- 1Department of Food Technology Research, National Nutrition and Food Technology Research Institute, Shahid Beheshti University of Medical Sciences, Tehran, Iran
- 2Food Safety Research Center (Salt), Semnan University of Medical Sciences, Semnan, Iran
Due to the concern of consumers about the presence of synthetic preservatives, researchers and food manufacturers have recently conducted extensive research on the limited use of these preservatives and the introduction and use of natural preservatives, such as herbal extracts and essential oils, bacteriocins, and antimicrobial enzymes. Lysozyme is a natural enzyme with antimicrobial activity that has attracted considerable attention to be potentially utilized in various industries. Since lysozyme is an intrinsic component of the human immune system and has low toxicity; it could be considered as a natural antimicrobial agent for use in food and pharmaceutical industries. Lysozyme exerts antimicrobial activity against microorganisms, especially Gram-positive bacteria, by hydrolyzing 1,4-beta-linkages between N-acetylmuramic acid and N-acetylglucosamine in the cell wall. In addition, increased antimicrobial activity of lysozyme against Gram-negative bacteria could be achieved by the modification of lysozyme through physical or chemical interactions. Lysozyme is presented as a natural preservative in mammalian milk and can be utilized as a bio-preservative in dairy products, such as cheese. Both bacteria and fungi can contaminate and spoil the cheese; especially the one that is made traditionally by raw milk. Furthermore, uncontrolled and improper processes and post-pasteurization contamination can participate in the cheese contamination. Therefore, besides common preservative strategies applied in cheese production, lysozyme could be utilized alone or in combination with other preservative strategies to improve the safety of cheese. Hence, this study aimed to review the antimicrobial properties of lysozyme as natural antimicrobial enzyme and its functionality in cheese.
Introduction
Food safety is considered as one of the most important challenges in the food industry. Microbial contamination and the presence of spoilage microorganism and especially the pathogens is known as one of the major concerns in food safety. Therefore, foodstuff production free from any microbial contamination is one of the priorities of food manufacturers and regulatory organizations (1, 2). In this regard, various thermal and non-thermal preservation strategies have been utilized to control or destroy microorganisms to ensure the safety of raw or processed food (3, 4). Furthermore, various preservatives, such as synthetic antimicrobial agents as supplementary approaches have been applied to hinder the microbial contamination of food products during processing, distribution, and storage. Although these synthetic antimicrobial agents are considered generally recognized as safe (GRAS), there has been a growing concern about using synthetic additives by consumers (5, 6). Therefore, attention has been increased to use various natural additives, such as sweeteners, coloring, antioxidants and antimicrobials agents obtained from microorganisms, plants, and animal (7, 8). Various natural antimicrobial agents, such as essential oils and herbal extract, bacteriocin, and antimicrobial enzymes have been identified (9–11).
Antimicrobial enzymes are widespread and have a key role in defending living organisms against bacterial attacks. These enzymes are now growingly used against microbial systems. As a group, they exert antimicrobial activity by directly attacking microorganisms, interfering with biofilm formation, biofilm destruction, and/or catalyzing reactions that resulted in the production of antimicrobial compounds. Different proteolytic, oxidative, and polysaccharide degrading enzymes are utilized against antimicrobial and antibiofilm systems (12). Various categories of antimicrobial enzymes are shown in Figure 1. One of these categories, as mentioned, is polysaccharide degrading enzymes, among which alginate lysase, amylase dispersin B, and lysozyme, are the most widely used.
Lysozyme, also called muramidase or N-acetylmuramic hydrolase, is a small, monomeric enzyme that hydrolyzes the β-1,4-linkages between N-acetyl-d-glucosamine and N-acetylmuramic acid residues in the peptidoglycan of bacterial cell walls (13). Lysozyme was discovered by Alexander Fleming, who accidentally recognized that a drop of his nasal mucosa caused the lysis of bacteria leading to discover a significant bacteriolytic element called lysozyme (14). Further investigations confirmed this finding, and at present, it is known that lysozyme exists in large quantities in tears, saliva, blood serum, human and cow milk, egg white of birds, and approximately in certain bacteria and bacteriophages (15–17). Lysozyme was the first enzyme that its primary amino acid sequence was characterized. Additionally, it was the first enzyme that its structure was defined by X-ray crystallography (18, 19). Lysozyme exerts antimicrobial activity against microorganisms, especially Gram-positive bacteria by hydrolyzing 1,4-beta-linkages in the bacterial cell walls. Hence this enzyme could be utilized in medical, pharmaceutical, and especially in the food industry as a natural food additives to control the bacterial growth in food products (20, 21). In the terms of availability, the chicken egg white is the most acceptable source of lysozyme. It has been widely reported that hen egg white lysozyme is generally utilized as a preservative in various products, such as fruits and vegetables, meat, milk, and dairy products (17, 22).
Since lysozyme is an intrinsic component of the human immune system, it seems that it has low toxicity in humans. It is specific for bacterial peptidoglycans, and therefore, no reaction between lysozyme and human tissue occurs (23). Since lysozyme is presented as a natural preservative in mammalian milk and due to the absorption of lysozyme by casein micelles and maintaining the activity of enzyme after absorption, this enzyme is likely to be appropriate bio-preservative in dairy products (24, 25). Among these, cheese, as one of the most popular dairy products, could be contaminated by various spoilage and pathogen microorganisms. Both bacteria and fungi can contaminate and spoil the cheese; especially the one that is made traditionally by raw milk (8, 26). Additionally, cheese could be contaminated by various microorganisms by post-pasteurization contaminants when the process is not properly controlled. Listeria monocytogenes, Salmonella, Escherichia coli, Staphylococcus aureus, and Bacillus cereus can be named as the most important food pathogen found in contaminated cheese (8, 27). Besides the concern about the presence of food pathogens, the spoilage of cheese may also occur, especially during ripening, such as late blowing by Clostridium tyrobutyricum (28). Therefore, it is required to prevent or control the microbial contamination of cheese during production, ripening, and storage. Apart from common preservative strategies applied in cheese production, there has been a growing interest in the application of natural antimicrobial agents, such as lysozyme alone or in combination with other preservative strategies (27, 29, 30). It has been stated that lysozyme could be applied to protect cheese against the development of off-flavors and curdling created by B. cereus and late blowing produced by C. tyrobutyricum (30–32). In this regard, this study aimed to review the antimicrobial activity of lysozyme as natural antimicrobial enzyme and its functionality in cheese.
Structure, Type and Properties of Lysozyme
One of the polysaccharide-degrading enzymes is lysozyme (E.C 3.2.1.17), and its structure was first illuminated in the 1960s by X-ray crystallography (12). Lysozyme is a glycosidase (a type of murein hydrolases) with a molecular weight of almost 11–22 kDa and the pH of isoelectric point of approximately 9.5–11 (15). This enzymatic protein is known as muramidase or N-acetyl muramide glycanohydrolases. Lysozyme is found in various sources, such as all living organisms, but avian egg whites, human and cow milk, mucus, tears, and saliva are the main sources (33). Lysozyme from hen egg white (HEWL) is considered as a major industrial source for practical uses because of its availability and cost-effectiveness. There are 0.3–0.4 g of lysozyme in each chicken egg (3.5% of total egg white proteins) with maximum enzymatic activity at pH of around 5 (21, 34).
