- 1Key Laboratory of Cold Chain Logistics Technology for Agro-Product, Ministry of Agriculture and Rural Affairs, Institute of Agro-Products Processing and Nuclear Agricultural Technology, Hubei Academy of Agricultural Sciences, Wuhan, China
- 2School of Environmental Ecology and Biological Engineering, Wuhan Institute of Technology, Wuhan, China
- 3School of Bioengineering and Food, Hubei University of Technology, Wuhan, China
This study described the quality and microbial influence on ozone water (OW) and ultra-high pressure (UHP) processing alone or in combination with refrigerated catfish fillets. The analysis parameters included total volatile base nitrogen (TVBN), thiobarbituric acid reactive substances (TBARs), chromaticity, microbial enumeration, 16S rRNA gene sequencing, electronic nose (E-nose), and sensory score. The study found that compared with the control (CK), ozone water combined with ultra-high pressure (OCU) delayed the accumulation of TVBN and TBARs. The results of sensory evaluation illustrated that OCU obtained a satisfactory overall sensory acceptability. The counting results suggested that compared to CK, OCU significantly (p < 0.05) delayed the stack of TVC, Enterobacteriaceae, Pseudomonas, lactic acid bacteria (LAB), and hydrogen sulfide-producing bacteria (HSPB) during the storage of catfish fillets. The sequencing results reflected that the dominant were Proteobacteria, Firmicutes, Bacteroidetes, and Actinobacteria at the phylum level, and the dominant were Acinetobacter, Pseudomonas, Lelliottia, Serratia, Shewanella, Yersinia, and Aeromonas at the genus level. The dominant was Acinetobacter in initial storage, while Pseudomonas and Shewanella were in anaphase storage. Based on the TVC and TVBN, the shelf life of catfish fillets was extended by at least 3 days compared to the control. In short, the combination of ozone water and ultra-high-pressure processing is a favorable strategy to control microbial quality and delay lipid oxidation during catfish storage.
Introduction
Channel catfish (Lctalurus punctatus) is the main cultured fish in the United States (1), while there are limited types of processed catfish products in China, mainly frozen fish fillets (2). The endogenous enzymatic reactions, oxidation, and microbial activities that occur after fish die could reduce sensory acceptance and nutritional value (3). Therefore, it is necessary to take timely preservation measures for catfish to maintain their economic value. Biological preservatives are natural and harmless, while their high acquisition cost and difficulty in adapting to industrialization are still critical reasons for not being commercialized (4). Certain chemical preservatives, for example, nitrite, are powerful in the preservation of meat products, but their potential carcinogenic ability keeps most consumers away (5). Based on the above, food processors are looking for harmless and efficient alternatives to extend the shelf life of catfish. Considering fresh aquatic products, non-pasteurization is the first choice. Common physical food processing technologies include electromagnetic field, cold plasma, irradiation, high pressure, high hydrostatic pressure, ozone, ultrasonic, microwave, ultraviolet, pulsed light, pulsed electric field, and shockwaves (6, 7).
Ozone is a powerful bactericide, which can quickly kill all kinds of pathogens, including protozoa, bacteria, and viruses due to its strong oxidizing property (8, 9). In the food industry, ozone water has been widely used in the disinfection of aquatic products. Pastoriza et al. (10) reported that the replacement treatment of ozone water resulted in a reduction in the total viable count (TVC) and hydrogen sulfide-producing bacteria (HSPB) compared to seawater cleaning and ice making. However, ozone water alone is not enough to effectively inhibit certain spoilage bacteria for example Pseudomonas. Tachikawa et al. (11) found that Pseudomonas fluorescens and Pseudomonas aeruginosa in biofilms were more resistant to ozone damage than suspension cells. Therefore, hurdle technology is used as a strategy to enhance the bactericidal effectiveness. Ultra-high pressure processing (UHP), also known as ultra-high hydrostatic pressure processing, is considered to be an emerging and promising physical alternative to heat sterilization (12). UHP (usually 100–800 MPa) has been proven to inactivate a variety of microbes and endogenous enzymes while retaining the sensory properties and nutritional value of food (6, 13–15). Specifically, UHP can cause membrane damage and increase the permeability of the cell membrane, which is the main reason for the destruction of microbes. In addition, the destruction of organelles and genetic material may also be critical reasons (16). In the food industry, UHP has been widely used as a pasteurization step after food packaging (17). Ye et al. (18) reported the effect of high-pressure treatment (300 MPa/20 min) on crab meat during storage at 4°C, and the results showed that the TVC (5.71 log10 CFU/g) on the 8th day was still lower than the limit value (6 log10 CFU/g) recommended by the International Committee of Microbiological Specializations on Food (ICMSF). But expensive equipment, localized packaging options, limited inactivation of spores, cooked appearance, and lipid oxidation are major restrictions to the widespread application of ultra-high pressure (13, 16).
Microbial activity and lipid oxidation are the main factors that limit the shelf life of fish (4). Two emerging technologies, ozone water and ultra-high pressure are considered harmless, effective, and economical methods, and are widely used in food technology. Ozone water or ultra-high pressure alone used to effectively control food spoilage has been widely reported. However, there are rare reports to probe the effects of ozone water combined with ultra-high pressure on refrigerated catfish for all we know. In this study, we checked the changes in the quality of refrigerated catfish fillets treated by single or combined ultra-high pressure through physicochemical parameters [total volatile base nitrogen (TVBN), thiobarbituric acid reactive substances (TBARs), chromaticity, and electronic nose], microbial analysis (microbial plate count and next-generation sequencing), and sensory evaluation.
Materials and Methods
Fillet Samples Preparation
The live catfish (mean weight and body length were about 2.0 ± 0.5 kg and 50 ± 5.0 cm, respectively) purchased from a local Wushang supermarket (Hongshan District, Wuhan, China), were transported to the laboratory in 1 h. Cleaned catfish were slaughtered, and then the back muscles were cut into small blocks (mean weight of about 50 g). All untreated fish fillets were divided into four groups as follows: ultrapure water immersion for 10 min set as the control (lot CK), 13.28 mg/L ozone water immersion for 10 min (lot OW), 200 MPa high-pressure treatment for 10 min (lot UHP), and first 13.28 mg/L ozone water immersion for 10 min followed by 200 MPa high-pressure treatment for 10 min (lot OCU). In detail, a gas–liquid mixer (HPSJ-25, Wuhan, China) combined with an ozone generator (GCQJ-1-3, Wuhan, China) was used to produce ozone water, and the flow rate was adjusted to change the concentration of ozone water. The soaked fish fillets were packed in cooking bags, and then the cooking bags were placed in a vacuum sealer (DZD-400/S, Tengtong Co. Ltd., Nantong, China). In addition, the packaged fillets from UHP and OCU were sent to an ultra-high pressure processing machine (HPPL2-600MPa/2L, Huataisenmiao Inc., Tianjin, China). All packaged fillets were stored at 4°C, and the physicochemical, microbiological, and sensory parameters of the catfish fillets were evaluated on the 0th, 3rd, 6th, 9th, and 12th days, respectively (unless otherwise specified).
Measurement of Total Volatile Base Nitrogen
Accurately 10.0 g minced catfish meats and 90.0 ml of 7.5% (w/v) trichloroacetic acid solution were homogenized at 5,000 rpm for 1 min, and then the mixture was filtered with a GE Whatman medium-speed qualitative filter paper after centrifuging at 5,000 rpm for 10 min at 4°C. The semi-micro-quantitative nitrogen method was used to determine TVBN in catfish fillets according to the fore method (19) with a slight modification. Concretely, 5 ml of obtained filtrate and 5 ml of 1% (w/v) magnesium oxide were boiled in a reaction chamber for 6 min, and the receiving parts were 10 ml of 2% (w/v) boric acid absorption solution and a few drops of indicator (methyl red and bromocresol green at a ratio of 1:5). After the reaction, 0.01 mol/L hydrochloric acid standard solution was used to turn the indicator blue–violet. TVBN of the reactant was converted by the volume of hydrochloric acid standard solution, and its value was expressed as mg N per 100 g of catfish fillets. Each catfish fillet was measured in triplicate.
Measurement of Thiobarbituric Acid Reactive Substances
Accurately 5 g of minced catfish muscles were homogenized with 45 ml of 20% (w/v) trichloroacetic acid solution. After standing for 30 min, the mixture was centrifuged at 5,000 rpm for 10 min at 4°C. The centrifugal liquid obtained was collected after passing through a GE Whatman medium-speed qualitative filter paper. About 5 ml of supernatant and 5 ml of 0.02 mol/L thiobarbituric acid (TBA) were seethed for 30 min. The reacting substance was measured at 532 nm using a UV-vis spectrophotometer (UV-3802, Unico Instrument Co. Ltd., China) after cooling in an ice bath. 1,1,3,3-Tetraethoxypropane was used in the preparation of the standard curve, and then TBARs was expressed as mg malondialdehyde (MDA) per kilogram of catfish fillets. Each catfish fillet was measured in triplicate.
Examination of Chromaticity
The L* (representing brightness value), a* (representing red or green value), and b* (representing yellow or blue value) of catfish fillets with a thickness of 40 mm were measured by a portable colorimeter (CR-400, Konica Minolta Inc., Japan), and the colorimeter was corrected by a white standard board (L* = 85.6, a* = 0.3162, b* = 0.3238) before testing. The chromaticity of three catfish fillets was measured in triplicate, and random testing parts of each catfish fillet were selected. The whiteness and chromatic aberration (ΔE) were expressed with the following equation (20, 21):
Where ΔL*, Δa*, and Δb* represent the aberration in the L*, a*, and b* values of different processing and raw catfish fillets.
