- 1Tea Research Institute, Chinese Academy of Agricultural Sciences, National Engineering Research Center for Tea Processing, Key Laboratory of Tea Biology and Resources Utilization, Hangzhou, China
- 2Department of Biological Sciences, Faculty of Science and Engineering, University of Limerick, Limerick, Ireland
- 3National Tea Quality Supervision and Inspection Center, Fujian, China
Tieguanyin oolong tea (TOT), a semi-oxidized tea originating from Anxi county in China, is categorized into jade TOT, medium-baked TOT, and deep-baked TOT, based on different baking processes. To study the effects of baking, chemical analysis, sensory evaluation, and bioactivity assessments of the three TOTs were conducted. The results indicated that the baking process promoted the formation of colored macromolecules (e.g., theabrownins), which affected the color of tea infusion. Free amino acids underwent the Maillard reaction and generated specific Maillard reaction products, such as 5-hydroxymethylfurfural and furfural, which modified the taste and aroma. Floral and fresh volatiles were remarkably reduced, while multiple new volatiles were produced, forming a typically baked aroma. The antioxidant activity and antibacterial activity were reduced after baking, which might be associated with the decrease of monomeric catechins. These results provide a scientific basis for understanding the changes caused by the baking process.
Introduction
Tieguanyin tea (TOT) is a semi-oxidized oolong tea from Anxi county, Fujian province, China. It is popular in south China and among Chinese expatriates in Southeast Asia. Jade TOT, medium-baked TOT, and deep-baked TOT are three significant types of TOTs. The jade TOT is produced by harvesting fresh tea leaves, withering, bruising, partial oxidation, fixing, shaping, and drying (1). The medium-baked TOT is produced by baking the jade TOT, and the deep-baked TOT is produced by baking the medium-baked TOT once more. The first baking is usually set at about 105°C for 3–8 h, and the second baking is set at about 115°C for 2–6 h. The three TOTs have distinguishing flavor characteristics and different target customers. The jade TOT is green, smells floral, tastes brisk and is preferred by females and youngsters (2). The deep-baked TOT is the favorite for the locals in Anxi county and is the most traditional and expensive TOT. Compared with the jade version, the deep-baked TOT color is darker, the aroma and the taste are more complex. The deep-baked TOT looks brownish, has a typical roast aroma, tastes mellow and thick with a strong sweet aftertaste. The sensory property of the medium-baked TOT falls in between the above two TOTs. Many customers feel that the flavor of medium-baked TOT is mediocre, not as distinctive as the other two TOTs. Therefore, medium-baked TOT is the least popular one among the three TOTs.
The flavor is caused by a specific combination of taste and aroma compounds. As a semi-oxidized tea, TOT not only contains flavor components originally from fresh tea leaves, but also flavor components generated during processes, especially the partial oxidation process. Plenty of them is oxidized intermediates, which may further convert to other substances under heat treatment or long-term storage (3), leading to the flavor alteration of TOT. Catechins are one of the flavor components which are remarkably changed during oolong tea processing. Catechins, featuring secondary metabolites in tea, are vital contributors to tea infusions’ bitter and astringent taste. However, they are chemically active, and part of them may undergo oxidation and polymerization to form new flavor molecules like theaflavins (TFs) and theasinensins during oolong tea processing (4). These intermediates can continue to form complex molecules under certain conditions (5). As catechins and their derivatives possess different sensory properties, the changes in these chemicals affect the sensory profile of the tea. Wang et al. found that by baking green tea, the composition and content of catechins were altered, resulting in a less astringent taste (6).
It is worth noting that many flavor compounds in tea are also bioactive compounds, suggesting that the alteration of bioactivity may occur along with the flavor alteration of tea. For example, catechins, mentioned above as major taste components, are the main bioactive components for the antioxidant and antibacterial activity of unfermented and semi-fermented tea (7). Lv et al. demonstrated that the content and composition of catechins in green teas made with different enzyme-inactivating processing technologies were varied, and the chemical composition was correlated to the sensory property and the antioxidant activity of green teas (8). Wang et al. proved that the baking process modified the sensory quality of Wuyi rock tea, as well as decreased the total phenolic content and free radical scavenging activity (9).
The baking process to produce medium-baked and deep-baked TOTs is conducted in a heated environment where the temperature exceeds over 100°C. Under this condition, not only catechins, but also other vulnerable components tend to transform into more stable products. To find out how baking dramatically converts the flavor and whether baking modifies the bioactivity, the differences among jade TOT, medium-baked TOT, and deep-baked TOT on the chemical composition, sensory quality, free radical scavenging activity, anti-advanced glycation end products (AGEs) activity, and antibacterial potential were investigated.
Materials and Methods
Tea Samples
The jade TOT (BT0), medium-baked TOT (BT1), and deep-baked TOT (BT2) samples were provided by Chanxinyuan (Fujian) Tea Industry Co., Ltd. (Fujian, China). BT0 was baked at 107°C for 210 min after 12-day storage to produce BT1 and then baked at 115°C for 150 min after 22-day storage to produce BT2.
Preparation of Infusions
Each tea sample was ground and filtered through a 60 Tyler mesh sieve. The tea powder was brewed with distilled water (3:500 w/v) at 100°C for 40 min, cooled to room temperature, and centrifuged at 8,000 g for 10 min to obtain the supernatant for the analysis of non-volatile chemical composition. Part of the supernatant was vacuum dried to prepare the tea extracts to assess antibacterial activity. The detailed parameters of vacuum drying were frozen at −30°C for 3 h and freeze-dried for 36 h (0–33 h increasing to 25°C and kept at 25°C for 3 h). The vacuum level was less than 50 Pa.
Sensory Evaluation and Instrumental Color
Based on the national standard GB/T 23776-2018 (10), the color, aroma, and taste of the three tea samples were evaluated by a professional sensory evaluation team consisting of seven qualified panelists, with ages ranging from 25 to 50 years old, three males and four females. The intensities of aroma and taste attributes were scored. Score 0–2 mean “extremely weak,” 2–4 mean “weak,” 4–6 mean “neutral,” 6–8 mean “strong,” and 8–10 mean “extremely strong.” Each evaluation was replicated three times on different days with a randomized order of samples for each test to assure reproducibility in the sensory analysis.
The color analysis of tea infusions was measured by a spectrophotometer (Konica Minolta, CM-3500d) by recording the CIE L*a*b* color space parameters.
To determine which part of the tea infusion contributed to the differential colors among samples, Vivaspin 20 ultrafiltration units (Product Nos. 28932358, 28932360, and 28932362, Cytiva, Marlborough, MA, United States) were used. Each tea infusion was filtered through membranes with a 3/10/50 kDa molecular weight cut-off, accordingly, via centrifuging at 4,000 g for 40 min at 37°C.
Determination of Non-volatile Chemical Composition
The total phenolic content, free amino acids, soluble proteins, soluble sugars, soluble polysaccharides, flavones, TFs, thearubigins (TRs), and theabrownins (TBs) were measured according to previously published methods (11). In brief, the contents of total polyphenols and free amino acids were determined based on the national standard GB/T 8313-2008 and GB/T 8314-2013, respectively (11). The content of soluble proteins was determined using a commercial protein assay kit (Bradford Protein Assay Kit, Product No. P0006, Beyotime Biotechnology, Haimen, China). The content of flavones was determined according to the following procedures. A 0.5 mL sample solution was added to 10 mL 1% aluminum trichloride, mixed, stayed at room temperature for 10 min, and read the absorbance at 420 nm. The contents of TFs, TRs, and TBs were determined by Robert’s method (12). The contents of eight catechins, gallic acid, and caffeine were determined using an HPLC method (13).
The content of soluble sugars was determined using the anthrone-sulfuric acid method. One milliliter sample solution was added to 4 mL anthrone-sulfuric acid (2 mg/mL), mixed, water-bathed at 100°C for 10 min, cooled to room temperature, and read the absorbance at 620 nm. The determination of soluble polysaccharides was the same as that of soluble sugars but with different pretreatment. The sample solution was added to 95% ethanol (1:5 v/v), stored at 4°C overnight, centrifuged at 8,500 g for 10 min to get the polysaccharide precipitation, and re-dissolve it with distilled water to prepare the solution for the anthrone-sulfuric acid assay. The contents of free amino acid components were determined using an amino acid analyzer (Hitachi 835-50, Tokyo, Japan) with a former established method (14).
