- College of Horticulture, Gansu Agricultural University, Lanzhou, China
Hydroponic culture has become a commercial planting model for leafy vegetables, herbs, and other plants with medicinal value. Methyl jasmonate (MeJA) is involved in primary and secondary plant metabolism; moreover, it regulates plant bioactive compounds and enhances the nutritional and medicinal value of plants. We performed targeted metabolomic analysis of the primary and secondary metabolites in substrate-grown and hydroponic Chinese chive leaves sprayed with MeJA (0, 300, 500, and 800 μM). Using ultra-performance liquid chromatography (UPLC), UPLC tandem mass spectrometry, and chemometric tools, and analyzed the antioxidant activity of these plants. We identified the biomarkers of amino acids (serine, proline, lysine, and arginine) and phenolic compounds (4-coumaric acid and protocatechuic acid) using chemometric tools to distinguish between substrate-grown and hydroponic Chinese chives treated with MeJA. MeJA (500 μM) treatment significantly increased the total sugar and amino acid (essential and non-essential amino acids and sulfur-containing amino acids) contents of hydroponically grown Chinese chives. However, the changes in total sugar and amino acid contents in Chinese chive grown in substrates showed the opposite trend. The organic acid content of hydroponically grown Chinese chives treated with MeJA decreased significantly, whereas that of substrate-grown plants treated with 300 μM MeJA increased significantly. Further, MeJA treatment significantly increased the phenolic content of substrate-grown Chinese chives. Treatment with 800 μM MeJA significantly increased the carotenoid content of substrate-grown Chinese chives and the phenolic content of hydroponic Chinese chives. In addition, the 500 μM MeJA treatment significantly increased the antioxidant activity of Chinese chives in both substrate-grown and hydroponic cultures, and promoted the accumulation of nutrients and bioactive substances. This treatment also improved the flavor quality of these plants and their nutritional and medicinal value. Thus, the results suggested that MeJA-treated plants could be used as value-added horticultural products.
Introduction
Allium species are known for their beneficial effects on human health and biological functions. These health benefits are attributed to the high content of bioactive compounds in Allium plants (1, 2). The Chinese chives (A. tuberosum Rottl. ex. Spreng.) have a long history of cultivation in China (3). As a perennial herbaceous root vegetable, it is one of the most widely used and studied species in the genus Allium (4). Chinese chives have a unique pungent garlic-like flavor and are rich in organic sulfur compounds, polysaccharides, phenols, flavonoids, and other bioactive substances. Moreover, the Chinese chives is rich in nutrients and has high medicinal value (5, 6). Chinese chives extract also plays an important role in the treatment of cardiovascular disease, diabetes, and cancer, and has antibacterial, anti-inflammatory, and antioxidant activities (7, 8). In addition, the characteristic flavor of the Chinese chives has received attention from researchers in recent years (9). As it is used for both food and medicine, improving the nutritional quality, bioactive substances, and antioxidant activity of the Chinese chives can help in increasing its potential nutritional and medicinal value. However, compared with the conventionally cultivated Chinese chives, research on the nutritional quality and bioactive substances of hydroponic Chinese chives has not been conducted extensively.
Plants are natural sources of ascorbic acid (10, 11), minerals (12, 13), and metabolites, such as sugar, amino acid, organic acid, phenolic compounds (14), flavonoids (15, 16), non-flavonoids, beta-carotene (17), betalains (18), and carotenoids (19, 20). Methyl jasmonate (MeJA) is an important cellular regulator that regulates plant development and defense responses to biotic and abiotic stresses (21, 22). In addition, MeJA is certified as a safe compound for all food items when used before harvesting (23). Phytohormone induction induces a response to adversity in plants, and is the main method of increasing the content of secondary metabolites in vegetables (24). Jasmonic acid (JA) and MeJA are the most commonly studied and widely used excitons that used to promote the accumulation of secondary metabolites in horticultural products to improve their nutritional quality (25). Exogenous MeJA can alter the levels of various primary metabolites, including plant sugars, organic acids, and amino acids (26). Treatment with MeJA promotes the production of bioactive compounds in broccoli (Brassica oleracea L. var. italica Plenck) (27). In addition, MeJA treatment promotes the biosynthesis of volatile compounds and the accumulation of secondary metabolites and non-volatile secondary metabolites (28, 29). Exogenous JA promotes the accumulation of β-carotene and the production of aromatic compounds (30). Liu et al. (31) found that MeJA promoted lycopene accumulation in tomato (Lycopersicon esculentum) by upregulating the expression of genes related to carotenoid biosynthesis. Shafiq et al. (32) reported that the application of MeJA promoted the accumulation of phenolic compounds, such as anthocyanin 3-galactoside, chlorogenic acid, and flavonols in the pericarp of apples (Malus pumila Mill.). Studies in the field of medical science have shown that a human diet rich in natural plant-synthesized polyphenols can reduce the risk of chronic and degenerative diseases, such as cancer. This is mainly because phenolic compounds rich in anthocyanins, flavonoids, and phenolic acids often exhibit strong free radical scavenging activity (33). MeJA treatment in pomegranate (Punica granatum L.) (34) and blackberry (Rubus fruticosus Pollich) (35) promotes bioactive compounds and enhances their antioxidant activities and properties beneficial for human health.
Despite their growing popularity, the nutritional quality and bioactive substances of Chinese chives have been relatively less studied, especially compared to those of other Allium vegetables, such as garlic and onions. To the best of our knowledge, previous studies have not reported the effects of MeJA treatment on primary and secondary metabolites in Chinese chives. In addition, there is a lack of information on the effects of MeJA treatment on primary and secondary metabolites in other Allium crops. Compared to soil cultivation, hydroponics can provide more efficient nutrient requirements for the plants (36). In addition, hydroponic cultivation mainly improves the yield and accumulation of functional components (37). We hypothesized that the two cultivation methods, substrate culture and hydroponic culture, could induce differences in the content of primary and secondary metabolites in Chinese chives, while the application of MeJA could promote primary and secondary metabolites in substrate-grown and hydroponic Chinese chives, consequently, increasing their antioxidant activity and medicinal value. The objective of this study was to determine how foliar spraying with MeJA affected the metabolites of substrate-grown and hydroponic Chinese chives. To better understand the metabolic fluxes between primary and secondary metabolites, we analyzed primary metabolites (including amino acids, organic acids, and sugars) and secondary metabolites (including phenolic compounds and carotenoids) in substrate-grown and hydroponic Chinese chives treated with MeJA. Our findings are important for the development of cultivation systems that can enhance the nutritional characteristics of vegetables.
Materials and Methods
Plant Material and Experimental Design
Seeds of A. tuberosum cv. “Chive God F1” were used as the experimental material. On May 8, 2020, the seedlings were cultivated in Wushan (34°25′–34°57′ N, 104°34′–105°08′ E), China, in the core demonstration area for Chinese chives. On July 20, 2021, we harvested the aboveground leaves and old roots of 1-year-old Chinese chive seedlings, removed the leaves and extra old roots while retaining 2–3 cm of old roots to promote the development of new roots. The plants were then transplanted into hydroponic and substrate cultivation systems in a glass solar greenhouse at Gansu Agricultural University in Lanzhou (36°03′ N, 103°40′ E) (Figure 1). The temperature and relative humidity conditions in the greenhouse were 20 ± 3°C/15 ± 3°C (day/night) and 60–70%, respectively. The nutrient solution for the hydroponic system was prepared according to Wu et al. (38) (Table 1), and the nutrient solution electrical conductivity and pH were 2.13 ms cm–1 and 6.15, respectively. Seedlings were fixed with cotton in 16 rectangular hydroponic boxes (0.37 m × 0.25 m × 0.2 m), each having 11 holes. Two seedlings were fixed in each hole; correspondingly, 88 plants were fixed in four boxes per treatment. The nutrient solution was continuously aerated with an air compressor and replaced every 3 d. In the substrate systems, the cultivation substrate, perlite, and vermiculite were provided by Gansu Green Energy Ruiqi Biotechnology Co., Ltd. (Tianzhu, China). The cultivation substrate, which was mainly composed of peat, coconut bran, cow dung, and perlite, was mixed with perlite, and vermiculite in a ratio of 3:1:1 in a 4-L plastic pot, and four seedlings were transplanted in each pot, that is, out of the total 88 pots for four treatments, 22 pots containing 88 seedlings were used per treatment. Each pot of substrate was supplied with 150 mL of the nutrient solution, the composition of which is listed in Table 1, on the first day after transplanting Chinese chive seedlings. In addition, substrate cultivation was watered once or twice per week.
The experiment was conducted in a completely randomized design with three replicates. The hydroponic Chinese chives were treated with the following exogenous hormones: an aqueous solution of MeJA (containing 0.1% ethanol and 0.1% Tween-20) at three concentrations (300 μM, HM300; 500 μM, HM500; and 800 μM, HM800) and a control solution (0 μM, HCK, containing 0.1% ethanol and 0.1% Tween-20). The substrate-grown Chinese chives were treated with the following exogenous hormones: an aqueous solution of MeJA (containing 0.1% ethanol and 0.1% Tween-20) at three concentrations (300 μM, SM300; 500 μM, SM500; and 800 μM, SM800) and a control solution (0 μM, SCK, containing 0.1% ethanol and 0.1% Tween-20). When the seedlings had grown to approximately 15 cm, 150 mL of the aforementioned aqueous solutions were sprayed on the plant leaves in each treatment group (i.e., total 88 plants) at 7:00–8:00 every morning from August 11, 2021, to August 17, 2021. After 35 d (August 24, 2021), Chinese chives were harvested from all treatment groups. In total, 60 Chinese chive plants were randomly selected for each treatment, with 20 plants in each of three biological replicates. All samples were ground to a powder in liquid nitrogen and stored at −80°C prior to analysis.
Analysis of Soluble Sugars and Organic Acids by High-Performance Liquid Chromatography
The sugar contents of samples were determined using High-Performance Liquid Chromatography (HPLC) (Agilent series 1100, Agilent Technologies, Santa Clara, CA, United States). The extraction and determination methods were based on Wei et al. (39), with minor modifications. Briefly, frozen Chinese chive powder (fresh weight, 0.5 g) was homogenized with ultrapure water (2.5 mL) and extracted by ultrasonication at 30°C for 60 min, followed by centrifugation at 10,000 × g for 10 min at 4°C. The supernatant (2 mL) was filtered through a 0.22-μm aqueous microporous membrane into a liquid chromatography vial for measurement. The conditions for separating the soluble sugars were as follows: detector, differential refractive index detector (Agilent series 1100, Agilent Technologies); column, LC-NH2 (4.6 × 250 mm); column temperature, 30°C; phase, acetonitrile: water (3:1, v: v); flow rate, 1.0 mL min–1; and injection volume, 25 μL. The concentrations of sugar compounds were determined based on the areas of their extracted chromatograms using the reference standards of fructose, glucose, and sucrose. Supplementary Figure 1 shows the chromatogram of sugars.
The levels of organic acids were determined using ultra-performance liquid chromatography (UPLC, Waters Corp., Milford, MA, United States). The extraction and determination methods were based on Coelho et al. (40), with minor modifications. Briefly, frozen Chinese chive powder (fresh weight, 1.5 g) was mixed with ultrapure water (7.5 mL) to extract the organic acids. The extract was centrifuged at 4°C and 10,000 × g for 10 min, and the supernatant was aspirated and filtered through a 0.22-μm aqueous phase microporous membrane into a liquid chromatography vial for measurement. The conditions for separating the organic acids were as follows: detector, UV detector; column, Thermo Hypersil COLD AQ (4.6 × 150 mm, 3 μm); column temperature, 30°C; phase, 20 mmol L–1 NaH2PO4 (pH = 2.7); flow rate, 0.5 mL min–1; and injection volume, 20 μL. The concentrations of organic acids were determined based on the areas of their extracted chromatograms using the reference standards of oxalic acid, citric acid, and malic acid. Supplementary Figure 2 shows the chromatogram of organic acids.
Analysis of Amino Acids by Ultra-Performance Liquid Chromatography Tandem Mass Spectrometry
The underivatized amino acids in Chinese chives were quantitatively analyzed by hydrophilic interaction chromatography (HILIC) using liquid chromatography/mass spectrometry (LC/MS) detection (Agilent 1290–6460, LC/MS, Agilent Technologies) (41). In brief, frozen Chinese chive powder (fresh weight, 0.1 g) was extracted with 0.5 M aqueous hydrochloric acid (1 mL). The solution was mixed by vortexing at 8,000 × g for 20 min, sonicating for 20 min in a 25°C water bath, and then centrifuging at 20,000 × g for 20 min. The supernatant (250 μL) was transferred to a liquid chromatographic vial with 15N-enriched deuterated internal standard (ISTD), and then diluted to 1 mL with 80% acetonitrile aqueous solution. The HPLC conditions were as follows: column, Agilent Infinity Lab Poroshell 120 HILIC-Z (2.1 × 100 mm, 2.7 μm); phase A, 20 mM ammonium formate (pH = 3) with water at a ratio of 9:1; phase B, 20 mM ammonium formate (pH = 3) with 90% aqueous acetonitrile at a ratio of 9:1; flow rate, 0.5 mL min–1; column temperature, 25°C; injection volume, 1 μL; total running time, 15 min; gradient time (min), 0, 11.5, and 12; and gradient concentration (%B), 100, 70, and 100. The mass spectrometry conditions were as follows: ionization mode, electrospray ionization in positive ion mode; dryer temperature, 330°C; gas flow rate, 13.0 L min–1; atomizer, 35 psi; sheath gas temperature, 390°C; sheath gas velocity, 12 L min–1; capillary voltage, 1,500 V; and nozzle voltage, 0 V. Supplementary Figure 3 shows the chromatogram of amino acids. The limit of detection (LOD) and limit of quantification (LOQ) values are listed in Supplementary Table 1.