Microbiological Evaluation
Microbial Enumeration
Precisely 10.0 g of chopped catfish muscles was thoroughly mixed with 90 ml of sterile 0.85% (w/v) saline and sterile grass beads to obtain a sample suspension. Then, 1 ml of the suspension was taken at an appropriate dilution to a petri dish containing approximately 20 ml of agar medium, while gently rotating the petri dish to fully mix the bacterial solution and the culture medium. After the agar medium had solidified, the plates were placed upside down into an artificial constant temperature incubator (HPX-9082 MBE, Boxun Inc., China). These procedures were completed in the ultra-clean workbench (HFsafe-1200LC, Lishen Inc., China). The strategy of microbial selective counting was customized based on the previous studies (22, 23): (a) the total viable count (TVC) was cultivated on Plate Count Agar (Hopebio, China) at 30°C for 3 days, (b) Enterobacteriaceae were incubated on Violet Red Bile Glucose Agar (Hopebio, China) at 30°C for 2 days, (c) Pseudomonas were cultivated on Cetrimide Fucidin Cephaloridine Agar (Hopebio, China) added with selective supplements at 30°C for 3 days, (d) lactic acid bacteria (LAB) were incubated on De Man Rogosa Sharpe Agar (Hopebio, China) at 30°C for 3 days, and (e) hydrogen sulfide-producing bacteria (HSPB) were incubated on Triple Sugar Iron Agar (Hopebio, China) at 30°C for 3 days (the production of black colonies). Two fish fillets from each treatment were respectively used for microbial enumeration. The counting results from the plates with 30–300 colonies were converted to the colony-forming units (CFU) and expressed in the form of log10 CFU/g.
Microbial Diversity
The 0th and 6th days of CK were used for sequencing analysis given the previously obtained results of the TVC threshold. Similarly, OW and UHP: the 0th and 9th days; OCU: the 0th and 12th days. The cotton swabs contaminated with the contents were stored at –80°C and then sent to Meiji Biomedical Technology Co. Ltd. (Shanghai, China) for microbial sequencing. The QIAamp® DNA Tool Mini Kit was used to extract bacterial DNA from swabs, and 2.0% agarose gel electrophoresis was used to determine the DNA quality. Universal primer 1 (338-F) and primer 2 (806-R) were used to amplify the V3-V4 region of the 16S rRNA gene according to Zhang et al. (24) with appropriate modification. The PCR mix included TE buffer, 10 ng of DNA template, 4 μl of 5 × FastPfu buffer, 0.4 μl of FastPfu DNA polymerase, 0.8 μl of primer 1, 0.8 μl of primer 2, and 2 μl of dNTP (2.5 mM). The PCR amplification procedures are listed as follows: 94°C for 2 min, and followed by 30 cycles: degeneration at 94°C for 1 min, renaturation at 55°C for 40 s, elongation at 72°C for 40 s, and finally, extension at 72°C for 10 min. The amplification process was completed in the ABI GeneAmp® 9700 PCR sprint. The concentration of amplified DNA was identified by 2.0% agarose gel electrophoresis, and the TruSeq® Nano DNA LT Sample Prep Kit (Illumina Inc., San Diego, CA, United States) was used to purify the amplified DNA, and then the sequencing library was established.
The Illumina HiSeq platform (Beijing Novogene Bioinformation Science and Technology Co. Ltd., China) was used to sequence the amplified DNA. The Flash (v1.2.11) software was used to splice, denoise, merge, and non-chimeric the sequences to obtain high-quality sequences. The Uparse (v7.0.1090) software clustered high-quality sequences into the same operational taxon units (OTU) with a similarity of 97%. OTU could be converted into a genus or a phylum. To obtain the species classification information corresponding to each OTU, the silva132/16S_bacteria database was used for taxonomic comparison of species classification, and Ribosomal Database Project (RDP) classifier (v2.11). The confidence percentage of species classification was set as 70%. This procedure was carried out on the free online platform from Shanghai Majorbio Bio-pharm Technology Co. Ltd. Plotting was based on data with software R (v3.3.1), including Principal Co-ordinates Analysis (PCoA), Barplot, and Heat-map to visualize bacteria community.
Electronic Nose Analysis
The electronic nose (E-Nose) (PEN3, AIRSENSE Co. Ltd., Germany) was used to analyze the odor difference of the crayfish samples. About 2.0 g of shredded catfish muscles and 4 ml of saturated saline were placed in a 20.0 ml headspace and sealed with an E-Z Crimper (Huifen Co. Ltd., China). The headspace bottle was placed in a 45°C water bath to equilibrate for 2 min and then analyzed in the E-nose system. The test parameters were as follows: flush time and measurement time were set as 100 and 120 s, respectively, and chamber flow was set as 600 ml/min. The E-nose system consists of 10 metal oxide sensors, which are sensitive to different volatile components. The response values of the sensor from 115 to 119 s were used to visualize the odor difference between the samples, and this procedure was completed in the software WinMuster built into the E-nose system. The samples from day 0 and day 12 were used for the E-nose test, and each sample was tested in triplicate.
Sensory Evaluation
Sensory evaluation was evaluated through four indexes: smell, color, muscle tissue, and elasticity, and slightly modified by Li et al. (25). Each indicator included four summary descriptive words based on sensory. The descriptors respectively represented four excellent quality levels (representing 8–9 score), good (representing 6–7 score), average (representing 4–5 score), and poor (representing 2–3 score). Five experienced food professionals (three males and two females) aged 22–26 in the laboratory scored the descriptors, and the results were averaged.
Statistical Analysis
Excel 2010 (Microsoft Corporation, United States) was used to calculate data, and Origin 9.4 (Origin Lab Corporation, Northampton, MA, United States) was used to form the chart. SPSS 18.0 for Windows (SPSS Inc., Chicago, IL, United States) was used for multiple comparisons (one-way analysis of variance with the Duncan method) between data, and a confidence interval of 95% (p < 0.05) was set as the significance level. The results were expressed as means ± standard deviation (SD).
Results and Discussion
Total Volatile Base Nitrogen
As shown in Figure 1, the TVC of the different treatments gradually increased with the prolonged storage. The TVBN value of all treatments was less than 12 mg/100 g on day 0. After that, the TVBN of CK increased rapidly and exceeded the freshwater fish threshold (20 mg/100 g) defined by the Chinese National Food Safety Standard (GB 2733–2015) on the 9th day. The combined treatment did not reach this spoilage point until the 12th day, which prolonged the shelf life by at least 3 days compared with the control. In brief, the TVBN of OCU was significantly (p < 0.05) lower than that of CK during the entire storage, which suggested that ozone water combined with ultra-high pressure could effectively reduce the accumulation of TVBN produced by spoilage bacteria and endogenous protein enzymes. Similarly, studies reported that TVBN of red mullet and coho salmon was reduced to varying degrees at different pressure levels compared to unpressured treatments (26, 27). On the other hand, we observed that the TVBN of UHP was lower than OW in general, and this phenomenon was more pronounced in anaphase storage (9–12 days).
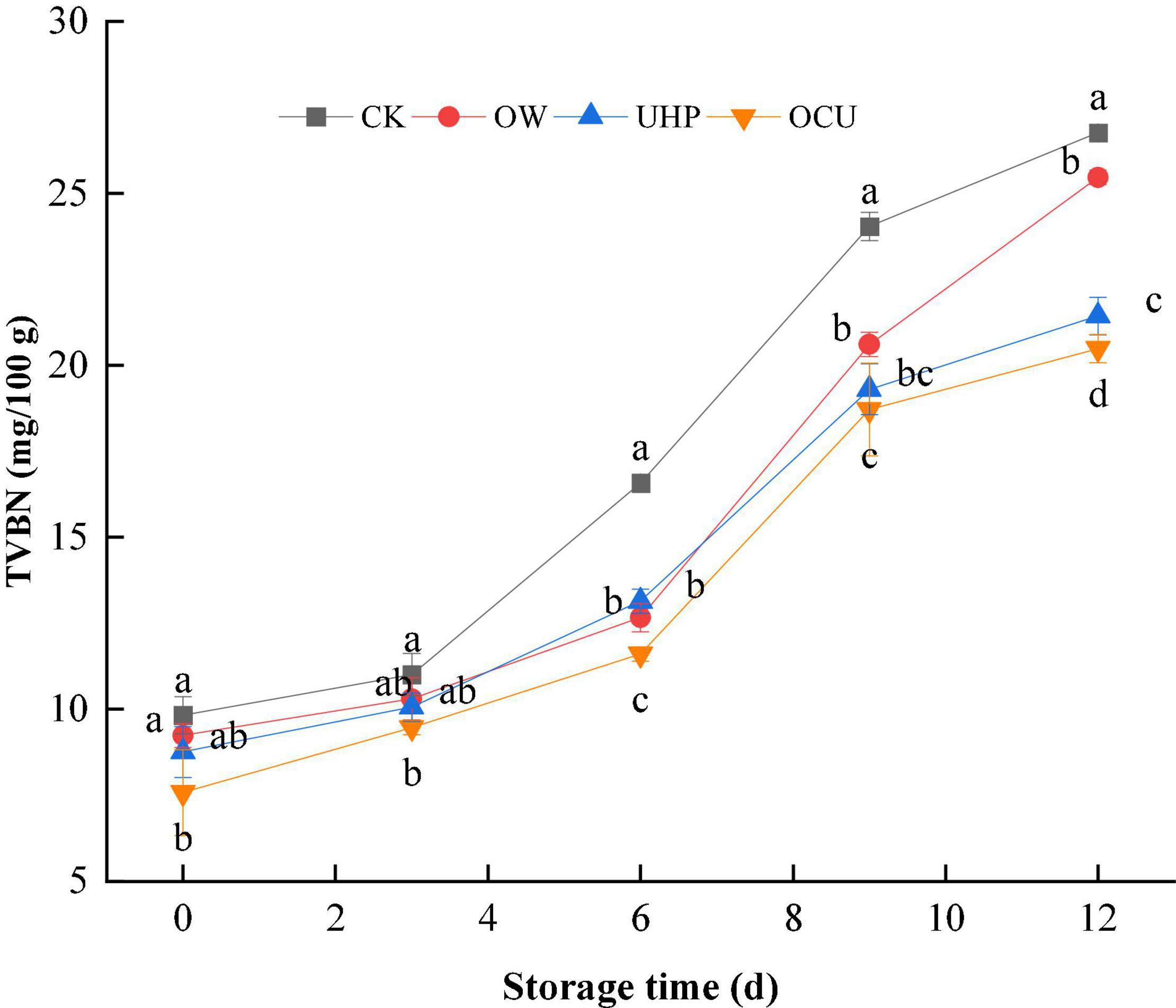
Figure 1. Changes in total volatile basic nitrogen (TVBN) of refrigerated catfish fillets. Error bars were derived from the standard deviation of means. Significant differences (p < 0.05) were expressed as alphabets (a, b, c, and d) in different treatments at the same storage time, respectively. CK, the control; OW, ozone water; UHP, ultra-high pressure; OCU, ozone water combined with ultra-high pressure.
