- 1Clinical Laboratory Sciences Department, College of Applied Medical Sciences, Taif University, Taif, Saudi Arabia
- 2High Altitude Research Center, Prince Sultan Medical Complex, Al-Hawiyah, Taif University, Taif, Saudi Arabia
- 3Radiological Sciences Department, College of Applied Medical Sciences, Taif University, Taif, Saudi Arabia
- 4Medical Biochemistry Department, National Research Centre, Cairo, Egypt
- 5Cancer Biology and Genetics Laboratory, Centre of Excellence for Advanced Sciences, National Research Centre, Cairo, Egypt
- 6Department of Pharmacology and Toxicology, Faculty of Pharmacy, Helwan University, Cairo, Egypt
Sargassum dentifolium, (Turner) C. Agarth, 1820, is an edible brown alga collected from red seashores, Egypt. Oral tongue squamous cell carcinoma (OTSCC) is an aggressive malignancy. Hypoxia leads to chemotherapeutic resistance. This work aimed to explore the anti-hypoxia effect of water-soluble polysaccharide fractions of S. dentifolium (SD1–SD3) in CAL-27 OTSCC cells. Cell cytotoxicity assay (MTT); cell death mode (DNA staining); total hypoxia (pimonidazole), HIF-1α (ELISA and immunocytochemistry), HIF-1β (ELISA), and hsa-miRNA-21-5p and hsa-miRNA-210-3p (qRT-PCR) were investigated. SD1 and SD2 showed a cytotoxic effect due to apoptosis. SD2 and SD3 decreased total cell hypoxia, inhibited miR-210 (p < 0.001 and p < 0.01), miR-21 (p < 0.01 and p < 0.05), and HIF-1α (p < 0.01 and p < 0.05), respectively. However, only SD3 suppressed HIF-1β (p < 0.05). In conclusion, SD2 showed a potential anti-hypoxia effect through amelioration of HIF-1α regulators, which may help in decreasing hypoxia-induced therapeutic resistance.
Introduction
Among brown algae, Sargassum is a common tropical/subtropical family that included 150 species (1). A battery of edible Sargassum algae that provide a rich source of biologically active polysaccharides, such as Sargassum stenophyllum, Sargassum latifolium, Sargassum fulvellum, and others [reviewed in (2)]. For example, polysaccharide extracts from Sargassum asperifolium polysaccharide extracts have been reported to act as tumor anti-initiation activity as well as anti-promotion property via their anti-inflammatory activity and a specific anti-progression activity against HepG2 (3). Similarly, the Gamal-Eldeen group (4) reported that S. latifolium polysaccharide extracts exhibit promising cancer chemopreventive activity as tumor anti-initiating and anti-promoting agents with specific anti-progression activity against leukemia. Additionally, Sargassum wightii polysaccharide extract has been proved to have anti-inflammatory activity (1), antimetastatic activity (5) and a preventive property against oxidative liver injury (6). Moreover, Sargassum duplicatum and S. ilicifolium in a mixture with other plants have been reported to enhance wound healing in skin (7).
Oral tongue squamous cell carcinoma (OTSCC), as one of the widespread oral cavity malignancies, is a strongly aggressive neoplasm that shows a fast local invasion/spread (8) as a result of a high growth rate and metastasis (9). Nearly 50% of patients have been lately diagnosed in Stages III and IV in the initial diagnostic examination (10). OTSCC has a significant elevated incidence in young to middle-age populations (8), with a poor prognosis, characteristic aggressiveness, and high mortality (19% of patients) (11). OTSCC often leads to functional problems in deglutition, mastication, and speech (12). Although chemotherapy may diminish tumor size and metastasis in OTSCC (12), still, the 5-year survival rate is ∼50% (13).
Hypoxia plays a serious role in the pathophysiology of several human disorders, such as cancer chronic lung disease and ischemic cardiovascular disease (14). In tumor microenvironment (TME), uncontrolled rapid proliferation restricts the oxygen availability (hypoxia) in all solid tumors, where a drop in the normal oxygen (2–9%) down to the hypoxic level <2% occurs (15). The adaption of tumor cells to hypoxia resulted in far aggressive and therapeutically resistant phenotypes. Hypoxia stimulates gene expression alterations followed by consequent proteomic changes that affect cellular and physiological functions (15). Among these changes, cells in TME hypoxic regions divide slowly and escape cytotoxic drugs, which are regularly targeting the rapidly dividing cells (16). In TME, hypoxia produces oxygen gradients that participate in the heterogeneity and plasticity of tumors and evoke aggressive and metastatic phenotypes. During hypoxia, the principal event is the stimulated expression of hypoxia-inducible factor-1α (HIF-1α) that implements a functional role in the cellular consequences prompted in response to hypoxia (17, 18).
Herbal nutraceuticals are functional phytochemicals derived from plants and algae (19). They are nontoxic edible supplements that exhibit broad-spectrum medicinal properties and provide efficient protection against many diseases including cancer (20). Growing studies demonstrate that herbal nutraceuticals could act as safe and effective agents against hypoxic cancer cells via successfully attenuating their growth, survival, and progression through the inhibition of HIF-1-signaling pathways (20). Recently, many hypoxia-inhibition strategies have been used as a therapeutic approach to treat cancer through regulating/targeting of HIF-1α to overcome the cell resistance due to hypoxia in solid tumors and through suppressing the hypoxia-stimulated resistance to chemotherapies (21). Among these strategies, using algal and herbal nutraceuticals for targeting downstream HIF-signaling pathways has been done through different approaches, including direct inhibition of HIFs using anticancer agents, blocking the dimerization of HIF-1α and β subunits, and silencing by HIFs-siRNA. Many of herbal and marine agents inhibit the hypoxia-associated resistance to chemotherapies by provoking the activation of an HIF-1α degradation cascade and diminishing the overexpressed HIF-1α in hypoxic tumors [reviewed in (20, 22)]. Extracts of marine algae and sponges from the NCI Open Repository have shown substantial activities as inhibitors for the HIF-1 activation in cell-based assays (23), for example, the red alga Laurencia intricata product inhibited HIF-1 activation (24).
