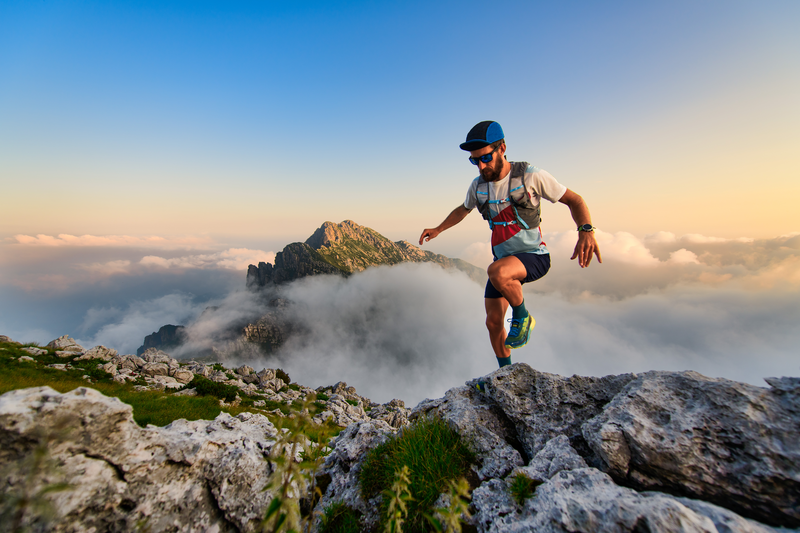
95% of researchers rate our articles as excellent or good
Learn more about the work of our research integrity team to safeguard the quality of each article we publish.
Find out more
REVIEW article
Front. Nutr. , 25 February 2022
Sec. Clinical Nutrition
Volume 9 - 2022 | https://doi.org/10.3389/fnut.2022.827286
Toxoplasma gondii is an obligate intracellular parasite that mainly infects warm-blooded animals including humans. T. gondii can encyst and persist chronically in the brain, leading to a broad spectrum of neurological sequelae. Despite the associated health threats, no clinical drug is currently available to eliminate T. gondii cysts. In a continuous effort to uncover novel therapeutic agents for these cysts, the potential of nutritional products has been explored. Herein, we describe findings from in vitro and in vivo studies that support the efficacy of plant-based foods and nutraceuticals against brain cyst burden and cerebral pathologies associated with chronic toxoplasmosis. Finally, we discuss strategies to increase the translatability of preclinical studies and nutritional products to address whether nutritional therapy can be beneficial for coping with chronic T. gondii infections in humans.
Toxoplasma gondii (T. gondii) is an obligate protozoan that infects mostly warm-blooded species including humans. Toxoplasmosis affects approximately a third of the human population. T. gondii has a few major clonal lineages, namely I, II, and III, and atypical genotypes of differing virulence and epidemiology (1). For example, the type II lineage is predominant in Europe and North America, and higher genetic diversity is observed in South America (2). T. gondii infection primarily occurs through the ingestion of oocysts or tissue cysts (3). Mother-to-foetus transmission and transmission through organ transplantation have also been observed (4, 5).
Following the ingestion of oocysts or cysts, the parasites invade intestinal epithelial cells and transform into tachyzoites. These tachyzoites replicate rapidly inside the parasitophorous vacuole (PV) (Figure 1) and ultimately rupture the PV membrane to infect the neighbouring cells (6). Tachyzoites are disseminated from the intestine to distant tissues by infecting and hijacking the migratory potential of immune cells (7–13). To reach the brain parenchyma, the parasites shuttle across the blood–brain barrier (BBB) within the immune cells or via transmigration of free tachyzoites across endothelial cells (7, 14–17). Unlike peripheral organs where the parasite presence is transient due to the ensuing immune responses [see reviews (18–20)], immune privilege sites such as the central nervous system (CNS) or the brain offer an environmental niche for the parasites (in this review, CNS and brain will be used interchangeably, with the brain being the main point of reference when CNS is used). Once inside the brain, the tachyzoites differentiate into slow-dividing bradyzoites within a cyst (Figure 1). A mature brain cyst (diameter: 50–70 μm) contains up to thousands of bradyzoites (21). Encysted bradyzoites are impervious to the host's immune system and antimicrobial drugs. Hence, T. gondii cysts can persist in the brain for a long time and establish a chronic infection. Neurons are the preferred cell type for T. gondii encystment, as observed in rodent models (22–24). The interaction between T. gondii cysts and human brain resident cells remains elusive. However, the limited data from autopsies have shown that cysts are present in neurons and astrocytes (25). In addition to exhibiting tropism for neurons, T. gondii encystment exhibits a high predilection to specific brain areas such as the amygdala, cerebral cortex, hippocampus, and cerebellum in rodents (26–30). The neuroanatomical distribution of cysts in humans is mainly evidenced through the autopsies of acquired immunodeficiency syndrome (AIDS) patients with toxoplasmic encephalitis (TE). TE lesions, which are an indication of cysts' presence and rupture, have been reported in the cerebral cortex, basal ganglia, and cerebellum (31–35).
Figure 1. Different stages of T. gondii infection in the host. Early or acute infection is characterised by the invasion and intracellular proliferation of tachyzoites in the parasitophorous vacuole (PV). Acute infection is often asymptomatic in healthy individuals. To establish life-long infection, T. gondii tachyzoites differentiate into bradyzoites within a cyst. Immune-privileged sites such as the brain provide a niche for T. gondii encystment. Chronic infection in the brain has been linked to the incidences of neuropsychiatric conditions. Upon immune suppression, encysted bradyzoites reconvert into tachyzoites, causing toxoplasmic encephalitis in immunocompromised patients.
The strong tropism and persistence of T. gondii cysts in the brain confirm that CNS toxoplasmosis impairs the complex brain functioning. Human infection produces a gamut of clinical manifestations, ranging from long-term behavioural and cognitive alterations, to mortality following reactivation of encysted bradyzoites upon immunosuppression (36).
Toxoplasmosis is an opportunistic infection in immunocompromised individuals. In reactivated toxoplasmosis, encysted bradyzoites transform back into tachyzoites and proliferate uncontrollably in the brain (Figure 1), causing widespread inflammation and critical morbidity. Reactivated toxoplasmosis develops in patients, such as those with human immunodeficiency virus (HIV)/AIDS, allogeneic haematopoietic stem cell transplant (allo-HSCT) and solid organ transplant (SOT) recipients (5, 37–39), because their T-cell repertoire is perturbed which weakens the host's antiparasitic responses. In HIV/AIDS patients, especially in those with a low CD4+ T-cell titre (<200/ml), reactivation of T. gondii brain cysts often manifests as TE (40). In allo-HSCT and SOT recipients, cerebral toxoplasmosis accounts for most post-transplant CNS infections, and TE remains the usual presentation (5, 38). Notably, in all cases, the clinical course of untreated TE is fatal.
Unlike in immunocompromised patients, brain cyst reactivation and TE are rare in immunocompetent adults with latent toxoplasmosis. T. gondii cysts persist in the brain for a long time. Latent CNS toxoplasmosis manifests asymptomatically in immunocompetent adults because cysts are largely quiescent. However, growing evidence shows that latent infection negatively affects neurocognitive functions. In intermediate rodent hosts, chronic infection is associated with behavioural changes such as reduced anxiety-like behaviour, neophobia, or more specifically, the fear of feline predators (26, 41, 42). Similar sequelae were also observed in infected humans. Latent CNS toxoplasmosis is consistently linked to schizophrenia (43–46). Various meta-analyses have also reported its probable association with anxiety disorders (47), bipolar disorder (43, 48), depression (49), epilepsy (50), and obsessive-compulsive disorder (51), with all these associations relying on the correlative data between T. gondii IgG seropositivity (a proxy for chronic infection) and disease incidence. The causal link between latent CNS toxoplasmosis and neuropsychiatric disorders in humans remains unclear. However, preclinical studies have suggested that neurological sequelae are triggered through the modulation of the brain and immune milieu. Detailing the neurobiology underlying the association between latent T. gondii infection and neuropsychiatric conditions is beyond the scope of this review [see reviews (52–55)]. However, this neurobiology can be explained by the influence of the parasite or cyst on: (1) specific brain regions for cognition, mood, and emotion processing; (2) neurotransmitter levels (56–61); (3) neuroinflammation (62, 63); and (4) neuroendocrine changes (64–66).
Chronic CNS toxoplasmosis has far-reaching consequences in humans. In immunocompetent individuals, T. gondii cysts establish a persistent brain infection that is linked to the incidence of neuropsychiatric disorders. Additionally, immunocompromised patients experience recrudescent latent infection. Given these dire consequences, treatments for preventing the formation or elimination of T. gondii cysts from the brain are urgently required to reduce public health burden.
The exponential rise in knowledge regarding T. gondii biology has proven beneficial to uncover new druggable targets for combating toxoplasmosis (67). Several prophylactic and therapeutic regimens have been adopted clinically [see reviews (68, 69)] (Figure 2, left). The standard treatment for toxoplasmosis involves a combination of pyrimethamine (Pyr) and sulfadiazine to inhibit the biosynthesis of folic acids (70)—the precursors for nucleic acid and protein synthesis in T. gondii tachyzoites. Folinic acid is often administered concurrently to minimise Pyr-induced haematologic toxicity (71). However, the Pyr–sulfadiazine treatment is associated with multiple adverse side effects, such as poor tolerability (72, 73), and emergence of T. gondii strains resistant to Pyr-based therapies (74). Other Pyr-based regimens such as the combination of Pyr with clindamycin (a protein synthesis inhibitor) or atovaquone (a mitochondrial inhibitor) have been adopted in patients with sulpha drug allergy (75, 76). Another regimen involving the combination of trimethoprim (an antagonist of folic acid synthesis) and sulfamethoxazole is also recommended (73). However, this drug combination is only adopted in lower-income countries where Pyr is unaffordable (77). Although other therapeutic options are available, none have been found to be more effective than Pyr–sulfadiazine.
Figure 2. Mechanistic targets of clinically approved anti-T. gondii drugs and the known targets of medicinal herbs, phytochemicals, and essential nutrients. (Left) Current clinical drugs target metabolic pathways in the tachyzoite. (Right) Nutritional products may alter bradyzoite biology and cyst wall integrity to alleviate cyst burden during chronic infection.
Unfortunately, all currently available therapeutic regimens are only effective against tachyzoites and cannot eliminate tissue cysts. Rational development of new and safe therapeutic interventions against chronic toxoplasmosis is urgently required.