Thiobarbituric Acid Reactive Substances
The TBARs monitoring of various secondary oxidation products is a valuable parameter to characterize lipid oxidative rancidity. As shown in Figure 2, with the storage prolongation, the TBARs of CK, OW, UHP, and OCU increased gradually. Previous studies have found that ozone water or ultra-high pressure processing could lead to increased TBARs (28–32). The TBARs of the three processing methods were lower than that of the control in early storage. The TBARs of OCU were significantly (p < 0.05) lower than that of CK during the entire storage, which suggested that ozone water combined with ultra-high pressure could effectively restrain rancidity. Pressurization could prevent the development of lipid oxidation by inhibiting the activity of endogenous enzymes (lipoxygenase, peroxidase, etc.) (15). Torres et al. (33) found that high-pressure treatment partially inhibited the development of lipid oxidation in Atlantic horse mackerel during frozen storage. The TBARs of OCU that we observed may be due to the better enzymatic inactivation effect of the combined treatment than either UHP or OW alone. The milder pressurization level used in this study (pressure is 200 MPa, and the holding time is only 10 min) may also be a reason for not complying with the UHP effect on lipid oxidation. The explanation of these phenomena was also classified as the differences in the composition and content of lipids in the species (34), the position of muscle containing heme proteins, and the pressurized level (such as pressure and duration) (31, 35).
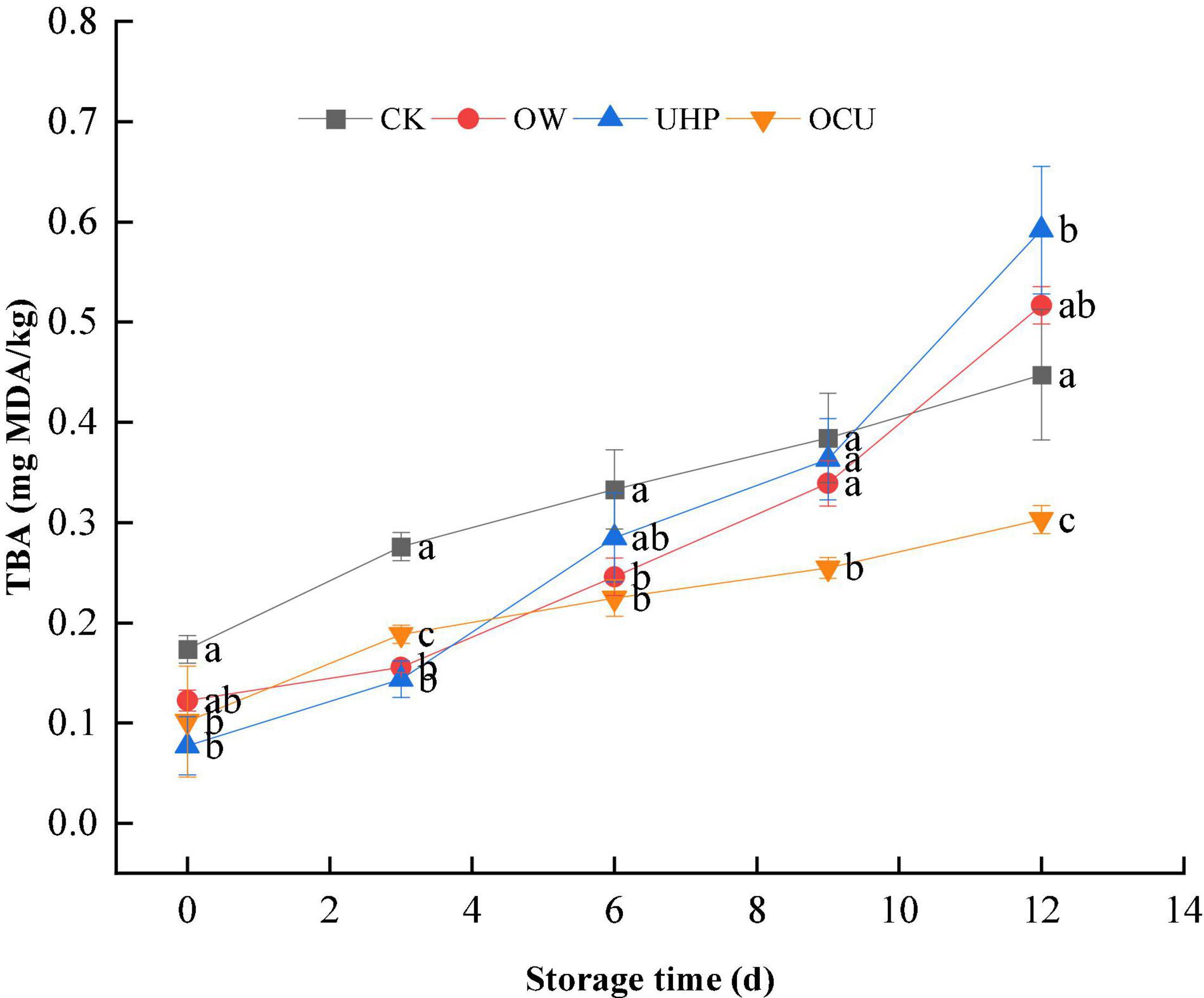
Figure 2. Changes in thiobarbituric acid reactive substances (TBARs) in refrigerated catfish fillets. Error bars were derived from the standard deviation of means. Significant differences (p < 0.05) were expressed as alphabets (a, b, c, and d) in different treatments at the same storage time, respectively. CK, the control; OW, ozone water; UHP, ultra-high pressure; OCU, ozone water combined with ultra-high pressure.
Chromaticity
The whiteness (Figure 3A) of OW was significantly (p < 0.05) lower than that of CK until the storage destination. In general, individual ozonation results in increased whiteness (36, 37). Ozone water causes the color difference of species. de Mendonça Silva et al. (32) found that ozone water processing did not affect the color of Nile tilapia fillets. These discrepancies may be due to factors such as the biological conditions of the species, storage conditions, and the treated load they receive. In addition, the whiteness of OCU was lower than that of CK until the 6th day. In detail, the whiteness of CK decreased from 61.48 on day 0 to 55.48 on day 6, while the whiteness of OCU increased from 52.38 on day 0 to 54.65 on day 6, indicating that OCU reduced the bleaching of the fillets compared to CK until storage middle. Subsequently, storage was prolonged, contrary to the serial decline of CK, the whiteness of UHP and OCU continued to rise. The results we observed reflected that catfish fillets treated with ultra-high pressure developed a cooked color, which may be attributed to the degeneration of the myofibrils and sarcoplasmic proteins that lead to changes in the surface of the meat (28). Similarly, studies have reported that high pressure processing contributed to the cooked appearance of meat (38, 39). Globin denaturation, oxidation of myoglobin, the disintegration of myofibrillar protein, lack of active pigments, and protein coagulation were also considered to be contributing factors to the whitish color (30, 40–43). And pressurization intensity could also affect color differences. Ragazzo-Sanchez et al. (44) reported that 600 MPa pressure treatment for 180 s did not affect the color of the shrimp paste samples. The converted ΔE (Figure 3B) and whiteness displayed the same trend. The L*, a*, and b* of raw catfish fillets were 51.74, 2.35, and 0.98, respectively (data not shown). Moreover, OCU contributed to the minimum of ΔE on day 0 (p < 0.05), and its increase was proportional to the prolonged storage. See the Supplementary Material for the actual photos of refrigerated catfish fillets.