The untargeted analysis was carried out using a previously established UPLC-QE-Orbitrap-MS method (11). The separation was performed on an ACQUITY UPLC HSS T3 column (1.8 μm, 2.1 mm × 100 mm, Waters, Milford, MA, United States) using a Dionex Ultimate 3000 RS system (Thermo Fisher). A 0.1% formic acid in water and acetonitrile was used as mobile phases A and B. The gradient changes of mobile phases were 0–1 min, 5% B; 1–2 min, 5–10% B; 2–6 min, 10–35% B; 6–8.5 min, 35–100% B; 8.5–9.5 min, 100% B; 9.5–10 min, 100–5% B; and 10–12 min, 5% B. The total flow rate was 0.3 mL/min. The column temperature was 40°C. The MS analysis was conducted using the QE-Orbitrap mass spectrometer (Thermo Scientific, United States) with electrospray ionization (ESI), operating in the positive and negative ionization full scan modes. Auxiliary gas and sheath gas flow rates were 10 and 45 (arbitrary units), respectively. The auxiliary gas heater temperature was 300°C. The capillary temperature was 320°C. The spray voltage was 3.1 kV and the S-lens RF level was 50 V. The normalized collision energy (NCE) was 30 eV. The resolution of the full scan and ddMS2 were 70,000 and 35,000, respectively. The full MS scan ranges were set from m/z 66.7 to 1,000. Data were acquired and processed using ThermoXcalibur 3.0 software (Thermo Scientific, United States). Tentative identification of non-volatiles was based on comparing retention time, m/z values, and MS/MS fragments with standards or data from databases (e.g., Massbank and MzCloud) when standards were unavailable. Relative quantitation was calculated by comparing the relative intensities of the parent ions among samples and presented in a heat map after converting to Z-scores of the rows.
Determination of Volatile Chemical Composition
The volatile chemical composition was investigated by the headspace solid-phase micro-extraction/gas chromatography-mass spectrometry (HS-SPME-GC-MS) (15). Before the extraction, the fiber of the SPME needle [50/30 μm divinylbenzene/carboxen/polydimethylsiloxane, StableFlex (2 cm), Product No. 57348-U, Supelco, Bellefonte, PA, United States] was kept at 250°C for 10 min to remove the remaining volatiles. A 0.5 g tea powder was added to a 50 mL glass vial and mixed with 5 mL boiling water and 30 μL ethyl caprate (internal standard). The glass vial was sealed immediately, gently vortexed, and incubated at 60°C. An SPME needle was inserted into the glass vial through the cap to absorb volatiles for 1 h. Then, the SPME needle was inserted into the injection port of GC to desorb volatiles at 250°C for 5 min.
Volatile organic compounds were determined by an Agilent 6890 gas chromatograph coupled with an Agilent HP 5973 mass selective detector (Agilent, Wilmington, DE, United States). The separation was performed on a DB-5MS capillary column (30 m × 250 μm × 0.25 μm) with the following GC conditions, which were the GC inlet temperature of 250°C, the split ratio of 15:1, the carrier gas (high purity helium) flow of 1.0 mL/min, the linear flow velocity of carrier gas of 40 cm/s. The column temperature was set as follows: 0–2 min, 40°C; 2–24.5 min, 40–85°C; 24.5–26.5 min, 85°C; 26.5–64.5 min, 85–180°C; 64.5–66.5 min, 180°C; 66.5–71.5 min, 180–230°C; and 71.5–73.5 min, 230°C.
The MS conditions were the temperature of the ion source of 230°C, the voltage of 70 eV, and the scan ranging from m/z 40 to 400. Tentative identification of volatiles was made by comparing the MS fragmentation patterns with data from the National Institute for Standards and Technology database (NIST 08, match percentage >80%). The relative abundance of each volatile was calculated by comparing the peak area of each compound to the total peak area.
Determination of in vitro Antioxidant Activity
The 2,2-diphenyl-1-picrylhydrazyl (DPPH) radical scavenging activity was assessed using the protocol described by Xu et al. (16). The 2,2′-azinobis-(3-ethylbenzothiazoline-6-sulfonic acid) (ABTS) scavenging activity was determined according to the protocol described by Re et al. (17). The hydroxyl radical scavenging activity was assessed using a commercial kit (Hydroxyl Free Radical Assay Kit, Product No. A018-1-1, Nanjing Jiancheng Bioengineering Institute, Nanjing, China), according to the manufacturer’s instructions. All analyses were conducted in triplicates.
Determination of Anti-advanced Glycation End Products Activity
The effects of TOTs on the formation of AGEs were investigated in bovine serum albumin (BSA) + glucose, BSA + methylglyoxal, and BSA + glyoxal systems, respectively, based on a previously published method with some modifications (18). In the BSA + glucose system, 200 μL of 15 mmol/L glucose, 200 μL of 30 mg/mL BSA, and 200 μL of tea infusion were mixed and incubated at 100°C for 1 h. The relative fluorescence was measured using a multi-functional microplate reader (excitation/emission = 370/440 nm) (Thermo Scientific Varioskan Flash, Waltham, MA, United States). In the BSA + methylglyoxal system, glucose was replaced by 200 μL of 1.5 mmol/L methylglyoxal. In the BSA + glyoxal system, glucose was replaced by 200 μL of 1.5 mmol/L glyoxal.
Determination of Antibacterial Activity
The minimum inhibitory concentrations (MICs) against Salmonella typhi [CMCC (B) 50071], Shigella flexneri [CMCC (B) 51572], β-hemolytic Streptococcus [CMCC (B) 32210], Staphylococcus aureus [CMCC (B) 26003], and Escherichia coli [CMCC (B) 44102] were determined using the micro-dilution method (19).
Each strain was plated on an agar plate and incubated at 37°C for 24 h. A single colony was used to inoculate 10 mL of sterile broth and incubated at 37°C for 24 h. Then the suspension was diluted to 2 × 105 CFU/mL. To investigate the effects of the three tea samples on the growth of each strain, BT0, BT1, and BT2 extracts were dissolved in sterile broth to prepare a 2 mg/mL solution and serially diluted to reach the final concentrations of 0.5, 1, and 2 mg/mL, respectively. A 100 μL bacterial suspension was mixed with 100 μL tea extract solution and then added to a 96-well plate. The positive control was 100 μL bacterial suspension mixed with 100 μL sterile broth. The 96-well plate was incubated overnight at 37°C and then observed. MICs are defined as the lowest concentration of an antimicrobial agent that would inhibit the visible growth of a microorganism after overnight incubation.
Statistical Analysis
The data are presented as the mean ± standard error of the mean (SEM). All experiments were carried out in triplicate and repeated in three independent sets of experiments. The results were analyzed with SPSS version 18.0 for Windows (SPSS, Chicago, IL, United States), using a one-way analysis of variance and a post hoc test (two-sided Dunnett’s test) to evaluate differences among groups. P-values < 0.05 were considered to be statistically significant.
Results and Discussion
Effects of Baking on the Non-volatiles and the Color of Infusion
The color of the infusion is an aspect to assess the sensory quality of tea. Sensory evaluation (Figure 1A) indicated that the baking process significantly darkened the color of tea infusion, turning it from honey yellow, golden yellow, to orange. Instrumental analysis of color (Figure 1B) showed that the a* value turned from −2.31 to −0.96, the b* value increased from 15.4 to 23.0, and the L* value decreased from 95.6 to 92.6 after twice baking. It indicated that the baking process brought more red and yellow tones but less luminosity to TOT infusion, which was in accordance with the results of the visual observation. Separating the tea infusion using centrifugal filters with 3, 10, and 50 kDa molecular weight cut-off (MWCO), accordingly, it was found that the color differences among the three tea infusions were mainly attributed to constituents that could not pass through the 10 kDa MWCO filter (Figure 1C). The orange/golden yellow pigments in BT2 had higher molecular weights than in BT1, suggesting that the baking process promoted the formation of colored macromolecules.