The lack of clinical drugs that eliminate T. gondii cysts from the brain has driven the search for effective therapeutics. At least 50 promising anti-cyst candidates have been identified through screening of compound libraries, target-based drug design, or repurposing of Food and Drug Administration (FDA)-approved drugs [see review (78)]. For example, imiquimod has recently been identified as a promising prophylactic and therapeutic drug against brain cyst burden and cyst reactivation through its immunomodulatory role (79). While the discovery of pharmacological cysticidal agents is underway, nutritional therapy has emerged as a new approach to cope with chronic T. gondii infection. Nutritional therapy is based on the idea that the food we eat can influence health and disease development. This idea has evolved from ancient times when pharmaceutical drugs were unavailable. Despite the advancement of modern medicine, food ingredients are being investigated for their therapeutic potential.
In this review, we evaluate the contribution of nutritional products—extracts from food plants, phytochemicals, vitamins, and minerals—towards the prevention (prophylactic) or treatment (therapeutic) of chronic brain toxoplasmosis (Table 1). These products robustly impacted the brain cyst burden and neurological sequelae in preclinical models of chronic toxoplasmosis. Interestingly, most of the anti-cyst nutritional products also interfere with the tachyzoite stage. In the first part, we highlight the nutritional products without a disease-related target (section Nutritional Products Without Identified Targets). In the next section, we discuss a small pool of nutritional products that targets the bradyzoite biology to interfere with brain cyst development (section Nutritional Products With Identified Targets: Interfering With Bradyzoite Biology and Cyst Development) (Figure 2, right).
Herbs are extensively used for culinary purposes and are a crucial part of the human diet and culture. Additionally, herbal extracts are recognised for their health-promoting effects (104, 105). Different cultural or societal backgrounds (e.g., traditional Chinese medicine and traditional Korean medicine) offer the propriety of herbal products for medicinal use.
Myristica fragrans (nutmeg), an aromatic evergreen plant, is extensively used as a food spice and in folk medicine to treat ailments (106). Its bioactive constituent myrislignan guards against acute cerebral toxoplasmosis by perturbing the tachyzoite's mitochondrial function (107, 108). Myristica fragrans is also effective against the bradyzoite stage. In mice infected with the type II ME49 T. gondii strain, ethanolic extract from dried fruits of M. fragrans mildly protected against brain cyst development (84).
Nigella sativa (black cumin), another food flavouring agent, has an enduring history of usage in folk medicine (109). Extensive clinical trials have highlighted the safety and efficacy of N. sativa in the treatment of inflammatory, auto-immune, and metabolic disorders (110). The volatile oil component of N. sativa acts synergistically with the current clinical drug Pyr to reduce T. gondii load and pathological insults to the liver and spleen of acutely infected mice (85). N. sativa seed oil also has prophylactic and therapeutic effects on chronic infection. In a murine infection model induced with ME49 T. gondii, parasite cysts were accumulated in the brain 5 weeks after infection, eventually leading to death of the mice (86). However, N. sativa seed oil administered before infection or a few days after infection significantly reduced the mortality and the brain cyst load in infected animals (86). The treated groups also exhibited less brain histopathological alterations compared with the untreated group (86). An enhanced brain level of inducible nitric oxide synthase (iNOS) was suggested to protect the treated animals against immunopathology (86). Interestingly, the prophylactic regimen is more effective than the therapeutic regimen in enhancing resistance to chronic toxoplasmosis (86).
Species of the Thymus genus, a group of aromatic herbs used as flavour additives, have been extensively studied for their antimicrobial activities (111, 112). Administering essential oils from Thymus broussonetii Boiss at the time of infection or a few days after infection prevented chronic brain toxoplasmosis. Mice infected with the type II Prugniaud (PRU) T. gondii strain developed intracerebral cysts after 3 weeks (91). However, animals treated with the oil extract did not exhibit brain cysts (91). In another study, ethanolic extract from the leaves and ariel parts of Thymus vulgaris reduced the severity of chronic infection. Administration of the herbal extract prophylactically to chronically infected mice has been shown to reduce the brain cyst load and ameliorate the inflammatory brain lesions (84, 92).
Polyphenols are produced by plants to combat environmental insults (113). In humans, dietary intake of fruits and vegetables rich in these phytochemicals promotes preventive and therapeutic benefits against various disorders such as cancer, cardiovascular diseases, chronic degenerative diseases, and metabolic syndromes (114). In addition to their medicinal application, these natural compounds are being targeted by the blooming nanotechnology industry. With nanotechnology, the stability, delivery, and release of dietary polyphenols to the brain tissue have markedly improved, greatly enhancing the clinical translatability of these compounds to treat CNS diseases (115).
The rhizome of Curcuma longa (turmeric), a spice best known for its use in curry, exhibits therapeutic potential against various diseases. Its potential can be attributed to the presence of curcumin, a highly pleiotropic compound that targets multiple biological signalling pathways (116). For example, curcumin targets the T. gondii detoxification pathway and blocks parasite growth by inhibiting T. gondii glyoxalase 1 activity (117). Extensive clinical trials have highlighted the safety and efficacy of curcumin in treating multiple human diseases (118). Curcumin has been designated as a Generally Recognised as Safe (GRAS) dietary compound by FDA. However, the bioactivity of curcumin is often limited by its low oral bioavailability (119). Nanocurcumin (encapsulation of curcumin in nanomaterials) has improved the solubility and therapeutic applications of curcumin. Curcumin and nanocurcumin have been widely investigated as antiparasitic agents (120). In an experimental model of acute toxoplasmosis, curcumin and curcumin nanoemulsion improved survival and reduced peritoneal tachyzoite load in the mice infected with the virulent type I RH T. gondii strain (82). In a chronic experimental paradigm, curcumin and the nanoemulsion administered shortly after infection with type II Tehran T. gondii were found to reduce the brain cyst number and cyst size in chronically infected mice (82). However, the nanoemulsion was found to be more effective in alleviating brain cyst burden than the native curcumin (82). Curcumin and a novel curcumin nanocomposite (prepared by reacting curcumin with amino-functionalised metal-organic frameworks) also reduced brain cyst load in chronically infected rats (83); the nanocomposite yielded a better effect than the native compound in alleviating cyst burden (83).
Resveratrol is a polyphenol enriched in multiple common food products such as berries, grapes, and peanuts. Several in vitro and in vivo studies have shown that similar to curcumin, resveratrol targets multiple cellular signalling molecules and can affect the initiation and progression of human diseases (121). The efficacy, safety, and pharmacokinetics of resveratrol have been investigated in numerous clinical trials, with the nutraceutical exhibiting promising therapeutic effects against cancer and neurological, cardiovascular, and metabolic diseases (122, 123). Nanotechnology-based formulations for resveratrol have been developed to enhance its bioactivity and bioavailability. Native resveratrol and the nanoparticles displayed synergy with sulfamethoxazole–trimethoprim (ST) to treat chronic T. gondii infection. In mice infected with type III VEG T. gondii, resveratrol alone showed no significant effect on the brain cyst burden (95–97); however, when co-administered with ST, resveratrol significantly reduced the number of brain cysts and increased the brain antioxidant levels (95–97). Specifically, the pairing of resveratrol nanoparticles with ST has the greatest effect on alleviating brain cyst burden, decreasing the levels of serum pro-inflammatory cytokines, and increasing the level of interleukin-10 (95). The anti-inflammatory effect could be partly mediated by the reduction in purinergic signalling (124, 125), which is a pro-inflammatory immunological pathway that is upregulated during cerebral infection (126). The resveratrol + ST regimen also mitigated downstream behavioural and cognitive impairments observed during chronic CNS infection. Chronically infected animals administered with resveratrol (free or nanoform) and ST spent less time in the open arms of the elevated plus-maze, displaying heightened anxiety behaviour compared with untreated animals (97). The regimen also led to memory improvement in the infected mice in the passive avoidance task (97). On the other hand, administration of free resveratrol or the nanoparticles or ST alone showed no effect on the neurological sequelae (97).
Pomegranate is a rich source of ellagitannins, a polyphenol class metabolised to urolithins by the human gut microbiota (127). Urolithin-A (UA) is regarded as powerful neuroprotectant in vivo against neurodegeneration, ischaemic neuronal injury, and brain ageing (128–135). In a pioneer human clinical trial, UA displayed a favourable safety profile and molecular signature for improved muscle health in elderly individuals (133). Our group recently showed that pomegranate and UA exhibit anti-T. gondii activity. Urolithin-A treatment interfered with intracellular tachyzoite growth and cyst formation in infected neural cells (94). In vivo, daily injection of UA shortly after PRU T. gondii infection reduced the severity of chronic CNS infection, which was evident from the smaller brain cysts in chronically infected mice than those in untreated animals (94). Interestingly, chronic infection reduces innate fear of mice towards predatory odour (26, 41, 42). However, UA attenuated the anxiolytic behaviour in chronically infected mice, as evident from the increased fear of these animals towards predatory odour compared with untreated animals (94).
Tanshinone IIA is a major lipophilic phytochemical isolated from the roots of Salvia miltiorrhiza Bunge (red sage). This herbal medicine is widely prescribed to treat cardiovascular and inflammatory diseases (136). In a chemical compound library screening to identify anti-T. gondii drugs, tanshinone IIA was considered active against in vitro tachyzoites and bradyzoites and thus a promising therapeutic candidate for acute and chronic infections (89). However, its effectiveness in vivo, particularly the effect against cerebral cyst burden, remains to be investigated.
Vitamins and minerals are essential micronutrients present in various foods that coordinate a range of human physiological functions. The consumption of different nutritious foods is the best approach to obtain vital nutrients. Vitamins C, D, and E act against T. gondii tachyzoites potentially by activating the NO-mediated mechanism to enhance parasite killing (137–139). However, vitamin E seems to be detrimental to chronic toxoplasmosis development. Dietary administration of vitamin E along with selenium prior to infection increased toxoplasmosis severity in infected mice, as evident from the higher cyst burden and severe meningoencephalitis in the brain tissues of these mice (100). The mechanism through which these micronutrients aggravate the phenotype remains unknown; however, preliminary evidence suggests that vitamin E and selenium perturb the protective cytokine expression (140).
Dietary intake of a small quantity of trace minerals, which play crucial roles at cellular and tissue levels, is important mainly for bodily functions and the immune system. Selenium, zinc, and copper are the trace minerals that influence the development of acute and chronic toxoplasmosis. Studies have explored the impact of biogenic minerals, which exhibit reduced toxicity due to the coating of plant molecules, on toxoplasmosis. Biogenic selenium orally administered to acutely infected mice reduced the peritoneal tachyzoite load and mortality (141). In chronic experiments, early supplementation with biogenic selenium, zinc, or copper prophylactically reduced the number of brain cysts or completely prevented their development (101–103). Additionally, these animals exhibited increased iNOS and reduced pro-inflammatory cytokine levels, which suggest the role of these biogenic minerals in strengthening the cellular immune system and protecting animals against harmful neuroinflammatory responses (101–103).
