- 1National R&D Center for Edible Fungus Processing Technology, Henan University, Kaifeng, China
- 2Functional Food Engineering Technology Research Center, Kaifeng, China
- 3Joint International Research Laboratory of Food & Medicine Resource Function, Kaifeng, China
- 4College of Physical Education, Henan University, Kaifeng, China
Proteomics and intestinal flora were used to determine the mechanism of immune modulatory effects of Flammulina velutipes polysaccharide on immunosuppressed mice. The results showed that compared with the model group, F. velutipes polysaccharide could increase thymus and spleen indices and improve thymus tissue structure in mice; IL-2 and IL-4 contents were significantly increased and IL-6 and TNF-α contents were significantly decreased; serum acid phosphatase (ACP), lactate dehydrogenase (LDH) and total antioxidant capacity (T-AOC) activities were increased (P < 0.05); in the liver, superoxide dismutase (SOD) and catalase (CAT) activities were increased (P < 0.001), while malondialdehyde (MDA) content was decreased (P < 0.001). Proteomics discovered that F. velutipes polysaccharides may exert immune modulatory effects by participating in signaling pathways such as immune diseases, transport and catabolism, phagosomes and influenza A, regulating the immune-related proteins Transferrin receptor protein 1 (TFRC) and Radical S-adenosyl methionine domain-containing protein 2 (RSAD2), etc. Gut microbial studies showed that F. velutipes polysaccharides could increase the abundance of intestinal flora and improve the flora structure. Compared to the model group, the content of short-chain fatty acids (SCFAs) and the relative abundance of SCFA-producers Bacteroides and Alloprevotella were increased in the F. velutipes polysaccharide administration group, while Lachnospiraceae_NK4A136_group and f_Lachnospiraceae_Unclassified decreased in relative abundance. Thus, F. velutipes polysaccharide may play an immunomodulatory role by regulating the intestinal environment and improving the balance of flora.
Introduction
Immunosuppression is a distinctive feature of immune disorders (1), which predispose to the development of tumors, infections, cardiovascular diseases and diabetes, so the improvement of immune modulatory effects has become an urgent problem. Edible mushrooms are collected and/or cultivated worldwide and considered to be an important part of a healthy human diet (2, 3). In recent years, the immune modulatory effect of edible mushrooms polysaccharides has attracted much attention (4). Schizophyllum commune polysaccharides can increase RAW264.7 cell activity and promote the production of large amounts of NO by cells to exert immune effects (5). Hericium erinaceus polysaccharide (HEP) can improve immune function by enhancing cellular and humoral immunity, macrophage phagocytosis and NK cell activity in mice, in addition, Sheng et al. found that HEP can upregulate intestinal SIgA secretion and activate MAPK and AKT cell signaling pathways (6).
Flammulina velutipes is one of the most popular edible mushrooms that contain triterpenes, polysaccharides, sterols and vitamins. F. velutipes polysaccharides have anti-inflammatory, antioxidant, anti-aging, immune modulatory, and intestinal flora activities (7–10). Earlier experiments of our group found that F. velutipes polysaccharides were non-toxic and could promote intestinal motility and improve constipation in rats (11). Liang obtained two polysaccharides CHFVP-1 (24.44 kDa) and CHFVP-2 (1,497 kDa) from F. velutipes and found that CHFVP-1 had procoagulant activity in vitro (12). A review of the literature shows that F. velutipes polysaccharides can modulate the intestinal microbiota of healthy mice and has potential immunomodulatory abilities (13, 14). However, the target proteins of immunomodulatory effects of this biologically active polysaccharide in immunocompromised mice remains to be investigated.
Proteomics can elucidate the composition of proteins and their action patterns in tissues and cells at a holistic level, can rapidly and precisely identify the key functional proteins between polysaccharides and immunity, lay the foundation for the study of disease mechanisms, drug action targets and new drug development (15–18). Zhao et al. investigated the key proteins and immune-related pathways stimulated by LPS after pretreatment with Hippophae rhamnoides polysaccharide in IPEC-J2 cells by proteomics techniques, and identified 42 key proteins related to immune pathways, and MAPKs/NF-κB signaling pathway may be the target for the efficacy of the drug (19). Yang et al. analyzed the effect of Taishan Pinus massoniana pollen polysaccharide on chicken peripheral blood lymphocyte proteome and identified 10 differentially expressed candidate proteins. Candidate proteins, and some differentially expressed proteins were associated with host innate immune response, stress-induced immune response and lipid synthesis-related pathways (20). The intestine is the largest immune organ and contains a large number of immune cells (21). The intestinal flora and its metabolites can play an important role in host immune homeostasis by promoting the development of the immune system, activating the immune response and regulating immune cell function (22). Numerous studies have shown that a variety of plant-derived polysaccharides can act directly as prebiotics or indirectly induce changes in the intestinal flora (23). Chen et al. found that food polysaccharide could reduce intestinal damage in immunosuppressed mice, regulate the composition of intestinal microorganisms, increase the levels of SCFAs, and exert intestinal immunomodulatory effects (24). Coptis chinensis Franch polysaccharides, when utilized by the intestinal flora, dynamically regulates the diversity, composition and distribution of the intestinal flora and has a regulatory effect on the intestinal immune microenvironment (25). The use of proteomic techniques and flora analysis will help to explore the mechanism of action of polysaccharide immunomodulation. Therefore, the immunosuppression model was established to investigate the immune modulatory mechanism of F. velutipes polysaccharides in immunocompromised mice, and to infer the target proteins of F. velutipes polysaccharides by proteomics in vivo.
Materials and Methods
Materials and Reagents
Cyclophosphamide: Jiangsu Hengrui Pharmaceutical Co., Ltd (batch number: 20031125); Lentinus edodes Polysaccharide Tablets: Hubei Guangren Pharmaceutical Co., Ltd (batch number: 1909080); Biochemical kit: Nanjing Jiancheng Institute of Biological Engineering; ELISA kit: Beijing Si Zhengbai Biotechnology Co., Ltd; Paraformaldehyde fixative: Wuhan Xavier Biotechnology Co., Ltd; antibody TFRC: abcam; antibody RSAD2: Wuhan Sanying Biotechnology Co. Multiskan GO full wavelength enzyme standardizer: Thermo Fisher; Analytical balance: Sartorius.
Extraction of Polysaccharides
Flammulina velutipes polysaccharides was extracted according to the literature (26) with the extraction rate of 1.49%.
Animals
Specific pathogen free (SPF) grade, male, Kunming (KM) mice, 4–5 weeks old, 18–22 g. All mice were placed in a light-dark cycle at 24 ± 2°C for 12 h. Mice were acclimated for 7 d before the start of the experiment and were fed standard chow and had free access to water. Animals were acclimatized and fed for 1 week. The study received ethical approval from the Ethics Committee of Henan University School of Medicine (HUSOM2021-76) and was conducted in accordance with the guidelines of the Ethics Committee of the Animal Experimentation Ethics Committee.
Establishment of Immunosuppressed Mouse Model
Fifty-four mice were randomly divided into 6 groups of 9 mice each according to body weight, namely, blank group (BC), model group (MC), positive control group (PC), high-dose group (HD, 200 mg/kg), medium-dose group (MD, 100 mg/kg) and low-dose group (LD, 50 mg/kg) of F. velutipes polysaccharide. The BC and MC groups were given saline (0.1 mL/10 g) daily by gavage, the PC group was administered Lentinus edodes polysaccharide tablets by gavage at 3 mg/kg body weight as a positive control once daily for 21 d. Except for the BC group, which was administered saline intraperitoneally, the remaining groups were molded with CTX at 80 mg/kg intraperitoneally on days 18, 19, 20, and 21 of the experiment.
Measurement of Organ Indices
The mice were fasted for 12 h after the last administration, and the body weight of each group was weighed. After removing the eyeballs, they were executed. The thymus and spleen tissues of the mice were immediately taken, washed with PBS solution, blotted dry on filter paper and weighed. The immune organ indices of mice were calculated according to the following formula.
Determination of Lactate Dehydrogenase and Acid Phosphatase Activity
The prepared sera were taken and the activity of LDH and ACP were determined by microplate method and microenzymatic assay, respectively, according to the instructions of the kit.
Determination of Cytokine Content in Mice Serum
Blood was taken into 1.5 mL EP tubes by the eyeball removal method, and serum was prepared by centrifugation at 4°C and 3,500 r/min for 10 min. The cytokines interleukin 2 (IL-2), interleukin 4 (IL-4), interleukin 6 (IL-6), and tumor necrosis factor α (TNF-α) were detected in the serum by ELISA according to the kit instructions.
Determination of Antioxidant Stress Capacity
The prepared serum was taken and the total antioxidant capacity (T-AOC) level in mice was determined according to the kit instructions. The mouse liver tissues were weighed accurately, and 10% tissue homogenate was prepared by homogenization method under ice water bath conditions according to the ratio of weight (g) to volume (mL) of 1:9, and the supernatant was centrifuged at 2,500 rpm/min for 10 min. The supernatant was taken and the malondialdehyde (MDA), superoxide dismutase (SOD) and catalase (CAT) were measured in mouse liver tissues according to the kit instructions, respectively.
Pathological Observation
The mouse thymus specimens were rinsed with PBS, placed in 4% paraformaldehyde solution for fixation, dehydrated with gradient ethanol solution, and paraffin-embedded sections. H&E staining was performed by hematoxylin-eosin for 5 min, followed by dehydration, sealing of the sections with neutral gum, microscopic examination, and image acquisition for analysis.
Proteomics Studies
The spleens of mice in the BC, MC, and HD groups were taken, and after washing, the spleen tissues of each three mice were combined into one sample, and the obtained samples were subjected to proteomic assay (27).
Western Blotting Analysis
According to the amount of protein supernatant, 1/4 of loading buffer was added and mixed, and then heated at 100°C for 10 min. Forty microgram of sample protein solution was taken and analyzed by SDS-PAGE method for protein TFRC and RSAD2.
Diversity Sequencing of Cecum Contents Flora
Under aseptic conditions, the cecum contents of mice were taken, the contents of three mice from each group were pooled, and the obtained samples were sequenced for flora diversity analysis (28).
Content Determination of SCFAs
The contents of acetic acid, propionic acid and butyric acid in cecum contents were analyzed with reference to literature (29).