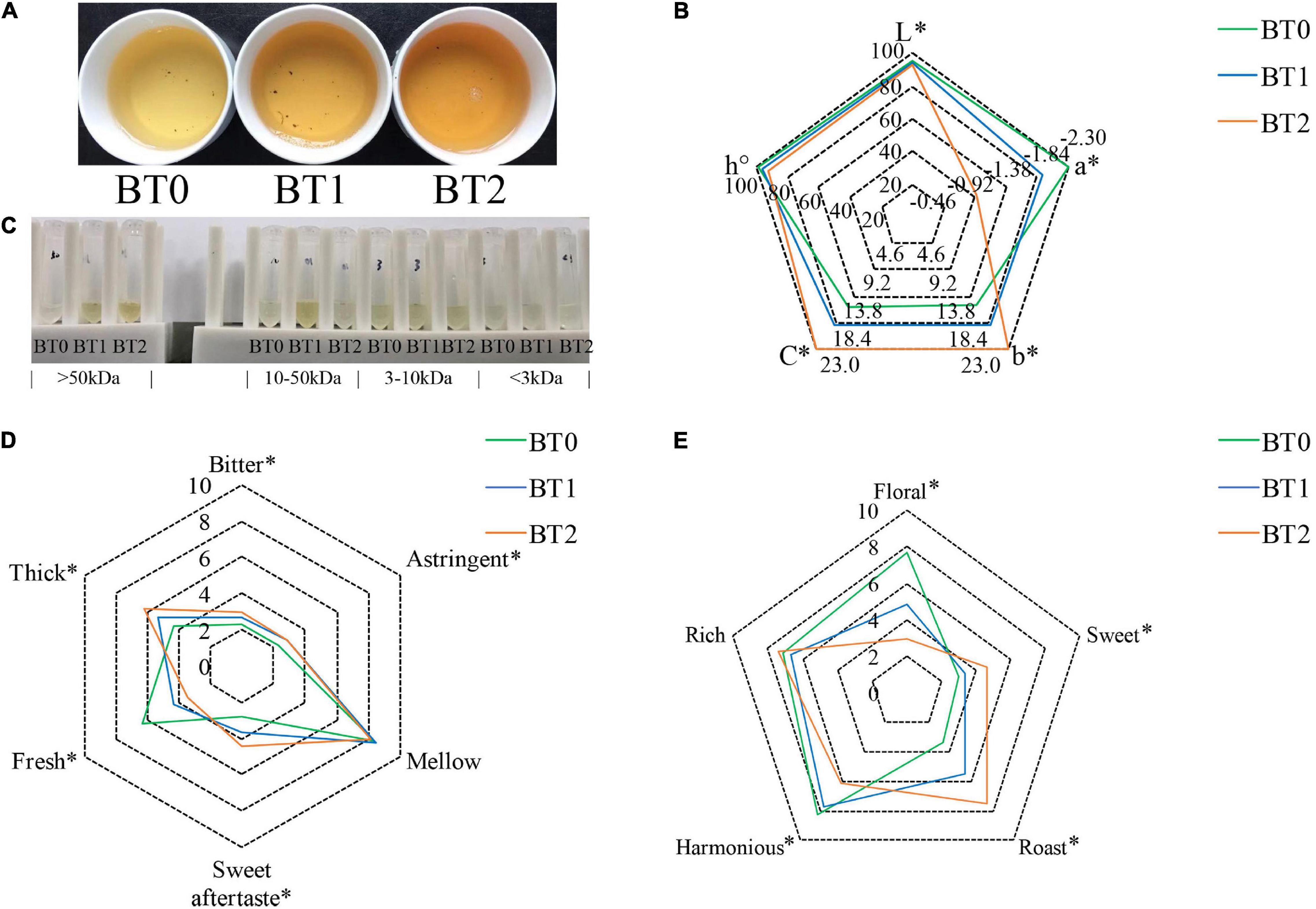
Figure 1. Sensory properties of jade (BT0), medium baked (BT1), and deep baked (BT2) Tieguanyin oolong teas. (A) Photo of tea infusions; (B) the color analysis determined by a spectrophotometer; (C) photo of tea infusion constituents separated using centrifugal filters with 3, 10, and 50 kDa molecular weight cut-off, accordingly; (D) taste characteristics and scores; and (E) aroma characteristics and scores. *P < 0.05 indicates significant difference.
Previous studies revealed that flavonol glycosides and tea pigments (e.g., TFs, TRs, and TBs) contributed to the color of tea infusion (20). As flavonol glycosides are small molecules, it is speculated that TRs and TBs may cause the differences on the color of TOT infusions. TRs and TBs are heterogeneous water-soluble polymers of catechins. TRs, whose average molecular weight range from ∼700 to 40,000 Da, usually present red color. TBs, characterized by their high molecular weight and complex structure, usually present brown color and are negatively related to the brightness of tea infusions. BT2 contained higher TRs and TBs than BT0 and BT1, and BT1 contained higher TBs than BT0 (Table 1). Deducing from the fact that the content of TRs in BT1 was insignificantly different from that in BT0, but the redness was enhanced in the BT1 infusion (Figure 1B), there might be something else attributing to the redness of TOT infusions besides TRs. The content of TBs was increased while the brightness of tea infusion was decreased as the baking degree increased, demonstrating that TBs reduced the brightness of TOT infusions. The cause of the increase of TRs and TBs might be the accelerated oxidization and polymerization of catechins induced by the heat and aerobic environment during the baking process (21). A 7.4 and 17.4% loss of total monomeric catechins were detected in BT1 and BT2 (Table 2), respectively, supporting the hypothesis.
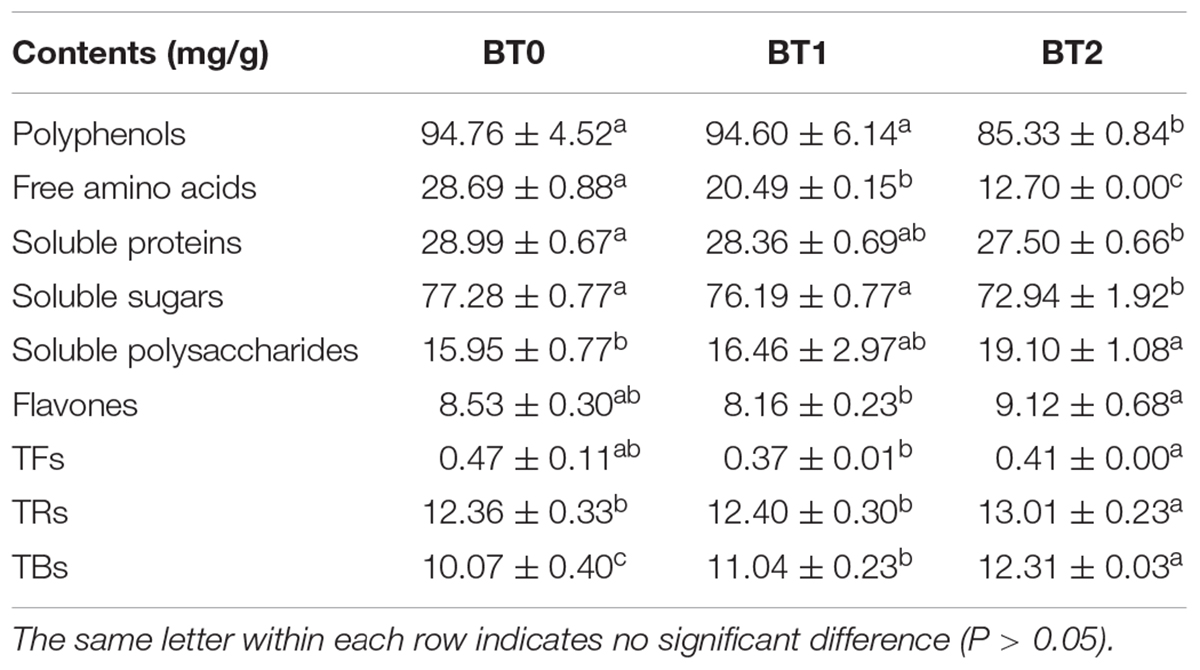
Table 1. The chemical compositions of jade (BT0), medium-baked (BT1), and deep-baked (BT2) Tieguanyin oolong teas.
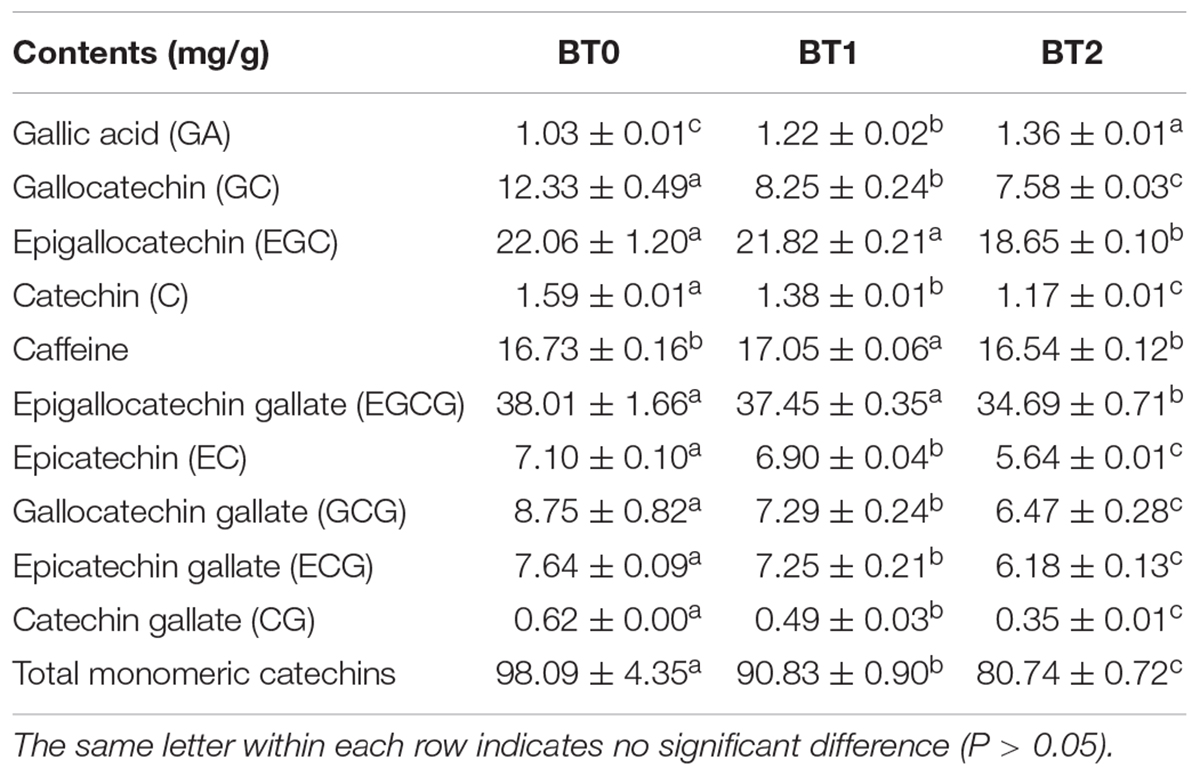
Table 2. The contents of monomeric catechins, gallic acid, and caffeine in jade (BT0), medium-baked (BT1), and deep-baked (BT2) Tieguanyin oolong teas.
