- 1Program in Food Production and Innovation, College of Integrated Science and Technology, Rajamangala University of Technology Lanna, Chiang Mai, Thailand
- 2Plant Bioactive Compound Laboratory, Faculty of Agriculture, Chiang Mai University, Chiang Mai, Thailand
- 3Faculty of Agro-Industry, School of Agro-Industry, Chiang Mai University, Chiang Mai, Thailand
- 4Cluster of Agro Bio-Circular-Green Industry (Agro BCG), Chiang Mai University, Chiang Mai, Thailand
- 5Department of Pharmaceutical Sciences, Faculty of Pharmacy, Chiang Mai University, Chiang Mai, Thailand
- 6Cluster of Research and Development of Pharmaceutical and Natural Products Innovation for Human or Animal, Chiang Mai University, Chiang Mai, Thailand
- 7Department of Plant and Soil Sciences, Faculty of Agriculture, Chiang Mai University, Chiang Mai, Thailand
Prebiotics are functional food ingredients that assist probiotic growth and render many other health benefits. Mango peel is the biomass of the processing industry and has recently been value-added as a dietary fiber pectin. Besides its general use as a food additive, mango peel pectin (MPP) is partially hydrolyzed by pectinase to obtain pectic oligosaccharides (POSs) that have recently gained attention as novel prebiotic products and in medical research. This review describes probiotic candidates responsible for the digestion of pectin derivatives and the advantages of POSs as functional additives and their current best retrieval options. Mango pectic oligosaccharide (MPOS) recovery from low methoxyl MPP from mango with prebiotic performance both in vivo and in vitro environments is discussed. Current research gaps and potential developments in the field are also explored. The overall worthiness of this article is the potential use of the cheap-green food processing bioresource for high-value components.
Introduction
Functional food is a type of food that is supplemented with bioactive ingredients (e.g., dietary fiber, probiotics, and antioxidants) and derived food ingredients. It can be consumed as part of a normal daily diet that provides health benefits and reduced the risk of chronic diseases beyond those provided by adequate nutrition. The newborns' gastrointestinal (GI) tracts were inoculated with organisms at an early stage of life due to the influence of maternal intestinal flora and diets (1). These include aerobic Gram-positive cocci, enterobacteria, and Lactobacilli, which are the primary colonizers. These bacteria rapidly consume O2, which enhances the growth of obligate anaerobic species, collectively known as gut microflora (2, 3). In particular, the microflora of breast-fed infants is dominated by a bifidobacterial population that purports to be in a better health condition than formula-fed babies (4). Human milk oligosaccharides (HMOs) are enriched with complex glycan compounds that are partially mediated by the modulation of the intestinal microbial ecology and immunological homeostasis associated with the prevention of intestinal diseases, improved general wellbeing, and reduced incidence of allergic symptoms (5, 6). Consequently, galacto-oligosaccharides (GOSs) are often the predominant prebiotic oligosaccharides used in infant diets. It is believed that including GOSs in infant formula boosts the population of bifidobacterial, reduces pathogenic inoculums, and boosts metabolic activity in immune system regulation (7). GOSs are typically synthesized from lactose through the enzymatic activity of β-galactosidase (as well as β-glucosidases and β-glycosidases) via transgalactosylation (8). Besides GOSs, other prebiotic oligosaccharides such as fructo-oligosaccharide (FOS) and/or polydextrose (PDX) are also prevalent components in breast milk, but they are fundamentally absent in cow's milk (9–11). In addition to the ability to enhance the growth of Bifidobacteria and Lactobacilli, short-chain fatty acids (SCFAs) are produced as the by-products of oligosaccharide fermentation. In adult humans, the SCFA has a stronger link in the prevention of colon cancer (12). Recently, POS has been proposed as a new class of prebiotics capable of in vivo synergistic empowerment of immunomodulation caused by GOS and FOS (13, 14). Mango peel is a potential biomass for dietary fiber recovery with 5–11% pectin depending on the extraction methods, varieties, and, also, fruit morphological characteristics (15, 16). As a food additive, mango peel pectin (MPP) has been utilized as a food additive to alter the texture and firmness of food products, and a carrier material for drugs and medicine (17, 18). The extracted MPP could be partially hydrolyzed by a pectinase enzyme to obtain MPOS as a prebiotic in human food (19).
Probiotics
Probiotics are defined by the World Health Organization as live microorganisms that enhance health benefits on the host when consumed in adequate amounts (20). They are non-pathogenic, beneficial, and active bacteria and yeast. The highly potent and commonly used probiotics are Lactobacillus spp., Bifidobacterium spp., Saccharomyces boulardii, Propionibacterium spp., Streptococcus spp., Bacillus spp., Enterococcus spp., and some specific strains of Escherichia coli (Table 1). Especially, the genus of Lactobacillus and Bifidobacterium of the Gram-positive, non-spore-forming, non-mobile, obligated are those of the facultative anaerobic bacteria. They are classified as lactic acid bacteria (LAB), which are catalase-negative bacterial species that can produce lactic acid as the main end-product of carbohydrate fermentation (23). LAB are generally used as food additives (Table 2) for health-promoting purposes.