Differentiation of tachyzoites into encysted bradyzoites is critical for the persistence of brain toxoplasmosis. Hence, the strategies that interfere with this transformation can reduce cyst formation. The molecular determinants that underlie this transformation have been extensively investigated. Recent discoveries of transcriptional programmes governing this switch have unveiled new therapeutic targets to cease parasite encystment (142–146).
Heat shock proteins (HSPs) are evolutionarily conserved proteins that are expressed in many organisms under stress (147). In in vitro culture, stressors such as an increase in pH and temperature can induce tachyzoite differentiation into bradyzoites (148). During this transformation, T. gondii upregulates HSP70 and HSP90 expressions (93, 149). HSP70 expression was also detected in the brain cysts of chronically infected mice (150). The molecular role of HSP during differentiation remains unexplored; however, a study demonstrated that HSP90-deficient parasites could not develop into bradyzoites (149). Quercetin, a ubiquitous flavonoid common in many fruits and vegetables, suppresses tachyzoite differentiation into bradyzoites in vitro plausibly by inhibiting T. gondii HSP70 and HSP90 synthesis (93). Although whether quercetin could influence the brain cyst burden in vivo is unknown, its ability to cross the BBB suggests its potential therapeutic value against chronic CNS toxoplasmosis (151, 152).
Following tachyzoite differentiation into bradyzoites, the PV membrane encapsulating the tachyzoites matures into the cyst wall (153). The cyst wall is composed of a loose inner layer facing the cyst matrix, a dense middle layer, and an outer membrane layer (154). This wall shields the bradyzoites from immune recognition and destruction. Given its role in survival and persistence of bradyzoites, the cyst wall is an attractive pharmacological target for preventing proper cyst development.
Alcoholic and oil extracts of Rosmarinus officinalis (rosemary), a medicinal herb commonly used as a culinary spice, exert remarkable prophylactic and therapeutic effects on chronic toxoplasmosis by perturbing cyst wall integrity. Administration of alcoholic and oil extracts from R. officinalis leaves to mice 1 week before infection or 2 weeks following infection with ME49 T. gondii significantly reduced the brain cyst burden, cyst viability, and histopathological insults compared with that in the untreated group (88). Ultrastructural analysis of the cyst through scanning electron microscopy revealed abnormal cyst morphology in the brain of the treated animals, with the cyst surface marked with multiple depressions, protrusions, and irregularities (88). Isolation and inoculation of these damaged cysts into naive mice did not produce successful secondary infection, highlighting a loss in cyst viability (88). Overall, R. officinalis extracts acted as a stronger therapeutic agent than a prophylactic agent.
Artemisinin, the bioactive constituent of the traditional Chinese herb Artemisia annua, and its derivatives represent a powerful class of medicines against malaria (155). The similarity in morphology and biochemistry of T. gondii and Plasmodium has led to the repurposing of artemisinin and its derivatives for toxoplasmosis treatment (156). Artesunate, artemether, and other novel derivatives have been reported to exhibit better potency than artemisinin against tachyzoite growth in vitro (80, 157–159). Artemiside and artemisone are two other derivatives that could control acute infection and protect chronically infected mice from reactivated toxoplasmosis (160). Artemisinin appears to act through multiple targets to control tachyzoite growth, for example, by interfering with the parasite's calcium homeostasis and mitochondrial physiology (161–163). The C-10 carba-linked unsaturated derivative CPH4-136 significantly reduced the brain cyst burden in chronically infected mice (81). In another study, co-administration of artesunate and dihydroartemisinin interfered with chronic toxoplasmosis progression by perturbing cyst wall integrity. Feeding artesunate–dihydroartemisinin to the mice chronically infected with the low-virulence DUR T. gondii strain resulted in smaller brain cysts and abnormal cyst morphology characterised by the alteration of inner membranes (80). However, these cysts remained viable and continued to produce secondary infection in mice (80).
T. gondii is auxotrophic for several essential metabolites and therefore depends on the host cell for nutrients. Bradyzoites can access the host's cytoplasm and scavenge nutrients, even when encysted, to meet their energy and anabolic requirements (164–166). T. gondii also modulates host's metabolic pathways during infection, thereby increasing the metabolite abundance to support toxoplasmosis progression (167). Metabolic profiling of the cerebral cortex in infected mice revealed a time-dependent upregulation of the metabolites associated with the unsaturated fatty acid (FA) biosynthesis pathway (168). This hints at the role of unsaturated FA in the pathogenesis and progression of cerebral toxoplasmosis. T. gondii bradyzoites scavenge and sequester the host's unsaturated FA as an energy source, a trait seemingly critical for proper cyst development (164). Strategies that change the host's reservoir of unsaturated FA could influence cyst development and the progression of chronic toxoplasmosis.
Lipids are a crucial energy source and structural element for cell membranes. Humans can synthesise almost all types of lipids except for unsaturated FA, which they need to acquire from diets including fish, vegetables, and nut-based oils (169). A few studies have highlighted the in vitro and in vivo efficacy of polyunsaturated FA against acute and chronic toxoplasmosis, possibly by inducing overt FA metabolism in T. gondii. Unsaturated FA made tachyzoites more vulnerable to lipotoxicity by inducing improper lipid storage in the parasites (164). Feeding mice with fish oil prevented toxoplasmosis potentially by disturbing the host's and parasite's FA metabolism and FA availability for parasite growth (99). Chronically infected transgenic mice with constantly elevated levels of omega-3 polyunsaturated FA exhibited lower brain cyst burden than wild-type mice (98).
Preclinical studies have highlighted the impact of nutritional products on the development of chronic CNS toxoplasmosis. However, as with all animal studies, the validity of the preclinical findings for clinical practice remains questionable. Here, we discuss the factors that could limit the translatability of animal studies and nutritional products and highlight potential strategies that could improve translational success.
Most of the aforementioned animal studies are in their pilot or preliminary stage. These studies have typically evaluated the therapeutic efficacy of a nutritional product with a specified administration route, a single or limited number of dosing, and within a predetermined treatment window period. Extensive optimisation and assessment of pharmacokinetics/pharmacokinetics of the nutritional products are required to translate the preclinical efficacy to human subjects. Moreover, chronic infection in animal models adopted in these studies was induced with a single T. gondii strain and in a predefined window period. Due to varying virulence across the parasite strains and the different incubation periods for chronic infection depending on the host species, no single animal model can reflect the development and progression of chronic toxoplasmosis in humans. Overall, the lack of extensive knowledge about the pharmacological properties of nutritional products and an appropriate infection model impedes clinical translation. To this end, delineating the ADMET (absorption, distribution, metabolism, excretion, and toxicity) profiles of the nutritional products can aid optimisation for subsequent preclinical therapeutic studies (170). Uncovering mechanistic targets of the nutritional products will also help in assessing the bioavailability, engagement, and expression of pharmacological activity at the target site. To improve the predictive translatability of animal models, the nutritional products must be tested in a large sample and in different infection models. Studies involving immunodeficient animal models (e.g., dexamethasone-induced immunosuppression) could also help in determining whether nutrition mitigates the recrudescence of latent CNS infection (79).
Rigour interrogation of animal studies can improve the translation process for clinical trials. However, the properties of the nutritional products per se could also limit their intrinsic translation value. Oral bioavailability is probably the greatest bottleneck that limits the therapeutic efficacy of nutritional products in humans. While polyphenols have shown an impact on brain cyst burden in animal models (section Phytochemicals), consumed polyphenols such as curcumin, resveratrol, and quercetin have very low oral bioavailability in humans due to extensive metabolism and rapid elimination from the body (171). Moreover, the water-soluble nature of polyphenols can remarkably impede their uptake across the BBB. Therefore, several studies have used nanodelivery systems to circumvent the difficulties associated with poor blood plasma and tissue bioavailability of polyphenols. Consequently, preclinical success has been achieved in improving the efficacy of curcumin and resveratrol to target T. gondii cysts in the brain (section Phytochemicals). The design of semisynthetic derivatives represents another strategy to improve bioavailability. Identification of the structural modifications that improve the stability of phytochemicals in the blood plasma can enhance therapeutic effectiveness. Successful examples include artemisinin derivatives, such as FDA-approved artesunate, artemether, and artemisone. These compounds have better plasma stability, low neurotoxicity, and high potency against toxoplasmosis (section Interfering with Cyst Wall Integrity). Overall, the improved blood plasma and tissue concentrations of nutritional compounds indicate that a low dosage may be required for therapy, which in turn may produce an effective treatment with fewer side effects in humans.
Side effects and tolerance are important considerations for the clinical translatability of any treatment. Most animal studies discussed in this review have considered the cell/tissue injury markers and survival rate as endpoints to evaluate nutritional product toxicity. None of the studies have reported significant toxicity of these products to the host cells and animals. Large-scale intervention trials conducted for curcumin, resveratrol, vitamins, and minerals for human diseases have also established the toxicology profiles for these products (118, 122), thereby laying a foundation for determining the dosing regimens of these products for chronic toxoplasmosis management in a straightforward manner.
Although targeting cyst development is an attractive strategy to interfere with the establishment of chronic brain infection, administering therapy solely targeting the bradyzoite stage may incur the risk of overt tachyzoite accumulation in peripheral tissues. While a healthy immune system can clear peripheral tachyzoites, immunocompromised patients may experience consequences of the parasite burden. Hence, the treatment for chronic brain toxoplasmosis should be effective against both tachyzoite and bradyzoite stages. Most nutritional products highlighted in the present review can target both parasite stages, representing a unique pool of products with dual pharmacological activities. Otherwise, an anti-tachyzoite drug needs to be co-administered with an anti-cyst agent to prevent overt tachyzoite accumulation. Exploring whether anti-cyst nutritive products can act synergistically with current clinical drugs (which are effective only against tachyzoites) to eliminate human infection would be interesting.
The absence of clinically appropriate biomarkers for measuring brain cyst burden is probably the most significant impediment in directly assessing the effectiveness of nutritional therapy in humans. Routine diagnosis of toxoplasmosis in humans relies on detection of parasite's DNA in body fluids through serologic testing or molecular techniques (172). Neuroimaging techniques that are particularly relevant to immunocompromised patients with CNS toxoplasmosis, such as magnetic resonance imaging (MRI) and computed tomography (CT), can efficiently determine TE severity based on ring-enhancing brain lesions (39). However, these tests do not provide direct readouts for the brain cyst burden. The lack of non-invasive techniques or practical quantitative biomarkers to monitor bradyzoite development in the brain makes it difficult to evaluate the efficacy of nutritional therapy in humans. This is particularly a problem when monitoring disease progression in immunocompetent patients with subclinical chronic infection. Consequently, nutritional therapy might need to be validated by monitoring clinical manifestations central to chronic CNS toxoplasmosis. In immunocompetent patients, the emerging association between chronic infection and neuropsychiatric conditions suggests that cognitive failure can predict a latent infection. Thus, the effectiveness of nutritional therapy can be evaluated by monitoring the severity of behavioural symptoms over the course of intervention. In schizophrenic patients with presumptive latent toxoplasmosis, two pilot studies have investigated the effect of co-administering artemisinin or artemether with their usual psychiatric medications and determined if the clinical benefits could be augmented by inhibiting brain parasitism (173, 174). Although the regimens were found to be safe and well-tolerated, adjunctive artemisinin or artemether did not alleviate psychotic symptoms or cognitive deficits (173, 174). In immunocompromised patients, MRI or CT can provide information on TE lesions, which are located proximal to the cysts.
