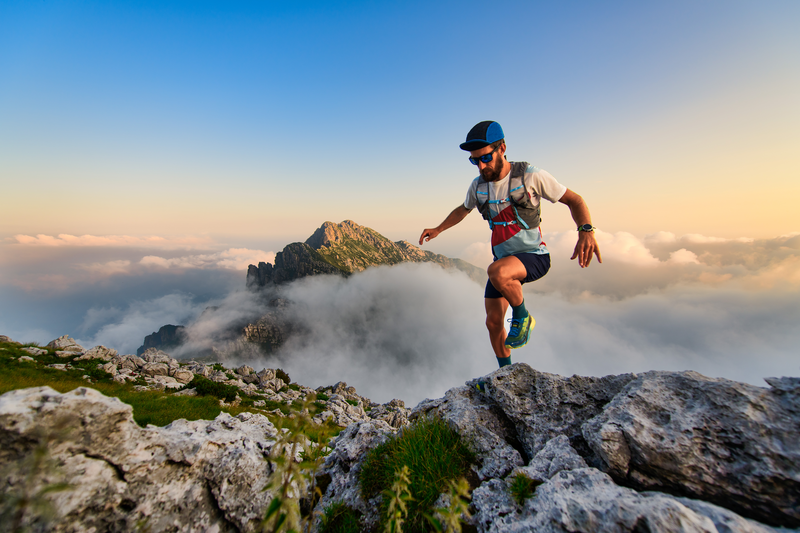
95% of researchers rate our articles as excellent or good
Learn more about the work of our research integrity team to safeguard the quality of each article we publish.
Find out more
ORIGINAL RESEARCH article
Front. Nutr. , 18 May 2022
Sec. Food Chemistry
Volume 9 - 2022 | https://doi.org/10.3389/fnut.2022.881464
This article is part of the Research Topic Rising Stars in Food Chemistry View all 28 articles
Laminaria japonica is rich in alginate (Alg) and galactofucan (GF) which have both been reported to regulate gut microbiota composition. To reveal the effect of L. japonica on human gut microbiota, the fecal microbiota of 12 volunteers before and after 14-day L. japonica intake was sequenced and compared, and the capabilities of the gut microbiota to utilize Alg and GF were also investigated. The 16S rRNA gene sequencing results demonstrated that Firmicutes/Bacteroidetes ratio could be balanced by L. japonica supplementation. The ability of gut microbiota to utilize Alg was significantly enhanced by L. japonica supplementation. Furthermore, the multiple linear regression analysis suggested that bacteria from Bacteroidaceae and Ruminococcaceae were positively correlated with Alg utilization while those from Erysipelotrichaceae, Bacteroidaceae, and Prevotellaceae participated in GF degradation. Moreover, the production of acetic acid and the total short-chain fatty acids (SCFAs) in fermentation were consistent with the consumption of Alg or GF, and propionic acid content was positively correlated with Alg consumption. In addition, the percentage of monosaccharides in the consumed GF after the fermentation suggested that gut microbiota from individuals could consume GF with different monosaccharide preferences. These findings shed a light on the impacts of dietary L. japonica on human health.
Laminaria japonica, one of the most widely consumed commercial edible seaweeds in the world (1, 2), has a high production in China, Japan, and Korea (3–5). It is rich in dietary fiber, such as alginate (Alg) and fucoidan, and their contents generally vary from 30 to 40% and 5 to 10% of the seaweed dry weight, respectively (6). Alg is an acidic polysaccharide composed of 1,4-linked β-D-mannuronate (M) and 1,4-linked α-L-guluronate (G) (7). Fucoidan is a class of sulfated polysaccharides in brown algae containing fucose and varying greatly in their structural characteristics (8). Galactofucan (GF) found in Laminaria japonica is a type of fucoidan, consisting of fucose and galactose residues (9).
Both Alg and fucoidan are indigestible fibers that could modulate the composition and/or activity of microorganisms in the large intestine, thus conferring a beneficial physiological effect on the host (10). For instance, Alg could prevent high-fat diet-induced metabolic syndrome and increase the abundance of Bacteroides (11), and relieve hyperglycemia in type 2 diabetic mice by enriching Bacteroides, Lactobacillus, Akkermansia, Alloprevotella, Weissella, and Enterorhabdus, and decreasing Turicibacter and Helicobacter in the intestinal tracts of mice (12). Furthermore, fucoidan shows a therapeutic effect on metabolic syndrome and the improvement of gut dysbiosis in association with an increase of Akkermansia population in the gut microbes of high-fat diet mice (13). More recently, researchers have suggested that fucoidan could improve insulin resistance in diet-induced obese mice by remodeling gut microbiota (14). It could be concluded that both Alg and fucoidan could modulate gut microbiota in animal models, suggesting the benefits of L. japonica consumption on the ecosystem of the intestinal tract. Therefore, it is necessary to evaluate the effect of L. japonica in human dietary intervention studies. In addition, more and more pieces of evidence demonstrate that the utilization of dietary fibers by gut microbiota could produce short-chain fatty acids (SCFAs) and other metabolites to benefit the host. However, bacteria responsible for the breakdown and utilization of Alg and fucoidan are largely unknown.
The present study aimed to evaluate the effects of dietary L. japonica supplementation on the gut microbiota of normal humans and the capabilities of the gut microbiota to utilize Alg and GF, and reveal bacteria involved in the utilization of Alg and GF. The microbial communities from twelve volunteers before and after the 14-day supplementation with L. japonica were compared. Furthermore, the fermentation of Alg and GF from L. japonica and SCFAs production by these fecal microbiota communities from different individuals were assessed.