Bioinformatics Analysis
The identified proteins and peptides were firstly obtained under a filtering criterion of 1% FDR (PSM-level FDR ≤ 0.01). The differential proteins were clustered and analyzed by Euclidean distance and systematic clustering method (Hierarchical Cluster). The identified differential proteins were also subjected to GO functional annotation, Pathway enrichment analysis, protein interactions analysis, and subcellular localization analysis.
The forward and reverse reads obtained from double-end sequencing were spliced two-by-two, and after quality filtering to remove chimeric sequences, the final valid sequences obtained were subjected to OTU clustering analysis by Vsearch (1.9.6) (sequence similarity was set to 97%), and the reference database Silva 132 was used for sequence comparison analysis, and the representative sequences of OTU were analyzed for species taxonomy. Based on the analysis results obtained from OTU, sample Alpha diversity analysis, Beta diversity analysis, and colony function prediction were performed.
Statistical Analysis
The results were expressed after arithmetic mean and standard deviation, and the data were statistically compared for significant differences by one-way analysis of variance (One-Way ANOVA) using SPSS 19.0 software.
Results
Effect of Flammulina velutipes Polysaccharide on Thymus Index and Spleen Index of Mice
In Table 1, compared with the BC group, the thymus index and spleen index of the MC group was decreased, indicating the model was established. Compared with the MC group, the spleen index of mice in the polysaccharide administration group and the thymus index in the PC and HD groups were significantly increased, the thymus index in the MD and LD groups had no the significant level, but it was an increasing trend compared with the MC group. It showed that F. velutipes polysaccharide could improve the atrophy of immunosuppressed mice constructed by CTX, and promote their development and enhance the immunity of the organism.
Effect of Flammulina velutipes Polysaccharide on ACP and LDH Activity in Mice
In Table 2, the ACP and LDH activity in serum of MC group was significantly reduced compared with BC group. After the administration treatment, the ACP vitality in the HD group was significantly increased compared with the MC group, and the LDH vitality in both the PC group and the administered group was improved, especially in the polysaccharide administered group. It indicated that the F. velutipes polysaccharide is beneficial to improve the ACP and LDH activity in mice.
Effect of Flammulina velutipes Polysaccharide on the Content of Cytokines in Mice Serum
In Figure 1, the levels of IL-2 and IL-4 were significantly decreased and the levels of IL-6 and TNF-α were extremely significantly increased in the MC group compared with the BC group (P < 0.001), indicating that cyclophosphamide could suppress immune activity in mice. The levels of IL-2, IL-4, IL-6, and TNF-α were improved by administration of the drug, and the best results were achieved in the HD group with highly significant levels (P < 0.001). It showed that F. velutipes polysaccharide could restore cyclophosphamide-induced immunosuppression and improve immune activity by regulating the levels of cytokines.
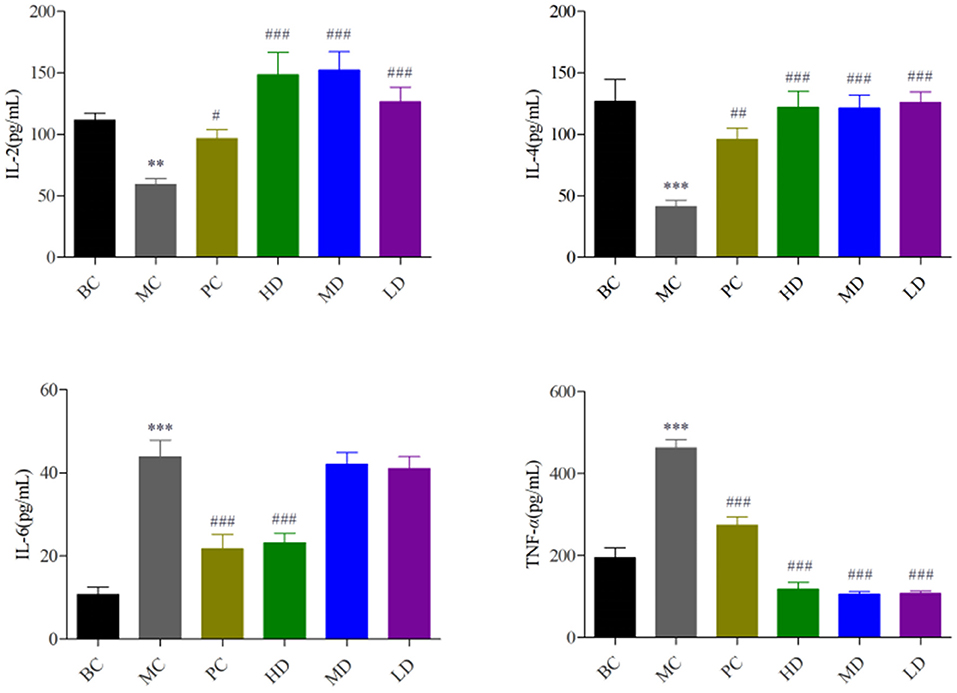
Figure 1. Effect of F. velutipes polysaccharide on serum cytokine content. Compared with BC group: ***P < 0.001, **P < 0.01; Compared with MC group: ###P < 0.001, ##P < 0.01, #P < 0.05.
Effect of Flammulina velutipes Polysaccharide on Antioxidant Capacity of Mice
In Figure 2, compared with the BC group, the T-AOC content and SOD and CAT activity in the MC group were highly significant decreased (P < 0.001) and the MDA activity was significantly increased (P < 0.001), indicating the model was established. Compared with the MC group, T-AOC content and SOD and CAT viability values were significantly increased and MDA activity was significantly decreased in the PC group and the high and medium dose administration groups, and SOD viability was significantly increased and MDA activity was highly significantly decreased in the low dose group, and it proved that F. velutipes polysaccharide had antioxidant capacity.
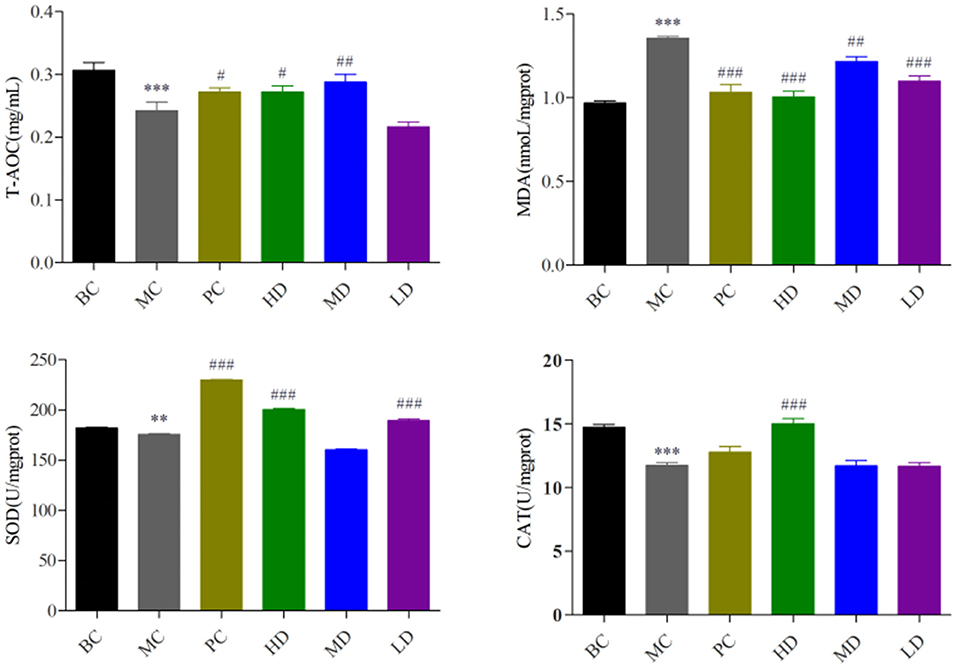
Figure 2. Effect of F. velutipes polysaccharide on antioxidant capacity of mice. Compared with BC group: ***P < 0.001, **P < 0.01; Compared with MC group: ###P < 0.001, ##P < 0.01, #P < 0.05.
Effect of Flammulina velutipes Polysaccharide on Pathological Changes of Mouse Thymus Organs
In Figure 3, the thymus cells of mice in BC group were abundant and well-arranged, there were no abnormalities, and the thymus staining was darker. Compared with the BC group, the thymus cells in the MC group were arranged in a disorganized and irregular manner with unclear edges, and the decrease of thymus cells led to the overall lighter staining of the thymus. Compared with the MC group, the treatment of F. velutipes polysaccharide alleviated the CTX-induced pathological changes in the thymus of mice, indicating that polysaccharide could alleviate the atrophy of the thymus caused by CTX and protect the thymus of mice.
Protein Identification and Protein GO (Gene Ontology) Annotation Analysis
A total of 717,312 secondary spectra were generated for the 3 sets of samples in this experiment. A total of 31,743 peptides and 5,975 proteins were identified under the “1% FDR” filtering criteria. The differentially expressed proteins between groups were compared by screening for differential ploidy and significance. In Figure 4A, the protein quantification results statistics were presented in the form of volcano plots. One hundred and thirty four differentially expressed proteins were identified in the MC group compared with the BC group, of which 52 were up-regulated and 82 were down-regulated. Compared with the MC group, there were 46 differentially expressed proteins after administration of F. velutipes polysaccharide treatment, of which 30 were up-regulated and 16 were down-regulated. The reproducibility of the quantification was assessed by the CV value, CV = standard deviation SD/mean, the lower the value, the better the reproducibility. In Figure 4B, the mean CV value was equal to 0.091 and the percentage of proteins with CV values within 20% was 93.6%, and the results indicated that the biological reproducibility was good between sample groups.