Chronic brain toxoplasmosis poses a significant health threat, causing severe neurological manifestations in immunocompetent adults and immunocompromised patients. Despite persistent efforts by the global disease control group, none of the drugs have been found to successfully eliminate T. gondii cysts from the brain. During the last few decades, the interest in repurposing nutritional products with a long-standing history of health benefits as medicinal sources for chronic CNS infection has increased. Preclinical studies have highlighted the promising potential of nutritive food products to prevent or interfere with disease progression; however, evidence supporting human clinical intervention is lacking. To improve the translation process, preclinical studies need to be conducted with the same rigour analogous to clinical trials. There is also a need to identify clinically appropriate biomarkers to monitor the progression of CNS toxoplasmosis. Finally, large-scale clinical trials are warranted to validate the relevance of nutritional therapy for managing human infection. Currently, polyphenols are probably the most promising candidates, as they have been investigated in multiple clinical trials for other human diseases.
ST and WHT prepared the manuscript. AV reviewed the submitted version. All authors made substantial intellectual contributions to the work and approved the final manuscript.
This work was financially supported by the Ministry of Education, Singapore (grant RG136/15) and Human Frontier Science Programme (grant RGP0062/2018).
The authors declare that the research was conducted in the absence of any commercial or financial relationships that could be construed as a potential conflict of interest.
All claims expressed in this article are solely those of the authors and do not necessarily represent those of their affiliated organizations, or those of the publisher, the editors and the reviewers. Any product that may be evaluated in this article, or claim that may be made by its manufacturer, is not guaranteed or endorsed by the publisher.
1. Shwab EK, Zhu X-Q, Majumdar D, Pena HF, Gennari SM, Dubey JP, et al. Geographical patterns of Toxoplasma gondii genetic diversity revealed by multilocus PCR-RFLP genotyping. Parasitology. (2014) 141:453–61. doi: 10.1017/S0031182013001844
2. Su C, Khan A, Zhou P, Majumdar D, Ajzenberg D, Dardé M-L, et al. Globally diverse Toxoplasma gondii isolates comprise six major clades originating from a small number of distinct ancestral lineages. Proc Nat Acad Sci USA. (2012) 109:5844–9. doi: 10.1073/pnas.1203190109
3. Pinto-Ferreira F, Caldart ET, Pasquali AKS, Mitsuka-Breganó R, Freire RL, Navarro IT. Patterns of transmission and sources of infection in outbreaks of human toxoplasmosis. Emerg Infect Dis. (2019) 25:2177. doi: 10.3201/eid2512.181565
4. Torgerson PR, Mastroiacovo P. The global burden of congenital toxoplasmosis: a systematic review. Bull World Health Organ. (2013) 91:501–8. doi: 10.2471/BLT.12.111732
5. Khurana S, Batra N. Toxoplasmosis in organ transplant recipients: evaluation, implication, and prevention. Trop Parasitol. (2016) 6:123. doi: 10.4103/2229-5070.190814
6. Blader IJ, Coleman BI, Chen C-T, Gubbels M-J. Lytic cycle of Toxoplasma gondii: 15 years later. Annu Rev Microbiol. (2015) 69:463–85. doi: 10.1146/annurev-micro-091014-104100
7. Lambert H, Hitziger N, Dellacasa I, Svensson M, Barragan A. Induction of dendritic cell migration upon Toxoplasma gondii infection potentiates parasite dissemination. Cell Microbiol. (2006) 8:1611–23. doi: 10.1111/j.1462-5822.2006.00735.x
8. Fuks JM, Arrighi RB, Weidner JM, Mendu SK, Jin Z, Wallin RP, et al. GABAergic signaling is linked to a hypermigratory phenotype in dendritic cells infected by Toxoplasma gondii. PLoS Pathog. (2012) 8:e1003051. doi: 10.1371/journal.ppat.1003051
9. Weidner JM, Kanatani S, Hernández-Castañeda MA, Fuks JM, Rethi B, Wallin RP, et al. Rapid cytoskeleton remodelling in dendritic cells following invasion by Toxoplasma gondii coincides with the onset of a hypermigratory phenotype. Cell Microbiol. (2013) 15:1735–52. doi: 10.1111/cmi.12145
10. Kanatani S, Fuks JM, Olafsson EB, Westermark L, Chambers B, Varas-Godoy M, et al. Voltage-dependent calcium channel signaling mediates GABAA receptor-induced migratory activation of dendritic cells infected by Toxoplasma gondii. PLoS Pathog. (2017) 13:e1006739. doi: 10.1371/journal.ppat.1006739
11. Ólafsson EB, Ross EC, Varas-Godoy M, Barragan A. TIMP-1 promotes hypermigration of Toxoplasma-infected primary dendritic cells via CD63–ITGB1–FAK signaling. J Cell Sci. (2019) 132:jcs225193. doi: 10.1242/jcs.225193
12. Drewry LL, Jones NG, Wang Q, Onken MD, Miller MJ, Sibley LD. The secreted kinase ROP17 promotes Toxoplasma gondii dissemination by hijacking monocyte tissue migration. Nat Microbiol. (2019) 4:1951–63. doi: 10.1038/s41564-019-0504-8
13. Ólafsson EB, Barragan A. The unicellular eukaryotic parasite Toxoplasma gondii hijacks the migration machinery of mononuclear phagocytes to promote its dissemination. Biol Cell. (2020) 112:239–50. doi: 10.1111/boc.202000005
14. Courret N, Darche S, Sonigo P, Milon G, Buzoni-Gâtel D, Tardieux I. CD11c-and CD11b-expressing mouse leukocytes transport single Toxoplasma gondii tachyzoites to the brain. Blood. (2006) 107:309–16. doi: 10.1182/blood-2005-02-0666
15. Lachenmaier SM, Deli MA, Meissner M, Liesenfeld O. Intracellular transport of Toxoplasma gondii through the blood–brain barrier. J Neuroimmunol. (2011) 232:119–30. doi: 10.1016/j.jneuroim.2010.10.029
16. Ueno N, Harker KS, Clarke EV, McWhorter FY, Liu WF, Tenner AJ, et al. Real-time imaging of Toxoplasma-infected human monocytes under fluidic shear stress reveals rapid translocation of intracellular parasites across endothelial barriers. Cell Microbiol. (2014) 16:580–95. doi: 10.1111/cmi.12239
17. Konradt C, Ueno N, Christian DA, Delong JH, Pritchard GH, Herz J, et al. Endothelial cells are a replicative niche for entry of Toxoplasma gondii to the central nervous system. Nat Microbiol. (2016) 1:1–8. doi: 10.1038/nmicrobiol.2016.1
18. Dupont CD, Christian DA, Hunter CA. Immune response and immunopathology during toxoplasmosis. Semin Immunopathol. (2012) 34:793–813. doi: 10.1007/s00281-012-0339-3
19. Sasai M, Pradipta A, Yamamoto M. Host immune responses to Toxoplasma gondii. Int Immunol. (2018) 30:113–9. doi: 10.1093/intimm/dxy004
20. Sasai M, Yamamoto M. Innate, adaptive, and cell-autonomous immunity against Toxoplasma gondii infection. Exp Mol Med. (2019) 51:1–10. doi: 10.1038/s12276-019-0353-9
21. Weiss LM, Kim K. The development and biology of bradyzoites of Toxoplasma gondii. Front Biosci. (2000) 5:D391–405. doi: 10.2741/Weiss
22. Ferguson D, Hutchison W. An ultrastructural study of the early development and tissue cyst formation of Toxoplasma gondii in the brains of mice. Parasitol Res. (1987) 73:483–91. doi: 10.1007/BF00535321
23. Melzer T, Cranston H, Weiss L, Halonen S. Host cell preference of Toxoplasma gondii cysts in murine brain: a confocal study. J Neuroparasitol. (2010) 1:N100505. doi: 10.4303/jnp/N100505
24. Cabral CM, Tuladhar S, Dietrich HK, Nguyen E, MacDonald WR, Trivedi T, et al. Neurons are the primary target cell for the brain-tropic intracellular parasite Toxoplasma gondii. PLoS Pathog. (2016) 12:e1005447. doi: 10.1371/journal.ppat.1005447
25. Alvarado-Esquivel C, Sánchez-Anguiano LF, Mendoza-Larios A, Hernández-Tinoco J, Pérez-Ochoa JF, Antuna-Salcido EI, et al. Prevalence of Toxoplasma gondii infection in brain and heart by immunohistochemistry in a hospital-based autopsy series in Durango, Mexico. Eur J Microbiol Immunol. (2015) 5:143–9. doi: 10.1556/1886.2015.00014
26. Vyas A, Kim S-K, Giacomini N, Boothroyd JC, Sapolsky RM. Behavioral changes induced by Toxoplasma infection of rodents are highly specific to aversion of cat odors. Proc Nat Acad Sci USA. (2007) 104:6442–7. doi: 10.1073/pnas.0608310104
27. Di Cristina M, Marocco D, Galizi R, Proietti C, Spaccapelo R, Crisanti A. Temporal and spatial distribution of Toxoplasma gondii differentiation into bradyzoites and tissue cyst formation in vivo. Infect Immun. (2008) 76:3491–501. doi: 10.1128/IAI.00254-08
28. Hermes G, Ajioka JW, Kelly KA, Mui E, Roberts F, Kasza K, et al. Neurological and behavioral abnormalities, ventricular dilatation, altered cellular functions, inflammation, and neuronal injury in brains of mice due to common, persistent, parasitic infection. J Neuroinflamm. (2008) 5:48. doi: 10.1186/1742-2094-5-48
29. Berenreiterová M, Flegr J, Kuběna AA, Němec P. The distribution of Toxoplasma gondii cysts in the brain of a mouse with latent toxoplasmosis: implications for the behavioral manipulation hypothesis. PLoS ONE. (2011) 6:e28925. doi: 10.1371/journal.pone.0028925
30. Dubey JP, Ferreira LR, Alsaad M, Verma SK, Alves DA, Holland GN, et al. Experimental toxoplasmosis in rats induced orally with eleven strains of Toxoplasma gondii of seven genotypes: tissue tropism, tissue cyst size, neural lesions, tissue cyst rupture without reactivation, and ocular lesions. PLoS ONE. (2016) 11:e0156255. doi: 10.1371/journal.pone.0156255
31. Lang W, Miklossy J, Deruaz J, Pizzolato G, Probst A, Schaffner T, et al. Neuropathology of the acquired immune deficiency syndrome (AIDS): a report of 135 consecutive autopsy cases from Switzerland. Acta Neuropathol. (1989) 77:379–90. doi: 10.1007/BF00687372
32. Strittmatter C, Lang W, Wiestler O, Kleihues P. The changing pattern of human immunodeficiency virus-associated cerebral toxoplasmosis: a study of 46 postmortem cases. Acta Neuropathol. (1992) 83:475–81. doi: 10.1007/BF00310023
33. Porter SB, Sande MA. Toxoplasmosis of the central nervous system in the acquired immunodeficiency syndrome. N Engl J Med. (1992) 327:1643–8. doi: 10.1056/NEJM199212033272306
34. Laing R, Flegg P, Brettle R, Leen C, Burns S. Clinical features, outcome and survival from cerebral toxoplasmosis in Edinburgh AIDS patients. Int J STD AIDS. (1996) 7:258–64. doi: 10.1258/0956462961917933
35. Arendt G, von Giesen HJ, Hefter H, Neuen-Jacob E, Roick H, Jablonowski H. Long-term course and outcome in AIDS patients with cerebral toxoplasmosis. Acta Neurol Scand. (1999) 100:178–84. doi: 10.1111/j.1600-0404.1999.tb00735.x
36. Daher D, Shaghlil A, Sobh E, Hamie M, Hassan ME, Moumneh MB, et al. Comprehensive overview of Toxoplasma gondii-induced and associated diseases. Pathogens. (2021) 10:1351. doi: 10.3390/pathogens10111351
37. Wang Z-D, Wang S-C, Liu H-H, Ma H-Y, Li Z-Y, Wei F, et al. Prevalence and burden of Toxoplasma gondii infection in HIV-infected people: a systematic review and meta-analysis. Lancet HIV. (2017) 4:e177–e88. doi: 10.1016/S2352-3018(17)30005-X
38. Dard C, Marty P, Brenier-Pinchart M-P, Garnaud C, Fricker-Hidalgo H, Pelloux H, et al. Management of toxoplasmosis in transplant recipients: an update. Expert Rev Anti Infect Ther. (2018) 16:447–60. doi: 10.1080/14787210.2018.1483721
39. Vidal JE. HIV-related cerebral toxoplasmosis revisited: current concepts and controversies of an old disease. J Int Assoc Provid AIDS Care. (2019) 18:2325958219867315. doi: 10.1177/2325958219867315
40. Kaplan JE, Benson C, Holmes KK, Brooks JT, Pau A, Masur H, et al. Guidelines for prevention and treatment of opportunistic infections in HIV-infected adults and adolescents. MMWR Recomm Rep. (2009) 58:1–207.
