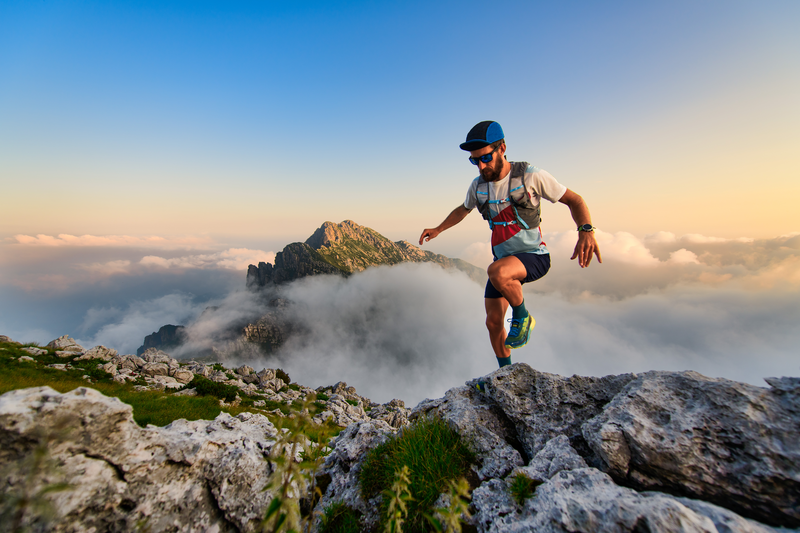
95% of researchers rate our articles as excellent or good
Learn more about the work of our research integrity team to safeguard the quality of each article we publish.
Find out more
MINI REVIEW article
Front. Nutr. , 04 July 2022
Sec. Nutrition and Metabolism
Volume 9 - 2022 | https://doi.org/10.3389/fnut.2022.886291
This article is part of the Research Topic Infant and Child Nutrition, Physical Activity, Oxidative Stress and Inflammatory Signaling View all 12 articles
Childhood obesity is identified as one of the major public health issues to increase the risk for cardiometabolic diseases and related complications in adulthood. The literature has supported inflammation and oxidative stress as the primary underlying mechanisms involved in the pathogenesis of obesity-related diseases. Epidemiological evidence consistently shows the benefits of physical activity in the improvement of obesity-mediated inflammation and oxidative stress status. In this narrative mini-review, the available scientific evidence on the potential effects of exercise in alleviating these susceptibilities in childhood obesity will be assessed.
Childhood obesity is considered as one of the most important public health issues, with approximately 5 times more likely to develop obesity in adulthood (1). Obesity-attributable illnesses, such as cardiovascular disease have contributed to more than two-thirds of deaths globally, according to data from the Global Burden of Disease study (2). One of the underlying mechanisms contributing to obesity-related diseases is the development of systemic low-grade inflammation derived from adipose tissue (3, 4). While chronic inflammation is often associated with increased oxidative stress (5), the literature has previously reported the role of oxidative stress in the pathogenesis of obesity-related diseases (6). Other possible contributors to elevated oxidative stress in obesity include, but not limited to, hyperglycemia, vitamin and mineral deficiencies, hyperleptinemia, endothelial dysfunction, and impaired mitochondrial function (7). Intricately, the damage of cellular proteins by reactive oxygen species (ROS) triggers the inflammatory responses to target the pattern recognition receptors, located either on the cell membrane [e.g., Toll-like receptors (TLRs)] (8) or in the cytoplasm [e.g., NOD-like receptors (NLR)] (9), thereby leading to the activation of various transcription factors, including NF-kB signaling (10) (Figure 1).
Figure 1. Inflammation and oxidative stress responses to childhood obesity and exercise in obese children. Localized inflammation in adipose tissue related to obesity triggers low-grade systemic inflammation, manifesting in a general increase in circulating inflammatory markers in obese children. Childhood obesity concomitates with an oxidative status imbalance, increasing the oxidative stress markers and decreasing the antioxidant systems. Different protocols of physical activity might revert the consequences of obesity on inflammatory processes and oxidative stress during child growth. 8-epi-PGF2α, 8-epi-prostaglandin F2 alpha; AOPPs, advanced oxidation protein products; CRP, C-reactive protein; GPX, glutathione peroxidase; GSH, reduced glutathione; IL-6, interleukin 6; LPO, lipoperoxides; MDA, malonaldehyde; ox-LDL, oxidized low-density lipoproteins; TNF-α, tumor necrosis factor alpha.
Increased physical activity in childhood obesity is an effective intervention to establish a healthy adult lifestyle in reducing the incidence of chronic diseases, such as diabetes (11, 12). In fact, the decline or lack of physical activity in different environments, especially in schools, could contribute to the development of overweight and obesity and their related health problems (13, 14), along with the associated proinflammatory state (15). In this regard, physical activity has been found to be the main intervention to lose or at least maintain body weight in overweight, obese, or severely obese adults, with the corresponding improvement in the cardiorespiratory fitness level (16). The maintenance of a physically active lifestyle in childhood is the basic to prevent or reduce the risk for cardiometabolic diseases and related complications in adulthood (17). Research in high-fat diet (HFD)-induced obese mice age ranged from 4 to 20 weeks has proven that exercise could prevent the obesity-entailed infiltration of inflammatory macrophages into adipose tissue, in support of a reduction in the pro-inflammatory cytokine production (18, 19). Furthermore, the suppression of neutrophil infiltration into fat tissue, along with the inhibition of the expression of elastase or monocyte chemoattractant protein-1 (MCP-1), was also observed with exercise training in this mouse model (20). Of important note, downregulation of inflammatory mediators/cytokines in an in vivo rat model of early obesity in response to exercise training has been found (21). In obese children, a meta-analysis has also revealed the beneficial effect of regular physical activity in the modulation of leptin, adiponectin, and interleukin (IL)-6 inflammatory markers (22). Furthermore, regular exercise contributes to the treatment of non-alcoholic fatty liver disease (NAFLD), with a reduction on intrahepatic fat, along with an increase in β-oxidation of fatty acids and a reduction in ROS via increased antioxidant enzyme activity (23). Although elevated oxidative stress has been shown to correlate with a deficiency of vitamin D (24) and index of insulin resistance in obese children (25), it is likely that lifestyle interventions including physical activity and dietary modification would improve oxidative status in this population (26) (Figure 1). Thus, this mini-review aims to evaluate the impact of childhood obesity on inflammation and oxidative stress status and the beneficial effects of exercise on these modifications.