In our recent work, we have reported the mechanistic anti-hypoxic role of the S. latifolium extract in HCT-116 cells, where the extract has been found to inhibit the hypoxia regulators miRNA-21 and miRNA-210, and consequently suppress HIF-1α and HIF-1β (25). We previously investigated the anti-cancer activity of the polysaccharide extract of the edible alga S. dentifolium that indicated its apoptotic activity via inducing histone acetylation. Additionally, the extract fractions showed potential anti-genotoxic, anti-mutagenic, and antioxidant activities (26). In the current study, we investigated the influence of S. dentifolium polysaccharide extracts on the hypoxia pathway in OTSCC as a continuation of our previous study and due to the lack of studies on S. dentifolium, which may help in decreasing hypoxia-induced therapeutic resistance in OTSCC.
Materials and Methods
Extraction of Sargassum dentifolium Polysaccharides
Sargassum dentifolium (Turner) C. Agardh was gathered from Hurghada, Red Sea governorate, Egypt, in December 2018. After several washes and drying, the algal mass was grounded by electric mill and sieved through 2-mm mesh. The extraction of water-soluble polysaccharides has been implemented according to the Zhuang group (27), with some modifications (26), as described in a schematic presentation in Figure 1. The powdered extract fractions (SD1, SD2, and SD3) were investigated for their anti-hypoxic effect.
Cytotoxicity and Cell Death Mode
Human tongue squamous carcinoma CAL-27 cells (ATCC, United States) were regularly cultured in supplemented DMEM. Chemicals, including celastrol and cell culture materials, were purchased from Sigma–Aldrich (VA, United States), unless mentioned. The cell vitality was tested using a metabolic cytotoxicity MTT assay (28) after 48 h of cell co-culturing with the extract fractions. The data are expressed as % of control cells (mean ± standard deviation). The half-maximal inhibitory concentration (IC50) was calculated for the extract fractions. Cells (5 × 103 cells/well) were co-cultured with IC50 of fractions, or celastrol, before being stained with ethidium bromide/acridine orange (100 μg/ml; V/V; EB/AO in PBS) (29). The stained cells were analyzed by a fluorescence microscope (n = 8; 200×; Axio Imager Z2, Carl Zeiss, Germany).
Estimation of Total Cellular Hypoxia, HIF-1α, and HIF-1β
Monitoring of the changes in the cellular hypoxia before/after the exposure to extracts was assessed by pimonidazole, a hypoxia-detection reagent, by microplate fluorometer for a qualitative assessment of hypoxia. CAL-27 cells were treated with 30% of IC50 of fractions for 6, 12, 24, and 48 h. In another experiment, cells (5 × 104 cells/well) were seeded with 30% of IC50 of fractions for 48 h. The cells were lysed by Cell Lysis Solution (#LSKCLS500; Merck, United States) that was supplemented with Protease Inhibitor Cocktail (#P8340; Merck, United States). The cell lysates were then submitted to the analysis with either Human HIF-1α ELISA Fluorescent Kit (#ab229433; Abcam, Germany) or Human ARNT/HIF-1 beta Colorimetric ELISA Kit (#LS-F9594; LifeSpan Biosciences, United States). For the immunocytochemical analysis, CAL-27 cells were cultured onto 8-chamber slides and then treated with 30% of IC50 of SD2 for 48 h. The cells were fixed with absolute methanol and then stained using a rabbit monoclonal anti-HIF-1α antibody (Abcam, ab179483), Goat Anti-Rabbit IgG-Phycoerythrin (Abcam, ab72465), and Hoechst 33342 (DNA counterstaining). The cells were analyzed under a fluorescence microscope (Zeiss, Goettingen, Germany), attached to a digital camera (AxioCam MRc3 S/N 4299, Zeiss, Germany) and equipped with an image analyzer (ZEN-11 edition software).
miR-210 and miR-21 Expression
CAL-27 cells (1 × 106 cells) were treated for 48 h, 30% of IC50 of fractions, and then, after, the total RNA was extracted by a miRNeasy RNA extraction kit (#217004, Qiagen, Germany). One microgram of RNA was submitted to reverse transcription by a miScript II RT kit (#218161, Qiagen, Germany). PCR amplification (3-ng cDNA) implemented by a miScript Sybr green PCR kit (#218073, Qiagen, Germany). Primers for U6 (#600750, Agilent technologies), as well as hsa-miR-21 and hsa-miR-210 (#MS00009079 and #MS00003801 Qiagen, Germany), were used. Calculations of the relative miRNA expression were carried out by ΔΔCt protocol (30) after normalization with U6 expression in control cells.
Statistical Analysis
Experimental analyses were repeated independently (n = 6), and the results were presented as mean ± SD. Graphpad Prism software V6 was used. Data were analyzed by Dunnett’s multiple comparisons test after one-way ANOVA. The data were considered significant when p < 0.05.
Results
Cell Viability and Cell Death Mode
The possible cytotoxicity effect of the extract fractions, compared with the anticancer drug celastrol, was investigated by MTT after 48 h of co-culturing with CAL-27 cells. The readings showed that the fractions exhibited a variable cytotoxic effect, where they reduced the cell viability with IC50 of 63.36, 50.27, and 99.49 μg/ml for SD1, SD2, and SD3, respectively (Figure 2A). Celastrol showed a concentration-dependent cytotoxicity with IC50 8.73 μg/ml. Except in the analysis of a cell death mode, 30% of IC50 of each fraction was used in all of the next investigations. The treatment with this IC50 percentage leads to a high viability percentage ranged from 84 to 90%, which represents a safe dose to evaluate the protein and genetic changes, as concluded from the concentration/viability equation. AO/EB was used as dual DNA-staining to monitor the cell death mode in CAL-27 cells. After 48 h of incubation with IC50 of each agent, the overall analysis of the harvested cells indicated that the treatment with celastrol resulted in a high remarkable increase in the percentages of early apoptotic cells (30.71%; p < 0.001) and late apoptotic/necrotic cells (19.12%; p < 0.001), compared to their corresponding control, 6.67 and 3.33%, respectively (Figure 2B). In the other hand, the treatment of cells with IC50 of fractions led to a noticeable elevation in the early apoptotic population in SD1-treated cells (40.01%; p < 0.001) and in SD2-treated cells (27.23%; p < 0.001) and a parallel increase in the percentage of late apoptotic/necrotic cells in SD1- and SD2-treated cells (p < 0.01 and p < 0.05, respectively), as shown in Figure 2B.