Effects of Baking on the Non-volatiles and Taste
Taste is the most crucial aspect of oolong tea’s sensory quality, based on GB/T 23776-2018. Sensory evaluation indicated that the baking process remarkably increased the thickness, sweet aftertaste, and bitterness, while reducing TOT infusion’s umami taste (Figure 1D).
Soluble polysaccharides were candidate contributors to the thickness of tea infusions. A 20% increase in the content of soluble polysaccharides was detected in BT2 (Table 1). It was reported that some polysaccharides increased the kokumi sensation (22), a taste impression combined of thickness, mouthfulness, and continuity, as they influenced the viscosity of fluids (23). Tea that tasted smooth and thick, such as ripe Pu’er tea and aged white tea, usually had abundant soluble polysaccharides. Heat treatment could promote the degradation of insoluble polysaccharides, thereby increasing the content of soluble polysaccharides in tea infusions. It was possible that the increase of soluble polysaccharides after baking also contributed to the thick taste. TRs might have an impact on the thickness of TOT infusions as well, because TRs were previously found to contribute to the mouth feel (thickness) (24).
Gallic acid, a degradation product of catechins, was associated with the sweet aftertaste of TOT infusions. Gallic acid was previously reported to improve the sweet aftertaste of tannase-treated autumn green tea (25). In this study, a 18 and 32% increase of gallic acid were detected in BT1 and BT2, respectively (Table 2), which was consistent with the gradual enhancement of sweet aftertaste after baking. Along with the increase of gallic acid, was the decrease of catechins. Among the eight catechins analyzed by HPLC, six were decreased after the first baking process, and eight were reduced after the second baking. Several dimeric catechins (e.g., theasinensins and procyanidins) were reduced after baking (Figure 2). In details, about 30% of TFs, 10% of theasinensins, and 30% of procyanidins were lost after twice baking. In a previous study, high-temperature processing (roasting) during tea production decreased monomeric catechins and increased gallic acid (26). Our results supported that the degradation of catechins were universal during the baking process of tea. Catechins and their derivatives are essential to the taste of oolong tea by enhancing bitterness and astringency (14). Monomeric catechins usually taste astringent and bitter, while dimeric catechins taste more astringent and less bitter than monomeric catechins (11). The bitterness and astringency were not reduced though catechins were decreased after baking (Figure 1D). On the contrary, both sensory attributes were enhanced, implying that other bitter and astringent compounds might be generated during the baking process.
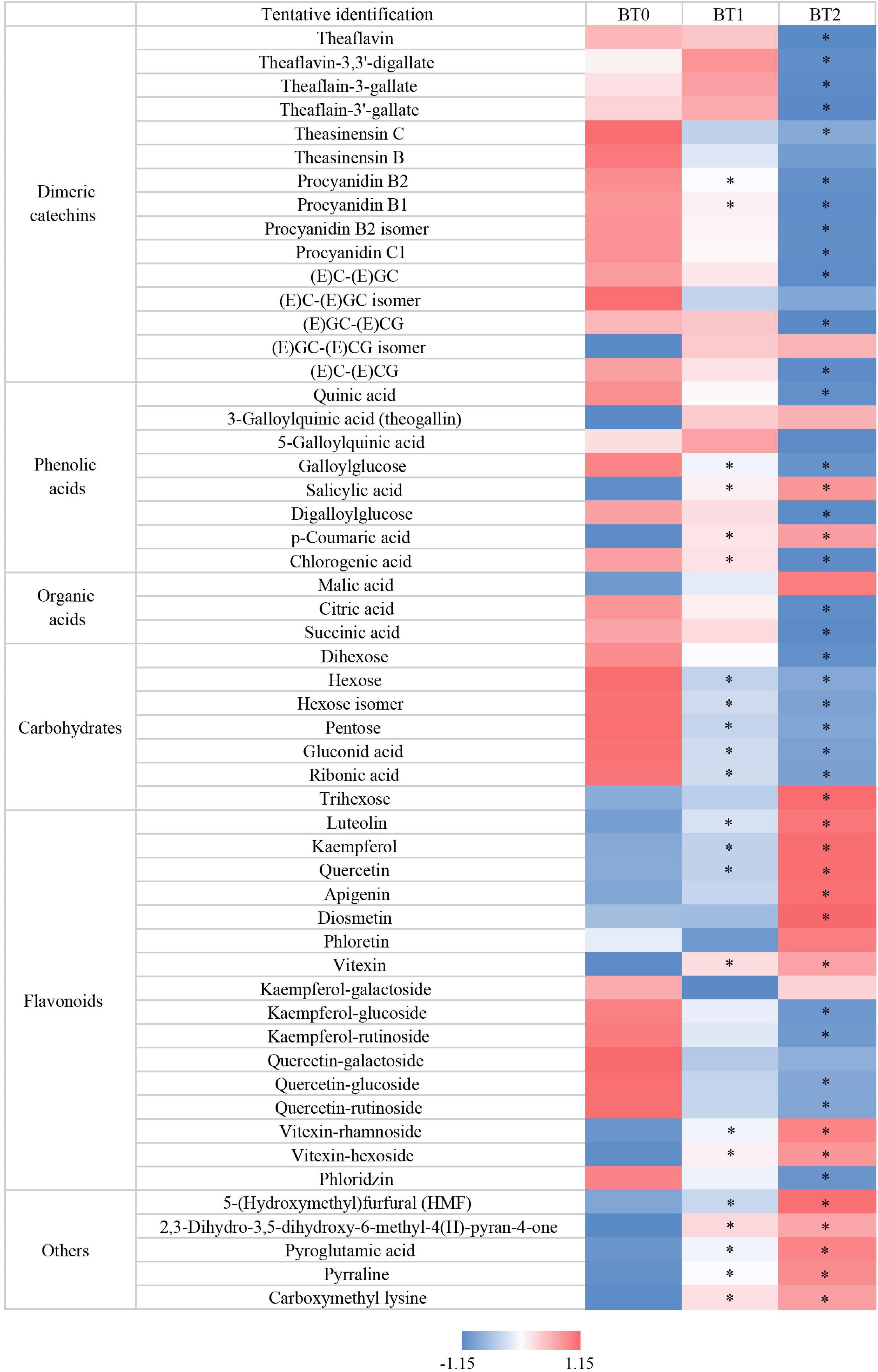
Figure 2. Heatmap of Z-score normalized relative abundances of non-volatiles identified by UPLC-QE-Orbitrap-MS. *P < 0.05 indicates significant difference.
It was assumed that the Maillard reaction was responsible to the bitterness of baked TOT infusions. The Maillard reaction is a chemical reaction between reducing sugars and amino acids upon the baking process, which produces a series of flavor compounds and modifies the sensory properties (e.g., bitterness) of baked foods (27, 28). Previous research demonstrated that the Maillard reaction impacted the quality of green tea and oolong tea (10, 29). In this study, 55% of total free amino acids was consumed after twice baking (Table 1). The contents of dihexose, hexoses, and pentose significantly decreased in baking. At the same time, the levels of 5-hydroxymethylfurfural (5-HMF) and 2,3-dihydro-3,5-dihydroxy-6-methyl-4(H)-pyran-4-one (DDMP), two typical intermediates of Maillard reaction with a bitter taste (30), in BT2 was 7.7-fold and 2.6-fold of that in BT0. These data proved the presence of the Maillard reaction during the baking process of TOT and its role in the chemical and taste changes of baked TOTs.