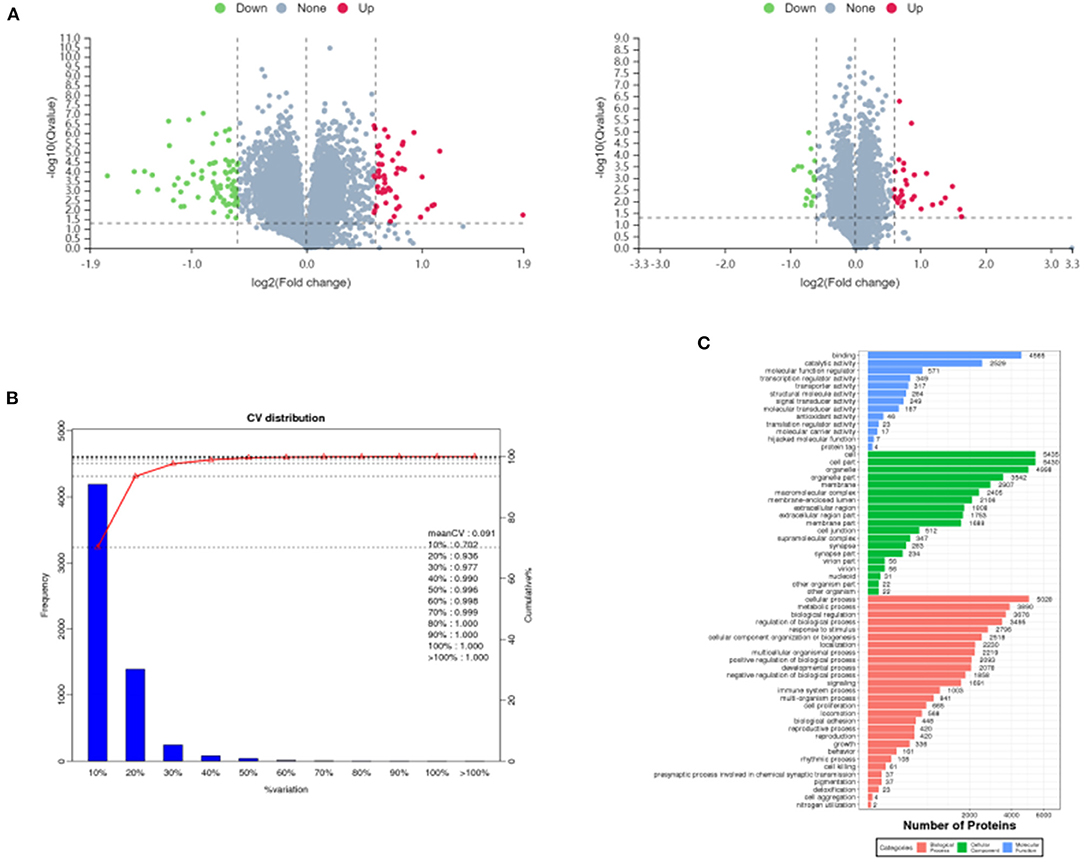
Figure 4. Volcano plots of differentially expressed proteins in MC/BC (left) and HD/MC (right) (A), distribution of CV values of replicate experiments (B) and GO annotation histogram (C).
All the identified proteins were compared with NR database for GO gene function annotation and enrichment analysis, and it was found that they mainly have molecular functions such as binding, catalytic activity, molecular function regulator, transcription regulator activity, etc.; contain cellular components such as cell, cell part, organelle, membrane, macromolecular complex, etc.; participate in cellular process, metabolic process, biological regulation, regulation of biological process, signaling, immune system process and other biological processes, as shown in Figure 4C.
GO Enrichment Analysis of Differential Proteins
The GO entries with significant enrichment of differential proteins were analyzed by clustering in Figure 5A, the horizontal axis represents the GO annotation entries, and the vertical axis represents the up- and down-regulated differential proteins. In Figure 5A, the GO functional classification of differential proteins in the HD group was more up-regulated compared with the MC group, among which cellular process, cell part, cell, binding was the more the GO entries were significantly different. The relationship network was used to observe the relationship between each GOterm in Figure 5B, the significantly enriched GOterms in the HD group and MC group were mainly related to the biological process function.
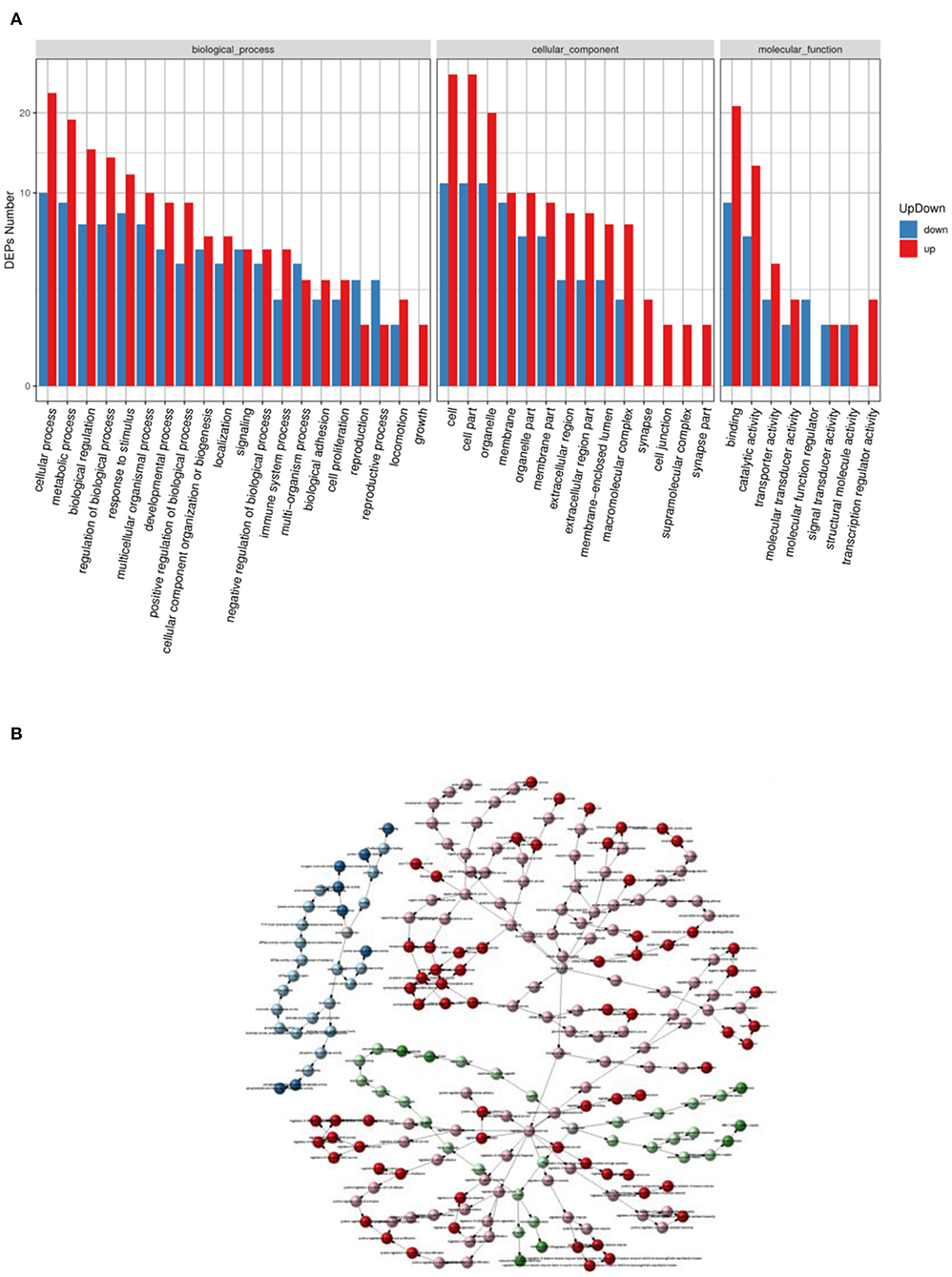
Figure 5. Statistical graph of up- and down-regulation of GO functional classification of differential proteins in HD and MC groups (A) and GOterm relationship network graph (B).
Differential Protein Pathway Enrichment Analysis and Subcellular Localization
Pathway enrichment analysis of differential proteins was performed by the Kyoto Encyclopedia of Genes and Genomes. In Figure 6A, the differentially expressed proteins in the HD and MC groups were mainly involved in transport and catabolism, signal transduction, infectious diseases: viral, immune diseases, carbohydrate metabolism, immune system and other metabolic pathways. The analysis revealed that TFRC (Transferrin receptor protein (1) and RSAD2 (Radical S-adenosyl methionine domain-containing protein (2) protein content changed significantly. The number of differential proteins annotated to the pathway was divided by all the proteins identified to the pathway as the RichFactor, and the larger the value, the larger the proportion of differential proteins in the pathway, and the size of its point represents the number of differential proteins annotated to the pathway. In Figure 6B, differential proteins could play a role in phagosome, antigen processing and presentation, autoimmune thyroid disease, and intestinal immune network for IgA production by participating in the pathway immune modulatory effects.
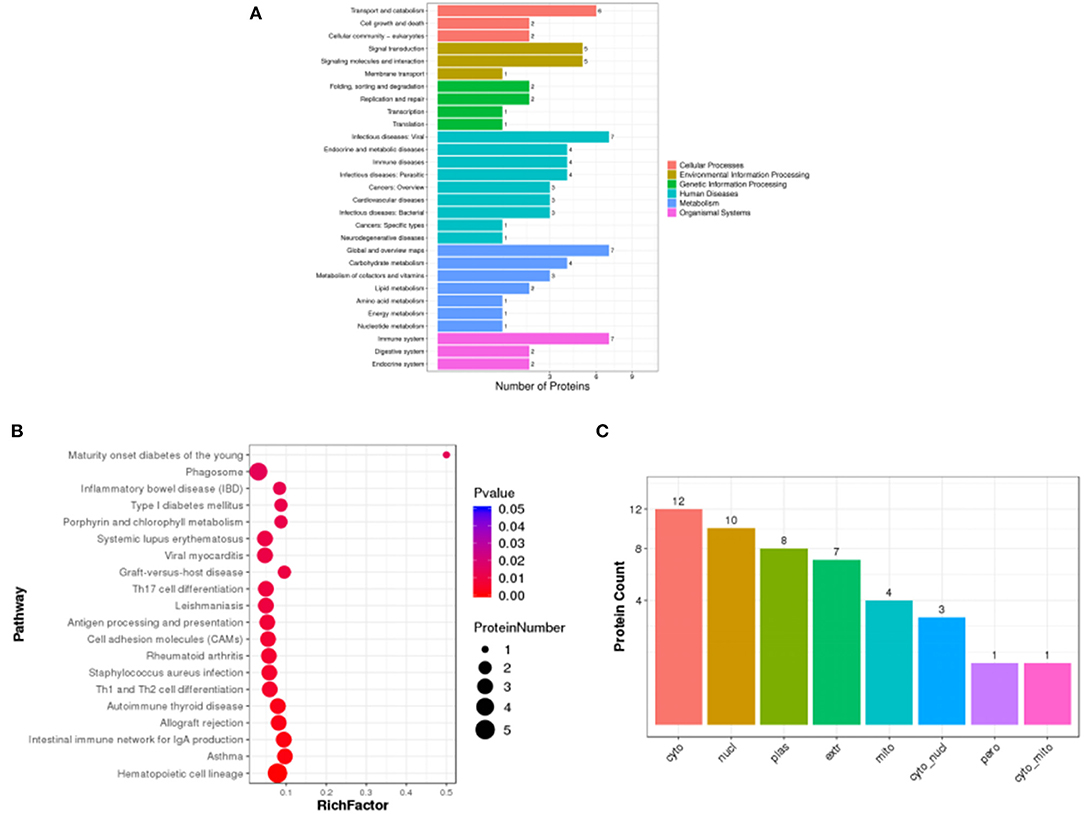
Figure 6. Statistical plots of Pathway classification of differential proteins (A), statistical plots of significantly enriched pathway (B) and histogram of subcellular localization (C) in HD and MC groups.