Lysozyme can split the bond between N-acetyl-D-glucosamine and N-acetyl-muramic acid residues of the peptidoglycan chains and thus act as an antimicrobial agent, especially against Gram-positive bacteria (21). In terms of its structure, lysozyme has 129 amino acids cross-linked by disulfide bridges in four different places of a single polypeptide chain. N-terminal and C-terminal amino acids in lysozyme structure are lysine and leucine, respectively (15, 35). The high thermal stability of lysozyme is due to the presence of the six helix regions and disulfide bridges in its structure. The α- and β-domain are two main domains in the polypeptide chain of lysozyme and the binding site is located between them in a large crevice. The active site consists of the amino acids of glutamic acid 35 (Glu35) and aspartate 52 (Asp52) that have important roles in lysozyme’s catalytic activities. Glu-35 gives a proton to the glycosidic oxygen, thus creating oxonium and carbenium ions and cleaving the substrate’s C–O bond. The carbenium ion interacts with the negatively charged Asp52 residue and forms the glycosyl intermediate. Then, the surrounding water provides a hydroxyl ion to combine with the carbenium ion and the lysozyme is separated from the glycosyl–enzyme intermediate. Thereby, the lysozyme molecule remains unchanged and the substrate is hydrolyzed (20).
Based on the differences in amino acid sequences and structural properties (tertiary structures), lysozymes are divided into three main types: c-type, g-type, and i-type (36). The properties of each type of lysozyme are presented in Table 1. The first type of lysozyme has been categorized as the chicken or conventional type (c-type), which human lysozyme and chicken egg-white lysozyme are related to this category. Although human lysozyme has shown higher antibacterial activity and thermal stability than lysozyme isolated from hen egg-white, its utilization has been limited due to its restricted available resources (33). The g-type lysozyme is a characteristic of goose egg-whites that its molecular weight is 21 kDa. Lysozymes of g-type were also found in rhea, cassowary, ostrich, black swan, and some fish species (37). The amount of cysteine and tryptophan is low in typical g-type lysozymes, which causes their thermal instability (38). Moreover, i-type lysozymes are the lysozymes isolated from invertebrates that are smaller than the lysozymes of g-type. The lysozymes of i-type have been identified in Nematoda, Mollusca, insects, Crustacea, Echinodermata, and Annelida (37). Although different types of lysozymes may be present in the same species simultaneously, they have different enzymatic and biochemical characteristics and can have different or complementary functions.
Antimicrobial Activity of Lysozyme
Lysozyme as an enzymatic globular protein has gained great attention in the pharmaceutical and food industries owing to its antibacterial function. As reported, all lysozymes can hydrolyze the β-(1,4)- glycosidic linkages between N-acetylmuramic acid (NAM) and N-acetylglucosamine (NAG) of peptidoglycan chains. Peptidoglycan specifies cellular shape and creates a good protection versus cellular turgor pressure, whereas lysozyme activity causes the loss of its integrity, bacterial cell lysis, and eventual cell death (39). The cell wall of Gram-positive bacteria consists of abandon peptidoglycan layers (about 40 layers) without any outer membrane to which polysaccharides, lipoteichoic acids, and teichoic acids are connected to peptidoglycan (40). Therefore, they are susceptible to being attacked by lysozyme. The level of teichoic acids in the cell wall of Gram-positive bacteria can affect the degree of their sensitivity to lysozymes because the negatively charged teichoic acids bind the lysozymes and reduce the enzymatic activity of lysozymes (36). Various technological and environmental factors, such as pH, temperature, salt concentration, and osmotic strength can affect the stability and antimicrobial activity of lysozyme in food and this varies based on the lysozyme types (32, 41). These effective factors on lysozyme activity have been critically reviewed by Proctor and Cunningham and Taylor et al. (23, 42). The maximum enzymatic activity of HEWL is observed at approximately pH 5 and lower stability and activity has been obtained in highly acidic (pH < 3.8) and alkaline conditions (43). The pH range of lysozyme activity is highly dependent on salt concentration. In a survey conducted on human lysozyme, the results showed that in the sodium chloride concentration of 0.05 M, lysozyme activity was greater at a wide range of pH (5–9) compared with the salt level of 0.1 M (44). Lysozyme non-specific activity can take place in the low level of salt, although high-ionic strength (0.2 M salt) inhibits its activity (41). The maximum enzymatic activity was obtained at an ionic strength of 0.1 in the presence of potassium salts (43). Garajov et al. reported that the specific activity of lysozyme increased with the decreasing concentration of sodium salts of acetate, chloride, bromide, thiocyanate, and perchlorate from 0.5 to 0.1 M (45). Due to the presence of four disulfide bonds in lysozyme structure, it was active at 100°C within 2 min at pH 4–5, but at pH 9, activity loss happened with heating for 5 min at boiling temperature (46). The temperature between 25 and 60°C had no effect on human lysozyme activity (44). However, at pH 6.2, 25% loss of the lytic activity of egg-white lysozyme was reported at 100°C within 20 min and 5% of activity was lost following treatments at 80°C for 30 min (47). Moreover, there is an evidence that lysozyme can be stored at 5°C for many years or at 30°C for several months (>6 months) without losing lytic activity in dry state. Lysozyme can be protected by polyols and sugars versus heat, because they affected hydrophobic interactions (44). Xing and Yaylayan stated that the presence of sugars could even maintain the enzymatic activity of lysozyme after milling (48). The most important factors that affect the catalytic rate of lysozyme are shown in Figure 2.
Based on the information obtained about the sensitivity of food-related bacteria to lysozyme, some Gram-positive bacteria, such as Micrococcus spp., Sarcina spp., C. tyrobutyricum, Clostridium thermosaccharolyticum, Bacillus stearothermophilus, and Bacillus coagulans were strongly lysed by egg-white lysozyme (46). On the other hand, lysozymes are ineffective against Gram-negative bacteria because they have a thin layer of peptidoglycan without teichoic acids covered by an outer membrane. The outer membrane contains phospholipids, lipopolysaccharides, and lipoproteins that act as a barrier and limit the access of lysozymes to peptidoglycan chains (36, 49, 50). Nonetheless, this barrier can be disrupted by membrane-permeabilizing agents, such as carvacrol, lactoferrin, organic acids, and ethylenediaminetetraacetic acid (EDTA) or conjugation of lysozyme to carbohydrates, denaturation of lysozyme, and the modification of lysozyme by linking with other compounds (polysaccharides, hydrophobic peptides, and fatty acids) (34). Several studies have reported that treatments, such as high hydrostatic pressure and pulsed electric field can increase the susceptibility of Gram-negative bacteria, such as E. coli to lysozyme (51, 52). A summary of used methods to improve the function of lysozymes against Gram-negative bacteria has been provided in Figure 3.
