranoanthocyanins does not contribute to increasing the antioxidant capacity concerning the antioxidant capacity conferred by the precursor anthocyanins, the greater stability of the pyranoanthocyanins may contribute to counteracting the loss of antioxidant capacity throughout aging.
One of the red wine aging techniques that has gained importance in recent years is aging-on-lees (AOL), which is characterized by the release of polysaccharides from the cell walls of yeast lees to the wine during aging (169). These polysaccharides can improve, among other attributes, the protection of phenolic compounds against oxidation, allowing, among other benefits, to maintain their antioxidant and anti-inflammatory capacity. In addition, the lees have a higher affinity for oxygen (170), so that in their presence the polyphenols can be protected against oxidation.
Although the content of anthocyanins may decrease during AOL (126, 171) due to adsorption by the lees (126), the application of lees of species such as S’codes ludwigii and S. pombe (169) may reduce the loss of anthocyanins. However, the lees must come from yeasts with low or no β-glucosidase activity, which can generate hydrolysis, and, therefore, the loss of anthocyanins (146).
Although most studies have focused on the quality of red wine in the aging phase, it has been reported that the addition of polysaccharides before alcoholic fermentation also has a positive effect (Table 5), increasing the content of anthocyanins, phenolic acids, tannins, and antioxidant capacity in the produced red wine (171).
The procedures described can be very useful in the winemaking process in order to improve the content and availability of phenolic compounds in the red wine, which once ingested may contribute to improving the health state of the consumer, without neglecting that these compounds cannot replace medicines, since their nature is not curative, but rather as components of the diet that, mainly, can help prevent certain pathologies.
Most of these technologies are mainly aimed at reducing the microbial load in the grape must and improving the physicochemical stability and sensory profile of red wine. However, they can be used to improve the content of bioactive compounds, considering that around 50% of the phenolic compounds are trapped in the red grape residue (pomace) (90) and that not always is used, for example, for food purposes (91).
It is also important to mention that, in most studies, the content of anthocyanins and derivatives, flavanols, and total polyphenols have been evaluated. However, the literature regarding the impact of these technologies on the content of other polyphenols is scarce. For example, in the case of resveratrol, which, according to the existing evidence, is the polyphenol that would provide the most health benefits.
The scaling up of these technologies is still a pending issue, considering that only high pressures, PEF and US, have been evaluated at the industrial level.
Regarding ultrasound, the OIV, through the Resolution OIV-OENO 616-2019, included its use for the pre-fermentative maceration stage to improve the extraction of phenolic compounds, among other benefits. In the market, it is possible to find systems based on this technology for applications in liquid foods, such as those commercialized by Industrial Sonomechanics (172).
In the case of PEFs, also the OIV (Resolution OIV-OENO 634-2020) has included their use in the pre-fermentative maceration stage, and there is currently industrial equipment for the processing of liquid foods up to 10,000 L h–1 and solid foods up to 70,000 kg h–1, commercialized by Elea Technology (173). At the winery level, PEF has been applied at intensities between 4.0 and 4.3 kV cm–1 on Garnacha musts with workflows up to 2,500 kg h–1 (128, 174) obtaining wines with better phenolic contents (Table 3). Regarding PEF intensity, it has applied high intensities between 17 and 30 kV cm–1 (Table 3), obtaining improvements in phenolic content and reduction of maceration time (115, 116). However, most of the studies have applied intensities lower than 7 kV cm–1 with good results (Table 3), which, in addition, would allow better adaptability at the industrial level, considering that the energy needed to produce high-intensity electric fields determines the characteristics of the PEF generator and, therefore, the cost of the PEF system (118, 130).
Commercially, UHPH systems, such as those offered by Homogenizing Systems, can be found with production capacities up to 1,000 L h–1 (175). However, on the company website it is not reported its specific application for winemaking. It is also possible to find the UHPH system patented by the Universidad Autónoma de Barcelona (176) and exploited by Ypsicon Advanced Technologies (177), equipped for winemaking with flow rates up to 50,000 L h–1. However, one of the challenges to overcome with this technology is its applicability in red musts containing peels and seeds (grape mash), considering that this technology has been evaluated in liquid foods with particle sizes of less than 0.5 mm (94). An alternative to taking advantage of the benefits of UHPH would be its sequential application in processes that include maceration in the presence of peels and seeds, with subsequent draining, for the later application of UHPH to the pure must, according to the methodology proposed by Vaquero et al. (110). It could also include the application of US, PEF, irradiation, or ozone in the maceration stage, with subsequent draining of the must and application of UHPH to reduce the microbial load and inactivate PPO, protecting polyphenols against oxidation.
Although the technologies described have shown efficacy to improve the extraction of phenolic compounds in the pre-fermentation stage, the decrease in anthocyanin content in wines made from grapes/must treated with HHP and e-beam irradiation is a disadvantage in the small-scale studies, which must be overcome for its industrial applicability, mainly by optimizing winemaking conditions that reduce the decrease in the general content of polyphenols (101, 105, 136).
Although the available literature reports that most of the studies have been carried out with small sample volumes and at the laboratory level, the results obtained have shown the potential of these technologies to improve the phenolic content in red wine, not without highlighting the need for further studies to optimize winemaking conditions with large volumes and in continuous flow systems, for their full implementation in the winery.
Finally, greater dissemination of the benefits of these technologies is necessary, allowing consumers to be aware of their advantages in the production of red wines, such as the reduction of the necessary doses of SO2, which has been related to different health problems in the consumer (95).
There is ample evidence regarding the benefits of wine on health, especially red wine, due to its higher content of phenolic compounds, which can help maintain good health and prevent diseases, being moderate consumption vitally important. The first part of the review summarizes some studies conducted, mostly with information available on the preclinical level, which contributes to a better understanding of the mechanisms by which phenolic compounds could act in the human body (clinical level), taking advantage of their antioxidant, anti-inflammatory, antitumor, antithrombotic, antiatherogenic, and antimicrobial activity, among others. According to the bibliography consulted, in the last years various studies address specific procedures aimed at improving the bioactive profile of red wine. In this sense, the second part of the review describes technological strategies that can contribute to increasing or at least maintaining, the content of polyphenols in red wine. In the pre-fermentation stage, extraction can be increased by treating the grape berries with high pressures, PEF, US, e-beam radiation, and ozone, while, at the fermentative level, yeasts with low adsorption of polyphenols, low anthocyanin-β-glucosidase (anthocyanase) activity, and high production of pyranoanthocyanins and/or their precursor molecules could be very useful. During aging, although in most cases the content of polyphenols can be reduced, AOL can maintain the levels of these compounds in the wine, in addition to chemical processes that modify its structure, such as the synthesis of pyranoanthocyanins, polymerization of anthocyanins and flavanols, anthocyanin-tannin condensation, among others, maintaining to a certain extent the bioactive profile of red wine, and, therefore, transmitting its health benefits to the consumer.
RV conceptualized, drafted, and revised the manuscript. ML-C drafted the manuscript. Both authors reviewed and approved the finalized version of the manuscript.
The authors declare that the research was conducted in the absence of any commercial or financial relationships that could be construed as a potential conflict of interest.
The handling editor AM declared a past collaboration with the author RV.
All claims expressed in this article are solely those of the authors and do not necessarily represent those of their affiliated organizations, or those of the publisher, the editors and the reviewers. Any product that may be evaluated in this article, or claim that may be made by its manufacturer, is not guaranteed or endorsed by the publisher.