Analysis of Phenolic Compounds by High-Performance Liquid Chromatography and Determination of Total Phenol Content
We analyzed polyphenols using an HPLC system (Waters Corp.) equipped with a 1525 pump and a 2998 photodiode array detector. The phenolic compounds were determined according to Liu et al. (42), with minor modifications. Frozen Chinese chive powder (fresh weight, 0.1 g) was added to methanol (2 mL) and placed in a refrigerator at 4°C for 60 min after shaking several times. The solution was centrifuged at 8,000 × g for 10 min at 4°C, and the supernatant was filtered through a 0.22-μm filter membrane into a liquid chromatography vial for detection. The conditions for separating the phenolic compounds were as follows: column, Waters Symmetry C18 column (4.6 × 250 mm, 5 μm); mobile phase, methanol and 1% acetic acid for gradient elution; flow rate, 1.1 mL min–1; column temperature, 30°C; and injection volume, 10 μL. The phenolic compounds were detected at 240, 280, and 322 nm (Table 2). P-hydroxybenzoic acid, protocatechuic acid, quercetin, chlorogenic acid, rutin, cinnamic acid, 4-coumaric acid, gallic acid, benzoic acid, ferulic acid, erucic acid, caffeic acid, artichoke element, kaempferol, and gentilic acid (Sigma-Aldrich, Burlington, MA, United States) were used as external standards. Supplementary Table 2 provides the retention time, wavelengths of maximum absorption in the visible region (λmax) and temporary identification of the phenolic components. Supplementary Figure 4 shows the phenolic acid chromatogram. The LOD and LOQ values are listed in Supplementary Table 3. Total phenol content was determined according to the method of Hand et al. (43) with minor modifications. Briefly, a crude extract of phenol was extracted with 50% methanol, mixed with distilled water, and then extracted with Folin-Ciocalteu phenol reagent and 20% sodium carbonate solution. After 30 min in a water at 50°C in the dark, the absorbance was measured at 760 nm using a UV-1780 spectrophotometer (Shimadzu Instruments, Suzhou, China). Total phenol content was calculated using a calibration curve for gallic acid.
Analysis of Carotenoids by High-Performance Liquid Chromatography
Carotenoids were determined according to Li et al. (44), with minor modifications. Frozen Chinese chive powder (fresh weight, 2 g) and butylated hydroxytoluene (0.1 g) were homogenized by grinding with a small volume of liquid nitrogen and transferred to a 50-mL centrifuge tube. This was added to 20% KOH-methanol solution (8 mL), and the mixture was sealed and placed in a constant water bath at 55°C for 30 min. The extract was added to a 1:2 solution of acetone: ethyl acetate, ultrasonicated for 40 min, and centrifuged at 8,000 r min–1 at 4°C. The supernatant was concentrated by rotary evaporation, and the volume was made up to 10 mL with acetone. For HPLC detection, the solution was filtered into the injection bottle with a 0.22-μm organic membrane. Samples were analyzed using an HPLC system (Analytical HPLC, 1260 Infinity II LC System; Agilent Technologies) coupled to a diode array detector. The conditions for separating the carotenoids were as follows: column, a special Welch Ultimate C30 column (4.6 × 250 mm, 5 μm) for carotenoids; column temperature, 30°C; flow rate, 1.0 mL min–1; injection volume, 20 μL; phase A, acetonitrile; phase B, water; phase C, methyl tertbutyl ether: methanol (V: V = 1: 1); and phase D, ethyl acetate. The carotenoids were detected at 470, 443, and 286 nm (Table 3) and quantified using the reference standards of α-carotene, monoepoxy zeaxanthin, zeaxanthin, lycopene, violaxanthin, lutein, and β-carotene. Supplementary Figure 5 shows the chromatogram of carotenoids. The LOD and LOQ values are listed (Supplementary Table 4).
Antioxidant Capacity of Chinese Chives
The 2, 2′-diphenyl-1-picrylhydrazyl radical (DPPH) assay for scavenging activity and ferric reducing/antioxidant capacity (FRAP) assay and 2,2′-azino-bis (3-ethylbenzothiazoline-6-sulfonic acid) (ABTS) assay for free radical scavenging activity were performed using a commercially available kit (Suzhou Keming, Suzhou, China) according to the manufacturer’s instructions. The sample was homogenized in an ice bath at a 1:10 ratio of sample fresh weight (g): volume of extract (mL), and then centrifuged at 10,000 × g for 10 min at 4°C. The supernatant was kept in ice for testing, and the supernatant and reagents were added to the enzyme plate in turn according to the instructions. The enzyme plates for the DPPH and FRAP assays were reacted for 20 min at 20–23°C. The absorbance was measured at 515 and 593 nm for DPPH and FRAP assays, respectively, using a multi-function microplate reader (Spectramax i3, Molecular Devices, Sunnyvale, CA, United States). A multifunctional microplate reader was used for the ABTS assay to detect the absorbance at 734 nm immediately after adding the reagents. Trolox was used to establish the standard curve, and the scavenging activity and reducing ability were expressed in Trolox equivalents (μmol Trolox g–l FW).
The oxygen radical absorbance capacity (ORAC) was determined using a kit (Huicheng Biological Technology, Shanghai, China) according to the manufacturer’s instructions. In brief, the sample was homogenized in an ice bath at a 1: 10 ratio of sample fresh weight (g): volume of ethanol (mL), and then centrifuged at 10,000 × g for 10 min at 4°C. The supernatant was placed in ice for further testing. The supernatant (50 μL) and 10 μL mL–1 sodium fluorescein (50 μL) was mixed in a black fluorescent enzyme plate and left to react in the dark for 30 min at 37°C. Subsequently, a free radical initiator was added, and the mixture was immediately subjected to fluorescence enzyme kinetic assay using a multi-function microplate reader (Synergy HTX, BioTek Instruments Ltd., Winooski, VT, United States). Readings were recorded every 3 min for 90 min at an excitation wavelength of 485 nm, an emission wavelength of 520 nm, and an incubation temperature of 37°C. The recordings were analyzed using the Trolox calibration curve and the area under the curve (AUC) of the fluorescence decay. The results were expressed as micro-moles of Trolox equivalent per mL. The AUC was calculated as follows:
where F0 is the initial fluorescence reading at 0 min, and Fn (n = 31) are the fluorescence readings taken every 3 min up to 90 min.
Statistical Analysis
All experimental data were analyzed using IBM SPSS Statistics version 21.0 (SPSS Inc., Chicago, IL, United States). The statistical significance of differences in treatment means was evaluated by Duncan’s multiple range test (p < 0.05). All data are presented as the mean ± standard error of three biological replicates. The figures were generated using Origin Pro 2021. For multivariate analysis, raw data were normalized by the sum, mean-centered, and divided by the square root of the standard deviation of each variable (Pareto scaling). We also conducted principal component analysis (PCA) and partial least squares discriminant analysis (PLS-DA), and calculated the variables importance in projection (VIP) scores. To evaluate differences between treated and untreated groups, heat maps (with Pearson’s correlation coefficients as a distance measure) were illustrated using the MetaboAnalyst 5.0 server (accessed on 20 October 2021).1
Results and Discussion
Effect of Methyl Jasmonate Treatments on Sugars and Organic Acids (Primary Metabolites) in Chinese Chive
The sugars and organic acid contents of substrate-grown and hydroponic Chinese chives treated with MeJA are shown in Figure 2. The fructose content of substrate-grown Chinese chives increased with the MeJA treatment (500–800 μM), and was significantly higher than that of the SCK group. In contrast, the MeJA treatment significantly reduced the glucose, sucrose, and total sugar contents of substrate-grown Chinese chives (Figure 2A). Tytgat et al. (45) reported that JA treatment reduced the sugar concentration of kale (Brassica oleracea L.), which is similar to our results for substrate-grown Chinese chives. However, treatment with 500 μM MeJA significantly increased the fructose, glucose, sucrose, and total sugar contents of hydroponic Chinese chives compared to those in the HCK group. In addition, we found that fructose and glucose constituted the main sugar fraction in hydroponic Chinese chives, accounting for 79–84% of the total sugars. In contrast, fructose constituted the main sugar fraction in substrate-grown Chinese chives, accounting for 10–65% of the total sugars. Liang et al. (46) reported that the MeJA treatment reduced the levels of most sugars in potted radish (Raphanus sativus L.), which was contrary to the results of our hydroponically grown plants. This suggested that the cultivation method, which results in high sugar content in hydroponic plants, is an important factor affecting the sugar contents of hydroponic and substrate-grown Chinese chives.
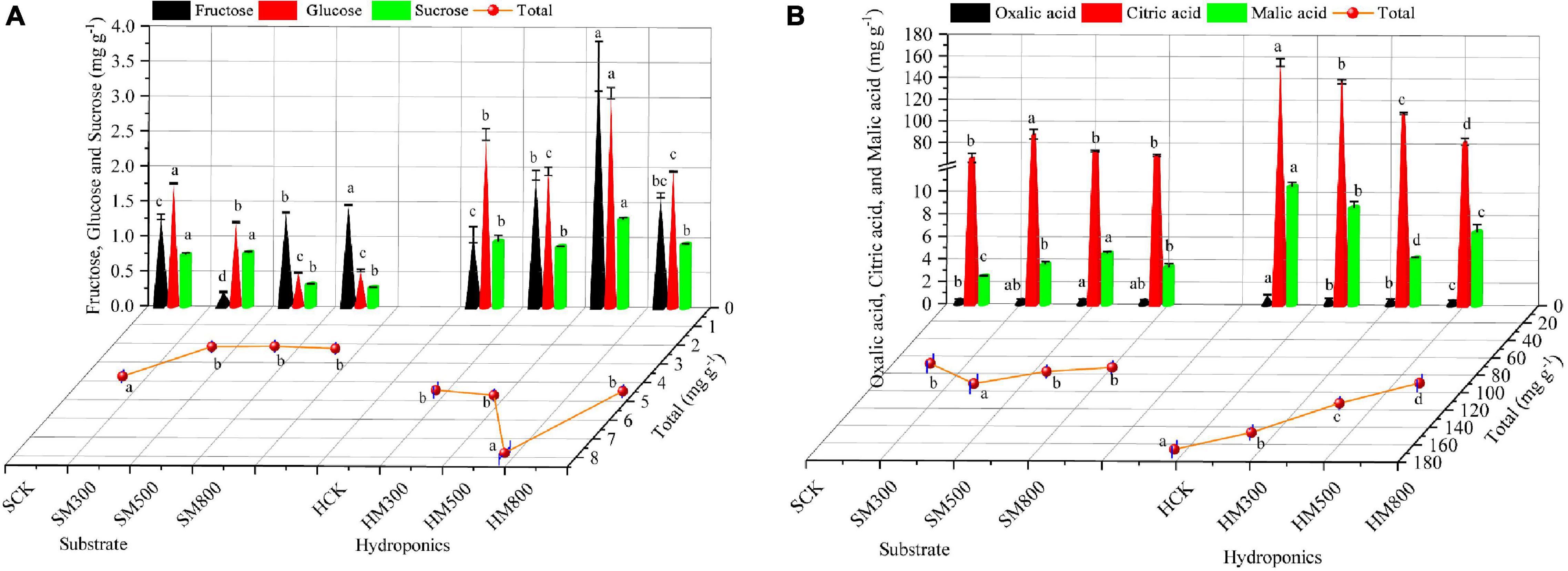
Figure 2. The sugar (A) and organic acid (B) contents of substrate-grown and hydroponic Chinese chives treated with methyl jasmonate (MeJA). Data represent the mean ± standard error (n = 3). Different lowercase letters indicate statistically significant differences according to Duncan’s multiple range tests (p < 0.05).
Citric acid is found in many fruits and vegetables, and its proper supplementation can promote appetite and enhance normal metabolism in the body (47). In this study, we found that citric acid constituted the main organic acid fraction in Chinese chives, accounting for 94–96% (substrate-grown) and 92–96% (hydroponic) of the total acid content. This was followed by malic acid, accounting for 3.6–5.9% (substrate-grown) and 3.7–7.3% (hydroponic) of the total acid content (Figure 2B). In addition, treatment with MeJA (300 μM) significantly promoted the citric and total acid contents of the substrate-grown Chinese chives. This is consistent with the findings of Kim et al. (48), who found that MeJA increased the levels of citric and organic acids in potted cabbage (Brassica oleracea L.). The MeJA treatment also significantly increased the malic acid content, which accelerates the scavenging of free radicals in human tissues (49). However, organic acid contents in hydroponic Chinese chives decreased significantly with increasing MeJA concentrations (Figure 2B). This indicated that the effects of MeJA on the organic acid content of Chinese chives differed between different cultivation methods. Oxalic acid is an anti-nutritional factor that reduces the effectiveness of calcium (a mineral element) in vegetables. It also combines with minerals in other foods to form oxalates, which are difficult for the body to absorb and may cause kidney and urinary tract stones with a poor long-term diet (50, 51). It is generally accepted that vegetables containing oxalic acid should not be consumed at the same time as foods high in calcium (52). In this study, we found that the oxalic acid content of hydroponically grown Chinese chive leaves (0.88 mg g–1) was higher than that of the substrate-grown Chinese chives (0.41 mg g–1). However, the oxalic acid content of the MeJA-treated hydroponically grown Chinese chives decreased significantly with increasing MeJA concentration. This offers a potential way to reduce the oxalic acid content of vegetables with high calcium content, thus, avoiding inadequate calcium absorption by the body due to the high oxalic acid content of vegetables.
