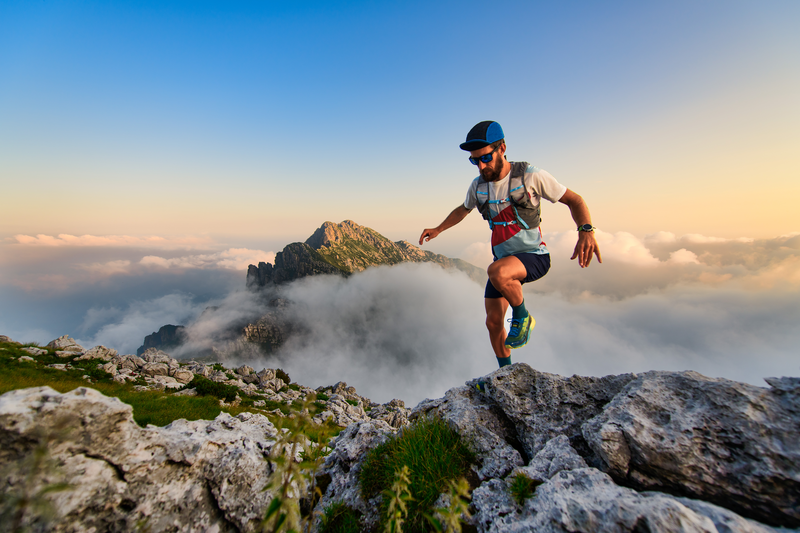
94% of researchers rate our articles as excellent or good
Learn more about the work of our research integrity team to safeguard the quality of each article we publish.
Find out more
ORIGINAL RESEARCH article
Front. Nutr. , 10 May 2022
Sec. Nutrition and Microbes
Volume 9 - 2022 | https://doi.org/10.3389/fnut.2022.888580
Salmonella enterica serovar Enteritidis (SE) is one of the most common pathogens associated with poultry health and foodborne Salmonellosis worldwide. The gut plays a pivotal role in inhibiting SE transintestinal transmission and contaminating poultry products. The nutritional status of vitamin D (VD) is involved in gut health apart from bone health. However, the impact of VD3 nutritional status on the gut health of Salmonella-challenged hens is rarely investigated. This study investigated the impact and possible mechanisms of VD3 nutritional status on the gut health of hens challenged with SE. Hens were fed basal diets with either 0 (deficient) or 3000 IU (sufficient) VD3/kg of diet, respectively. After 10 weeks of feeding, half of the hens were orally inoculated with either SE (1 × 109 CFU /bird). Results indicated that VD3 sufficiency reversed the disruptive effects on the laying performance of hens caused by Salmonella challenge or VD3 insufficiency by promoting VD3 metabolism. In addition, VD3 sufficiency ameliorated gut injury induced by either Salmonella or VD3 deficiency, shown by reducing Salmonella load and histopathological scores, suppressing TLR4-mediated inflammatory responses, and increasing expression of TJs along with decreasing pro-apoptotic protein expression and the number of TUNEL-positive cells in the jejunum. Besides, VD3 enriched the abundance of probiotics, such as Lactobacillus and Bacilli, and restored the balance of gut microflora. Collectively, dietary VD3 sufficient supplementation could alleviate Salmonella or VD3 deficiency-induced intestinal damage of hens via modulating intestinal immune, barrier function, apoptosis along with gut microbiota composition, revealing that VD3 could act as a novel nutritional strategy defending Salmonella invasion in hens.
Salmonella enterica serovar Enteritidis (S. Enteritidis, SE), one of the most prevalent serotypes of Salmonella, is a common foodborne pathogen that enters the human food chain through animal products, particularly raw poultry products resulting in human salmonellosis worldwide (1). S. Enteritidis infection in chicks can result in asymptomatic cecal colonization, gut dysbiosis, intestinal mild inflammation (2, 3), and gut barrier damage along with the invasion of internal organs and poultry carcasses (4). In addition, its infection on hens would induce SE transintestinal transmission and colonizing reproductive tissues (ovaries, oviduct, and testis), and persistent shedding of S. enteritidis in feces and eggs laid by infected hens (5). The report revealed that more than 90% of human salmonellosis was related to the Salmonella contamination in eggs and chicken, of which SE is the most frequent serotype inducing human salmonellosis (6, 7). Thus, infected chickens and contaminated poultry products are one of the most important carriers of SE threatening human health, which was considered to be one of the major sources of human salmonellosis worldwide (8). Therefore, reducing S. Enteritidis colonization and invasion in the intestinal tract of chickens, maintaining gut barrier integrity and intestinal microbial flora balance, along with improving intestinal mucosal immune defense ability would prevent Salmonella to invade the internal organs, ovaries, oviduct, and eggs and help minimize the contamination of poultry products and salmonellosis in humans.
Contamination of poultry products caused by Salmonella infection occurred frequently in the poultry industry. Biosecurity management and pharmaceutical approaches, such as vaccination, competitive exclusion, and antibiotic treatments, can help reduce the S. Enteritidis burden in poultry (2, 9). However, the use of in-feed growth-promoting antibiotics was gradually banned in recent decades, and the use of therapeutic antibiotics in aquaculture is also been reduced due to the risks associated with antibiotics use in some countries or areas (10). Supplemented with non-antibiotic and non-nutritional additives, such as probiotics, prebiotics, organic acids, short and medium-chain fatty acids, essential oils, nitro-compounds, and bacteriophages, in feed or drinking water to control Salmonella infection become popular on farms. Methods above were reported to help hosts resistance against Salmonella infection via enhancing innate immune functions and inhibiting inflammatory responses, modulating the balance of intestinal microbiota and competitively eliminating Salmonella colonization, repairing damaged intestinal barrier structure, killing directly or inhibiting indirectly the colonization, growth, and organ invasion of Salmonella ultimately (11–13). Meanwhile, dietary interventions with fortified nutrients (vitamins, minerals) were also suggested to enhance innate immune response and disease resistance against Salmonella infection in hens (14).
Vitamin D (VD) is a fat-soluble vitamin and pleiotropic hormone that has conventional roles in modulating calcium and phosphate absorption in the intestine, sustaining the homeostasis of calcium and phosphate, and bone mineralization along with skeletal health (15). Interestingly, in the past decades, accumulating data has shown that VD possessed several extra-skeletal effects, such as immunomodulatory, anti-inflammatory, anti-viral, anti-oxidative, anti-allergy, anti-apoptosis actions, and cancer prevention, through VDR-mediated regulation on gene expressions, mainly because VDR and/or VD 1α-hydroxylase were found to express in various tissues and cells (16, 17). Also, growing pieces of evidence from mammals' experiments have demonstrated that VD and VDR have an important role in maintaining gut homeostasis through enhancing the intestinal mucosal barrier integrity, sensing gut microbiota, preventing pathogens colonization and invasion, modulating innate and acquired immunity in the gut together with suppressing gut inflammation (18). VD deficiency or lack of VDR results in intestinal microbiota dysbiosis, inflammation, and barrier impairment along with increased susceptibility to infection and mucosal damage as well as disease exacerbation (19). It was suggested that a VD supplement could suppress intestinal mucosal inflammation and ameliorate intestinal injury by promoting antimicrobial peptides expressions, maintaining gut microflora eubiosis, along with enhancing tight junction (TJ) proteins expression (20, 21). Ulteriorly, supplementation with VD3 or its active form has been a beneficial approach to control enteric pathogens, such as Helicobacter pylori, Clostridium difficile, Salmonella, and Citrobacter rodentium infection and inflammatory bowel disease in mammals (22). Thus, the maintenance of an adequate VD status seems to provide a great preventive health potential.
Vitamin D is also one of the key dietary factors for hens. The essentiality of VD in hen feed is particularly significant for profitable egg and meat production in the modern poultry industry where the birds are raised indoors (23). VD3 has traditionally been used as a major source of VD by the poultry industry. Official recommendations the nutrient requirement for normal egg production and shell quality is 500 IU of cholecalciferol/kg feed. In China, the recommended common levels of supplemented VD3 specified by the China National Standardization Management Committee (NY/T33-2004) is 1,600 IU/kg feed for commercial laying hens, 2,000 IU/kg in diets for the commercial egg breeders, while 3,000–5,000 IU/kg diet is recommended by commercial producers. When laying hens are fed a diet deficient or completely devoid of VD3, the signs of deficiency are characterized by thin eggshells, repressed egg production, cracked eggshells, elevated mortality of birds, and low Ca and P utilization alone with skeletal diseases, and welfare issues (23). It was demonstrated VD deficiency aggravated the CD8+ T cell deficiency, decreased cell-mediated immune responses, and impaired macrophages development, resulting in higher susceptibility to pathogen infection and ultimately decreased growth performance in poultry (24). Additionally, previous studies showed that VD3 or its activated form (25-hydroxyvitamin D3) could serve as prophylactic ways to control pathogens infection and alleviate negative effects caused by pathogens, such as Salmonella and Coccidia infection in broilers (25).