It was aware that the occurrence of the Maillard reaction was accompanied by the consumption of free amino acids. A total of 17 free amino acids were detected, including 14 proteinogenic amino acids and 3 non-protein amino acids (i.e., theanine, β-aminoisobutyric acid, and γ-aminobutyric acid) (Table 3). Theanine and glutamic acid, major umami compounds in tea (31), were the two most abundant free amino acids. Together, the two accounted for about 60% of total free amino acids in TOT infusions. However, merely 24.3% of theanine and 32.7% of glutamic acid remained after baking twice, which led to the reduced umami taste of baked TOT infusions (Figure 1D). Our previous study suggested that the free amino acid content was positively correlated to the taste quality of TOTs (1). The baking-induced decrease of free amino acids might hamper the taste of TOTs, but benefit the formation of typical roast aroma via the Maillard reaction.
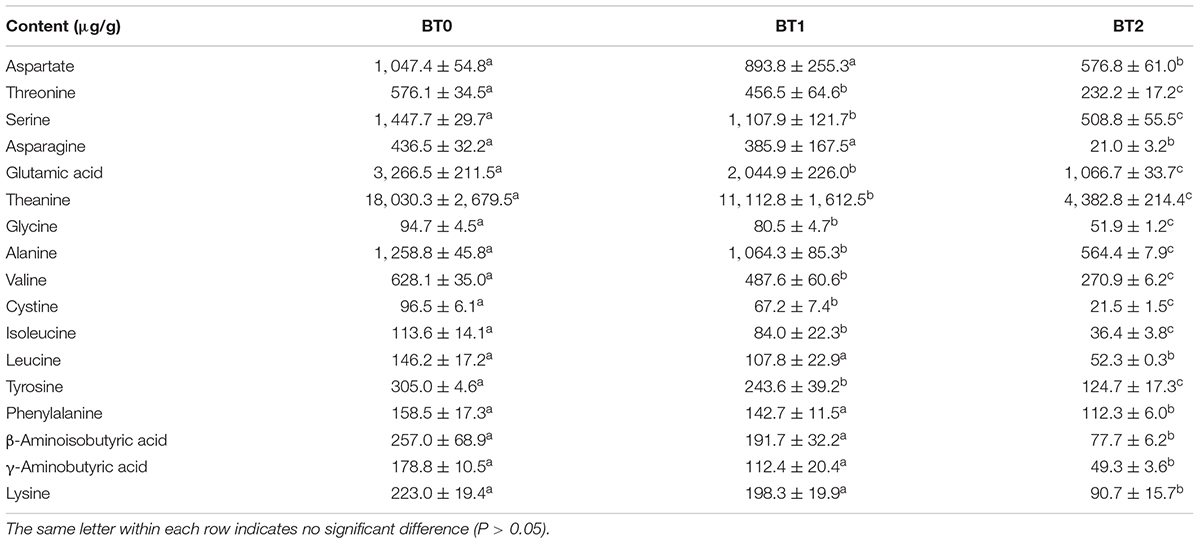
Table 3. The contents of free amino acid components in jade (BT0), medium-baked (BT1), and deep-baked (BT2) Tieguanyin oolong teas.
Effects of Baking on the Volatiles and Aroma
Aroma is the second important aspect of oolong tea’s sensory quality, based on GB/T 23776-2018. Sensory evaluation indicated that the baking process transformed the aroma from floral to sweet and roast (Figure 1E).
The changes of volatiles after baking were the basis of the changes in the aroma. Table 4 indicated that the baking process significantly increased the number of volatiles. A total of 74, 85, and 102 volatiles were identified in BT0, BT1, and BT2, respectively. Among them, 61 volatiles were detected in all three samples. BT2 contained more unique volatiles than the other two. It indicated that the baking process promoted the formation of new volatiles, many of which belonged to heterocycles.
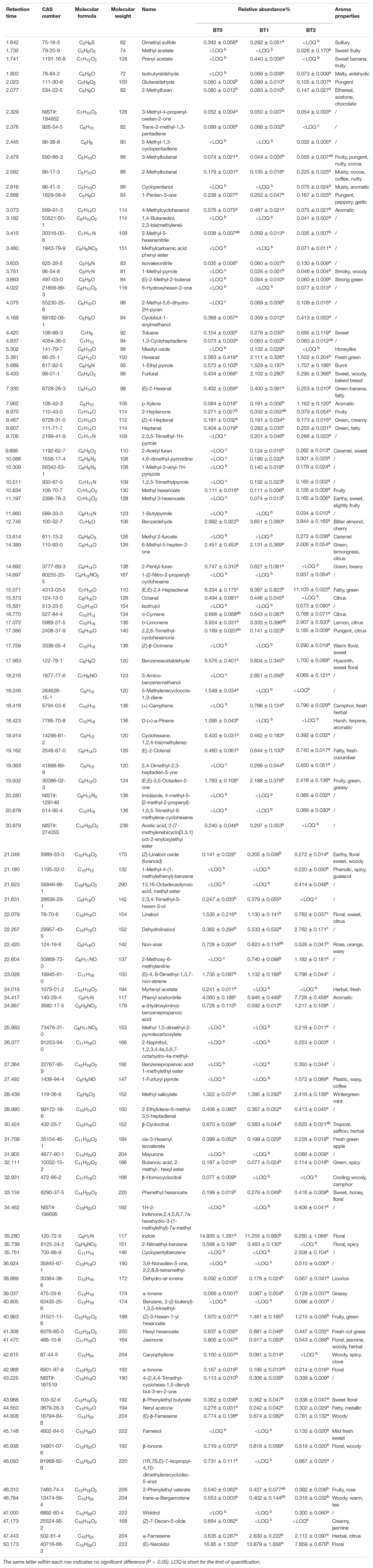
Table 4. Volatile compounds in jade (BT0), medium-baked (BT1), and deep-baked (BT2) Tieguanyin oolong teas determined by GC-MS.
Floral volatiles were the main volatiles detected in BT0. Although the number of floral volatiles did not vary considerably among the three teas, their relative abundances did. The relative abundances of floral volatiles accounted for 46.6% of total volatiles in BT0. (E)-Nerolidol, indole, and benzeneacetaldehyde were the top three abundant floral volatiles in BT0, making up 37.4% of total volatiles. The baking process decreased the contents of floral volatiles. The loss of floral volatiles occurred during the first and second baking stages, but was more severe during the second stage. The relative abundance of floral volatiles dropped to 19.7% in BT2. Previous study indicated that high temperature hampered the floral volatiles in TOT, i.e., β-ionone, jasmine, and nerolidol (32). Our results were partially in accordance with it. The differences might be caused by the differences in the raw material and the conditions of heat treatment. In addition, multiple green volatiles [e.g., cis-3-hexenyl isovalerate, heptanal, hexyl hexanoate, (Z)-3-hexen-1-yl hexanoate, hexanal, and 2-pentyl-furan], citrus volatiles (e.g., octanal and D-limonene), and herbal volatiles (e.g., α-farnesene and myrtenyl acetate) were reduced or disappeared after baking. The sum of the relative abundances of green/citrus/herbal volatiles decreased from 16.4 to 8.7%, leading to a less refreshing aroma in BT2.
Meanwhile, the types and relative abundances of sweet, caramel, and roast volatiles were significantly increased. Six, 12, and 14 sweet, caramel, and roast volatiles were identified in BT0, BT1, and BT2, accounting for 1.6, 5.3, and 11.0% of total volatiles, respectively. The relative abundances of toluene, phenethyl hexanoate, furfural, and 1-ethyl pyrrole gradually increased with baking. The relative abundances of these volatiles in BT2 were at least twice as much as that in BT0, respectively. Notably, the relative abundance of furfural, a volatile with a sweet and baked bready aroma and a typical intermediate of the Maillard reaction, was 11-fold higher in BT2 than that in BT0. Methyl 3-hexanoate, 2-acetyl furan, and 1-methyl-pyrrole, which existed in BT1 and BT2 but not in BT0, were enriched in BT2. Methyl acetate, farnesol, methyl 2-furoate, and 1-furfuryl pyrrole were merely detected in BT2. Earlier study revealed that 1-ethyl pyrrole was positively correlated with the grade of deep-baked TOT, while methyl acetate was initially decreased but then increased with the declining grade of deep-baked TOT (33). In this study, the two volatiles were accumulated in BT2, implying that the baking process was important for the formation of the aroma property of deep-baked TOT. It was noticed that many volatiles generated or accumulated during baking were pyrroles, furans, and their derivatives, which were Maillard reaction products, indicating that the baking process mainly reshaped the aroma via the Maillard reaction.
In addition to volatiles with known aroma characteristics, 27 volatiles detected in BT2 but not in BT0 were with unknown aroma characteristics. The contents of these compounds accounted for 14.4% of total volatiles. Most of them were complex structures, containing at least one cyclic ring. Little information on the aroma properties of these compounds could be found. It is necessary to use gas chromatography-olfactometry to investigate the aroma properties of these compounds and assess whether they contribute to the aroma of tea in further studies.