1. Levy E, Delvin E, Marcil V, Spahis S. Can phytotherapy with polyphenols serve as a powerful approach for the prevention and therapy tool of novel coronavirus disease 2019 (COVID-19)? Am J Physiol Endocrinol Metab. (2020) 319:E689–708. doi: 10.1152/ajpendo.00298.2020
2. Zhang H, Tsao R. Dietary polyphenols, oxidative stress and antioxidant and anti-inflammatory effects. Curr Opin Food Sci. (2016) 8:33–42. doi: 10.1016/j.cofs.2016.02.002
3. Iriti M, Varoni EM. Cardioprotective effects of moderate red wine consumption: polyphenols vs. ethanol. J Appl Biomed. (2014) 12:193–202. doi: 10.1021/jf802050h
4. Jeong SI, Shin JA, Cho S, Kim HW, Lee JY, Kang JL, et al. Resveratrol attenuates peripheral and brain inflammation and reduces ischemic brain injury in aged female mice. Neurobiol Aging. (2016) 44:74–84. doi: 10.1016/j.neurobiolaging.2016.04.007
5. Noguer MA, Cerezo AB, Donoso Navarro E, Garcia-Parrilla MC. Intake of alcohol-free red wine modulates antioxidant enzyme activities in a human intervention study. Pharmacol Res. (2012) 65:609–14. doi: 10.1016/j.phrs.2012.03.003
6. Chong E, Chang SL, Hsiao YW, Singhal R, Liu SH, Leha T, et al. Resveratrol, a red wine antioxidant, reduces atrial fibrillation susceptibility in the failing heart by PI3K/AKT/eNOS signaling pathway activation. Hear Rhythm. (2015) 12:1046–56. doi: 10.1016/j.hrthm.2015.01.044
7. Lingua MS, Fabani MP, Wunderlin DA, Baroni MV. From grape to wine: changes in phenolic composition and its influence on antioxidant activity. Food Chem. (2016) 208:228–38. doi: 10.1016/j.foodchem.2016.04.009
8. Champ CE, Kundu-Champ A. Maximizing polyphenol content to uncork the relationship between wine and cancer. Front Nutr. (2019) 6:44. doi: 10.3389/fnut.2019.00044
9. Doustimotlagh AH, Eftekhari M. Glucose-6-phosphate dehydrogenase inhibitor for treatment of severe COVID-19: polydatin. Clin Nutr ESPEN. (2021) 43:197–9. doi: 10.1016/j.clnesp.2021.02.021
10. Carocho M, Ferreira ICFR. A review on antioxidants, prooxidants and related controversy: natural and synthetic compounds, screening and analysis methodologies and future perspectives. Food Chem Toxicol. (2013) 51:15–25. doi: 10.1016/j.fct.2012.09.021
11. Lü JM, Lin PH, Yao Q, Chen C. Chemical and molecular mechanisms of antioxidants: experimental approaches and model systems. J Cell Mol Med. (2010) 14:840–60. doi: 10.1111/j.1582-4934.2009.00897.x
12. Craft BD, Kerrihard AL, Amarowicz R, Pegg RB. Phenol-based antioxidants and the in vitro methods used for their assessment. Compr Rev Food Sci Food Saf. (2012) 11:148–73. doi: 10.1111/j.1541-4337.2011.00173.x
13. Gupta A, Birhman K, Raheja I, Kumar Sharma S, KumarKar H. Quercetin: a wonder bioflavonoid with therapeutic potential in disease management. Asian Pacific J Trop Dis. (2016) 6:248–52. doi: 10.3390/antiox9111105
14. Furlan AL, Jobin ML, Buchoux S, Grélard A, Dufourc EJ, Géan J. Membrane lipids protected from oxidation by red wine tannins: a proton NMR study. Biochimie. (2014) 107(Part A):82–90. doi: 10.1016/j.biochi.2014.07.008
15. McAnulty LS, Miller LE, Hosick PA, Utter AC, Quindry JC, McAnulty SR. Effect of resveratrol and quercetin supplementation on redox status and inflammation after exercise. Appl Physiol Nutr Metab. (2013) 38:760–5. doi: 10.1139/apnm-2012-0455
16. Garcia-Alonso M, Rimbach G, Sasai M, Nakahara M, Matsugo S, Uchida Y, et al. Electron spin resonance spectroscopy studies on the free radical scavenging activity of wine anthocyanins and pyranoanthocyanins. Mol Nutr Food Res. (2005) 49:1112–9. doi: 10.1002/mnfr.200500100
17. Shen MY, Hsiao G, Liu CL, Fong TH, Lin KH, Chou DS, et al. Inhibitory mechanisms of resveratrol in platelet activation: pivotal roles of p38 MAPK and NO/cyclic GMP. Br J Haematol. (2007) 139:475–85. doi: 10.1111/j.1365-2141.2007.06788.x
18. Singh N, Agrawal M, Doré S. Neuroprotective properties and mechanisms of resveratrol in in vitro and in vivo experimental cerebral stroke models. ACS Chem Neurosci. (2013) 4:1151–62. doi: 10.1021/cn400094w
19. Spanier G, Xu H, Xia N, Tobias S, Deng S, Wojnowski L, et al. Resveratrol reduces endothelial oxidative stress by modulating the gene expression of superoxide dismutase 1 (SOD1), glutathione peroxidase 1 (GPx1) and NADPH oxidase subunit (Nox4). J Physiol Pharmacol An Off J Polish Physiol Soc. (2009) 60(Suppl 4):111–6.
20. Rubiolo JA, Mithieux G, Vega FV. Resveratrol protects primary rat hepatocytes against oxidative stress damage: activation of the Nrf2 transcription factor and augmented activities of antioxidant enzymes. Eur J Pharmacol. (2008) 591:66–72. doi: 10.1016/j.ejphar.2008.06.067
21. Ghanim H, Sia CL, Korzeniewski K, Lohano T, Abuaysheh S, Marumganti A, et al. A resveratrol and polyphenol preparation suppresses oxidative and inflammatory stress response to a high-fat, high-carbohydrate meal. J Clin Endocrinol Metab. (2011) 96:1409–14. doi: 10.1210/jc.2010-1812
22. Innamorato NG, Rojo AI, García-Yagüe ÁJ, Yamamoto M, de Ceballos ML, Cuadrado A. The transcription factor Nrf2 is a therapeutic target against brain inflammation. J Immunol. (2008) 181:680–9. doi: 10.4049/jimmunol.181.1.680
23. Rojo AI, Innamorato NG, Martín-Moreno AM, De Ceballos ML, Yamamoto M, Cuadrado A. Nrf2 regulates microglial dynamics and neuroinflammation in experimental Parkinson’s disease. Glia. (2010) 58:588–98. doi: 10.1002/glia.20947
24. Peng Y, Zhang H, Liu R, Mine Y, McCallum J, Kirby C, et al. Antioxidant and anti-inflammatory activities of pyranoanthocyanins and other polyphenols from staghorn sumac (Rhus hirta L.) in Caco-2 cell models. J Funct Foods. (2016) 20:139–47. doi: 10.1016/j.jff.2015.10.026
25. Chiva-Blanch G, Urpi-Sarda M, Llorach R, Rotches-Ribalta M, Guilleń M, Casas R, et al. Differential effects of polyphenols and alcohol of red wine on the expression of adhesion molecules and inflammatory cytokines related to atherosclerosis: a randomized clinical trial. Am J Clin Nutr. (2012) 95:326–34. doi: 10.3945/ajcn.111.022889
26. Sacanella E, Vázquez-Agell M, Mena MP, Antúnez E, Fernández-Solá J, Nicolás JM, et al. Down-regulation of adhesion molecules and other inflammatory biomarkers after moderate wine consumption in healthy women: a randomized trial. Am J Clin Nutr. (2007) 86:1463–9. doi: 10.1093/ajcn/86.5.1463
27. Estruch R, Sacanella E, Badia E, Antúnez E, Nicolás JM, Fernández-Solá J, et al. Different effects of red wine and gin consumption on inflammatory biomarkers of atherosclerosis: a prospective randomized crossover trial: effects of wine on inflammatory markers. Atherosclerosis. (2004) 175:117–23. doi: 10.1016/j.atherosclerosis.2004.03.006