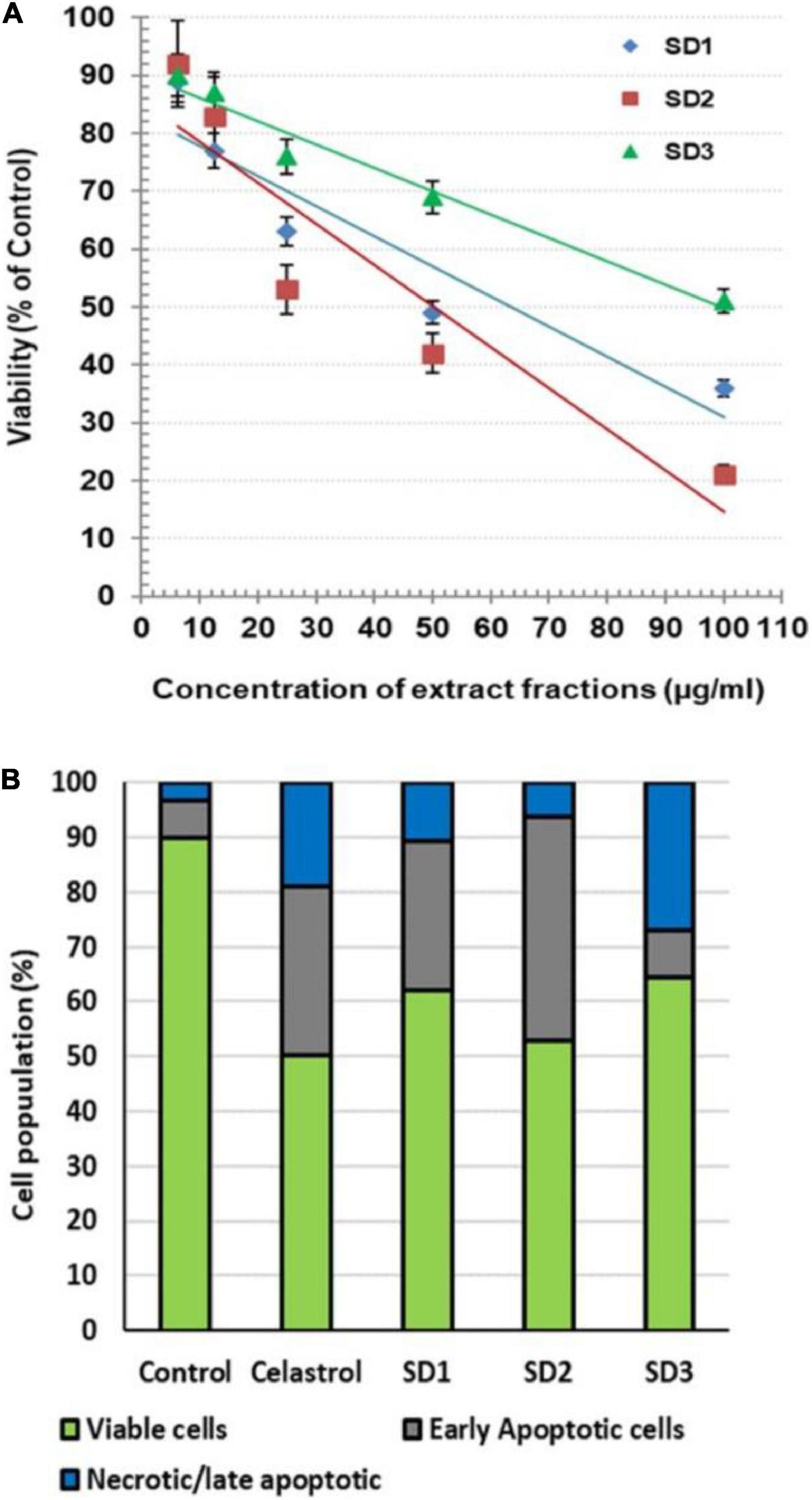
Figure 2. (A) Cytotoxic effect of S dentifolium extract fractions (SD1, SD2, and SD3) on CAL-27 cells after 48 h of incubation, as assayed by MTT. The viability is expressed as percentage of control (mean ± SD; n = 8). (B) A segmented histogram indicates the percentage of cell population distribution of vital, early apoptotic, and late apoptotic/necrotic cells after being treated with IC50 of celastrol or S dentifolium fractions (SD1, SD2, and SD3) for 48 h compared to control.
Monitoring of Cellular Hypoxia
Follow-up of time-dependent alterations in the cellular hypoxia was carried out by pimonidazole, an exogenous nontoxic 2-nitroimidazole. It interacts with the proteins and forms fluorescence adducts, especially with thiol groups, in the hypoxic cells. The fluorescence intensity of these adducts was qualitatively estimated by a microplate fluorescence reader to assess hypoxia occurrence. The cells were co-cultured with 30% of IC50 of each fraction for 6, 12, 24, and 48 h. The experiment indicated that SD1 led to a nonsignificant decrease in cell hypoxia (p > 0.05), as shown in Figure 3A. In the other hand, SD2 and SD3 resulted in a dramatic time-dependent inhibition in the cell hypoxia, especially after 24 and 48 h (p < 0.01; p < 0.001, respectively), Figure 3A, where the high maximal inhibitory time for hypoxia (t50) for SD2 and SD2 was 25.38 and 31.71 h, respectively.