Effect of Methyl Jasmonate Treatments on Amino Acids (Primary Metabolites) in Chinese Chive
The levels of 18 amino acids in the substrate-grown and hydroponic Chinese chives treated with MeJA are listed in Table 4. Substrate-grown and hydroponic Chinese chives were rich in lysine (73.66 and 62.50 mg g–1), threonine (1.44 and 1.41 mg g–1), phenylalanine (1.51 and 1.45 mg g–1), tryptophan (0.34 and 0.47 mg g–1), leucine (1.76 and 1.65 mg g–1) (1.84 and 1.75 mg g–1), isoleucine (1.84 and 1.75 mg g–1), valine (2.28 and 2.12 mg g–1), and methionine (1.76 and 1.14 mg g–1). Collectively, these accounted for 55 and 54% of the total amino acid content in substrate-grown and hydroponic Chinese chives, respectively, indicating that these plants were good sources of essential amino acids (EAAs). Among non-essential amino acids (NEAAS), glutamate levels were the highest in substrate-grown and hydroponic Chinese chives (26.02 and 18.72 mg g–1, respectively), whereas cysteine levels were the lowest. Amino acids are often involved in various biochemical mechanisms, such as protein synthesis, cell signaling, osmoregulation, and metabolic regulation (53). Tytgat et al. (45) reported that JA treatment reduced the levels of amino acids in kale, and Liang et al. (46) found that MeJA application reduced amino acid content in Brassica. These are similar to our results of substrate-grown Chinese chives, which showed significantly lower levels of total essential amino acids (TEAAs), total non-essential amino acids (TNEAAs), and total amino acids (TAAs) compared to SCK after the MeJA treatment. However, the MeJA (500 and 800 μM) treatments significantly increased the levels of TEAAs, TNEAAs, and TAAs in hydroponic Chinese chives compared to those in HCK. This effect was primarily due to a significant increase in the levels of EAAs (such as lysine, isoleucine, valine, and methionine) and NEAAS (such as glycine, aspartic acid, proline, and glutamic acid).
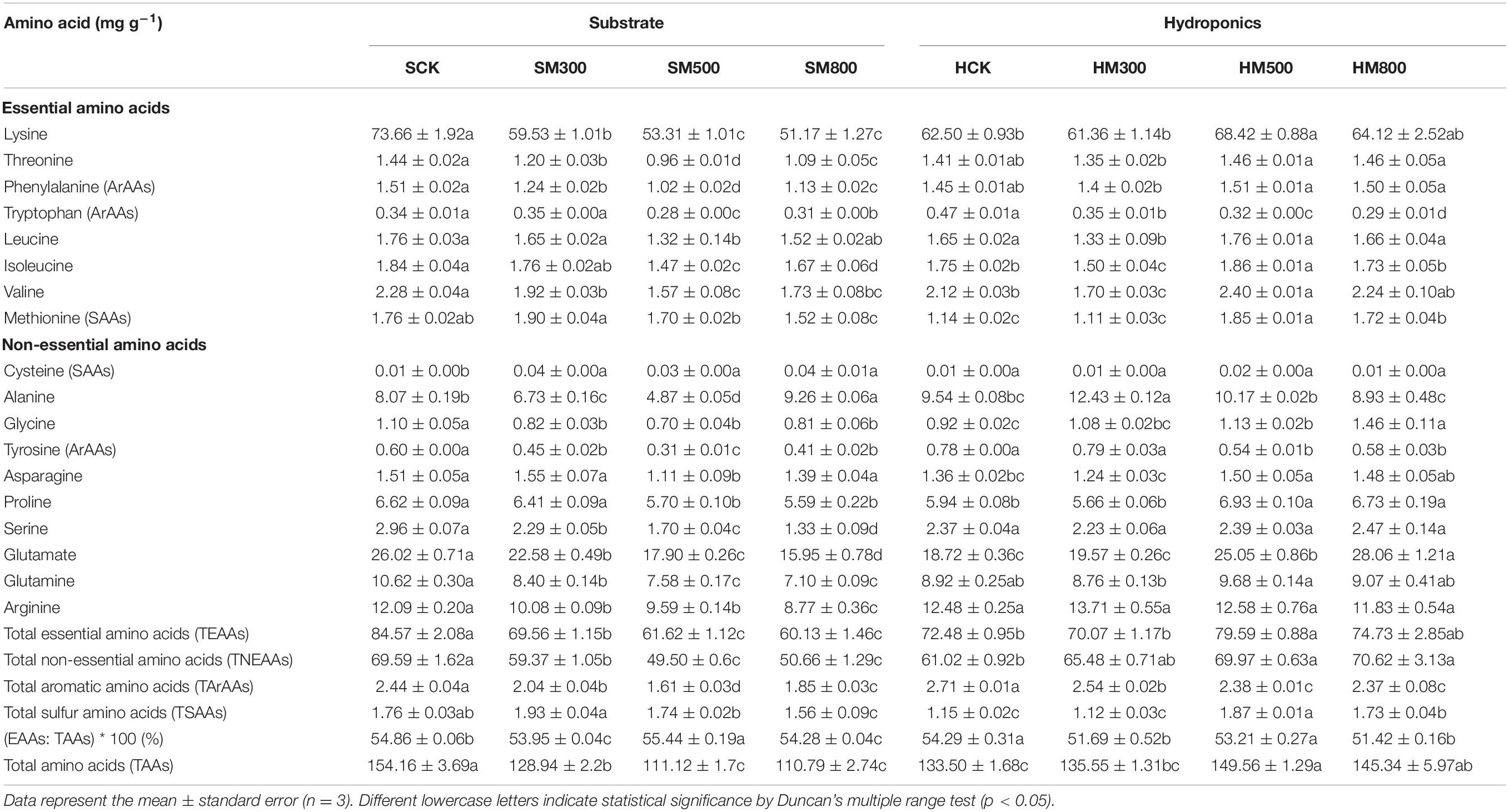
Table 4. Effect of MeJA treatments on the amino acid contents of substrate-grown and hydroponic Chinese chive.
Most amino acids are aliphatic amino acids and function as precursors to aromatic compounds (54). Phenylalanine, tryptophan, and tyrosine are aromatic amino acids that participate in the shikimate pathway, which plays an important role in the aroma development in fruits (55, 56). We found that total aromatic amino acids were inhibited to varying degrees by the MeJA treatment in both substrate-grown and hydroponic Chinese chives. This was probably because MeJA treatment accelerates amino acid deamination and decarboxylation, and degradation of proteins and heat-sensitive amino acids (such as tyrosine) (57). Further studies are required to determine the physiological and molecular mechanisms underlying the effects of MeJA on amino acid synthesis and degradation. Sulfur-containing amino acids are known to play an important role in the production of flavor substances in the Maillard reaction (58). The characteristic flavor and aroma of Chinese chives is produced by the alliinase-catalyzed hydrolysis of metabolites, such as S-alk (alkene) cysteine sulfoxides during cell rupture (59). Volatile components mainly include organosulfur compounds, of which methionine produces methyl sulfide as the main bioactive substance. Methyl sulfide provides the pungent aroma of Chinese chives (60), rather than the fruity aroma provided by aromatic amino acids. We showed that the MeJA treatment significantly increased the cysteine content (sulfur amino acids) in the substrate-grown Chinese chives; moreover, treatment with 500 and 800 μM MeJA significantly increased the methionine content (51–62%) of hydroponic Chinese chives. This suggested that the sulfur-containing amino acids are the primary reason for the flavor of Chinese chives. In addition, sulfur-containing amino acids have an antioxidant capacity comparable to that of tert-butylhydroquinone under deep-frying conditions, and can therefore, be added to foods as antioxidants to extend their shelf life (61).
Effect of Methyl Jasmonate Treatment on Primary Metabolite Profiles in Chinese Chive
An unsupervised principal component analysis (PCA) of primary metabolites (amino acids) using UPLC-MS/MS data confirmed a high degree of reproducibility between the three biological replicates and treatments. These results helped to determine that the MeJA-treated substrate-grown or hydroponic Chinese chives contained the most and least amino acid metabolites, respectively (Figures 3A, 4A). The PC1 and PC2 axes for the substrate-grown Chinese chives explained 79.4% of the total variance (59.3 and 20.1%, respectively) (Figure 3A), and those for hydroponically grown Chinese chives explained 88.7% of the total variance (66 and 22.7%, respectively) (Figure 4A). The PCA score plot clearly distinguished the MeJA-treated and control groups in a systematic manner according to the amino acids in Chinese chives. This indicated that treatment with different concentrations of MeJA had a considerable effect on the amino acid content of Chinese chives.
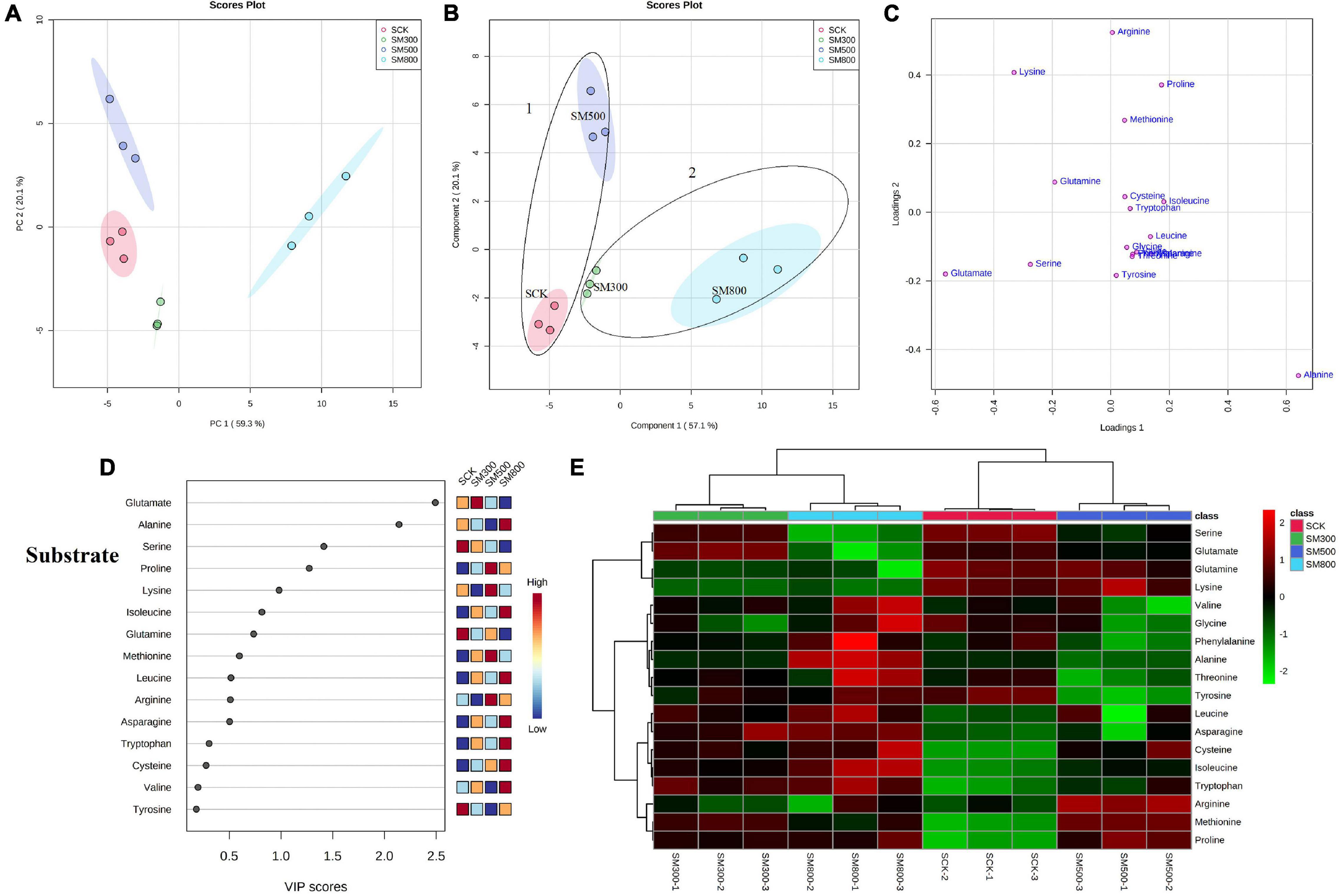
Figure 3. Statistical analyses of bioactive metabolites in substrate-grown Chinese chive treated with methyl jasmonate (MeJA). (A) An unsupervised principal component analysis (PCA) score plot of amino acid metabolites. (B) A partial least squares discriminant analysis (PLS-DA) score plot of amino acid metabolites. (C) PLS-DA score plots loaded with different amino acid metabolites. (D) VIP scores of amino acid metabolites in PLS-DA. The scores from low to high determine the importance of variables. The colored boxes on the right show the relative concentration of each metabolite from low (blue) to high (red). (E) Heat map of amino acid concentrations. The colored areas correspond to the concentrations of different amino acids from low (green) to high (red) in groups treated with different concentrations of MeJA. Each row represents an amino acid, and each column represents a treatment.