Subcellular localization of proteins is an important part of protein function annotation. Protein subcellular localization prediction was performed by WoLF PSORT software. In Figure 6C, the differential protein subcellular localization of HD and MC differential proteins were more distributed in cyto (cytosol), nucl (nucleus), plas (plasma membrane) and extr (extracellular).
Protein Validation
In Figure 7, the protein expression in the spleen of TFRC and RSAD2 mice was highly significantly down-regulated in the MC group compared with the BC group (P < 0.001), and highly significantly up-regulated in the HD group compared with the MC group (P < 0.001), which is consistent with the results of proteomics.
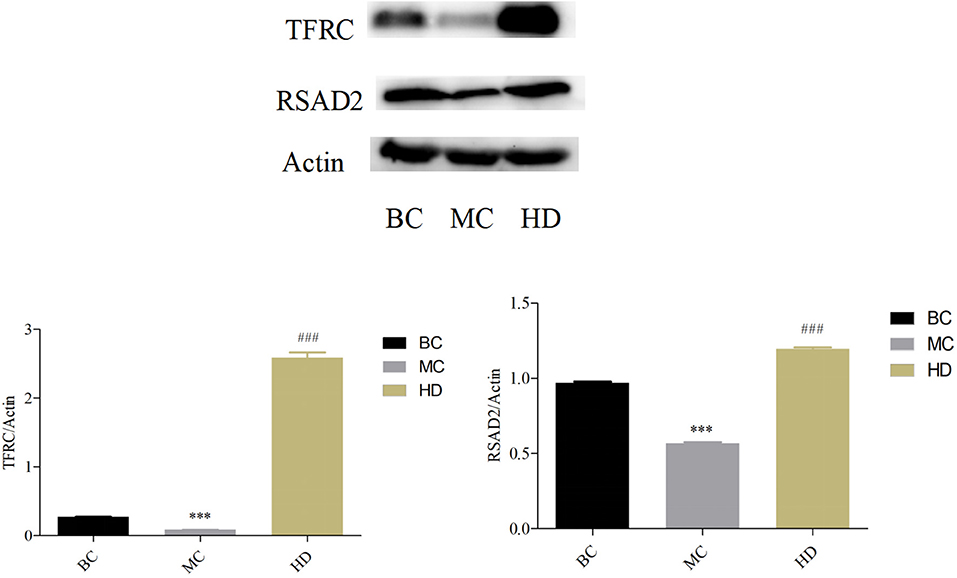
Figure 7. Effect of F. velutipes polysaccharide on the expression levels of TFRC and RSAD2 proteins in mouse spleen. Compared with BC group: ***P < 0.001; Compared with MC group: ###P < 0.001.
Taxonomic Analysis of Intestinal Flora Species
OTUs clustering analysis was performed on mouse intestinal microorganisms, and the Top 30 dominant species were selected for analysis by comparing the database for Silva_132 16S rRNA database. In Figure 8A, at the phylum level, the phylum Firmicutes and the phylum Bacteroidetes were the major dominant groups in the mouse intestinal flora, accounting for more than 95% of all bacteria, followed by the phylum Proteobacteria. The relative abundance of Firmicutes decreased, the relative abundance of Bacteroidetes increased and Proteobacteria showed no significant change in the F. velutipes polysaccharide administration group compared with the MC group.
To further understand the changes in the intestinal flora, the relative abundance of the dominant flora was analyzed at the genus level in Figure 8B. Compared to the MC group, the relative abundance of Lachnospiraceae_NK4A136_group and f_Lachnospiraceae_Unclassified was reduced in the blank and high and medium dose administration groups. While the relative abundance of f_Muribaculaceae_Unclassified, Bacteroides, and Alloprevotella was relatively increased in the HD and MD groups compared with the MC group.
Colony Diversity Analysis and PICRUSt Functional Prediction Analysis
The alpha diversity of the flora was carried out by randomly sampling the sample sequences. Alpha diversity allows assessment of species abundance and diversity. In Figure 9A, the Chao1 index was higher in the HD and LD groups than in the MC group, indicating that the high and low dose groups of F. velutipes polysaccharide could increase the abundance of intestinal flora in immunosuppressed mice. Figure 9B showed that the Shannon index value increased in the LD group compared with the MC group, and there was no significant change in the HD and MD groups, indicating that the low-dose administration group could improve the diversity of intestinal flora in mice.
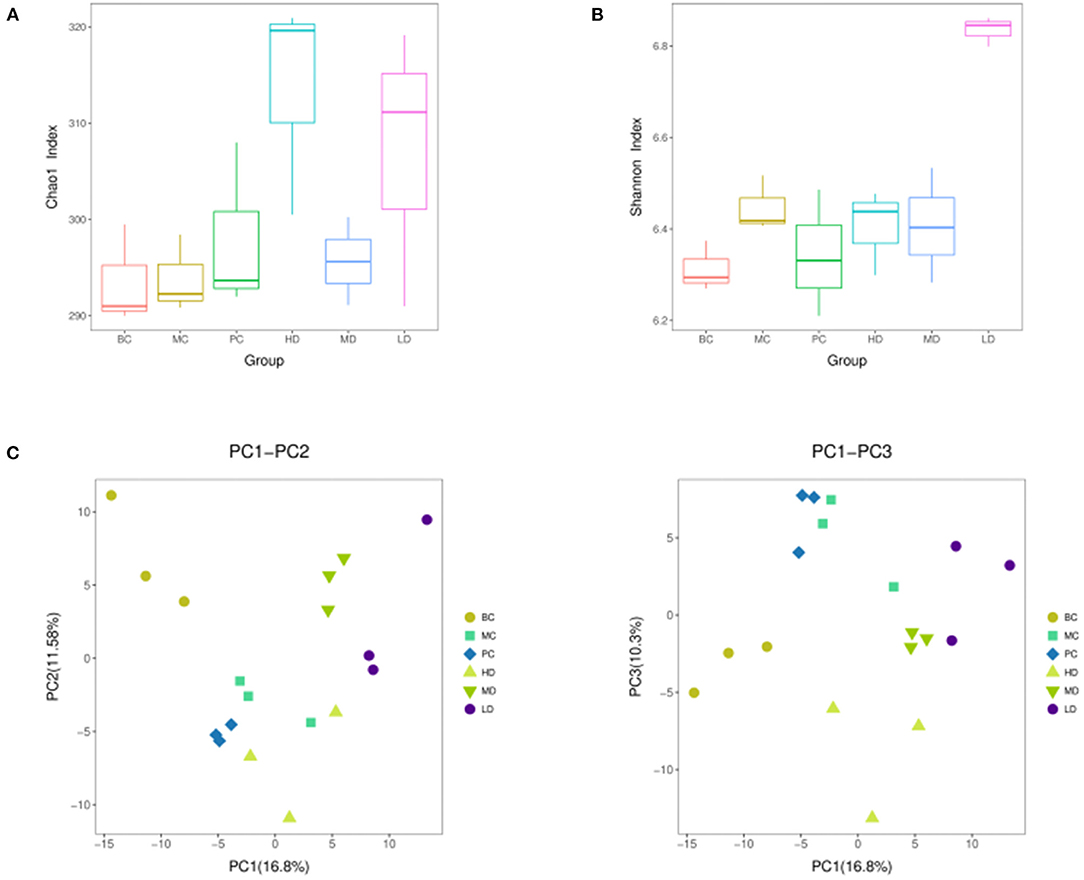
Figure 9. Chao 1 index (A), Shannon index (B) based on alpha diversity analysis, PCA analysis performed on the distribution of strain communities (C).
In order to reflect the diversity differences among different samples, the samples were analyzed by beta diversity between groups. In Figure 9C, the composition of intestinal flora of immunosuppressed mice treated with F. velutipes polysaccharide was significantly different from that of mice in the MC group, indicating that F. velutipes polysaccharide had a good effect on the structural composition of intestinal microorganisms in immunosuppressed mice.
PICRUSt Functional Prediction Analysis
The metabolic functions of the colony were predicted based on the PICRUSt analysis platform. In Figure 10, the metabolic functions of the colony were concentrated in energy production and conversion, amino acid transport and metabolism, carbohydrate transport and metabolism, lipid transport and metabolism, signal transduction mechanisms, etc. It can be predicted that the intestinal flora may play a regulatory role by affecting the signaling pathways of amino acid, carbohydrate, lipid transport and metabolism and signal transduction.
Effect of Flammulina velutipes Polysaccharide on the Content of SCFAs
The effects of F. velutipes polysaccharide on the content of SCFAs were determined by extracting SCFAs from the intestine of mice. In Table 3, compared with BC, the contents of acetic acid, propionic acid and butyric acid were all down-regulated in the MC group, and the contents of acetic acid and butyric acid reached a significant level (P < 0.05), indicating that CTX had an inhibitory effect on the production of SCFAs. Compared with the MC group, the content of SCFAs was improved in both the PC and polysaccharide treatment groups, with the content of acetic acid, propionic acid and butyric acid reaching significant levels in the HD group. It was speculated that F. velutipes polysaccharide might regulate the changes of intestinal microbial composition by improving the content of SCFAs, with the most obvious improvement in the high-dose group.