41. Berdoy M, Webster JP, Macdonald DW. Fatal attraction in rats infected with Toxoplasma gondii. Proc Roy Soc Lond B Biol Sci. (2000) 267:1591–4. doi: 10.1098/rspb.2000.1182
42. Vyas A. Mechanisms of host behavioral change in Toxoplasma gondii rodent association. PLoS Pathog. (2015) 11:e1004935. doi: 10.1371/journal.ppat.1004935
43. Sutterland A, Fond G, Kuin A, Koeter M, Lutter R, Van Gool T, et al. Beyond the association. Toxoplasma gondii in schizophrenia, bipolar disorder, and addiction: systematic review and meta-analysis. Acta Psychiatr Scand. (2015) 132:161–79. doi: 10.1111/acps.12423
44. Xiao J, Prandovszky E, Kannan G, Pletnikov MV, Dickerson F, Severance EG, et al. Toxoplasma gondii: biological parameters of the connection to schizophrenia. Schizophr Bull. (2018) 44:983–92. doi: 10.1093/schbul/sby082
45. Fond G, Boyer L, Schürhoff F, Berna F, Godin O, Bulzacka E, et al. Latent toxoplasma infection in real-world schizophrenia: results from the national FACE-SZ cohort. Schizophr Res. (2018) 201:373–80. doi: 10.1016/j.schres.2018.05.007
46. Sutterland AL, Mounir DA, Ribbens JJ, Kuiper B, van Gool T, de Haan L. Toxoplasma gondii infection and clinical characteristics of patients with schizophrenia: a systematic review and meta-analysis. Schizophr Bull Open. (2020) 1:sgaa042. doi: 10.1093/schizbullopen/sgaa042
47. Markovitz AA, Simanek AM, Yolken RH, Galea S, Koenen KC, Chen S, et al. Toxoplasma gondii and anxiety disorders in a community-based sample. Brain Behav Immun. (2015) 43:192–7. doi: 10.1016/j.bbi.2014.08.001
48. de Barros JLVM, Barbosa IG, Salem H, Rocha NP, Kummer A, Okusaga OO, et al. Is there any association between Toxoplasma gondii infection and bipolar disorder? A systematic review and meta-analysis. J Affect Disord. (2017) 209:59–65. doi: 10.1016/j.jad.2016.11.016
49. Nayeri Chegeni T, Sharif M, Sarvi S, Moosazadeh M, Montazeri M, Aghayan SA, et al. Is there any association between Toxoplasma gondii infection and depression? A systematic review and meta-analysis. PLoS ONE. (2019) 14:e0218524. doi: 10.1371/journal.pone.0218524
50. Sadeghi M, Riahi SM, Mohammadi M, Saber V, Aghamolaie S, Moghaddam SA, et al. An updated meta-analysis of the association between Toxoplasma gondii infection and risk of epilepsy. Trans R Soc Trop Med Hyg. (2019) 113:453–62. doi: 10.1093/trstmh/trz025
51. Nayeri Chegeni T, Sarvi S, Amouei A, Moosazadeh M, Hosseininejad ZA, Aghayan S, et al. Relationship between toxoplasmosis and obsessive compulsive disorder: A systematic review and meta-analysis. PLoS Neglect Trop Dis. (2019) 13:e0007306. doi: 10.1371/journal.pntd.0007306
52. Tedford E, McConkey G. Neurophysiological changes induced by chronic Toxoplasma gondii infection. Pathogens. (2017) 6:19. doi: 10.3390/pathogens6020019
53. Tyebji S, Seizova S, Hannan AJ, Tonkin CJ. Toxoplasmosis: a pathway to neuropsychiatric disorders. Neurosci Biobehav Rev. (2019) 96:72–92. doi: 10.1016/j.neubiorev.2018.11.012
54. Milne G, Webster JP, Walker M. Toxoplasma gondii: an underestimated threat? Trends Parasitol. (2020) 36:P959–69. doi: 10.1016/j.pt.2020.08.005
55. Tong WH, Pavey C, O'Handley R, Vyas A. Behavioral biology of Toxoplasma gondii infection. Parasit Vectors. (2021) 14:77. doi: 10.1186/s13071-020-04528-x
56. Stibbs H. Changes in brain concentrations of catecholamines and indoleamines in Toxoplasma gondii infected mice. Ann Trop Med Parasitol. (1985) 79:153–7. doi: 10.1080/00034983.1985.11811902
57. Ihara F, Nishimura M, Muroi Y, Mahmoud ME, Yokoyama N, Nagamune K, et al. Toxoplasma gondii infection in mice impairs long-term fear memory consolidation through dysfunction of the cortex and amygdala. Infect Immun. (2016) 84:2861–70. doi: 10.1128/IAI.00217-16
58. Mahmoud ME, Fereig R, Nishikawa Y. Involvement of host defense mechanisms against Toxoplasma gondii infection in anhedonic and despair-like behaviors in mice. Infect Immun. (2017) 85:e00007–17. doi: 10.1128/IAI.00007-17
59. Li Y, Viscidi RP, Kannan G, McFarland R, Pletnikov MV, Severance EG, et al. Chronic Toxoplasma gondii infection induces anti-N-methyl-d-aspartate receptor autoantibodies and associated behavioral changes and neuropathology. Infect Immunity. (2018) 86:e00398–18. doi: 10.1128/IAI.00398-18
60. David CN, Frias ES, Szu JI, Vieira PA, Hubbard JA, Lovelace J, et al. GLT-1-dependent disruption of CNS glutamate homeostasis and neuronal function by the protozoan parasite Toxoplasma gondii. PLoS Pathog. (2016) 12:e1005643. doi: 10.1371/journal.ppat.1005643
61. Mahmoud ME, Ihara F, Fereig RM, Nishimura M, Nishikawa Y. Induction of depression-related behaviors by reactivation of chronic Toxoplasma gondii infection in mice. Behav Brain Res. (2016) 298:125–33. doi: 10.1016/j.bbr.2015.11.005
62. Boillat M, Hammoudi P-M, Dogga SK, Pagès S, Goubran M, Rodriguez I, et al. Neuroinflammation-associated aspecific manipulation of mouse predator fear by Toxoplasma gondii. Cell Rep. (2020) 30:320.e6–34.e6. doi: 10.1016/j.celrep.2019.12.019
63. Martynowicz J, Augusto L, Wek RC, Boehm SL, Sullivan WJ. Guanabenz reverses a key behavioral change caused by latent toxoplasmosis in mice by reducing neuroinflammation. MBio. (2019) 10:e00381–19. doi: 10.1128/mBio.00381-19
64. Tong WH, Abdulai-Saiku S, Vyas A. Testosterone reduces fear and causes drastic hypomethylation of arginine vasopressin promoter in medial extended amygdala of male mice. Front Behav Neurosci. (2019) 13:33. doi: 10.3389/fnbeh.2019.00033
65. Singh DK, Hari Dass SA, Abdulai-Saiku S, Vyas A. Testosterone acts within the medial amygdala of rats to reduce innate fear to predator odor akin to the effects of Toxoplasma gondii infection. Front Psychiatry. (2020) 11:630. doi: 10.3389/fpsyt.2020.00630
66. Abdulai-Saiku S, Tong WH, Vyas A. Behavioral manipulation by Toxoplasma gondii: does brain residence matter? Trends Parasitol. (2021) 37:381–90. doi: 10.1016/j.pt.2020.12.006
67. Hajj RE, Tawk L, Itani S, Hamie M, Ezzeddine J, El Sabban M, et al. Toxoplasmosis: current and emerging parasite druggable targets. Microorganisms. (2021) 9:2531. doi: 10.3390/microorganisms9122531
68. Dunay IR, Gajurel K, Dhakal R, Liesenfeld O, Montoya JG. Treatment of toxoplasmosis: historical perspective, animal models, and current clinical practice. Clin Microbiol Rev. (2018) 31:e00057–17. doi: 10.1128/CMR.00057-17
69. Konstantinovic N, Guegan H, Stäjner T, Belaz S, Robert-Gangneux F. Treatment of toxoplasmosis: current options and future perspectives. Food Waterborne Parasitol. (2019) 15:e00036. doi: 10.1016/j.fawpar.2019.e00036
70. Leport C, Raffi F, Matheron S, Katlama C, Regnier B, Saimot AG, et al. Treatment of central nervous system toxoplasmosis with pyrimethamine/sulfadiazine combination in 35 patients with the acquired immunodeficiency syndrome: efficacy of long-term continuous therapy. Am J Med. (1988) 84:94–100. doi: 10.1016/0002-9343(88)90014-9
71. Van Delden C, Hirschel B. Folinic acid supplements to pyrimethamine-sulfadiazine for Toxoplasma encephalitis are associated with better outcome. J Infect Dis. (1996) 173:1294–5. doi: 10.1093/infdis/173.5.1294
72. Ben-Harari RR, Goodwin E, Casoy J. Adverse event profile of pyrimethamine-based therapy in toxoplasmosis: a systematic review. Drugs R D. (2017) 17:523–44. doi: 10.1007/s40268-017-0206-8
73. Hernandez AV, Thota P, Pellegrino D, Pasupuleti V, Benites-Zapata VA, Deshpande A, et al. A systematic review and meta-analysis of the relative efficacy and safety of treatment regimens for HIV-associated cerebral toxoplasmosis: is trimethoprim-sulfamethoxazole a real option? HIV Med. (2017) 18:115–24. doi: 10.1111/hiv.12402
74. Montazeri M, Mehrzadi S, Sharif M, Sarvi S, Tanzifi A, Aghayan SA, et al. Drug resistance in Toxoplasma gondii. Front Microbiol. (2018) 9:2587. doi: 10.3389/fmicb.2018.02587