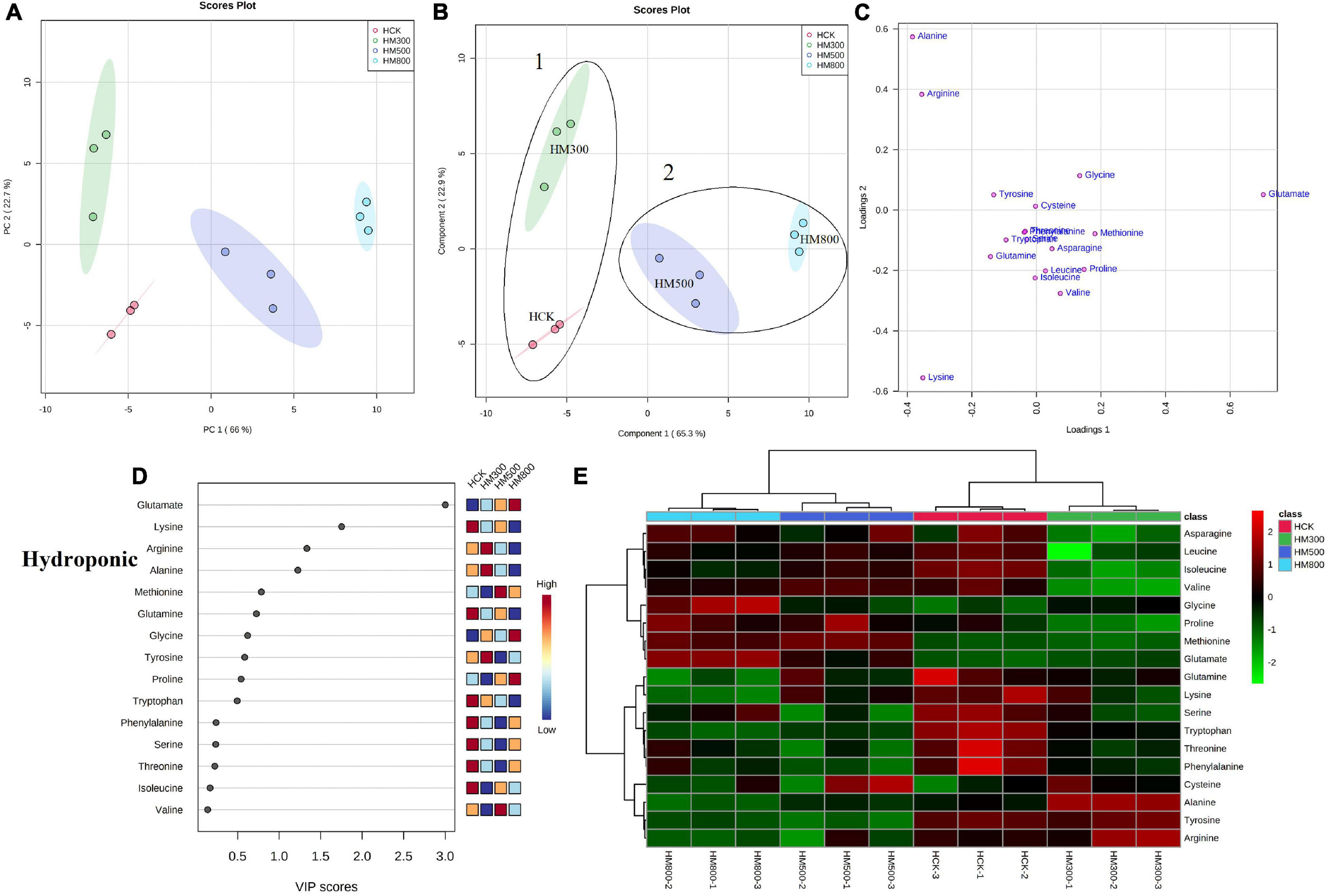
Figure 4. Statistical analyses of bioactive metabolites in hydroponic Chinese chive treated with methyl jasmonate (MeJA). (A) An unsupervised principal component analysis (PCA) score plot of amino acid metabolites. (B) A partial least squares discriminant analysis (PLS-DA) score plot of amino acid metabolites. (C) PLS-DA score plots loaded with different amino acid metabolites. (D) VIP scores of amino acid metabolites in PLS-DA. The scores from low to high determine the importance of variables. The colored boxes on the right show the relative concentration of each metabolite from low (blue) to high (red). (E) Heat map of amino acid concentrations. The colored areas correspond to the concentrations of different amino acids from low (green) to high (red) in groups treated with different concentrations of MeJA. Each row represents an amino acid, and each column represents a treatment.
To obtain more specific and meaningful information regarding the metabolites, we used PLS-DA to determine the metabolite changes in Chinese chives treated with different concentrations of MeJA. PLS-DA uses a general algorithm based on reduced dimensional discriminant analysis, which provides predictive and descriptive analyses for the selection of discriminant variables (62). This analysis has the advantage of not relying on specific distributions, resulting in more accurate predictive and descriptive models (63). We found that the PLS-DA models for substrate-grown and hydroponic Chinese chives fitted well (R2 = 0.97 and 0.99, respectively) and were highly predictable (Q2 = 0.88 and 0.96, respectively), thus, allowing us to predict the changes in amino acid metabolites. The PC1 and PC2 axes of the substrate-grown Chinese chives explained 77.2% of the total variance (57.1 and 20.1%, respectively) (Figure 3B), and those of the hydroponic Chinese chives explained 88.2% of the total variance (65.3 and 22.9%, respectively) (Figure 4B). In the PLS-DA score plot, the substrate-grown or hydroponic Chinese chives treated with different concentrations of MeJA were divided into two major groups according to the levels of 18 amino acids. Thus, different concentrations of MeJA and control treatments had significant effects on the amino acid content of the substrate-grown or hydroponic Chinese chives. Based on the proximity of data points, the SCK and SM500 groups of the substrate-grown Chinese chives showed similar amino acid metabolisms. The PLS-DA loading plots indicated that the greater the distance between the metabolite points and the origin, the greater the contribution of the metabolite to the total variation (64) (Figures 3C, 4C). Among the substrate-grown Chinese chives, lysine and glutamine were positively loaded on PC2 and separated the 500 μM MeJA-treated group from other treatment groups. Glycine, valine, phenylalanine, threonine, leucine, tyrosine, and alanine were positively loaded on PC1 and separated the 800 μM MeJA-treated group from other treatment groups. Serine and glutamate showed negative loading on PC1 and PC2 and separated HCK and the 300 μM MeJA-treated group from other treatment groups (Figures 3B,C). Therefore, these 11 amino acid metabolites explained most of the variations between substrate-grown Chinese chives treated with different concentrations of MeJA. Among the hydroponic plants, glutamate and glycine were positively loaded on PC1, separating the 800 μM MeJA-treated group from the other treatment groups. Alanine, arginine, and tyrosine were positively loaded on PC2, separating the 300 μM MeJA-treated group from the other treatment groups. Lysine, glutamine, tryptophan, serine, phenylalanine, and threonine were loaded on PC1 and PC2, separating HCK from other treatment groups. Threonine, leucine, proline, asparagine, and methionine were positively loaded on PC1 and PC2, separating the 800 μM MeJA-treated group from the other treatment groups (Figures 4B,C). Therefore, these 16 amino acid metabolites explained most of the variations between the hydroponic Chinese chives treated with different concentrations of MeJA.
To further elucidate the amino acid metabolites in Chinese chives treated with different concentrations of MeJA, we performed a hierarchical cluster analysis using the entire dataset of identified metabolites. The results yielded different clusters of samples with similar chemical composition, and we prepared a heat map based on metabolite concentrations in all samples. The heat map includes the data of the 18 amino acid metabolites identified in the substrate-grown or hydroponic Chinese chives (Figures 3E, 4E). To some extent, the hierarchical cluster analysis confirmed the two main clusters observed in the PLS-DA score plots (Figures 3B, 4B), which can be inferred from the branches at the top of the heat map (Figures 3E, 4E). In addition, the heat map indicated the trends of amino acid metabolic composition in the substrate-grown or hydroponic Chinese chives treated with different concentrations of MeJA. The substrate-grown Chinese chives in the SCK group contained higher levels of serine and glutamine, the 300 μM MeJA-treated group contained higher levels of glutamate, the 500 μM MeJA-treated group contained higher levels of arginine; and the 800 μM MeJA-treated group contained higher levels of alanine (Figure 3E). The HCK treatment group of hydroponic Chinese chives contained high levels of threonine, phenylalanine, and tryptophan, the 300 μM MeJA-treated group contained high levels of alanine, the 500 μM MeJA-treated group contained high levels of cysteine and methionine, and the 800 μM MeJA-treated group contained high levels of glycine and glutamate (Figure 4E).
In the PLS-DA analysis, we used the VIP scores to measure the strength of influence and explanatory power of each amino acid metabolite on the categorical discrimination of the sample. Higher VIP scores indicate greater differences between treatments, and are useful for selecting biomarkers that differ between treatments (48). To provide the most meaningful interpretation of the results, we considered only the top metabolites with VIP scores > 1 (65) (Figures 3D, 4D). The top four amino acid metabolites (VIP scores > 1) in the substrate-grown Chinese chives were (from highest to lowest) glutamate, alanine, serine, and proline, which had the highest relative concentrations in the SM300, SM800, SCK, and SM500 treatments, respectively. These patterns allowed us to distinguish between the treatments (Figure 3D). The top four amino acid metabolites (VIP scores > 1) in the hydroponic Chinese chives were glutamate, lysine, arginine, and alanine. Glutamate and lysine showed the highest relative concentrations in the HM800 and HCK treatments, respectively, whereas arginine and alanine showed the highest relative concentration in the HM300 treatment (Figure 4D). The higher VIP scores for these compounds suggested that they are important biomarkers for describing variations in the primary metabolites in Chinese chives.
Effect of Methyl Jasmonate Treatments on Phenolic Compounds (Secondary Metabolites) and Total Phenol Content in Chinese Chive
The levels of 15 phenolic compounds in the substrate-grown and hydroponic Chinese chives treated with MeJA are listed in Table 5. The MeJA treatment significantly increased (2.8–47%) the phenolic content of the substrate-grown Chinese chives compared with that of SCK, and the 500 μM MeJA treatment had the most significant effect. This was consistent with our previous study, where the application of 500 μM MeJA increased the total phenol contents in Chinese chives (66). The phenolic compounds of hydroponic Chinese chives treated with 500 and 800 μM MeJA were significantly higher than those of the HCK group. Phenolic compounds are known to benefit human health, and can be used as functional foods (67). Phenolics includes coumarins, phenolic acids, such as hydroxybenzoic acids (68) and hydroxycinnamic acids (69), flavonoids, such as flavonols (70), flavones (71), flavanols (72), flavanones (73), isoflavones, anthocyanins, chalcones and non-flavonoids, such as tannins, lignans, and stilbenes. Flavonoids, which are widely found in plants, have antioxidant, anticancer, antibacterial, and antimutagenic activities (74), and exhibit high levels of antioxidant activity as plant secondary metabolites (75). Among the substrate-grown Chinese chives, the total phenolic acid content was significantly lower in the MeJA-treated groups; however, the content of total flavonoids in the MeJA-treated groups was significantly higher than that in the control group. In the hydroponic plants, the total flavonoid and total phenolic acid contents of groups treated with 300 and 500 μM MeJA were significantly higher than those of the control group. This suggested that the effect of the MeJA treatment on the flavonoid and phenolic acid contents of Chinese chives depended mainly on the cultivation methods. Baek et al. reported similar results after applying MeJA treatment to pak choi (Brassica rapa L. ssp. chinensis) in soil and hydroponic systems (76). Chinese chives contain high levels of ferulic acid, which has a strong antioxidant capacity and can lower triglyceride levels (77). Protocatechuic acid and rutin have strong free radical scavenging abilities and inhibit lipid peroxidation (78, 79). In the present study, the MeJA treatment in substrate-grown Chinese chives significantly increased the levels of phenolic acids, such protocatechuic acid (6.0–33%), p-hydroxybenzoic acid (1.4–12%), chlorogenic acid (3.8–4.5%), ferulic acid (0.4–4.1%), and erucic acid (5.1–12.5%), and flavonoids, such as rutin (23–351%). In addition, the levels of cinnamic acid, gentilic acid, caffeic acid, cynarin, kaempferol, and quercetin also increased to different degrees. Among the hydroponic Chinese chives, treatment with MeJA (500 and 800 μM) significantly increased the levels of protocatechuic acid (20 and 404%, respectively), chlorogenic acid (2.0 and 4.1%, respectively), and 4-coumaric acid (12 and 22%, respectively) compared with those in the HCK treatment. Treatment with a low concentration of MeJA (300 μM) also significantly increased the levels of 4-coumaric acid, gentilic acid, caffeic acid, cynarin, rutin, and quercetin. These results indicated that the MeJA-treated substrate-grown Chinese chives had higher levels of most phenolic components compared to the hydroponic Chinese chives. In addition, the changes in the total phenol content of MeJA-treated substrate-grown and hydroponic Chinese chives showed similar trends to those of the total phenolic compounds detected by HPLC (Supplementary Figure 6).
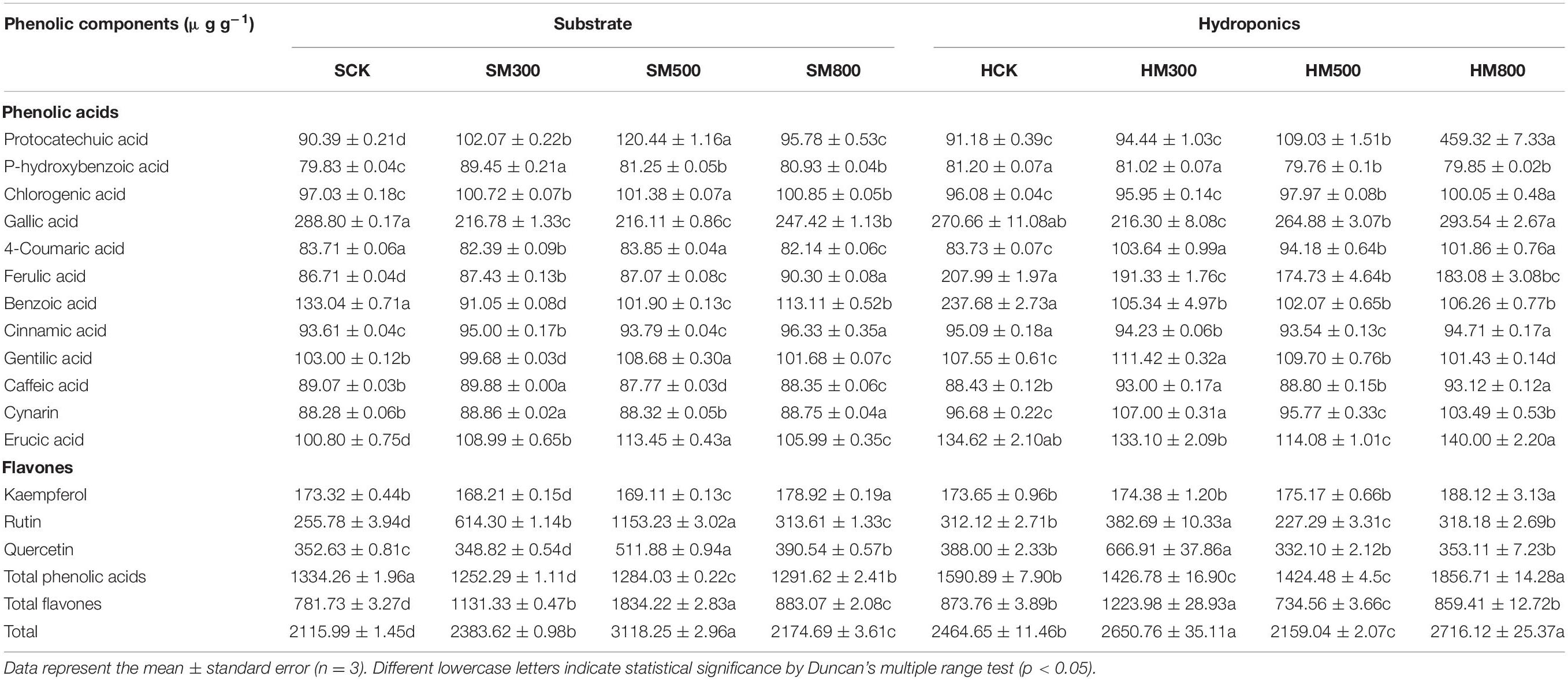
Table 5. Effect of MeJA treatments on the phenolic component contents of substrate-grown and hydroponic Chinese chive.