A recent review has reported significant alterations in the quantity and activation of both peripheral and tissue immune cells in obese children, although the obesity-related immune function in childhood still remains to be further investigated due to the limited data from the literature (27). As presented in Table 1.1A, increased levels of C-reactive protein (CRP) and neutrophil count were found to positivity correlate with body fat mass in children and adolescents aged 7–18 years (28, 29). Similarly, Vehapoglu et al. (30) reported increased levels of CRP, white blood cell, and neutrophil count, as well as the neutrophil/lymphocyte ratio, which were positively correlated with BMI and index of insulin resistance, demonstrating the obesity-induced chronic low-grade inflammation in childhood (2–11 years). In agreement with these findings, an increase in the circulating levels of proinflammatory cytokines such as leptin, tumor necrosis factor (TNF)-α, IL-6, and CRP was also observed in obese compared to normal-weight children and adolescents aged 2–18 years (30–39), with a concomitant reduction of adiponectin levels in a similar age group (5–18 years) (34, 37, 40). Additionally, higher levels of plasma hs-CRP, TFN-α, and leptin were reported in both overweight and obese children, while MCP-1 was only found to be higher in the obese than overweight and control subjects with no differences in IL-6 and adiponectin among groups (41). In this regard, obese children (4–14 years) with carbohydrate metabolic impairment have been shown to exhibit an elevation in the concentrations of pro-inflammatory cytokines (e.g., CRP, IL6, TNF-α) compared to controls (36). Importantly, Carolan et al. (34) further examined the phenotype and activation of immune cells and found a shift of pro-inflammatory M1 macrophages as evidenced by elevated soluble CD163 level, along with a greater capacity of peripheral blood mononuclear cells (PBMCs) to produce IL-1β ex vivo following lipopolysaccharide (LPS) stimulation in obese vs. non-obese children and adolescents aged 6–18 years. A similar observation was also reported by Schipper et al. (42), showing that obese children (6–16 years) elicited an increase in the numbers of classical (CD14++CD16–) monocytes, as well as the levels of CD11b and IL-6 after LPS treatment compared to non-obese children. Taken together, these findings might partially support the macrophages infiltration and activation associated with obesity-induced inflammation as previously discussed. Additionally, in an interesting study evaluating the levels of several inflammatory cytokines in the saliva of children with dental caries, results showed increased levels of IL-6 and IL-15 in overweight/obese compared to normal-weight children aged 3–8 years (43). In an attempt to evaluate the gender effect of childhood obesity in the circulating levels of inflammatory cytokines, Murdolo et al. (38) showed that obese girls presented elevated IL-8, IL-18, MCP-1, and soluble intercellular adhesion molecule-1 levels (sICAM-1), whereas only obese boys exhibited increased macrophage migration inhibitory factor (MIF) levels. However, although the concentration of appetite-stimulating hormone, adiponectin, was higher in normal-weight girls compared to boys age ranged from 5 to 13 years, no sex by BMI group interaction was found in this study (38). Overall, these outcomes confirm the obesity-induced chronic low-grade inflammation during childhood and propose the elevation of immune cell activation, such as macrophage infiltration, with limited gender influence during puberty.
Table 1. Effects of childhood obesity (1) and exercise (2) in obese children on inflammation (A) and oxidative stress (B) markers.
Both epidemiologic and longitudinal research have supported that regular exercise or increased physical activity is an effective strategy in reducing systemic low-level inflammation in individuals with obesity and related conditions (44–46). As summarized in Table 1.2A, Nascimento et al. (47) conducted an 8-month longitudinal study in investigating the effects of aerobic exercise training on the inflammatory status in childhood (5–17 years) obesity and observed a reduction in the levels of TNF-α and CRP, along with an improvement in lipid profile and insulin resistance. These findings are partially supported by Nemet et al. (48), showing an increase in adiponectin and a decrease in CRP, along with no changes in leptin and IL-6 levels in obese self-referred children aged 6–13 years following a 3-month combined nutritional-behavioral-physical activity intervention. Interestingly, Woo et al. (37) found a significant increase but a reduction in the serum levels of adiponectin and leptin, respectively, following a minimum of 12 weeks of aerobic training in obese children (the average age of 11 years), despite no change in the percentage of body fat. In contrast, Kelly et al. (49, 50) did not observe any effect of 8 weeks of stationary cycling intervention in plasma CRP, IL-6, TNF-α, adiponectin, and leptin levels, as well as change in weight or body composition in overweight children in the same range of age. Similarly, no significant difference was found in serum adiponectin, IL-6, and CRP levels in overweight and obese girls aged 9–15 years in response to 12-week aerobic training (51). Additionally, although Blüher et al. (51) reported a decrease in serum leptin following the obesity therapy program, including 150 min/week of physical activity for 39 weeks, no change was observed in adiponectin and CRP levels in these overweight/obese children, independently of gender, age, or pubertal stage. Finally, in a recent study examining the effect of a 12-week combined strength and endurance training program in PBMCs of obese pediatric patients aged 7–12 years, a lower NLRP3 inflammasome (a regulator of innate immune activity/pro-inflammatory response) activation, but not TLR4 was reported (52). Similar contradictions have been described in obese adolescents conducting long-term resistance and aerobic exercise programs, showing either decreases or no changes in pro-inflammatory markers such as CRP, IL-6, leptin, or TNF-α (53–58). Additionally, while a reduction in the number of neutrophils and the expression of TNF-α was observed after a 6-month high-intensity exercise, an opposite response was found with the low-intensity protocol (53). Thus, these contradictory findings in the improvement of exercise-mediated inflammation in childhood obesity may be the result of various intensities and/or duration of the intervention, and other factors including dietary changes could also alter these inflammatory profiles.