28. Queipo-Ortuño MI, Boto-Ordóñez M, Murri M, Gomez-Zumaquero JM, Clemente-Postigo M, Estruch R, et al. Influence of red wine polyphenols and ethanol on the gut microbiota ecology and biochemical biomarkers. Am J Clin Nutr. (2012) 95:1323–34. doi: 10.3945/ajcn.111.027847
29. Chiva-Blanch G, Urpi-Sarda M, Ros E, Valderas-Martinez P, Casas R, Arranz S, et al. Effects of red wine polyphenols and alcohol on glucose metabolism and the lipid profile: a randomized clinical trial. Clin Nutr. (2013) 32:200–6. doi: 10.1016/j.clnu.2012.08.022
30. Estruch R, Sacanella E, Mota F, Chiva-Blanch G, Antúnez E, Casals E, et al. Moderate consumption of red wine, but not gin, decreases erythrocyte superoxide dismutase activity: a randomised cross-over trial. Nutr Metab Cardiovasc Dis. (2011) 21:46–53. doi: 10.1016/j.numecd.2009.07.006
31. Chiva-Blanch G, Urpi-Sarda M, Ros E, Arranz S, Valderas-Martínez P, Casas R, et al. Dealcoholized red wine decreases systolic and diastolic blood pressure and increases plasma nitric oxide. Circ Res. (2012) 111:1065–8. doi: 10.1161/CIRCRESAHA.112.275636
32. Apostolidou C, Adamopoulos K, Lymperaki E, Iliadis S, Papapreponis P, Kourtidou-Papadeli C. Cardiovascular risk and benefits from antioxidant dietary intervention with red wine in asymptomatic hypercholesterolemics. Clin Nutr ESPEN. (2015) 10:E224–33. doi: 10.1016/j.clnesp.2015.08.001
33. Hansel B, Thomas F, Pannier B, Bean K, Kontush A, Chapman MJ, et al. Relationship between alcohol intake, health and social status and cardiovascular risk factors in the urban Paris-Ile-De-France cohort: is the cardioprotective action of alcohol a myth? Eur J Clin Nutr. (2010) 64:561–8. doi: 10.1038/ejcn.2010.61
34. Perissinotto E, Buja A, Maggi S, Enzi G, Manzato E, Scafato E, et al. Alcohol consumption and cardiovascular risk factors in older lifelong wine drinkers: the Italian longitudinal study on aging. Nutr Metab Cardiovasc Dis. (2010) 20:647–55. doi: 10.1016/j.numecd.2009.05.014
35. Röhrl C, Stangl H. HDL endocytosis and resecretion. Biochim Biophys Acta Mol Cell Biol Lipids. (2013) 1831:1626–33. doi: 10.1016/j.bbalip.2013.07.014
36. Rifler J-P, Lorcerie F, Durand P, Delmas D, Ragot K, Limagne E, et al. A moderate red wine intake improves blood lipid parameters and erythrocytes membrane fluidity in post myocardial infarct patients. Mol Nutr Food Res. (2012) 56:345–51. doi: 10.1002/mnfr.760
37. Droste DW, Iliescu C, Vaillant M, Gantenbein M, De Bremaeker N, Lieunard C, et al. A daily glass of red wine associated with lifestyle changes independently improves blood lipids in patients with carotid arteriosclerosis: results from a randomized controlled trial. Nutr J. (2013) 12:147. doi: 10.1186/1475-2891-12-147
38. Sahebkar A. Effects of resveratrol supplementation on plasma lipids: a systematic review and meta-analysis of randomized controlled trials. Nutr Rev. (2013) 71:822–35. doi: 10.1111/nure.12081
39. Wallace TC, Slavin M, Frankenfeld CL. Systematic review of anthocyanins and markers of cardiovascular disease. Nutrients. (2016) 8:32. doi: 10.3390/nu8010032
40. Li H, Förstermann U. Red wine and cardiovascular health. Circ Res. (2012) 111:959–61. doi: 10.1161/circresaha.112.278705
41. Chondrogianni N, Kapeta S, Chinou I, Vassilatou K, Papassideri I, Gonos ES. Anti-ageing and rejuvenating effects of quercetin. Exp Gerontol. (2010) 45:763–71. doi: 10.1016/j.exger.2010.07.001
42. Kim YA, Kim GY, Park KY, Choi YH. Resveratrol inhibits nitric oxide and prostaglandin E2 production by lipopolysaccharide-activated C6 microglia. J Med Food. (2007) 10:218–24. doi: 10.1089/jmf.2006.143
43. Wang Q, Tang XN, Yenari MA. The inflammatory response in stroke. J Neuroimmunol. (2007) 184:53–68.
44. Wang X, Zhu L, Huang J, Yin YG, Kong XQ, Rong QF, et al. Resveratrol-induced augmentation of telomerase activity delays senescence of endothelial progenitor cells. Chin Med J (Engl). (2011) 124:4310–5.
45. Park SJ, Ahmad F, Philp A, Baar K, Williams T, Luo H, et al. Resveratrol ameliorates aging-related metabolic phenotypes by inhibiting cAMP phosphodiesterases. Cell. (2012) 148:421–33. doi: 10.1016/j.cell.2012.01.017
46. Li Z, Pang L, Fang F, Zhang G, Zhang J, Xie M, et al. Resveratrol attenuates brain damage in a rat model of focal cerebral ischemia via up-regulation of hippocampal Bcl-2. Brain Res. (2012) 1450:116–24. doi: 10.1016/j.brainres.2012.02.019
47. Szmitko PE, Verma S. Cardiology patient pages. Red wine and your heart. Circulation. (2005) 111:e10–1. doi: 10.1161/01.CIR.0000151608.29217.62
48. Kennedy DO, Wightman EL, Reay JL, Lietz G, Okello EJ, Wilde A, et al. Effects of resveratrol on cerebral blood flow variables and cognitive performance in humans: a double-blind, placebo-controlled, crossover investigation. Am J Clin Nutr. (2010) 91:1590–7. doi: 10.3945/ajcn.2009.28641
49. Bowers JL, Tyulmenkov VV, Jernigan SC, Klinge CM. Resveratrol acts as a mixed agonist/antagonist for estrogen receptors α and β. Endocrinology. (2000) 141:3657–67. doi: 10.1210/endo.141.10.7721
50. Lisabeth L, Bushnell C. Stroke risk in women: the role of menopause and hormone therapy. Lancet Neurol. (2012) 11:82–91. doi: 10.1016/S1474-4422(11)70269-1
51. Huang W, Chen Z, Wang Q, Lin M, Wu S, Yan Q, et al. Piperine potentiates the antidepressant-like effect of trans-resveratrol: involvement of monoaminergic system. Metab Brain Dis. (2013) 28:585–95. doi: 10.1007/s11011-013-9426-y
52. Ogle WO, Speisman RB, Ormerod BK. Potential of treating age-related depression and cognitive decline with nutraceutical approaches: a mini-review. Gerontology. (2012) 59:23–31. doi: 10.1159/000342208
53. Pathak L, Agrawal Y, Dhir A. Natural polyphenols in the management of major depression. Expert Opin Investig Drugs. (2013) 22:863–80. doi: 10.1517/13543784.2013.794783
54. Decean H, Fischer-Fodor E, Tatomir C, Perde-Schrepler M, Somfelean L, Burz C, et al. Vitis vinifera seeds extract for the modulation of cytosolic factors BAX-α and NF-KB involved in UVB-induced oxidative stress and apoptosis of human skin cells. Clujul Med. (2016) 89:72–81. doi: 10.15386/cjmed-508
55. Kwon JY, Lee KW, Kim JE, Jung SK, Kang NJ, Hwang MK, et al. Delphinidin suppresses ultraviolet B-induced cyclooxygenases-2 expression through inhibition of MAPKK4 and PI-3 kinase. Carcinogenesis. (2009) 30:1932–40. doi: 10.1093/carcin/bgp216
56. Vicentini FTMC, Simi TRM, Del Ciampo JO, Wolga NO, Pitol DL, Iyomasa MM, et al. Quercetin in w/o microemulsion: in vitro and in vivo skin penetration and efficacy against UVB-induced skin damages evaluated in vivo. Eur J Pharm Biopharm. (2008) 69:948–57. doi: 10.1016/j.ejpb.2008.01.012