Effect of the Methyl Jasmonate Treatment on Secondary Metabolite Profiles in Chinese Chive
The PCA analysis showed that the PC1 and PC2 axes for the substrate-grown Chinese chives explained 96.9% of the total variance (88.7 and 8.2%, respectively) (Figure 5A), and those for hydroponic plants explained 82.9% of the total variance (48.9 and 34%, respectively) (Figure 6A). The PCA score plot distinguished the MeJA-treated groups from controls systematically and clearly based on the phenolic compounds in Chinese chives. The PLS-DA score plot showed that the PC1 and PC2 axes for the substrate-grown Chinese chives explained 94.2% of the total variance (85.4 and 8.8%, respectively) (Figure 5B), and those for hydroponic Chinese chives explained 70.3% of the total variance (45.5 and 24.8%, respectively) (Figure 6B). Based on the sample distribution in the PLS-DA plot, we hypothesized that there are two large groups in the substrate-grown Chinese chives and three large groups in the hydroponic Chinese chives (Figures 5B, 6B). Based on the proximity of data points, the SCK and SM800 groups of the substrate-grown Chinese chives showed similar phenolic metabolism and were different from the SM300 and SM500 groups. Among the hydroponic Chinese chives, the HCK and HM300 groups had similar phenolic metabolism. Further, among the substrate-grown Chinese chives, the metabolite 4-coumaric acid was loaded positively on PC2, gallic acid was loaded positively on PC1, and p-hydroxybenzoic acid was loaded negatively on PC1 and PC2. Thus, these three phenolic metabolites separated the treatment groups of the substrate-grown Chinese chives (Figures 5B,C) and explained most of the variation in plants treated with different MeJA concentrations. For the hydroponic Chinese chives, 4-coumaric acid, gallic acid, kaempferol, cynarin, and erucic acid were negatively loaded on PC1; gentilic acid, cinnamic acid, rutin, caffeic acid, and p-hydroxybenzoic acid were negatively loaded on PC2; and benzoic acid, protocatechuic acid, and ferulic acid were positively loaded on PC1. Thus, these 13 phenolic metabolites separated the various treatment groups of the hydroponic Chinese chives (Figures 6B,C) and explained most of the variation between plants treated with different concentrations of MeJA. The hierarchical clustering heat map showed 15 phenolic compound metabolites identified in the substrate-grown or hydroponic cultures of Chinese chives (Figures 5E, 6E). The SCK group contained higher levels of amino acids than other substrate-grown Chinese chives (Figure 5E), and the HM500 treatment group of hydroponic plants contained a higher level of amino acids than other hydroponics (Figure 6E). The VIP scores plot showed that in the substrate-grown Chinese chives, the top six phenolic metabolites (VIP scores > 1) were 4-coumaric acid, ferulic acid, gentilic acid, benzoic acid, p-hydroxybenzoic acid, and erucic acid (Figure 5D). In the hydroponic Chinese chives, the top three phenolic metabolites (VIP scores > 1) were protocatechuic acid, gentilic acid, and 4-coumaric acid (Figure 6D). The higher VIP scores for these compounds suggested that they were important biomarkers for describing variations in the phenolic compounds of the primary metabolites in Chinese chives.
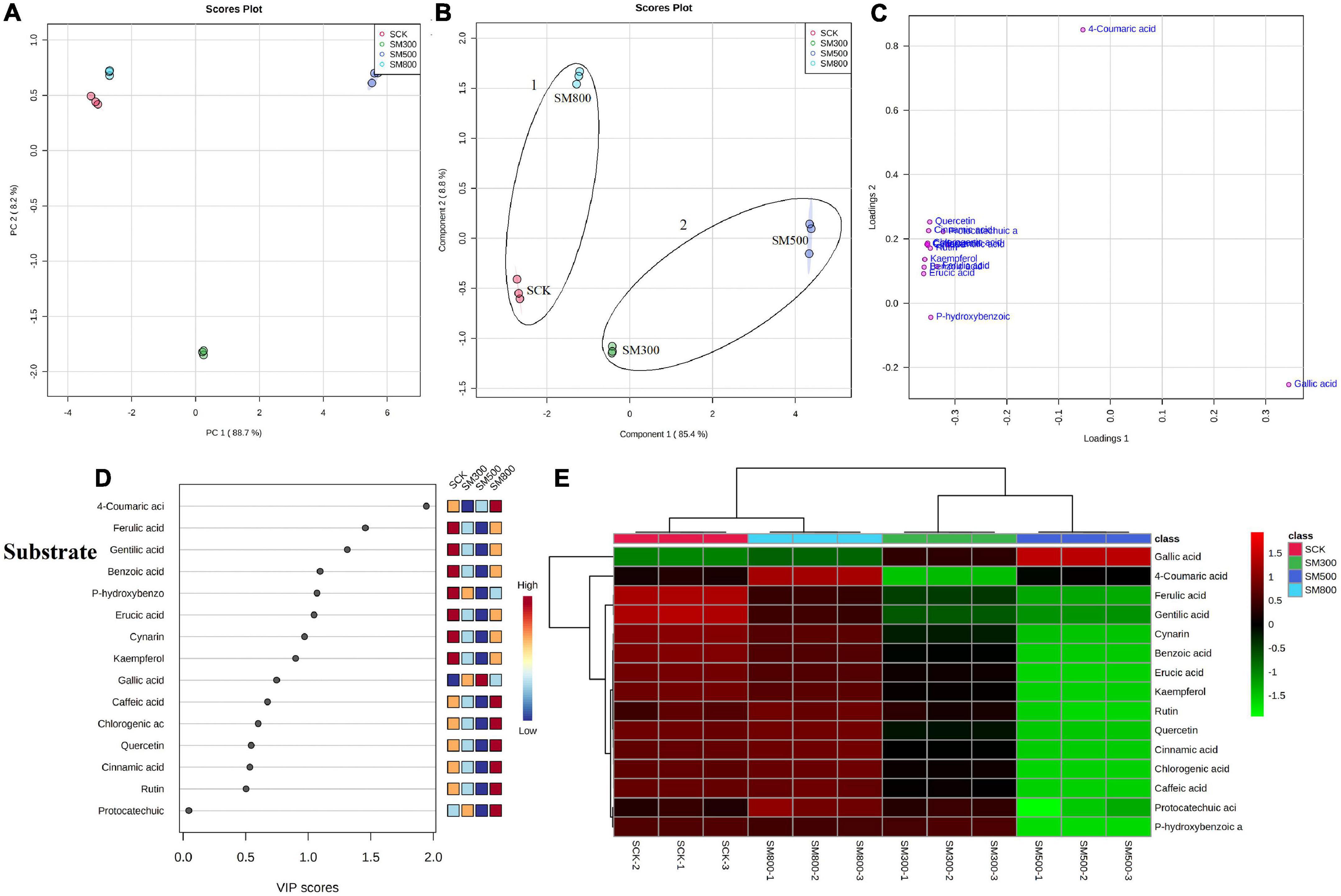
Figure 5. Statistical analyses of bioactive metabolites in substrate-grown Chinese chive treated with methyl jasmonate (MeJA). (A) An unsupervised principal component analysis (PCA) score plot of phenolic metabolites. (B) A partial least squares discriminant analysis (PLS-DA) score plot of phenolic metabolites. (C) PLS-DA score plots loaded with different phenolic metabolites. (D) VIP scores of phenolic metabolites in PLS-DA. The scores from low to high determine the importance of variables. The colored boxes on the right show the relative concentration of each metabolite from low (blue) to high (red). (E) Heat map of phenolic concentrations. The colored areas correspond to the concentrations of different amino acids from low (green) to high (red) in groups treated with different concentrations of MeJA. Each row represents a phenolic, and each column represents a treatment.
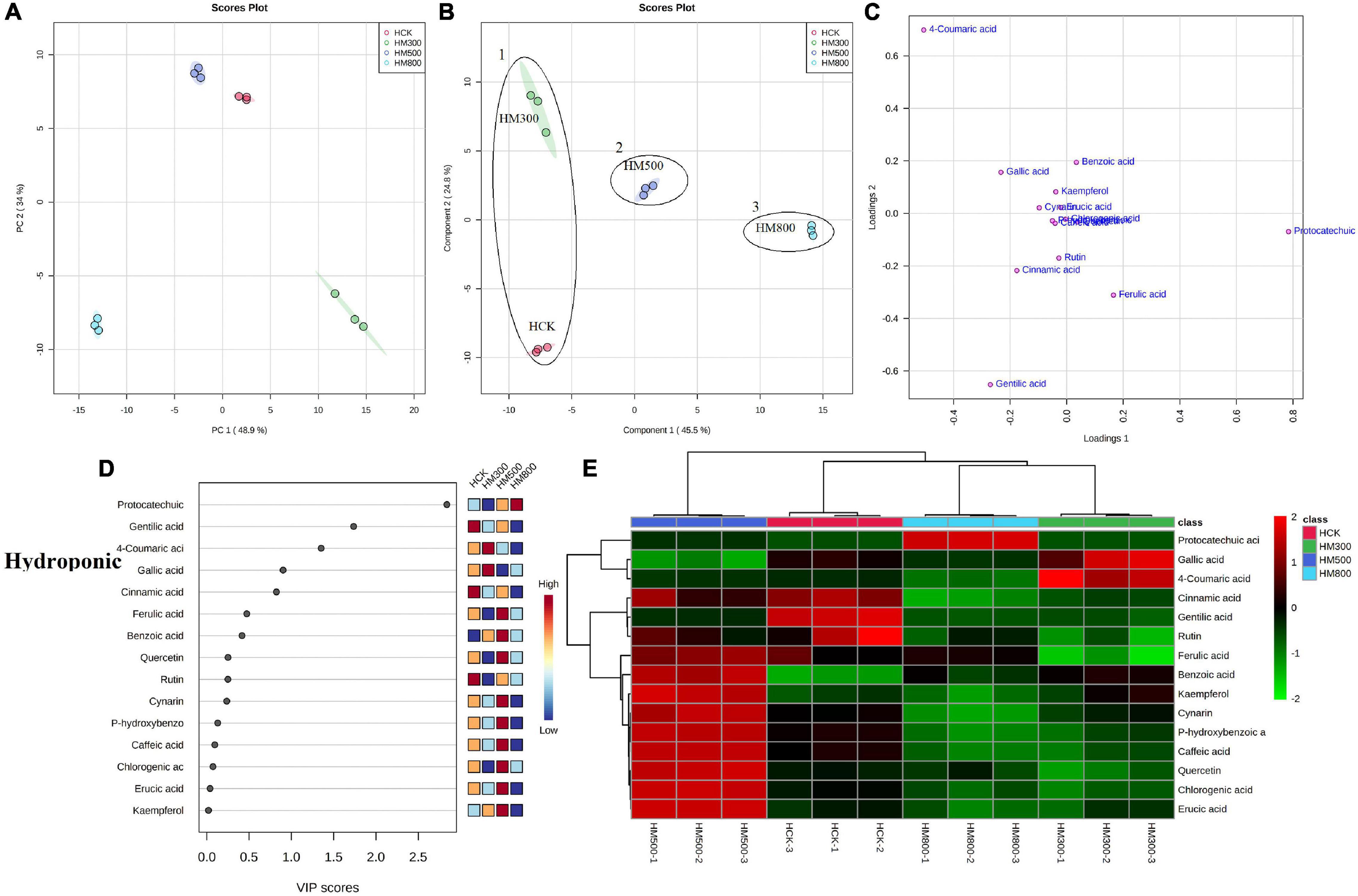
Figure 6. Statistical analyses of bioactive metabolites in hydroponic Chinese chive treated with methyl jasmonate (MeJA). (A) An unsupervised principal component analysis (PCA) score plot of phenolic metabolites. (B) A partial least squares discriminant analysis (PLS-DA) score plot of phenolic metabolites. (C) PLS-DA score plots loaded with different phenolic metabolites. (D) VIP scores of phenolic metabolites in PLS-DA. The scores from low to high determine the importance of variables. The colored boxes on the right show the relative concentration of each metabolite from low (blue) to high (red). (E) Heat map of phenolic concentrations. The colored areas correspond to the concentrations of different amino acids from low (green) to high (red) in groups treated with different concentrations of MeJA. Each row represents a phenolic, and each column represents a treatment.