Discussion
Thymus and spleen are two important immune organs for the proliferation and growth of human immune cells, and the immune organ index is known as the main indicator of immune function (30). It has been shown that polysaccharide can significantly increase thymus index and spleen index in immunosuppressed mice (31, 32). CTX is a chemotherapeutic drug that has a suppressive effect on the body's immune system, which is manifested by a decrease in spleen and thymus index (1). It was found that the thymus and spleen indices of mice in the MC group were significantly lower than those of normal mice, indicating that the immune function of mice was suppressed by CTX, and when the polysaccharide was gavaged, the thymus and spleen indices of mice in each dose group increased to different degrees, and the spleen indices of the polysaccharide administration group and the thymus indices of the HD group increased significantly, and the thymus tissue structure was improved, indicating that F. velutipes polysaccharide could improve the immune organ damage and enhance the immunity of the organism, which is consistent with the results of Zhang et al. (33). Panax japonicus polysaccharide can improve spleen and thymus indices of immunosuppressed mice induced by cyclophosphamide.
Macrophages arise from the differentiation of monocytes and play a unique role in the immune system with the function of activating the innate immune response (34). Macrophage enzyme activity can reflect the functional status of macrophages. ACP is the marker enzyme of macrophage lysosomal enzymes in higher animals, and its activity reflects the degree of macrophage activation. LDH is an enzyme necessary for intracellular glucose enzymes, and the energy required by phagocytes is also derived from glycolysis. Lactic acid produced during LDH enzymes can cause a decrease in intracellular pH in macrophages, which facilitates the immune response of macrophages and is one of the hallmarks of macrophage activation (35). Paecilomyces sinensis polysaccharides can increase the activity of LDH and ACP in rat and human monocytes AMϕ and PMϕ (36). Water-soluble Ginseng marc polysaccharide can activate macrophages by regulating the activity of lysosomal phosphatases, affecting the ability of lysosomal enzymes to respond appropriately to exogenous substances and increasing the proportion of phagocytic macrophages (37). The ACP activity in the HD group and LDH in the polysaccharide administration group were significantly increased after the administration treatment, indicating that ACP and LDH activities in immunocompromised mice can be regulated by the F. velutipes polysaccharide.
Cytokines have an important regulatory role in cell growth, cell differentiation and cell-cell interactions and have a significant effect on the immune inflammatory response (38). In this experiment, IL-2 and IL-4 levels were significantly decrease and TNF-α and IL-6 levels were significantly increase in the MC group compared to the BC group, indicating that CTX has a suppressive effect on the immune function of the body. IL-2 is mainly expressed by Th1 cells and is a soluble factor that mediates T cell proliferation and has an important role in the innate and adaptive immune system (39). IL-4 is mainly expressed by Th2 cells and is an important anti-inflammatory cytokine that plays an important role in humoral immunity and has an important regulatory role in the immune function and inflammatory process of the body. Wen et al. found that low molecular-weight seleno-aminopolysaccharides significantly increased cyclophosphamide-induced secretion of serum cytokines IL-2 and IL-4 in immunosuppressed mice to restore immune function (40). It is consistent with our research, F. velutipes polysaccharides can significantly increase the levels of serum cytokines IL-2 and IL-4 to exert immune modulatory effects. IL-6 and TNF-α have dual effects on the body. Moderate concentrations can enhance the immune function of the organism, regulate a variety of immune cells, and have a protective effect on the organism, but large production and release can disrupt the immune balance of the organism, produce an overly violent immune response, present toxic effects, and cause damage to the organism (41, 42). Cheng et al. (43) showed that Bupleurum smithii var. Parvifolium polysaccharides can inhibit lipopolysaccharide induced production of pro-inflammatory cytokines IL-6 and TNF-α. It has been reported that CTX can induce elevation of inflammatory cytokines TNF-α, IL-6 through activation of nuclear factor-κB (NF-κB) and p38 mitogen-activated protein kinase (p38-MAPK) (44–46). F. velutipes polysaccharides could exert immune modulatory effects by inhibiting the CTX-induced increase in the levels of pro-inflammatory cytokines IL-6 and TNF-α.
CTX causes immunosuppression along with damage to the liver and antioxidant enzymes, induces oxidative stress in the body, generates large amounts of ROS, causes oxidative damage to immune cells (47–49), and further decreases the immune function of the body. Excess ROS leads to an increase in the membrane lipid peroxidation product MDA (50, 51), which reflects the overall level of lipid peroxidation. SOD is an important oxygen radical scavenger widely present in living organisms, catalyzing the disproportionation of superoxide anions and converting them into hydrogen peroxide, which is decomposed into H2O and O2 catalyzed by CAT (52–54). Antioxidant the proteins SOD and CAT can act as cellular detoxification systems to prevent ROS damage (55). T-AOC represents the total antioxidant capacity of the organism. It has been shown that polysaccharides can enhance antioxidant activity in immunosuppressed mice (26). Our research found that F. velutipes polysaccharide could reduce the level of MDA and alleviate oxidative stress in the body by increasing the activity of SOD, CAT and T-AOC, which is consistent with the results of Xu et al. (56).
Proteomics research involves the large-scale detection, identification and characterization of proteins, making it highly promising for biomarker discovery in many diseases (57). Chen et al. (58) investigated the effect of Sargassum fusiforme polysaccharides (SFP) on the antioxidant capacity of liver tissue in mice by proteomics techniques. The effect of SFP on the antioxidant capacity of mouse liver tissues was investigated by proteomic techniques, and 38 out of 49 protein spots were found to be up-regulated and 11 down-regulated. Functional analysis revealed that the differentially expressed proteins were mainly involved in redox, amino acid metabolism and energy metabolism, and the results indicated that SFP could regulate antioxidant enzymes to scavenge excess free radicals and prevent oxidative damage. Jiang et al. investigated the immune modulatory function of Durio zibethinus Rind polysaccharide at the proteomic level and found a total of 13 shared differential proteins by comparing the differential proteins in untreated, immunosuppressed and D. zibethinus Rind polysaccharide-treated mice. These shared differential proteins were mostly associated with biological functions such as lolalization, biological regulation and immune system process. Liang et al. (59) used proteomics to find that Nigella sativa seed polysaccharides could participate in immune regulation by regulating metabolism-related pathways such as Autoimmune thyroid disease, Primary immunodeficiency, and PI3K-Akt signaling pathway through the regulation of differential proteins such as PI3K and PTEN. The aim of this study was to investigate the immune modulatory effects of F. velutipes polysaccharide at the proteomic level through the quantitative technique of homogeneous isotope labeling. By comparing the differential proteins in spleen tissues of different treated mice, 52 differential proteins were up-regulated and 82 differential proteins were down-regulated in the MC group compared with the BC group, and 30 differential proteins were up-regulated and 16 differential proteins were down-regulated in F. velutipes polysaccharide treated group compared with the MC group. These differential proteins were mostly associated with functions such as biological regulation, immune system process and signal transducer activity, and were involved in transport and catabolism, infectious diseases: viral, immune diseases, phagosome and other pathways to play immune regulatory roles. Differential protein function enrichment analysis revealed that among the immune-related proteins, TFRC and RSAD2 were significantly upregulated in HD compared with MC group. Iron is essential for the generation of immune responses and is required for the growth, proliferation and differentiation of immune cells (60). Iron deficiency leads to a decrease in the number of T cells, a lower proportion of mature T cells and suppressed cytokine synthesis (61–63). Cellular iron uptake is largely dependent on iron transporters (64, 65). The transferrin receptor is a cell surface receptor that mediates iron uptake through receptor-mediated endocytosis and is required for cellular iron uptake (66, 67). It has been suggested that mutations in the transferrin receptor gene may lead to severe combined immunodeficiency (68). Promoting the expression of transferrin receptor protein (TFRC) increases cellular iron uptake and helps to enhance host antitumor immunity (69). RSAD2 is a key enzyme of the innate immune response, localized to the cytoplasmic face of the endoplasmic reticulum (70) and/or to lipid droplets via the N-terminal hydrophobic structural domain (71), and its expression is induced by interferon-dependent or non-dependent pathways (72). The inhibition of GAPDH activity by the ddhCTP product of RSAD2 radical SAM activity may improve the rate of NADPH regeneration by affecting upstream metabolic pathways and increasing the flux of the pentose phosphate pathway (PPP). It is also able to increase the rate of reduction of glutathione disulfide (GSSG) to reduced glutathione (GSH), thus protecting cells from reactive oxygen species (ROS) damage (73). In macrophages, the cellular activity of RSAD2 may provide a protective mechanism for cells against viral infection or other conditions that increase ROS levels (74). The results of the present study showed that F. velutipes polysaccharide may alleviate CTX-induced oxidative stress capacity as well as increase the body's iron uptake and immune response through upregulation of TFRC and RSAD2 protein expression, and exert immune modulatory effects through drug metabolism-related pathways such as immune diseases, transport and catabolism, phagosomes and influenza A.