Although, the above research showed that VD3 could serve as a prophylactic or therapeutic way to control enteric inflammation and pathogens infection in humans and broilers. However, to date, there are few studies investigating the influences of VD3 nutritional status on gut health of the Salmonella enteritis–challenged hens. Therefore, the objective of this study was to evaluate the impact of VD3 nutritional status on gut health of the Salmonella-challenged laying hens by determining intestinal Salmonella load, intestinal pathological changes, barrier function, apoptotic status, and immune function as well as the gut microbiome, to reveal the association between VD3 status and Salmonella resistance of laying hens, providing a feasible nutritional approach to mitigate the deleterious impacts on hens health caused by Salmonella infection and reduce the risk of Salmonella pollution on poultry products.
All the procedures implemented in this study were approved by the China Agricultural University Animal Care and Use Committee (statement no. CAU20170601-2). All efforts were made to minimize animal suffering.
A total of 144 Salmonella-negative Jinghong-1 strain layers at 32 weeks of age (a China local layer breed) were selected and randomly assigned into two groups, with 12 replicates per group and six birds per replicate. Hens were fed a corn-soybean-based meal mash diet without supplemental cholecalciferol (0 IU cholecalciferol /kg diet; VD3-deficient group) and the basal diet contained 3,000 IU cholecalciferol per kg diet (VD3-sufficient group) for 10 weeks, respectively. After 10 weeks of pre-feed, half of the hens in each group were orally gavaged with Salmonella enterica (1 × 109 CFU /bird), whereas another half of the birds with sterile medium. All hens were re-assigned to 4 groups based on VD3 and Salmonella treatment, containing hens fed a VD-deficient diet with or without Salmonella challenge (group VD0S and VD0), and hens fed the VD-sufficient groups challenged with or without Salmonella (group VD3000S and VD3000). All infected hens (group VD0S and VD3000S) were reared in a separate room to avoid cross-infection. The basal diet was formulated to meet or exceeded the Chinese Feeding Standard of Chickens (NY/T33-2004) and nutrient requirements of laying hens except for VD3. The basal composition and nutrient levels of the diet are presented in Table 1. Hens were housed in climate-controlled experimental layer rooms and kept in 3-layer complete ladder cages at three birds per cage (40 cm × 45 cm × 45 cm) equipped with water nipples, respectively. All birds were fed ad libitum and allowed to access water freely throughout the entire experimental period. The lighting schedule was maintained on a cycle of 16 h light and 8 h dark. The average house temperature in the chicken house was 24.5 ± 0.5°C. The infection trial lasted for 2 weeks.
The egg number and total egg weight of each replicate pen were recorded daily, and the feed intake of each replicate was determined weekly. The laying rate, average egg weight, average feed intake, and feed to egg ratio were calculated before (from 1 to 10 weeks) and after SE infection (from 11 to 12 weeks). Hens were monitored twice daily for clinical expression of disease. Mortalities were recorded daily for 2 weeks post-SE infection.
Salmonella enterica strain CVCC3377 was inoculated on Xylose Lysine Deoxycholate (XLD) Agar at 37°C for 24 h. The single colonies were selected and inoculated into Luria-Bertani (LB) broth overnight at 37°C with shaking. Inoculation dose dilutions of SE were made based on a concentration estimated from the optical density at 600 nm applied to an SE growth curve regression equation. Actual SE inoculation doses were then confirmed by serial plate dilutions of the inoculum.
On 7 days post-infection (dpi), one bird per replicate was randomly selected, blood samples were collected via wing venipuncture and clotted at room temperature, and then centrifuged at 3,000 × g for 5 min at 4°C to collect serum for calcium and 25-OH-VD measure. After the blood collection, all birds were killed by cervical dislocation, the middle segments of the jejunum (approximately 1 cm) were cut off and gently rinsed with ice-cold sterile saline to remove internal digesta, and then immediately frozen with liquid nitrogen and stored at −80°C for gene and protein expression determination. Subsequently, the distal jejunum segments were fixed in 4% paraformaldehyde immediately for H&E staining and immunohistochemistry analysis. Cecal digesta samples were put into sterile tubes, snap-frozen in liquid nitrogen, and transferred to −80°C for DNA extraction and gut microbiome analysis.
Serum calcium content was measured using commercial colorimetric assay kits (Nanjing Jiancheng Bioengineering Institute, Nanjing, China). Serum 25-OH-VD concentrations were determined by ultrahigh-performance liquid chromatography–tandem mass spectrometry as previously described (26). A standard curve was obtained using dilutions of a 25-OH-VD standard (Iso Sciences, USA).
The number of Salmonella cells in the cecal content was analyzed using the plate pouring method as previously described (3). Bacterial concentration was expressed in colony-forming units (lg CFU/g of content).
The fixed jejunum tissues were processed in graded ethanol, then embedded in paraffin wax, cut into 5 μm thickness slides using a microtome (Leica Microsystems K.K., Tokyo, Japan), and stained with H&E. The slides were scanned (Leica Aperio CS2, Germany) for histopathological analysis at 200 × magnification using an Apeio Image Scope (Version 12.0.1; Leica Biosystems, Buffalo Grove, USA). Histopathological scoring refers to a standard described previously (27). The combined pathological score for jejunum tissue specimen was determined as the sum of following scores: (1) Inflammation (score 0-3): 0 = no inflammatory-cell infiltration; 1 = slight inflammatory-cell infiltration; 2 = moderate inflammatory-cell infiltration; 3 = severe inflammatory-cell infiltration. (2) Extent of lesions (score 0-3): 0 = No lesion; 1 = Lesion in the mucosal layer; 2 = Lesion in the mucosal layer and submucosa; 3 = Transparent cell wall. (3) Crypt Damage (score 0-4): 0 = No lesion in crypt; 1 = 1/3 crypt lesion; 2 = 2/3 crypt lesion; 3 = only the epithelial surface was intact; 4 = Crypt and epithelium not visible. It ranges between 0 and 10 arbitrary units and covers the levels of inflammation in the intestine.
The apoptotic cells in the jejunum tissues were detected using the terminal deoxynucleotidyl transferase dUTP nick end labeling (TUNEL) assay kit (Roche Diagnostics GmbH, Mannheim, USA) in accordance with the manufacturer's protocol. Briefly, sections (5 μm) were deparaffinized in xylene, rehydrated in decreasing concentrations of ethanol, boiled in Citra buffer solution for 10 min, and digested in 0.5% pepsin for 60 min at 37°C, before endogenous peroxidase was quenched in 3% hydrogen peroxide following incubating with proteinase K. The slides were incubated with the terminal deoxynucleotidyl transferase (TdT)/digoxin-dUTP mixture for 2 h at 37°C. Following washes with TBS three times, the slides were enclosed with anti-digoxin-biotin conjugated antibody diluted 1:100 in blocking reagent and incubated for 30 min at 37°C. Subsequently, the sections were incubated with streptavidin-biotin complex (SABC) for 1 h at 37°C. Apoptotic cells were detected by a microscope (80i, Nikon Eclipse, Tokyo, Japan) after incubation in the 3,3′-diaminobenzidine (DAB) chromogen for approximately 6 min and slides were counterstained with hematoxylin. The integrated optical density (IOD) of TUNEL-positive cells was assessed by a digital microscope camera system (Nikon DS-Ri1, Japan) and Image-Pro Plus 6.0 software (Media Cybernetics, Inc., MD, USA) image analysis software.
Total RNA was extracted from the jejunum samples using Trizol reagent (Invitrogen Life Technologies, Carlsbad, CA, USA) according to the manufacturer's instructions. The concentration and purity of total RNA were determined using a NanoDrop-2000 spectrophotometer (Thermo Fisher Scientific, Waltham, MA, USA). Then, complementary DNA (cDNA) was synthesized using Primer Script™ RT reagent Kit (Takara) according to the manufacturer's instructions. Using the synthesized cDNA as a template, RT-qPCR was performed in Applied Biosystems' 7,500 Fast Real-Time PCR System81iUSA), with SYBR Premix Ex Taq TMQ20 kit (Takara Biotechnology Co. Ltd., Dalian, China) in accordance with the manufacturer's guidelines. Relative mRNA levels were calculated using the 2 −ΔΔCT method and normalized with β-actin. Relative expressions for target genes were calculated using that of the VD-supplemented but without the SE infection group as the reference (set to 1). The primer sequences used in this study were designed and synthesized by Sangon (China) and listed in Supplementary Tables 1, 2.