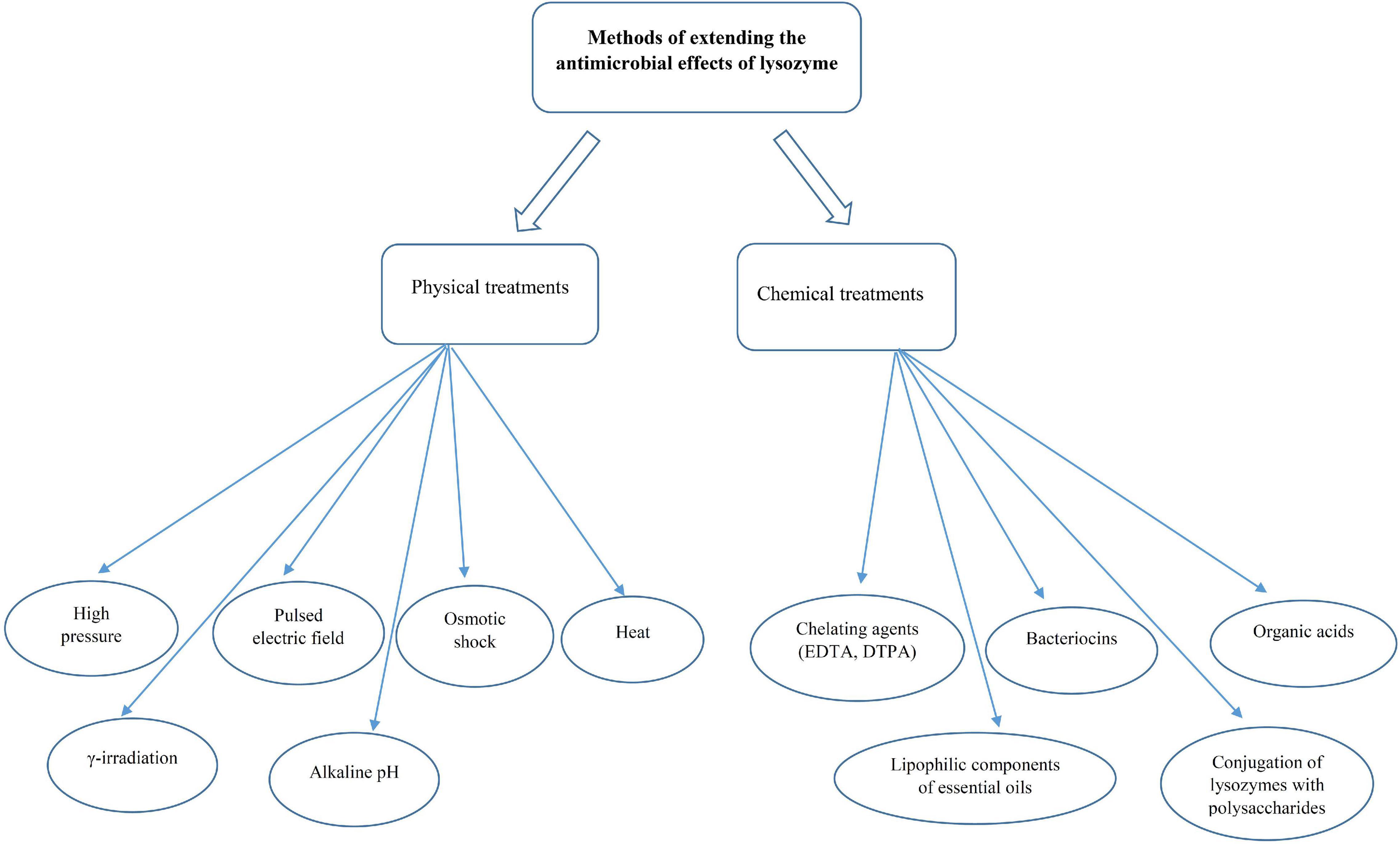
Figure 3. Common methods to increase the lysozyme activity against the growth of Gram-negative bacteria.
In addition to the enzymatic activity of lysozymes as a bactericidal action, they have non-lytic activity to kill bacteria related to their hydrophobic, structural, and cationic features. Lysozyme as a cationic protein can form pores in bacterial membranes through the electrostatic interactions between protein and components of the bacterial membrane (phospholipids) and induce cell lysis as a result of membrane leakage without peptidoglycan hydrolysis. Evidence has shown that the complete or partial denaturation of lysozyme using heat, dithiothreitol (DTT), or site-directed mutagenesis does not reduce its bactericidal effect against Gram-negative and Gram-positive bacteria due to its non-enzymatic antimicrobial properties. The bactericidal activity of denatured lysozyme is attributed to the cationic properties and the increase of hydrophobic amino acid residues on its surface owing to conformational changes (46).
Apart from the antibacterial action, lysozyme has a weak activity against yeast, because mannoprotein and fibrous β-(1,3) glucans are the main compounds of yeast cell wall and the chitinase activity of lysozyme is not strong (53, 54). Additionally, lysozyme can inhibit the growth of viruses and fungi, such as Aspergillus niger and Penicillium that is associated with its charge, rather than its enzymatic action (49). In addition, Lysozyme exhibits antiviral activity by forming complexes with the DNA of viruses (15, 37).
Application of Lysozyme in Cheese
The high demand for natural food preservatives has made lysozyme an increasingly important natural antimicrobial enzyme in food processing and preservation. Due to the high potential of lysozyme, it could be utilized in various practical applications, such as food, pharmaceutical, and chemical industries (55, 56). As mentioned before, lysozyme as a natural antimicrobial compound can hydrolyze β-(1,4) glycosidic linkage between N-acetylmuramic acid and N acetylglucosamine found in the peptidoglycan, the main component of the cell wall of both Gram-positive and Gram-negative bacteria (57). Therefore, it can be used to inhibit bacterial growth, especially Gram-positive spoilage bacteria, in various food products, such as wine and unpasteurized beer and meat and dairy products (41, 51, 58). In the dairy industry, lysozyme could be applied in cheese making to inhibit the growth of spoilage bacteria and prevent the texture deterioration, blowing, and unpleasant taste of various cheeses (59, 60). The antimicrobial activity of lysozyme and its functionality in cheese is discussed in this part.
Bester et al. investigated the effect of various lysozyme levels on the growth of vegetative cells of C. tyrobutyricum and germination of its spore forms, the growth of cheese starter culture and coliform bacteria in culture media. They found that the vegetative growth of C. tyrobutyricum BZ15 and C611 was prevented by lysozyme at the levels equal to and greater than 250 units/ml. Furthermore, the stimulation of spores carried out in the presence of lysozyme and the maximum stimulation was observed at 250 units/ml and the spores of strain C611 exhibited faster germination in the presence of lysozyme. Three isolates of coliforms were inhibited by lysozyme at 500 and 1,000 U/ml, and only one isolated was stimulated by lysozyme. In addition, they indicated that the activity of starter cultures was not affected by various concentrations of lysozyme (up to 2,500 U/ml). They stated that the growth of four lactobacilli and the quality of the cheese obtained by these bacteria were not affected by lysozyme at levels of 250 U/ml (61). On the other hand, López-Pedemonte et al. studied the effect of the addition of nisin or lysozyme in the sensitivity of B. cereus ATCC 9139 to high hydrostatic pressure (HHP) in cheese and found that the sensitivity of spores to HHP was not affected by the addition of lysozyme (22.4 mg/ml), while the presence of nisin (1.56 mg/ml) significantly increased the sensitivity of the spores. They concluded that HHP at mild temperatures in combination with the addition of nisin may be helpful for improving the safety and preservation of cheeses made from raw milk (30). Similarly, Sudagidan and Yemenicioğlu evaluated the antimicrobial effect of nisin (0.5, 2.5, 12.5, and 25 μg/ml) and lysozyme (1, 2, 3, 4, and 5 mg/ml) on the growth and biofilm formation of 25 strains of S. aureus that isolated from cheese (12 strains) and raw milk (13 strains) and figured out that an application of nisin at a level of 25 μg/ml inhibited the growth of all tested strains. In contrast, no inhibition on the growth of tested strain was observed when lysozyme was utilized at levels up to 5 mg/ml. Besides, the results showed that nisin at the growth inhibitory concentration prevented the biofilm formation of strains, while lysozyme was able to inactivate only six strains considerably. Their results showed that the biofilm formation capacity is likely to be affected by the changed bacterial growth kinetics in the presence of lysozyme. They indicated that there might be a potential risk of S. aureus growth in dairy products when lysozyme is utilized as a biopreservative (27). Conversely, Amiri et al. studied the antimicrobial characteristics of native and dextran-conjugated lysozyme (400 μg/ml) against E. coli and S. aureus in cheese curd and found that native and modified lysozyme was effective against E. coli at a level of 400 μg/ml in the cheese curd and the glycosylated enzyme was more significant that native enzyme in E. coli reduction. Moreover, both lysozyme and modified enzymes were similarly effective against S. aureus at 400 μg/ml. As far as we know, lysozyme attacks only glycosidic bonds that presented in the peptidoglycan of bacterial cell wall. On the other hand, due to the presence of hydrophobic material, such as lipopolysaccharide associated with the thin peptidoglycan layer of Gram-negative bacteria, native lysozyme can hardly penetrate and lyse the cell wall of Gram-negative bacteria. Therefore, it is necessary to improve the surface properties of lysozyme. Their results revealed that the improvement of antimicrobial activity of the lysozyme-dextran conjugate against Gram-negative bacteria, such as E. coli could be probably ascribed to the excellent surfactant properties and the remaining lytic activity of lysozyme-dextran conjugate. Generally, they stated that due to the improved solubility of lysozyme-dextran conjugate at various temperatures and pH values as well as better surfactant properties in comparison with native enzyme lysozyme, lysozyme-dextran conjugate could considered as a suitable antimicrobial enzyme (62).