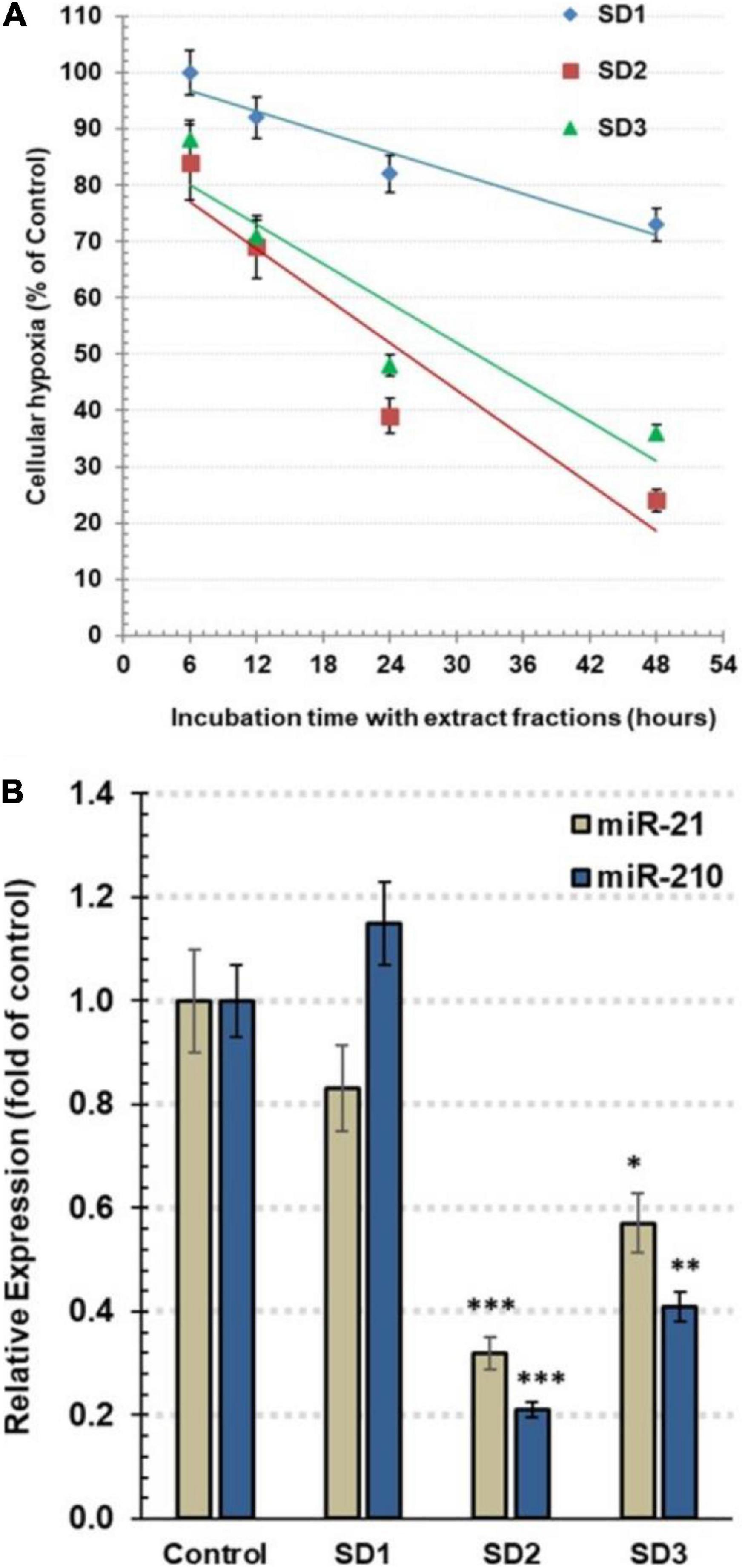
Figure 3. (A) Estimation of the total hypoxia degree by pimonidazole in CAL-27 cells. The cells were co-cultured with 30% of IC50 of each fraction for 6, 12, 24, and 48 h. The results are expressed as percentage of control (mean ± SD; n = 6), where control was 1,374 IFU. (B) The relative expression of hsa-miRNA-21-5p and hsa-miRNA-210-3p in CAL-27 cells after being treated with 30% of IC50 of fractions for 48 h. Data are expressed as mean ± SD, n = 8. *p < 0.05, **p < 0.01, and ***p < 0.001 compared to the corresponding control.
miR-210 and miR-21 Expression
To explore the influence of the fractions on the epigenic regulators of cellular hypoxia, the expression of miR-210 and miR-21 was traced by qRT-PCR. As a master hypoxamiR, the oncomiR miR-210 is upregulated, generally, in tumor cells. Perusing of the effect of extract fractions (30% of IC50) after 48 h, the results revealed that miR-210 expression was significantly diminished in SD2- and SD3-treated cells, reaching.21 ± 0.03 (p < 0.001) and.42 ± 0.06 (p < 0.01) fold of the control cells, respectively (Figure 3B). As a potent oncomiR, miR-21 shows a high expression in various tumor cell types. Furthermore, it acts as a pivotal hypoxamiR during a hypoxia cascade. The treatment with 30% of IC50 of fractions for 48 h resulted in a dramatic downregulation of miR-21 expression in the cells treated with SD2 and SD3 down to 0.32 ± 0.04 (p < 0.01) and 0.57 ± 0.08 (p < 0.05) fold of the control cells, respectively (Figure 3B). These findings suggest that SD2 is a potent inhibitor of hypoxia and its regulators.
Detection of HIF-1α and HIF-1β
Hypoxia-inducible factor-1α and HIF-1β are hypoxia indicators that are controlled, generally, by miR-210 and by miR-21 among other miRNAs. Using ELISA, the influence of the fractions on the protein concentrations of HIF-1α and HIF-1β was investigated in CAL-27 cells after 48 h from treatment. Commonly, the elevated HIF-1α is an indication of cellular hypoxia. SD2 was the most potent HIF-1α inhibitor among the tested fractions (p < 0.01) after 48 h of treatment (Figure 4A). The immunochemical analysis of the cells confirmed the diminished HIF-1α concentration in SD2-treated cells (Figure 4B), compared to untreated cells.