About 0.2 g of frozen jejunal mucosa was homogenized and lysed in 1 mL of ice-cold radioimmunoprecipitation assay (RIPA) lysis buffer containing 10 μL PMSF [50 mmoL/L Tris-HCl (pH 7.4), 150 mmoL/L NaCl, 1% nondiet P (NP)-40,.1% SDS, 1.0 mmoL/L PMSF, 1 mmoL/L Na3VO4, 1 mmoL/L sodium fluoride (NaF), and protease and phosphatase inhibitor cocktail] (P1045, Beyotime Biotechnology, Co., Ltd., Beijing). The homogenate lysate was centrifuged at 12,000 × g for 15 min at 4°C to remove cellular debris, and then the supernatant was collected for total protein determination using a BCA protein assay kit (BTYA0301, Biodragon Immunotechnologies Co., Ltd., Beijing, China). Equal amounts of extracted protein (40 μg) were subjected to electrophoresis on 8, 10, or 12% SDS-PAGE gels (Tris-glycine-sodium dodecyl sulfate-polyacrylamide gel electrophoresis), and then transferred onto methanol presoaked polyvinylidene difluoride (PVDF) membranes (IPVH000101, Millipore, USA). The membranes were inoculated with 3% skimmed-milk solution in Tris-buffered saline containing 0.05% Tween-20 (TBST blocking solution) at 37°C for 1 h, and then were incubated with primary antibodies. Rabbit anti-mouse polyclonal primary antibodies against chicken B cell lymphoma (Bcl-2) and Fas were purchased from Wanlei Biotech Co., Ltd. (Shenyang, China), and p53, Caspase-3, and glyceraldehyde 3-phosphate dehydrogenase (GAPDH), were purchased from Suzhou Biodragon Immunotechnology Co. Ltd. (Suzhou, China, Supplementary Table 3). Rabbit anti-chicken polyclonal primary antibodies against chicken Claudin-1, Claudin-4, Occludin, and ZO-1 recombinant protein antigens were prepared in our laboratory (patents were being authorized). And then membranes were washed an additional three times with TBST and incubated with secondary goat anti-rabbit horseradish-peroxidase (HRP)-conjugated IgG (BF-3008, Biodragon Immunotechnologies, Co., Ltd., Beijing, China) at room temperature for 3 h. The filters were then stripped and reprobed with antibodies to total glyceraldehyde 3-phosphate dehydrogenase (GAPDH, YM 3029, immunoway, USA) to demonstrate equal loading. After incubation, the chemiluminescence signal was detected by using the enhanced/super ECL chemiluminescent kit (BF06053, Biodragon Immunotechnologies Co., Ltd., Beijing, China) and a luminescence imager (Tanon 5200, Tanon Science & Technology Co., Ltd., Shanghai, China). Detection and quantification of protein band density were determined using image-J (version 1.50i) software. The results are expressed as relative intensity compared to those of the normal controls.
Genomic DNA of cecal digesta at 7 dpi was extracted using PowerSoil® DNA Isolation Kit (ANBIOSCI Tech Ltd., USA) according to the manufacturer's instructions. The concentration, purity, and integrity of the isolated genomic DNA were measured using a NanoDrop ND-1,000 (Thermo Scientific, Wilmington, DE), and agarose gel electrophoresis, respectively. The qualified genomic DNA was used as a template for the V3~V4 region of bacterial 16S rRNA gene amplification with barcoded primer pair 338F: 5′-ACTCCTACGGGAGGCAGCA-3′ and 806R: 5′-GGACTACHVGGGTWTCTAAT-3′. Amplicon libraries were constructed and sequenced on Illumina HiSeq 2,500 platform (Illumina, San Diego, USA) at Biomarker Technologies Co. Ltd. (Beijing, China). The sequencing data were merged using FLASH (version 1.2.11) to get raw tags. Raw tags were subjected to filtration (Trimmomatic, version.33) and chimera sequences removed (UCHIME, version 8.1) to obtain effective tags. UCLUST was used to cluster effective tags into operational taxonomic units (OTUs) at a similarity level of 97% with Quantitative Insights into Microbial Ecology (QIIME) software (version 1.8.0) (28). Afterward, based on the Silva taxonomic database, OTUs were annotated. Venn diagram, Rarefaction curve, and bacteria relative abundance were created with R software (version 2.15.3). Alpha diversity, including ACE, Chao 1, Simpson, and Shannon index, was investigated by Mothur (vision 1.30), and the significance of these items was determined using the Mann-Whitney U test. Beta diversity was calculated from a binary-jaccard distance (PERMANOVA/ANOSIM analysis) in QIIME software. Two-sided Student's t-test was used to determine the significance of the differences between groups. Line Discriminant Analysis (LDA) Effect Size (LEfSe, http://huttenhower.sph.harvard.edu/lefse/) tool was used to determine statistically different biomarkers between groups (LDA value = 4) based on the taxonomic files obtained from the QIIME analysis (29). The raw sequences used in our study had been uploaded at the Sequence Read Archive of the National Center for Biotechnology Information, with the study accession number PRJNA759703. The functions of the cecum metagenomes were predicted using Phylogenetic investigation of communities by reconstruction of unobserved states (PICRUSt) analysis based on high-quality sequences (30).
All results are displayed as means ± SEM. For the normally distributed data (serum calcium and 25-OH-VD concentration, productive performance, intestinal histopathological scores, cecal Salmonella load, intestinal PCNA-positive and TUNEL-positive cell numbers, relative mRNA expression, protein expression), the main effect and interaction effects were analyzed using the general linear model (GLM) procedure in SPSS 19.0 (SPSS Inc, Chicago, Illinois, USA). The one-way ANOVA and multiple comparisons were performed when interactive effects differed significantly. α-diversity and β-diversity were analyzed using the Mann-Whitney U test. The relative abundance of microorganisms obtained from 16S rRNA sequencing was analyzed using the Kruskal-Wallis test to compare the difference between two groups or all four treatments. P < 0.05 was considered significant.
Figure 1 showed that the concentrations of serum calcium/in VD3 sufficient groups (2.19 ± 0.09 mmol/L; 24.6 ± 1.37 ng/mL) were remarkably higher than those in VD3-deficient groups (1.91 ± 0.76 mmol/L; 6.76 ± 1.11 ng/mL) regardless of Salmonella infection (P = 0.006), indicating that hens fed diets without VD3 supplementation for 10 weeks were in a state of VD deficiency [deficiency <12 ng/ml; insufficiency was between 12 and 20 ng/ml (31)], whereas hens given VD3 at 3000IU/kg diet were VD sufficient. In addition, the Salmonella challenge reduced serum 25-OH-VD, and calcium (1.88 ± 0.06 mmol/L; 13.89 ± 2.63 ng/ml) concentration relative to the non-infected groups (P < 0.05, 2.22 ±0.09 mmol/L; 17.5 ± 3.16 ng/ml), suggesting that Salmonella challenge disturbed the absorption and metabolism of VD3 in hens, resulting in reduced serum calcium and VD levels. However, there was no notable interactive effect between VD3 addition and SE challenge on serum calcium and 25-OH-VD levels.
Figure 1. VD3 enhanced serum (A) calcium and (B) 25-OH-VD concentration of laying hens. Data are represented as the mean (n = 6) ± SEM; VD0, hens with VD3-free diet and no infection; VD3000, hens with VD3-sufficient diet and no infection; VD0S, hens with VD3-free diet and Salmonella infection; VD3000S, hens with VD3-sufficient diet and Salmonella infection; VD, vitamin D3; SE, Salmonella enteritidis.
Data on the productive performance of hens fed diets with different levels of VD3 from 1 to 10 weeks before SE infection have been presented in our published paper (32). Data on the productive performance of hens fed diets with 0 or 3,000 IU VD3/kg of the diet with or without SE infection during 11 to 12 weeks was shown in this study (Table 2). Results indicated that supplemental VD3 at 3,000 IU per kg of diet significantly increased laying rate, egg weight, and feed intake remarkably improved feed to egg ratio (P < 0.01) but had no effect on mortality when compared with the VD3-free groups (P = 0.441). Salmonella challenge significantly decreased laying rate and egg weight (P < 0.05), trended to decrease the feed intake (P = 0.095), but notably increased the feed to egg ratio and death ratio (P < 0.05). There were interactive effects between VD3 addition and Salmonella challenge on egg weight as well as feed intake (P < 0.05). SE-infected hens without VD3 supplementation exhibited the lowest egg weight and feed intake compared with the other three groups. The non-infected hens fed VD-deficient diets showed the lowest egg thickness and eggshell strength as compared to the other three group hens. And there was an interactive trend of VD3 and challenge on feed egg ratio (P = 0.063). SE-infected hens fed VD3-deficient diets displayed the highest FCR relative to the other three group hens.
Histopathological scores in the jejunum were detected by H&E (Figure 2). Results showed the jejunal morphology was destroyed by SE challenge and VD3 deficiency (P < 0.05). Compared with the VD3 sufficiency and non-Salmonella infected group, both the single Salmonella-infected hens and the VD3-deficiency hens showed extensive granulocytes and mono-nuclear cells infiltration in the mucosa and submucosa, ulceration of the epithelial layer, crypt damage of gut wall in the jejunum, resulting in substantially higher histopathology scores, indicating that either Salmonella infection or VD3 deficiency-induced intestinal mucosa impairment and intestinal inflammation. In contrast, only mild inflammation in the jejunum was observed in the infected hens that received VD3 sufficient supplementation compared to that of the single Salmonella infection hens, suggesting that dietary VD3 sufficient supplementation might alleviate intestinal inflammatory injury.