Furthermore, Sinigaglia et al. examined the antimicrobial activity of lysozyme (0.25 mg/ml) in combination with various levels of ethylenediaminetetraacetic disodium salt (Na2-EDTA) (10, 20, and 50 mmol/L) against spoilage microorganisms, such as coliforms and Pseudomonadaceae as well as the functional microbiota of mozzarella cheese during 8 days storage at 4°C. They understood that lysozyme and Na2-EDTA could be effective in preventing the growth of coliforms and Pseudomonadaceae during 7 days storage. In addition, they reported that the functional microbiota of mozzarella cheese, such as lactic acid bacteria, was not affected by the addition of these active compounds and finally, they concluded that the shelf life of mozzarella cheese could be prolonged by utilizing lysozyme and Na2-EDTA in the conditioning brine (59). Similarly, Scharfen et al. investigated the effect of human lysozyme (270 μg/ml) transgenic goat milk on the functionality of lactic acid bacteria in cheese making and found that lysozyme transgenic goat milk did not have an adverse effect on the growth of lactic acid bacteria during cheese-making process and could be potentially used to enhance the characteristics of raw milk cheese production (28). Additionally, Conte et al. investigated the effect of various concentrations of lysozyme (125, 250, and 500 mg/kg) with Na2-EDTA (50 mM) with or without modified-atmosphere packaging (MAP) (95:5 CO2:N2) conditions on the microbiological and sensory quality of burrata cheese during 9 days at 8°C. They found that the combination of lysozyme/Na2-EDTA and MAP resulted in a higher microbiological acceptability limit (days) for coliforms and Pseudomonas spp. compared with control or the samples containing active substance and stored in the air atmosphere. They stated that the growth of aerobic, Gram-negative bacteria, such as Pseudomonas spp. could be decreased by the bacteriostatic effect of CO2 that extends the lag phase of tested bacteria. Furthermore, they understood that regardless of the packaging strategy, the cheese’s quality showed decreasing manner during storage. They concluded that lysozyme/Na2-EDTA in combination with MAP, especially at the highest lysozyme concentrations (500 mg/kg) could be considered as an effective strategy against spoilage microorganisms (63). Moreover, Doosh et al. examined the effect of hen egg-white lysozyme (250 and 300 mg/kg) on the microbial properties and shelf-life of Iraqi soft cheese made from buffalo milk during 15 days storage at 6°C. They observed that the application of lysozyme prevented the development of total count of bacteria, the count of psychrophilic bacteria, and yeast and mold compared with the untreated sample during 15 days storage. Sensory evaluation showed no significant differences among lysozyme-treated samples and control at zero time, confirming that lysozyme addition had no adverse effect on consumer acceptance. Furthermore, after 3 days of storage lysozyme-treated samples, especially the one containing 300 mg/kg obtained the highest scores in terms of texture, flavor, and bitterness compared with control. These high scores could be attributed to the antimicrobial activity of lysozyme that prevents bacterial growth, particularly psychotropic bacteria, which is responsible for the lipolysis and proteolysis of cheese during storage (25). Additionally, Al-Baarri et al. studied the possibility of shelf-life improvement of Indonesian soft milk cheese (dangke) using lysozyme and lactoperoxidase systems. In this regard, the microbiological properties of dangke samples placed in sterile pure water, lysozyme, lactoperoxidase system (LPS), and a combination of lysozyme and LPS were evaluated during 18 h at room temperature. They figured out that the sample of cheese immersed in sterile pure water had the highest bacterial count at 0 h compared with the treated samples. The lysozyme-treated cheese sample exhibited the lowest total number of bacteria. Furthermore, they found that the microbial growth was inhibited in treated samples for 8 h. The highest antimicrobial activity was observed in the cheese immersed in the combination of LPS and lysozyme followed by lysozyme. They indicated that the antimicrobial activity of lysozyme could be ascribed to its ability to destroy the structure of cell wall bacteria and fungi (64). In a study carried out by Sozbilen and Yemenicioğlu, the effect of pH (4 and 6) and temperature (50 or 60°C for 45 min) on the optimum activity of lysozyme–nisin combination (500–15.6 μg/ml) against Listeria innocua in the test buffer was investigated. They understood that heating at pH 4.5 and 6 in combination with lysozyme and nisin resulted in the higher inactivation of L. innocua compared with heating alone or heating combined with lysozyme or nisin. Furthermore, they indicated that the antimicrobial activity of lysozyme–nisin combination at 50°C was not pH-dependent. Additionally, they found that milk heating at 50°C preserved 73 and 63% activity of nisin and lysozyme, respectively. They concluded that heating at 50°C in combination with lysozyme and nisin could be applicable as mild treatment in the production of traditional cheese made from raw milk to improve the safety of the cheese and aroma and flavor. Moreover, they reported that the changes of pH range from 2.5–4 to 4.50–6.50 resulted in an increase in the activities of lysozyme from 204–2,039 to 17,705–48,295 U/ml range, respectively. Therefore, to achieve the acceptable antimicrobial properties of lysozyme, the pH of food products should be taken into consideration (29).
Besides the direct usage of lysozyme in cheese or cheese brine, the application of lysozyme as an antimicrobial agent in the packaging, such as film and coating is investigated. Duan et al. examined the antimicrobial activity of chitosan composite film containing lysozyme against various microorganisms inoculated onto the surface of Mozzarella cheese. They observed that chitosan-lysozyme composite films and coatings remarkably decreased the growth of L. monocytogenes, E. coli, Pseudomonas fluorescens, and mold in Mozzarella cheese than laminated films and coatings. This can be ascribed to the higher concentration of chitosan and lysozyme in chitosan-lysozyme composite than in laminated films and coatings. They indicated that beside the peptidoglycan hydrolysis, the non-enzymatic antimicrobial action of lysozyme involving membrane disruption due to the positive charge of lysozyme could be suggested for the antimicrobial properties of lysozyme, especially against Gram- negative bacteria. They claimed that chitosan composite films in combination with lysozyme could be utilized in cheese packaging to control the post-processing microbial contaminants of cheese and therefore, enhance the microbial safety of cheese products (65). Similarly, Mehyar et al. studied the effect of chitosan coating containing lysozyme (60% (w/w) lysozyme/chitosan solution) on the shelf-life, microbial, and sensory properties of Halloumi cheese and found that coating increased the shelf-life of cheese as well as having no adverse effect on the sensory properties of cheese. They concluded that by using chitosan-lysozyme coating in the production of Halloumi cheese, the brine could be reduced from 15 to 10% while the same inhibitory effects against most contaminating microorganisms and shelf-life would be achieved (66).