The intestinal flora has an important role in immune system development and regulation of immune function (75). Dysfunctional gut microbial structure affects physiological processes such as energy metabolism, immune regulation and liver injury in humans (76–78). Niu et al. evaluated the effect of Pinus massoniana pollen polysaccharides (PPPS) on the intestinal flora of mice by 16S rRNA high-throughput sequencing technology and showed that PPPS can regulate the composition of mouse intestinal microorganisms and increase the proportion of probiotic bacteria, and also regulate the systemic immune system by modulating the immunosuppressive status of lymphocytes in Peyer's patches (79). N. sativa seed polysaccharides can exert immune modulatory effects by improving the structure of the intestinal flora, increasing flora diversity, and regulating metabolic pathways such as lipid metabolism, polysaccharide synthesis and signal transduction (59). The results of the present study showed that F. velutipes polysaccharides improved the composition and diversity of the intestinal flora of mice in a CTX-induced immunosuppression model. At the phylum level, the thick-walled phylum Firmicutes and Bacteroidetes were the predominant intestinal flora in mice, it is consistent with the Tremaroli et al. research. Bacteroidetes and Firmicutes accounted for more than 90% of the total intestinal microorganisms (80). It has been shown that Bacteroidetes can interact with cellular receptors using lipopolysaccharide and flagellin components to enhance the immune response through cytokine synthesis (81), and that an increase in thick-walled Bacteroidetes promotes energy absorption by the body, leading to obesity (82). In the present study, F. velutipes polysaccharides decreased the relative abundance of Firmicutes and increased the relative abundance of Bacteroidetes, which had a beneficial regulatory effect on the intestinal flora. The literature reports that intestinal microorganisms have a large system of carbohydrate-active enzymes that can further utilize polysaccharides (83). After entering the intestine, polysaccharides are converted into SCFAs by microbial metabolism (84). Short-chain fatty acids are not only an important source of energy for intestinal epithelial cells, but it also regulates the production of inflammatory factors and reduces intestinal inflammation and tumorigenesis (85, 86). Among the various microbial metabolites, acetic acid, butyric acid, and propionic acid are the key bacterial metabolites that promote the development and maintenance of the immune system (87). In the present study, it was found that F. velutipes polysaccharides increased the content of acetic acid, propionic acid, and butyric acid, with the most significant increase in the high-dose administration group. Tan et al. (88) found that the intervention of Bacteroides reduced the destruction of intestinal flora by LPS treatment, maintained the integrity of the intestinal epithelium, had a role in promoting intestinal homeostasis, and its content was proportional to the acetic acid content in short-chain fatty acids (89). Propionate is a health-promoting microbial fermentation metabolite in the human gut that provides energy to the intestine and plays an inhibitory role in the development of disease (90). Butyrate is the preferred source of energy for colon cells and is locally consumed, and has been more extensively studied in inflammation and cancer, where it inhibits colorectal cancer and inflammation (87). Alloprevotella belongs to the genus Bacteroides of the phylum Synechococcus and is SCFA-producers, whose abundance is negatively correlated with obesity and diabetes (91, 92). Lachnospiraceae_NK4A136 group is a discriminatory feature of intestinal dysfunction (93), and Wang et al. (94) studied found that Chrysanthemum morifolium polysaccharides could improve intestinal dysfunction by decreasing the abundance of Lachnospiraceae_NK4A136_group flora and increasing the relative abundance of beneficial bacteria. In the present study, the relative abundance of Lachnospiraceae_NK4A136_group flora was decreased and the relative abundance of Bacteroides and Alloprevotella was increased in the group administered with high and medium doses of F. velutipes polysaccharide compared with the MC group, which regulated the balance of flora in the intestine of mice.
In conclusion, F. velutipes polysaccharide can protect immune organs of immunosuppressed mice, improve serum cytokine levels, enhance the antioxidant capacity of the body, promote the increase of intestinal SCFAs content, regulate the expression of proteins TFRC and RSAD2, promote energy metabolism, increase the abundance of flora, improve the structure of flora and maintain the homeostasis of the intestinal environment. The results suggest that F. velutipes polysaccharide has potential immune modulatory effects.
Data Availability Statement
The original contributions presented in the study are included in the article/supplementary material, further inquiries can be directed to the corresponding author/s.
Ethics Statement
The animal study was reviewed and approved by Ethics Committee of Henan University School of Medicine (HUSOM2021-76).
Author Contributions
QL and QZ: conceptualization, investigation, methodology, software, and writing-original draft preparation. XH and JW: data curation, formal analysis, and visualization. CM and XX: supervision, software, and validation. WK: resources, funding acquisition, project administration, and writing-reviewing and editing. All authors contributed to the article and approved the submitted version.
Funding
This work was supported by Major Public Welfare Projects in Henan Province (201300110200), Research on Precision Nutrition and Health Food, Department of Science and Technology of Henan Province (CXJD2021006), and The Key Project in Science and Technology Agency of Henan Province (212102110019 and 202102110283).
Conflict of Interest
The authors declare that the research was conducted in the absence of any commercial or financial relationships that could be construed as a potential conflict of interest.
Publisher's Note
All claims expressed in this article are solely those of the authors and do not necessarily represent those of their affiliated organizations, or those of the publisher, the editors and the reviewers. Any product that may be evaluated in this article, or claim that may be made by its manufacturer, is not guaranteed or endorsed by the publisher.
References
1. Zhu GX, Jiang Y, Yao Y, Wu N, Luo J, Hu MW, et al. Ovotransferrin ameliorates the dysbiosis of immunomodulatory function and intestinal microbiota induced by cyclophosphamide. Food Funct. (2019) 10:1109–22. doi: 10.1039/C8FO02312C
2. Barzee TJ, Cao L, Pan ZL, Zhang RH. Fungi for future foods. J. Funct Foods. (2021) 1:25–37. doi: 10.1016/j.jfutfo.2021.09.002
3. USDA. Dietary Guidelines for Americans, 2020-2025. 9th ed (2020). Available online at: DietaryGuidelines.gov (accessed August 26, 2021).
4. Yin ZH, Liang ZH, Li CQ, Wang JM, Ma CY, Kang WY. Immunomodulatory effects of polysaccharides from edible fungus: a review. Food Sci Hum Well. (2021) 10:393–400. doi: 10.1016/j.fshw.2021.04.001
5. Yelithao K, Surayot U, Lee CS, Palanisamy S, Prabhu NM, Lee JH, et al. Studies on structural properties and immune-enhancing activities of glycomannans from Schizophyllum commune. Carbohydr Polym. (2019) 218:37–45. doi: 10.1016/j.carbpol.2019.04.057
6. Sheng XT, Yan JM, Meng Y, Kang YY, Han Z, Tai GH, et al. Immunomodulatory effects of Hericium erinaceus derived polysaccharides are mediated by intestinal immunology. Food Funct. (2017) 8:1020–7. doi: 10.1039/C7FO00071E
7. Wu DM, Duan WQ, Liu Y, Cen Y. Anti-inflammatoryeffect of the polysaccharides of golden needle mushroom in burnedrats. Int J Biol Macromol. (2010) 46:100–3. doi: 10.1016/j.ijbiomac.2009.10.013
8. Zhang ZF, Lv GY, He WQ, Shi LG, Pan HJ, Fan LF. Effects of extraction methods on the antioxidant activities of polysaccharides obtained from Flammulina velutipes. Carbohydr Polym. (2013) 98:1524–31. doi: 10.1016/j.carbpol.2013.07.052
9. Yuan FF, Gao Z, Liu WB, Li HP, Zhang YW, Feng Y, et al. Characterization, antioxidant, anti-aging and organ protective effects of sulfated polysaccharides from Flammulina velutipes. Molecules. (2019) 24:3517. doi: 10.3390/molecules24193517
10. Ye JF, Wang XD, Wang K, Deng YD, Yang YC, Ali R, et al. A novel polysaccharide isolated from Flammulina velutipes, characterization, macrophage immunomodulatory activities and its impact on gut microbiota in rats. J Anim Physiol Anim Nutr. (2020) 104:735–48. doi: 10.1111/jpn.13290
11. Xin X, Zheng KW, Niu YY, Song MM, Kang WY. Effect of Flammulina velutipes (golden needle mushroom, eno-kitake) polysaccharides on constipation. Open Chem. (2018) 16:155–62. doi: 10.1515/chem-2018-0017
12. Liang ZH, Zheng KW, Zhao QC, Shao WJ, Li CQ, Wang JM, et al. Structural identification and coagulation effect of Flammulina Velutipes polysaccharides. App Sci. (2021) 11:1736. doi: 10.3390/app11041736
13. Zhao RQ, Fang DL, Ji Y, Chen X, Ma GX, Su AX, et al. In vitro and in vivo functional characterization of an immune activation Flammulina velutipes polysaccharide based on gut microbiota regulation. Food Agric Immunol. (2020) 1:667–86. doi: 10.1080/09540105.2020.1754345
14. Zhao RQ, Hu QH, Ma GX, Su AX, Xie MH, Li XF, et al. Effects of Flammulina velutipes polysaccharide on immune response and intestinal microbiota in mice. J Funct Foods. (2019) 56:255–64. doi: 10.1016/j.jff.2019.03.031
15. Kahn P. From genome to proteome: looking at a cell's proteins. Science. (1995) 270:369–70. doi: 10.1126/science.270.5235.369
16. Pandey A, Mann M. Proteomics to study genes and genomes. Nature. (2000) 405:837–46. doi: 10.1038/35015709
17. Almeida AM, Bassols A, Bendixen E, Bhide M, Ceciliani F, Cristobal S, et al. Animal board invited review: advances in proteomics for animal and food sciences. Animal. (2014) 9:1–17. doi: 10.1017/S1751731114002602
18. Nanjundan M, Byers LA, Carey MS, Siwak DR, Raso MG, Diao LX, et al. Proteomic profiling identifies pathways dysregulated in non-small cell lung cancer and an inverse association of AMPK and adhesion pathways with recurrence. J Thorac Oncol. (2010) 5:1894–904. doi: 10.1097/JTO.0b013e3181f2a266
19. Zhao L, Geng TT, Sun KC, Su S, Zhao Y, Bao N, et al. Proteomic analysis reveals the molecular mechanism of Hippophae rhamnoides polysaccharide intervention in LPS-induced inflammation of IPEC-J2 cells in piglets. Int J Biol Macromol. (2020) 164:3294–304. doi: 10.1016/j.ijbiomac.2020.08.235
20. Yang SF, Zhao ZC, Zhang AY, Jia FJ, Song MX, Huang ZL, et al. Proteomics analysis of chicken peripheral blood lymphocyte in Taishan Pinus massoniana pollen polysaccharide regulation. PloS ONE. (2018) 13:e0208314. doi: 10.1371/journal.pone.0208314
21. Round JL, Mazmanian SK. The gut microbiota shapes intestinal immune responses during health and disease. Nat Rev Immunol. (2009) 9:313–23. doi: 10.1038/nri2515
22. Thomas S, Izard J, Walsh E, Batich K, Chongsathidkiet P, Clarke G, et al. The host microbiome regulates and maintains human health: a primer and perspective for non-microbiologists. Cancer Res. (2017) 77:1783–812. doi: 10.1158/0008-5472.CAN-16-2929
23. Tropini C, Earle KA, Huang KC, Sonnenburg JL. The gut microbiome: connecting spatial organization to function. Cell Host Microbe. (2017) 21:433–42. doi: 10.1016/j.chom.2017.03.010
24. Chen SP, Wang JQ, Fang QY, Nan D, Fang QY, Cui SW, et al. A polysaccharide from natural Cordyceps sinensis regulates the intestinal immunity and gut microbiota in mice with cyclophosphamide-induced intestinal injury. Food Funct. (2021) 12:6271–82. doi: 10.1039/D1FO00596K
25. Chen QQ, Ren RR, Zhang QQ, Wu JJ, Zhang YF, Xue MS, et al. Coptis chinensis Franch polysaccharides provide a dynamically regulation on intestinal microenvironment, based on the intestinal flora and mucosal immunity. J Ethnopharmacol. (2020) 267:113542. doi: 10.1016/j.jep.2020.113542
26. Niu YY, Dong J, Jiang HM, Wang JM, Liu ZH, Ma CY, et al. Effects of polysaccharide from Malus halliana Koehne flowers in cyclophosphamide-induced immunosuppression and oxidative stress on mice. Oxidative Med Cell Longev. (2020) 2020:1603735. doi: 10.1155/2020/1603735
27. Jiang HM, Wang JM, Liang QX, Jiang SJ, Ma CY, Zhang, et al. Mechanism of intestinal flora and proteomics on regulating immune function of Durio zibethinus rind polysaccharide. Oxid Med Cell Longev. (2021) 2021:6614028. doi: 10.1155/2021/6614028
28. Jiang HM, Dong J, Jiang SJ, Liang QX, Zhang Y, Liu ZH, et al. Effect of Durio zibethinus rind polysaccharide on functional constipation and intestinal microbiota in rats. Food Res Int. (2020) 136:109316. doi: 10.1016/j.foodres.2020.109316
29. Dong J, Liang QX, Niu Y, Jiang SJ, Zhou L, Wang JM, et al. Effects of Nigella sativa seed polysaccharides on type 2 diabetic mice and gut microbiota. Int J Biol Macromol. (2020) 159:725–38. doi: 10.1016/j.ijbiomac.2020.05.042
30. Sun H, Ni XQ, Song X, Wen B, Zhou Y, Zou FQ, et al. Fermented Yupingfeng polysaccharides enhance immunity by improving the foregut microflora and intestinal barrier in weaning rex rabbits. Appl Microbiol Biotechnol. (2016) 100:8105–20. doi: 10.1007/s00253-016-7619-0
31. Gong Y, Wu J, Li ST. Immuno-enhancement effects of Lycium ruthenicum Murr. polysaccharide on cyclophosphamide-induced immunosuppression in mice. Int J Clin Exp Med. (2015) 8:20631–7.