Figure 2. VD3 alleviated jejunal pathology in Salmonella infected hens (× 400 magnification; scale bar: 20 μm). Data are represented as the mean (n = 6) ± SEM; The photomicrographs showed obvious inflammatory-cell infiltration (black arrows), ulceration of the epithelial layer, necrotic cell clusters with shrunken nucleoli or karyorrhexis and karyolysis (red arrows), and connective tissue hyperplasia and inflammatory cell infiltration in the submucosal and muscle layer (crypt damage, yellow arrows). VD0, hens with VD3-free diet and no infection; VD3000, hens with VD3-sufficient diet and no infection; VD0S, hens with VD3-free diet and Salmonella infection; VD3000S, hens with VD3-sufficient diet and Salmonella infection; VD, vitamin D3; SE, Salmonella enteritidis.
As shown in Figure 3, no Salmonella was detected in the cecum of the two non-infected groups. Dietary VD3 addition down-regulated the number of Salmonella gene copies in cecal content nevertheless SE challenge up-regulated it significantly (P < 0.001). Infected hens given VD3 sufficient supplementation significantly decreased Salmonella numbers in cecal content compared with infected hens, indicating that supplemental VD3 could inhibit the growth of Salmonella in the cecum of infected hens (P < 0.001).
Figure 3. VD3 lowered Salmonella load in cecal content of Salmonella infected hens. Data are represented as the mean (n = 6) ± SEM; Bars with different letters show a significant difference between groups (P < 0.05); VD0, hens with VD3-free diet and no infection; VD3000, hens with VD3-sufficient diet and no infection; VD0S, hens with VD3-free diet and Salmonella infection; VD3000S, hens with VD3-sufficient diet and Salmonella infection; VD, vitamin D3; SE, Salmonella enteritidis.
Apoptotic cells were identified by TUNEL staining. The apoptotic cell had round and shrunken shapes and the nuclei were darkly stained. As illustrated in Figure 4A, VD sufficiency significantly decreased the TUNEL-positive cells population compared with VD deficiency (P < 0.001), nevertheless TUNEL-positive cells in the jejunum markedly increased in the single SE-infected hens compared with the non-infected group (P < 0.001). In addition, a marked reduction of TUNEL-positive cells was observed in the jejunum of the SE-infected hens given sufficient VD3 supplementation (P < 0.05). Western blotting results showed that SE infection tended to decrease the Bcl-2 protein transcription (P = 0.059), while VD sufficiency significantly increased anti-apoptosis Bcl-2 protein expression abundance, notably lowering the protein amount of p53 (P < 0.05, Figure 4B). Furthermore, relative to the infected hens without VD3 addition, infected hens given VD3-sufficient diets notably decreased Caspase-3 protein levels in the jejunum mucosa (P < 0.05). Based on these results, we suggested that dietary VD3 sufficient supplementation could attenuate Salmonella-induced intestinal cells apoptosis through modulating apoptosis-related proteins expression.
Figure 4. VD3 suppressed jejunal apoptosis response of Salmonella infected hens. (A) Percentage of TdT-mediated dUTP nick-End labeling (TUNEL)-positive cells. The red arrow points apoptotic cells in the jejunal crypt, and the yellow arrow represents the apoptotic cells in the villus. Integral optical density (IOD) represented the TUNEL expression. Magnification = 400×. (B) Western blot analysis for the expression levels of apoptosis-relative protein. Data are represented as the mean (n = 6) ± SEM; Bars with different letters show a significant difference between groups (P < 0.05); VD0, hens with VD3-free diet and no infection; VD3000, hens with VD3-sufficient diet and no infection; VD0S, hens with VD3-free diet and Salmonella infection; VD3000S, hens with VD3-sufficient diet and Salmonella infection; VD, vitamin D3; SE, Salmonella enteritidis; Bcl-2, B-cell lymphoma-2; GAPDH, glyceraldehyde-3-phosphate dehydrogenase.
As displayed in Table 3, Salmonella infection didn't affect intestinal VD-metabolism related genes expression, but remarkably upregulated toll-like receptor (TLR)-4, tumor necrosis factor (TNF)-α, interleukin (IL)-8, and interferon (IFN)-γ genes expression (P < 0.05), tended to up-regulate genes expressions of myeloid differentiation primary response protein 88 (MyD88) and IL-1β (0.05 < P < 0.1) as compared to the non-infected hens, suggesting that Salmonella infection caused intestinal inflammation via activating TLR4-mediated pathway. Supplemental VD3 significantly upregulated cytochrome P450 family 24 subfamilies A member 1 (CYP24A1), 25-hydroxylase-encoding gene cytochrome P450 family 2 subfamily R member 1 (CYP2R1), and TLR-2 genes expressions (P < 0.05), tended to upregulate 1α-hydroxylase-encoding gene cytochrome P450 family 24 subfamily B member 1 (CYP27B1), and cytochrome P450 family 3 subfamily A member 37 (CYP3A37) gene expressions (.05 < P < 0.1), and notably downregulated TNF-α mRNA levels (P = 0.053) and showed a decreased trend for TLR-4 and MyD88, and VDR gene expression in the jejunum (0.05 < P < 0.1) compared with the VD3-deficient hens, indicating that VD deficiency could induce intestinal inflammation, whereas intestinal inflammation induced by VD deficiency could be inhibited by VD sufficiency. SE-infected hens given the VD3-sufficient diets markedly upregulated jejunal CYP27B1 and TLR-2 but downregulated TLR-4, MyD88, IFN-γ, TNF-α, IL-1β as well as IL-8 mRNA levels (P < 0.05).
Table 3. VD3 facilitated VD3 metabolism, regulated inflammatory pathway and strengthened barrier function of jejunum of Salmonella infected hens.
Compared with VD3-deficient groups, Claudin-1 gene expression was up-regulated by dietary VD3 sufficient supplementation, but mucin-2 was down-regulated (P < 0.05, Table 3). Although the SE challenge had no influence on the barrier function-related genes and mucin-2 expression, there were notable interactive effects on Occludin and ZO-1 mRNA expression between VD3 administration and Salmonella challenge (P < 0.05). Further analysis revealed SE-infected hens given VD3 sufficient addition displayed a remarkable increase in Occludin and ZO-1 mRNA levels compared with the SE-infected alone hens (P < 0.05). In accordance with mRNA expression results, immune-blotting showed that SE challenge significantly reduced the amount of Claudin-1 and Claudin-4 protein (P < 0.05, Figure 5), showed a declined trend in ZO-1 protein amounts (P = 0.079), indicating SE infection disrupted intestinal tight junction. In contrast, VD3 sufficient addition remarkably up-regulated the protein levels of Claudin-4 as well as ZO-1 (P < 0.05) and trended to increase the Claudin-1 protein amount (P = 0.061) regardless of infection. Further, SE-infected hens received VD sufficiency markedly upregulated Claudin-4 protein expression level, indicating a barrier restoring effect of VD3 sufficient addition on laying hens under the SE challenge.
Figure 5. VD3 boosted jejunal barrier integrity of Salmonella infected hens. Data are represented as the mean (n = 6) ± SEM; Bars with different letters show a significant difference between groups (P < 0.05); VD0, hens with VD3-free diet and no infection; VD3000, hens with VD3-sufficient diet and no infection; VD0S, hens with VD3-free diet and Salmonella infection; VD3000S, hens with VD3-sufficient diet and Salmonella infection; VD, vitamin D3; SE, Salmonella enteritidis; ZO-1, zonula occludens-1; GAPDH, glyceraldehyde-3-phosphate dehydrogenase; VD, vitamin D3; SE, Salmonella enteritidis.