Additionally, it has been reported that composite edible film containing lysozyme prevented the growth of microorganisms both on the surface and inside the region of Gouda cheese during ripening (67). It has been stated that nano-laminate coating containing lysozyme was able to extend the shelf-life of Coalho cheese and coated cheese sample exhibited lower pH, lipid peroxidation, values of mass loss and microorganisms’ proliferation in comparison with the uncoated sample (68).
Jalilzadeh et al. evaluated the application of lysozyme-xanthan (200, 400, and 500 ppm) gum in whey protein-based edible coating on the shelf life of ultra-filtrated white cheese. They figured out that the growth of Penicillium chrysogenum, E. coli O157:H7, and S. aureus was significantly inhibited by application coating incorporating lysozyme-xanthan gum conjugate in comparison with uncoated cheese and therefore, the shelf life of coated sample increased. Their results showed that lactic acid bacteria were not affected by edible coating during 60 days of ripening. Due to the antimicrobial effect of tested edible film and having no adverse effect on the sensory properties of ultra-filtrated white cheese, they stated that whey protein-based edible coating in combination with lysozyme-xanthan could be applicable in increasing the shelf life of ultra-filtrated cheese (69). Generally, it can be stated that the application of edible film and coating in combination with natural antimicrobial enzymes, such as lysozyme can be considered as an alternative material for the food industry to improve the safety of food products, such as cheese, especially during storage and ripening to prevent post-processing contamination. Table 2 listed selected publications on the application of lysozyme in cheese.
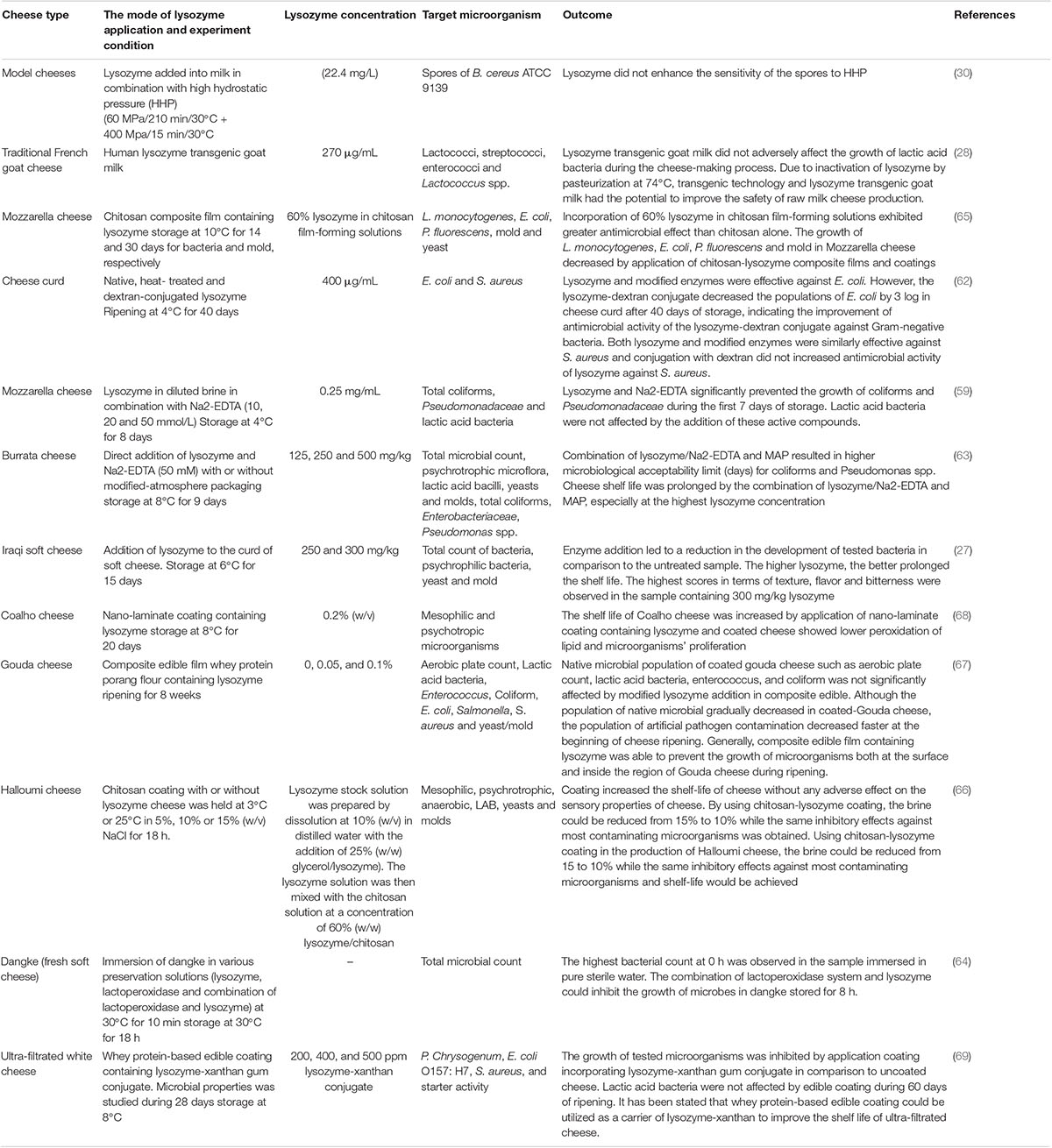
Table 2. Selected publications regarding the effects of lysozyme addition on the microbial properties of cheese.
Regulatory Status and Toxicology
One of the controversial topics about lysozyme is its role as an allergen. Some studies indicated that lysozyme acts as a weak allergen, while others support the opposite idea (70). As far as we know, egg white is considered allergenic to sensitive individuals, especially children and this sensitivity is related to allergenic proteins in eggs, such as conalbumin (ovotransferrin), ovomucoid, ovalbumin, and lysozyme. Allergic reactions generated by egg white lysozyme were lower in animals and humans than in other egg proteins, such as ovalbumin and albumin (41). Although significant amounts of IgE in the serum of egg-allergic patients have been bonded by lysozyme, the clinical significance of this reaction is unclear (41). It has been reported that lysozyme from egg white has insignificance acute, subacute, and chronic toxicity in animals (23). Furthermore, the long-term consumption of lysozyme as an endogenous food ingredient in eggs, milk as well as the long historic use of lysozyme in cheese that has traditionally been sold over the counter without prepackaging and labeling could be considered as an argument for the safety of lysozyme (23, 41). It has been stated that neither side effects nor immunological responses have been identified in egg-sensitive individuals with or without lysozyme sensitization after consuming lysozyme-containing cheese (71). Therefore, it is likely that the application of egg-white lysozyme in foods at permitted levels could not be considered as a health concern. Lysozyme has been declared as generally recognized as safe (GRAS) by the U.S. Food and Drug Administration (FDA) for use in cheese. Due to the allergenic potential of lysozyme, the FDA has concluded that “Egg white lysozyme” labeling is mandatory for bulk and packaged foods containing lysozyme (72). Additionally, it has been reported by the Joint FAO/WHO Expert Committee on Food Additives (JECFA) that lysozyme is derived from edible animal tissue commonly utilized as food and therefore can be designated as class I enzyme and considered as a food. It has been stated by JECFA that the intake of the low amounts of lysozyme in cheese made from treated milk was not a risk to consumer health (73). It has been approved by many countries to utilize hen egg-white lysozyme to control the growth of spoilage organisms in foods (74). European Additives Directive approved lysozyme as a preservative (E1105) to prevent the late blowing in ripened cheese (23).