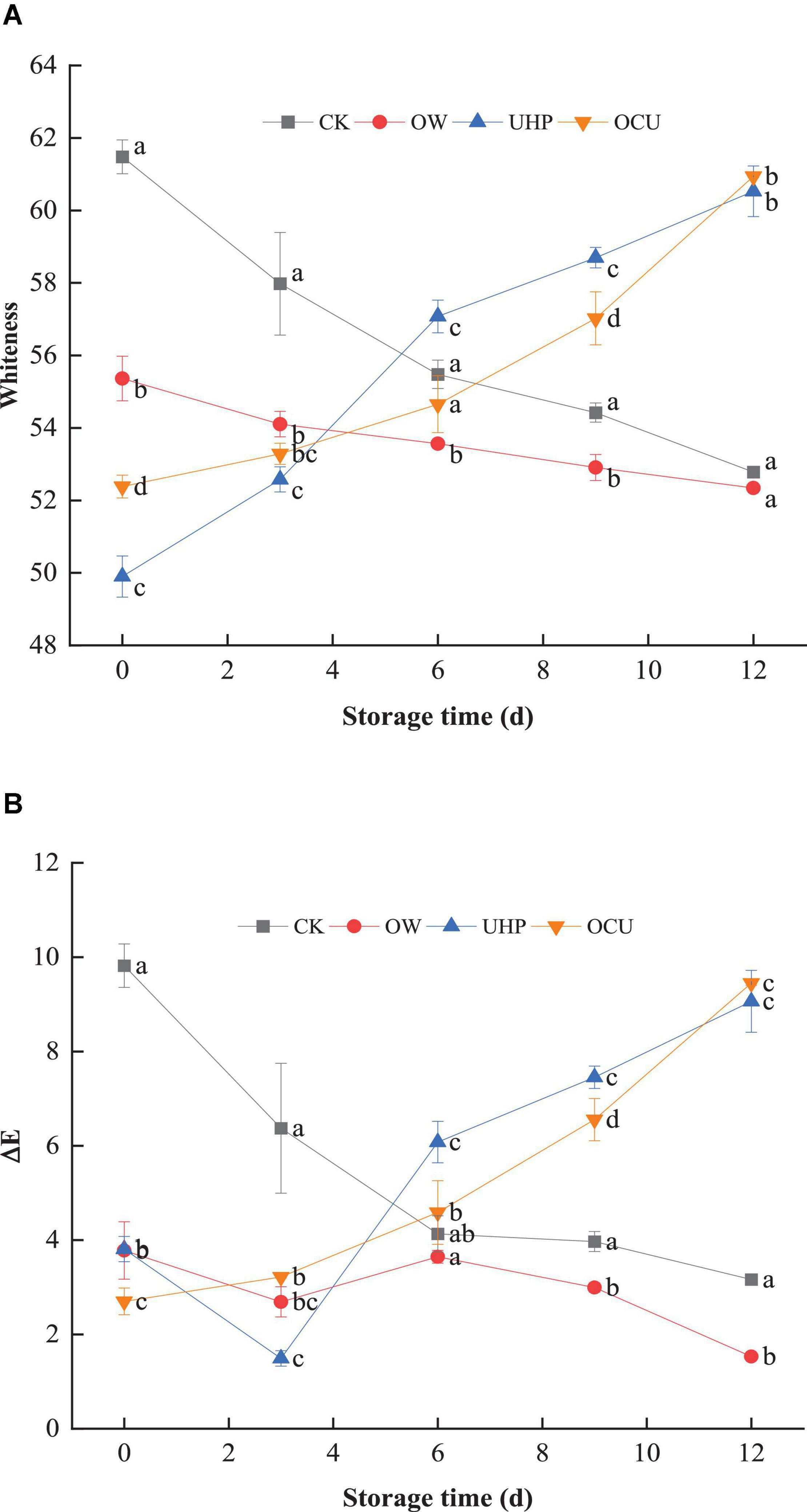
Figure 3. Panels (A,B) were respectively whiteness and chromatic aberration of refrigerated catfish fillets. Error bars were derived from the standard deviation of means. Significant differences (p < 0.05) were expressed as alphabets (a, b, c, and d) in different treatments at the same storage time, respectively. CK, the control; OW, ozone water; UHP, ultra-high pressure; OCU, ozone water combined with ultra-high pressure.
Microbial Count
As shown in Figure 4A, the TVC of different processing was positively correlated with prolonged storage. Specifically, TVC of OW, UHP, and OCU was 4.28, 4.88, and 4.06 log10 CFU/g at day 0, respectively, which were all lower than 5.35 log10 CFU/g of CK. The initial bacterial count of CK was relatively high and exceeded the threshold (6 log10 CFU/g) stipulated in Al–Dagal et al. (45) on the 6th day. The OCU did not reach this spoilage point until the 9th day, which prolonged the shelf life by at least 3 days compared with the control. The microbial activity was also evaluated by chemical parameters, for example, TVBN (27). Dramatically, the TVC results we observed were consistent with the formerly described TVBN. The latest evidence confirmed that there was a significant correlation between alkaline volatile nitrogen and microbial proliferation (46). In brief, TVC of OCU was significantly (p < 0.05) lower than that of CK during the entire storage, which suggested that ozone water combined with ultra-high pressure could effectively reduce the accumulation of bacteria and fungi. On the other hand, we observed that the microbial loading of UHP was lower than OW in general, and this phenomenon was more significant (p < 0.05) in 6 –12 days. Zhao et al. (47) found that Toona sinensis treated with UHP reduced more TVC compared with ozonation. The lower initial microbial load of OW may be attributed to the sublethal damage to the cells. After the harsh stress is eliminated, cell metabolism can be partially or completely repaired (44, 48). The explanation of OCU may be attributed to ultra-high pressure further ruptured the cells damaged by oxidation, resulting in irreversible degeneration. Then, we emphasized the potential of ozone water combined with ultra-high pressure to control the microbial quality of catfish fillets.
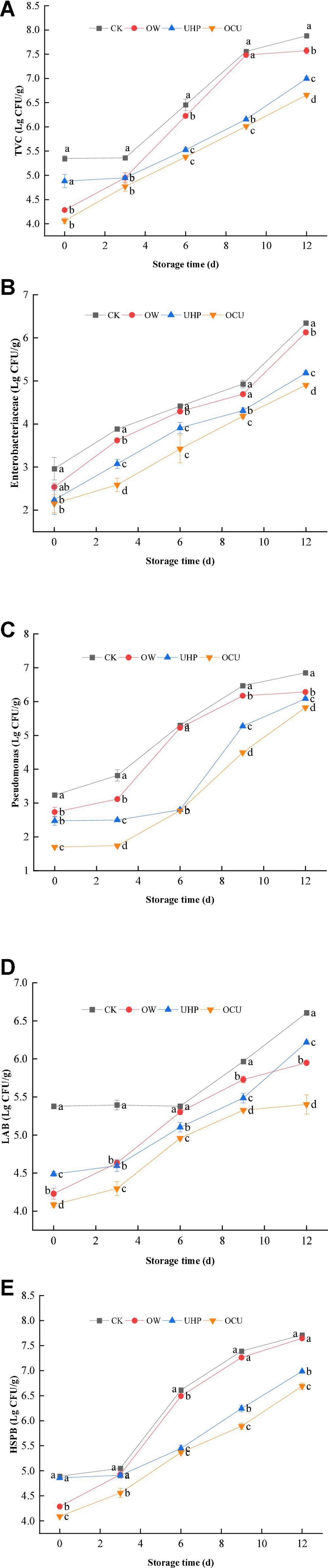
Figure 4. Microbial count of refrigerated catfish fillets, including (A) total viable count (TVC), (B) Enterobacteriaceae, (C) Pseudomonas, (D) lactic acid bacteria (LAB), and (E) hydrogen sulfide-producing bacteria (HSPB). Error bars were derived from the standard deviation of means. Significant differences (p < 0.05) were expressed as alphabets (a, b, c, and d) in different treatments at the same storage time, respectively. CK, the control; OW, ozone water; UHP, ultra-high pressure; OCU, ozone water combined with ultra-high pressure.
Enterobacteriaceae (Figure 4B), Pseudomonas (Figure 4C), LAB (Figure 4D), and HSPB (Figure 4E) gradually increased during storage. Both Enterobacteriaceae and Pseudomonas from combined treatment were significantly (p < 0.05) less than from the control during storage. In summary, the combined processing postponed the accumulation of the main genera of Proteobacteria in the entire storage. Spoilage microorganisms such as Pseudomonas and Shewanella could metabolize free amino acids in the muscles to produce volatile nitrogen compounds (49), resulting in unacceptable sensory quality. The counting results showed that Pseudomonas has the potential to survive in ozone water based on a large increase of OW in mid-to-late storage. And preceding reports also stated that Pseudomonas has the advantage of tolerating a fairly high dose of ozone water (50). The results we found suggested that UHP could effectively rupture the cell membrane of Pseudomonas. Similarly, ultra-high pressure reduced the initial load of Pseudomonas (27). Moreover, Rivas-Canedo et al. (51) reported that the presence of some gram-negative bacteria such as Pseudomonas and Aeromonas was directly related to accelerated lipid oxidation. Our reduced Pseudomonas may also contribute to the reduced TBARs as described earlier. The oxygen-free environment caused by vacuum is conducive to facultative anaerobes propagation, which also explained that certain LAB can become dominant bacteria in later storage (52). In short, the LAB of ultra-high-pressure treatments was significantly (p < 0.05) reduced compared with the control. The study reported that the same pressure (200 MPa) treatment can effectively reduce the LAB of salmon fillets during refrigeration, no matter at the beginning or end of storage (53). Compared to the control, the LAB of beef and chicken breast pressure-treated at 400 MPa/10 min decreased significantly (51). H2S is described as a strong characteristic spoilage odor of “spoiled eggs.” In general, the trend of HSPB was not much different from that of TVC. Ozone water lethality to HSPB during entire storage was mild on the whole except for the significant (p < 0.05) decline on day 6. The low initial bacterial load of OW was explained as ozone water processing may cause sublethal bacterial damage, and this damage gradually recovers as prolonged storage. Compared with OW, OCU significantly (p < 0.05) reduced the load of HSPB during storage, which also indicated that the inactivation efficacy of the combined treatment mainly came from ultra-high pressure. A previous study found that Shewanella (a representative genus producing hydrogen sulfide) achieved varying degrees of reduction through ultra-high pressure levels (27). In brief, the combination of ozone water and ultra-high-pressure processing has achieved a significant bacterial inactivation amount.