75. Rolston KV, Hoy J. Role of clindamycin in the treatment of central nervous system toxoplasmosis. Am J Med. (1987) 83:551–4. doi: 10.1016/0002-9343(87)90769-8
76. Kovacs JA. Efficacy of atovaquone in treatment of toxoplasmosis in patients with AIDS. Lancet. (1992) 340:637–8. doi: 10.1016/0140-6736(92)92172-C
77. Goswami RP, Goswami RP, Rahman M, Ray Y, Tripathi SK. Alternative treatment approach to cerebral toxoplasmosis in HIV/AIDS: experience from a resource-poor setting. Int J STD AIDS. (2015) 26:864–9. doi: 10.1177/0956462414560594
78. Montazeri M, Mehrzadi S, Sharif M, Sarvi S, Shahdin S, Daryani A. Activities of anti-Toxoplasma drugs and compounds against tissue cysts in the last three decades (1987 to 2017), a systematic review. Parasitol Res. (2018) 117:3045–57. doi: 10.1007/s00436-018-6027-z
79. Hamie M, Najm R, Deleuze-Masquefa C, Bonnet PA, Dubremetz J-F, El Sabban M, et al. Imiquimod targets toxoplasmosis through modulating host toll-like receptor-MyD88 signaling. Front Immunol. (2021) 12:555. doi: 10.3389/fimmu.2021.629917
80. Sarciron ME, Saccharin C, Petavy AF, Peyron F. Effects of artesunate, dihydroartemisinin, and an artesunate-dihydroartemisinin combination against Toxoplasma gondii. Am J Trop Med Hyg. (2000) 62:73–6. doi: 10.4269/ajtmh.2000.62.73
81. Schultz TL, Hencken CP, Woodard LE, Posner GH, Yolken RH, Jones-Brando L, et al. A thiazole derivative of artemisinin moderately reduces Toxoplasma gondii cyst burden in infected mice. J Parasitol. (2014) 100:516–21. doi: 10.1645/13-451.1
82. Azami SJ, Teimouri A, Keshavarz H, Amani A, Esmaeili F, Hasanpour H, et al. Curcumin nanoemulsion as a novel chemical for the treatment of acute and chronic toxoplasmosis in mice. Int J Nanomed. (2018) 13:7363. doi: 10.2147/IJN.S181896
83. El-Shafey AAM, Hegab MHA, Seliem MME, Barakat AMA, Mostafa NE, Abdel-Maksoud HA, et al. Curcumin@metal organic frameworks nano-composite for treatment of chronic toxoplasmosis. J Mater Sci Mater Med. (2020) 31:90. doi: 10.1007/s10856-020-06429-y
84. Farag TI, Salama MA, Yahia SH, Elfeqy RA. THERAPEUTIC EFFICACY OF THYMUS VULGARIS AND MYRISTICA FRAGRANCE HOUTT. (NUTMEG) ETHANOLIC EXTRACTAGAINST TOXOPLASMOSIS IN MURINE MODEL. J Egypt Soc Parasitol. (2019) 49:73–9. doi: 10.21608/jesp.2019.68288
85. Mady RF, El-Hadidy W, Elachy S. Effect of Nigella sativa oil on experimental toxoplasmosis. Parasitol Res. (2016) 115:379–90. doi: 10.1007/s00436-015-4759-6
86. Rayan HZ, Wagih H, Atwa M. Efficacy of black seed oil from Nigella sativa against murine infection with cysts of Me49 strain of Toxoplasma gondii. PUJ. (2011) 4:165–76.
87. González-Minero FJ, Bravo-Díaz L, Ayala-Gómez A. Rosmarinus officinalis L (Rosemary): an ancient plant with uses in personal healthcare and cosmetics. Cosmetics. (2020) 7:77. doi: 10.3390/cosmetics7040077
88. Hamed EFA, Mostafa NE, Fawzy EM, Ibrahim MN, Attia RA, Salama MA. The delayed death-causing nature of Rosmarinus officinalis leaf extracts and their mixture within experimental chronic toxoplasmosis: therapeutic and prophylactic implications. Acta Trop. (2021) 2021:105992. doi: 10.1016/j.actatropica.2021.105992
89. Murata Y, Sugi T, Weiss LM, Kato K. Identification of compounds that suppress Toxoplasma gondii tachyzoites and bradyzoites. PLoS ONE. (2017) 12:e0178203. doi: 10.1371/journal.pone.0178203
90. Elhabazi K, Dicko A, Desor F, Dalal A, Younos C, Soulimani R. Preliminary study on immunological and behavioural effects of Thymus broussonetii Boiss, an endemic species in Morocco. J Ethnopharmacol. (2006) 103:413–9. doi: 10.1016/j.jep.2005.08.034
91. Dahbi A, Bellete B, Flori P, Hssaine A, Elhachimi Y, Raberin H, et al. The effect of essential oils from Thymus broussonetii Boiss on transmission of Toxoplasma gondii cysts in mice. Parasitol Res. (2010) 107:55–8. doi: 10.1007/s00436-010-1832-z
92. Eraky MA, El-Fakahany AF, El-Sayed NM, Abou-Ouf EA-R, Yaseen DI. Effects of Thymus vulgaris ethanolic extract on chronic toxoplasmosis in a mouse model. Parasitol Res. (2016) 115:2863–71. doi: 10.1007/s00436-016-5041-2
93. Weiss LM, Ma YF, Takvorian PM, Tanowitz HB, Wittner M. Bradyzoite development in Toxoplasma gondii and the hsp70 stress response. Infect Immun. (1998) 66:3295–302. doi: 10.1128/IAI.66.7.3295-3302.1998
94. Tan S, Tong WH, Vyas A. Urolithin-A attenuates neurotoxoplasmosis and alters innate response towards predator odor. Brain, Behavior, & Immunity-Health. (2020) 8:100128. doi: 10.1016/j.bbih.2020.100128
95. Bottari NB, Baldissera MD, Tonin AA, Rech VC, Nishihira VS, Thomé GR, et al. Effects of sulfamethoxazole-trimethoprim associated to resveratrol on its free form and complexed with 2-hydroxypropyl-β-cyclodextrin on cytokines levels of mice infected by Toxoplasma gondii. Microb Pathog. (2015) 87:40–4. doi: 10.1016/j.micpath.2015.07.013
96. Bottari NB, Baldissera MD, Tonin AA, Rech VC, Nishihira VS, Thomé GR, et al. Sulfamethoxazole-trimethoprim associated with resveratrol for the treatment of toxoplasmosis in mice: influence on the activity of enzymes involved in brain neurotransmission. Microb Pathog. (2015) 79:17–23. doi: 10.1016/j.micpath.2015.01.001
97. Bottari NB, Baldissera MD, Tonin AA, Rech VC, Alves CB, D'Avila F, et al. Synergistic effects of resveratrol (free and inclusion complex) and sulfamethoxazole-trimetropim treatment on pathology, oxidant/antioxidant status and behavior of mice infected with Toxoplasma gondii. Microb Pathog. (2016) 95:166–74. doi: 10.1016/j.micpath.2016.04.002
98. Choi J-W, Lee J, Lee J-H, Park B-J, Lee EJ, Shin S, et al. Omega-3 polyunsaturated fatty acids prevent Toxoplasma gondii infection by inducing autophagy via AMPK activation. Nutrients. (2019) 11:2137. doi: 10.3390/nu11092137
99. Zhang J, Qin X, Zhu Y, Zhang S, Zhang X-W, Lu H. Mechanism of dexamethasone in the context of Toxoplasma gondii infection. Parasitology. (2017) 144:1551–9. doi: 10.1017/S0031182017001111
100. McCarthy SM, Davis CD. Prooxidant diet provides protection during murine infection with Toxoplasma gondii. J Parasitol. (2003) 89:886–94. doi: 10.1645/GE-3032
101. Keyhani A, Ziaali N, Shakibaie M, Kareshk AT, Shojaee S, Asadi-Shekaari M, et al. Biogenic selenium nanoparticles target chronic toxoplasmosis with minimal cytotoxicity in a mouse model. J Med Microbiol. (2020) 69:104–10. doi: 10.1099/jmm.0.001111
102. Albalawi AE, Alanazi AD, Alyousif MS, Sepahvand A, Ebrahimi K, Niazi M, et al. The high potency of green synthesized copper nanoparticles to prevent the Toxoplasma gondii infection in mice. Acta Parasitol. (2021) 66:1472–9. doi: 10.1007/s11686-021-00451-y
103. Saadatmand M, Al-Awsi GRL, Alanazi AD, Sepahvand A, Shakibaie M, Shojaee S, et al. Green synthesis of zinc nanoparticles using Lavandula angustifolia Vera. extract by microwave method and its prophylactic effects on Toxoplasma gondii infection. Saudi J Biol Sci. (2021) 28:6454–60. doi: 10.1016/j.sjbs.2021.07.007
104. Craig WJ. Health-promoting properties of common herbs. Am J Clin Nutr. (1999) 70:491s−9s. doi: 10.1093/ajcn/70.3.491s
105. Tapsell LC, Hemphill I, Cobiac L, Sullivan DR, Fenech M, Patch CS, et al. Health benefits of herbs and spices: the past, the present, the future. Med J Aust. (2006) 185:S1–24. doi: 10.5694/j.1326-5377.2006.tb00548.x
106. Arumugam G, Purushotham B, Swamy MK. Myristica fragrans Houtt.: botanical, pharmacological, and toxicological aspects. In: Swamy M, Akhtar M, editors. Natural Bio-active Compounds. Singapore: Springer (2019). p. 81–106. doi: 10.1007/978-981-13-7205-6_4
107. Pillai S, Mahmud R, Lee WC, Perumal S. Anti-parasitic activity of Myristica fragrans Houtt. essential oil against Toxoplasma gondii parasite. APCBEE Proc. (2012) 2:92–6. doi: 10.1016/j.apcbee.2012.06.017