Conclusion
Due to the growing concerns about the applying synthetic additives in food, much effort has been made in the food industry for alternatives to these additives, especially from natural sources to improve the safety and shelf-life of food products. Because of antimicrobial and antibiofilm activity of antimicrobial enzymes, they have been recently considered as a novel natural antimicrobial agent in food preservation. Due to the widespread dispersion of lysozyme in nature and the antimicrobial activity of this enzyme against various bacteria, especially Gram-positive, lysozyme has the potential to be considered a safe food additives in food products. Based on the differences in amino acid sequences and structural properties, lysozymes are categorized into three main types: c-type, g-type, and i-type. Lysozyme from HEWL is considered a major industrial source for practical uses because of its availability and cost-effectiveness. All lysozymes exert antimicrobial activity by hydrolyzing the β-(1,4)-glycosidic linkages between N-acetylmuramic acid (NAM) and N-acetylglucosamine (NAG) of peptidoglycan chains and this activity is affected by various factors, such as ionic strength, presence of lysozyme inhibitors, pH, salt content, water activity, presence of potentiating chemicals, and temperature. Various chemical and physical treatments have been applied to improve the sensitivity of Gram-negative bacteria against lysozyme. Lysozyme is presented as a natural preservative in mammalian milk and therefore, it could be utilized in dairy products, such as cheese and as this study revealed, lysozyme could be applied in cheese making to inhibit the growth of spoilage bacteria and therefore to prevent the texture deterioration, blowing, and unpleasant taste of various cheeses. Moreover, besides the direct usage of lysozyme in cheese or cheese brine, lysozyme could be applied as an antimicrobial agent in the packaging, such as film and coating to preserve its functionality and antimicrobial activity during storage and ripening to prevent post-processing contamination. Generally it could be stated that lysozyme could be considered as an alternative material alone or as part of hurdle technology for the food industry to improve the safety of food products, such as cheese. Additionally, it is suggested to study the application of lysozyme in combination with other antimicrobial enzymes and novel technologies, such as high-intensity pulsed electric field, cold plasma, and irradiation to enhance the antimicrobial efficiency of lysozyme in cheese making in future investigations.
Author Contributions
MY and NK designed the study. NK, EK, and MK wrote the manuscript. MY, NK, and SS critically revised the manuscript. All authors listed have approved it for publication, contributed to the article, and approved the submitted version.
Conflict of Interest
The authors declare that the research was conducted in the absence of any commercial or financial relationships that could be construed as a potential conflict of interest.
Publisher’s Note
All claims expressed in this article are solely those of the authors and do not necessarily represent those of their affiliated organizations, or those of the publisher, the editors and the reviewers. Any product that may be evaluated in this article, or claim that may be made by its manufacturer, is not guaranteed or endorsed by the publisher.
References
1. Khorshidian N, Khanniri E, Mohammadi M, Mortazavian AM, Yousefi M. Antibacterial activity of pediocin and pediocin-producing bacteria against Listeria monocytogenes in meat products. Front Microbiol. (2021) 12:709959. doi: 10.3389/fmicb.2021.709959
2. Yousefi M, Khorshidian N, Hosseini H. Potential application of essential oils for mitigation of Listeria monocytogenes in meat and poultry products. Front Nutr. (2020) 7:577287. doi: 10.3389/fnut.2020.577287
3. Bahrami A, Moaddabdoost Baboli Z, Schimmel K, Jafari SM, Williams L. Efficiency of novel processing technologies for the control of Listeria monocytogenes in food products. Trends Food Sci Technol. (2020) 96:61–78. doi: 10.1016/j.tifs.2019.12.009
4. Mathys A. Perspective of micro process engineering for thermal food treatment. Front Nutr. (2018) 5:24. doi: 10.3389/fnut.2018.00024
5. Carocho M, Barreiro MF, Morales P, Ferreira ICFR. Adding molecules to food, pros and cons: a review on synthetic and natural food additives. Compr Rev Food Sci Food Safety. (2014) 13:377–99. doi: 10.1111/1541-4337.12065
6. Silva MM, Lidon FC. Food preservatives – an overview on applications and side effects. Emirates J Food Agric. (2016) 28:366–73. doi: 10.9755/ejfa.2016-04-351
7. Ferreira S, Sanchez G, Alves MJ, Fraqueza MJ. Editorial: natural compounds in food safety and preservation. Front Nutr. (2021) 8:759594. doi: 10.3389/fnut.2021.759594
8. Khorshidian N, Yousefi M, Khanniri E, Mortazavian AM. Potential application of essential oils as antimicrobial preservatives in cheese. Innov Food Sci Emerg Technol. (2018) 45:62–72. doi: 10.1016/j.ifset.2017.09.020
9. Polat Yemiş G, Delaquis P. Natural compounds with antibacterial activity against Cronobacter spp. in powdered infant formula: a review. Front Nutr. (2020) 7:595964. doi: 10.3389/fnut.2020.595964
10. Barroug S, Chaple S, Bourke P. Combination of natural compounds with novel non-thermal technologies for poultry products: a review. Front Nutr. (2021) 8:628723. doi: 10.3389/fnut.2021.628723
11. Carocho M, Morales P, Ferreira ICFR. Natural food additives: quo vadis? Trends Food Sci Technol. (2015) 45:284–95. doi: 10.1016/j.tifs.2015.06.007
12. Thallinger B, Prasetyo EN, Nyanhongo GS, Guebitz GM. Antimicrobial enzymes: an emerging strategy to fight microbes and microbial biofilms. Biotechnol J. (2013) 8:97–109. doi: 10.1002/biot.201200313
13. Zhang H, Fu G, Zhang D. Cloning, characterization, and production of a novel lysozyme by different expression hosts. J Microbiol Biotechnol J. (2014) 24:1405–12. doi: 10.4014/jmb.1404.04039
14. Fleming A. On a remarkable bacteriolytic element found in tissues and secretions. Proc R Soc Lond Ser B. (1922) 93:306–17. doi: 10.1098/rspb.1922.0023
15. Lesnierowski G, Stangierski J. What’s new in chicken egg research and technology for human health promotion? – a review. Trends Food Sci Technol. (2018) 71:46–51. doi: 10.1016/j.tifs.2017.10.022
16. Wu T, Jiang Q, Wu D, Hu Y, Chen S, Ding T, et al. What is new in lysozyme research and its application in food industry? A review. Food Chem. (2019) 274:698–709. doi: 10.1016/j.foodchem.2018.09.017
17. Syngai GG, Ahmed G. Chapter 11 - lysozyme: a natural antimicrobial enzyme of interest in food applications. In: Kuddus M editor. Enzymes in Food Biotechnology. Cambridge, MA: Academic Press (2019). p. 169–79.
18. Phillips DC. The three-dimensional structure of an enzyme molecule. Sci Am. (1966) 215:78–93. doi: 10.1038/scientificamerican1166-78
19. Blake C, Koenig D, Mair G, North A, Phillips D, Sarma V. Structure of hen egg-white lysozyme: a three-dimensional Fourier synthesis at 2 Å resolution. Nature. (1965) 206:757–61. doi: 10.1038/206757a0
20. Callewaert L, Michiels CW. Lysozymes in the animal kingdom. J Biosci. (2010) 35:127–60. doi: 10.1007/s12038-010-0015-5
21. Shahmohammadi A. Lysozyme separation from chicken egg white: a review. Eur Food Res Technol. (2018) 244:577–93. doi: 10.1007/s00217-017-2993-0
22. Gyawali R, Ibrahim SA. Natural products as antimicrobial agents. Food Control. (2014) 46:412–29. doi: 10.1016/j.foodcont.2014.05.047
23. Taylor TM, Johnson EA, Larson AE. Lysozyme. In: Davidson PM, Sofos JN, Branen AL editors. Antimicrobials in Food. Boca Raton, FL: CRC Press (2020). p. 445–74.