The analysis of the 16S rRNA sequence showed that the cecal microbiota was prominently dominated by Firmicutes, Bacteroidetes, Proteobacteria, Fusobacteria together with Actinobacteria at the phylum level among all groups (Figure 6A). VD3 treatment had no influence on the relative abundance of those phyla, but the SE challenge remarkably decreased the abundance of Proteobacteria (P = 0.039, Table 4). What's more, dietary VD3 sufficient inclusion tended to inhibit SE-induced a decrease in the relative abundance of Fusobacteria compared to the single SE challenge (P = 0.079). At the gena level, microflora was dominated by Lactobacillus, Peptoclostridium, Bacteroides, Lawsonia as well as the Rikenellaceae_RC9_gut_group (Figure 6B). Analysis noted that SE infection tended to decrease Lawsonia (P = 0.086) but increased Rikenellaceae_RC9_gut_group relative abundance, respectively (P = 0.098, Table 4). Dietary VD3 sufficient supplementation enriched the abundance of Lactobacillus (P = 0.006). Our results also found that alpha diversity of cecal microflora was not affected by VD3 nutritional status and Salmonella challenge (P > 0.05, Table 5). However, a significant difference in β diversity was observed between groups VD0, VD0S, and VD3000 (P < 0.05, Figure 6C). Additionally, the β diversity of the VD3000S group was significantly different from the group VD0S (P < 0.001). Linear discriminant analysis coupled with effect size measurements (LEfSe) analysis showed that Barnesiella, Escherichia Shigella, Enterobacteriaceae, Enterobacteriales, Clostridiales, and Clostridia were rather abundant in the VD0 group (Figure 6D). Adding VD3 at 3,000 IU/kg enriched the abundance of Lactobacillus, Lactobacillaceae, Lactobacillales as well as Bacilli. SE infection up-regulated the abundance of Ruminococcaceae, Family X AD3011. Nevertheless, SE infected given VD3 sufficient addition enhanced an uncultured Bacillus abundance in cecal content compared with infected hens and the VD3-free hens. PICRUSt analysis indicated that sufficient supplementation with VD3 up-regulated the translation, nucleotide metabolism as well as replication and repair pathways, whereas it down-regulated cell motility, global and overview maps, amino acid metabolism, and carbohydrate metabolism pathways of cecal microbiota (P < 0.001, Figure 6E), when compared with VD0 group. Similarly, under the SE challenge, dietary VD3 increased the membrane transport, translation, nucleotide metabolism, replication, and repair pathways, but decreased amino acid metabolism pathways of cecal microbiota in level 2 (P < 0.001, Figure 6F).
Figure 6. VD3 increased the abundance of fecal Lactobacillus to alleviated intestinal damage of Salmonella infected hens. Relative abundance of cecal microbiota at the (A) phylum level (B) geneus level. (C) Differential cecum microbiota community (β-diversity) between groups. (D) Differential enrichment of cecal microbiota between groups measured using LEfSe (LDA score = 4). The microbial pathways grouped into level-2 functional categories using PICRUSt between groups (E) VD0 and VD3000 and (F) VD3000 and VD3000S. Data are represented as the mean (n = 6) ± SEM; *P < 0.05, **P < 0.01. VD0, hens with VD3-free diet and no infection; VD3000, hens with VD3-sufficient diet and no infection; VD0S, hens with VD3-free diet and Salmonella infection; VD3000S, hens with VD3-sufficient diet and Salmonella infection.
Table 4. Changes in the composition of the gut microbiota cecal microbiota of laying hens challenged with Salmonella (%).
Table 5. Changes in the diversity of the gut microbiota cecal microbiota of laying hens challenged with Salmonella.
In this study, we first investigated the changes in the productive performance of laying hens at different VD3-metabolic statues (deficient or sufficient) with or without SE infection. A significant decrease in egg production and egg weight, and a notable increase in feed to egg ratio, were observed in hens that received a VD3-free diet, indicating that dietary long-term VD3 deficiency had significant negative effects on the productive performance of laying hens. However, these changes in productive performance caused by VD deficiency were reversed by dietary VD3 sufficient addition, which was consistent with previous reports (32, 33). Additionally, data of this study also showed that Salmonella infection had adverse effects on productive performance of laying hens which were similar to previous studies, while these negative effects caused by SE challenge were lightened by VD3 sufficient supplementation (3,000 IU per kg of diet), suggesting that dietary VD3 sufficient supplementation could reverse the negative impact of productive performance caused by Salmonella infection or VD deficiency in laying hens.
The major circulating form of VD is the 25-OH-VD, whose serum levels are regarded as an indicator of VD metabolic status, availability, and biological functions (34). In the study, we observed that serum Ca and 25-OH-VD concentration were notably decreased by Salmonella challenge or VD3 insufficiency or both treatments, reflecting that either Salmonella challenge or dietary VD3 deficiency treatment or both could reduce calcium and VD3 absorption in the gut, thereby resulting in intestinal malabsorption syndromes and VD deficiency status. In contrast, feeding sufficient VD3 significantly reversed this trend caused by Salmonella challenge or dietary VD3 deficiency, suggesting that dietary VD3 sufficient supplementation could improve the VD nutritional status of hens that were exposed to either Salmonella challenge or dietary VD3 deficiency. VD3 exerts various biological functions, mainly through regulating VD metabolic enzymes 25-hydroxylase (CYP2R1), 1α-hydroxylase (CYP27B1), and CYP24A1 activity, along with the VDR signal pathway (35, 36). Meanwhile, results of RT-PCR analysis showed that VD3 sufficient supplementation upregulated CYP2R1 and CYP24A1 genes expressions, tended to upregulate CYP27B1 and CYP3A37 gene expressions, showing dietary VD3 sufficiency facilitated the metabolic pathway of VD, resulting in increasing serum 25-OH-VD level (36). Thus, we suggested that changes in productive performance caused by dietary VD3 levels and Salmonella infection were possibly related to Ca and 25-OH-VD contents in serum. Dietary addition of sufficient VD3 contributed to the improvement of production performance in our study was partially ascribed to the observed higher serum calcium and 25-OH-VD concentration and upregulated intestinal CYP24A1, CYP2R1, and CYP27B1 genes expression.
A plethora of studies has demonstrated that VD3 and VDR signal pathways play a pivotal role in intestinal homeostasis through modulating intestinal barrier function (TJs and AJs), microbiome composition, as well as immune responses. Moreover, undesirable VD nutritional status or loss of VDR has long been associated with intestinal barrier impairment, dysbiosis, inflammation, and disease in humans and mice (21, 37). However, the effect of VD nutritional status on the intestinal health of Salmonella-infected hens has been rarely reported. In this study, we further investigated the effect of VD nutritional status on intestinal cells apoptosis index, intestinal pathological scores, and cecal Salmonella load of the Salmonella-infected hens. Results indicated that SE challenge or VD3 insufficiency or both increased intestinal histopathological scores, the number of intestinal TUNEL-positive cells together with Salmonella colonization levels, while VD3 sufficiency reversed these alterations caused by Salmonella infection or VD3 insufficiency, as indicated by a reduction in Salmonella carrier, TUNEL-positive cells percentage as well as gut inflammation, suggesting that VD3 deficiency promoted overgrowth of Salmonella in inflamed gut, increased the susceptibility to Salmonella infection, deteriorated intestinal damage severity. While VD3 sufficiency could restrain Salmonella proliferation and colonization in the gut together with decrease intestinal inflammation and apoptosis, resulting in ameliorating gut damage caused by Salmonella infection or VD deficiency. Similar results were also reported by previous research using mice or humans as models (38, 39), indicating that VD3 nutritional status is associated with disease susceptibility and the degree of gut injury.
Intestinal epithelial cells' apical junctional proteins, including Claudins, Occludins, ZOs, junctional adhesion molecules (JAMs), and E-cadherins, play a vital role in regulating intestinal permeability, defense against pathogen infection, and inflammation response (40). TLR-mediated signaling pathways and apoptosis response play core regulative roles in responding to Salmonella infection and epithelial barrier integrity (27, 41). In order to reveal the correlation between VD3 nutritional status and gut health of the Salmonella-infected or VD-deficient hens in deep, intestinal mucosal immune-related genes, apoptosis-related proteins, and tight junction proteins expressions profiles were analyzed further. In this study, Salmonella infection activated TLR4-mediated immune responses, induced pro-inflammatory cytokines and chemokines expression (IFN-γ, TNF-α, IL-1β, and IL-8), and suppressed intestinal tight junction Claudin-1, Claudin-4 protein, and ZO-1 protein amounts in laying hens, which was in consistent with previous results using chicken models (3, 42). Meanwhile, the protein amount of pro-apoptotic p53 and caspase-3 in the jejunum was elevated by the SE challenge. Additionally, the study also found that the effects of VD3 deficiency on intestinal immune responses, intestinal cells apoptosis, and tight junction proteins expression of hens were similar to that of the Salmonella challenge. Based on the above changes, we suggested that SE infection or VD deficiency, or both treatments lead to intestinal inflammation and intestinal epithelial cells apoptosis, possibly resulting in elevated intestinal mucosal permeability and the impairment of gut barrier integrity in laying hens. In contrast, those alterations in the gut induced by Salmonella infection or VD3 deficiency were restrained by VD3 sufficient administration in our study, as evidenced by downregulating TLR-mediated inflammatory cytokines (TLR4, MyD88, and TNF-a) genes expressions, upregulating Claudin-1 gene and protein, Claudin-4 and ZO-1 protein abundances in the VD-sufficient hens irrespective of Salmonella infection relative to VD deficiency. Moreover, upregulation in Claudin-4 protein, anti-apoptosis protein Bcl-2 amount, and VDR, Occludin as well as ZO-1 mRNA levels; together with downregulation in TLR-4, MyD88, IFN-γ, TNF-α, IL-1β, and IL-8 mRNA levels in the gut of the Salmonella-infected hens given enough VD3 obtained in our study, suggesting that VD3 sufficiency can effectively prevent intestinal inflammation and intestinal epithelial cells apoptosis triggered by SE infection, and protected intestinal epithelial barrier integrity. Similar results have been observed in humans and mice when faced with stress and pathological conditions (38). Meanwhile, VD3 sufficient supply enhanced TLR-2 mRNA levels in Salmonella-infected hens compared with the infected hens with VD insufficiency was detected in our study. VDR and TLR-2 signal pathways had been reported to be positively involved in anti-infection, anti-inflammatory, anti-oxidative and anti-apoptosis activities as well as intestinal barrier-protective effects, especially under inflammation conditions (43), therefore upregulated level of TLR-2 together with intestinal VDR mRNA amounts obtained in Salmonella-infected hens received enough VD3, indicating further confirmed our suggestion. Collectively, our results suggested that VD3 sufficient supply could prevent intestinal barrier impairment induced by either Salmonella or VD deficiency, and improve gut health in laying hens, possibly by reducing intestinal mucosal inflammation and boosting tight junction proteins expression together with inhibiting intestinal epithelial cells apoptosis. Our data obtained from the present study also suggests that VD3 sufficient supply may be beneficial for controlling Salmonella infection in laying hens.