Effect of the Methyl Jasmonate Treatments on Carotenoids (Secondary Metabolites) in Chinese Chive
Carotenoid is a lipophilic antioxidant and can detoxify various forms of reactive oxygen species (ROS) (80). The levels of different carotenoids in the substrate-grown and hydroponic Chinese chives treated with MeJA are listed in Table 6. β-carotene showed the highest levels (2027.16 and 1466.89 μg g–1) in the substrate-grown and hydroponic Chinese chives, followed by lycopene (223.74 and 121.46 μg g–1, respectively), lutein (159.21 and 196.54 μg g–1, respectively) and monocyclic zeaxanthin (77.43 and 63.58 μg g–1, respectively). Carotenoids include, β-carotene, α-carotene (81), and xanthophylls, such as zeaxanthin, neoxanthin, violaxanthin, lutein (82), and lycopene, which have antioxidants capacity (83, 84). The carotenoids in Chinese chives mainly included β-carotene. Lutein enhances the immune function and improves the antioxidant capacity of the body, protects vision, and reduces the severity of age-related macular degeneration (64, 85). The levels of most carotenoids, such as lutein, violaxanthin, zeaxanthin, and α-carotene, were significantly higher in the substrate-grown Chinese chives treated with MeJA (500 μM) than in the SCK group. Treatment with 800 μM MeJA significantly increased the total carotenoid content (37%), mainly because this concentration significantly increased the most abundant β-carotene in Chinese chives (48%). These results were similar to those of Pérez et al. (86). Previous studies have shown that β-carotene content is genotypically determined (64). However, we found that the β-carotene content in the substrate-grown Chinese chives was significantly higher than that in the hydroponic Chinese chives with or without MeJA treatment, suggesting that cultivation method is also a key factor in determining the β-carotene content in Chinese chives. A study on tomato fruits reported that JA treatment had a dose-dependent effect on carotenoid accumulation; that is, when the concentration of JA was > 0.5 μM, carotenoid biosynthesis was inhibited in tomato (31). In our study, hydroponic Chinese chives treated with MeJA (300 μM) showed significantly higher levels of zeaxanthin, α-carotene, and lutein compared to those in the HCK group. Treatment with 500 μM MeJA significantly increased the levels of violet xanthin. However, the carotenoid content in the hydroponic Chinese chives treated with MeJA (500 μM) showed an overall decreasing trend. In addition, the carotenoid content in the substrate-grown Chinese chives was higher than that in the hydroponic Chinese chives with or without MeJA treatment. This may have been because in leafy vegetables, carotenoid levels depend on various factors, such as cultivar species, cultivation method, maturity, and environmental conditions (87). Future studies should investigate the effect of different concentrations of MeJA on carotenoid biosynthesis to improve our understanding on the accumulation of carotenoids in hydroponic Chinese chives.
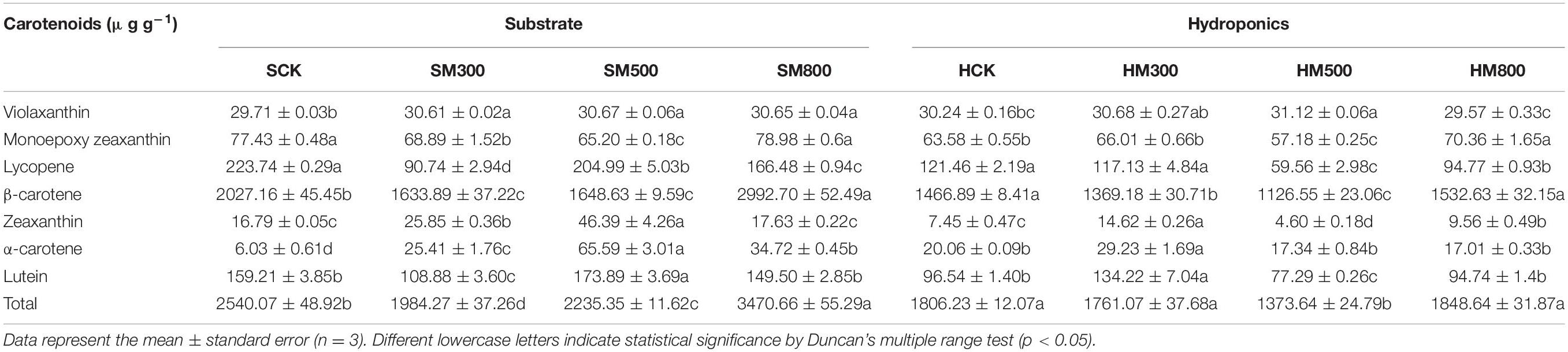
Table 6. Effect of MeJA treatments on the carotenoid contents of substrate-grown and hydroponic Chinese chive.
Effect of the Methyl Jasmonate Treatments on the Antioxidant Capacities of Chinese Chive
The antioxidant activities in the substrate-grown and hydroponic Chinese chives treated with MeJA are listed in Figures 7A–D. The ABTS (Figure 7B), FRAP (Figure 7C), and ORAC (Figure 7D) assays showed that the antioxidant activities of the substrate-grown and hydroponic Chinese chives treated with MeJA (500 μM) were significantly higher than those in the SCK and HCK control groups, respectively. Exogenous MeJA can increase the antioxidant activity of plants (88), which is consistent with our findings. The DPPH activities of hydroponic Chinese chives treated with MeJA and of substrate-grown Chinese chives treated with 300 μM MeJA were significantly higher than that of the HCK group (Figure 7A). Both phenolics and flavonoids are strongly associated with antioxidant activity (DPPH and ABTS) in Amaranthus hypochondriacus (89), A. tricolor (84), A. blitum (90), weedy species (91), stem amaranth (92), green morph amaranth (93), and red morph amaranth (94), which are corroborative to the present findings. Phenolic compounds are usually positively associated with the antioxidant activity of plant foods (95). In addition, polyphenols have an ideal chemical structure for scavenging free radicals, and higher levels of polyphenols in plants are associated with stronger free radical scavenging ability (96, 97). The correlation heat map further confirmed the relationship between antioxidant activity and phenolic content of the substrate-grown (Figure 7F) and hydroponic (Figure 7E) Chinese chives. The correlation coefficients indicated the strength of the correlation between the two, and we considered only strong correlations by setting the threshold value to 0.5. In the substrate-grown Chinese chives, we observed strong positive correlations between DPPH activity and the levels of rutin, eruic acid, and p-hydroxybenzoic acid; ABTS and the levels of rutin, protocatechuic acid, and 4-coumaric acid; FRAP and the levels of quercetin and gentilic acid; and ORAC and the levels of kaempferol, cinnamic acid, chlorogenic acid, and ferulic acid (Figure 7F). In the hydroponic Chinese chives, we found positive correlations between ABTS activity and the levels of quercetin, cynarin, caffeic acid, gentilic acid, and 4-coumaric acid; FRAP and the level of 4-coumaric acid; and ORAC and the levels of kaempferol, gallic acid, and chloro-genic acid (Figure 7E). This further indicated that an increase in the level of phenolic compounds might be associated with the improved antioxidant capacity of the substrate-grown and hydroponic Chinese chives following the MeJA treatment. Following the MeJA treatment, the ORAC of the substrate-grown and hydroponic Chinese chives increased in a dose-dependent manner. This was similar to the trend of changes observed in the levels of phenolic compounds after the MeJA treatment. In addition to phenolic compounds, sulfur-containing compounds (66), vitamin C (98), chlorophylls (99), carotenoids (100), and polysaccharides (101) also showed strong antioxidant activity.
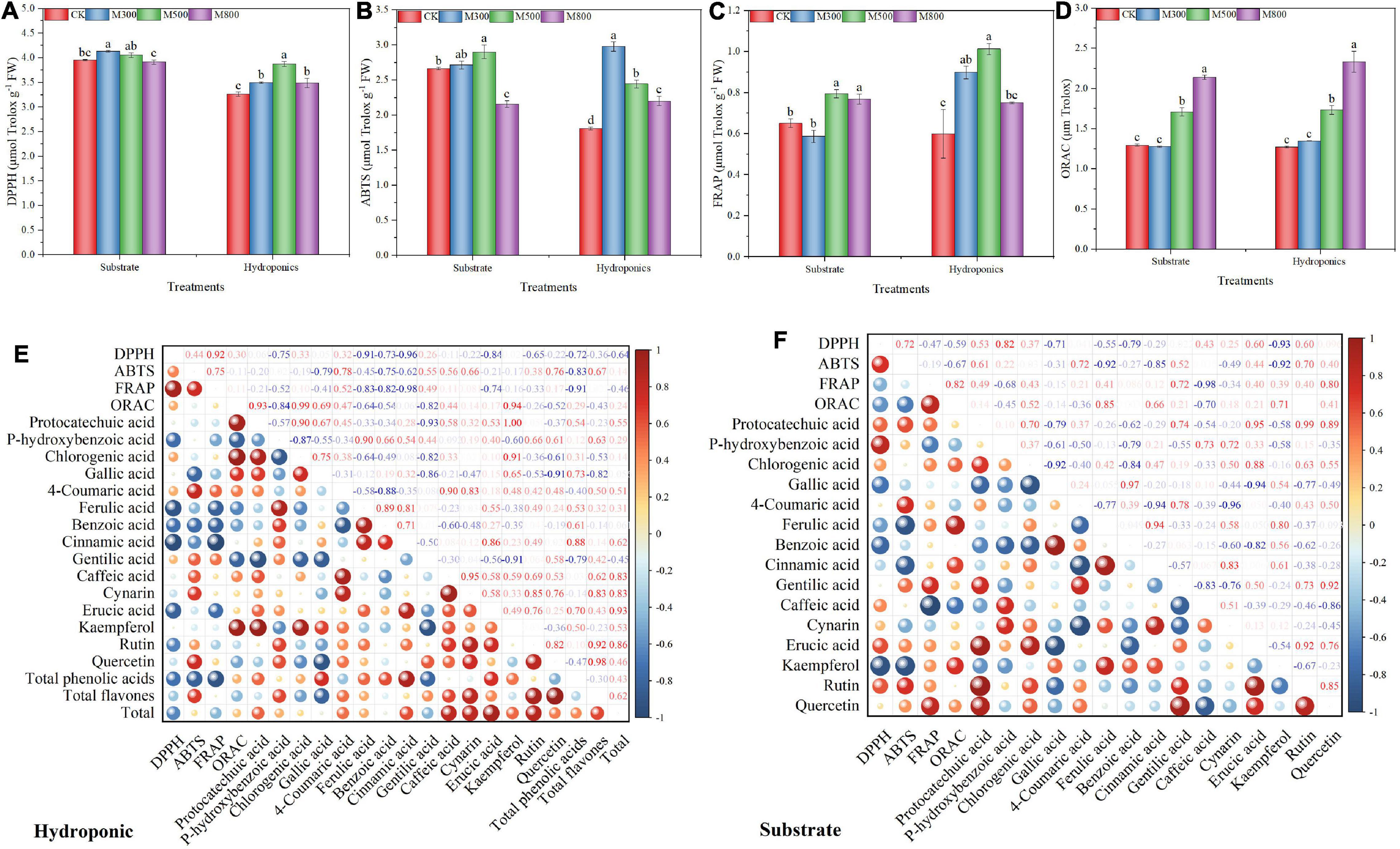
Figure 7. The 2,2-diphenyl-1-picrylhydrazyl (DPPH) radical scavenging capacity (A), Trolox-equivalent antioxidant capacity (ABTS) (B), ferric-reducing antioxidant power (FRAP) (C), and oxygen radical absorbance capacity (ORAC) (D) of Chinese chives grown in substrate or hydroponics as affected by methyl jasmonate (MeJA) treatments. Data represent the mean ± standard error (n = 3). Different lowercase letters indicate statistical significance, by Duncan’s multiple range tests (p < 0.05). The heat map shows the Pearson correlation analysis between the observed parameters of antioxidant capacity and the observed parameters of phenolic compounds in MeJA treated hydroponic (E) or substrate (F) Chinese chive. The values in the heat map are Pearson’s correlation coefficient. The size and color depth of the colored balls correspond to the strength of the correlation, ranging from weak (small balls, blue) to strong (large balls, red).
Conclusion
Our results showed that the MeJA-treated Chinese chives showed significant differences in primary and secondary metabolites depending on the cultivation method. After treating the Chinese chives with MeJA, we distinguished between substrate-grown and hydroponic Chinese chives using amino acids and phenolic compounds. The MeJA treatment significantly increased the phenolic content of the substrate-grown Chinese chives and decreased the organic acids content of the hydroponic Chinese chives. Treatment with MeJA (500 μM) significantly increased the total sugar and amino acid (essential and non-essential amino acids and sulfur-containing amino acids) contents of hydroponically grown Chinese chives. However, the total sugar and amino acid contents of the substrate-grown Chinese chives showed trends contrasting to those of hydroponic Chinese chives. In addition, treatment with 500 μM MeJA significantly increased the antioxidant activity of both substrate-grown and hydroponic Chinese chives. The nutritional quality and bioactive substances of Chinese chives were comprehensively analyzed using metabolomics. The results of metabolites under different cultivation methods and MeJA treatments can contribute to increasing the nutritional quality and medicinal value of substrate-grown and hydroponic Chinese chives, and to some extent in promoting the flavor quality of Chinese chives, and promoting Chinese chives as a value-added horticultural product.
Data Availability Statement
The original contributions presented in the study are included in the article/Supplementary Material, further inquiries can be directed to the corresponding author/s.
Author Contributions
CW, JZ, JX, and JY conceived and designed the experiments. CW, JZ, and YG analyzed the data. CW wrote the manuscript. CW, JZ, JLi, TN, and JLv involved in the related discussion. JX, JZ, and BP improved the quality of the manuscript. All authors have read and agreed to the published version of the manuscript.
Funding
This research was funded by the National Key Research and Development Program of China (2016YFD0201005), the Special Fund for Technical System of Melon and Vegetable Industry of Gansu Province (GARS-GC-1), and the Special Fund for Guiding Scientific and Technological Innovation and Development of Gansu Province (2018ZX-02), China.
Conflict of Interest
The authors declare that the research was conducted in the absence of any commercial or financial relationships that could be construed as a potential conflict of interest.
Publisher’s Note
All claims expressed in this article are solely those of the authors and do not necessarily represent those of their affiliated organizations, or those of the publisher, the editors and the reviewers. Any product that may be evaluated in this article, or claim that may be made by its manufacturer, is not guaranteed or endorsed by the publisher.