24. Roos ALD, Walstra P, Geurts TJ. The association of lysozyme with casein. Int Dairy J. (1998) 8:319–24. doi: 10.1016/S0958-694600053-3
25. Doosh KS, Abdul-Rahman SM. Effect of lysozyme isolated from hen egg white in elongation the shelf life of Iraqi soft cheese made from buffalo milk. Pak J Nutr. (2014) 13:635. doi: 10.3923/pjn.2014.635.641
26. Khorshidian N, ghasemzadeh-mohammadi V, Yousefi M, Mortazavian AM. Comparison of antifungal properties of Lactobacillus rhamnosus and Lactobacillus reuteri with potassium sorbate in the iranian uf-feta cheese. J Microbiol Biotechnol Food Sci. (2021):e3318. doi: 10.15414/jmbfs.3318
27. Sudagidan M, Yemenicioğlu A. Effects of nisin and lysozyme on growth inhibition and biofilm formation capacity of Staphylococcus aureus strains isolated from raw milk and cheese samples. J Food Protect. (2012) 75:1627–33. doi: 10.4315/0362-028X.JFP-12-001
28. Scharfen EC, Mills DA, Maga EA. Use of human lysozyme transgenic goat milk in cheese making: effects on lactic acid bacteria performance. J Dairy Sci. (2007) 90:4084–91. doi: 10.3168/jds.2006-808
29. Sozbilen GS, Yemenicioğlu A. Antilisterial effects of lysozyme-nisin combination at temperature and pH ranges optimal for lysozyme activity: test of key findings to inactivate Listeria in raw milk. LWT. (2021) 137:110447. doi: 10.1016/j.lwt.2020.110447
30. López-Pedemonte TJ, Roig-Sagués AX, Trujillo AJ, Capellas M, Guamis B. Inactivation of spores of Bacillus cereus in cheese by high hydrostatic pressure with the addition of nisin or lysozyme. J Dairy Sci. (2003) 86:3075–81. doi: 10.3168/jds.S0022-030273907-1
31. Bottazzi V, Battistotti B, Rebecchi A, Bertuzzi S. Germination of Clostridium spores and the action of lysozyme in Grana cheese. Latte. (1996) 11:80.
32. Masschalck B, Michiels CW. Antimicrobial properties of lysozyme in relation to foodborne vegetative bacteria. Crit Rev Microbiol. (2003) 29:191–214. doi: 10.1080/713610448
33. Wu H, Cao D, Liu T, Zhao J, Hu X, Li N. Purification and characterization of recombinant human lysozyme from eggs of transgenic chickens. PLoS One. (2015) 10:e0146032. doi: 10.1371/journal.pone.0146032
34. Vilcacundo R, Méndez P, Reyes W, Romero H, Pinto A, Carrillo W. Antibacterial activity of hen egg white lysozyme denatured by thermal and chemical treatments. Sci Pharm. (2018) 86:48. doi: 10.3390/scipharm86040048
35. Takahashi M, Takahashi H, Okakura Y, Ichikawa M, Kuda T, Kimura B. Impact of pH and protein hydrophobicity on norovirus inactivation by heat-denatured lysozyme. PLoS One. (2020) 15:e0237888. doi: 10.1371/journal.pone.0237888
36. Ragland SA, Criss AK. From bacterial killing to immune modulation: recent insights into the functions of lysozyme. PLoS Pathog. (2017) 13:e1006512. doi: 10.1371/journal.ppat.1006512
37. Tullio V, Spaccapelo R, Polimeni M. Lysozymes in the animal kingdom. In: Prato M editor. Human and Mosquito Lysozymes. Cham: Springer (2015). p. 45–57. doi: 10.1007/978-3-319-09432-8_3
38. Farkye NY, Bansal N. Enzymes indigenous to milk| other enzymes. In: Fuquay JW editor. Encyclopedia of Dairy Sciences (Second Edition). San Diego, CA: Academic Press (2011). p. 327–34.
39. Primo ED, Otero LH, Ruiz F, Klinke S, Giordano W. The disruptive effect of lysozyme on the bacterial cell wall explored by an in-silico structural outlook. Biochem Mol Biol Educ. (2018) 46:83–90. doi: 10.1002/bmb.21092
40. Gutiérrez D, Briers Y. Lysins breaking down the walls of gram-negative bacteria, no longer a no-go. Curr Opin Biotechnol. (2021) 68:15–22. doi: 10.1016/j.copbio.2020.08.014
41. Silvetti T, Morandi S, Hintersteiner M, Brasca M. Chapter 22 - use of hen egg white lysozyme in the food industry. In: Hester PY editor. Egg Innovations and Strategies for Improvements. San Diego, CA: Academic Press (2017). p. 233–42.
42. Proctor VA, Cunningham FE, Fung DYC. The chemistry of lysozyme and its use as a food preservative and a pharmaceutical. CRC Crit Rev Food Sci Nutr. (1988) 26:359–95. doi: 10.1080/10408398809527473
43. Ercan D, Demirci A. Recent advances for the production and recovery methods of lysozyme. Crit Rev Biotechnol. (2016) 36:1078–88. doi: 10.3109/07388551.2015.1084263
44. Yang B, Wang J, Tang B, Liu Y, Guo C, Yang P, et al. Characterization of bioactive recombinant human lysozyme expressed in milk of cloned transgenic cattle. PLoS One. (2011) 6:e17593. doi: 10.1371/journal.pone.0017593
45. Garajová K, Balogová A, Dušeková E, Sedláková D, Sedlák E, Varhaè R. Correlation of lysozyme activity and stability in the presence of Hofmeister series anions. Biochim Biophys Acta Proteins Proteom. (2017) 1865:281–8. doi: 10.1016/j.bbapap.2016.11.016
47. Smolelis AN. Hartsell S. Factors affecting the lytic activity of lysozyme. J. Bacteriol. (1952) 63:665–74. doi: 10.1128/jb.63.5.665-674.1952
48. Xing H, Yaylayan V. Mechanochemically induced controlled glycation of lysozyme and its effect on enzymatic activity and conformational changes. J Agric Food Chem. (2019) 67:3249–55. doi: 10.1021/acs.jafc.9b00070
49. Feng K, Wen P, Yang H, Li N, Lou WY, Zong MH, et al. Enhancement of the antimicrobial activity of cinnamon essential oil-loaded electrospun nanofilm by the incorporation of lysozyme. RSC Adv. (2017) 7:1572–80. doi: 10.1039/C6RA25977D
50. Shehadul Islam M, Aryasomayajula A, Selvaganapathy PR. A review on macroscale and microscale cell lysis methods. Micromachines. (2017) 8:83. doi: 10.3390/mi8030083
51. Liburdi K, Benucci I, Esti M. Lysozyme in wine: an overview of current and future applications. Compr Rev Food Sci Food Safety. (2014) 13:1062–73.