Intestinal microbiota and its metabolites play a critical role in regulating host physiology, immune responses, and diseases resistance ability (44). Evidence from mammal experimental studies and clinical trials suggested that there were potential associations between VD3, gut microbiota, and gut health, namely, the effect of VD3 on health is partially mediated through the microbiome (45, 46). To investigate the underlying mechanisms of the VD3 status on the gut health of the Salmonella-infected hens, the cecal microbial composition was further investigated. The results revealed that the alpha diversity of the microbiota profile was not different between the VD3 nutritional status and Salmonella challenge in our study, which was similar to the results of research on humans and mice (47). However, both VD3 treatment and SE challenge remarkably modified β-diversity, which indicated that VD3 administration, SE challenge altered intestinal bacterial community profiles. LEfSe analysis observed that some potential detrimental microbes, including Barnesiella, Escherichia Shigella, Enterobacteriaceae, Enterobacteriales, Clostridiales, and Clostridia, were enriched in VD3-deficient hens, while sufficient VD3 sufficient addition favored the growth of some potential health-beneficial microbes, including Lactobacillales, Lactobacillaceae, and Lactobacillus, as well as Bacilli, implying that VD nutritional status was correlated with gut microbiome composition in laying hens irrespective of Salmonella infection. In similar with our findings, accumulating pieces of evidence from human and mice studies has shown that the composition of the gut microbiome can be altered by VD3 status, especially under disease challenge conditions, and the effects of VD3 on intestinal microbiome interactions related to gut dysbiosis and gut inflammation (48). In mammals, supplemental VD3 could increase the abundance of potential health-beneficial bacterial genera (such as Lactobacillus), and lowered the abundance of potential detrimental or inflammation-related microbiota genera in the gut, possibly by regulating the production of antimicrobial peptides, such as cathelicidin and β-defensin (49). Additionally, VD deficiency increases the relative abundance of opportunistic pathogens which, in the context of intestinal barrier dysfunction, may favor pathogen bacterial translocation and systemic infection and inflammation (50). Lactobacillus spp. was reported to exhibit beneficial effects on intestinal health, and intestinal barrier integrity, and had stronger anti-inflammatory effects when confronted with pathogens infection, including Salmonella (51). Also, Lactobacillus treatment could contribute to the increase in circulating VD, improve the VDR signaling and reduce the inflammatory response in the gut (52, 53). Higher serum 25-OH-VD levels decreased intestinal inflammation and improved gut barrier structure observed in the Salmonella-infected or VD3-deficient hens following sufficient VD3 supplementation in our study might be attributed to the increase of Latcobacillus abundance in the gut. At the same time, VD3 sufficient addition enriched uncultured Bacillus abundance in the cecal content of the Salmonella-infected given as compared to the single infected hens and the VD3-free hens. Some Bacillus spp were considered to be beneficial microbiota and used as feed additives and antibiotic alternatives due to secreting digestive enzymes, producing anti-microbial substances along with possessing immune-regulatory and anti-inflammatory effects (54). Further, more studies had showed that probiotics Bacillus spp. was widely used to advance feed absorption and productive performance, promote immunity, improve gut health and enhance resistance to enteric pathogens, such as Salmonella, Escherichia coli, and Clostridium perfringens, infection in poultry (3). Collectively, these findings suggested VD nutritional status affects the composition of gut bacterial microbiota, and VD deficiency could bring about gut dysbiosis and gut barrier integrity impairment, while supplementation of sufficient VD3 had a positive influence on health-beneficial microbes in the Salmonella-infected hens or the VD3-deficient hens. In other words, the protective effect of VD3 sufficiency on the gut health of the Salmonella-infected hens was possibly by promoting the establishment of a healthy microbiota and inhibiting potential detrimental microbes. Improved laying performance, decreased mortality, and alleviated gut inflammation observed in hens supplied with sufficient VD3 may be possibly attributed to improving gut microbial composition induced by VD3 sufficient addition. PICRUSt analysis indicated that nucleotide metabolism, translation as well as replication, and repair pathways involved in cell viability and growth were enriched in the hens that received VD3 sufficient addition regardless of Salmonella infection (55). Meanwhile, the membrane transport pathway associated with the survival of bacteria in the gut ecosystem was also increased in the Salmonella-infected hens given sufficient VD3, suggesting that VD3 sufficient supplementation exerted a growth-promoting, restorative role for gut microflora of hens under an undesirable state of nutrition or pathogen challenge (56). Improved intestinal microbiota in hens following VD3 sufficient administration whether infected with Salmonella or not was obtained in our study to support our suggestion. In addition, cell motility and amino acid metabolism pathways were suppressed by dietary VD3 sufficiency relative to VD deficiency. Further, only amino acid metabolism pathways were downregulated in the Salmonella-infected hens given sufficient VD3 as compared to the single Salmonella-infected hens. Cell motility is the determinant step of pathogen bacteria, such as Salmonella, in early local invasion (57). The unabsorbed amino acids in the hind-gut would be metabolized into polyamines, such as putrescine, spermidine, and spermine, which could damage the gut (58). Thus, the down-regulated cell motility and amino acid metabolism pathways suggested that VD3 might have anti-infective and barrier-protecting functions via modulating the function of gut microbiota. Surprisingly, carbohydrate metabolism pathways were enriched in the VD3-deficient group. As reported, indigestible carbohydrate could be fermented by cecal microflora into short-chain fatty acids which functions as trophic and immune regulatory effects (59). Combined with the phenomenon of potential harmful microbiota, such as Barnesiella, Escherichia Shigella, Enterobacteriaceae, Enterobacteriales, Clostridiales, and Clostridia, were abundant in VD3-deficient hens, we suggested that carbohydrate metabolism pathways enriched in VD3-deficient hens may be associated with the production of inflammatory metabolites and intestinal inflammation. Taken together, observation in the present study indicated that VD3 sufficient supplementation might be a valuable way to manipulate the composition of the bacterial microbiome and protect against gastrointestinal injury in hens. Additional studies are needed to further understand the relationship between VD3 levels, gut bacteria, and gut health using fecal microbiota transplantation.
In summary, we presented evidence that the VD3 status is associated with gut health and disease resistance of laying hens. Concretely speaking, VD3 insufficiency or deficiency are much more susceptible to Salmonella, leading to intestinal microflora disturbance and gut mucosal injury together with extensive intestinal inflammatory responses in laying hens. While dietary supplementation with sufficient VD3 is able to enhance intestinal barrier junctions by inducing junction protein expression, restoring the balance of intestinal microbiota, and alleviating intestinal damage and intestinal inflammation induced by Salmonella in laying hens. The data also first identified that adequate VD3 in feed could act as a novel nutritional strategy to defend against Salmonella infection in hens. It should be noted that well-defined studies on optimal dose and underlying action mechanisms of VD3 modulating gut health of hens are required in future research.
The datasets presented in this study can be found in online repositories. The names of the repository/repositories and accession number(s) can be found below: NCBI [accession: PRJNA759703].
The animal study was reviewed and approved by CAU20170601-2.
ZW designed the research, evaluated the test details, and revised the manuscript. YqG and WA performed the experiments. FG analyzed the data and wrote the manuscript. WZ, SW, and YH participated in the revision of the manuscript. YmG and QM provided calculation and operation support. All authors contributed to data interpretation and approved the final version of the manuscript.
This research was funded by the Natural Science Foundation of China (No. 32172774).
The authors declare that the research was conducted in the absence of any commercial or financial relationships that could be construed as a potential conflict of interest.
All claims expressed in this article are solely those of the authors and do not necessarily represent those of their affiliated organizations, or those of the publisher, the editors and the reviewers. Any product that may be evaluated in this article, or claim that may be made by its manufacturer, is not guaranteed or endorsed by the publisher.