Oxidative stress is another underlying mechanism involved in the pathogenesis of obesity-related diseases. Regarding the oxidative status (Table 1.1B), research has demonstrated lower antioxidant activities and higher oxidation products in obese vs. non-obese children aged 6–12 years (59). Thus, an increase in total oxidant capacity (TOC) (39) and a decrease in total antioxidant capacity (TAC) (39, 40), as well as a depletion in total antioxidant status (TAS) and total thiols, and a rise in total oxidation status (TOS) (30) were reported in obese children aged 2–13 years compared to their normal-weight counterparts. Moreover, the erythrocyte activities of superoxide dismutase (SOD), catalase, glutathione peroxidase (GPX), glutathione reductase (GR), and reduced glutathione concentration (GSH) were lower in obese children aged 6–12 years than their counterparts, whereas these antioxidant activities in erythrocyte (59) and serum TAS (29) were increased in overweight compared to normal-weight children aged 7–15 years, suggesting the inability to counterbalance the detrimental effects or the highly production of ROS in obesity. The results of this oxidative stress status from these obese children are in agreement with the study by Lechuga–Sancho et al. (36), demonstrating lower erythrocyte levels of catalase, GSH, and TAC in children aged 4–14 years. However, the contradictory findings have been reported including an increase in SOD and no differences in catalase and GPX activity in erythrocytes (60), elevated GPX and no changes in SOD in PBMCs (37), and no differences in plasma SOD and GSH levels (33) between obese and normal-weight children aged 6–12 years. On the other hand, obese children aged 6–12 years exhibited elevated levels of erythrocyte malondialdehyde (MDA) as well as both plasma oxidized low-density lipoproteins (ox-LDL) and advanced oxidation protein products (AOPPs), while no difference was reported in these markers between overweight and normal-weight groups (59). In support of these results, elevated levels of ox-LDL (61, 62) and 8-epi-prostaglandin F2 alpha (31, 33, 36, 40) have been shown to be higher in obese than normal-weight children aged 4–18 years, along with an elevation in circulating levels of MDA, and AOPPs (31). Importantly, Codoñer–Franch et al. (63) have previously demonstrated an increase in plasma MDA and carbonyl groups (CG), as well as erythrocyte GPX activity levels, along with decreased levels in erythrocyte GSH, in severely obese children only, whereas the levels of α-tocopherol and β-carotene, lipophilic antioxidants that act in biomembranes as scavengers of free radicals, remained similar as obese and normal-weight children aged 6–14 years. Although another investigation by Codoñer–Franch et al. (64) showed that the levels of plasma lipoperoxides (LPO), CG, and the antioxidants α-tocopherol and β-carotene, as well as the GPX erythrocyte activity were increased in obese children in the same range of age, plasma MDA levels and erythrocyte GSH concentration did not differ between groups. Similar results have been presented in obese infants aged 3–5 years, showing higher serum peroxidized lipids, specifically thiobarbituric acid-reacting substances (TBARS), plasmatic ox-LDLs and oxidized glutathione (GSSG) levels, as well as lower plasmatic GSH values, compared with their matched controls (65). Thus, the inconsistence of the above findings could potentially be due to the wide range of age groups utilized in these studies. To further clarify this potential effect of age, Rupérez et al. (66) differentiated children aged 3–17 years during pre/pubertal period and found that TAC levels were lower in prepubertal but higher in pubertal overweight/obese children than normal-weight children. Furthermore, the advanced glycation end products (AGEs) formation (a facilitator of ROS), measured as skin autofluorescence, was increased in obese children and adolescents aged 4–18 years, although no differences were shown in the group of children under 10 years old (67). These observations might be partially supported by the transient decline in insulin sensitivity during puberty attributable to lack of glycemic control (68, 69). In contrast, a high level of serum ox-LDL observed in obese children (the mean age = 11 years) was not correlated with sex, age, and pubertal status (62). It is reasonably speculated that the metabolic status and the presence of the metabolic syndrome could have influenced these reported results, as evidenced by Rupérez et al. (66) with lower carotenes and tocopherols levels in metabolically unhealthy vs. healthy children aged 3–17 years. In this regard, Faienza et al. (35) presented similar results, demonstrating a higher level of diacron reactive oxygen metabolites (d-ROMs) in obese children and prepubertal subjects (the average age of 11 years) with metabolic syndrome, with a negative relationship between total antioxidant capacity and standardized BMI. Thus, these findings suggest that an early onset in the pathogenesis of obesity-mediated diseases by elevated oxidative stress may occur during pediatric age.
Although there is limited research investigating the impact of physical activity interventions on childhood obesity-associated oxidative stress (Table 1.2B), acute aerobic cycling exercise seems to exert contrary effects on oxidative markers in obese children. Specifically, Ahmadian et al. (70) reported a decrease in MDA levels after an acute progressive test until volitional exhaustion in both obese and normal-weight children under the age of 13 years, as well as in their asthmatic counterparts (70), Paltoglou et al. (71) reported an increase in the levels of TBARS and protein carbonyls (PCs), as well as in TAC, and catalase, along with a decrease in the production of GSH and GSH/GSSG in both prepubertal and pubertal obese children following an acute bout of maximal aerobic exercise. These responses to acute aerobic exercise were also observed in their prepubertal and pubertal normal-weight counterparts. On the other hand, neither an 8-week nor 13-week aerobic training program improved the levels of plasma 8-isoprostane (50) or serum F2-isoprostane (72) in overweight/obese children aged 7–11 years. Contrary to these results, a 12-week training exercise was effective to increase both SOD and GPX mRNA expression in PBMCs from both obese and normal-weight children (the mean age = 11 years) (37). Interestingly, this study reported that the expression of SOD and GPX mRNA remained elevated until completion of a 24-week training intervention in the obese training group, but not the obese detraining group who participated in the 12-week training plus 12-week detraining program. Similarly, an elevation in plasma levels of SOD and GPX following 24 weeks of exercise program in both obese training and detraining groups was observed, whereas only obese detraining group showed a decrease in GPX and no changes in SOD level at the mid-training (12 weeks) (37). The phenomenon of this observation might present a different behavior or time course of these antioxidant enzyme responses, depending on the oxidation-reduction transcription factors involved in the regulatory processes. Overall, these results indicate that chronic exercise could possibly elicit protective adaptations against oxidative damage in childhood obesity as the effective training interventions have been administered in adult obesity (73, 74).