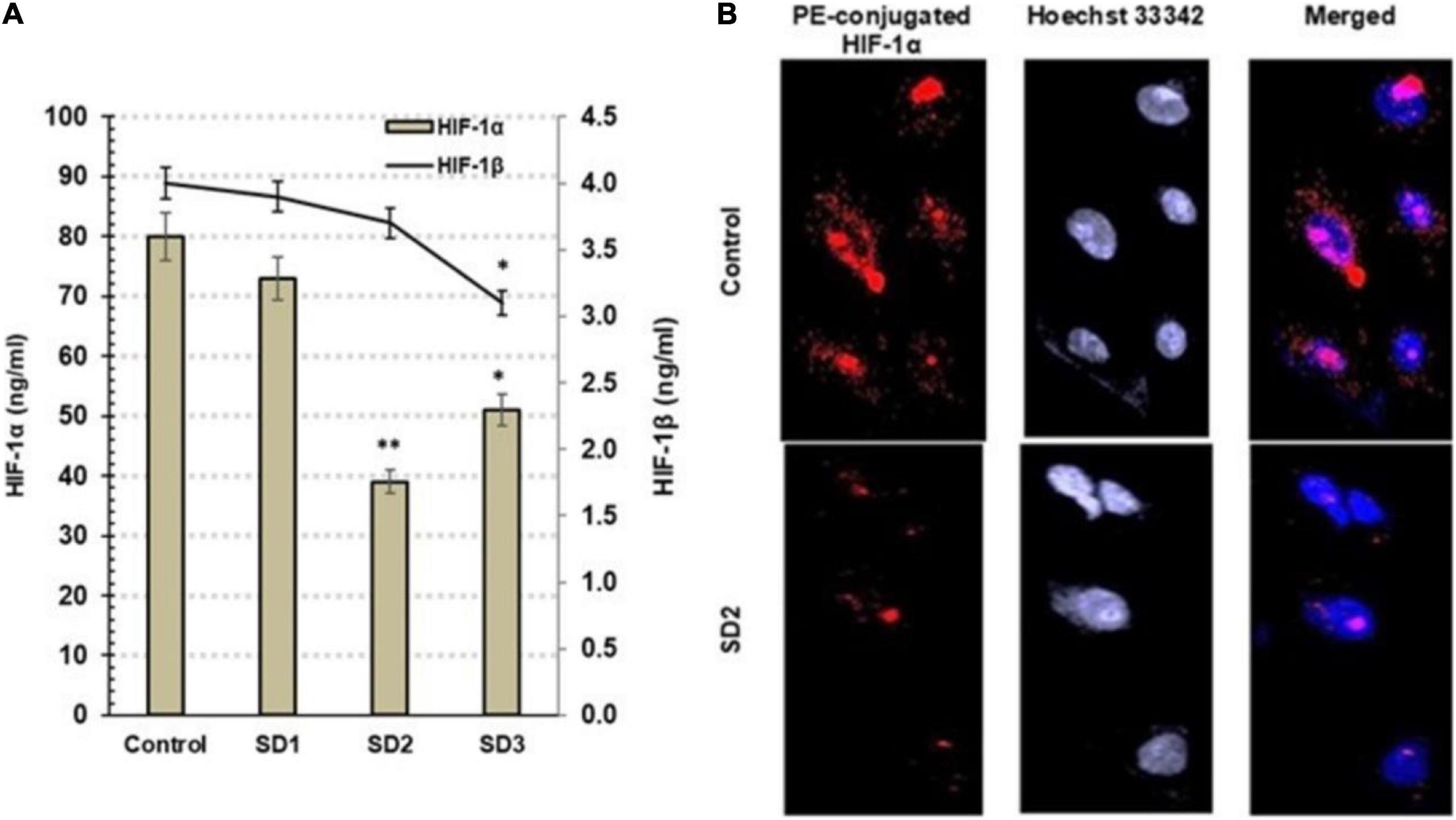
Figure 4. (A) Estimation of HIF-1α and HIF-1β by ELISA in CAL-27 cells after being treated with 30% of IC50 of each extract fraction for 48 h, compared to control cells, after 48 h. Data are expressed as (mean ± SD in ng/ml, n = 6). *p < 0.05, and **p < 0.01 compared with the corresponding control. (B) SD2 treatment led to an inhibited HIF-1α; the immunocytochemical staining was implemented by a PE-conjugated HIF-1α antibody (red) and the nuclei counterstaining with DAPI (blue). SD2-treated cells showed a lower HIF-1α concentration than control. The cells were analyzed under fluorescence microscope (magnification ×200).
Discussion
Herbal nutraceuticals suppress the pathways that induce HIF-1α synthesis and enhance its degradation and reduce its downstream-signaling events (20). Above exerting these anti-cancer activities, herbal nutraceuticals have been reported to (a) reverse hypoxia-stimulated drug resistance, (b) ameliorate the therapeutic index of cancer therapies against hypoxic tumors, and (3) diminish the harmful adverse effects of those therapies. The mechanisms of action of these nutraceuticals and their potent chemo- and radio-sensitization on hypoxic tumor cells are variable and depend on the nutraceuticals type and properties [reviewed in 20, 22]. Therefore, the current study investigated the effect of S. dentifolium polysaccharide extracts on the hypoxia pathway in OTSCC, which may provide a new adjacent agent that lowers OTSCC hypoxia-associated resistance to chemotherapies.
Under normoxic conditions, in the ubiquitin-dependent mechanism, O2-dependent prolyl hydroxylation targets α subunit of the ubiquitin–proteasome pathway and, hence, degrades HIF-1α (31). Subsequently, HIF-1α remains in a steady state and in a low concentration that prevents the formation of the transcriptional functional complex: HIF-1α/HIF-1β. In the other hand, in severe hypoxic conditions, HIF-1α subunit is mainly the responding part of the HIF-1 complex, and the degradation pathway is inhibited; therefore, HIF-1α concentration is rapidly elevated (31). HIF-1α aggregates in the cytoplasm and then transfers to the nucleus to heterodimerize with the β subunit; binds to hypoxia response elements of target genes, which results in overexpression of hypoxia-regulated genes (14). HIF-1α implicates in the regulation of the cell proliferation, motility, survival, apoptosis, cellular metabolism, and angiogenesis, while the HIF-1β subunit occurs constitutively within cytoplasm. In the current study, exploring the effect of S. dentifolium fractions on the cell viability indicated that the fractions exhibited a variable cytotoxic effect on CAL-27 cells in the following order: SD2 > SD1 > SD3. The most potent fraction of the lowest IC50 was SD2 due to high percentages of early and late apoptosis.
Hypoxia markers, such as misonidazole, pimonidazole, nitroimidazole, were used to trace cellular the O2 profile (32). They have the capability to detect hypoxia due to their production of detectable adducts at low O2 tension. For example, pimonidazole is covalently bound to thiol-containing cellular macromolecules in hypoxia cells when the O2 tension is below 10-mm Hg, <1.3% (32). In the current study, screening of hypoxia status in CAL-27 cells by pimonidazole after co-culture with S. dentifolium fractions for different intervals indicated that SD2 was more potent than SD3 in the time-dependent inhibition of hypoxia, especially after 24 and 48 h. It is known that the regulation of HIF-1α is disturbed as a response to the cellular hypoxia status and/or as a subsequent reflection of genetic alterations that occur in tumor pathophysiology, including tumor invasion, angiogenesis, and cell survival (32). Therefore, exploring the status of HIF-1α protein may be the target mechanism of the extract fraction to inhibit cellular hypoxia. In this study, HIF-1α was found to be basically higher in CAL-27 cells compared to the treated cells. HIF-1α protein was significantly diminished when the cells were treated with SD2 or SD3. The study findings confirmed that SD2 strongly inhibited hypoxia through targeting HIF-1α.