Instant shredded kelp was provided by Sichuan Jixiangju Food Co., Ltd. (Sichuan, China). Dried L. japonica was obtained from Fujian Yida Food Co., Ltd. (Fujian, China). Alg was purchased from Qingdao Bright Moon Seaweed Group Co., Ltd. Its molecular weight was determined as 1,311 kDa according to the reported method (15). GF was prepared from L. japonica according to our previous method and its monosaccharide composition was fucose (Fuc): xylose (Xyl): galactose (Gal): glucose (Glc): galacturonic acid (GalA): manmose (Man) = 9.8: 1.0: 16.4: 1.8: 6.0: 12.7 as reported by us previously (15), and the average molecular weight of GF was determined as 627 kDa. Peptone and yeast extract were purchased from Oxoid (Thermo Co., Ltd, Massachusetts, USA). Monosaccharide standards, such as rhamnose (Rha), Xyl, Gal, GalA, Glc, glucuronic acid (GlcA), Man, arabinose (Ara), and Fuc were purchased from Sigma Chemical Co. (St. Louis, MO, USA). The ingredients of bacterial media were purchased from Qingdao Hope Bio-Technology Co., Ltd. All other chemical reagents with analytical grades used in this study were purchased from Sinopharm Chemical Reagent Co., Ltd. (Shanghai, China).
In this study, 12 subjects were chosen from six male and six female volunteer students (aged 20–22 years old) of the School of Food Science and Technology, Dalian Polytechnic University. The volunteers consumed L. japonica 50 g/day for 14 days. The participants had no gastrointestinal disease or antibiotics treatment for at least 3 months. The study was supported by the Ethics Committee of Dalian Polytechnic University and all volunteers provided a well-informed and signed consent before entering the trial. The general characteristics of the 12 individuals during intervention are shown in Supplementary Table S1. The body mass index (BMI) of volunteers was 18.5–23.9 which is in the healthy weight range. The volunteers were asked not to take any medication that could affect the gastrointestinal tract during the trial.
The fecal samples obtained from the 12 normal volunteers before (Be) and after (Af) 14-day dietary intake of L. japonica were labeled as Be.1, Be.2, Be.3 to Be.12 and Af.1, Af.2, Af.3 to Af.12, respectively. The PowerFecal™ DNA Isolation Kit (MO BIO, USA) was applied to isolate the genomic DNA from the feces, and the analysis of all the DNA samples was conducted by Novogene Bioinformatics Technology Co., Ltd. (Beijing, China). For each sample, the V3-V4 region of 16S rRNA was chosen for amplification using the forward primer 515F and the reverse primer 806R and sequenced by Ion S5TMXL platform. The analysis was based on sequenced reads and operational taxonomic units (OTUs).
The preparation of growth medium was performed according to the method reported previously (16). Briefly, 1 L of the basal nutrient medium was composed of 2 g peptone, 2 g yeast extract, 0.02 g hemin, 0.5 g L -cysteine, 0.5 g bile salts, 0.1 g NaCl, 0.04 g K2HPO4, 0.04 g KH2PO4, 0.01 g MgSO4·7H2O, 0.01 g CaCl2·6H2O, 2 g NaHCO3, 1 mg resazurin, 2 ml Tween-80, and 10 μl vitamin K. The whole substrates were dissolved in distilled water and autoclaved at 121°C for 15 min.
A total of 24 fecal samples as mentioned in Section High-Throughput Sequencing Analysis were used in vitro fermentation. Then, 1 g of feces from the donor was diluted in a 9-ml modified physiological saline solution containing 9 g/L of NaCl and blended using a magnetic stirrer to yield 10% (w/v) fecal slurry. Then, the fecal slurry was centrifuged at 4°C (500 rpm, 5 min). After that, 1.0 ml of the fecal suspension was added to 9.0 ml of the basal nutrient medium containing 3 g/L of Alg or 3 g/L of GF. All samples were incubated at 37°C in an anaerobic incubator (Electrotek, UK), and 1.5 ml fermentation samples were collected at 0 and 48 h and then stored at −80°C for further analysis. Each experiment was independently replicated 3 times.
The results were expressed as the proportion of residual Alg or GF (%) based on the contents of carbohydrates before and after fermentation. Residual Alg content was measured by using the meta-hydroxydiphenyl colorimetric method with Alg as standard (17) and residual GF content was determined using the phenol-sulfuric acid method with Gal as standard (18), respectively.
The contents of SCFAs, such as acetic acid, propionic acid, and butyric acid were measured according to the procedure reported with some modifications (19). Briefly, 500 μl of the fermentation broth was transferred into a 2-ml centrifuge tube, and the solution was acidified by adding 10 μl of sulfuric acid (50%, v/v). Subsequently, 900 μl of diethyl ether and 100 μl of 500 μg/ml 2-ethylbutyric acid (internal standard) solution were added to the sample. The solution was then mixed through vortex oscillation 2 times for 3 min. After being centrifuged (12,000 rpm, 15 min), the supernatant was transferred into a tube with 250 mg of Na2SO4. The mixed solution was centrifuged at 4°C and the supernatant was loaded by the GC (2010-plus, Shimadzu) equipped with a flame ionization detector (FID), and a Rtx®-Wax column (30 m × 0.25 mm × 0.25 μm) was used. For GC analysis, the initial column temperature was 100°C with a 1-min hold, then programmed to 180°C at a temperature ramp of 5°C/min and maintained for 4 min; the injector temperature was 250°C; the flow rates of hydrogen, air, and nitrogen make up gas were 40, 300, and 3 ml/min, respectively.