Finally, oxidative stress is frequently associated with obesity-induced inflammation. Although the mechanisms for childhood obesity-induced oxidative stress remain to be investigated, leptin has been proposed as an important contributor, due to its role in mediating pro-inflammatory state in obese individuals (75, 76). Particularly, increased leptin has been shown to modulate the production of oxidative stress biomarkers, such as reduced nitric oxide (NO), increased superoxide (O2–) and peroxynitrite (ONOO-) in both human endothelial cells and the endothelium of obese mice (77, 78). With a reduction in the circulating level of leptin following training in children and adolescences aged 7–18 years (79) and its potential relationship with oxidative stress (80), this could partially support the role of exercise interventions for oxidative adaptions in alleviating susceptibility to obesity-associated oxidative stress.
Results from this mini-review evidence that localized inflammation in adipose tissue triggers low-grade systemic inflammation in childhood obesity. Similarly, an increase in oxidative stress markers, as well as a decrease in antioxidant markers in obese children present an imbalance in oxidative stress status. However, the effects of physical activity on the inflammatory and oxidative stress responses in growing obese kids still remain inconclusive. Regarding oxidative status, although the studies available to date are not sufficient to conclude the beneficial effect of exercise in obese children, the influence of puberty in childhood obesity-mediated oxidative stress is warranted for further investigation. Moreover, the research addressing the role of several key hormones (e.g., testosterone, estrogen, and growth hormone) on the obesity-associated inflammatory and oxidative stress processes in the different growth stages of children is needed to further discover their exercise modulation, including a limited age range with controlling for biological and chronological age. In summary, additional insight into how physical activity interventions influence the cellular adaptations of inflammation and oxidative stress is necessary to better understand the importance of exercise as an antagonist to the current childhood obesity epidemic.
BE, MJC, and JG-G conceptualized the manuscript. BE and MR-V performed the literature search. BE, C-JH, and MJC performed manuscript drafting. MJC and JG-G supervised and revised the manuscript. All authors contributed to the article and approved the submitted version.
This project was supported by grants from the EXERNET Research Network on Physical Exercise and Health (09/UPB/19 and 45/UPB/20) and the IN MOTU SALUS Research Network on Molecular and Cellular Basis of Physical Exercise for Health and Performance (14/UPB/19 and 09/UPB/20).
The authors declare that the research was conducted in the absence of any commercial or financial relationships that could be construed as a potential conflict of interest.
All claims expressed in this article are solely those of the authors and do not necessarily represent those of their affiliated organizations, or those of the publisher, the editors and the reviewers. Any product that may be evaluated in this article, or claim that may be made by its manufacturer, is not guaranteed or endorsed by the publisher.
8-epi-PGF2 α or 8-isoprostane or F2 isoprostate or F2-IsoP, 8-epi-prostaglandin F2 alpha; AGEs, advanced glycation end products; AOPPs, advanced oxidation protein products; BAP, biological antioxidant potential; BMI, body mass index; CASP-1, caspase 1; CG, carbonyl groups; CD, cluster of differentiation; CD14++CD16–, classical monocytes; CD14+CD16++, non-classical monocytes; ConA, concanavalin A; CRP, C-reactive protein; d, days; d-ROMs, diacron reactive oxygen metabolites; EGF, epidermal growth factor; F, female; GPX or GSH-Px, glutathione peroxidase; GSH, reduced glutathione; GSSG, oxidized glutathione; HGF, Hepatocyte growth factor; HRR, heart rate recovery; hs-CRP, high sensitivity CRP; IFN- γ, interferon-gamma; IL, interleukin; IP-10, IFN- γ -induced protein 10; LC, lymphocyte count; LPO, lipoperoxides; LPS, lipopolysaccharide; M, male; MCP-1, monocyte chemoattractant protein 1; MDA, malonaldehyde; MIF, macrophage migration inhibitory factor; NC, neutrophil count; NF-kB, nuclear factor NF-kappa-B; NLR, neutrophil/lymphocyte ratio; NLRP3, nod like receptor family pyrin domain containing 3; ob, obese; OPN, osteopontin; ow, overweight; OSI, Oxidative stress index; ox-LDL, oxidized low-density lipoproteins; pb, pubertal; PBMCs, peripheral blood mononuclear cells; PCs, protein carbonyls; ppb, prepubertal; RANTES, regulated on activation, normal T-cell expressed and secreted; RBP, retinol-binding protein; sCD163, soluble hemoglobin scavenger receptor CD163; sICAM, soluble intercellular adhesion molecule; SOD, superoxide dismutase; sTNFR, soluble tumor necrosis factor receptor; TAC, total antioxidant capacity; TAG, triacylglycerols; TAS, total antioxidant status; TBARS, thiobarbituric acid reactive substances; tGSH, total glutathione equivalents; TIMP-1, metalloproteinase inhibitor 1; TLR4, toll like receptor 4; TNF- α, tumor necrosis factor-alpha; TOC, total oxidant capacity; TOS, Total oxidant status; VO2max, maximal oxygen uptake; vs., versus; w, week; WBC, white blood cell.