Microbial Diversity
Most OTU belonged to four bacteria phyla (Figure 5A), namely Proteobacteria, Firmicutes, Bacteroidetes, and Actinobacteria. Based on the results on day 0, the relative abundance of the most abundant phylum Proteobacteria from UHP and OCU decreased compared to CK, but excluding OW. In detail, the relative abundance of CK, OW, UHP, and OCU was 60.03, 68.82, 19.85, and 22.16%, respectively. The results emphasized that ultra-high pressure has better bacteria reduction ability than ozone water, which complied with our previous results of TVBN and TVC. Generally, compared with gram-negative bacteria, gram-positive bacteria survived more due to their thicker cell walls with 40 layers of peptidoglycan and their ability to resist ultra-high pressure (44).
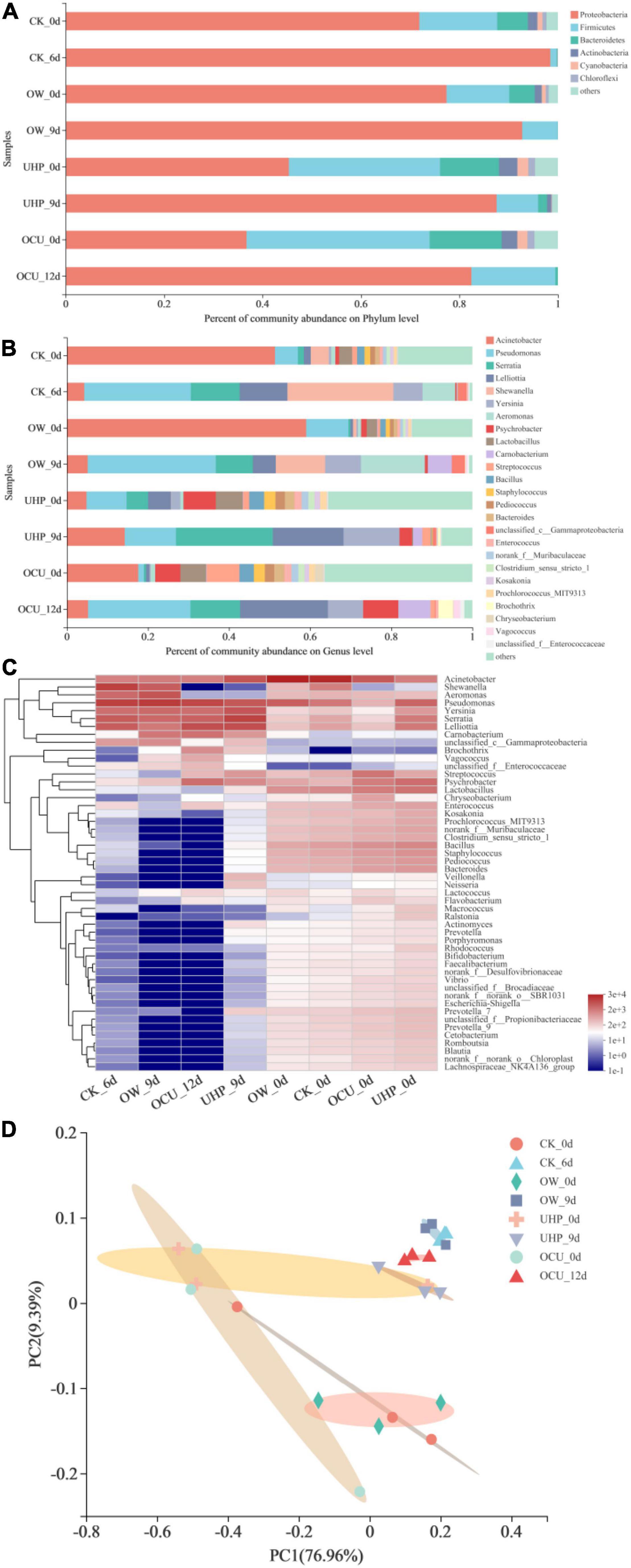
Figure 5. The relative abundance of bacterial community phylum (A) and genus (B) level in refrigerated catfish fillets. Among panel (B), genera with a relative abundance of less than 1% (all samples) were classified as “other”; panel (C) was bacterial community analysis at the genus level, presented in the form of a Heat-map (readers can choose the online version of this article to refer to the colors in the Heat-map); panel (D) was principal co-ordinates analysis (PCoA) at the species level. CK, the control; OW, ozone water; UHP, ultra-high pressure; OCU, ozone water combined with ultra-high pressure.
A total of twenty-five of relative abundance value >1% (at least 1 sample) was identified at the genus level (Figure 5B). OTU of most genera increased with prolonged storage. On day 0, the combined processing significantly reduced the relative abundance of Acinetobacter and Pseudomonas belonging to the phylum Proteobacteria, while the ozone water processing alone stimulated the vitality of genera. Concretely, Acinetobacter and Pseudomonas were reduced by 33.73 and 4.26%, respectively. In this work, Acinetobacter showed high relative abundance in initial storage, while Pseudomonas became the most dominant bacteria in anaphase storage, which may be attributed to competition between species. A previous study reported that Acinetobacter, which dominated fresh fish, was replaced by Pseudomonas or Aeromonas under different treatments (54). In addition, the abundances of Lelliottia, Serratia, Shewanella, and Yersinia belonging to the phylum Proteobacteria were less than 1% (all samples) after combined processing (OCU). Serratia belonging to Enterobacteriaceae was widely considered to be typical conditional rot-causing bacteria (55, 56). Belletti et al. (57) expressed the fact that Serratia was affected by high pressure. In short, the results at the genus level corresponded to those at the phylum level as well as the Enterobacteriaceae count. At the same time, these phenomena indicated that ozone water combined with ultra-high pressure effectively deactivated Enterobacteriaceae of the Proteobacteria. Shewanella belonging to Proteobacteria was recognized as the main contributor to hydrogen sulfide during the storage of aquatic products (58). As for Shewanella, its relative abundance was reduced in the ultra-high-pressure treatments (UHP and OCU) compared to the control during storage, and the effective reduction of Shewanella in ultra-high pressure followed the previous HSPB count result. On the other hand, certain gram-positive bacteria such as Lactobacillus, Bacillus, Staphylococcus, Pediococcus, and Enterococcus were insensitive to ultra-high-pressure processing given increased relative abundance in initial storage. Due to the presence of thick film or endospores, the gram-positive bacteria can effectively withstand ultra-high pressure (48). The study reported that the gram-negative bacteria group and coliforms decreased more than TVC and LAB under the same pressure level treatment (51).
The top fifty microbial genera in total abundance emerged in the Heat-map (Figure 5C). The colors of most genera that characterize relative abundance had obvious differences between the early and late storages, which indicated that the dominant microbiota had changed. The Principal Co-ordinates Analysis (PCoA) plot showing species clustering is exhibited in Figure 5D. The PCoA visualized the differences in microbial species between different processing methods. The first principal component (PC1) and the second principal component (PC2) were 44.33 and 20.08%, respectively. In initial storage, OTU were clustered into two areas, one formed between UHP and OCU, and the other between CK and OW. The species composition similarity between UHP and OCU was attributed to the inactivated effects of ultra-high pressure on microbial communities. In addition, OW was closest to CK due to the limitation of the bactericidal effect of ozone water. Consistent with the previous microbial enumeration results, the inactivation of ultra-high pressure was more recognized than that of ozone water.
Electronic Nose
Electronic nose technology was considered a simple and fast parameter for characterizing complex odors in food samples (59). Figure 6 is principal components analysis (PCA), showing the odor clustering of refrigerated catfish fillets from different processing. The sample variance of PC1 and PC2 was 96.63 and 2.33%, respectively, which almost characterized the volatile odor components of all catfish fillets. An overlap area was created between CK, OW, UHP, and OCU due to fresh samples, which suggested that the contribution of the samples with several processing methods to the collection of volatile odors was almost equal in the initial storage. The obvious odor difference between the samples was reflected in the anaphase storage. The interlaced area of CK and OW may be attributed to the weaker bactericidal effect of ozone water. There was a great overlap area between UHP and OCU, indicating that the odor properties of the two were approximately equal. Generally, volatile odors are mainly derived from metabolites produced by protein degradation and fat oxidation during storage as well as microbial catabolites (18). The odor distance of the ultra-high-pressure processing (UHP and OCU) on day 12 was closer to that of the fresh samples, probably because UHP inhibited the growth of putrefying bacteria and reduced the generation of some spoilage odors, which still requires further study to confirm.