57. Chen J, Cai D, Zhang Y. Rapid determination of lipid peroxidation using a novel pyridoxamine-participating ferrous oxidation-sulfosalicylic acid spectrophotometric method. Food Chem. (2016) 211:637–44. doi: 10.1016/j.foodchem.2016.05.106
58. Didžiapetriene J, Bublevič J, Smailyte G, Kazbariene B, Stukas R. Significance of blood serum catalase activity and malondialdehyde level for survival prognosis of ovarian cancer patients. Medicina (Kaunas). (2014) 50:204–8. doi: 10.1016/j.medici.2014.09.001
59. Jang M, Cai L, Udeani GO, Slowing KV, Thomas CF, Beecher CWW, et al. Cancer chemopreventive activity of resveratrol, a natural product derived from grapes. Science. (1997) 275:218–20. doi: 10.1126/science.275.5297.218
60. Youn HS, Lee JY, Fitzgerald KA, Young HA, Akira S, Hwang DH. Specific inhibition of MyD88-independent signaling pathways of TLR3 and TLR4 by resveratrol: molecular targets are TBK1 and RIP1 in TRIF complex. J Immunol. (2005) 175:3339–46. doi: 10.4049/jimmunol.175.5.3339
61. Zhu W, Qin W, Zhang K, Rottinghaus GE, Chen YC, Kliethermes B, et al. Trans-resveratrol alters mammary promoter hypermethylation in women at increased risk for breast cancer. Nutr Cancer. (2012) 64:393–400. doi: 10.1080/01635581.2012.654926
62. Wolter F, Ulrich S, Stein J. Molecular mechanisms of the chemopreventive effects of resveratrol and its analogs in colorectal cancer: key role of polyamines? J Nutr. (2004) 134:3219–22. doi: 10.1093/jn/134.12.3219
63. Patel KR, Brown VA, Jones DJL, Britton RG, Hemingway D, Miller AS, et al. Clinical pharmacology of resveratrol and its metabolites in colorectal cancer patients. Cancer Res. (2010) 70:7392–9. doi: 10.1158/0008-5472.CAN-10-2027
64. Vaid M, Prasad R, Singh T, Jones V, Katiyar SK. Grape seed proanthocyanidins reactivate silenced tumor suppressor genes in human skin cancer cells by targeting epigenetic regulators. Toxicol Appl Pharmacol. (2012) 26:122–30. doi: 10.1016/j.taap.2012.06.013
65. Cao HH, Tse AKW, Kwan HY, Yu H, Cheng CY, Su T, et al. Quercetin exerts anti-melanoma activities and inhibits STAT3 signaling. Biochem Pharmacol. (2014) 87:424–34. doi: 10.1016/j.bcp.2013.11.008
66. Ramos S. Cancer chemoprevention and chemotherapy: dietary polyphenols and signalling pathways. Mol Nutr Food Res. (2008) 52:507–26. doi: 10.1002/mnfr.200700326
67. Pandey PR, Okuda H, Watabe M, Pai SK, Liu W, Kobayashi A, et al. Resveratrol suppresses growth of cancer stem-like cells by inhibiting fatty acid synthase. Breast Cancer Res Treat. (2011) 130:387–98. doi: 10.1007/s10549-010-1300-6
68. Mukherjee S, Dudley JI, Das DK. Dose-dependency of resveratrol in providing health benefits. Dose-Response. (2010) 8:478–500. doi: 10.2203/dose-response.09-015.Mukherjee
69. Malik A, Afaq S, Shahid M, Akhtar K, Assiri A. Influence of ellagic acid on prostate cancer cell proliferation: a caspase-dependent pathway. Asian Pac J Trop Med. (2011) 4:550–5. doi: 10.1016/S1995-7645(11)60144-2
70. Yun JM, Afaq F, Khan N, Mukhtar H. Delphinidin, an anthocyanidin in pigmented fruits and vegetables, induces apoptosis and cell cycle arrest in human colon cancer HCT116 cells. Mol Carcinog. (2009) 48:260–70. doi: 10.1002/mc.20477
71. Feng R, Wang SY, Shi YH, Fan J, Yin XM. Delphinidin induces necrosis in hepatocellular carcinoma cells in the presence of 3-methyladenine, an autophagy inhibitor. J Agric Food Chem. (2010) 58:3957–64. doi: 10.1021/jf9025458
72. Hafeez B, Siddiqui IA, Asim M, Malik A, Afaq F, Adhami VM, et al. A dietary anthocyanidin delphinidin induces apoptosis of human prostate cancer PC3 cells in vitro and in vivo: involvement of nuclear factor-κB signaling. Cancer Res. (2008) 68:8564–72. doi: 10.1158/0008-5472.CAN-08-2232
73. Prasad R, Katiyar SK. Grape seed proanthocyanidins inhibit migration potential of pancreatic cancer cells by promoting mesenchymal-to-epithelial transition and targeting NF-κB. Cancer Lett. (2013) 334:118–26. doi: 10.1016/j.canlet.2012.08.003
74. Cvorovic J, Tramer F, Granzotto M, Candussio L, Decorti G, Passamonti S. Oxidative stress-based cytotoxicity of delphinidin and cyanidin in colon cancer cells. Arch Biochem Biophys. (2010) 501:151–7. doi: 10.1016/j.abb.2010.05.019
75. Aires V, Limagne E, Cotte AK, Latruffe N, Ghiringhelli F, Delmas D. Resveratrol metabolites inhibit human metastatic colon cancer cells progression and synergize with chemotherapeutic drugs to induce cell death. Mol Nutr Food Res. (2013) 57:1170–81. doi: 10.1002/mnfr.201200766
76. Singh CK, Pitschmann A, Ahmad N. Resveratrol-zinc combination for prostate cancer management. Cell Cycle. (2014) 13:1867–74. doi: 10.4161/cc.29334
77. Daglia M, Stauder M, Papetti A, Signoretto C, Giusto G, Canepari P, et al. Isolation of red wine components with anti-adhesion and anti-biofilm activity against Streptococcus mutans. Food Chem. (2010) 119:1182–8. doi: 10.1016/j.foodchem.2009.08.037
78. Thimothe J, Bonsi IA, Padilla-Zakour OI, Koo H. Chemical characterization of red wine grape (Vitis vinifera and Vitis interspecific hybrids) and pomace phenolic extracts and their biological activity against Streptococcus mutans. J Agric Food Chem. (2007) 55:10200–7. doi: 10.1021/jf0722405
79. Muñoz-González I, Thurnheer T, Bartolomé B, Moreno-Arribas MV. Red wine and oenological extracts display antimicrobial effects in an oral bacteria biofilm model. J Agric Food Chem. (2014) 62:4731–7. doi: 10.1021/jf501768p
80. Napimoga MH, Clemente-Napimoga JT, Macedo CG, Freitas FF, Stipp RN, Pinho-Ribeiro FA, et al. Quercetin inhibits inflammatory bone resorption in a mouse periodontitis model. J Nat Prod. (2013) 76:2316–21. doi: 10.1021/np400691n
81. Tamaki N, Orihuela-Campos R, Inagaki Y, Fukui M, Nagata T, Ito HO. Resveratrol improves oxidative stress and prevents the progression of periodontitis via the activation of the Sirt1/AMPK and the Nrf2/antioxidant defense pathways in a rat periodontitis model. Free Radic Biol Med. (2014) 75:222–9. doi: 10.1016/j.freeradbiomed.2014.07.034
82. Boto-Ordóñez M, Urpi-Sarda M, Queipo-Ortuño MI, Tulipani S, Tinahones FJ, Andres-Lacueva C. High levels of bifidobacteria are associated with increased levels of anthocyanin microbial metabolites: a randomized clinical trial. Food Funct. (2014) 5:1932–8. doi: 10.1039/c4fo00029c
83. Smulders FJM, Norrung B, Budka H. (eds). Foodborne Viruses and Prions and their Significance for Public Health. (Vol. 13). Wageningen: Wageningen Academic Publishers (2013). p. 97–97.