HIF-1β is identical to vertebrate aryl hydrocarbon receptor nuclear translocator (ARNT) (33). It is known that HIF-1β is over-expressed in experimental hypoxic conditions and metastatic tumor cells (34). Contrary to early reports, HIF-1β has a critical function in hypoxic response, where recent reports advocate that HIF-1β concentrations are not consistent during hypoxia. Instead, HIF-1β is fluctuated in response to hypoxic conditions, parallel to HIF-1α, as its heterodimeric partner (34, 35). In the present study, HIF-1β concentration showed a nonsignificant alteration in SD1- and SD2-treated cells, while SD3 treatment led to a noticed inhibition in HIF-1β concentration. Recently, HIF-1β has been reported to be required for full activation of NF-κB in cells in response to canonical and non-canonical stimuli (36), where HIF-1β binds TRAF6 gene and regulates its expression independently from HIF-1α. Moreover, exogenous TRAF6 expression is capable to recover all of the cellular hypoxic phenotypes in absence of HIF-1β. These findings elaborated that HIF-1β is an essential regulator of NF-κB (37). Therefore, the inhibition of HIF-1β by SD3 may provide not only a role in the inhibition of the hypoxia pathway but also a suppression of the NF-κB activation process, a suggestion that needs further investigations.
The majority of miRNAs are alleged to function through RNA-target silencing complexes as a consequence of the impaired base pairing of 30-UTR of certain mRNAs to their target genes; hence, it closes the mRNA translation of these genes or stimulates a direct damaging cleavage (8). A fundamental role is implemented by miRNAs in the upregulation/downregulation of genes in the balance of cell growth/apoptosis (8). Imperfect expression of miRNA often accelerates cancer progression (38). miRNAs are mainly tending to be either oncogenes or tumor suppressors in cancers, including oral cancer (38). OncomiRs, oncogenic miRNAs, are dramatically overexpressed in a way that evoked the neoplastic initiation successively into the tumor progression stage. In the other hand, the tumor suppressive miRNAs play an important role in halting or blocking the tumor development (39). miRNAs expression in OTSCC dominantly controls the activation of gene transcription regulators: protein kinase Ca and HIF-1α that are mainly stimulating the transcription of hypoxamiRs: miR-210 and miR-21 (38). Disturbance of miRNAs in OTSCC resulted in a concomitant induction in cell division, growth rate, anti-apoptosis, metastasis, and drug resistance (38).
miR-210 is an essential responding factor to the hypoxia occurrence in the microenvironment of the endothelial cells, which controls the cells when they are dividing, differentiating, and migrating (40). Previously, fundamental microRNAs functions were recognized in critical cellular pathways in TME. Overexpressed miR-210 was observed to participate in the stabilization of HIF- 1α in TME, in hypoxia (41), and that this overexpression was noticed in head and neck cancerous patients (42). The effect of the extract fractions on the expression of hypoxia-controlling miRNAs (miR-21 and miR-210) was determined. The treatment of OTSCC cells with the fractions SD2 and SD3 dramatically diminished the miR-210 expression. Similarly, the treatment with SD2 and SD3 led to a dramatic down-expression of miR-21 in the cells. miR-21 is overexpressed in most of cancers and possesses several oncogenic functions in carcinomas including OTSCC (43), where miR-21 is correlated with depleted apoptosis (44). Other reports have been reported that using anti-miR-21 to block miR-21 triggers apoptosis and diminishes anchorage-based division of OTSCC cells and likewise suppresses mass xenografts formation of OTSCC in immunocompromised animals (38, 45). A previous report claimed that, in OTSCC, miR-21 decreased the apoptosis through silencing of tropomyosin 1 gene (45). Patients with oral cancer show a successful initial response to chemotherapy; however, a later relapse regularly occurs due to hypoxia-stimulated drug resistance, which limits the chemotherapy effectiveness and its application (46). Therefore, approaches to minimizing the cellular hypoxia through edible complementary agents may facilitate a lower drug resistance and potentiate the efficiency of radio/chemotherapies in oral cancer. Accordingly, the findings of this study deduce S. dentifolium extracts as a promising adjacent candidate to chemotherapies for inhibiting hypoxia-induced drug resistance in TME.
Conclusion
The extract fractions of the edible alga S. dentifolium were used in this investigation. Through separate mechanistic amelioration of HIF-1α and HIF-1β and their regulators—miRNA-21 and miRNA-210 - the fractions SD2 and SD3 revealed a possible anti-hypoxia impact in Tongue Squamous Cell Carcinoma (CAL27) cells. The findings imply that S. dentifolium could be used as a complementary dietary agent in cancer therapy to reduce hypoxia-related therapeutic resistance in solid tumors.
Data Availability Statement
The original contributions presented in the study are included in the article/supplementary material, further inquiries can be directed to the corresponding author.
Author Contributions
AG-E: study concept/design and writing manuscript. BR: statistical analysis. SE-D, NH, AA, HJB, and MA: practical work. ER: alga extraction. All authors contributed to the article and approved the submitted version.
Funding
The authors extend their appreciation to the Deputyship for Research and Enovation, Ministry of Education of Saudi Arabia for funding this research work through the project number 1-441-132.
Conflict of Interest
The authors declare that the research was conducted in the absence of any commercial or financial relationships that could be construed as a potential conflict of interest.
The reviewer [AAT] declared a shared affiliation with the authors [SE-D] to the handling editor at the time of review.
Publisher’s Note
All claims expressed in this article are solely those of the authors and do not necessarily represent those of their affiliated organizations, or those of the publisher, the editors and the reviewers. Any product that may be evaluated in this article, or claim that may be made by its manufacturer, is not guaranteed or endorsed by the publisher.
Acknowledgments
We gratefully acknowledge the support of the Deanship of Scientific Research, Taif University.
References
1. Dar A, Baig HS, Saifuallah SM, Ahmad VU, Yasmeen N, Nizamuddin M. Effect of seasonal variation on the anti-inflammatory activity of Sargassum wightii growing on the N Arabian sea coast of Pakistan. J Exp Mar Biol Ecol. (2007) 351:1–9.