The monosaccharide composition analysis of GF after 48 h fermentation was performed as the previous method with a little modification (20). In brief, after dialyzed and dried, the sample was dissolved in 2 ml of 2 M trifluoroacetic acid (TFA) at 121°C for 2 h. Then, excess TFA was removed with deionized water by reduced pressure distillation. The residue was re-dissolved in 500 μl of Na2CO3 (0.5 mol/L) solution and maintained at 30°C for 45 min. After the reduction using sodium borohydride, acetic acid was added to the solution. Sodium in the obtained solution was removed by passing through a cation exchange column. Excess borohydride was decomposed by co-distillation with methanol. The resultant solution was heated at 85°C for 2 h to convert the aldose salt to lactone. The reagent of pyridine and n-propylamine (1 ml each) were added to dissolve the residue and then kept at 55°C for 30 min to react. Then, the solution was dried in vacuum. After adding 1 ml pyridine and 1 ml acetic anhydride, the solution was kept at room temperature overnight. The resulting solution was extracted 3 times using dichloromethane. Neutral sugars and uronic acids were subsequently determined by GC using the method reported previously (21).
A multivariate linear regression model was used to investigate the associations between Alg or GF utilization and bacterial OTUs. The relative abundances of bacterial OTUs with an abundance larger than 5% in at least one sample were included in the regression model as confounding factors. Data were analyzed using SPSS version 20.0 software (SPSS Inc., Chicago, IL, USA). All the data were presented as means ± standard derivation (SD). Comparison between two groups/samples was performed using Student's t-test. The comparison of multiple samples was conducted by one-way analysis of variance (ANOVA) procedure followed by the Tukey's Honestly Significant Difference (HSD) test. Correlations were analyzed using Pearson's correlation. The values of p < 0.05 were regarded as statistically significant. *p < 0.05, **p < 0.01, and ***p < 0.001.
The effects of a 14-day supplementation with L. japonica on gut microbiota structures were evaluated by comparing the gut microbes of the volunteers before (Be group) and after (Af group) the dietary intervention. The gut microbiota compositions in most of the individuals varied obviously after supplementation with L. japonica both at phylum (Figure 1A) and genus (Figure 1B) levels. Then, the changes of Bacteroidetes and Firmicutes were observed at the individual level. The change directions of Bacteroidetes (Figure 2A) and Firmicutes (Figure 2B) in these 12 individuals were not consistent. However, interestingly, those with the original Bacteroidetes abundance higher than 0.6 (Nos. 7, 10, and 12), grouped as H-B, all down-adjusted the Bacteroidetes after the intake of L. japonica while those with Bacteroidetes lower than 0.6 (Nos. 1, 2, 4, 5, 6, 8, 9, and 11), grouped as L-B, demonstrated an opposite trend, except No. 3. Further significance analysis excluding No. 3 indicated that L. japonica intake could significantly reduce Bacteroidetes in the H-B group but increase Bacteroidetes in the L-B group. Moreover, the original Firmicutes abundance of more than 0.36 showed a reduced population after the intervention of L. japonica. Further analysis demonstrated that the modulation of Firmicutes in the H-B group and the L-B group was opposite to Bacteroidetes. Similar patterns were observed in Firmicures/Bacteroidetes (divided based on the line at 0.6) (Figure 2C). Previous studies have established the correlation of an increase in the Firmicutes-to-Bacteroidetes ratio to the higher energy harvesting from diet and the pathology of obesity (22–24). Dietary fiber was effective to downregulate the Firmicutes-to-Bacteroidetes ratio in obese patients and thus attenuated obesity (25–28). However, in the model of dextran sulfate sodium (DSS)-induced inflammatory mice, supplementation with polysaccharides could upregulate the Firmicutes-to-Bacteroidetes ratio and alleviate intestinal inflammation (29). In the present study, L. japonica supplementation balanced the population of Firmicutes to Bacteriodetes ratio in individuals, which may be associated with the intake of dietary fiber in L. japonica. The different changed directions of the microbiota abundance between the H-B group and the L-B group may be explained by the variation among inter-individuals.
Figure 1. The compositions of fecal gut microbiota before (Be) and after (Af) the 14-day dietary Laminaria japonica intake at the phylum level (A) and genus level (B).
Figure 2. The phylum Bacteroidetes abundance (A), phylum Firmicutes abundance (B), and Firmicute/Bacteroidetes ratio (C) in individuals Be and Af the 14-day dietary L. japonica intake and the comparations of microbiota abundance in the H-B group and the L-B group. H-B group, original Bacteroidetes abundance higher than 0.6; L-B group, original Bacteroidetes abundance lower than 0.6. *p < 0.05 and **p < 0.01.