84. Ono K, Nakane H. Mechanisms of inhibition of various cellular DNA and RNA polymerases by several flavonoids. J Biochem. (1990) 108:609–13. doi: 10.1093/oxfordjournals.jbchem.a123251
85. Wu Y, Crich D, Pegan SD, Lou L, Hansen MC, Booth C, et al. Polyphenols as potential inhibitors of SARS-CoV-2 RNA dependent RNA polymerase (RdRp). Molecules. (2021) 26:7438. doi: 10.3390/molecules26247438
86. Wang S-C, Chen Y, Wang Y-C, Wang W-J, Yang C-S, Tsai C-L, et al. Tannic acid suppresses SARS-CoV-2 as a dual inhibitor of the viral main protease and the cellular TMPRSS2 protease. Am J Cancer Res. (2020) 10:4538–46.
87. Shohan M, Nashibi R, Mahmoudian-Sani MR, Abolnezhadian F, Ghafourian M, Alavi SM, et al. The therapeutic efficacy of quercetin in combination with antiviral drugs in hospitalized COVID-19 patients: a randomized controlled trial. Eur J Pharmacol. (2022) 914:174615. doi: 10.1016/j.ejphar.2021.174615
88. Clouser CL, Chauhan J, Bess MA, Van Oploo JL, Zhou D, Dimick-Gray S, et al. Anti-HIV-1 activity of resveratrol derivatives and synergistic inhibition of HIV-1 by the combination of resveratrol and decitabine. Bioorganic Med Chem Lett. (2012) 22:6642–6. doi: 10.1016/j.bmcl.2012.08.108
89. Mittra I, de Souza R, Bhadade R, Madke T, Shankpal PD, Joshi M, et al. Resveratrol and copper for treatment of severe COVID-19: an observational study (RESCU 002). medRxiv [Preprint]. (2020). doi: 10.1101/2020.07.21.20151423v1
90. Stockley CS, Høj PB. Better wine for better health: Fact or fiction? Aust J Grape Wine Res. (2005) 11:127–38. doi: 10.1111/j.1755-0238.2005.tb00284.x
91. Merino-Miñano MF, Luján-Herrera GE, Vejarano R. Thermal stability of anthocyanins in grape skin extracts from red winemaking residues. In: Proceedings of the 6th Brazilian Technology Symposium (BTSym’20). BTSym 2020 Smart Innovation, Systems and Technologies [Internet]. Cham: Springer (2021). p. 233
92. Federico Casassa L, Bolcato EA, Sari SE, Fanzone ML, Jofré VP. Combined effect of prefermentative cold soak and SO2 additions in barbera D’Asti and Malbec wines: anthocyanin composition, chromatic and sensory properties. LWT Food Sci Technol. (2016) 66:134–42. doi: 10.1016/j.lwt.2015.10.026
93. Kostadinović S, Wilkens A, Stefova M, Ivanova V, Vojnoski B, Mirhosseini H, et al. Stilbene levels and antioxidant activity of Vranec and Merlot wines from Macedonia: effect of variety and enological practices. Food Chem. (2012) 135:3003–9. doi: 10.1016/j.foodchem.2012.06.118
94. Morata A, Guamis B. Use of UHPH to obtain juices with better nutritional quality and healthier wines with low levels of SO2. Front Nutr. (2020) 7:598286. doi: 10.3389/fnut.2020.598286
95. Guerrero RF, Cantos-Villar E. Demonstrating the efficiency of sulphur dioxide replacements in wine: a parameter review. Trends Food Sci Technol. (2015) 42:27–43. doi: 10.1016/j.tifs.2014.11.004
96. Raposo R, Ruiz-Moreno MJ, Garde-Cerdán T, Puertas B, Moreno-Rojas JM, Zafrilla P, et al. Replacement of sulfur dioxide by hydroxytyrosol in white wine: Influence on both quality parameters and sensory. LWT Food Sci Technol. (2016) 65:214–21. doi: 10.1016/j.lwt.2015.08.005
97. Goldberg DM, Ng E, Karumanchiri A, Diamandis EP, Soleas GJ. Resveratrol glucosides are important components of commercial wines. Am J Enol Vitic. (1996) 47:415–20.