108. Zhang J, Si H, Li B, Zhou X, Zhang J. Myrislignan exhibits activities against Toxoplasma gondii RH strain by triggering mitochondrial dysfunction. Front Microbiol. (2019) 10:2152. doi: 10.3389/fmicb.2019.02152
109. Yimer EM, Tuem KB, Karim A, Ur-Rehman N, Anwar F. Nigella sativa L. (black cumin): a promising natural remedy for wide range of illnesses. Evid Based Complement Alternat Med. (2019) 2019:1528635. doi: 10.1155/2019/1528635
110. Tavakkoli A, Mahdian V, Razavi BM, Hosseinzadeh H. Review on clinical trials of black seed (Nigella sativa) and its active constituent, thymoquinone. J Pharmacopunct. (2017) 20:179. doi: 10.3831/KPI.2017.20.021
111. Salehi B, Abu-Darwish MS, Tarawneh AH, Cabral C, Gadetskaya AV, Salgueiro L, et al. Thymus spp. plants-Food applications and phytopharmacy properties. Trends Food Sci Technol. (2019) 85:287–306. doi: 10.1016/j.tifs.2019.01.020
112. Nieto G. A review on applications and uses of Thymus in the food industry. Plants. (2020) 9:961. doi: 10.3390/plants9080961
113. Cheynier V, Comte G, Davies KM, Lattanzio V, Martens S. Plant phenolics: recent advances on their biosynthesis, genetics, and ecophysiology. Plant Physiol Biochem. (2013) 72:1–20. doi: 10.1016/j.plaphy.2013.05.009
114. Zhang Y-J, Gan R-Y, Li S, Zhou Y, Li A-N, Xu D-P, et al. Antioxidant phytochemicals for the prevention and treatment of chronic diseases. Molecules. (2015) 20:21138–56. doi: 10.3390/molecules201219753
115. Saeedi M, Eslamifar M, Khezri K, Dizaj SM. Applications of nanotechnology in drug delivery to the central nervous system. Biomed Pharmacother. (2019) 111:666–75. doi: 10.1016/j.biopha.2018.12.133
116. Hewlings SJ, Kalman DS. Curcumin: a review of its' effects on human health. Foods. (2017) 6:92. doi: 10.3390/foods6100092
117. Goo Y-K, Yamagishi J, Ueno A, Terkawi MA, Aboge GO, Kwak D, et al. Characterization of Toxoplasma gondii glyoxalase 1 and evaluation of inhibitory effects of curcumin on the enzyme and parasite cultures. Parasit Vectors. (2015) 8:654. doi: 10.1186/s13071-015-1268-5
118. Salehi B, Stojanović-Radić Z, Matejić J, Sharifi-Rad M, Kumar NVA, Martins N, et al. The therapeutic potential of curcumin: a review of clinical trials. Eur J Med Chem. (2019) 163:527–45. doi: 10.1016/j.ejmech.2018.12.016
119. Liu W, Zhai Y, Heng X, Che FY, Chen W, Sun D, et al. Oral bioavailability of curcumin: problems and advancements. J Drug Target. (2016) 24:694–702. doi: 10.3109/1061186X.2016.1157883
120. Rai M, Ingle AP, Pandit R, Paralikar P, Anasane N, Santos CAD. Curcumin and curcumin-loaded nanoparticles: antipathogenic and antiparasitic activities. Expert Rev Anti Infect Ther. (2020) 18:367–79. doi: 10.1080/14787210.2020.1730815
121. Koushki M, Amiri-Dashatan N, Ahmadi N, Abbaszadeh HA, Rezaei-Tavirani M. Resveratrol: a miraculous natural compound for diseases treatment. Food Sci Nutr. (2018) 6:2473–90. doi: 10.1002/fsn3.855
122. Berman AY, Motechin RA, Wiesenfeld MY, Holz MK. The therapeutic potential of resveratrol: a review of clinical trials. NPJ Precis Oncol. (2017) 1:1–9. doi: 10.1038/s41698-017-0038-6
123. Singh AP, Singh R, Verma SS, Rai V, Kaschula CH, Maiti P, et al. Health benefits of resveratrol: evidence from clinical studies. Med Res Rev. (2019) 39:1851–91. doi: 10.1002/med.21565
124. Bottari NB, Pillat MM, Schetinger MRC, Reichert KP, Machado V, Assmann CE, et al. Resveratrol-mediated reversal of changes in purinergic signaling and immune response induced by Toxoplasma gondii infection of neural progenitor cells. Purinergic Signal. (2019) 15:77–84. doi: 10.1007/s11302-018-9634-3
125. Bottari NB, Reichert KP, Fracasso M, Dutra A, Assmann CE, Ulrich H, et al. Neuroprotective role of resveratrol mediated by purinergic signalling in cerebral cortex of mice infected by Toxoplasma gondii. Parasitol Res. (2020) 119:2897–905. doi: 10.1007/s00436-020-06795-0
126. Moreira-Souza ACA, Rangel TP, Silva S, Figliuolo VR, Savio LEB, Schmitz F, et al. Disruption of purinergic receptor P2X7 signaling increases susceptibility to cerebral toxoplasmosis. Am J Pathol. (2019) 189:730–8. doi: 10.1016/j.ajpath.2019.01.001
127. Espin JC, Larrosa M, Garcia-Conesa MT, Tomas-Barberan F. Biological significance of urolithins, the gut microbial ellagic acid-derived metabolites: the evidence so far. Evid Based Complement Alternat Med. (2013) 2013:270418. doi: 10.1155/2013/270418
128. Gasperotti M, Passamonti S, Tramer F, Masuero D, Guella G, Mattivi F, et al. Fate of microbial metabolites of dietary polyphenols in rats: is the brain their target destination? ACS Chem Neurosci. (2015) 6:1341–52. doi: 10.1021/acschemneuro.5b00051
129. Ryu D, Mouchiroud L, Andreux PA, Katsyuba E, Moullan N, Nicolet-Dit-Felix AA, et al. Urolithin A induces mitophagy and prolongs lifespan in C. elegans and increases muscle function in rodents. Nat Med. (2016) 22:879–88. doi: 10.1038/nm.4132
130. Yuan T, Ma H, Liu W, Niesen DB, Shah N, Crews R, et al. Pomegranate's neuroprotective effects against Alzheimer's disease are mediated by urolithins, its ellagitannin-gut microbial derived metabolites. ACS Chem Neurosci. (2016) 7:26–33. doi: 10.1021/acschemneuro.5b00260
131. Ahsan A, Zheng YR, Wu XL, Tang WD, Liu MR, Ma SJ, et al. Urolithin A-activated autophagy but not mitophagy protects against ischemic neuronal injury by inhibiting ER stress in vitro and in vivo. CNS Neurosci Ther. (2019) 25:976–86. doi: 10.1111/cns.13136
132. Gong Z, Huang J, Xu B, Ou Z, Zhang L, Lin X, et al. Urolithin A attenuates memory impairment and neuroinflammation in APP/PS1 mice. J Neuroinflamm. (2019) 16:62. doi: 10.1186/s12974-019-1450-3
133. Andreux PA, Blanco-Bose W, Ryu D, Burdet F, Ibberson M, Aebischer P, et al. The mitophagy activator urolithin A is safe and induces a molecular signature of improved mitochondrial and cellular health in humans. Nat Metab. (2019) 1:595–603. doi: 10.1038/s42255-019-0073-4
134. Chen P, Chen F, Lei J, Li Q, Zhou B. Activation of the miR-34a-mediated SIRT1/mTOR signaling pathway by urolithin A attenuates d-galactose-induced brain aging in mice. Neurotherapeutics. (2019) 16: 1269–82. doi: 10.2139/ssrn.3337879
135. Kujawska M, Jourdes M, Kurpik M, Szulc M, Szaefer H, Chmielarz P, et al. Neuroprotective effects of pomegranate juice against parkinson's disease and presence of ellagitannins-derived metabolite—urolithin A—in the brain. Int J Mol Sci. (2020) 21:202. doi: 10.3390/ijms21010202
136. Guo R, Li L, Su J, Li S, Duncan SE, Liu Z, et al. Pharmacological activity and mechanism of tanshinone IIA in related diseases. Drug Des Devel Ther. (2020) 14:4735–48. doi: 10.2147/DDDT.S266911
137. Rajapakse R, Uring-Lambert B, Andarawewa KL, Rajapakse R, Abou-Bacar A, Marcellin L, et al. 1,25 (OH) 2D3 inhibits in vitro and in vivo intracellular growth of apicomplexan parasite Toxoplasma gondii. J Steroid Biochem Mol Biol. (2007) 103:811–4. doi: 10.1016/j.jsbmb.2006.12.058
138. Ghaffarifar F, Pour MA, Sharifi Z, Asl AD, Al-Kawaz E. The effect of vitamin D3 alone and mixed with IFN-γ on tachyzoites of Toxoplasma gondii (RH strain) proliferation and nitric oxide (NO) production in infected macrophages of BALB/C mice. Iran J Parasitol. (2010) 5:48.
139. Jalalizadegan B, Ghaffarifar F, Fallah S, Elmi T, Namazi MJ, Vafa Shoar F, et al. The effect of vitamins C and E on nitric oxide elevation to enhance killing of phagocytised Tachizoites of Toxoplasma gondii in BALB/c mice. J Sabz Univ Med Sci. (2019) 26:515–25.
140. Watson L, Cohen R, Padigala K. Impact of Pro-oxidant Diet on Cytokine Production in Mice Infected with Toxoplasma gondii. Western Kentucky University (2018).