Effects of Baking on the Chemical Composition and Bioactivity
Chemical analysis revealed that the baking process dramatically altered the compositions and contents of catechins and their derivatives, flavones, free amino acids, and soluble sugars, many of which were bioactive compounds (Tables 1, 2, 3 and Figure 2). Hence, it was wondered whether the baking process also altered the bioactivity of TOTs. Antioxidant property is a featuring bioactivity of oolong tea. Free radicals are highly oxidizing and may damage macromolecules in the living system via inducing oxidative stress. Scavenging free radicals is an important strategy for antioxidants to exert their functions. Thus, free radical scavenging assays are often applied to evaluate the antioxidant activity. Recently, anti-AGEs formation assays are gaining attention as another way to evaluate antioxidant activity. Reactive oxygen species and free radicals participate in the formation of AGEs, and AGEs can induce oxidative stress via binding to their cell surface receptor (34). Many antioxidants possess inhibitory effects on the formation of AGEs (35). In this study, the above two assays were conducted to assess the antioxidant activity of three TOTs (Figures 3A–F). Antibacterial property is another featuring bioactivity of oolong tea. The MICs against five bacteria were used to determine the antibacterial activity (Figure 3G).
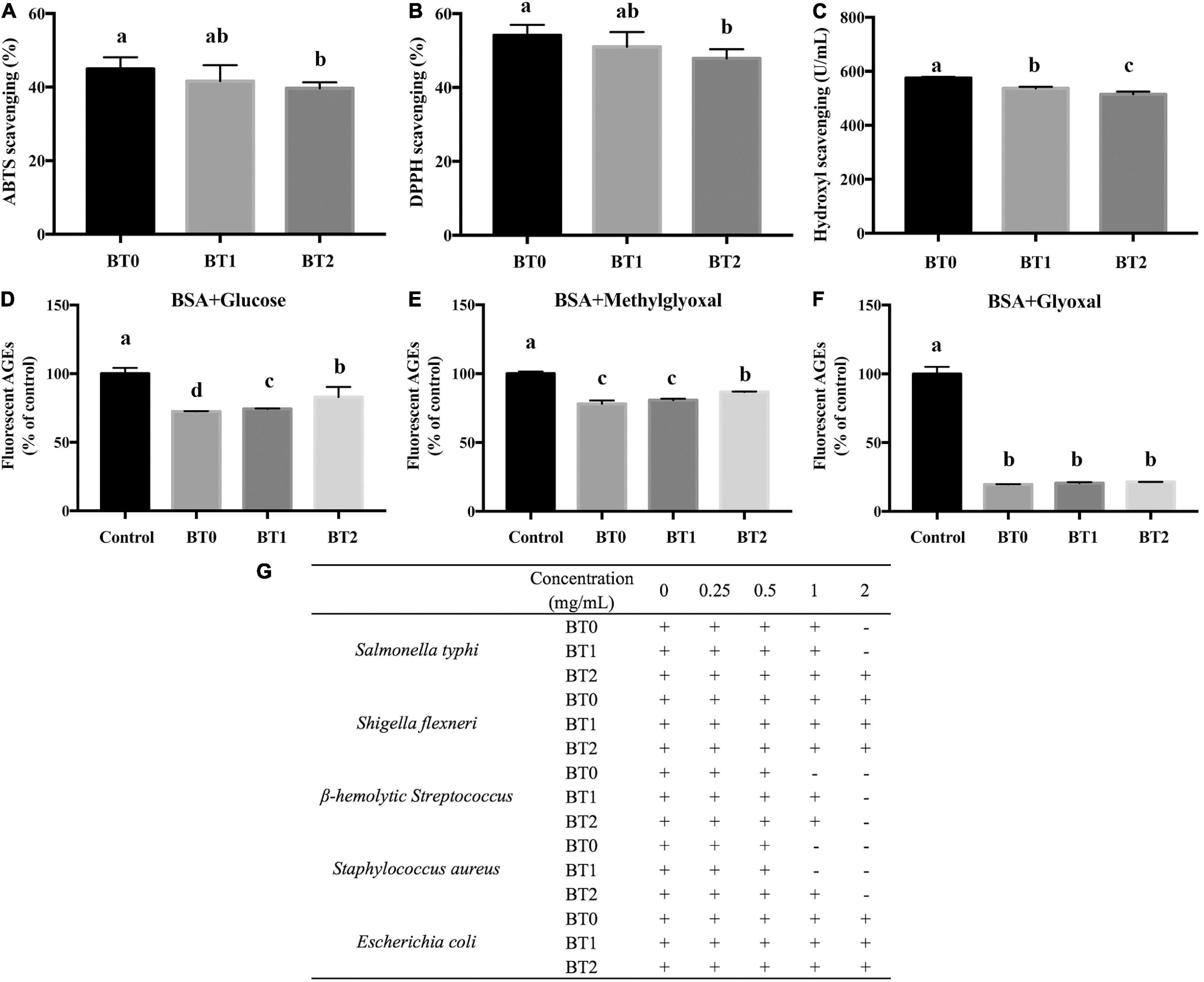
Figure 3. Bioactivities of jade (BT0), medium baked (BT1), and deep baked (BT2) Tieguanyin oolong teas. (A) ABTS scavenging activity; (B) DPPH scavenging activity; (C) hydroxyl scavenging activity; relative fluorescent AGEs formation in the bovine serum albumin (BSA) + glucose system (D), BSA + methylglyoxal system (E), and BSA + glyoxal system (F); (G) minimum inhibitory concentrations of the three tea extracts against five bacteria. “+” means visible growth of the bacteria, while “–” means no visible bacterial growth. The same letter within each column indicates no significant difference (P > 0.05).
The effects of baking on the free radical scavenging activity are disadvantageous. The ABTS, DPPH, and hydroxyl radical scavenging activities of BT2 were significantly reduced compared with BT0 (Figures 3A–C). Earlier researches suggested that the ethyl acetate fraction of TOT, which had higher contents of phenolics, flavonoids, procyanidins, sugars, and catechin monomers, exhibited stronger antioxidant capacity than the n-butanol fraction and water fraction of TOT (36). Su et al. demonstrated that monomeric catechins played critical roles in anti-oxidation (37). It was reported that the trans-catechins possessed higher free radical scavenging activity than the corresponding cis-epicatechins, and the gallated catechins possessed higher antioxidant activity than the corresponding non-gallated catechins (38). Lee et al. tested the ABTS and DPPH scavenging activities of seven monomeric catechins. Compared with non-gallated catechins, gallated catechins did exhibit stronger ABTS and DPPH scavenging activities (39). Su et al. demonstrated that two major catechins, i.e., epigallocatechin gallate (EGCG) and epigallocatechin (EGC), contributed significantly to oolong tea’s DPPH and superoxide radical scavenging activities. Guo et al. investigated the hydroxyl radical scavenging activity of four epi-form catechins. The results indicated that their ability to scavenge hydroxyl radicals decreased in the order of epicatechin gallate (ECG) > epicatechin (EC) > EGCG > EGC (40). In our research, the changing trends of catechins contents, particularly the changing trends of epi-form catechins contents, were consistent with the changing trends of free radical scavenging activity during baking. It suggested that the decrease of catechins was an important cause of the baking-mediated decreased free radical scavenging activity of TOT.
The effects of baking on inhibiting the formation of AGEs are inconsistent in different reaction systems. Dietary AGEs are formed in foods during the thermal process, and they are a main source of AGEs in the body (41). The formation of AGEs begins between a carbonyl group of reducing sugar (e.g., glucose) and a free amino group, which generates an unstable Schiff base. It spontaneously cyclizes and undergoes the Amadori rearrangement, producing intermediates with highly reactive carbonyl groups, such as glyoxal and methylglyoxal (42). These products further react with free amino groups to form stable AGEs. Many AGEs are highly oxidant and pro-inflammatory, usually regarded as harmful to health (43). In this study, the baking process weakened the activity of TOTs in blocking the formation of AGEs in the BSA + glucose reaction system and the BSA + methylglyoxal reaction system, while hardly affecting that in the BSA + glyoxal reaction system (Figures 3D–3F). Earlier research indicated that catechins, including catechin (C), EC, ECG, EGC, and EGCG, could inhibit the formation of AGEs via scavenging reactive oxygen species (44). Sang et al. found that EGCG efficiently trapped reactive dicarbonyl compounds (methylglyoxal and glyoxal) to prevent the formation of AGEs (45). Besides catechins, several flavonols and flavones also had anti-AGEs activity. Quercetin, kaempferol, apigenin, and luteolin were proved to inhibit the formation of AGEs by trapping reactive dicarbonyls (46). A further study revealed that the hydroxyl groups at the 3′-, 4′-, 5-, and 7-positions affected the inhibitory activity (47). Although the contents of catechins decreased, the contents of several flavonols and flavones were increased after baking (Figure 2). It might explain why the anti-AGEs activity was hardly reduced in the BSA + glyoxal reaction system.