98. Morata A, Escott C, Loira I, López C, Palomero F, González C. Emerging non-thermal technologies for the extraction of grape anthocyanins. Antioxidants. (2021) 10:1863. doi: 10.3390/antiox10121863
99. Rapeanu G, Van Loey A, Smout C, Hendrickx M. Thermal and high pressure inactivation kinetics of Victoria grape polyphenol oxidase: from model systems to grape must. J Food Process Eng. (2006) 29:269–86. doi: 10.1111/j.1745-4530.2006.00063.x
100. Morata A, Loira I, Vejarano R, González C, Callejo MJ, Suárez-Lepe JA. Emerging preservation technologies in grapes for winemaking. Trends Food Sci Technol. (2017) 67:36–43. doi: 10.1016/j.tifs.2017.06.014
101. Morata A, Loira I, Vejarano R, Bañuelos MA, Sanz PD, Otero L, et al. Grape processing by high hydrostatic pressure: effect on microbial populations, phenol extraction and wine quality. Food Bioprocess Technol. (2015) 8:277–86. doi: 10.1007/s11947-014-1405-8
102. Corrales M, Toepfl S, Butz P, Knorr D, Tauscher B. Extraction of anthocyanins from grape by-products assisted by ultrasonics, high hydrostatic pressure or pulsed electric fields: a comparison. Innov Food Sci Emerg Technol. (2008) 9:85–91. doi: 10.1016/j.ifset.2007.06.002
103. Corrales M, Butz P, Tauscher B. Anthocyanin condensation reactions under high hydrostatic pressure. Food Chem. (2008) 110:627–35. doi: 10.1016/j.foodchem.2008.02.055
104. Santos MC, Nunes C, Ferreira AS, Jourdes M, Teissedre PL, Rodrigues A, et al. Comparison of high pressure treatment with conventional red wine aging processes: impact on phenolic composition. Food Res Int. (2019) 116:223–31. doi: 10.1016/j.foodres.2018.08.018
105. Escott C, Vaquero C, del Fresno JM, Bañuelos MA, Loira I, Han S, et al. Pulsed light effect in red grape quality and fermentation. Food Bioprocess Technol. (2017) 10:1540–7. doi: 10.1007/s11947-017-1921-4
106. Christofi S, Malliaris D, Katsaros G, Panagou E, Kallithraka S. Limit SO2 content of wines by applying high hydrostatic pressure. Innov Food Sci Emerg Technol. (2020) 62:102342. doi: 10.1016/j.ifset.2020.102342
107. Bañuelos MA, Loira I, Guamis B, Escott C, Del Fresno JM, Codina-Torrella I, et al. White wine processing by UHPH without SO2. Elimination of microbial populations and effect in oxidative enzymes, colloidal stability and sensory quality. Food Chem. (2020) 332:127417. doi: 10.1016/j.foodchem.2020.127417
108. Comuzzo P, Calligaris S. Potential applications of high pressure homogenization in winemaking: a review. Beverages. (2019) 5:56. doi: 10.3390/beverages5030056
109. Loira I, Morata A, Bañuelos MA, Puig-Pujol A, Guamis B, González C, et al. Use of ultra-high pressure homogenization processing in winemaking: control of microbial populations in grape musts and effects in sensory quality. Innov Food Sci Emerg Technol. (2018) 50:50–6. doi: 10.1016/j.ifset.2018.10.005
110. Vaquero C, Escott C, Loira I, Guamis B, del Fresno JM, Quevedo JM, et al. Cabernet Sauvignon red must processing by UHPH to produce wine without SO2: the colloidal structure, microbial and oxidation control, colour protection and sensory quality of the wine. Food Bioprocess Technol. (2022) 15:620–34. doi: 10.1007/s11947-022-02766-8
111. Vejarano R. Non-Saccharomyces in winemaking: source of mannoproteins, nitrogen, enzymes, and antimicrobial compounds. Fermentation. (2020) 6:76. doi: 10.3390/fermentation6030076
112. Vejarano R, Gil-Calderón A. Commercially available non-Saccharomyces yeasts for winemaking: current market, advantages over Saccharomyces, biocompatibility, and safety. Fermentation. (2021) 7:171. doi: 10.3390/fermentation7030171
113. Harte F. Food processing by high-pressure homogenization. In: V Balasubramaniam, G Barbosa-Cánovas, H Lelieveld editors. Food Engineering Series [Internet]. New York, NY: Springer (2016). p. 123–41. doi: 10.1007/978-1-4939-3234-4_7
114. Puig A, Olmos P, Quevedo JM, Guamis B, Mínguez S. Microbiological and sensory effects of musts treated by high-pressure homogenization. Food Sci Technol Int. (2008) 14(Suppl. 5):5–11.
115. Vaquero C, Loira I, Raso J, Álvarez I, Delso C, Morata A. Pulsed electric fields to improve the use of non-saccharomyces starters in red wines. Foods. (2021) 10:1472. doi: 10.3390/foods10071472
116. Leong SY, Treadwell M, Liu T, Hochberg M, Sack M, Mueller G, et al. Influence of pulsed electric fields processing at high-intensity electric field strength on the relationship between anthocyanins composition and colour intensity of Merlot (Vitis vinifera L.) musts during cold maceration. Innov Food Sci Emerg Technol. (2020) 59:102243. doi: 10.1016/j.ifset.2019.102243
117. López N, Puértolas E, Condón S, Álvarez I, Raso J. Application of pulsed electric fields for improving the maceration process during vinification of red wine: influence of grape variety. Eur Food Res Technol. (2008) 227:1099–107. doi: 10.1016/j.ifset.2016.12.008
118. Maza MA, Martínez JM, Delso C, Camargo A, Raso J, Álvarez I. PEF-dependency on polyphenol extraction during maceration/fermentation of Grenache grapes. Innov Food Sci Emerg Technol. (2020) 60:102303.
119. El Darra N, Grimi N, Maroun RG, Louka N, Vorobiev E. Pulsed electric field, ultrasound, and thermal pretreatments for better phenolic extraction during red fermentation. Eur Food Res Technol. (2013) 236:47–56. doi: 10.1007/s00217-012-1858-9
120. El Darra N, Grimi N, Vorobiev E, Maroun RG, Louka N. Pulsed electric field-assisted cold maceration of Cabernet Franc and Cabernet Sauvignon grapes. Am J Enol Vitic. (2013) 64:476–84. doi: 10.5344/ajev.2013.12098
121. El Darra N, Rajha HN, Ducasse MA, Turk MF, Grimi N, Maroun RG, et al. Effect of pulsed electric field treatment during cold maceration and alcoholic fermentation on major red wine qualitative and quantitative parameters. Food Chem. (2016) 213:352–60. doi: 10.1016/j.foodchem.2016.06.073
122. López-Alfaro I, González-Arenzana L, López N, Santamaría P, López R, Garde-Cerdán T. Pulsed electric field treatment enhanced stilbene content in Graciano, Tempranillo and Grenache grape varieties. Food Chem. (2013) 141:3759–65. doi: 10.1016/j.foodchem.2013.06.082
123. Amri A, Chaumeil JC, Sfar S, Charrueau C. Administration of resveratrol: what formulation solutions to bioavailability limitations? J Control Release. (2012) 158:182–93. doi: 10.1016/j.jconrel.2011.09.083
124. Leong SY, Burritt DJ, Oey I. Evaluation of the anthocyanin release and health-promoting properties of Pinot Noir grape juices after pulsed electric fields. Food Chem. (2016) 196:833–41. doi: 10.1016/j.foodchem.2015.10.025
125. Delsart C, Cholet C, Ghidossi R, Grimi N, Gontier E, Gény L, et al. Effects of pulsed electric fields on Cabernet Sauvignon grape berries and on the characteristics of wines. Food Bioprocess Technol. (2014) 7:424–36. doi: 10.1021/jf904035v
126. Loira I, Vejarano R, Morata A, Ricardo-Da-Silva JM, Laureano O, González MC, et al. Effect of Saccharomyces strains on the quality of red wines aged on lees. Food Chem. (2013) 139:1044–51. doi: 10.1016/j.foodchem.2013.01.020