32. Li WJ, Li L, Zhen WY, Wang LF, Pan M, Lv JQ, et al. Ganoderma atrum polysaccharide ameliorates ROS generation and apoptosis in spleen and thymus of immunosuppressed mice. Food Chem Toxicol. (2017) 99:199–208. doi: 10.1016/j.fct.2016.11.033
33. Zhang J, Li CY, Li JP, Guo R, Wang H, Pan J, et al. Immunoregulation on mice of low immunity and effects on five kinds of human cancer cells of Panax japonicus polysaccharide. Evid Based Complement Altern Med. (2015) 2015:839697. doi: 10.1155/2015/839697
34. Cui LN, Chen L, Yang G, Li YK, Qiao ZH, Liu Y, et al. Structural characterization and immunomodulatory activity of a heterogalactan from Panax ginseng flowers. Food Res Int. (2021) 140:109859. doi: 10.1016/j.foodres.2020.109859
35. Chen HR. Study on immunological enhancemen and free radical pharmacology of potentilla anserine polysaccharide. [dissertation]. Gansu Agricultural University, Lanzhou, China (2008).
36. Chen XM, Lu JX, Zhang YD, He JT, Guo XZ, Tian GY, et al. Studies of macrophage immuno-modulating activity of polysaccharides isolated from Paecilomyces tenuipes. Int J Biol Macromol. (2008) 43:252–6. doi: 10.1016/j.ijbiomac.2008.06.004
37. Lim TS, Na K, Choi EM, Chung JY, Hwang JK. Immunomodulating activities of polysaccharides isolated from Panax ginseng. J Med Food. (2004) 7:1–6. doi: 10.1089/109662004322984626
38. Aringer M, Smolen JS. Cytokine expression in lupus kidneys. Lupus. (2005) 14:13–18. doi: 10.1191/0961203305lu2053oa
39. Yang Y, Lundqvist A. Immunomodulatory effects of IL-2 and IL-15; implications for cancer immunotherapy. Cancers. (2020) 12:3586. doi: 10.3390/cancers12123586
40. Wen ZS, Tang Z, Gu LX, Xiang XW, Qu YL. Immunomodulatory effect of low molecular-weight seleno-aminopolysaccharide on immunosuppressive mice. Int J Biol Macromol. (2019) 123:1278–88. doi: 10.1016/j.ijbiomac.2018.10.099
41. Baumeister D, Akhtar R, Ciufolini S, Pariante CM, Mondelli V. Childhood trauma and adulthood inflammation: a meta-analysis of peripheral C-reactive protein, interleukin-6 and tumour necrosis factor-α. Mol Psychiatry. (2016) 21:642–9. doi: 10.1038/mp.2015.67
42. Cleynen I, Moerkercke WV, Billiet T, Vandecandelaere P, Vande Casteele NV, Breynaert C, et al. Characteristics of skin lesions associated with anti-tumor necrosis factor therapy in patients with inflammatory bowel disease: a cohort study. Ann Intern Med. (2016) 164:10–22. doi: 10.7326/M15-0729
43. Cheng XQ, Li H, Yue XL, Xie J, Zhang YY, Di HY, et al. Macrophage immunomodulatory activity of the polysaccharides from the roots of Bupleurum smithii var. parvifolium. J Ethnopharmacol. (2010) 130:363–8. doi: 10.1016/j.jep.2010.05.019
44. El-Kholy AA, Elkablawy MA, El-Agamy DS. Lutein mitigates cyclophosphamide induced lung and liver injury via NF-κB/MAPK dependent mechanism. Biomed Pharmacother. (2017) 92:519–27. doi: 10.1016/j.biopha.2017.05.103
45. Iqubal A, Syed MA, Haque MM, Najmi AK, Ali J, Haque SE. Effect of nerolidol on cyclophosphamide-induced bone marrow and hematologic toxicity in Swiss albino mice. Exp Hematol. (2020) 82:24–32. doi: 10.1016/j.exphem.2020.01.007
46. Iqubal A, Syed MA, Najmi AK, Azam F, Barreto GE, Iqubal MK, et al. Nano-engineered nerolidol loaded lipid carrier delivery system attenuates cyclophosphamide neurotoxicity-Probable role of NLRP3 inflammasome and caspase-1. Exp Hematol. (2020) 334:113464. doi: 10.1016/j.expneurol.2020.113464
47. Tong J, Mo QG, Ma BX, Ge LL, Zhou G, Wang YW. The protective effects of Cichorium glandulosum seed and cynarin against cyclophosphamide and its metabolite acrolein-induced hepatotoxicity in vivo and in vitro. Food Funct. (2017) 8:209–19. doi: 10.1039/C6FO01531J
48. Singh C, Prakash C, Tiwari KN, Mishra S, Kumar V. Premna integrifolia ameliorates cyclophosphamide-induced hepatotoxicity by modulation of oxidative stress and apoptosis. Biomed Pharmacother. (2018) 107:634–43. doi: 10.1016/j.biopha.2018.08.039
49. Nafees S, Ahmad ST, Arjumand W, Rashid S, Ali N, Sultana S. Modulatory effects of gentisic acid against genotoxicity and hepatotoxicity induced by cyclophosphamide in Swiss albino mice. J Pharm Pharmacol. (2012) 64:259–67. doi: 10.1111/j.2042-7158.2011.01393.x
50. Shi AM, Shi HT, Wang Y, Liu X, Cheng Y, Li H, et al. Activation of Nrf2 pathway and inhibition of NLRP3 inflammasome activation contribute to the protective effect of chlorogenic acid on acute liver injury. Int Immunopharmacol. (2018) 54:125–30. doi: 10.1016/j.intimp.2017.11.007
51. Lu Y, Hu DM, Ma SB, Zhao X, Wang S, Wei G, et al. Protective effect of wedelolactone against CCl4-induced acute liver injury in mice. Int Immunopharmacol. (2016) 34:44–52. doi: 10.1016/j.intimp.2016.02.003
52. Kebieche M, Lakroun Z, Lahouel M, Bouayed J, Meraihi Z, Soulimani R. Evaluation of epirubicin-induced acute oxidative stress toxicity in rat liver cells and mitochondria, and the prevention of toxicity through quercetin administration. Exp Toxicol Pathol. (2009) 61:161–7. doi: 10.1016/j.etp.2008.06.002
53. Wang QF, Nie P, Hou YH, Wang YT. Purification, biochemical characterization and DNA protection against oxidative damage of a novel recombinant superoxide dismutase from psychrophilic bacterium Halomonas sp. ANT108. Protein Expres Purif. (2020) 173:105661. doi: 10.1016/j.pep.2020.105661
54. Hou RL, Liu X, Wu XP, Zheng MF, Fu JS. Therapeutic effect of natural melanin from edible fungus Auricularia auricula on alcohol-induced liver damage in vitro and in vivo. Food Sci Hum Well. (2021) 10:514–22. doi: 10.1016/j.fshw.2021.04.014
55. Lin XH, Yang F, Huang J, Jiang S, Tang YP, Li JR. Ameliorate effect of pyrroloquinoline quinone against cyclophosphamide-induced nephrotoxicity by activating the Nrf2 pathway and inhibiting the NLRP3 pathway. Life Sci. (2020) 256:117901. doi: 10.1016/j.lfs.2020.117901
56. Xu YY, Li YH, Lu YX Feng XB Tian GT, Liu QH. Antioxidative and hepatoprotective activities of a novel polysaccharide (LSAP) from Lepista sordida mycelia, Food Sci Hum Well. (2021) 10:536–44. doi: 10.1016/j.fshw.2021.04.016
57. Chan PP, Wasinger VC, Leong RW. Current application of proteomics in biomarker discovery for inflammatory bowel disease. World J Gastrointest Pathophysiol. (2016) 7:27–37. doi: 10.4291/wjgp.v7.i1.27
58. Chen PC, Zhang Y, Xu M, Chen HJ, Zou HX, Zhang X, et al. Proteomic landscape of liver tissue in old male mice that are long-term treated with polysaccharides from Sargassum fusiforme. Food Funct. (2020) 11:3632. doi: 10.1039/D0FO00187B
59. Liang QX, Dong J, Wang SY, Shao WJ, Ahmed AF, Zhang Y, et al. Immunomodulatory effects of Nigella sativa seed polysaccharides by gut microbial and proteomic technologies. Int J Biol Macromol. (2021) 184:483–496. doi: 10.1016/j.ijbiomac.2021.06.118
60. Beard JL. Iron biology in immune function, muscle metabolism and neuronal functioning. J Nutr. (2001) 131:568–80S. doi: 10.1093/jn/131.2.568S
61. Bonaccorsi-Riani E, Danger R, Lozano JJ, Martinez-Picola M, Kodela E, Mas-Malavila R, et al. Iron deficiency impairs intra-hepatic lymphocyte mediated immune response. PLoS ONE. (2015) 10:e0136106. doi: 10.1371/journal.pone.0136106
62. Kuvibidila SR, Velez M, Gardner R, Penugonda K, Chandra LC, Yu L. Iron deficiency reduces serum and in vitro secretion of interleukin-4 in mice independent of altered spleen cell proliferation. Nutr Res. (2012) 32:107–15. doi: 10.1016/j.nutres.2011.12.005
63. Kuvibidila SR, Kitchens D, Baliga BS. In vivo and in vitro iron deficiency reduces protein kinase C activity and translocation in murine splenic and purified T cells. J Cell Biochem. (1999) 74:468–78.