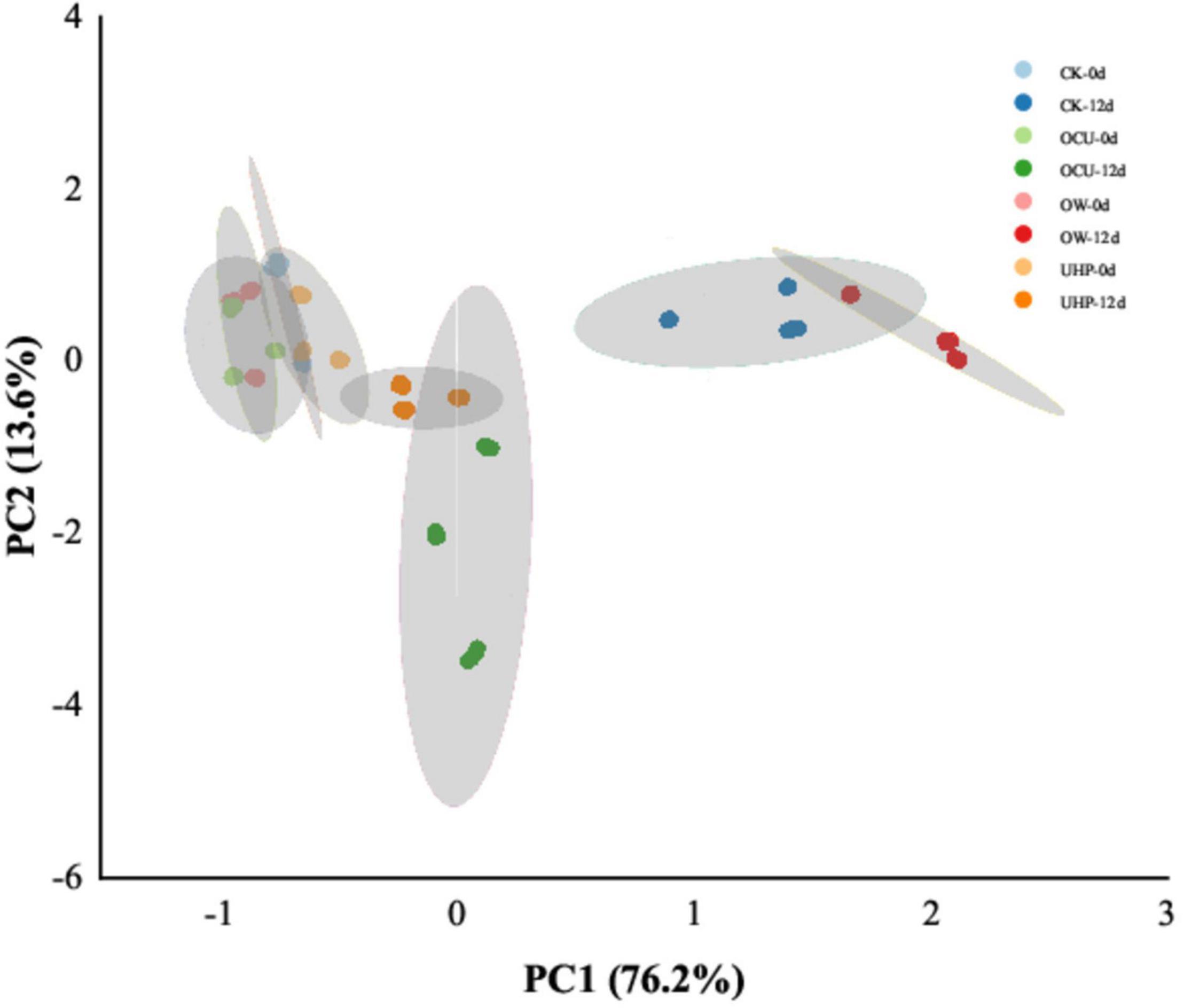
Figure 6. Principal components analysis (PCA) of electronic nose (E-nose) odor clustering in refrigerated catfish fillets. CK, the control; OW, ozone water; UHP, ultra-high pressure; OCU, ozone water combined with ultra-high pressure.
Sensory Score
Figure 7 shows the overall sensory acceptability of the samples during storage. The sensory scores of all samples decreased with prolonged storage. The scores of UHP and OCU on day 0 were 7.60 and 7.64, respectively, which attribute to cooked color caused by ultra-high-pressure treatment. From the 3rd day, OCU consistently maintained the highest overall acceptability compared with other samples. A score of 5 could be considered a threshold for not being accepted by consumers (60). CK (4.04 score), OW (4.08 score), and UHP (4.40 score) all reached levels of sensory rejection on day 9, while OCU (4.24) reached this threshold on day 12. Sensory rejection is related to microbial consumption of meat nutrients (61). The reason for the high sensory score of OCU in our results can be considered to be the synergistic inactivation effect of ozone water combined with ultra-high pressure. These sensory scores followed the changes in E-nose, indicating that the combined treatment effectively improved the sensory quality of refrigerated catfish fillets.
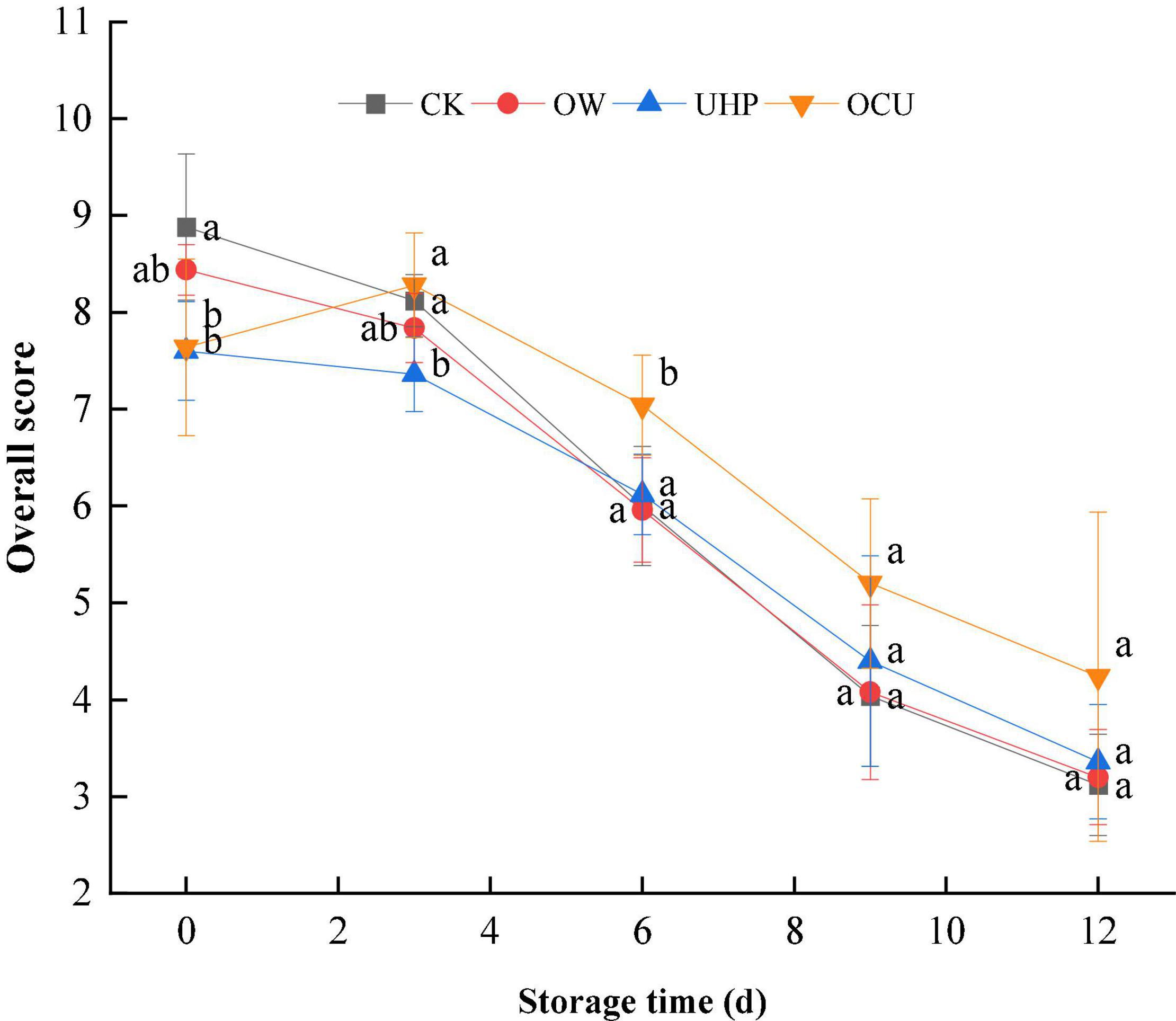
Figure 7. Overall sensory score of refrigerated catfish fillets. CK, the control; OW, ozone water; UHP, ultra-high pressure; OCU, ozone water combined with ultra-high pressure. Significant differences (p < 0.05) were expressed as lowercase alphabets in different treatments at the same storage time, respectively. CK, the control; OW, ozone water; UHP, ultra-high pressure; OCU, ozone water combined with ultra-high pressure.
Conclusion
This study suggested that ozone water combined with ultra-high pressure effectively decreased TVBN value and microbial load in catfish fillets during refrigeration. All analytical results suggested that the combined treatment inhibited catfish fillet spoilage, especially showing a significant reduction of Enterobacteriaceae, Pseudomonas, LAB, and HSPB. On the other hand, 16S rRNA gene sequencing analysis showed that the dominant bacteria in fresh catfish fillets were Acinetobacter, while the dominant bacteria in spoiled catfish fillets were mainly Pseudomonas and Shewanella. Further work needs to be performed to better understand the potential synergistic active mechanisms of ozone water and ultra-high pressure on spoilage bacteria, and to apply this method to extend the shelf life of catfish fillets during refrigeration.
Data Availability Statement
The data presented in this study are deposited in the National Center for Biotechnology Information (NCBI) repository, accession number PRJNA837024.
Author Contributions
YL: experiments, statistical analysis of data, visualization, and manuscript writing and revision. MZ: experiments and manuscript revision. YQ: experimental design and conceptualization. GX: project proposal and research methodology. LWe: supervision. LWa, WW, LS, AD, and XL: review and editing. All authors contributed to the article and approved the submitted version.
Funding
This work was funded by the China Agriculture Research System (CARS-46) and the Major Project of Scientific and Technological R&D of Hubei Agricultural Scientific and Technological Innovation Center (2020-620-000-002-03).