141. Shakibaie M, Ezzatkhah F, Gabal E, Badparva E, Jahanbakhsh S, Mahmoudvand H. Prophylactic effects of biogenic selenium nanoparticles on acute toxoplasmosis: an in vivo study. Ann Med Surg. (2020) 54:85–8. doi: 10.1016/j.amsu.2020.04.010
142. Huang S, Holmes MJ, Radke JB, Hong D-P, Liu T-K, White MW, et al. Toxoplasma gondii AP2IX-4 regulates gene expression during bradyzoite development. MSphere. (2017) 2:e00054–17. doi: 10.1128/mSphere.00054-17
143. Hong D-P, Radke JB, White MW. Opposing transcriptional mechanisms regulate Toxoplasma development. Msphere. (2017) 2:e00347–16. doi: 10.1128/mSphere.00347-16
144. Radke JB, Worth D, Hong D, Huang S, Sullivan WJ Jr, Wilson EH, et al. Transcriptional repression by ApiAP2 factors is central to chronic toxoplasmosis. PLoS Pathog. (2018) 14:e1007035. doi: 10.1371/journal.ppat.1007035
145. Waldman BS, Schwarz D, Wadsworth II MH, Saeij JP, Shalek AK, Lourido S. Identification of a master regulator of differentiation in Toxoplasma. Cell. (2020) 180:359.e16–72.e16. doi: 10.1016/j.cell.2019.12.013
146. Song X, Lin M, Li M, Yang X, Liu J, Liu Q. Toxoplasma gondii metacaspase 2 is an important factor that influences bradyzoite formation in the Pru strain. Parasitol Res. (2020) 119:2287–98. doi: 10.1007/s00436-020-06722-3
147. Feder ME, Hofmann GE. Heat-shock proteins, molecular chaperones, and the stress response: evolutionary and ecological physiology. Annu Rev Physiol. (1999) 61:243–82. doi: 10.1146/annurev.physiol.61.1.243
148. Soete M, Camus D, Dubrametz J. Experimental induction of bradyzoite-specific antigen expression and cyst formation by the RH strain of Toxoplasma gondii in vitro.Exp Parasitol. (1994) 78:361–70. doi: 10.1006/expr.1994.1039
149. Sun H, Zhuo X, Zhao X, Yang Y, Chen X, Yao C, et al. The heat shock protein 90 of Toxoplasma gondii is essential for invasion of host cells and tachyzoite growth. Parasite. (2017) 24:22. doi: 10.1051/parasite/2017023
150. Barenco PVC, Lourenco EV, Cunha-Júnior JP, Almeida KC, Roque-Barreira MC, Silva DAO, et al. Toxoplasma gondii 70 kDa heat shock protein: systemic detection is associated with the death of the parasites by the immune response and its increased expression in the brain is associated with parasite replication. PLoS ONE. (2014) 9:e96527. doi: 10.1371/journal.pone.0096527
151. Huebbe P, Wagner AE, Boesch-Saadatmandi C, Sellmer F, Wolffram S, Rimbach G. Effect of dietary quercetin on brain quercetin levels and the expression of antioxidant and Alzheimer's disease relevant genes in mice. Pharmacol Res. (2010) 61:242–6. doi: 10.1016/j.phrs.2009.08.006
152. Ishisaka A, Ichikawa S, Sakakibara H, Piskula MK, Nakamura T, Kato Y, et al. Accumulation of orally administered quercetin in brain tissue and its antioxidative effects in rats. Free Radic Biol Med. (2011) 51:1329–36. doi: 10.1016/j.freeradbiomed.2011.06.017
153. Guevara RB, Fox BA, Bzik DJ. Toxoplasma gondii parasitophorous vacuole membrane-associated dense granule proteins regulate maturation of the cyst wall. Msphere. (2020) 5:e00851–19. doi: 10.1128/mSphere.00851-19
154. Dubey J, Lindsay D, Speer C. Structures of Toxoplasma gondii tachyzoites, bradyzoites, and sporozoites and biology and development of tissue cysts. Clin Microbiol Rev. (1998) 11:267–99. doi: 10.1128/CMR.11.2.267
155. Martino E, Tarantino M, Bergamini M, Castelluccio V, Coricello A, Falcicchio M, et al. Artemisinin and its derivatives. ancient tradition inspiring the latest therapeutic approaches against malaria. Fut Med Chem. (2019) 11:1443–59. doi: 10.4155/fmc-2018-0337
156. Secrieru A, Costa IC, O'Neill PM, Cristiano ML. Antimalarial agents as therapeutic tools against toxoplasmosis—a short bridge between two distant illnesses. Molecules. (2020) 25:1574. doi: 10.3390/molecules25071574
157. Jones-Brando L, D'Angelo J, Posner GH, Yolken R. In vitro inhibition of Toxoplasma gondii by four new derivatives of artemisinin. Antimicrob Agents Chemother. (2006) 50:4206–8. doi: 10.1128/AAC.00793-06
158. D'Angelo JG, Bordón C, Posner GH, Yolken R, Jones-Brando L. Artemisinin derivatives inhibit Toxoplasma gondii in vitro at multiple steps in the lytic cycle. J Antimicrob Chemother. (2009) 63:146–50. doi: 10.1093/jac/dkn451
159. Hencken CP, Jones-Brando L, Bordón C, Stohler R, Mott BT, Yolken R, et al. Thiazole, oxadiazole, and carboxamide derivatives of artemisinin are highly selective and potent inhibitors of Toxoplasma gondii. J Med Chem. (2010) 53:3594–601. doi: 10.1021/jm901857d
160. Dunay IR, Chan WC, Haynes RK, Sibley LD. Artemisone and artemiside control acute and reactivated toxoplasmosis in a murine model. Antimicrob Agents Chemother. (2009) 53:4450–6. doi: 10.1128/AAC.00502-09
161. Nagamune K, Beatty WL, Sibley LD. Artemisinin induces calcium-dependent protein secretion in the protozoan parasite Toxoplasma gondii. Eukaryot Cell. (2007) 6:2147–56. doi: 10.1128/EC.00262-07
162. Nagamune K, Moreno SN, Sibley LD. Artemisinin-resistant mutants of Toxoplasma gondii have altered calcium homeostasis. Antimicrob Agents Chemother. (2007) 51:3816–23. doi: 10.1128/AAC.00582-07
163. Rosenberg A, Luth MR, Winzeler EA, Behnke M, Sibley LD. Evolution of resistance in vitro reveals mechanisms of artemisinin activity in Toxoplasma gondii. Proc Natl Acad Sci USA. (2019) 116:26881–91. doi: 10.1073/pnas.1914732116
164. Nolan SJ, Romano JD, Kline JT, Coppens I. Novel approaches to kill Toxoplasma gondii by exploiting the uncontrolled uptake of unsaturated fatty acids and vulnerability to lipid storage inhibition of the parasite. Antimicrob Agents Chemother. (2018) 62:e00347–18. doi: 10.1128/AAC.00347-18
165. Paredes-Santos T, Martins-Duarte E, de Souza W, Attias M, Vommaro R. Toxoplasma gondii reorganizes the host cell architecture during spontaneous cyst formation in vitro. Parasitology. (2018) 145:1027–38. doi: 10.1017/S0031182017002050
166. Kannan G, Thaprawat P, Schultz TL, Carruthers VB. Acquisition of host cytosolic protein by Toxoplasma gondii Bradyzoites. Msphere. (2021) 6:e00934–20. doi: 10.1128/mSphere.00934-20
167. Olson WJ, Martorelli Di Genova B, Gallego-Lopez G, Dawson AR, Stevenson D, Amador-Noguez D, et al. Dual metabolomic profiling uncovers Toxoplasma manipulation of the host metabolome and the discovery of a novel parasite metabolic capability. PLoS Pathog. (2020) 16:e1008432. doi: 10.1371/journal.ppat.1008432
168. Ma J, He J-J, Hou J-L, Zhou C-X, Zhang F-K, Elsheikha HM, et al. Metabolomic signature of mouse cerebral cortex following Toxoplasma gondii infection. Parasit Vect. (2019) 12:1–11. doi: 10.1186/s13071-019-3623-4
169. Glick NR, Fischer MH. The role of essential fatty acids in human health. J Evid Based Complementary Altern Med. (2013) 18:268–89. doi: 10.1177/2156587213488788
170. Morgan P, Van Der Graaf PH, Arrowsmith J, Feltner DE, Drummond KS, Wegner CD, et al. Can the flow of medicines be improved? Fundamental pharmacokinetic and pharmacological principles toward improving Phase II survival. Drug Discov Today. (2012) 17:419–24. doi: 10.1016/j.drudis.2011.12.020
171. Teng H, Chen L. Polyphenols and bioavailability: an update. Crit Rev Food Sci Nutr. (2019) 59:2040–51. doi: 10.1080/10408398.2018.1437023
172. Bastien P. Molecular diagnosis of toxoplasmosis. Trans Roy Soc Trop Med Hyg. (2002) 96(Suppl 1):S205–15. doi: 10.1016/S0035-9203(02)90078-7
173. Dickerson F, Stallings C, Vaughan C, Origoni A, Goga J, Khushalani S, et al. Artemisinin reduces the level of antibodies to gliadin in schizophrenia. Schizophr Res. (2011) 129:196–200. doi: 10.1016/j.schres.2011.04.010
174. Wang HL, Xiang YT Li QY, Wang XP, Liu ZC, Hao SS, et al. The effect of artemether on psychotic symptoms and cognitive impairment in first-episode, antipsychotic drug-naive persons with schizophrenia seropositive to Toxoplasma gondii. J Psychiatr Res. (2014) 53:119–24. doi: 10.1016/j.jpsychires.2014.02.016
Keywords: brain, CNS, cyst, chronic toxoplasmosis, nutrition, intervention, parasite, Toxoplasma gondii
Citation: Tan S, Tong WH and Vyas A (2022) Impact of Plant-Based Foods and Nutraceuticals on Toxoplasma gondii Cysts: Nutritional Therapy as a Viable Approach for Managing Chronic Brain Toxoplasmosis. Front. Nutr. 9:827286. doi: 10.3389/fnut.2022.827286
Received: 01 December 2021; Accepted: 31 January 2022;
Published: 25 February 2022.
Edited by:
Yosra A. Helmy, The Ohio State University, United StatesReviewed by:
Hiba Ahmad El Hajj, American University of Beirut, LebanonCopyright © 2022 Tan, Tong and Vyas. This is an open-access article distributed under the terms of the Creative Commons Attribution License (CC BY). The use, distribution or reproduction in other forums is permitted, provided the original author(s) and the copyright owner(s) are credited and that the original publication in this journal is cited, in accordance with accepted academic practice. No use, distribution or reproduction is permitted which does not comply with these terms.
*Correspondence: Sijie Tan, c2p0YW5AbnR1LmVkdS5zZw==
Disclaimer: All claims expressed in this article are solely those of the authors and do not necessarily represent those of their affiliated organizations, or those of the publisher, the editors and the reviewers. Any product that may be evaluated in this article or claim that may be made by its manufacturer is not guaranteed or endorsed by the publisher.
Research integrity at Frontiers
Learn more about the work of our research integrity team to safeguard the quality of each article we publish.