2. Mayer AM, Rodríguez AD, Berlinck RG, Hamann MT. Marine pharmacology in 2003-4: marine compounds with anthelmintic antibacterial, anticoagulant, antifungal, anti-inflammatory, antimalarial, antiplatelet, antiprotozoal, antituberculosis, and antiviral activities; affecting the cardiovascular, immune and nervous systems, and other miscellaneous mechanisms of action. Comp Biochem Physiol Toxicol Pharmacol. (2007) 145:553–81. doi: 10.1016/j.cbpc.2007.01.015
3. Raafat EM, Gamal-Eldeen AM, El-Hussieny EA, Ahmed EF, Eissa AA. Polysaccharide extracts of the brown alga Sargassum asperifolium possess in vitro cancer chemopreventive properties. Nat Prod Res. (2014) 28:2304–11. doi: 10.1080/14786419.2014.926351
4. Gamal-Eldeen AM, Ahmed EF, Abo-Zeid MA. In vitro cancer chemopreventive properties of polysaccharide extract from the brown alga, Sargassum latifolium. Food Chem Toxicol. (2009) 47:1378–84. doi: 10.1016/j.fct.2009.03.016
5. Delma C, Ramalingam K, Pandian V, Baskar A, Savarimuthu I, Thangavelu B, et al. Inhibition of tumor cell migration and angiogenesis by sulphated polysaccharides from Sargassum wightii. Greville. Cancer Prev Res. (2008) 1(7 Suppl.):A5.
6. Josephine A, Nithya K, Amudha G, Veena CK, Preetha SP, Varalakshmi P. Role of sulphated polysaccharides from Sargassum wightii in cyclosporine A-induced oxidative liver injury in rats. BMC Pharmacol. (2008) 8:4. doi: 10.1186/1471-2210-8-4
7. Husen SA, Syadzha MF, Setyawan MF, Pudjiastuti P, Ansori ANM, Susilo RJK, et al. Evaluation of the combination of Sargassum duplicatum, Sargassum ilicifolium, Abelmoschus esculentus, and Garcinia mangostana extracts for open wound healing in diabetic mice. SRP. (2020) 11:888–92.
8. Ye H, Yu T, Temam S, Ziober BL, Wang J, Schwartz JL, et al. Transcriptomic dissection of tongue squamous cell carcinoma. BMC Genomics. (2008) 9:69. doi: 10.1186/1471-2164-9-69
9. Sano D, Myers JN. Metastasis of squamous cell carcinoma of the oral tongue. Cancer Metastasis Rev. (2007) 26:645–62.
10. Wong TS, Liu XB, Wong BY, Ng RW, Yuen AP, Wei WI. Mature miR-184 as potential oncogenic microRNA of squamous cell carcinoma of tongue. Clin Cancer Res. (2008) 14:2588–92. doi: 10.1158/1078-0432.CCR-07-0666
11. Almangush A, Heikkinen I, Mäkitie AA, Coletta RD, Läärä E, Leivo I, et al. Prognostic biomarkers for oral tongue squamous cell carcinoma: a systematic review and meta-analysis. Br J Cancer. (2017) 117:856–66.
12. Yu T, Tang B, Sun X. Development of inhibitors targeting hypoxia-inducible factor 1 and 2 for cancer therapy. Yonsei Med J. (2017) 58:489–96.
13. Warnakulasuriya S. Global epidemiology of oral and oropharyngeal cancer. Oral Oncol. (2009) 45:309–16. doi: 10.1016/j.oraloncology.2008.06.002
15. Roma-Rodrigues C, Mendes R, Baptista PV, Fernandes AR. Targeting tumor microenvironment for cancer therapy. Int J Mol Sci. (2019) 20:840.
16. Birner P, Schindl M, Obermair A, Plank C, Breitenecker G, Oberhuber G. Overexpression of hypoxia-inducible factor 1alpha is a marker for an unfavorable prognosis in early-stage invasive cervical cancer. Cancer Res. (2000) 60:4693–6.
17. McKeown SR. Defining normoxia, physoxia and hypoxia in tumours-implications for treatment response. Br J Radiol. (2014) 87:20130676. doi: 10.1259/bjr.20130676
18. Huang Y, Lin D, Taniguchi CM. Hypoxia inducible factor. HIF. in the tumor microenvironment: friend or foe? Sci China Life Sci. (2017) 60:1114–24. doi: 10.1007/s11427-017-9178-y
19. Dillard CJ, German JB. Phytochemicals: nutraceuticals and human health. J Sci Food Agric. (2000) 80:1744–56.
20. Nalini D, Selvaraj J, Kumar GS. Herbal nutraceuticals: safe and potent therapeutics to battle tumor hypoxia. J Cancer Res Clin Oncol. (2020) 146:1–18. doi: 10.1007/s00432-019-03068-x
21. Jing X, Yang F, Shao C, Wei K, Xie M, Shen H, et al. Role of hypoxia in cancer therapy by regulating the tumor microenvironment. Mol Cancer. (2019) 18:157.