The utilization of Alg and GF by the fecal microbiota of 12 volunteers before and after the 14-day L. japonica intake was evaluated by determining the remaining sugar contents after 48 h of fermentation. As shown in Figure 3A, the residual content of Alg differed greatly among the samples in both the Be group and the Af group. However, for one-half of the participants, the Alg content after fermentation in the Af group was significantly lower than that in the Be group. Moreover, Figure 3B showed that there was a significant difference between the Be group and the Af group in residual Alg content, indicating that L. japonica intake could enhance the capability of fecal microbiota to metabolize Alg. Unlike Alg consumption, the GF content after fermentation in the Af group did not show more utilization by fecal microbiota than that in the Be group (Figure 3C) and no significant change in the GF utilization between the Be group and the Af group was observed (Figure 3D). It has been well documented that some dietary fibers could regulate the gut microbiota community when these microbiota consuming fibers. As the major component of L. japonica, Alg (30–40% of dry weight) (30) was supposed to show a far more important effect on gut microbiota than GF. Moreover, the degradation rates of Alg and GF by gut microbiota differed because of their different chemical structures, and obviously, Alg was favored more by gut microbiota than GF. Of note, L. japonica intake enhanced the Alg fermentation ability of fecal bacteria in Nos. 3, 4, 8, 9, 11, and 12. Of the individuals with the greatest consumption of Alg, Nos. 4, 8, 9, and 11 showed increased Bacteroidetes but No. 3 and 12 showed a reduction in Bacteroidetes (Figure 2A). Altogether, these results implied the discrepancy of Alg or GF utilization efficacy among individuals after L. japonica intake. This finding was consistent with the previous report that the fecal microbiota from different individuals differed in their ability to utilize polysaccharides (31). Previous studies have demonstrated that chondroitin sulfate could be readily metabolized to varying extents by diverse microbial consortiums (32). Recent studies revealed that the regulation results of gut microbiota by resistant starch varied greatly due to inter-individual differences in gut microbiota populations (33). These studies suggest that the beneficial outcomes of polysaccharides are influenced by the inter-individual variation of microbiome structure.
Figure 3. The comparisons of alginate (Alg) and galactofucan (GF) utilization by fecal microbiota collected Be and Af L. japonica intake in individuals (A,C) and in groups (B,D). *p < 0.05, **p < 0.01, ***p < 0.001; ns, no significant difference.
The multiple linear regression analysis is a regression model with more than one independent variable which is applied to determine the correlations among variables having cause-effect relationships and to make predictions for the topic by using the relationships (31, 34, 35). To identify the determinants of bacterial OTUs to Alg or GF utilization, the association of fecal bacterial OTUs with the consumption of the Alg or GF was analyzed by multiple linear regression analysis. The results are shown in Table 1, and the multiple linear regression equation was obtained as follows: the consumption of Alg = 0.356–4.450OTU18 + 3.841OTU200-3.222OTU75 + 1.064OTU28 + 0.288OTU7, with a correlation coefficient of 0.908, and the consumption of GF = 0.177 + 7.744OTU66 + 5.714OTU1060 + 3.774OTU589-2.565OTU18, with a correlation coefficient of 0.798. Totally, 5 OTUs and 4 OTUs were the significant variables attributed to Alg and GF utilization, respectively. The multiple linear regression analysis showed that the consumption of Alg was positively related (B > 0) with Bacteroide smassiliensis (OTU200), Ruminococcus bromii (OTU28), and a genus of Ruminococcaceae (OTU7), and negatively related (B < 0) with OTU18 (Roseburia inulinivorans) and a genus of Muribaculaceae (OTU75). The consumption of GF had a positive correlation with a genus of Erysipelotrichaceae (OTU66), Bacteroides dorei (OTU1060), and a genus of Prevotellaceae (OTU589), and a negative correlation with R. inulinivorans (OTU18). Bacteroides massiliensis and a genus of Erysipelotrichaceae (OTU66) were the most powerful bacteria to metabolize Alg and GF, respectively. In addition, a multi-collinearity analysis demonstrated no interaction among these variables (tolerance > 0.1). Bacteroidaceae and Prevotellaceae, which are reported as the major gut microbiota families participating in the degradation of numerous polysaccharides (36–38), also play important roles in the utilization of Alg and GF. Moreover, it has been reported that most of the Alg-active bacteria are from Bacteroides (39), and Bacteroides could also be enriched by fucoidan (13), suggesting their possible participation in GF degradation in the gut. In addition, the members of the Ruminococcaceae family could also take part in the Alg utilization, as indicated by its potential power to degrade indigestible polysaccharides (40, 41). Erysipelotrichaceae, which is involved in the fermentation of plant polysaccharides (42), also demonstrated its attribution in GF consumption. Nevertheless, although Roseburia and Muribaculaceae are associated with carbohydrate metabolism (43, 44), they had an unexpected negative association with the consumption of Alg and/or GF. Notably, the bacteria members contributing to the degradation of Alg and GF were discrepant, indicating that different carbohydrate-active enzymes (CAZymes) were involved in the metabolism of the 2 polysaccharides. The inhomogeneity of the regulation on these microbiotas by L. japonica supplementation suggested the bacteria members participating in the polysaccharide degradation varied in individuals.
Table 1. A multiple linear regression analysis between bacterial OTUs (%) and alginate (Alg) or galactofucan (GF) consumption (%).