1. Simmonds M, Llewellyn A, Owen CG, Woolacott N. Predicting adult obesity from childhood obesity: a systematic review and meta-analysis. Obes Rev. (2016) 17:95–107. doi: 10.1111/obr.12334
2. Afshin A, Forouzanfar MH, Reitsma MB, Sur P, Estep K, Lee A, et al. Health Effects of overweight and obesity in 195 countries over 25 years. N Engl J Med. (2017) 377:13–27. doi: 10.1056/NEJMoa1614362
3. Karczewski J, Śledzińska E, Baturo A, Jończyk I, Maleszko A, Samborski P, et al. Obesity and inflammation. Eur Cytokine Netw. (2018) 29:83–94. doi: 10.1684/ecn.2018.0415
4. Juárez-Fernández M, Porras D, Petrov P, Román-Sagüillo S, García-Mediavilla MV, Soluyanova P, et al. The synbiotic combination of Akkermansia muciniphila and quercetin ameliorates early obesity and NAFLD through gut microbiota reshaping and bile acid metabolism modulation. Antioxidants (Basel). (2021) 10:2001. doi: 10.3390/antiox10122001
5. Rius-Pérez S, Torres-Cuevas I, Millán I, Ortega Á, Pérez S. Pgc-1α, inflammation, and oxidative stress: an integrative view in metabolism. Oxid Med Cell Longev. (2020) 2020:1452696. doi: 10.1155/2020/1452696
6. Savini I, Catani MV, Evangelista D, Gasperi V, Avigliano L. Obesity-associated oxidative stress: strategies finalized to improve redox state. Int J Mol Sci. (2013) 14:10497–538. doi: 10.3390/ijms140510497
7. Manna P, Jain SK. Obesity, oxidative stress, adipose tissue dysfunction, and the associated health risks: causes and therapeutic strategies. Metab Syndr Relat Disord. (2015) 13:423–44. doi: 10.1089/met.2015.0095
8. Ringseis R, Eder K, Mooren FC, Krüger K. Metabolic signals and innate immune activation in obesity and exercise. Exerc Immunol Rev. (2015) 21:58–68.
9. Martins JD, Liberal J, Silva A, Ferreira I, Neves BM, Cruz MT. Autophagy and inflammasome interplay. DNA Cell Biol. (2015) 34:274–81. doi: 10.1089/dna.2014.2752
10. Lingappan K. Nf-K b in oxidative stress. Curr Opin Toxicol. (2018) 7:81–6. doi: 10.1016/j.cotox.2017.11.002
11. Hallal PC, Victora CG, Azevedo MR, Wells JC. Adolescent physical activity and health: a systematic review. Sports Med. (2006) 36:1019–30.
12. Kumar S, Kelly AS. Review of childhood obesity: from epidemiology, etiology, and comorbidities to clinical assessment and treatment. Mayo Clin Proc. (2017) 92:251–65. doi: 10.1016/j.mayocp.2016.09.017
13. Hills AP. Exercise: an integral and non-negotiable component of a healthy lifestyle. Eur J Clin Nutr. (2018) 72:1320–2. doi: 10.1038/s41430-018-0254-3
14. Yuksel HS, Şahin FN, Maksimovic N, Drid P, Bianco A. School-based intervention programs for preventing obesity and promoting physical activity and fitness: a systematic review. Int J Environ Res Public Health. (2020) 17:347. doi: 10.3390/ijerph17010347
15. Merlin M, de Oliveira HH, Passos MEP, Momesso CM, Dos Santos de Oliveira LC, Santana JE, et al. Relationship between children physical activity, inflammatory mediators and lymphocyte activation: possible impact of social isolation (Covid-19). Sport Sci Health. (2020) 17:431–9. doi: 10.1007/s11332-020-00719-2
16. Jakicic JM, Davis KK. Obesity and physical activity. Psychiatr Clin North Am. (2011) 34:829–40. doi: 10.1016/j.psc.2011.08.009
17. Hills AP, Andersen LB, Byrne NM. Physical activity and obesity in children. Br J Sports Med. (2011) 45:866–70. doi: 10.1136/bjsports-2011-090199
18. Kawanishi N, Mizokami T, Yano H, Suzuki K. Exercise attenuates M1 macrophages and Cd8+ T cells in the adipose tissue of obese mice. Med Sci Sports Exerc. (2013) 45:1684–93. doi: 10.1249/MSS.0b013e31828ff9c6
19. Kawanishi N, Niihara H, Mizokami T, Yano H, Suzuki K. Exercise training attenuates adipose tissue fibrosis in diet-induced obese mice. Biochem Biophys Res Commun. (2013) 440:774–9. doi: 10.1016/j.bbrc.2013.10.004
20. Kawanishi N, Niihara H, Mizokami T, Yada K, Suzuki K. Exercise training attenuates neutrophil infiltration and elastase expression in adipose tissue of high-fat-diet-induced obese mice. Physiol Rep. (2015) 3:e12534. doi: 10.14814/phy2.12534
21. Carbajo-Pescador S, Porras D, García-Mediavilla MV, Martínez-Flórez S, Juarez-Fernández M, Cuevas MJ, et al. Beneficial effects of exercise on gut microbiota functionality and barrier integrity, and gut-liver crosstalk in an in vivo model of early obesity and non-alcoholic fatty liver disease. Dis Model Mech. (2019) 12:dmm039206. doi: 10.1242/dmm.039206
22. Sirico F, Bianco A, D’Alicandro G, Castaldo C, Montagnani S, Spera R, et al. Effects of physical exercise on adiponectin, leptin, and inflammatory markers in childhood obesity: systematic review and meta-analysis. Child Obes. (2018) 14:207–17. doi: 10.1089/chi.2017.0269
23. Farzanegi P, Dana A, Ebrahimpoor Z, Asadi M, Azarbayjani MA. Mechanisms of beneficial effects of exercise training on non-alcoholic fatty liver disease (NAFLD): roles of oxidative stress and inflammation. Eur J Sport Sci. (2019) 19:994–1003. doi: 10.1080/17461391.2019.1571114
24. Codoñer-Franch P, Tavárez-Alonso S, Simó-Jordá R, Laporta-Martín P, Carratalá-Calvo A, Alonso-Iglesias E. Vitamin D status is linked to biomarkers of oxidative stress, inflammation, and endothelial activation in obese children. J Pediatr. (2012) 161:848–54. doi: 10.1016/j.jpeds.2012.04.046
25. Ozgen IT, Tascilar ME, Bilir P, Boyraz M, Guncikan MN, Akay C, et al. Oxidative stress in obese children and its relation with insulin resistance. J Pediatr Endocrinol Metab. (2012) 25:261–6. doi: 10.1515/jpem-2011-0397
26. Montero D, Walther G, Perez-Martin A, Roche E, Vinet A. Endothelial dysfunction, inflammation, and oxidative stress in obese children and adolescents: markers and effect of lifestyle intervention. Obes Rev. (2012) 13:441–55. doi: 10.1111/j.1467-789X.2011.00956.x
27. Fang X, Henao-Mejia J, Henrickson SE. Obesity and immune status in children. Curr Opin Pediatr. (2020) 32:805–15. doi: 10.1097/MOP.0000000000000953
28. Singer K, Eng DS, Lumeng CN, Gebremariam A, Lee JM. The Relationship between body fat mass percentiles and inflammation in children. Obesity (Silver Spring). (2014) 22:1332–6. doi: 10.1002/oby.20710
29. Jaksic M, Martinovic M, Gligorovic-Barhanovic N, Vujacic A, Djurovic D, Nedovic-Vukovic M. Association between inflammation, oxidative stress, vitamin D, copper and zinc with pre-obesity and obesity in school children from the city of Podgorica, Montenegro. J Pediatr Endocrinol Metab. (2019) 32:951–7. doi: 10.1515/jpem-2019-0086
30. Vehapoglu A, Turkmen S, Goknar N, Özer Ö. Reduced antioxidant capacity and increased subclinical inflammation markers in prepubescent obese children and their relationship with nutritional markers and metabolic parameters. Redox Rep. (2016) 21:271–80.