Supplementary Material
The Supplementary Material for this article can be found online at: https://www.frontiersin.org/articles/10.3389/fnut.2022.859035/full#supplementary-material
Footnotes
References
1. Nicastro HL, Ross SA, Milner JA. Garlic and onions: their cancer prevention properties. Cancer Prev Res. (2015) 8:181–9. doi: 10.1158/1940-6207.CAPR-14-0172
2. Zeng Y, Li Y, Yang J, Pu X, Du J, Yang X, et al. Therapeutic role of functional components in alliums for preventive chronic disease in human being. Evid Based Complement Alternat Med. (2017) 2017:9402849. doi: 10.1155/2017/9402849
3. Lanciotti L. The Book of Odes. Chinese Text, Transcription and Translation. New York, NY: JSTOR (1950).
4. Poojary MM, Putnik P, Kovačević DB, Barba FJ, Lorenzo JM, Dias DA, et al. Stability and extraction of bioactive sulfur compounds from Allium genus processed by traditional and innovative technologies. J Food Compost Anal. (2017) 61:28–39. doi: 10.1016/j.jfca.2017.04.007
5. Hu G, Lu Y, Wei D. Chemical characterization of Chinese chive seed (Allium tuberosum Rottl.). Food Chem. (2006) 99:693–7. doi: 10.1016/j.foodchem.2005.08.045
6. Zhang W-N, Zhang H-L, Lu C-Q, Luo J-P, Zha X-Q. A new kinetic model of ultrasound-assisted extraction of polysaccharides from Chinese chive. Food Chem. (2016) 212:274–81. doi: 10.1016/j.foodchem.2016.05.144
7. Hong J, Chen TT, Hu P, Yang J, Wang SY. Purification and characterization of an antioxidant peptide (GSQ) from Chinese leek (Allium tuberosum Rottler) seeds. J Funct Foods. (2014) 10:144–53. doi: 10.1016/j.jff.2014.05.014
8. Hur HJ, Lee AS. Protective effect of Allium tuberosum extract on vascular inflammation in tumor necrosis factor-α-induced human vascular endothelial cells. J Cancer Prev. (2017) 22:228. doi: 10.15430/JCP.2017.22.4.228
9. Liu N, Tong J, Hu M, Ji Y, Wu Z. Transcriptome landscapes of multiple tissues highlight the genes involved in the flavor metabolic pathway in Chinese chive (Allium tuberosum). Genomics. (2021) 113:2145–57. doi: 10.1016/j.ygeno.2021.05.005
10. Sarker U, Islam MT, Rabbani MG, Oba S. Genotypic diversity in vegetable amaranth for antioxidant, nutrient and agronomic traits. Indian J Genet Pl Breed. (2017) 77:173–6. doi: 10.5958/0975-6906.2017.00025.6
11. Sarker U, Oba S. Response of nutrients, minerals, antioxidant leaf pigments, vitamins, polyphenol, flavonoid and antioxidant activity in selected vegetable amaranth under four soil water content. Food Chem. (2018) 252:72–83. doi: 10.1016/j.foodchem.2018.01.097
12. Sarker U, Islam T, Rabbani G, Oba S. Genotype variability in composition of antioxidant vitamins and minerals in vegetable amaranth. Genetika. (2015) 47:85–96. doi: 10.2298/GENSR1803995C
13. Chakrabarty T, Sarker U, Hasan M, Rahman M. Variability in mineral compositions, yield and yield contributing traits of stem amaranth (Amaranthus lividus). Genetika. (2018) 50:995–1010. doi: 10.2298/GENSR1501085S
14. Sarker U, Islam MT, Rabbani MG, Oba S. Variability in total antioxidant capacity, antioxidant leaf pigments and foliage yield of vegetable amaranth. J Integr Agric. (2018) 17:1145–53. doi: 10.1016/S2095-3119(17)61778-7
15. Sarker U, Islam MT, Rabbani MG, Oba S. Phenotypic divergence in vegetable amaranth for total antioxidant capacity, antioxidant profile, dietary fiber, nutritional and agronomic traits. Acta Agric Scand. (2018) 68:67–76. doi: 10.1080/09064710.2017.1367029
16. Sarker U, Oba S. Salinity stress enhances color parameters, bioactive leaf pigments, vitamins, polyphenols, flavonoids and antioxidant activity in selected Amaranthus leafy vegetables. J Sci Food Agric. (2019) 99:2275–84. doi: 10.1002/jsfa.9423
17. Sarker U, Islam MT, Rabbani MG, Oba S. Genotypic variability for nutrient, antioxidant, yield and yield contributing traits in vegetable amaranth. J Food Agri Environ. (2014) 12:168–74. doi: 10.1234/4.2014.5378
18. Sarker U, Islam T, Rabbani G, Oba S. Antioxidant leaf pigments and variability in vegetable amaranth. Genetika. (2018) 50:209–20. doi: 10.2298/GENSR1801209S
19. Sarker U, Islam MS, Rabbani MG, Oba S. Variability, heritability and genetic association in vegetable amaranth (Amaranthus tricolor L.). Spanish J Agric Res. (2015) 13:17. doi: 10.5424/sjar/2015132-6843
20. Keleş D, Özgen Ş, Saraçoğlu O, Ata A, Özgen M. Antioxidant potential of Turkish pepper (Capsicum annuum L.) genotypes at two different maturity stages. Turk J Agric For. (2016) 40:542–51. doi: 10.3906/tar-1601-24
21. Pauwels L, Inzé D, Goossens A. Jasmonate-inducible gene: what does it mean? Trends Plant Sci. (2009) 14:87–91. doi: 10.1016/j.tplants.2008.11.005
22. Jang G, Shim JS, Jung C, Song JT, Lee HY, Chung PJ, et al. Volatile methyl jasmonate is a transmissible form of jasmonate and its biosynthesis is involved in systemic jasmonate response in wounding. Plant Biotechnol Rep. (2014) 8:409–19. doi: 10.1007/s11816-014-0331-6
23. Bradbury S. Methyl jasmonate; exemption from the requirement of a tolerance. federal register. a rule by the environmental protection agency (EPA). Fed Regist. (2013) 78:13–9.
24. Baenas N, García-Viguera C, Moreno DA. Elicitation: a tool for enriching the bioactive composition of foods. Molecules. (2014) 19:13541–63. doi: 10.3390/molecules190913541
25. Koo AJ, Gao X, Daniel Jones A, Howe GA. A rapid wound signal activates the systemic synthesis of bioactive jasmonates in Arabidopsis. Plant J. (2009) 59:974–86. doi: 10.1111/j.1365-313x.2009.03924.x
26. Kim HS. Functional Studies of Lignin Biosynthesis Genes and Putative Flowering Gene in Miscanthus x giganteus and Studies on Indolyl Glucosinolate Biosynthesis and Translocation in Brassica oleracea. Champaign, IL: University of Illinois at Urbana-Champaign (2010).
27. Baenas N, García-Viguera C, Moreno DA. Biotic elicitors effectively increase the glucosinolates content in brassicaceae sprouts. J Agric Food Chem. (2014) 62:1881–9. doi: 10.1021/jf404876z
28. Cheong J-J, Do Choi Y. Methyl jasmonate as a vital substance in plants. TRENDS Genetics. (2003) 19:409–13. doi: 10.1016/S0168-9525(03)00138-0
29. Sun G, Yang Y, Xie F, Wen J-F, Wu J, Wilson IW, et al. Deep sequencing reveals transcriptome re-programming of Taxus× media cells to the elicitation with methyl jasmonate. PLoS One. (2013) 8:e62865. doi: 10.1371/journal.pone.0062865
30. Fan X, Mattheis JP, Fellman JK. A role for jasmonates in climacteric fruit ripening. Planta. (1998) 204:444–9. doi: 10.1007/s004250050278
31. Liu L, Wei J, Zhang M, Zhang L, Li C, Wang Q. Ethylene independent induction of lycopene biosynthesis in tomato fruits by jasmonates. J Exp Bot. (2012) 63:5751–61. doi: 10.1093/jxb/ers224
32. Shafiq M, Singh Z, Khan AS. Time of methyl jasmonate application influences the development of ‘Cripps Pink’apple fruit colour. J Sci Food Agric. (2013) 93:611–8. doi: 10.1002/jsfa.5851
33. Weng C-J, Yen G-C. Chemopreventive effects of dietary phytochemicals against cancer invasion and metastasis: phenolic acids, monophenol, polyphenol, and their derivatives. Cancer Treat Rev. (2012) 38:76–87. doi: 10.1016/j.ctrv.2011.03.001
34. Sayyari M, Babalar M, Kalantari S, Martinez-Romero D, Guillen F, Serrano M, et al. Vapour treatments with methyl salicylate or methyl jasmonate alleviated chilling injury and enhanced antioxidant potential during postharvest storage of pomegranates. Food Chem. (2011) 124:964–70. doi: 10.1016/j.foodchem.2010.07.036
35. Wang SY, Bowman L, Ding M. Methyl jasmonate enhances antioxidant activity and flavonoid content in blackberries (Rubus sp.) and promotes antiproliferation of human cancer cells. Food Chem. (2008) 107:1261–9. doi: 10.1016/j.foodchem.2007.09.065
36. Palermo M, Paradiso R, De Pascale S, Fogliano V. Hydroponic cultivation improves the nutritional quality of soybean and its products. J Agric Food Chem. (2012) 60:250–5. doi: 10.1021/jf203275m
37. Miyagi A, Uchimiya H, Kawai-Yamada M. Synergistic effects of light quality, carbon dioxide and nutrients on metabolite compositions of head lettuce under artificial growth conditions mimicking a plant factory. Food Chem. (2017) 218:561–8. doi: 10.1016/j.foodchem.2016.09.102
38. Wu ZH, Maruo T, Shinohara Y. Effect of total nitrogen concentration of nutrient solution in DFT system on the initial growth and nutrient uptake of Chinese chive (Allium tuberosum Rottler ex Spreng.). J Jpn Soc Hortic Sci. (2008) 77:173–9. doi: 10.2503/jjshs1.77.173
39. Wei X, Wei Y, Liu L, Wang X, Yang Y, Sun B, et al. Determination of sugar and acid components and contents in fruits of 4 blueberry varieties by high performance liquid chromatography. Chin Fruit Trees. (2013) 3:64–7. doi: 10.16626/j.cnki.issn1000-8047.2013.03.029
40. Coelho EM, da Silva Padilha CV, Miskinis GA, de Sá AGB, Pereira GE, de Azevêdo LC, et al. Simultaneous analysis of sugars and organic acids in wine and grape juices by HPLC: method validation and characterization of products from northeast Brazil. J Food Compost Anal. (2018) 66:160–7. doi: 10.1016/j.jfca.2017.12.017
41. Kennedy A, Bivens A. Method for analyzing underivatized amino acids using liquid mass spectrometry system. Agilent Technol Appl Note. (2017) 12:1–8.
42. Liu F. Optimization of Detection Methods for Polyphenols in Tomato Fruit and Comprehensive Evaluation of Quality. Lanzhou: Gansu Agricultural University (2021).
43. Hand MJ, Taffouo VD, Nouck AE, Nyemene K, Brice T, Meguekam TL, et al. Effects of salt stress on plant growth, nutrient partitioning, chlorophyll content, leaf relative water content, accumulation of osmolytes and antioxidant compounds in pepper (Capsicum annuum L.) cultivars. Not Bot Horti Agrobot Cluj Napoca. (2017) 45:481–90. doi: 10.15835/nbha45210928
44. Li J, Xie J, Yu J, Lv J, Zhang J, Wang X, et al. Reversed-phase high-performance liquid chromatography for the quantification and optimization for extracting 10 kinds of carotenoids in pepper (Capsicum annuum L.) leaves. J Agric Food Chem. (2017) 65:8475–88. doi: 10.1021/acs.jafc.7b02440
45. Tytgat TOG, Verhoeven KJF, Jansen JJ, Raaijmakers CE, Bakx-Schotman T, McIntyre LM, et al. Plants know where it hurts: root and shoot jasmonic acid induction elicit differential responses in Brassica olracea. PLoS One. (2013) 8:14. doi: 10.1371/journal.pone.0065502
46. Liang Y-S, Choi YH, Kim HK, Linthorst HJM, Verpoorte R. Metabolomic analysis of methyl jasmonate treated Brassica rapa leaves by 2-dimensional NMR spectroscopy. Phytochemistry. (2006) 67:2503–11. doi: 10.1016/j.phytochem.2006.08.018
47. Wang D. Development and application progress of citric acid (sodium) chemical intermediates: technology. Industry Ed. (2004) 1:7.