22. Ikeda H, Kakeya H. Targeting hypoxia-inducible factor 1 (HIF-1) signaling with natural products toward cancer chemotherapy. J Antibiot. (2021) 74:687–95 doi: 10.1038/s41429-021-00451-0
23. Nagle DG, Zhou YD. Marine natural products as inhibitors of hypoxic signaling in tumors. Phytochem Rev. (2009) 8:415–29. doi: 10.1007/s11101-009-9120-1
24. Mohammed KA, Hossain CF, Zhang L, Bruick RK, Zhou YD, Nagle DG. Laurenditerpenol, a new diterpene from the tropical marine alga Laurencia intricata that potently inhibits HIF-1 mediated hypoxic signaling in breast tumor cells. J Nat Prod. (2004) 67:2002–7. doi: 10.1021/np049753f
25. Gamal-Eldeen AM, Raafat BM, El-Daly SM, Fahmy CA, Almehmadi MM, Althobaiti F. Inhibitory effect of Sargassum latifolium extract on hypoxia pathway in colon cancer cells. J Sci Food Agric. (2021) 33:600–6. doi: 10.9755/ejfa.2021.v33.i7.2727
26. Gamal-Eldeen AM, Abo-Zeid MA, Ahmed EF. Anti-genotoxic effect of the Sargassum dentifolium extracts: prevention of chromosomal aberrations, micronuclei, and DNA fragmentation. Exp Toxicol Pathol. (2013) 65:27–34. doi: 10.1016/j.etp.2011.05.005
27. Zhuang C, Itoh H, Mizuno T, Ito H. Antitumor active fucoidan from the brown seaweed, umitoranoo. Sargassum thunbergii. Biosci Biotech Biochem. (1995) 59:563–7. doi: 10.1271/bbb.59.563
28. Hansen MB, Nielsen SE, Berg K. Re-examination and further development of a precise and rapid dye method for measuring cell growth/cell kill. J Immunol Methods. (1989) 119:203–10. doi: 10.1016/0022-1759(89)90397-9
29. Baskić D, Popović S, Ristić P, Arsenijević NN. Analysis of cycloheximide-induced apoptosis in human leukocytes: fluorescence microscopy using annexin V/propidium iodide versus acridin orange/ethidium bromide. Cell Biol Int. (2006) 30:924–32. doi: 10.1016/j.cellbi.2006.06.016
30. Livak KJ, Schmittgen TD. Analysis of relative gene expression data using real-time quantitative PCR and the 2(-Delta Delta C(T)) method. Methods. (2001) 25:402–08. doi: 10.1006/meth.2001.1262
31. Mylonis I, Simos G, Paraskeva E. Hypoxia-inducible factors and the regulation of lipid metabolism. Cells. (2019) 2019:8. doi: 10.3390/cells8030214
32. Challapalli A, Carroll L, Aboagye EO. Molecular mechanisms of hypoxia in cancer. Clin Transl Imag. (2017) 5:225–53. doi: 10.1007/s40336-017-0231-1
33. Dery MA, Michaud MD, Richard DE. Hypoxia-inducible factor 1: regulation by hypoxic and non-hypoxic activators. Int J Biochem Cell Biol. (2004) 37:535–40. doi: 10.1016/j.biocel.2004.08.012
34. Ercin ME, Bozdoğan Ö, Çavuşoğlu T, Bozdoğan N, Atasoy P, Koçak M. Hypoxic gene signature of primary and metastatic melanoma cell lines: focusing on HIF-1β and NDRG-1. Balkan Med J. (2019) 37:15–23. doi: 10.4274/balkanmedj.galenos.2019.2019.3.145
35. Zagorska A, Dulak J. HIF-1: the knowns and unknowns of hypoxia sensing. Acta Biochim Pol. (2004) 51:563–85.
36. van Uden P, Kenneth NS, Webster R, Müller HA, Mudie S, Rocha S. Evolutionary conserved regulation of HIF-1β by NF-κB. PLoS Genet. (2011) 7:e1001285. doi: 10.1371/journal.pgen.1001285
37. D’Ignazio L, Shakir D, Batie M, Muller HA, Rocha S. HIF-1β positively regulates NF-κB activity via direct control of TRAF6. Int J Mol Sci. (2020) 21:3000.
38. Wu BH, Xiong XP, Jia J, Zhang WF. MicroRNAs: new actors in the oral cancer scene. Oral Oncol. (2011) 47:314–9. doi: 10.1016/j.oraloncology.2011.03.019
39. Varol N, Konac E, Gurocak OS, Sozen S. The realm of microRNAs in cancers. Mol Biol Rep. (2011) 38:1079–89. doi: 10.1007/s11033-010-0205-0
40. Fasanaro P, D’Alessandra Y, Di Stefano V, Melchionna R, Romani S, Pompilio G, et al. MicroRNA-210 modulates endothelial cell response to hypoxia and inhibits the receptor tyrosine kinase ligand ephrin-A3. J Biol Chem. (2008) 283:15878–83. doi: 10.1074/jbc.M800731200
41. Puisségur MP, Mazure NM, Bertero T, Pradelli L, Grosso S, Robbe-Sermesant K, et al. miR-210 is overexpressed in late stages of lung cancer and mediates mitochondrial alterations associated with modulation of HIF-1 activity. Cell Death Differ. (2011) 18:465–78. doi: 10.1038/cdd.2010.119
42. Gee HE, Camps C, Buffa FM, Patiar S, Winter SC, Betts G, et al. hsa-mir-210 is a marker of tumor hypoxia and a prognostic factor in head and neck cancer. Cancer. (2010) 116:2148–58. doi: 10.1002/cncr.25009
43. Selcuklu SD, Donoghue MT, Spillane C. miR-21 as a key regulator of oncogenic processes. Biochem Soc Trans. (2009) 37:918–25.
44. Li J, Huang H, Sun L, Yang M, Pan C, Chen W, et al. miR-21 indicates poor prognosis in tongue squamous cell carcinomas as an apoptosis inhibitor. Clin Cancer Res. (2009) 15:3998–4008. doi: 10.1158/1078-0432.CCR-08-3053
45. Blower PE, Chung JH, Verducci JS, Lin S, Park JK, Dai Z, et al. MicroRNAs modulate the chemosensitivity of tumor cells. Mol Cancer Ther. (2008) 7:1–9.
Keywords: Sargassum dentifolium, miR-21, miR-210, HIF-1α, HIF-1β, CAL-27 OTSCC cells
Citation: Gamal-Eldeen AM, Raafat BM, Alrehaili AA, El-Daly SM, Hawsawi N, Banjer HJ, Raafat EM and Almehmadi MM (2022) Anti-hypoxic Effect of Polysaccharide Extract of Brown Seaweed Sargassum dentifolium in Tongue Squamous Cell Carcinoma. Front. Nutr. 9:854780. doi: 10.3389/fnut.2022.854780
Received: 14 January 2022; Accepted: 14 February 2022;
Published: 24 March 2022.
Edited by:
Mostafa Waly, Sultan Qaboos University, OmanReviewed by:
Preeja Prabhakar, Sultan Qaboos University, OmanAshraf A. Tabll, National Research Centre, Egypt
Ala-Eddin Al Moustafa, Qatar University, Qatar
Martina Smolic, Josip Juraj Strossmayer University of Osijek, Croatia
Copyright © 2022 Gamal-Eldeen, Raafat, Alrehaili, El-Daly, Hawsawi, Banjer, Raafat and Almehmadi. This is an open-access article distributed under the terms of the Creative Commons Attribution License (CC BY). The use, distribution or reproduction in other forums is permitted, provided the original author(s) and the copyright owner(s) are credited and that the original publication in this journal is cited, in accordance with accepted academic practice. No use, distribution or reproduction is permitted which does not comply with these terms.
*Correspondence: Amira M. Gamal-Eldeen, amabdulaziz@tu.edu.sa, aeldeen7@yahoo.com