64. Arosio P, Elia L, Poli M. Ferritin, cellular iron storage and regulation. IUBMB Life. (2017) 69:414–22. doi: 10.1002/iub.1621
65. Gao GF, Li J, Zhang YT, Chang YZ. Cellular iron metabolism and regulation. Adv Exp Med Biol. (2019) 1173:21–32. doi: 10.1007/978-981-13-9589-5_2
66. Levy JE, Jin O, Fujiwara Y, Kuo F, Andrews NC. Transferrin receptor is necessary for development of erythrocytes and the nervous system. Nat. Genet. (1999) 21:369. doi: 10.1038/7727
67. Shen Y, Li X, Dong DD, Zhang B, Xue YR, Shang P, Transferrin receptor 1 in cancer: a new sight for cancer therapy. Am J Cancer Res. (2018) 8:916–31.
68. Jabara HH, Boyden SE, Chou J, Ramesh N, Massaad MJ, Benson H, et al. A missense mutation in TFRC, encoding transferrin receptor 1, causes combined immunodeficiency. Nat Genet. (2016) 48:74–8. doi: 10.1038/ng.3465
69. Song J, Liu TT, Yin Y, Zhao W, Lin ZQ, Yin YX, et al. The deubiquitinase OTUD1 enhances iron transport and potentiates host antitumor immunity. EMBO Rep. (2021) 22:e51162. doi: 10.15252/embr.202051162
70. Hinson ER, Cresswell P. The N-terminal amphipathic a-helix of viperin mediates localization tothe cytosolic face of the endoplasmic reticulum and inhibits protein secretion. J Biol Chem. (2009) 284:4705–4712. doi: 10.1074/jbc.M807261200
71. Hinson ER, Cresswell P. The antiviral protein, viperin, localizes to lipid droplets via its Nterminal amphipathic a-helix. Proc Natl Acad Sci USA. (2009) 106:20452–7. doi: 10.1073/pnas.0911679106
72. Severa M, Coccia EM, Fitzgerald KA. Toll-like receptor-dependent and -independent viperin gene expression and counter-regulation by PRDI-binding factor-1/BLIMP1. J Biol Chem. (2006) 281:26188–95. doi: 10.1074/jbc.M604516200
73. Ebrahimi KH, Vowles J, Browne C, McCullagh J, James WS. ddhCTP produced by the radical-SAM activity of RSAD2 (viperin) inhibits the NAD+-dependent activity of enzymes to modulate metabolism. FEBS Lett. (2020) 594:1631–44. doi: 10.1002/1873-3468.13778
74. Schwarz KB. Oxidative stress during viral infection: a review. Free Radic Biol Med. (1996) 21:641–9. doi: 10.1016/0891-5849(96)00131-1
75. Kamada N, Seo SU, Chen GY, Núñez G. Role of the gut microbiota in immunity and inflammatory disease. Nat Rev Immunol. (2013) 13:321–35. doi: 10.1038/nri3430
76. Zhang XW, Ma CW, Xu PP, Tang J, Xu XF. Gut microbiota, host health, and polysaccharides. Biotechnol Adv. (2013) 31:237–318. doi: 10.1016/j.biotechadv.2012.12.009
77. Liu CJ, Cheng LY, Ji LL, Li F, Zhan YX, Wu BT, et al. Intestinal microbiota dysbiosis play a role in pathogenesis of patients with primary immune thrombocytopenia. Thromb Res. (2020) 190:11–19. doi: 10.1016/j.thromres.2020.03.012
78. Hong YZ, Shen MY, Huang LX, Wu T, Xie JH. Mesona chinensis Benth polysaccharides alleviates liver injury by beneficial regulation of gut microbiota in cyclophosphamide-induced mice. Food Sci Hum Well. (2022) 11:74–84. doi: 10.1016/j.fshw.2021.07.009
79. Niu XY, Shang HQ, Chen SY, Chen RC, Huang J, Miao YQ, et al. Effects of Pinus massoniana pollen polysaccharides on intestinal microenvironment and colitis in mice. Food Funct. (2020) 12:252–66. doi: 10.1039/D0FO02190C
80. Tremaroli V, Bäckhed F. Functional interactions between the gut microbiota and host metabolism. Nature. (2012) 489:242–9. doi: 10.1038/nature11552
81. Stojanov S, Berlec A, Štrukelj B. The influence of probiotics on the Firmicutes/Bacteroidetes ratio in the treatment of obesity and inflammatory bowel disease. Microorganism. (2020) 8:1715–31. doi: 10.3390/microorganisms8111715
82. Roy TL, Llopis M, Lepage P, Bruneau A, Rabot S, Bevilacqua C, et al. Intestinal microbiota determines development of non-alcoholic fatty liver disease in mice. Gut. (2013) 62:1787–94. doi: 10.1136/gutjnl-2012-303816
83. Kaoutari AE, Armougom F, Gordon JI, Raoult D, Henrissat B. The abundance and variety of carbohydrate-active enzymes in the human gut microbiota. Nat. Rev. Microbiol. (2013) 11:497–504. doi: 10.1038/nrmicro3050
84. Lovegrove A, Edwards CH, Noni ID, Patel H, EI SN, Grassby T, et al. Role of polysaccharides in food, digestion, and health. Crit Rev Food Sci. (2017) 57:237. doi: 10.1080/10408398.2014.939263
85. Donohoe DR, Garge N, Zhang XX, Sun W, O'Connell TM, Bunger MK, et al. The microbiome and butyrate regulate energy metabolism and autophagy in the mammalian colon. Cell Metab. (2011) 13:517–26. doi: 10.1016/j.cmet.2011.02.018
86. Inagaki A, Sakata T. Dose-dependent stimulatory inhibitory effects of luminal serosal n-butyric acid on epithelial cell proliferation of pig distal colonic mucosa. J Nutr Sci Vitaminol. (2005) 51:156–60. doi: 10.3177/jnsv.51.156
87. Koh A, Vadder F D, Kovatcheva-Datchary P, Bäckhed F. From dietary fiber to host physiology: short-chain fatty acids as key bacterial metabolites. Cell. (2016) 165:1332–45. doi: 10.1016/j.cell.2016.05.041
88. Tan HZ, Zhao JX, Zhang H, Zhai QX, Chen W. Novel strains of Bacteroides fragilis and Bacteroides ovatus alleviate the LPS-induced inflammation in mice. Appl Microbiol Biotechnol. (2019) 103:2353–65. doi: 10.1007/s00253-019-09617-1
89. Rui Y, Wan P, Chen GJ, Xie MH, Sun Y, Zeng XX, et al. Simulated digestion and fermentation in vitro by human gut microbiota of intra- and extra-cellular polysaccharides from Aspergillus cristatus. LWT Food Sci Technol. (2019) 116:108508–17. doi: 10.1016/j.lwt.2019.108508
90. Hosseini E, Grootaert C, Verstraete W, Wiele TV. Propionate as a health-promoting microbial metabolite in the human gut. Nutr. Rev. (2011) 69:245–58. doi: 10.1111/j.1753-4887.2011.00388.x
91. Hu RK, Guo WL, Huang ZR, Li L, Liu B, Lv XC, et al. Anti-diabetic effect of aloin via JNK-IRS1/PI3K pathways and regulation of gut microbiota. J Funct Foods. (2018) 46:403–12. doi: 10.1016/j.jff.2018.05.020
92. Shang QS, Song GR, Zhang MF, Shi JJ, Xu CY, Hao JJ, et al. Dietary fucoidan improves metabolic syndrome in association with increased Akkermansia population in the gut microbiota of high-fat diet-fed mice. J Funct Foods. (2017) 28:138–46. doi: 10.1016/j.jff.2016.11.002
93. Caparrós-Martín JA, Lareu RR, Ramsay JP, Peplies J, Reen FJ, Headlam HA, et al. Statin therapy causes gut dysbiosis in mice through a PXR-dependent mechanism. Microbiome. (2017) 5:95. doi: 10.1186/s40168-017-0312-4
Keywords: Flammulina velutipes, Flammulina velutipes polysaccharide, immunomodulation, proteomics, intestinal flora
Citation: Liang Q, Zhao Q, Hao X, Wang J, Ma C, Xi X and Kang W (2022) The Effect of Flammulina velutipes Polysaccharide on Immunization Analyzed by Intestinal Flora and Proteomics. Front. Nutr. 9:841230. doi: 10.3389/fnut.2022.841230
Received: 22 December 2021; Accepted: 06 January 2022;
Published: 28 January 2022.
Edited by:
Bin Du, Hebei Normal University of Science and Technology, ChinaCopyright © 2022 Liang, Zhao, Hao, Wang, Ma, Xi and Kang. This is an open-access article distributed under the terms of the Creative Commons Attribution License (CC BY). The use, distribution or reproduction in other forums is permitted, provided the original author(s) and the copyright owner(s) are credited and that the original publication in this journal is cited, in accordance with accepted academic practice. No use, distribution or reproduction is permitted which does not comply with these terms.
*Correspondence: Changyang Ma, bWFjYXlhMTAyNEBzaW5hLmNvbQ==; Xuefeng Xi, eHVlZmVuZzM1MDI4NkBzaW5hLmNvbQ==; Wenyi Kang, a2FuZ3dlbnlAaG90bWFpbC5jb20=
†These authors have contributed equally to this work