In the light of the fact that gut microbiota variation will lead to the distinct metabolic functions for Alg and GF, SCFAs, the most important microbial metabolites of dietary polysaccharides, produced by human microbiota from different individuals were compared and the correlation analysis between SCFAs and the Alg or GF consumption was further conducted. Fecal samples with the highest (Af.3) or lowest (Be.9) Alg utilization capabilities and those with more (Af.4 and Af.9) or less (Be.1 and Be.6) Bacteroidetes were chosen for the assay of SCFAs production. As shown in Figures 4A,B, among these samples, Af.3 and Be.3 produced the most acetic acid in the fermentation of Alg and GF, respectively, and of note, they were the most potent to degrade Alg and GF (as shown in Section Effects of L. Japonica Supplementation on the Capabilities of Fecal Microbiota to Metabolize Alg and GF), respectively. Moreover, Af.3 and Be.3 showed a comparatively high production of propionic acid in Alg and GF fermentation, respectively (Figures 4C,D). However, no significant differences among individuals were observed in the butyric acid concentration for both Alg and GF fermentation (Figures 4E,F). In addition, Af.3 and Be.3, which afforded the most acetic acid, produced the highest amounts of the total SCFAs in the fermentation of Alg and GF, respectively (Figures 4G,H). Subsequently, the correlation analysis showed that the production of the acetic acid and the total SCFAs were positively correlated with the consumption of both Alg and GF (Figure 5), and the production of propionic acid was positively associated with the utilization of Alg. It is well known that acetic acid and propionic acid are both beneficial for host health (45). For instance, acetic acid can serve as an energy source for gut microbiota (46) and peripheral tissues (47). Propionic acid can be converted into glucose by intestinal gluconeogenesis, leading to satiety and decreased hepatic glucose production (48). The differences in the productions of SCFAs among the fecal samples were related to the abilities of the gut microbiota to degrade Alg or GF, and SCFAs production was greatly correlated with Alg or GF utilization. Considering the important roles of SCFAs in host health, individuals with more powerful gut microbiota to utilize Alg and GF could benefit more from L. japonica consumption. Thus, for a great number of normal humans, a 14-day supplementation with L. japonica could strengthen the capability of gut microbiota to metabolize Alg in L. japonica, so to enhance their benefits from L. japonica consumption.
Figure 4. The productions of acetic acid (A,B), propionic acid (C,D), butyric acid (E,F), and total short-chain fatty acids (SCFAs) (G,H) after the fermentation of Alg and GF for 48 h. Graph bars marked with different letters on top represent statistically significant results (p < 0.05) based on one-way analysis of variance (ANOVA) followed by the Tukey's Honestly Significant Difference (HSD) test, whereas bars labeled with the same letter correspond to results that show no statistically significant differences.
Figure 5. Correlation analysis between Alg and GF consumption with the contents of acetic acid (A,B), propionic acid (C,D), butyric acid (E,F), and total SCFAs (G,H).
It has been well documented that the polysaccharides are metabolized by some CAZymes which are specialized to catalyze certain types of glycosic linkage (49). Due to the substrate specificity of these enzymes in the microbiota, the percentage of monosaccharides in the consumed GF after fermentation could reflect the utilization preferences of gut microbiota. Be.1, Be.3, and Be.12 with higher GF consumption (35.25, 64.54, and 45.09%, respectively) were chosen for monosaccharide composition analysis. As shown in Figure 6, GF was mainly composed of Fuc and Gal with the percentages of 30.83 and 46.18%, respectively. After 48 h of fermentation, the percentage of Gal consumption in Be.1 and Be.12 accounted for 65.79 and 70.23%, larger than that in the original GF. Of note, Be.3, with the most amount of GF consumption, demonstrates a relative ratio of Fuc and Gal similar to that of original GF. The above results demonstrated that Gal residues in GF were more ready to be utilized by intestinal microbiota in Be.1 and Be.12 so as to benefit the growth of beneficial bacteria in the gut. It has been reported that monosaccharides linked to a side chain were more susceptible to being broken down into monomers or dimmers compared with those linked to the backbone (50). It could be hypothesized that Gal residues in GF were possibly linked to the side chains of GF or the glycosidic linkages to Gal were easy to be broken down, resulting in the higher consumption rate of Gal. Whereas, gut microbes of Be.3 may degrade GF by consuming Gal and Fuc residues in GF. The above results indicated that GF could be fermented by specific intestinal microbiota and utilized with a different strategy.
Figure 6. The percentage of monosaccharides in the consumed GF after fermentation by different fecal samples. Be.1, Be.3, and Be.12 were fecal samples obtained from the volunteer Nos. 1, 3, and 12 before the 14-day dietary intake of L. japonica, respectively.
The present study demonstrated that a 14-day L. japonica supplementation could balance Firmicutes/Bacteroidetes ratio. The Alg utilization capability was significantly enhanced by L. japonica supplementation for half of the volunteers. Gut bacteria from Bacteroidaceae and Ruminococcaceae were positively correlated with Alg utilization while those from Erysipelotrichaceae, Bacteroidaceae, and Prevotellaceae contributed to the degradation of GF. Of note, the production of acetic acid and the total SCFAs in fermentation were consistent with the consumption of Alg and GF, and the production of propionic acid was positively correlated with the utilization of Alg. Thus, L. japonica supplementation could strengthen the Alg utilization so to enhance SCFAs production. In addition, gut microbiota communities showed different monosaccharide preferences in GF consumption. The present study promoted the understanding of the impacts of dietary L. japonica on human health.
The datasets presented in this study can be found in online repositories. The names of the repository/repositories and accession number(s) can be found below: NCBI; PRJNA835688
The studies involving human participants were reviewed and approved by Ethics Committee of Dalian Polytechnic University. The patients/participants provided their written informed consent to participate in this study.