31. Codoñer-Franch P, Tavárez-Alonso S, Murria-Estal R, Megías-Vericat J, Tortajada-Girbés M, Alonso-Iglesias E. Nitric oxide production is increased in severely obese children and related to markers of oxidative stress and inflammation. Atherosclerosis. (2011) 215:475–80. doi: 10.1016/j.atherosclerosis.2010.12.035
32. Mărginean CO, Meliţ LE, Huţanu A, Ghiga DV, Săsăran MO. The adipokines and inflammatory status in the era of pediatric obesity. Cytokine. (2020) 126:154925. doi: 10.1016/j.cyto.2019.154925
33. Oliver SR, Rosa JS, Milne GL, Pontello AM, Borntrager HL, Heydari S, et al. Increased oxidative stress and altered substrate metabolism in obese children. Int J Pediatr Obes. (2010) 5:436–44. doi: 10.3109/17477160903545163
34. Carolan E, Hogan AE, Corrigan M, Gaotswe G, O’Connell J, Foley N, et al. The impact of childhood obesity on inflammation, innate immune cell frequency, and metabolic microrna expression. J Clin Endocrinol Metab. (2014) 99:E474–8. doi: 10.1210/jc.2013-3529
35. Faienza MF, Francavilla R, Goffredo R, Ventura A, Marzano F, Panzarino G, et al. Oxidative stress in obesity and metabolic syndrome in children and adolescents. Horm Res Paediatr. (2012) 78:158–64. doi: 10.1159/000342642
36. Lechuga-Sancho AM, Gallego-Andujar D, Ruiz-Ocaña P, Visiedo FM, Saez-Benito A, Schwarz M, et al. Obesity induced alterations in redox homeostasis and oxidative stress are present from an early age. PLoS One. (2018) 13:e0191547. doi: 10.1371/journal.pone.0191547
37. Woo J, Shin KO, Yoo JH, Park S, Kang S. The effects of detraining on blood adipokines and antioxidant enzyme in korean overweight children. Eur J Pediatr. (2012) 171:235–43. doi: 10.1007/s00431-011-1518-2
38. Murdolo G, Nowotny B, Celi F, Donati M, Bini V, Papi F, et al. Inflammatory adipokines, high molecular weight adiponectin, and insulin resistance: a population-based survey in prepubertal schoolchildren. PLoS One. (2011) 6:e17264. doi: 10.1371/journal.pone.0017264
39. Rowicka G, Dyląg H, Ambroszkiewicz J, Riahi A, Weker H, Chełchowska M. Total oxidant and antioxidant status in prepubertal children with obesity. Oxid Med Cell Longev. (2017) 2017:5621989. doi: 10.1155/2017/5621989
40. Araki S, Dobashi K, Yamamoto Y, Asayama K, Kusuhara K. Increased plasma isoprostane is associated with visceral fat, high molecular weight adiponectin, and metabolic complications in obese children. Eur J Pediatr. (2010) 169:965–70. doi: 10.1007/s00431-010-1157-z
41. Chang CJ, Jian DY, Lin MW, Zhao JZ, Ho LT, Juan CC. Evidence in obese children: contribution of hyperlipidemia, obesity-inflammation, and insulin sensitivity. PLoS One. (2015) 10:e0125935. doi: 10.1371/journal.pone.0125935
42. Schipper HS, Nuboer R, Prop S, van den Ham HJ, de Boer FK, Kesmir Ç, et al. Systemic inflammation in childhood obesity: circulating inflammatory mediators and activated Cd14++ monocytes. Diabetologia. (2012) 55:2800–10. doi: 10.1007/s00125-012-2641-y
43. Ramírez-De Los Santos S, López-Pulido EI, Medrano-González IDC, Becerra-Ruiz JS, Alonso-Sanchez CC, Vázquez-Jiménez SI, et al. Alteration of cytokines in saliva of children with caries and obesity. Odontology. (2021) 109:11–7. doi: 10.1007/s10266-020-00515-x
44. Diniz TA, Antunes BM, Little JP, Lira FS, Rosa-Neto JC. Exercise training protocols to improve obesity, glucose homeostasis, and subclinical inflammation. In: PC Guest editor. Physical Exercise and Natural and Synthetic Products in Health and Disease. Methods in Molecular Biology. (Vol. 2343), Humana, NY: Springer US (2022). doi: 10.1007/978-1-0716-1558-4_7
45. Valeria Oliveira de Sousa B, de Freitas DF, Monteiro-Junior RS, Mendes IHR, Sousa JN, Guimarães VHD, et al. Physical exercise, obesity, inflammation and neutrophil extracellular traps (Nets): a review with bioinformatics analysis. Mol Biol Rep. (2021) 48:4625–35. doi: 10.1007/s11033-021-06400-2
46. Gonzalez-Gil AM, Elizondo-Montemayor L. The role of exercise in the interplay between myokines, hepatokines, osteokines, adipokines, and modulation of inflammation for energy substrate redistribution and fat mass loss: a review. Nutrients. (2020) 12:1899. doi: 10.3390/nu12061899
47. Nascimento H, Alves AI, Medeiros AF, Coimbra S, Catarino C, Bronze-da-Rocha E, et al. Impact of a school-based intervention protocol - Acorda Project - on adipokines in an overweight and obese pediatric population. Pediatr Exerc Sci. (2016) 28:407–16. doi: 10.1123/pes.2015-0261
48. Nemet D, Oren S, Pantanowitz M, Eliakim A. Effects of a multidisciplinary childhood obesity treatment intervention on adipocytokines, inflammatory and growth mediators. Horm Res Paediatr. (2013) 79:325–32. doi: 10.1159/000348732