52. Salazar O, Asenjo JA. Enzymatic lysis of microbial cells. Biotechnol Lett. (2007) 29:985–94. doi: 10.1007/s10529-007-9345-2
54. Dadkhodazade E, Khanniri E, Khorshidian N, Hosseini SM, Mortazavian AM, Moghaddas Kia E. Yeast cells for encapsulation of bioactive compounds in food products: a review. Biotechnol Prog. (2021) 37:e3138. doi: 10.1002/btpr.3138
55. Leśnierowski G. Lysozyme and its modified forms: properties, potential for its production and application. In: Watson RR, De Meester F editors. Handbook of Eggs in Human Function. (Vol. 9), Wageningen: Wageningen Academic Publishers (2015). p. 483
56. Wichgers Schreur PJ, Van Weeghel C, Rebel JM, Smits MA, van Putten JP, Smith HE. Lysozyme resistance in Streptococcus suis is highly variable and multifactorial. PLoS One. (2012) 7:e36281. doi: 10.1371/journal.pone.0036281
57. Ormus S, Oulahal N, Noël C, Degraeve P, Gharsallaoui A. Effect of low methoxyl (LM) pectin complexation on the thermal and proteolytic inactivation of lysozyme: a kinetic study. Food Hydrocolloids. (2015) 43:812–8. doi: 10.1016/j.foodhyd.2014.08.016
58. Ntzimani AG, Giatrakou VI, Savvaidis IN. Combined natural antimicrobial treatments (EDTA, lysozyme, rosemary and oregano oil) on semi cooked coated chicken meat stored in vacuum packages at 4°C: microbiological and sensory evaluation. Innov Food Sci Emerg. Technol. (2010) 11:187–96. doi: 10.1016/j.ifset.2009.09.004
59. Sinigaglia M, Bevilacqua A, Corbo MR, Pati S, Del Nobile MA. Use of active compounds for prolonging the shelf life of mozzarella cheese. Int Dairy J. (2008) 18:624–30. doi: 10.1016/j.idairyj.2007.11.022
60. Stocco G, Cipolat-Gotet C, Cecchinato A, Calamari L, Bittante G. Milk skimming, heating, acidification, lysozyme, and rennet affect the pattern, repeatability, and predictability of milk coagulation properties and of curd-firming model parameters: a case study of Grana Padano. J Dairy Sci. (2015) 98:5052–67. doi: 10.3168/jds.2014-9146
61. Bester BH, Lombard SH. Influence of lysozyme on selected bacteria associated with gouda cheese. J Food Prot. (1990) 53:306–11. doi: 10.4315/0362-028X-53.4.306
62. Amiri S, Ramezani R, Aminlari M. Antibacterial activity of dextran-conjugated lysozyme against Escherichia coli and Staphylococcus aureus in cheese curd. J Food Prot. (2008) 71:411–5. doi: 10.4315/0362-028X-71.2.411
63. Conte A, Brescia I, Del Nobile MA. Lysozyme/EDTA disodium salt and modified-atmosphere packaging to prolong the shelf life of burrata cheese. J Dairy Sci. (2011) 94:5289–97. doi: 10.3168/jds.2010-3961
64. Al-Baarri A, Legowo AM, Arum SK, Hayakawa S. Extending shelf life of Indonesian soft milk cheese (dangke) by lactoperoxidase system and lysozyme. Int J Food Sci. (2018) 2018:4305395. doi: 10.1155/2018/4305395
65. Duan J, Park SI, Daeschel M, Zhao Y. Antimicrobial chitosan-lysozyme (CL) films and coatings for enhancing microbial safety of mozzarella cheese. J Food Sci. (2007) 72:M355–62. doi: 10.1111/j.1750-3841.2007.00556.x
66. Mehyar GF, Al Nabulsi AA, Saleh M, Olaimat AN, Holley RA. Effects of chitosan coating containing lysozyme or natamycin on shelf-life, microbial quality, and sensory properties of Halloumi cheese brined in normal and reduced salt solutions. J Food Process Preserv. (2018) 42:e13324. doi: 10.1111/jfpp.13324
67. Setyowati E, Manab A. Gouda cheese microbial controlling during ripening using composite edible film containing lysozyme. Int J Curr Microbiol Appl Sci. (2016) 5:748–56. doi: 10.20546/ijcmas.2016.505.076
68. de S, Medeiros BG, Souza MP, Pinheiro AC, Bourbon AI, Cerqueira MA, et al. Physical characterisation of an alginate/lysozyme nano-laminate coating and its evaluation on ‘Coalho’ cheese shelf life. Food Bioprocess Technol. (2014) 7:1088–98. doi: 10.1007/s11947-013-1097-5
69. Jalilzadeh A, Hesari J, Peighambardoust SH, Javidipour I. The effect of whey protein-based edible coating containing natamycin and lysozyme-xanthan gum conjugate on the shelf life of ultrafiltrated white cheese. J Food Bioprocess Eng. (2020) 3:168–77. doi: 10.22059/jfabe.2020.306083.1068
70. Brasca M, Morandi S, Silvetti T, Rosi V, Cattaneo S, Pellegrino L. Different analytical approaches in assessing antibacterial activity and the purity of commercial lysozyme preparations for dairy application. Molecules. (2013) 18:6008–20. doi: 10.3390/molecules18056008
71. Rossi F, Iaconelli A, Fiorentini L, Zito F, Donati MB, De Cristofaro ML, et al. Immunological response in egg-sensitive adults challenged with cheese containing or not containing lysozyme. J Am Coll Nutr. (2012) 31:385–91. doi: 10.1080/07315724.2012.10720463
72. United States Food and Drug Administration. Direct food substances affirmed as GRAS: egg white lysozyme. Tentative final rule. Fed Reg. (1998) 63:12421–12426.
73. Joint FAO/WHO Expert Committee on Food Additives (JECFA). Toxicological Evaluation of Certain Food Additives and Contaminants. Rome: World Health Organization (1993).
74. Wang C, Shelef LA. Behavior of Listeria monocytogenes and the spoilage microflora in fresh cod fish treated with lysozyme and EDTA. Food Microbiol. (1992) 9:207–13. doi: 10.1016/0740-002080048-9
75. Zheng W, Tian C, Chen X. Molecular characterization of goose-type lysozyme homologue of large yellow croaker and its involvement in immune response induced by trivalent bacterial vaccine as an acute-phase protein. Immunol Lett. (2007) 113:107–16. doi: 10.1016/j.imlet.2007.08.001
76. Cong L, Yang X, Wang X, Tada M, Lu M, Liu H, et al. Characterization of an i-type lysozyme gene from the sea cucumber Stichopus japonicus, and enzymatic and nonenzymatic antimicrobial activities of its recombinant protein. J Biosci Bioeng. (2009) 107:583–8. doi: 10.1016/j.jbiosc.2009.01.016
Keywords: antimicrobial enzyme, lysozyme, peptidoglycan, dairy, cheese
Citation: Khorshidian N, Khanniri E, Koushki MR, Sohrabvandi S and Yousefi M (2022) An Overview of Antimicrobial Activity of Lysozyme and Its Functionality in Cheese. Front. Nutr. 9:833618. doi: 10.3389/fnut.2022.833618
Received: 11 December 2021; Accepted: 09 February 2022;
Published: 09 March 2022.
Edited by:
Ilke Uysal-Unalan, Aarhus University, DenmarkReviewed by:
Magda Janalíková, Tomas Bata University in Zlín, CzechiaElena Arranz, Universidad Complutense de Madrid, Spain
Derya Alkan, Mu ð la University, Turkey
Copyright © 2022 Khorshidian, Khanniri, Koushki, Sohrabvandi and Yousefi. This is an open-access article distributed under the terms of the Creative Commons Attribution License (CC BY). The use, distribution or reproduction in other forums is permitted, provided the original author(s) and the copyright owner(s) are credited and that the original publication in this journal is cited, in accordance with accepted academic practice. No use, distribution or reproduction is permitted which does not comply with these terms.
*Correspondence: Mojtaba Yousefi, bS55b3VzZWZpQHNlbXVtcy5hYy5pcg==; bS55b3VzZWZpMjAwNkBnbWFpbC5jb20=