XZ: investigation, data curation, and writing-original draft. CS: formal analysis. CC: data curation. GG: methodology. LH: writing-review and editing. ZW: project administration and supervision. SS: conceptualization, writing- original draft, supervision, project administration, and funding acquisition. BZ: project administration. All authors contributed to the article and approved the submitted version.
This work was funded by the National Key Research and Development Program of China (No. 2019YFD0902005).
The authors declare that the research was conducted in the absence of any commercial or financial relationships that could be construed as a potential conflict of interest.
All claims expressed in this article are solely those of the authors and do not necessarily represent those of their affiliated organizations, or those of the publisher, the editors and the reviewers. Any product that may be evaluated in this article, or claim that may be made by its manufacturer, is not guaranteed or endorsed by the publisher.
The Supplementary Material for this article can be found online at: https://www.frontiersin.org/articles/10.3389/fnut.2022.881464/full#supplementary-material
1. Gao J, Lin L, Sun B, Zhao M. Comparison study on polysaccharide fractions from Laminaria japonica: structural characterization and bile acid binding capacity. J Agric Food Chem. (2017) 65:9790–8. doi: 10.1021/acs.jafc.7b04033
2. Peng Z, Liu M, Fang Z, Wu J, Zhang Q. Composition and cytotoxicity of a novel polysaccharide from brown alga (Laminaria japonica). Carbohydr Polym. (2012) 89:1022–6. doi: 10.1016/j.carbpol.2012.03.043
3. Wang J, Zhang Q, Zhang Z, Song H, Li P. Potential antioxidant and anticoagulant capacity of low molecular weight fucoidan fractions extracted from Laminaria japonica. Int J Biol Macromol. (2010) 46:6–12. doi: 10.1016/j.ijbiomac.2009.10.015
4. Gao J, Lin L, Sun B, Zhao M. A comparison study on polysaccharides extracted from Laminaria japonica using different methods: structural characterization and bile acid-binding capacity. Food Funct. (2017) 8:3043–52. doi: 10.1039/C7FO00218A
5. Xing R, Liu S, Yu H, Chen X, Qin Y, Li K, et al. Extraction and separation of fucoidan from Laminaria japonica with chitosan as extractant. Biomed Res Int. (2013) 2013:193689. doi: 10.1155/2013/193689
6. Gotteland M, Riveros K, Gasaly N, Carcamo C, Magne F, Liabeuf G, et al. The pros and cons of using algal polysaccharides as prebiotics. Front Nutr. (2020) 7. doi: 10.3389/fnut.2020.00163
7. O'Sullivan L, Murphy B, McLoughlin P, Duggan P, Lawlor PG, Hughes H, et al. Prebiotics from marine macroalgae for human and animal health applications. Mar Drugs. (2010) 8:2038–64. doi: 10.3390/md8072038
8. Koh HSA, Lu J, Zhou W. Structure characterization and antioxidant activity of fucoidan isolated from Undaria pinnatifida grown in New Zealand. Carbohydr Polym. (2019) 212:178–85. doi: 10.1016/j.carbpol.2019.02.040
9. Usoltseva RV, Malyarenko OS, Anastyuk SD, Shevchenko NM, Silchenko AS, Zvyagintseva TN, et al. The structure of fucoidan from Sargassum oligocystum and radiosensitizing activity of galactofucans from some algae of genus Sargassum. Int J Biol Macromol. (2021) 183:1427–35. doi: 10.1016/j.ijbiomac.2021.05.128
10. Bindels LB, Delzenne NM, Cani PD, Walter J. Towards a more comprehensive concept for prebiotics. Nat Rev Gastro Hepat. (2015) 12:303–10. doi: 10.1038/nrgastro.2015.47
11. Ejima R, Akiyama M, Sato H, Tomioka S, Yakabe K, Kimizuka T, et al. Seaweed dietary fiber sodium alginate suppresses the migration of colonic inflammatory monocytes and diet-induced metabolic syndrome via the gut microbiota. Nutrients. (2021) 13. doi: 10.3390/nu13082812
12. Liu J, Wu S, Cheng Y, Liu Q, Su L, Yang Y, et al. Sargassum fusiforme alginate relieves hyperglycemia and modulates intestinal microbiota and metabolites in type 2 diabetic mice. Nutrients. (2021) 13:2887. doi: 10.3390/nu13082887
13. Shang Q, Song G, Zhang M, Shi J, Xu C, Hao J, et al. Dietary fucoidan improves metabolic syndrome in association with increased Akkermansia population in the gut microbiota of high-fat diet-fed mice. J Funct Foods. (2017) 28:138–46. doi: 10.1016/j.jff.2016.11.002
14. Zhang Y, Liu J, Mao G, Zuo J, Li S, Yang Y, et al. Sargassum fusiforme fucoidan alleviates diet-induced insulin resistance by inhibiting colon-derived ceramide biosynthesis. Food Funct. (2021) 12:8440–53. doi: 10.1039/D1FO01272J
15. Zhang T, Wu S, Ai C, Wen C, Liu Z, Wang L, et al. Galactofucan from Laminaria japonica is not degraded by the human digestive system but inhibits pancreatic lipase and modifies the intestinal microbiota. Int J Biol Macromol. (2021) 166:611–20. doi: 10.1016/j.ijbiomac.2020.10.219