127. Puértolas E, Saldaña G, Condón S, Álvarez I, Raso J. Evolution of polyphenolic compounds in red wine from Cabernet Sauvignon grapes processed by pulsed electric fields during aging in bottle. Food Chem. (2010) 119:1063–70. doi: 10.1111/j.1750-3841.2009.01343.x
128. Maza MA, Martínez JM, Cebrián G, Sánchez-Gimeno AC, Camargo A, Álvarez I, et al. Evolution of polyphenolic compounds and sensory properties of wines obtained from Grenache grapes treated by pulsed electric fields during aging in bottles and in oak barrels. Foods. (2020) 9:542. doi: 10.3390/foods9050542
129. Comuzzo P, Voce S, Grazioli C, Tubaro F, Marconi M, Zanella G, et al. Pulsed electric field processing of red grapes (cv. Rondinella): modifications of phenolic fraction and effects on wine evolution. Foods. (2020) 9:414. doi: 10.3390/foods9040414
130. Ricci A, Parpinello GP, Banfi BA, Olivi F, Versari A. Preliminary study of the effects of pulsed electric field (PEF) treatments in wines obtained from early-harvested Sangiovese grapes. Beverages. (2020) 6:34. doi: 10.3390/beverages6020034
131. Chemat F, Zill-E-Huma KMK. Applications of ultrasound in food technology: processing, preservation and extraction. Ultrason Sonochem. (2011) 18:813–35. doi: 10.1016/j.ultsonch.2010.11.023
132. Plaza EG, Jurado R, Iniesta JA, Bautista-a-Ortín AB. High power ultrasounds: a powerful, non-thermal and green technique for improving the phenolic extraction from grapes to must during red wine vinification. In: Proceedings of the BIO Web of Conferences. Punta del Este (2019). p. 02001. doi: 10.1051/bioconf/20191202001
133. Tiwari BK, Patras A, Brunton N, Cullen PJ, O’Donnell CP. Effect of ultrasound processing on anthocyanins and color of red grape juice. Ultrason Sonochem. (2010) 17:598–604. doi: 10.1016/j.ultsonch.2009.10.009
134. Osete-Alcaraz A, Bautista-Ortín AB, Ortega-Regules AE, Gómez-Plaza E. Combined use of pectolytic enzymes and ultrasounds for improving the extraction of phenolic compounds during vinification. Food Bioprocess Technol. (2019) 12:1330–9. doi: 10.1007/s11947-019-02303-0
135. Dranca F, Oroian M. Kinetic improvement of bioactive compounds extraction from red grape (Vitis vinifera Moldova) pomace by ultrasonic treatment. Foods. (2019) 8:353. doi: 10.3390/foods8080353
136. Morata A, Bañuelos MA, Tesfaye W, Loira I, Palomero F, Benito S, et al. Electron beam irradiation of wine grapes: effect on microbial populations, phenol extraction and wine quality. Food Bioprocess Technol. (2015) 8:1845–53. doi: 10.1007/s11947-015-1540-x
137. Gupta S, Padole R, Variyar PS, Sharma A. Influence of radiation processing of grapes on wine quality. Radiat Phys Chem. (2015) 111:46–56. doi: 10.1016/j.radphyschem.2015.02.019
138. Augustine S, Kudachikar VB, Vanajakshi V, Ravi R. Effect of combined preservation techniques on the stability and microbial quality and retention of anthocyanins in grape pomace stored at low temperature. J Food Sci Technol. (2013) 50:332–8. doi: 10.1007/s13197-011-0325-0
139. Błaszak M, Nowak A, Lachowicz S, Migdał W, Ochmian I. E-beam irradiation and ozonation as an alternative to the sulphuric method of wine preservation. Molecules. (2019) 24:3406. doi: 10.3390/molecules24183406
140. Bellincontro A, Catelli C, Cotarella R, Mencarelli F. Postharvest ozone fumigation of Petit Verdot grapes to prevent the use of sulfites and to increase anthocyanin in wine. Aust J Grape Wine Res. (2017) 23:200–6. doi: 10.1111/ajgw.12257
141. Paissoni MA, Río Segade S, Giacosa S, Torchio F, Cravero F, Englezos V, et al. Impact of post-harvest ozone treatments on the skin phenolic extractability of red winegrapes cv Barbera and Nebbiolo (Vitis vinifera L.). Food Res Int. (2017) 98:68–78. doi: 10.1016/j.foodres.2016.11.013
142. Botondi R, De Sanctis F, Moscatelli N, Vettraino AM, Catelli C, Mencarelli F. Ozone fumigation for safety and quality of wine grapes in postharvest dehydration. Food Chem. (2015) 188:641–7. doi: 10.1016/j.foodchem.2015.05.029
143. Laureano J, Giacosa S, Río Segade S, Torchio F, Cravero F, Gerbi V, et al. Effects of continuous exposure to ozone gas and electrolyzed water on the skin hardness of table and wine grape varieties. J Texture Stud. (2016) 47:40–8. doi: 10.1111/jtxs.12158
144. Rodoni L, Casadei N, Concellón A, Chaves Alicia AR, Vicente AR. Effect of short-term ozone treatments on tomato (Solanum lycopersicum L.) fruit quality and cell wall degradation. J Agric Food Chem. (2010) 58:594–9. doi: 10.1021/jf9029145
145. González-Neves G, Gil G, Barreiro L. Influence of grape variety on the extraction of anthocyanins during the fermentation on skins. Eur Food Res Technol. (2008) 226:1349–55. doi: 10.1007/s00217-007-0664-2
146. Wightman JD, Wrolstad RE. β-glucosidase activity in juice-processing enzymes based on anthocyanin analysis. J Food Sci. (1996) 61:544–8. doi: 10.1111/j.1365-2621.1996.tb13153.x
147. Barcia MT, Pertuzatti PB, Rodrigues D, Gómez-Alonso S, Hermosín-Gutiérrez I, Godoy HT. Occurrence of low molecular weight phenolics in Vitis vinifera red grape cultivars and their winemaking by-products from São Paulo (Brazil). Food Res Int. (2014) 62:500–13. doi: 10.1016/j.foodres.2014.03.051
148. Morata A, Gómez-Cordovés MC, Suberviola J, Bartolomé B, Colomo B, Suárez-Lepe JA. Adsorption of anthocyanins by yeast cell walls during the fermentation of red wines. J Agric Food Chem. (2003) 51:4084–8. doi: 10.1021/jf021134u
149. Morata A, Gómez-Cordovés MC, Colomo B, Suárez JA. Cell wall anthocyanin adsorption by different Saccharomyces strains during the fermentation of Vitis vinifera L. cv Graciano grapes. Eur Food Res Technol. (2005) 220:341–6. doi: 10.1007/s00217-004-1053-8
150. Weiskirchen S, Weiskirchen R. Resveratrol: how much wine do you have to drink to stay healthy? Adv Nutr. (2016) 7:706–18. doi: 10.3945/an.115.011627
151. Escott C, López C, Loira I, González C, Bañuelos MA, Tesfaye W, et al. Improvement of must fermentation from late harvest cv. Tempranillo grapes treated with pulsed light. Foods. (2021) 10:1416. doi: 10.3390/foods10061416
152. Morata A, Vejarano R, Ridolfi G, Benito S, Palomero F, Uthurry C, et al. Reduction of 4-ethylphenol production in red wines using HCDC+ yeasts and cinnamyl esterases. Enzyme Microb Technol. (2013) 52:99–104. doi: 10.1016/j.enzmictec.2012.11.001
153. Suárez-Lepe JA, Morata A. New trends in yeast selection for winemaking. Trends Food Sci Technol. (2012) 23:39–50. doi: 10.1016/j.tifs.2011.08.005
154. Suárez R, Suárez-Lepe JA, Morata A, Calderón F. The production of ethylphenols in wine by yeasts of the genera Brettanomyces and Dekkera: a review. Food Chem. (2007) 102:10–21. doi: 10.1016/j.foodchem.2006.03.030
155. Mansfield AK, Zoecklein BW, Whiton RS. Quantification of glycosidase activity in selected strains of Brettanomyces bruxellensis and Oenococcus oeni. Am J Enol Vitic. (2002) 53:303–7.