Conflict of Interest
The authors declare that the research was conducted in the absence of any commercial or financial relationships that could be construed as a potential conflict of interest.
Publisher’s Note
All claims expressed in this article are solely those of the authors and do not necessarily represent those of their affiliated organizations, or those of the publisher, the editors and the reviewers. Any product that may be evaluated in this article, or claim that may be made by its manufacturer, is not guaranteed or endorsed by the publisher.
Supplementary Material
The Supplementary Material for this article can be found online at: https://www.frontiersin.org/articles/10.3389/fnut.2022.880370/full#supplementary-material
Abbreviations
OW, ozone water; UHP, ultra-high pressure; OCU, ozone water combined with ultra-high pressure; TVBN, total volatile basic nitrogen; TBARs, thiobarbituric acid reactive substances; MDA, malondialdehyde; Δ E, chromatic aberration; TVC, total viable count; LAB, lactic acid bacteria; HSPB, hydrogen sulfide-producing bacteria; OTU, operational taxon units; PCoA, principal co-ordinates analysis; E-nose, electronic nose; PCA, principal components analysis.
References
1. Liu Z, Liu S, Yao J, Bao L, Zhang J, Li Y, et al. The channel catfish genome sequence provides insights into the evolution of scale formation in teleosts. Nat Commun. (2016) 7:11757. doi: 10.1038/ncomms11757
2. Zhong L, Song C, Chen X, Deng W, Xiao Y, Wang M, et al. Channel catfish in china: historical aspects, current status, and problems. Aquaculture. (2016) 465:367–73. doi: 10.1016/j.aquaculture.2016.09.032
3. Karoui R, Hassoun A. Efficiency of rosemary and basil essential oils on the shelf-life extension of Atlantic mackerel (Scomber scombrus) fillets stored at 2°C. J AOAC Int. (2019) 100:335–44. doi: 10.5740/jaoacint.16-0410
4. Hussain MA, Sumon TA, Mazumder SK, Ali MM, Jang WJ, Abualreesh MH, et al. Essential oils and chitosan as alternatives to chemical preservatives for fish and fisheries products: a review. Food Control. (2021) 129:108244. doi: 10.1016/j.foodcont.2021.108244
5. Anand S, Sati N. Artificial preservatives and their harmful effects: looking toward nature for safer alternatives. Int J Pharm Sci Res. (2013) 4:2496. doi: 10.13040/ijpsr.0975-8232.4(7).2496-01
6. Deng LZ, Mujumdar AS, Pan Z, Vidyarthi SK, Xu J, Zielinska M, et al. Emerging chemical and physical disinfection technologies of fruits and vegetables: a comprehensive review. Crit Rev Food Sci Nutr. (2020) 60:2481–508. doi: 10.1080/10408398.2019.1649633
7. Shi H, Shahidi F, Wang J, Huang Y, Zou Y, Xu W, et al. Techniques for postmortem tenderisation in meat processing: effectiveness, application and possible mechanisms. Food Prod Process Nutr. (2021) 3:21. doi: 10.1186/s43014-021-00062-0
8. Rodrigues AAZ, Queiroz M, Neves AA, Oliveira AF, Prates LHF, Freitas JF, et al. Use of ozone and detergent for removal of pesticides and improving storage quality of tomato. Food Res Int. (2019) 125:108626. doi: 10.1016/j.foodres.2019.108626
9. da Silva S, Luvielmo M, Geyer M, Pra I. Potencialidades do uso do ozônio no processamento de alimentos potential use of ozone in the food processing. Semina Ciências Agrárias. (2011) 32:659–82. doi: 10.5433/1679-0359.2011v32n2p659
10. Pastoriza L, Bernárdez M, Sampedro G, Cabo ML, Herrera JJR. Use of sterile and ozonized water as a strategy to stabilize the quality of stored refrigerated fresh fish. Food Control. (2008) 19:772–80. doi: 10.1016/j.foodcont.2007.08.003
11. Tachikawa M, Yamanaka K, Nakamuro K. Studies on the disinfection and removal of biofilms by ozone water using an artificial microbial biofilm system. Ozone Sci Eng. (2009) 31:3–9. doi: 10.1080/01919510802586566
12. Abera G, Yildiz F. Review on high-pressure processing of foods. Cogent Food Agric. (2019) 5:1568725. doi: 10.1080/23311932.2019.1568725
13. Truong BQ, Buckow R, Stathopoulos CE, Nguyen MH. Advances in High-pressure processing of fish muscles. Food Eng Rev. (2014) 7:109–29. doi: 10.1007/s12393-014-9084-9
14. Aubourg SP. Impact of high-pressure processing on chemical constituents and nutritional properties in aquatic foods: a review. Int J Food Sci Technol. (2018) 53:873–91. doi: 10.1111/ijfs.13693
15. Vázquez M, Fidalgo LG, Saraiva JA, Aubourg SP. Preservative effect of a previous high-pressure treatment on the chemical changes related to quality loss in frozen hake (Merluccius merluccius). Food Bioprocess Technol. (2017) 11:293–304. doi: 10.1007/s11947-017-2010-4
16. Zhao YM, de Alba M, Sun DW, Tiwari B. Principles and recent applications of novel non-thermal processing technologies for the fish industry-a review. Crit Rev Food Sci Nutr. (2019) 59:728–42. doi: 10.1080/10408398.2018.1495613
17. Sevenich R, Mathys A. Continuous versus discontinuous ultra-high-pressure systems for food sterilization with focus on ultra-high-pressure homogenization and high-pressure thermal sterilization: a review. Compr Rev Food Sci Food Saf. (2018) 17:646–62. doi: 10.1111/1541-4337.12348
18. Ye T, Chen X, Chen Z, Yao H, Wang Y, Lin L, et al. Quality and microbial community of high pressure shucked crab (Eriocheir sinensis) meat stored at 4°C. J Food Process Preserv. (2021) 45:e15330. doi: 10.1111/jfpp.15330
19. Yu D, Zhao W, Yang F, Jiang Q, Xu Y, Xia WA. Strategy of ultrasound-assisted processing to improve the performance of bio-based coating preservation for refrigerated carp fillets (Ctenopharyngodon idellus). Food Chem. (2021) 345:128862. doi: 10.1016/j.foodchem.2020.128862
20. Cao Y, Li B, Fan X, Wang J, Zhu Z, Huang J, et al. Synergistic recovery and enhancement of gelling properties of oxidatively damaged myofibrillar protein by L-lysine and transglutaminase. Food Chem. (2021) 358:129860. doi: 10.1016/j.foodchem.2021.129860
21. Wang Y-Y, Yan J-K, Ding Y, Rashid MT, Ma H. Effect of sweep frequency ultrasound and fixed frequency ultrasound thawing on gelling properties of myofibrillar protein from quick-frozen small yellow croaker and its possible mechanisms. Lwt. (2021) 150:111922. doi: 10.1016/j.lwt.2021.111922
22. Carrion-Granda X, Fernandez-Pan I, Jaime I, Rovira J, Mate JI. improvement of the microbiological quality of ready-to-eat peeled shrimps (Penaeus vannamei) by the use of chitosan coatings. Int J Food Microbiol. (2016) 232:144–9. doi: 10.1016/j.ijfoodmicro.2016.05.029
23. Li B, Wang X, Gao X, Ma X, Zhang L, Mei J, et al. Shelf-life extension of large yellow croaker (Larimichthys crocea) using active coatings containing lemon verbena (Lippa citriodora Kunth.) essential oil. Front Nutr. (2021) 8:678643. doi: 10.3389/fnut.2021.678643
24. Zhang J, Li Y, Yang X, Liu X, Hong H, Luo Y. Effects of oregano essential oil and nisin on the shelf life of modified atmosphere packed grass carp (Ctenopharyngodon idellus). Lwt. (2021) 147:111609. doi: 10.1016/j.lwt.2021.111609
25. Li P, Peng Y, Mei J, Xie J. Effects of microencapsulated eugenol emulsions on microbiological, chemical and organoleptic qualities of farmed Japanese sea bass (Lateolabrax japonicus) during cold storage. Lwt. (2020) 118:108831. doi: 10.1016/j.lwt.2019.108831
26. Erkan N, Üretener G, Alpas H. Effect of high pressure (Hp) on the quality and shelf life of red mullet (Mullus surmelutus). Innovative Food Sci Emerg Technol. (2010) 11:259–64. doi: 10.1016/j.ifset.2010.01.001
27. Aubourg SP, Tabilo-Munizaga G, Reyes JE, Rodríguez A, Pérez-Won M. Effect of high-pressure treatment on microbial activity and lipid oxidation in chilled coho salmon. Eur J Lipid Sci Technol. (2010) 112:362–72. doi: 10.1002/ejlt.200900173
28. Chevalier D, Le Bail A, Ghoul M. Effects of high pressure treatment (100–200 Mpa) at low temperature on turbot (Scophthalmus maximus) muscle. Food Res Int. (2001) 34:425–9. doi: 10.1016/s0963-9969(00)00187-3
29. Yagiz Y, Kristinsson HG, Balaban MO, Marshall MR. Effect of high pressure treatment on the quality of rainbow trout (Oncorhynchus mykiss) and Mahi Mahi (Coryphaena hippurus). J Food Sci. (2007) 72:C509–15. doi: 10.1111/j.1750-3841.2007.00560.x
30. Han G, Chen Q, Xia X, Liu Q, Kong B, Wang H. High hydrostatic pressure combined with moisture regulators improves the tenderness and quality of beef jerky. Meat Sci. (2021) 181:108617. doi: 10.1016/j.meatsci.2021.108617