16. Ding Y, Yan Y, Peng Y, Chen D, Mi J, Lu L, et al. In vitro digestion under simulated saliva, gastric and small intestinal conditions and fermentation by human gut microbiota of polysaccharides from the fruits of Lycium barbarum. Int J Biol Macromol. (2019) 125:751–60. doi: 10.1016/j.ijbiomac.2018.12.081
17. Blumenkrantz N, Asboe-Hansen G. New method for quantitative determination of uronic acids. Anal Biochem. (1973) 54:484–9. doi: 10.1016/0003-2697(73)90377-1
18. DuBois M, Gilles KA, Hamilton JK, Rebers PA, Smith F. Colorimetric method for determination of sugars and related substances. Anal Biochem. (1956) 28:350–6. doi: 10.1021/ac60111a017
19. Zhu Z, Zhu B, Sun Y, Ai C, Wu S, Wang L, et al. Sulfated polysaccharide from sea cucumber modulates the gut microbiota and its metabolites in normal mice. Int J Biol Macromol. (2018) 120:502–12. doi: 10.1016/j.ijbiomac.2018.08.098
20. Chen X, Tang R, Liu T, Dai W, Liu Q, Gong G, et al. Physicochemical properties, antioxidant activity and immunological effects in vitro of polysaccharides from Schisandra sphenanthera and Schisandra chinensis. Int J Biol Macromol. (2019) 131:744–51. doi: 10.1016/j.ijbiomac.2019.03.129
21. Gray GR, Ballou CE. Isolation and characterization of a polysaccharide containing 3-O-methyl-D-mannose from Mycobacterium phlei. J Biol Chem. (1971) 246:6835–42. doi: 10.1016/S0021-9258(19)45922-X
22. Turnbaugh PJ, Ley RE, Mahowald MA, Magrini V, Mardis ER, Gordon JI. An obesity-associated gut microbiome with increased capacity for energy harvest. Nature. (2006) 444:1027–31. doi: 10.1038/nature05414
23. Turnbaugh PJ, Hamady M, Yatsunenko T, Cantarel BL, Duncan A, Ley RE, et al. A core gut microbiome in obese and lean twins. Nature. (2009) 457:480–4. doi: 10.1038/nature07540
24. Turnbaugh PJ, Ridaura VK, Faith JJ, Rey FE, Knight R, Gordon JI. The effect of diet on the human gut microbiome: a metagenomic analysis in humanized gnotobiotic mice. Sci Transl Med. (2009) 1. doi: 10.1126/scitranslmed.3000322
25. Chen J, Liu J, Yan C, Zhang C, Pan W, Zhang W, et al. Sarcodon aspratus polysaccharides ameliorated obesity-induced metabolic disorders and modulated gut microbiota dysbiosis in mice fed a high-fat diet. Food Funct. (2020) 11:2588–602. doi: 10.1039/C9FO00963A
26. Liu M, Li X, Zhou S, Wang TTY, Zhou S, Yang K, et al. Dietary fiber isolated from sweet potato residues promotes a healthy gut microbiome profile. Food Funct. (2020) 11:689–99. doi: 10.1039/C9FO01009B
27. Shi L-l, Li Y, Wang Y, Feng Y. MDG-1, an Ophiopogon polysaccharide, regulate gut microbiota in high-fat diet-induced obese C57BL/6 mice. Int J Biol Macromol. (2015) 81:576–83. doi: 10.1016/j.ijbiomac.2015.08.057
28. Chen P, Chen X, Hao L, Du P, Li C, Han H, et al. The bioavailability of soybean polysaccharides and their metabolites on gut microbiota in the simulator of the human intestinal microbial ecosystem (SHIME). Food Chem. (2021) 36. doi: 10.1016/j.foodchem.2021.130233
29. Gou Y, Sun J, Liu J, Chen H, Kan J, Qian C, et al. Structural characterization of a water-soluble purple sweet potato polysaccharide and its effect on intestinal inflammation in mice. J Funct Foods. (2019) 61:103502. doi: 10.1016/j.jff.2019.103502
30. Liu Z, Zhang Y, Ai C, Wen C, Dong X, Sun X, et al. Gut microbiota response to sulfated sea cucumber polysaccharides in a differential manner using an in vitro fermentation model. Food Res Int. (2021) 148. doi: 10.1016/j.foodres.2021.110562
31. Uyanik GK, Güler N. A study on multiple linear regression analysis. Procedia Soc Behav Sci. (2013) 106:234–40. doi: 10.1016/j.sbspro.2013.12.027
32. Shang Q, Yin Y, Zhu L, Li G, Yu G, Wang X. Degradation of chondroitin sulfate by the gut microbiota of Chinese individuals. Int J Biol Macromol. (2016) 86:112–8. doi: 10.1016/j.ijbiomac.2016.01.055
33. Cherbuy C, Bellet D, Robert V, Mayeur C, Schwiertz A, Langella P. Modulation of the caecal gut microbiota of mice by dietary supplement containing resistant starch: impact is donor-dependent. Front Microbiol. (2019) 10:1234. doi: 10.3389/fmicb.2019.01234
34. Han X, Niu Y, Guo X, Hu Y, Yan W, He M. Age-related changes of intraocular pressure in elderly people in southern China: lingtou eye cohort study. PLoS ONE. (2016) 11:e0151766. doi: 10.1371/journal.pone.0151766
35. Orlandi M, Escudero-Casao M, Licini G. Nucleophilicity prediction via multivariate linear regression analysis. J Org Chem. (2021) 86:3555–64. doi: 10.1021/acs.joc.0c02952
36. Oliphant K, Allen-Vercoe E. Macronutrient metabolism by the human gut microbiome: major fermentation by-products and their impact on host health. Microbiome. (2019) 7. doi: 10.1186/s40168-019-0704-8