156. Benito S, Palomero F, Morata A, Uthurry C, Suárez-Lepe JA. Minimization of ethylphenol precursors in red wines via the formation of pyranoanthocyanins by selected yeasts. Int J Food Microbiol. (2009) 132:145–52. doi: 10.1016/j.ijfoodmicro.2009.04.015
157. García-Alonso M, Rimbach G, Rivas-Gonzalo JC, De Pascual-Teresa S. Antioxidant and cellular activities of anthocyanins and their corresponding vitisins A – Studies in platelets, monocytes, and human endothelial cells. J Agric Food Chem. (2004) 52:3378–84. doi: 10.1021/jf035360v
158. McDougall GJ, Fyffe S, Dobson P, Stewart D. Anthocyanins from red wine – Their stability under simulated gastrointestinal digestion. Phytochemistry. (2005) 66:2540–8. doi: 10.1016/j.phytochem.2005.09.003
159. Benito S, Morata A, Palomero F, del Carmen González M, Suárez-Lepe JA. Formation of vinylphenolic pyranoanthocyanins by Saccharomyces cerevisiae and Pichia guillermondii in red wines produced following different fermentation strategies. Food Chem. (2011) 124:15–23. doi: 10.1016/j.foodchem.2010.05.096
160. Bañuelos MA, Loira I, Escott C, Del Fresno JM, Morata A, Sanz PD, et al. Grape processing by high hydrostatic pressure: effect on use of non-Saccharomyces in must fermentation. Food Bioprocess Technol. (2016) 9:1769–78. doi: 10.1007/s11947-016-1760-8
161. Loira I, Morata A, Comuzzo P, Callejo MJ, González C, Calderón F, et al. Use of Schizosaccharomyces pombe and Torulaspora delbrueckii strains in mixed and sequential fermentations to improve red wine sensory quality. Food Res Int. (2015) 76:325–33. doi: 10.1016/j.foodres.2015.06.030
162. Vejarano R. Saccharomycodes ludwigii, control and potential uses in winemaking processes. Fermentation. (2018) 4:71. doi: 10.3390/fermentation4030071
163. Domizio P, Romani C, Lencioni L, Comitini F, Gobbi M, Mannazzu I, et al. Outlining a future for non-Saccharomyces yeasts: Selection of putative spoilage wine strains to be used in association with Saccharomyces cerevisiae for grape juice fermentation. Int J Food Microbiol. (2011) 147:170–80. doi: 10.1016/j.ijfoodmicro.2011.03.020
164. Abalos D, Vejarano R, Morata A, González C, Suárez-Lepe JA. The use of furfural as a metabolic inhibitor for reducing the alcohol content of model wines. Eur Food Res Technol. (2011) 232:663–9. doi: 10.1007/s00217-011-1433-9
165. Vejarano R, Morata A, Loira I, González MC, Suárez-Lepe JA. Theoretical considerations about usage of metabolic inhibitors as possible alternative to reduce alcohol content of wines from hot areas. Eur Food Res Technol. (2013) 237:281–90. doi: 10.1007/s00217-013-1992-z
166. Modig T, Lidén G, Taherzadeh MJ. Inhibition effects of furfural on alcohol dehydrogenase, aldehyde dehydrogenase and pyruvate dehydrogenase. Biochem J. (2002) 363:769–76. doi: 10.1042/0264-6021:3630769
167. Monagas M, Bartolomé B, Gómez-Cordovés C. Evolution of polyphenols in red wines from Vitis vinifera L. during aging in the bottle. Eur Food Res Technol. (2005) 220:331–40. doi: 10.1007/s00217-004-1109-9
168. Rice-Evans C, Miller NJ. Total antioxidant status in plasma and body fluids. Methods Enzymol. (1994) 234:279–93. doi: 10.1016/0076-6879(94)34095-1
169. Palomero F, Morata A, Benito S, Calderón F, Suárez-Lepe JA. New genera of yeasts for over-lees aging of red wine. Food Chem. (2009) 112:432–41. doi: 10.1016/j.foodchem.2008.05.098
170. Salmon JM. Interactions between yeast, oxygen and polyphenols during alcoholic fermentations: practical implications. LWT Food Sci Technol. (2006) 39:959–65. doi: 10.1016/j.lwt.2005.11.005
171. Yue XF, Jing SS, Ni XF, Zhang KK, Fang YL, Zhang ZW, et al. Anthocyanin and phenolic acids contents influence the color stability and antioxidant capacity of wine treated with mannoprotein. Front Nutr. (2021) 8:691784. doi: 10.3389/fnut.2021.691784
172. Industrial Sonomechanics Llc. Food & Beverage [Internet]. (2022). Available online at: https://www.sonomechanics.com/food-beverages/ (accessed April 11, 2022).
173. Elea Technology. Find the Right Elea PEF Advantage System for your Wine Production [Internet]. (2022). Available online at: https://elea-technology.com/systems/ (accessed April 11, 2022).
174. Luengo E, Franco E, Ballesteros F, Álvarez I, Raso J. Winery trial on application of pulsed electric fields for improving vinification of Garnacha grapes. Food Bioprocess Technol. (2014) 7:1457–64. doi: 10.1007/s11947-013-1209-2
175. Homogenising Systems Ltd. Ultra High Pressure Homogenizers [Internet]. (2022). Available online at: https://www.homogenisingsystems.com/index.html (accessed April 11, 2022).
176. Guamis-Lopez B, Trujillo-Mesa AJ, Ferragut-Pérez V, Quevedo-Terré JM, Lopez-Pedemonte T, Buffa-Dunat MN. Continuous System and Procedure of Sterilization and Physical Stabilization of Pumpable Fluids by Means of Ultrahigh Pressure Homogenization. Spain Patent Application PCT/EP2011/003572. Barcelona: Universidad Autónoma de Barcelona (2012).
177. Ypsicon Advanced Technologies S.L. Applications for wine [Internet]. (2022). Available online at: https://www.ypsicon.com/main.asp?Familia=174&Subfamilia=280&cerca=familia&pag=1 (accessed April 11, 2022).
178. Trichopoulou A, Bamia C, Trichopoulos D. Anatomy of health effects of mediterranean diet: Greek EPIC prospective cohort study. BMJ. (2009) 338:b2337. doi: 10.1136/bmj.b2337
179. Puértolas E, López N, Saldaña G, Álvarez I, Raso J. Evaluation of phenolic extraction during fermentation of red grapes treated by a continuous pulsed electric fields process at pilot-plant scale. J Food Eng. (2010) 98:120–5. doi: 10.1016/j.jfoodeng.2009.12.017
180. Vicaş SI, Bandici L, Teuşdea AC, Turcin V, Popa D, Bandici GE. The bioactive compounds, antioxidant capacity, and color intensity in must and wines derived from grapes processed by pulsed electric field. CyTA J Food. (2017) 15:553–62. doi: 10.1080/19476337.2017.1317667
181. González M, Barrios S, Budelli E, Pérez N, Lema P, Heinzen H. Ultrasound assisted extraction of bioactive compounds in fresh and freeze-dried Vitis viniIIfera cv Tannat grape pomace. Food Bioprod Process. (2020) 124:378–86. doi: 10.1016/j.fbp.2020.09.012
182. Benito Á, Calderón F, Benito S. The combined use of Schizosaccharomyces pombe and Lachancea thermotolerans – effect on the anthocyanin wine composition. Molecules. (2017) 22:739. doi: 10.3390/molecules22050739
Keywords: red wine, polyphenols, antioxidant, anti-inflammatory, disease prevention, emerging technologies, extraction
Citation: Vejarano R and Luján-Corro M (2022) Red Wine and Health: Approaches to Improve the Phenolic Content During Winemaking. Front. Nutr. 9:890066. doi: 10.3389/fnut.2022.890066
Received: 05 March 2022; Accepted: 05 May 2022;
Published: 25 May 2022.
Edited by:
Antonio Morata, Polytechnic University of Madrid, SpainReviewed by:
Marcos Maza, Universidad Nacional de Cuyo, ArgentinaCopyright © 2022 Vejarano and Luján-Corro. This is an open-access article distributed under the terms of the Creative Commons Attribution License (CC BY). The use, distribution or reproduction in other forums is permitted, provided the original author(s) and the copyright owner(s) are credited and that the original publication in this journal is cited, in accordance with accepted academic practice. No use, distribution or reproduction is permitted which does not comply with these terms.
*Correspondence: Ricardo Vejarano, cmljYXJkby52ZWphcmFub0B1cG4uZWR1LnBl
Disclaimer: All claims expressed in this article are solely those of the authors and do not necessarily represent those of their affiliated organizations, or those of the publisher, the editors and the reviewers. Any product that may be evaluated in this article or claim that may be made by its manufacturer is not guaranteed or endorsed by the publisher.
Research integrity at Frontiers
Learn more about the work of our research integrity team to safeguard the quality of each article we publish.