31. Ramirez-Suarez JC, Morrissey MT. Effect of high pressure processing (Hpp) on shelf life of albacore tuna (Thunnus alalunga) minced muscle. Innov Food Sci Emerg Technol. (2006) 7:19–27. doi: 10.1016/j.ifset.2005.08.004
32. de Mendonça Silva AM, Gonçalves AA. Effect of aqueous ozone on microbial and physicochemical quality of Nile tilapia processing. J Food Process Preserv. (2017) 41:e13298. doi: 10.1111/jfpp.13298
33. Torres JA, Vázquez M, Saraiva JA, Gallardo JM, Aubourg SP. Lipid damage inhibition by previous high pressure processing in white muscle of frozen horse mackerel. Eur J Lipid Sci Technol. (2013) 115:1454–61. doi: 10.1002/ejlt.201300027
34. Montiel R, De Alba M, Bravo D, Gaya P, Medina M. Effect of high pressure treatments on smoked cod quality during refrigerated storage. Food Control. (2012) 23:429–36. doi: 10.1016/j.foodcont.2011.08.011
35. Campus M. High pressure processing of meat, meat products and seafood. Food Eng Rev. (2010) 2:256–73. doi: 10.1007/s12393-010-9028-y
36. Aday MS, Caner C. Individual and combined effects of ultrasound, ozone and chlorine dioxide on strawberry storage life. LWT Food Sci Technol. (2014) 57:344–51. doi: 10.1016/j.lwt.2014.01.006
37. Glowacz M, Rees D. Exposure to ozone reduces postharvest quality loss in red and green chilli peppers. Food Chem. (2016) 210:305–10. doi: 10.1016/j.foodchem.2016.04.119
38. Chéret R, Chapleau N, Delbarre-Ladrat C, Verrez-Bagnis V, Lamballerie MD. Effects of high pressure on texture and microstructure of sea bass (Dicentrarchus labrax L.) fillets. J Food Sci. (2005) 70:e477–83. doi: 10.1111/j.1365-2621.2005.tb11518.x
39. Techarang J, Apichartsrangkoon A. Physical, chemical and rheological parameters of pressurized Swai-fish (Pangasius hypophthalmus) emulsion incorporating fermented soybeans. Food Bioprod Process. (2015) 94:649–56. doi: 10.1016/j.fbp.2014.09.002
40. Ma Y, Yuan Y, Bi X, Zhang L, Xing Y, Che Z. Tenderization of yak meat by the combination of papain and high-pressure processing treatments. Food Bioprocess Technol. (2019) 12:681–93. doi: 10.1007/s11947-019-2245-3
41. Liu C, Gu Z, Lin X, Wang Y, Wang A, Sun Y, et al. Effects of high hydrostatic pressure (Hhp) and storage temperature on bacterial counts, color change, fatty acids and non-volatile taste active compounds of oysters (Crassostrea ariakensis). Food Chem. (2021) 372:131247. doi: 10.1016/j.foodchem.2021.131247
42. Lowder AC, Mireles Dewitt CA. Impact of high pressure processing on the functional aspects of beef muscle injected with salt and/or sodium phosphates. J Food Process Preserv. (2014) 38:1840–8. doi: 10.1111/jfpp.12155
43. Canto ACVCS, Lima BRCC, Cruz AG, Lázaro CA, Freitas DGC, Faria JAF, et al. Effect of high hydrostatic pressure on the color and texture parameters of refrigerated caiman (Caiman crocodilus Yacare) tail meat. Meat Sci. (2012) 91:255–60. doi: 10.1016/j.meatsci.2012.01.023
44. Ragazzo-Sanchez JA, Gutierrez-Sanchez Q, Ramirez-de-Leon JA, Ortiz-Basurto RI, Calderon-Santoyo M. Application of high hydrostatic pressure on pacific white shrimp (Litopenaeus vannamei) pate: microbiological, physicochemical and consumer acceptance. Food Sci Technol Int. (2018) 24:713–23. doi: 10.1177/1082013218792955
45. Al-Dagal MM, Bazaraa WA. Extension of shelf life of whole and peeled shrimp with organic acid salts and bifidobacteria. J Food Protect. (1999) 62:51–6. doi: 10.4315/0362-028X-62.1.51
46. Yu D, Wu L, Regenstein JM, Jiang Q, Yang F, Xu Y, et al. Recent advances in quality retention of non-frozen fish and fishery products: a review. Crit Rev Food Sci Nutr. (2020) 60:1747–59. doi: 10.1080/10408398.2019.1596067
47. Zhao f, Sun x-j, Yu P-F, Chen W, Liu C-J. Effects of different cold sterilization technology on quality change in toona sinensis during storage. Food Res Dev. (2018) 39:188–92. doi: 10.3969/j.issn.1005-6521.2018.04.035
48. Zhang Z-H, Wang L-H, Zeng X-A, Han Z, Brennan CS. Non-thermal technologies and its current and future application in the food industry: a review. Int J Food Sci Technol. (2019) 54:1–13. doi: 10.1111/ijfs.13903
49. Parlapani FF, Michailidou S, Anagnostopoulos DA, Koromilas S, Kios K, Pasentsis K, et al. Bacterial communities and potential spoilage markers of whole blue crab (Callinectes sapidus) stored under commercial simulated conditions. Food Microbiology. (2019) 82:325–33. doi: 10.1016/j.fm.2019.03.011
50. Fournaud J, Lauret R. Influence of ozone on the surface microbial flora of frozen beef and during thawing. Industr Aliment Agric. (1972) 89:585–9.
51. Rivas-Canedo A, Fernandez-Garcia E, Nunez M. Volatile compounds in fresh meats subjected to high pressure processing: effect of the packaging material. Meat Sci. (2009) 81:321–8. doi: 10.1016/j.meatsci.2008.08.008
52. Zhang J, Li Y, Liu X, Lei Y, Regenstein JM, Luo Y. Characterization of the microbial composition and quality of lightly salted grass carp (Ctenopharyngodon idellus) fillets with vacuum or modified atmosphere packaging. Int J Food Microbiol. (2019) 293:87–93. doi: 10.1016/j.ijfoodmicro.2018.12.022
53. Park DH, Jung JG, Jung BR, Kim G, Lee H, Kim HA, et al. Changes in salmon (Oncorhynchus keta) flesh quality following ultra-high pressure treatment and 30 D of chilled storage. J Food Sci. (2015) 80:M142–6. doi: 10.1111/1750-3841.12714
54. Liu X, Zhang Y, Li D, Luo Y. Characterization of the microbiota in lightly salted bighead carp (Aristichthys nobilis) fillets stored at 4 degrees C. Food Microbiol. (2017) 62:106–11. doi: 10.1016/j.fm.2016.10.007
55. Lavizzari T, Breccia M, Bover-Cid S, Vidal-Carou MC, Veciana-Nogues MT. Histamine, cadaverine, and putrescine produced in vitro by Enterobacteriaceae and pseudomonadaceae isolated from spinach. J Food Prot. (2010) 73:385–9. doi: 10.4315/0362-028x-73.2.385
56. Marino M, Maifreni M, Moret S, Rondinini G. The capacity of Enterobacteriaceae species to produce biogenic amines in cheese. Lett Appl Microbiol. (2000) 31:169–73. doi: 10.1046/j.1365-2672.2000.00783.x
57. Belletti N, Garriga M, Aymerich T, Bover-Cid S. Inactivation of Serratia liquefaciens on dry-cured ham by high pressure processing. Food Microbiol. (2013) 35:34–7. doi: 10.1016/j.fm.2013.03.001
58. Yang SP, Xie J, Qian YF. Determination of spoilage microbiota of pacific white shrimp during ambient and cold storage using next-generation sequencing and culture-dependent method. J Food Sci. (2017) 82:1178–83. doi: 10.1111/1750-3841.13705
59. Zhao D, Hu J, Chen W. Analysis of the relationship between microorganisms and flavour development in dry-cured grass carp by high-throughput sequencing, volatile flavour analysis and metabolomics. Food Chem. (2022) 368:130889. doi: 10.1016/j.foodchem.2021.130889
60. Li Y, Yang Z, Li J. Shelf-life extension of pacific white shrimp using algae extracts during refrigerated storage. J Sci Food Agric. (2017) 97:291–8. doi: 10.1002/jsfa.7730
Keywords: ultra-high pressure, ozone water, catfish fillets, lipid oxidation, microbial enumeration, 16S rRNA gene sequencing
Citation: Ling Y, Zhou M, Qiao Y, Xiong G, Wei L, Wang L, Wu W, Shi L, Ding A and Li X (2022) Effects of Ozone Water Combined With Ultra-High Pressure on Quality and Microorganism of Catfish Fillets (Lctalurus punctatus) During Refrigeration. Front. Nutr. 9:880370. doi: 10.3389/fnut.2022.880370
Received: 21 February 2022; Accepted: 11 April 2022;
Published: 06 July 2022.
Edited by:
Qiang Xia, Ningbo University, ChinaReviewed by:
Wenshui Xia, Jiangnan University, ChinaTao Yin, Huazhong Agricultural University, China
Copyright © 2022 Ling, Zhou, Qiao, Xiong, Wei, Wang, Wu, Shi, Ding and Li. This is an open-access article distributed under the terms of the Creative Commons Attribution License (CC BY). The use, distribution or reproduction in other forums is permitted, provided the original author(s) and the copyright owner(s) are credited and that the original publication in this journal is cited, in accordance with accepted academic practice. No use, distribution or reproduction is permitted which does not comply with these terms.
*Correspondence: Yu Qiao, cWlhb3l1NDEyQHNpbmEuY29t; Guangquan Xiong, eGlvbmdndWFuZ3F1YW5AMTYzLmNvbQ==
†These authors have contributed equally to this work and share first authorship