The Supplementary Material for this article can be found online at: https://www.frontiersin.org/articles/10.3389/fnut.2022.888580/full#supplementary-material
Figure S1. Action mechanisms of VD3 modulating gut health of hens with VD3 deficiency and/or Salmonella challenge: VD3 sufficiency ameliorated gut injury induced by either Salmonella and/or VD3 deficiency via promoting VD3 metabolism, reducing Salmonella load, suppressing pro-inflammatory status, increasing expression of TJs along with decreasing apoptotic responses. Besides, VD3 restored the balance of gut microflora by enriching the abundance of probiotics such as Lactobacillus and Bacilli.
1. Jajere SM. A review of Salmonella enterica with particular focus on the pathogenicity and virulence factors, host specificity and antimicrobial resistance including multidrug resistance. Vet World. (2019) 12:504–21. doi: 10.14202/vetworld.2019.504-521
2. Shao Y, Wang Z, Tian X, Guo Y, Zhang H. Yeast β-d-glucans induced antimicrobial peptide expressions against Salmonella infection in broiler chickens. Int J Biol Macromol. (2016) 85:573–84. doi: 10.1016/j.ijbiomac.2016.01.031
3. Zhen W, Shao Y, Gong X, Wu Y, Geng Y, Wang Z, et al. Effect of dietary Bacillus coagulans supplementation on growth performance and immune responses of broiler chickens challenged by Salmonella enteritidis. Poult Sci. (2018) 97:2654–66. doi: 10.3382/ps/pey119
4. van Cuyck H, Farbos-Granger A, Leroy P, Yith V, Guillard B, et al. MLVA polymorphism of Salmonella enterica subspecies isolated from humans, animals, and food in Cambodia. BMC Res Notes. (2011) 4:306–306. doi: 10.1186/1756-0500-4-306
5. Smith AM, Ismail H, Henton MM, Keddy KH, Surveillance Network GS. Similarities between Salmonella Enteritidis isolated from humans and captive wild animals in South Africa. J Infect Dev Ctries. (2014) 8:1615–9. doi: 10.3855/jidc.5393
6. Mughini-Gras L, Enserink R, Friesema I, Heck M, van Duynhoven Y, van Pelt W. Risk factors for human salmonellosis originating from pigs, cattle, broiler chickens and egg laying hens: a combined case-control and source attribution analysis PloS one. (2014) 9:e87933–e87933. doi: 10.1371/journal.pone.0087933
7. The The European Union summary report on trends and sources of zoonoses zoonotic agents and food-borne outbreaks in 2016. Efsa J. (2017) 15: e05077. doi: 10.2903/j.efsa.2017.5077
8. Koutsoumanis K, Allende A, Alvarez-Ordóñez A, Bolton D, Bover-Cid S, Chemaly M, et al. Salmonella control in poultry flocks and its public health impact. Efsa J. (2019) 17:e05596. doi: 10.2903/j.efsa.2019.5596
9. Vandeplas S, Dubois Dauphin R, Beckers Y, Thonart P, Théwis A. Salmonella in chicken: current and developing strategies to reduce contamination at farm level. J Food Prot. (2010) 73:774–85. doi: 10.4315/0362-028x-73.4.774
10. Cheng G, Hao H, Xie S, Wang X, Dai M, Huang L, et al. Antibiotic alternatives: the substitution of antibiotics in animal husbandry? Front Microbiol. (2014) 5:217. doi: 10.3389/fmicb.2014.00217
11. Al-Khalaifa H, Al-Nasser A, Al-Surayee T, Al-Kandari S, Al-Enzi N, Al-Sharrah T, et al. Effect of dietary probiotics and prebiotics on the performance of broiler chickens. Poult Sci. (2019) 98:4465–79. doi: 10.3382/ps/pez282
12. Gong H, Yang Z, Celi P, Yan L, Ding X, Bai S, et al. Effect of benzoic acid on production performance, egg quality, intestinal morphology, and cecal microbial community of laying hens. Poult Sci. (2021) 100:196–205. doi: 10.1016/j.psj.2020.09.065
13. Kemgang T S, Kapila S, Shanmugam VP, Reddi S, Kapila R. Fermented milk with probiotic Lactobacillus rhamnosus S1K3 (MTCC5957) protects mice from salmonella by enhancing immune and non-immune protection mechanisms at intestinal mucosal level. J Nutr Biochem. (2016) 30:62–73. doi: 10.16/j.jnutbio.2015.11.018
14. Shojadoost B, Yitbarek A, Alizadeh M, Kulkarni R R, Astill J, Boodhoo N, et al. Centennial review: effects of vitamins A, D, E, and C on the chicken immune system. Poult Sci. (2021) 100:100930. doi: 10.1016/j.psj.2020.12.027
15. Gil Á, Plaza-Diaz J, Mesa MD. Vitamin D: Classic and Novel Actions Ann Nutr Metab. (2018) 72:87–95. doi: 10.1159/000486536
16. Worm M, Heine G, Radbruch A. Immunomodulation by vitamin D. Allergol Select. (2018) 2:62–6. doi: 10.5414/alx1430e
17. Schmitt AK, Puppa MA, Wessels I, Rink L. Vitamin D3 and zinc synergistically induce regulatory T cells and suppress interferon-γ production in mixed lymphocyte culture. J Nutr Biochem. (2022) 102:108942. doi: 10.1016/j.jnutbio.2022.108942
18. Clark A, Mach N. Role of vitamin D in the hygiene hypothesis: the interplay between vitamin D, Vitamin D receptors, gut microbiota, and immune response. Front Immunol. (2016) 7:627. doi: 10.3389/fimmu.2016.00627
19. Teymoori-Rad M, Shokri F, Salimi V, Marashi SM. The interplay between vitamin D and viral infections. Rev Med Virol. (2019) 29:e2032. doi: 10.1002/rmv.2032
20. Garcia PM, Moore J, Kahan D, Hong MY. Effects of vitamin D supplementation on inflammation, colonic cell kinetics, and microbiota in colitis: a review. Molecules. (2020) 25:2300. doi: 10.3390/molecules25102300
21. Fakhoury HMA, Kvietys PR, AlKattan W, Anouti FA, Elahi MA, Karras SN, et al. Vitamin D and intestinal homeostasis: barrier, microbiota, and immune modulation. J Steroid Biochem Mol Biol. (2020) 200:105663. doi: 10.1016/j.jsbmb.2020.105663
22. Lin YD, Arora J, Diehl K, Bora SA, Cantorna MT. Vitamin D Is Required for ILC3 Derived IL-22 and Protection from citrobacter rodentium infection. Front Immunol. (2019) 10:1. doi: 10.3389/fimmu.2019.00001
23. Adhikari R, White D, House JD, Kim WK. Effects of additional dosage of vitamin D(3), vitamin D(2), and 25-hydroxyvitamin D(3) on calcium and phosphorus utilization, egg quality and bone mineralization in laying hens. Poult Sci. (2020) 99:364–73. doi: 10.3382/ps/pez502
24. Pender, Michael P. CD8+ T-Cell deficiency, epstein-barr virus infection, vitamin D deficiency, and steps to autoimmunity: a unifying hypothesis. Autoimmune Dis. (2012). 2012:189096. doi: 10.1155/2012/189096
25. Fatemi SA, Elliott KEC, Bello A, Peebles ED. Effects of the in ovo injection of vitamin D(3) and 25-hydroxyvitamin D(3) in Ross 708 broilers subsequently challenged with coccidiosis I performance, meat yield and intestinal lesion incidence(1,2,3). Poult Sci. (2021) 100:101382. doi: 10.1016/j.psj.2021.101382
26. Vijayendra Chary A, Hemalatha R, Seshacharyulu M, Vasudeva Murali M, Jayaprakash D, Dinesh Kumar B. Vitamin D deficiency in pregnant women impairs regulatory T cell function. J Steroid Biochem Mol Biol. (2015) 147:48–55. doi: 10.1016/j.jsbmb.2014.11.020
27. Wu Y, Zhen W, Geng Y, Wang Z, Guo Y. Pretreatment with probiotic Enterococcus faecium NCIMB 11181 ameliorates necrotic enteritis-induced intestinal barrier injury in broiler chickens. Sci Rep. (2019) 9:10256. doi: 10.1038/s41598-019-46578-x
28. Edgar RC. Search and clustering orders of magnitude faster than BLAST. Bioinformatics. (2010) 26:2460–1. doi: 10.1093/bioinformatics/btq461
29. Segata N, Izard J, Waldron L, Gevers D, Miropolsky L, Garrett W S, et al. Metagenomic biomarker discovery and explanation. Genome Biol. (2011) 12:R60. doi: 10.1186/gb-2011-12-6-r60
30. Morgan GIL. Predictive functional profiling of microbial communities using 16S rRNA marker gene sequences. Nature biotechnol. (2013) 9:814–21. doi: 10.1038/nbt.2676
31. Holick MF, Binkley NC, Bischoff-Ferrari HA, Gordon CM, Hanley DA, Heaney RP, et al. Evaluation, treatment, and prevention of vitamin D deficiency: an Endocrine Society clinical practice guideline. J Clin Endocrinol Metab. (2011) 96:1911–30. doi: 10.1210/jc.2011-0385