49. Kelly AS, Wetzsteon RJ, Kaiser DR, Steinberger J, Bank AJ, Dengel DR. Inflammation, insulin, and endothelial function in overweight children and adolescents: the role of exercise. J Pediatr. (2004) 145:731–6. doi: 10.1016/j.jpeds.2004.08.004
50. Kelly AS, Steinberger J, Olson TP, Dengel DR. In the absence of weight loss, exercise training does not improve adipokines or oxidative stress in overweight children. Metabolism. (2007) 56:1005–9. doi: 10.1016/j.metabol.2007.03.009
51. Nassis GP, Papantakou K, Skenderi K, Triandafillopoulou M, Kavouras SA, Yannakoulia M, et al. Aerobic exercise training improves insulin sensitivity without changes in body weight, body fat, adiponectin, and inflammatory markers in overweight and obese girls. Metabolism. (2005) 54:1472–9. doi: 10.1016/j.metabol.2005.05.013
52. Quiroga R, Nistal E, Estébanez B, Porras D, Juárez-Fernández M, Martínez-Flórez S, et al. Exercise training modulates the gut microbiota profile and impairs inflammatory signaling pathways in obese children. Exp Mol Med. (2020) 52:1048–61. doi: 10.1038/s12276-020-0459-0
53. Tenório TRS, Balagopal PB, Andersen LB, Ritti-Dias RM, Hill JO, Lofrano-Prado MC, et al. Effect of low- versus high-intensity exercise training on biomarkers of inflammation and endothelial dysfunction in adolescents with obesity: a 6-month randomized exercise intervention study. Pediatr Exerc Sci. (2018) 30:96–105. doi: 10.1123/pes.2017-0067
54. Dias I, Farinatti P, De Souza MG, Manhanini DP, Balthazar E, Dantas DL, et al. Effects of resistance training on obese adolescents. Med Sci Sports Exerc. (2015) 47:2636–44. doi: 10.1249/MSS.0000000000000705
55. Ben Ounis O, Elloumi M, Zouhal H, Makni E, Denguezli M, Amri M, et al. Effect of individualized exercise training combined with diet restriction on inflammatory markers and Igf-1/Igfbp-3 in obese children. Ann Nutr Metab. (2010) 56:260–6. doi: 10.1159/000275888
56. Shih KC, Janckila AJ, Kwok CF, Ho LT, Chou YC, Chao TY. Effects of exercise on insulin sensitivity, inflammatory cytokines, and serum tartrate-resistant acid phosphatase 5a in obese chinese male adolescents. Metabolism. (2010) 59:144–51. doi: 10.1016/j.metabol.2009.06.035
57. Mendelson M, Michallet AS, Monneret D, Perrin C, Estève F, Lombard PR, et al. Impact of exercise training without caloric restriction on inflammation, insulin resistance and visceral fat mass in obese adolescents. Pediatr Obes. (2015) 10:311–9. doi: 10.1111/ijpo.255
58. Vasconcellos F, Seabra A, Cunha F, Montenegro R, Penha J, Bouskela E, et al. Health markers in obese adolescents improved by a 12-week recreational soccer program: a randomised controlled trial. J Sports Sci. (2016) 34:564–75. doi: 10.1080/02640414.2015.1064150
59. Albuali WH. Evaluation of oxidant-antioxidant status in overweight and morbidly obese saudi children. World J Clin Pediatr. (2014) 3:6–13. doi: 10.5409/wjcp.v3.i1.6
60. Sfar S, Boussoffara R, Sfar MT, Kerkeni A. Antioxidant enzymes activities in obese tunisian children. Nutr J. (2013) 12:18. doi: 10.1186/1475-2891-12-18
61. Kelly AS, Jacobs DR, Sinaiko AR, Moran A, Steffen LM, Steinberger J. Relation of circulating oxidized Ldl to obesity and insulin resistance in children. Pediatr Diabetes. (2010) 11:552–5. doi: 10.1111/j.1399-5448.2009.00640.x
62. Calcaterra V, De Giuseppe R, Biino G, Mantelli M, Marchini S, Bendotti G, et al. Relation between circulating oxidized-Ldl and metabolic syndrome in children with obesity: the role of hypertriglyceridemic waist phenotype. J Pediatr Endocrinol Metab. (2017) 30:1257–63. doi: 10.1515/jpem-2017-0239
63. Codoñer-Franch P, Boix-García L, Simó-Jordá R, Del Castillo-Villaescusa C, Maset-Maldonado J, Valls-Bellés V. Is obesity associated with oxidative stress in children? Int J Pediatr Obes. (2010) 5:56–63. doi: 10.3109/17477160903055945
64. Codoñer-Franch P, Pons-Morales S, Boix-García L, Valls-Bellés V. Oxidant/antioxidant status in obese children compared to pediatric patients with Type 1 Diabetes Mellitus. Pediatr Diabetes. (2010) 11:251–7. doi: 10.1111/j.1399-5448.2009.00565.x
65. Carmona-Montesinos E, Velazquez-Perez R, Pichardo Aguirre E, Rivas-Arancibia S. Obesity, oxidative stress, and their effect on serum heme oxygenase-1 concentrations and insulin in children aged 3 to 5 years in a pediatric hospital of the Ministry of Health Cdmx. Child Obes. (2016) 12:474–81. doi: 10.1089/chi.2016.0155
66. Rupérez AI, Mesa MD, Anguita-Ruiz A, González-Gil EM, Vázquez-Cobela R, Moreno LA, et al. Antioxidants and oxidative stress in children: influence of puberty and metabolically unhealthy status. Antioxidants (Basel). (2020) 9:618. doi: 10.3390/antiox9070618
67. Lentferink YE, van Teeseling L, Knibbe CAJ, van der Vorst MMJ. Skin autofluorescence in children with and without obesity. J Pediatr Endocrinol Metab. (2019) 32:41–7. doi: 10.1515/jpem-2018-0237