37. Kinross JM, Darzi AW, Nicholson JK. Gut microbiome-host interactions in health and disease. Genome Med. (2011) 3. doi: 10.1186/gm228
38. Cox LM, Cho I, Young SA, Anderson WHK, Waters BJ, Hung S-C, et al. The nonfermentable dietary fiber hydroxypropyl methylcellulose modulates intestinal microbiota. FASEB J. (2013) 27:692–702. doi: 10.1096/fj.12-219477
39. Fu T, Pan L, Shang Q, Yu G. Fermentation of alginate and its derivatives by different enterotypes of human gut microbiota: Towards personalized nutrition using enterotype-specific dietary fibers. Int J Biol Macromol. (2021) 183:1649–59. doi: 10.1016/j.ijbiomac.2021.05.135
40. Christopherson MR, Dawson JA, Stevenson DM, Cunningham AC, Bramhacharya S, Weimer PJ, et al. Unique aspects of fiber degradation by the ruminal ethanologen Ruminococcus albus 7 revealed by physiological and transcriptomic analysis. BMC Genom. (2014) 15. doi: 10.1186/1471-2164-15-1066
41. Kim H, Lee I, Kwon Y, Kim BC, Ha S, Lee J-h, et al. Immobilization of glucose oxidase into polyaniline nanofiber matrix for biofuel cell applications. Biosens Bioelectron. (2011) 26:3908–13. doi: 10.1016/j.bios.2011.03.008
42. Hao YT, Wu SG, Jakovlic I, Zou H, Li WX, Wang GT. Impacts of diet on hindgut microbiota and short-chain fatty acids in grass carp (Ctenopharyngodon idellus). Aquac Res. (2017) 48:5595–605. doi: 10.1111/are.13381
43. David LA, Maurice CF, Carmody RN, Gootenberg DB, Button JE, Wolfe BE, et al. Diet rapidly and reproducibly alters the human gut microbiome. Nature. (2014) 505:559–63. doi: 10.1038/nature12820
44. Lagkouvardos I, Lesker TR, Hitch TCA, Galvez EJC, Smit N, Neuhaus K, et al. Sequence and cultivation study of Muribaculaceae reveals novel species, host preference, and functional potential of this yet undescribed family. Microbiome. (2019) 7. doi: 10.1186/s40168-019-0637-2
45. Zhou W, Yan Y, Mi J, Zhang H, Lu L, Luo Q, et al. Simulated digestion and fermentation in vitro by human gut microbiota of polysaccharides from bee collected pollen of Chinese wolfberry. J Agric Food Chem. (2018) 66:898–907. doi: 10.1021/acs.jafc.7b05546
46. Peng X, Li S, Luo J, Wu X, Liu L. Effects of dietary fibers and their mixtures on short chain fatty acids and microbiota in mice guts. Food Funct. (2013) 4:932–8. doi: 10.1039/c3fo60052a
47. Wong J, de Souza R, Kendall C, Emam A, Jenkins D, Wong J, et al. Colonic health: fermentation and short chain fatty acids. J Clin Gastroenterol. (2006) 40:235–43. doi: 10.1097/00004836-200603000-00015
48. Koh A, De Vadder F, Kovatcheva-Datchary P, Bäckhed F. From dietary fiber to host physiology: short-chain fatty acids as key bacterial metabolites. Cell. (2016) 165:1332–45. doi: 10.1016/j.cell.2016.05.041
49. Cockburn DW, Koropatkin NM. Polysaccharide degradation by the intestinal microbiota and its influence on human health and disease. J Mol Biol. (2016) 428:3230–52. doi: 10.1016/j.jmb.2016.06.021
Keywords: Laminaria japonica, polysaccharide, fermentation, alginate, fucoidan, gut microbiota
Citation: Zhang X, Su C, Cao C, Gong G, Huang L, Wang Z, Song S and Zhu B (2022) Gut Microbiota of Individuals Could Be Balanced by a 14-Day Supplementation With Laminaria japonica and Differed in Metabolizing Alginate and Galactofucan. Front. Nutr. 9:881464. doi: 10.3389/fnut.2022.881464
Received: 22 February 2022; Accepted: 21 March 2022;
Published: 18 May 2022.
Edited by:
Biao Yuan, China Pharmaceutical University, ChinaReviewed by:
Yujiao Sun, Shaanxi University of Science and Technology, ChinaCopyright © 2022 Zhang, Su, Cao, Gong, Huang, Wang, Song and Zhu. This is an open-access article distributed under the terms of the Creative Commons Attribution License (CC BY). The use, distribution or reproduction in other forums is permitted, provided the original author(s) and the copyright owner(s) are credited and that the original publication in this journal is cited, in accordance with accepted academic practice. No use, distribution or reproduction is permitted which does not comply with these terms.
*Correspondence: Zhongfu Wang, d2FuZ3poZkBud3UuZWR1LmNu; Shuang Song, c29uZ3MxMDA4QDE2My5jb20=
Disclaimer: All claims expressed in this article are solely those of the authors and do not necessarily represent those of their affiliated organizations, or those of the publisher, the editors and the reviewers. Any product that may be evaluated in this article or claim that may be made by its manufacturer is not guaranteed or endorsed by the publisher.
Research integrity at Frontiers
Learn more about the work of our research integrity team to safeguard the quality of each article we publish.