The effects of baking on antibacterial activity are disadvantageous (Figure 3G). The MIC is the lowest concentration of a substance which prevents the visible growth of a bacterium or bacteria. A substance with low MICs is considered an effective antimicrobial agent. Compared with BT2, the MICs of BT0 and BT1 against S. typhi and S. aureus were lower, respectively. The MIC of BT0 against β-hemolytic Streptococcus was lower than the MIC of BT1. The results indicated that the baking process attenuated the inhibition of TOTs against certain bacteria. Chou et al. proved that oolong tea had inhibitory effects on several micro-organisms (48). Monomeric polyphenols, which had a high affinity to proteins, were regarded as active antibacterial compounds (49). Some volatiles also displayed antibacterial activity, and there were synergistic interactions among specific volatiles. α-Pinene was capable of suppressing S. aureus (50). Indole, the second most abundant volatile in BT0, inhibited Streptococcus mutans. The combination of β-caryophyllene or δ-cadinene enhanced the inhibitory effects of indole against bacteria (51). The contents of monomeric catechins and the volatiles mentioned above (e.g., indole, α-pinene, and caryophyllene) were decreased after baking. The change was in accordance with the reduced antibacterial activity of BT1 and BT2. It implied that the loss of monomeric catechins and some volatiles during the baking process might be responsible for the baking-mediated decreased antibacterial activity of TOT.
Conclusion
This study assessed and compared the chemical composition, sensory quality, and bioactivity of three TOTs with different baking degrees. According to the results, the baking process dramatically modified the chemical composition of TOTs, particularly in catechins, amino acids, and volatiles. The baking-induced changes of chemical components led to the darkened color of tea infusion, roast aroma, and enhanced sweet aftertaste. Since many flavor components are also bioactive components, the changes of chemical components also affected bioactivity. Adverse impacts of the baking process on the free radical scavenging activity and antibacterial activity were observed, probably due to the decrease of monomeric catechins. The results obtained herein fill the gap on the effects of the baking process of oolong tea and indicate that the baking process might be a double-edged sword, which enriches the flavor while attenuating the bioactivity. Future studies can focus on exploring the superior bioactivity of baked TOTs and developing processing technologies of TOTs that retain the typical sensory quality of baked TOTs but with less loss of bioactive components.
Data Availability Statement
The raw data supporting the conclusions of this article will be made available by the authors, without undue reservation.
Author Contributions
YG, J-FY, and Y-QX conceived and designed the experiments. YG, Q-QC, FW, J-XC, X-BZ, and Y-QX performed the experiments. YG, Q-QC, Y-HC, and J-QW analyzed the data. YG and DG wrote the manuscript. All authors have read and agreed to the final version of the manuscript.
Funding
This research was supported by the National Natural Science Foundation of China (31872709), the Central Level, Scientific Research Project (Y2021CG06), the China Agriculture Research System of MOF and MARA (CARS-19), and the Innovation Project for the Chinese Academy of Agricultural Sciences.
Conflict of Interest
The authors declare that the research was conducted in the absence of any commercial or financial relationships that could be construed as a potential conflict of interest.
Publisher’s Note
All claims expressed in this article are solely those of the authors and do not necessarily represent those of their affiliated organizations, or those of the publisher, the editors and the reviewers. Any product that may be evaluated in this article, or claim that may be made by its manufacturer, is not guaranteed or endorsed by the publisher.
References
1. Xu YQ, Liu PP, Shi J, Gao Y, Wang QS, Yin JF. Quality development and main chemical components of Tieguanyin oolong teas processed from different parts of fresh shoots. Food Chem. (2018) 249:176–83. doi: 10.1016/j.foodchem.2018.01.019
3. Wen W. The Research on Quality Chemistry and Anti-Inflammatory of Anxi Tieguanyin Tea. Changsha: Hunan Agricultural University (2018).
4. Zhang J, Cui H, Xue J, Wang W, Wang W, Le T, et al. Adsorption equilibrium and thermodynamics of tea theasinensins on HP20-A high-efficiency macroporous adsorption resin. Foods. (2021) 10:2971. doi: 10.3390/foods10122971
5. Hua J, Wang H, Yuan H, Yin P, Wang J, Guo G, et al. New insights into the effect of fermentation temperature and duration on catechins conversion and formation of tea pigments and theasinensins in black tea. J Sci Food Agric. (2021) 102:2750–60. doi: 10.1002/jsfa.11616
6. Wang JQ, Fu YQ, Chen JX, Wang F, Feng ZH, Yin JF, et al. Effects of baking treatment on the sensory quality and physicochemical properties of green tea with different processing methods. Food Chem. (2022) 380:132217. doi: 10.1016/j.foodchem.2022.132217
7. Narukawa M, Noga C, Ueno Y, Sato T, Misaka T, Watanabe T. Evaluation of the bitterness of green tea catechins by a cell-based assay with the human bitter taste receptor hTAS2R39. Biochem Biophys Res Commun. (2011) 405:620–5. doi: 10.1016/j.bbrc.2011.01.079
8. Lv HP, Liang MZ, Zhang Y, WAng LB, Lin Z. Major chemical components and antioxidant activity in tea infusion of tea products obtained from the special tea germplasm ‘Zijuan’ using different processing technologies. Food Sci. (2016) 37:122–7.
9. Wang L, Guan XQ, Yu NH, Lin ZQ, Zhang YL. Effect of different baking degree on antioxidant activity and quality of Wuyi rock tea. Food Sci Technol. (2020) 45:68–74.
10. Zhu YM, Dong JJ, Jin J, Liu JH, Zheng XQ, Lu JL, et al. Roasting process shaping the chemical profile of roasted green tea and the association with aroma features. Food Chem. (2021) 353:129428. doi: 10.1016/j.foodchem.2021.129428
11. Gao Y, Wang JQ, Fu YQ, Yin JF, Shi J, Xu YQ. Chemical composition, sensory properties and bioactivities of Castanopsis lamontii buds and mature leaves. Food Chem. (2020) 316:126370. doi: 10.1016/j.foodchem.2020.126370
12. Roberts EAH, Smith RF. Spectrophotometric measurements of theaflavins and thearubigins in black tea liquors in assessments of quality in teas. Analyst. (1961) 86:94–8. doi: 10.1039/an9618600094
13. Xu YQ, Hu XF, Tang P, Jiang YW, Yuan HB, Du QZ, et al. The major factors influencing the formation of sediments in reconstituted green tea infusion. Food Chem. (2015) 172:831–5. doi: 10.1016/j.foodchem.2014.09.143
14. Liu PP, Yin JF, Chen GS, Wang F, Xu YQ. Flavor characteristics and chemical compositions of oolong tea processed using different semi-fermentation times. J Food Sci Technol. (2018) 55:1185–95. doi: 10.1007/s13197-018-3034-0
15. Xu YQ, Chen JX, Du QZ, Yin JF. Improving the quality of fermented black tea juice with oolong tea infusion. J Food Sci Technol. (2017) 54:3908–16. doi: 10.1007/s13197-017-2849-4
16. Xu YQ, Gao Y, Granato D. Effects of epigallocatechin gallate, epigallocatechin and epicatechin gallate on the chemical and cell-based antioxidant activity, sensory properties, and cytotoxicity of a catechin-free model beverage. Food Chem. (2021) 339:128060. doi: 10.1016/j.foodchem.2020.128060
17. Re R, Pellegrini N, Proteggente A, Pannala A, Yang M, Rice-Evans C. Antioxidant activity applying an improved ABTS radical cation decolorization assay. Free Radic Biol Med. (1999) 26:1231–7. doi: 10.1016/s0891-5849(98)00315-3
18. Li XM, Deng RH, Kong YH, Xia QQ, Lv LS. Inhibitory effect of rutin on the formation of advanced glycation end products (AGEs) from bovine serum albumin. Food Sci. (2014) 35:85–9.
19. Eloff JN. A sensitive and quick microplate method to determine the minimal inhibitory concentration of plant extracts for bacteria. Planta Med. (1998) 64:711–3. doi: 10.1055/s-2006-957563
20. Zhang L. Effects of Baking Technics on the Quality of Wuyi Rock-Essence Tea. Chongqing: Southwest Universiy (2018).
21. Lin YP, Liu SB, Huang YB, Zhan SQ, Zhang JM, Chen RB. Effect of baking degrees on the quality of Wuyi Rock Tea “Dahongpao”. Food Res Dev. (2020) 41:49–54.