48. Kim MJ, Chiu YC, Kim NK, Park HM, Lee CH, Juvik JA, et al. Cultivar-specific changes in primary and secondary metabolites in pak choi (Brassica Rapa, Chinensis Group) by methyl jasmonate. Int J Mol Sci. (2017) 18:17. doi: 10.3390/ijms18051004
49. Wang R. Research progress on production and application of L-malic acid. Chem Times. (2002) 5:1–7. Doi: CNKI:SUN:HGJS.0.2002-05-000
50. Zhang Y, Lin X, Zhang Y, Zheng SJ, Du S. Effects of nitrogen levels and nitrate/ammonium ratios on oxalate concentrations of different forms in edible parts of spinach. J Plant Nutr. (2005) 28:2011–25. doi: 10.1080/01904160500311086
51. Bohn T, Davidsson L, Walczyk T, Hurrell RF. Fractional magnesium absorption is significantly lower in human subjects from a meal served with an oxalate-rich vegetable, spinach, as compared with a meal served with kale, a vegetable with a low oxalate content. Br J Nutr. (2004) 91:601–6. doi: 10.1079/BJN20031081
52. Jiang J. Eliminating oxalate from vegetables is beneficial for calcium absorption. Fam Med. (2009) 5:1.
53. Wu G. Functional amino acids in growth, reproduction, and health. Adv Nutr. (2010) 1:31–7. doi: 10.3945/an.110.1008
54. Prasanna V, Prabha T, Tharanathan R. Fruit ripening phenomena–an overview. Crit Rev Food Sci Nutr. (2007) 47:1–19. doi: 10.1080/10408390600976841
55. Wang X, Zeng L, Liao Y, Zhou Y, Xu X, Dong F, et al. An alternative pathway for the formation of aromatic aroma compounds derived from L-phenylalanine via phenylpyruvic acid in tea (Camellia sinensis (L.) O. Kuntze) leaves. Food Chem. (2019) 270:17–24. doi: 10.1016/j.foodchem.2018.07.056
56. Rivero Meza SL, de Castro Tobaruela E, Benedetti Pascoal G, Louro Massaretto I, Purgatto E. Post-harvest treatment with methyl jasmonate impacts lipid metabolism in tomato pericarp (Solanum lycopersicum L. cv. Grape) at different ripening stages. Foods. (2021) 10:877. doi: 10.3390/foods10040877
57. Deng Y, Luo YL, Wang YG, Zhao YY. Effect of different drying methods on the myosin structure, amino acid composition, protein digestibility and volatile profile of squid fillets. Food Chem. (2015) 171:168–76. doi: 10.1016/j.foodchem.2014.09.002
58. Ye Y, Bie Z, Tang L, Tian Y. Study on pretreatment conditions for determination of sulfur-containing amino acids in food. Food and Ferment Industry. (2021) 47:236–42. doi: 10.13995/j.cnki.11-1802/ts.025336
59. Wall MM, Corgan JN. Relationship between pyruvate analysis and flavor perception for onion pungency determination. HortScience. (1992) 27:1029–30. doi: 10.21273/HORTSCI.27.9.1029
60. Yabuki Y, Mukaida Y, Saito Y, Oshima K, Takahashi T, Muroi E, et al. Characterisation of volatile sulphur-containing compounds generated in crushed leaves of Chinese chive (Allium tuberosum Rottler). Food Chem. (2010) 120:343–8. doi: 10.1016/j.foodchem.2009.11.028
61. Heng H, Ong XL, Chow P. Antioxidant action and effectiveness of sulfur-containing amino acid during deep frying. J Food Sci Technol. (2020) 57:1150–7. doi: 10.1007/s13197-019-04150-5
62. Lee LC, Liong C-Y, Jemain AA. Partial least squares-discriminant analysis (PLS-DA) for classification of high-dimensional (HD) data: a review of contemporary practice strategies and knowledge gaps. Analyst. (2018) 143:3526–39. doi: 10.1039/x0xx00000x
63. Carvalho FV, Santana LF, da Silva VDA, Costa SL, Zambotti-Villelae L, Colepicolo P, et al. Combination of a multiplatform metabolite profiling approach and chemometrics as a powerful strategy to identify bioactive metabolites in Lepidium meyenii (Peruvian maca). Food Chem. (2021) 364:130453. doi: 10.1016/j.foodchem.2021.130453
64. Phahlane CJ, Laurie SM, Shoko T, Manhivi VE, Sivakumar D. An evaluation of phenolic compounds, carotenoids, and antioxidant properties in leaves of South African cultivars, Peruvian 199062.1 and USA’s beauregard. Front Nutr. (2021) 8:773550. doi: 10.3389/fnut.2021.773550
65. Xia J, Psychogios N, Young N, Wishart DS. MetaboAnalyst: a web server for metabolomic data analysis and interpretation. Nucleic Acids Res. (2009) 37:W652–60. doi: 10.1093/nar/gkp356
66. Wang C, Lv J, Xie J, Yu J, Li J, Zhang J, et al. Effects of preharvest methyl jasmonate and salicylic acid treatments on the growth, quality, volatile components and antioxidant systems of Chinese chives. Front Plant Sci. (2021) 12:767335. doi: 10.3389/fpls.2021.767335
67. Jung J-K, Lee S-U, Kozukue N, Levin CE, Friedman M. Distribution of phenolic compounds and antioxidative activities in parts of sweet potato (Ipomoea batata L.) plants and in home processed roots. J Food Compos Anal. (2011) 24:29–37. doi: 10.1016/j.jfca.2010.03.025
68. Sarker U, Oba S. Phenolic profiles and antioxidant activities in selected drought-tolerant leafy vegetable amaranth. Sci Rep. (2020) 10:18287. doi: 10.1038/s41598-020-71727-y
69. Sarker U, Oba S. Drought stress enhances nutritional and bioactive compounds, phenolic acids and antioxidant capacity of Amaranthus leafy vegetable. BMC Plant Biol. (2018) 18:258. doi: 10.1186/s12870-018-1484-1
70. Sarker U, Oba S. Antioxidant constituents of three selected red and green color Amaranthus leafy vegetable. Sci Rep. (2019) 9:1–11. doi: 10.1038/s41598-019-52033-8
71. Sarker U, Oba S. Polyphenol and flavonoid profiles and radical scavenging activity in leafy vegetable Amaranthus gangeticus. BMC Plant Biol. (2020) 20:499. doi: 10.1186/s12870-020-02700-0
72. Sarker U, Oba S. Nutraceuticals, phytochemicals, and radical quenching ability of selected drought-tolerant advance lines of vegetable amaranth. BMC Plant Biol. (2020) 20:564. doi: 10.1186/s12870-020-02780-y
73. Sarker U, Hossain MN, Iqbal MA, Oba S. Bioactive components and radical scavenging activity in selected advance lines of salt-tolerant vegetable amaranth. Front Nutr. (2020) 7:587257. doi: 10.3389/fnut.2020.587257
74. Ghasemzadeh A, Jaafar HZ, Rahmat A. Antioxidant activities, total phenolics and flavonoids content in two varieties of Malaysia young ginger (Zingiber officinale Roscoe). Molecules. (2010) 15:4324–33. doi: 10.3390/molecules15064324
75. Zhang R, Zhang B-L, He T, Yi T, Yang J-P, He B. Increase of rutin antioxidant activity by generating maillard reaction products with lysine. Bioorg Med Chem Lett. (2016) 26:2680–4. doi: 10.1016/j.bmcl.2016.04.008
76. Baek MW, Choi HR, Solomon T, Jeong CS, Lee O-H, Tilahun S. Preharvest methyl jasmonate treatment increased the antioxidant activity and glucosinolate contents of hydroponically grown pak choi. Antioxidants. (2021) 10:131. doi: 10.3390/antiox10010131
77. Srinivasan M, Sudheer AR, Menon VP. Recent advances in Indian herbal drug researchguest editor: thomas paul asir devasagayamferulic acid: therapeutic potential through its antioxidant property. J Bioorg Med Chem Lett Clin Biochem Nutr. (2007) 40:92–100. doi: 10.3164/jcbn.40.92
78. Kerry NL, Abbey M. Red wine and fractionated phenolic compounds prepared from red wine inhibit low density lipoprotein oxidation in vitro. Atherosclerosis. (1997) 135:93–102. doi: 10.1016/S0021-9150(97)00156-1
79. Sarker U, Oba S. Augmentation of leaf color parameters, pigments, vitamins, phenolic acids, flavonoids and antioxidant activity in selected Amaranthus tricolor under salinity stress. Sci Rep. (2018) 8:12349. doi: 10.1038/s41598-018-30897-6
80. Sarker U, Oba S. Catalase, superoxide dismutase and ascorbate-glutathione cycle enzymes confer drought tolerance of Amaranthus tricolor. Sci Rep. (2018) 8:1–12. doi: 10.1038/s41598-018-34944-0
81. Sarker U, Oba S. Color attributes, betacyanin, and carotenoid profiles, bioactive components, and radical quenching capacity in selected Amaranthus gangeticus leafy vegetables. Sci Rep. (2021) 11:1–14. doi: 10.1038/s41598-021-91157-8
82. Sarker U, Oba S. Leaf pigmentation, its profiles and radical scavenging activity in selected Amaranthus tricolor leafy vegetables. Sci Rep. (2020) 10:1–10. doi: 10.1038/s41598-020-66376-0
83. Sarker U, Oba S. Drought Stress Effects on Growth, ROS Markers, Compatible Solutes, Phenolics, Flavonoids, and Antioxidant Activity in Amaranthus tricolor. Appl Biochem Biotechnol. (2018) 186:999–1016. doi: 10.3389/fpls.2020.559876
84. Sarker U, Oba S. The response of salinity stress– induced a. tricolor to growth, anatomy, physiology, non-enzymatic and enzymatic antioxidants. Front Plant Sci. (2020) 11:559876. doi: 10.1007/s12010-018-2784-5
85. Rohini V, Goodrow-Kotyla EF, Wooten BR, Wilson TA, Nicolosi RJ. Consumption of 2 and 4 egg yolks/d for 5 wk increases macular pigment concentrations in older adults with low macular pigment taking cholesterol-lowering statins. Am J Clin Nutr. (2009) 90:1272–9. doi: 10.3945/ajcn.2009.28013
86. Pérez AG, Sanz C, Richardson DG, Olías JM. Methyl jasmonate vapor promotes β-carotene synthesis and chlorophyll degradation in golden delicious apple peel. J Plant Growth Regulator. (1993) 12:163–7. doi: 10.1007/BF00189648
87. Nyathi MK, Du Plooy CP, Van Halsema GE, Stomph TJ, Annandale JG, Struik PC. The dual-purpose use of orange-fleshed sweet potato (Ipomoea batatas var. Bophelo) for improved nutritional food security. Agric Water Manag. (2019) 217:23–37. doi: 10.1016/j.agwat.2019.02.029
88. Szymanowska U, Złotek U, Karaś M, Baraniak B. Anti-inflammatory and antioxidative activity of anthocyanins from purple basil leaves induced by selected abiotic elicitors. Food Chem. (2015) 172:71–7. doi: 10.1016/j.foodchem.2014.09.043
89. Sarker U, Oba S. Nutritional and bioactive constituents and scavenging capacity of radicals in Amaranthus hypochondriacus. Sci Rep. (2020) 10:1–10. doi: 10.1038/s41598-020-71714-3
90. Sarker U, Oba S. Nutrients, minerals, pigments, phytochemical, and radical scavenging activity in Amaranthus blitum leafy vegetable. Sci Rep. (2020) 10:3868. doi: 10.1038/s41598-020-59848-w
91. Sarker U, Oba S. Nutraceuticals, antioxidant pigments, and phytochemicals in the leaves of Amaranthus spinosus and Amaranthus viridis weedy species. Sci Rep. (2019) 9:20413. doi: 10.1038/s41598-019-50977-5
92. Sarker U, Oba S, Daramy M. Nutrients, minerals, antioxidant pigments and phytochemicals, and antioxidant capacity of the leaves of stem amaranth. Sci Rep. (2020) 10:3892. doi: 10.1038/s41598-020-60252-7
93. Sarker U, Hossain MM, Oba S. Nutritional and antioxidant components and antioxidant capacity in green morph Amaranthus leafy vegetable. Sci Rep. (2020) 10:1–10. doi: 10.1038/s41598-020-57687-3
94. Sarker U, Oba S. Protein, dietary fiber, minerals, antioxidant pigments and phytochemicals, and antioxidant activity in selected red morph Amaranthus leafy vegetable. PLoS One. (2019) 14:e0222517. doi: 10.1371/journal.pone.0222517
95. Duan S-C, Kwon S-J, Eom S-H. Effect of thermal processing on color, phenolic compounds, and antioxidant activity of Faba bean (Vicia faba L.) leaves and seeds. Antioxidants. (2021) 10:1207. doi: 10.3390/antiox10081207
96. Sokół-Łętowska A, Oszmiański J, Wojdyło A. Antioxidant activity of the phenolic compounds of hawthorn, pine and skullcap. Food Chem. (2007) 103:853–9. doi: 10.1016/j.foodchem.2006.09.036
97. Ghasemzadeh A, Omidvar V, Jaafar HZ. Polyphenolic content and their antioxidant activity in leaf extract of sweet potato (Ipomoea batatas). J Med Plants Res. (2012) 6:2971–6. doi: 10.5897/JMPR11.135396
98. Podsêdek A. Natural antioxidants and antioxidant capacity of Brassica vegetables: a review. LWT Food Sci Technol. (2007) 40:1–11. doi: 10.1016/j.lwt.2005.07.023
99. Lanfer-Marquez UM, Barros RM, Sinnecker P. Antioxidant activity of chlorophylls and their derivatives. Food Res Int. (2005) 38:885–91. doi: 10.1016/j.foodres.2005.02.012
100. Londhe JS, Devasagayam TP, Foo LY, Ghaskadbi SS. Antioxidant activity of some polyphenol constituents of the medicinal plant Phyllanthus amarus Linn. Redox Rep. (2008) 13:199–207. doi: 10.1179/135100008X308984
Keywords: hydroponic agriculture, methyl jasmonate, antioxidant capacity, plant metabolites, Chinese chive, Allium tuberosum, metabolic profile
Citation: Wang C, Zhang J, Lv J, Li J, Gao Y, Patience BE, Niu T, Yu J and Xie J (2022) Effect of Methyl Jasmonate Treatment on Primary and Secondary Metabolites and Antioxidant Capacity of the Substrate and Hydroponically Grown Chinese Chives. Front. Nutr. 9:859035. doi: 10.3389/fnut.2022.859035
Received: 20 January 2022; Accepted: 09 March 2022;
Published: 05 April 2022.
Edited by:
Dharini Sivakumar, Tshwane University of Technology, South AfricaReviewed by:
Umakanta Sarker, Bangabandhu Sheikh Mujibur Rahman Agricultural University, BangladeshMartin Maboko, Tshwane University of Technology, South Africa
Copyright © 2022 Wang, Zhang, Lv, Li, Gao, Patience, Niu, Yu and Xie. This is an open-access article distributed under the terms of the Creative Commons Attribution License (CC BY). The use, distribution or reproduction in other forums is permitted, provided the original author(s) and the copyright owner(s) are credited and that the original publication in this journal is cited, in accordance with accepted academic practice. No use, distribution or reproduction is permitted which does not comply with these terms.
*Correspondence: Jianming Xie, eGllamlhbm1pbmdAZ3NhdS5lZHUuY24=
†These authors have contributed equally to this work and share first authorship