32. Geng Y, Ma Q, Wang Z, Guo Y. Dietary vitamin D(3) supplementation protects laying hens against lipopolysaccharide-induced immunological stress. Nutr Metab (Lond). (2018) 15:58. doi: 10.1186/s12986-018-0293-8
33. Wang J, Qiu L, Gong H, Celi P, Yan L, Ding X, et al. Effect of dietary 25-hydroxycholecalciferol supplementation and high stocking density on performance, egg quality, and tibia quality in laying hens. Poult Sci. (2020) 99:2608–15. doi: 10.1016/j.psj.2019.12.054
34. Zerwekh J. Blood biomarkers of Vitamin D status. Am J Clin Nutr. (2008) 87:1087S-91S. doi: 10.1093/ajcn/87.4.1087S
35. Pu Y, Zhu G, Xu Y, Zheng S, Tang B, Huang H, et al. Association between vitamin D exposure and head and neck cancer: a systematic review with meta-analysis. Front Immunol. (2021) 12:7226. doi: 10.3389/fimmu.2021.627226
36. El-Boshy M, BaSalamah M A, Ahmad J, Idris S, Mahbub A, Abdelghany A H, et al. Vitamin D protects against oxidative stress, inflammation and hepatorenal damage induced by acute paracetamol toxicity in rat. Free Radical Biology and Medicine. (2019) 141:310–21. https://doi.org/10.1016/j.freeradbiomed.2019.06.030
37. Raftery T, Merrick M, Healy M, Mahmud N, O'Morain C, Smith S, et al. Vitamin D status is associated with intestinal inflammation as measured by fecal calprotectin in crohn's disease in clinical remission. Dig Dis Sci. (2015) 60:2427–35. doi: 10.1007/s10620-015-3620-1
38. Huang FC, Huang SC. Active vitamin D3 attenuates the severity of Salmonella colitis in mice by orchestrating innate immunity. Immun Inflamm Dis. (2021) 9:481–91. doi: 10.1002/iid3.408
39. Ananthakrishnan AN, Nguyen GC, Bernstein CN. AGA clinical practice update on management of inflammatory bowel disease in elderly patients: expert review. Gastroenterology. (2021) 160:445–51. doi: 10.1053/j.gastro.2020.08.060
40. Turner J R. Intestinal mucosal barrier function in health and disease. Nat Rev Immunol. (2009) 9:799–809. doi: 10.1038/nri2653
41. Ouyang L, Shi Z, Zhao S, Wang FT, Zhou TT, Liu B, et al. Programmed cell death pathways in cancer: a review of apoptosis, autophagy and programmed necrosis. Cell Prolif. (2012) 90:487–98. doi: 10.1111/j.1365-2184.2012.00845.x
42. Adhikari P, Lee CH, Cosby DE, Cox NA, Kim WK. Effect of probiotics on fecal excretion, colonization in internal organs and immune gene expression in the ileum of laying hens challenged with Salmonella Enteritidis. Poult Sci. (2019) 98:1235–42. doi: 10.3382/ps/pey443
43. Yuki T, Yoshida H, Akazawa Y, Komiya A, Sugiyama Y, Inoue S. Activation of TLR2 enhances tight junction barrier in epidermal keratinocytes. J Immunol. (2011) 187:3230–7. doi: 10.4049/jimmunol.1100058
44. Fan L, Liu B, Han Z, Ren W. Insights into host-microbe interaction: What can we do for the swine industry? Animal Nutrition. (2021) 7:17–23. doi: 10.1016/j.aninu.2020.10.003
45. Tangestani H, Boroujeni H K, Djafarian K, Emamat H, Shab-Bidar S. Vitamin D and the gut microbiota: a narrative literature review. Clin Nutr Res. (2021) 10:181–91. doi: 10.7762/cnr.2021.10.3.181
46. Bellerba F, Muzio V, Gnagnarella P, Facciotti F, Chiocca S, Bossi P, et al. The association between vitamin D and gut microbiota: a systematic review of human studies. Nutrients. (2021) 13:3378. doi: 10.3390/nu13103378
47. Naderpoor N, Mousa A, Fernanda Gomez Arango L, Barrett HL, Dekker Nitert M, de Courten B. Effect of vitamin D supplementation on faecal microbiota: a randomised clinical trial. Nutrients. (2019) 11:2888. doi: 10.3390/nu11122888
48. Luthold RV, Fernandes GR. Franco-de-Moraes AC, Folchetti LG, Ferreira SR. Gut microbiota interactions with the immunomodulatory role of vitamin D in normal individuals. Metabolism. (2017) 69:76–86. doi: 10.1016/j.metabol.2017.01.007
49. Ranjbar R, Vahdati SN, Tavakoli S, Khodaie R, Behboudi H. Immunomodulatory roles of microbiota-derived short-chain fatty acids in bacterial infections. Biomed Pharmacotherapy. (2021) 141:111817. doi: 10.1016/j.biopha.2021.111817
50. Zuo K, Li J, Xu Q, Hu C, Gao Y, Chen M, et al. Dysbiotic gut microbes may contribute to hypertension by limiting vitamin D production. Clin Cardiol. (2019) 42:710–9. doi: 10.1002/clc.23195
51. Mayorgas A, Dotti I, Salas A. Microbial metabolites, postbiotics, and intestinal epithelial function. Mol Nutr Food Res. (2020) 65: 2000188. doi: 10.1002/mnfr.202000188
52. Schäffler H, Herlemann D P, Klinitzke P, Berlin P, Kreikemeyer B, Jaster R, et al. Vitamin D administration leads to a shift of the intestinal bacterial composition in Crohn's disease patients, but not in healthy controls. J Dig Dis. (2018) 19:225–34. doi: 10.1111/1751-2980.12591
53. Yoon S, Wu S, Zhang YG, Lu R, Petrof E, Yuan L, et al. Probiotic regulation of vitamin D receptor in intestinal inflammation. Gastroenterology. (2011) 5:140: doi: 10.1016/S0016-5085(11)60075-9
54. Muras A, Romero M, Mayer C, Otero A. Biotechnological applications of Bacillus licheniformis. Crit Rev Biotechnol. (2021) 41:609–27. doi: 10.1080/07388551.2021.1873239
55. Duval M, Simonetti A, Caldelari I, Marzi S. Multiple ways to regulate translation initiation in bacteria: mechanisms, regulatory circuits, dynamics. Biochimie. (2015) 114:18–29. doi: 10.1016/j.biochi.2015.03.007
56. Lyons PP, Turnbull JF, Dawson KA, Crumlish M. Phylogenetic and functional characterization of the distal intestinal microbiome of rainbow trout Oncorhynchus mykiss from both farm and aquarium settings. J App Microbiol. (2017) 122:347–63. doi: 10.1111/jam.13347
57. Lin Y, Xu S, Zeng D, Ni X, Zhou M, Zeng Y, et al. Disruption in the cecal microbiota of chickens challenged with Clostridium perfringens and other factors was alleviated by Bacillus licheniformis supplementation. PLoS ONE. (2017) 12:e0182426. doi: 10.1371/journal.pone.0182426
58. Bonaiuto E, Grancara S, Martinis P, Stringaro A, Paolo MLD. A novel enzyme with spermine oxidase properties in bovine liver mitochondria: Identification and kinetic characterization. Free Rad Biol Med. (2015) 81:88–99. doi: 10.1016/j.freeradbiomed.2015.01.001
Keywords: Vitamin D, Salmonella, intestinal health, gut microbiota, laying hen
Citation: Guo F, Geng Y, Abbas W, Zhen W, Wang S, Huang Y, Guo Y, Ma Q and Wang Z (2022) Vitamin D3 Nutritional Status Affects Gut Health of Salmonella-Challenged Laying Hens. Front. Nutr. 9:888580. doi: 10.3389/fnut.2022.888580
Received: 03 March 2022; Accepted: 06 April 2022;
Published: 10 May 2022.
Edited by:
Guillermo Tellez-Isaias, University of Arkansas, United StatesReviewed by:
Victor Manuel Petrone-García, National Autonomous University of Mexico, MexicoCopyright © 2022 Guo, Geng, Abbas, Zhen, Wang, Huang, Guo, Ma and Wang. This is an open-access article distributed under the terms of the Creative Commons Attribution License (CC BY). The use, distribution or reproduction in other forums is permitted, provided the original author(s) and the copyright owner(s) are credited and that the original publication in this journal is cited, in accordance with accepted academic practice. No use, distribution or reproduction is permitted which does not comply with these terms.
*Correspondence: Zhong Wang, d2FuZ3poQGNhdS5lZHUuY24=
Disclaimer: All claims expressed in this article are solely those of the authors and do not necessarily represent those of their affiliated organizations, or those of the publisher, the editors and the reviewers. Any product that may be evaluated in this article or claim that may be made by its manufacturer is not guaranteed or endorsed by the publisher.
Research integrity at Frontiers
Learn more about the work of our research integrity team to safeguard the quality of each article we publish.