22. Lin ST, Hao GX, Long M, Lai F, Li QQ, Xiong YM, et al. Oyster (Ostrea plicatula Gmelin) polysaccharides intervention ameliorates cyclophosphamide-Induced genotoxicity and hepatotoxicity in mice via the Nrf2-ARE pathway. Biomed Pharmacother. (2017) 95:1067–71. doi: 10.1016/j.biopha.2017.08.058
23. Passos CP, Costa RM, Ferreira SS, Lopes GR, Cruz MT, Coimbra MA. Role of coffee caffeine and chlorogenic acids adsorption to polysaccharides with impact on brew immunomodulation effects. Foods. (2021) 10:378. doi: 10.3390/foods10020378
24. Zhu K, Ouyang J, Huang J, Liu Z. Research progress of black tea thearubigins: a review. Crit Rev Food Sci Nutr. (2021) 61:1556–66. doi: 10.1080/10408398.2020.1762161
25. Cao QQ, Zou C, Zhang YH, Du QZ, Yin JF, Shi J, et al. Improving the taste of autumn green tea with tannase. Food Chem. (2019) 277:432–7. doi: 10.1016/j.foodchem.2018.10.146
26. Jiang H, Zhang MT, Wang DX, Yu F, Zhang N, Song CK, et al. Analytical strategy coupled to chemometrics to differentiate Camellia sinensis tea types based on phenolic composition, alkaloids, and amino acids. J Food Sci. (2020) 85:3253–63. doi: 10.1111/1750-3841.15390
27. Lindenmeier M, Faist V, Hofmann T. Structural and functional characterization of pronyl-lysine, a novel protein modification in bread crust melanoidins showing in vitro antioxidative and phase I/II enzyme modulating activity. J Agr Food Chem. (2002) 50:6997–7006. doi: 10.1021/jf020618n
28. Jiang D, Peterson DG. Identification of bitter compounds in whole wheat bread. Food Chem. (2013) 141:1345–53. doi: 10.1016/j.foodchem.2013.03.021
29. Xiang LW, Chen WT. Effects of Maillard reaction on the quality of oolong tea. Chem Eng Equipment. (2012) 7:13–7.
30. Li H. Study on the Formation Pathway and Regulation Mechanism of Main Bitter Compounds in Maillard Reaction. Guangzhou: South China University of Technology (2019).
31. Guo X, Ho CT, Schwab W, Wan X. Effect of the roasting degree on flavor quality of large-leaf yellow tea. Food Chem. (2021) 347:129016. doi: 10.1016/j.foodchem.2021.129016
32. Cao QQ, Fu YQ, Wang JQ, Zhang L, Wang F, Yin JF, et al. Sensory and chemical characteristics of Tieguanyin oolong tea after roasting. Food Chem X. (2021) 12:100178. doi: 10.1016/j.fochx.2021.100178
33. Wang W, Jin S, Guo Y. Exploration of a method of distinguishing different nongxiang tieguanyin tea grades based on aroma determined by GC-MS combined with chemometrics. Molecules. (2019) 24:1707. doi: 10.3390/molecules24091707
34. Dzib-Guerra WD, Escalante-Erosa F, Garcia-Sosa K, Derbre S, Blanchard P, Richomme P, et al. Anti-advanced glycation end-product and free radical scavenging activity of plants from the Yucatecan Flora. Pharmacognosy Res. (2016) 8:276–80. doi: 10.4103/0974-8490.188883
35. Starowicz M, Zielinski H. Inhibition of advanced glycation end-product formation by high antioxidant-leveled spices commonly used in European Cuisine. Antioxidants (Basel). (2019) 8:100. doi: 10.3390/antiox8040100
36. Wang Y, Kong D, Gao Y, Ying L, Huang Q, Xu P. Chemical characterization and bioactivity of phenolics from Tieguanyin oolong tea. J Food Biochem. (2019) 43:e12894. doi: 10.1111/jfbc.12894
37. Su XG, Duan J, Jiang YM, Duan XW, Chen F. Polyphenolic profile and antioxidant activities of oolong tea infusion under various steeping conditions. Int J Mol Sci. (2007) 8:1196–205. doi: 10.3390/i8121196
38. Guo Q, Zhao B, Shen S, Hou J, Hu J, Xin W. ESR study on the structure-antioxidant activity relationship of tea catechins and their epimers. Biochim Biophys Acta. (1999) 1427:13–23. doi: 10.1016/s0304-4165(98)00168-8
39. Lee LS, Kim SH, Kim YB, Kim YC. Quantitative analysis of major constituents in green tea with different plucking periods and their antioxidant activity. Molecules. (2014) 19:9173–86. doi: 10.3390/molecules19079173
40. Guo Q, Zhao B, Li M, Shen S, Xin W. Studies on protective mechanisms of four components of green tea polyphenols against lipid peroxidation in synaptosomes. Biochim Biophys Acta. (1996) 1304:210–22. doi: 10.1016/s0005-2760(96)00122-1
41. Nowotny K, Schroter D, Schreiner M, Grune T. Dietary advanced glycation end products and their relevance for human health. Ageing Res Rev. (2018) 47:55–66. doi: 10.1016/j.arr.2018.06.005
42. Popova EA, Mironova RS, Odjakova MK. Non-enzymatic glycosylation and deglycating enzymes. Biotechnol Biotechnol Equipment. (2010) 24:1928–35. doi: 10.2478/v10133-010-0066-7
43. Uribarri J, Woodruff S, Goodman S, Cai W, Chen X, Pyzik R, et al. Advanced glycation end products in foods and a practical guide to their reduction in the diet. J Am Diet Assoc. (2010) 110:911–6. doi: 10.1016/j.jada.2010.03.018
44. Wu CH, Yen GC. Inhibitory effect of naturally occurring flavonoids on the formation of advanced glycation endproducts. J Agric Food Chem. (2005) 53:3167–73. doi: 10.1021/jf048550u
45. Sang S, Shao X, Bai N, Lo CY, Yang CS, Ho CT. Tea polyphenol (-)-epigallocatechin-3-gallate: a new trapping agent of reactive dicarbonyl species. Chem Res Toxicol. (2007) 20:1862–70. doi: 10.1021/tx700190s
46. Ronsisvalle S, Panarello F, Longhitano G, Siciliano EA, Montenegro L, Panico A. Natural flavones and flavonols: relationships among antioxidant activity, glycation, and metalloproteinase inhibition. Cosmetics. (2020) 7:71. doi: 10.3390/cosmetics7030071
47. Matsuda H, Wang T, Managi H, Yoshikawa M. Structural requirements of flavonoids for inhibition of protein glycation and radical scavenging activities. Bioorg Med Chem. (2003) 11:5317–23. doi: 10.1016/j.bmc.2003.09.045
48. Chou CC, Lin LL, Chung KT. Antimicrobial activity of tea as affected by the degree of fermentation and manufacturing season. Int J Food Microbiol. (1999) 48:125–30. doi: 10.1016/s0168-1605(99)00034-3
49. Sasaki H, Matsumoto M, Tanaka T, Maeda M, Nakai M, Hamada S, et al. Antibacterial activity of polyphenol components in oolong tea extract against Streptococcus mutans. Caries Res. (2004) 38:2–8. doi: 10.1159/000073913
50. Raman A, Weir U, Bloomfield SF. Antimicrobial effects of tea-tree oil and its major components on Staphylococcus aureus, Staph. epidermidis and Propionibacterium acnes. Lett Appl Microbiol. (1995) 21:242–5. doi: 10.1111/j.1472-765x.1995.tb01051.x
Keywords: oolong tea, baking process, free radical scavenging activity, anti-advanced glycation end products, antibacterial, sensory properties, catechins
Citation: Gao Y, Cao Q-Q, Chen Y-H, Granato D, Wang J-Q, Yin J-F, Zhang X-B, Wang F, Chen J-X and Xu Y-Q (2022) Effects of the Baking Process on the Chemical Composition, Sensory Quality, and Bioactivity of Tieguanyin Oolong Tea. Front. Nutr. 9:881865. doi: 10.3389/fnut.2022.881865
Received: 23 February 2022; Accepted: 06 April 2022;
Published: 16 May 2022.
Edited by:
Jinkai Zheng, Institute of Food Science and Technology (CAAS), ChinaReviewed by:
Sean F. O’Keefe, Virginia Tech, United StatesLijun Sun, Northwest A&F University, China
Copyright © 2022 Gao, Cao, Chen, Granato, Wang, Yin, Zhang, Wang, Chen and Xu. This is an open-access article distributed under the terms of the Creative Commons Attribution License (CC BY). The use, distribution or reproduction in other forums is permitted, provided the original author(s) and the copyright owner(s) are credited and that the original publication in this journal is cited, in accordance with accepted academic practice. No use, distribution or reproduction is permitted which does not comply with these terms.
*Correspondence: Yong-Quan Xu, yqx33@126.com; Jun-Feng Yin, yinjf@tricaas.com