68. Kelsey MM, Pyle L, Hilkin A, Severn CD, Utzschneider K, Van Pelt RE, et al. The impact of obesity on insulin sensitivity and secretion during pubertal progression: a longitudinal study. J Clin Endocrinol Metab. (2020) 105:e2061–8. doi: 10.1210/clinem/dgaa043
69. Amiel SA, Sherwin RS, Simonson DC, Lauritano AA, Tamborlane WV. Impaired insulin action in puberty. A contributing factor to poor glycemic control in adolescents with diabetes. N Engl J Med. (1986) 315:215–9. doi: 10.1056/NEJM198607243150402
70. Ahmadian M, Dabidi Roshan V, Rezvan K, Leicht AS. Impact of exercise in high-humidity on heart rate variability and salivary oxidative stress in obese and lightweight asthmatic children. J Sports Med Phys Fitness. (2020) 60:779–85. doi: 10.23736/S0022-4707.20.10312-8
71. Paltoglou G, Fatouros IG, Valsamakis G, Schoina M, Avloniti A, Chatzinikolaou A, et al. Antioxidation improves in puberty in normal weight and obese boys, in positive association with exercise-stimulated growth hormone secretion. Pediatr Res. (2015) 78:158–64. doi: 10.1038/pr.2015.85
72. Dennis BA, Ergul A, Gower BA, Allison JD, Davis CL. Oxidative stress and cardiovascular risk in overweight children in an exercise intervention program. Child Obes. (2013) 9:15–21. doi: 10.1089/chi.2011.0092
73. Roh HT, So WY. The effects of aerobic exercise training on oxidant-antioxidant balance, neurotrophic factor levels, and blood-brain barrier function in obese and non-obese men. J Sport Health Sci. (2017) 6:447–53. doi: 10.1016/j.jshs.2016.07.006
74. Bianchi VE, Ribisl PM. Reactive oxygen species response to exercise training and weight loss in sedentary overweight and obese female adults. J Cardiopulm Rehabil Prev. (2015) 35:263–7. doi: 10.1097/HCR.0000000000000114
75. La Cava A. Leptin in inflammation and autoimmunity. Cytokine. (2017) 98:51–8. doi: 10.1016/j.cyto.2016.10.011
76. Fernández-Sánchez A, Madrigal-Santillán E, Bautista M, Esquivel-Soto J, Morales-González A, Esquivel-Chirino C, et al. Inflammation, oxidative stress, and obesity. Int J Mol Sci. (2011) 12:3117–32. doi: 10.3390/ijms12053117
77. Yamagishi S, Amano S, Inagaki Y, Okamoto T, Takeuchi M, Inoue H. Pigment epithelium-derived factor inhibits leptin-induced angiogenesis by suppressing vascular endothelial growth factor gene expression through anti-oxidative properties. Microvasc Res. (2003) 65:186–90. doi: 10.1016/s0026-2862(03)00005-0
78. Korda M, Kubant R, Patton S, Malinski T. Leptin-induced endothelial dysfunction in obesity. Am J Physiol Heart Circ Physiol. (2008) 295:H1514–21. doi: 10.1152/ajpheart.00479.2008
79. Blüher S, Panagiotou G, Petroff D, Markert J, Wagner A, Klemm T, et al. Effects of a 1-year exercise and lifestyle intervention on irisin, adipokines, and inflammatory markers in obese children. Obesity (Silver Spring). (2014) 22:1701–8. doi: 10.1002/oby.20739
80. Magherini F, Fiaschi T, Marzocchini R, Mannelli M, Gamberi T, Modesti PA, et al. Oxidative stress in exercise training: the involvement of inflammation and peripheral signals. Free Radic Res. (2019) 53:1155–65. doi: 10.1080/10715762.2019.1697438
Keywords: adipokines, antioxidant systems, cytokines, children, exercise, inflammation, oxidative stress, physical activity
Citation: Estébanez B, Huang C-J, Rivera-Viloria M, González-Gallego J and Cuevas MJ (2022) Exercise Outcomes in Childhood Obesity-Related Inflammation and Oxidative Status. Front. Nutr. 9:886291. doi: 10.3389/fnut.2022.886291
Received: 28 February 2022; Accepted: 08 June 2022;
Published: 04 July 2022.
Edited by:
Julio J. Ochoa, University of Granada, SpainReviewed by:
Hermann Zbinden-Foncea, Universidad Finis Terrae, ChileCopyright © 2022 Estébanez, Huang, Rivera-Viloria, González-Gallego and Cuevas. This is an open-access article distributed under the terms of the Creative Commons Attribution License (CC BY). The use, distribution or reproduction in other forums is permitted, provided the original author(s) and the copyright owner(s) are credited and that the original publication in this journal is cited, in accordance with accepted academic practice. No use, distribution or reproduction is permitted which does not comply with these terms.
*Correspondence: Brisamar Estébanez, Yi5lc3RlYmFuZXpAdW5pbGVvbi5lcw==
†These authors share senior authorship
Disclaimer: All claims expressed in this article are solely those of the authors and do not necessarily represent those of their affiliated organizations, or those of the publisher, the editors and the reviewers. Any product that may be evaluated in this article or claim that may be made by its manufacturer is not guaranteed or endorsed by the publisher.
Research integrity at Frontiers
Learn more about the work of our research integrity team to safeguard the quality of each article we publish.