The health mechanisms of these probiotic genera are described in Figure 1, which includes (1) increased adhesion to the intestinal mucosa, (2) enhancement of the epithelial barrier, (3) inhibition of pathogen adhesion, (4) production of antimicroorganism substance, (5) competitive exclusion of pathogenic microorganisms (i.e., acid and SCFA), and (6) modulation of the immune system (25, 26). Lactobacillus reuteri is a well-studied probiotic bacterium that colonizes a large number of mammals. It has been clarified as a heterofermentative species that can grow in oxygen-limited atmospheres and colonize the GI tract of humans and animals (27). It can also survive in a variety of pH conditions, employ multiple mechanisms for pathogenic microorganism inhibition, and secrete many antimicrobial intermediates (28–30). B. animalis is considered a natural inhibitor of human and other mammalian GI tracts, as well as widely supplemented in numerous fermented dairy products (31). The major subspecies of B. animalis include animalis and lactis, of which the latter subspecies is regarded as technologically suitable to use as a probiotic adjunct due to its resistance against acid, bile, and oxygen than other members of the genus (32, 33). Clinical properties for health advancement and/or disease defense of the probiotics are to provide symptom relief to individuals with common GI symptoms, irritable bowel syndrome, and constipation and to boost the immune response (34). The survival rate of probiotics in gastric conditions depends on the types of prebiotics and their resources. Larsen et al. (35) claimed that Lactobacillus fermentum PCC and L. reuteri RC-14 were more resistant to gastric tract in the presence of different pectin types. The variable amount of polygalacturonic acids in the backbone was also crucial for bacterial protection (35). The protective function of pectin is linked primarily to the complex fluctuation in surface charges and, as a result, influences the pectin-bacteria electrostatic interactions. Additionally, Corcoran et al. (36) and Hernandez-Hernandez et al. (37) suggested that the survival improvement of Lactobacilli in the gastric juice by prebiotic oligosaccharides was in association with the presence of metabolizable sugars, which could maintain pH homeostasis by increasing ATP generation.
Types of Prebiotics as Functional Food Ingredients
Prebiotics are short-chain carbohydrates (SCCs) that are non-digestible by digestive enzymes in humans and sometimes known as resistant SCCs because they are only fermented in the intestinal tract (38). It encourages the growth and/or activity of one or a limited number of intestinal bacteria that reside in the gut rather than introducing the exogenous species (39). Non-digested carbohydrate (CHO) molecules, saccharides (di-, oligo-, and poly-), resistant starches, and sugar polyols have been claimed to have the potentiality of prebiotic (40). The carbohydrate sources are identified as prebiotic properties that achieve the following criteria; (a) resistance to gastric acidity and mammalian enzymes, (b) susceptibility to fermentation by gut bacteria, and (c) ability to enhance the viability and/or activity of beneficial microorganisms (41). For these, GOS, FOS, and inulin are commercially accepted for food-grade prebiotics. GOSs and FOSs are non-digestible carbohydrates derived from lactose that can be found naturally in human milk. Inulin and fructan are known as prebiotics derived from soluble dietary fibers, which are vastly obtained from plants such as asparagus, chicory, tomatoes, mango, onion, and garlic (40, 42). Prebiotic compounds are classified based on chemical structures, chain length or degree of polymerization, and applications (43). Most of the functional food prebiotics are saccharide derivatives, which are mostly of plant origin. Besides, protein or peptide and lipid prebiotics can be naturally found (44). The different types of prebiotics and their plant sources are summarized in Table 3. Inulin is a polysaccharide fructan that produces SCFAs that are extracted from fruits and vegetables. The fatty acids such as propionate, butyrate, and acetate endure the reduction effect of lipids and cholesterol and possibly attain a reduction in hypertension risk (58). Similarly, pectic oligosaccharides (POSs), which are the products of partial hydrolysis of pectin, are currently classified as emerging prebiotics, but only limited studies are there to support their use at the commercial level (59).
Pectic Oligosaccharides
Pectic oligosaccharide is a non-digestible oligosaccharide that possesses prebiotic activity. POS beneficially affects the host by selectively enhancing the growth and/or activity of one or a limited number of Bifidobacteria and Lactobacilli in the colon (56, 60). The colonic fermentation of POS generates SCFA, which provides a great variety of health effects, including inhibition of pathogenic bacteria, constipation relief, reduction in blood glucose levels, improvement in mineral absorption, reduction of colonic cancer, and modulation of the immune system (13). POS also has a potential inhibitory on the growth of entero-putrefactive and pathogenic organisms (61, 62). Pectic polysaccharide (i.e., pectin) extracted from various sources is cut into smaller chains of POS using different preparations, viz. enzymatic, chemical, and physical techniques (63, 64). The techniques for POS preparation from several raw materials and biomasses are comprehensively collected as shown in Table 4. Enzymatic treatment has been extensively applied for POS production due to the specificity and selectivity as well as minimum adverse chemical modifications of products (74, 75). The hydrolysis enzyme of pectin has been used, which acts synergistically to produce POS (64, 76, 77). The methyl esters of galacturonic acid residues are cleaved by pectin methyl esterase (PME) (78). This enzyme acts before polygalacturonase (PG). PG degrades the glycosidic bond of the α-(1,4)-polygalacturonan in a random position (79). Nevertheless, the less esterified the structure of the pectin substrate, the greater the activity of PG (80). Meanwhile, pectin lyase (PL) highly catalyzes the esterified pectin, producing unsaturated methyloligogalacturonates through transelimination of glycosidic linkages (81). Physical pretreatments, including hydrothermal, dynamic high-pressure microfluidization (DHPM), and irradiation, have been adapted to partially degrade the raw materials for oligosaccharide release (71, 82, 83). Using the hydrothermal method, arabino and galacto-oligosaccharides (GOSs) were effectively obtained from various bioresources. While the chemical hydrolysis of pectin for the production of POS has been limitedly explored. This is because there are some disadvantages to the chemical process, including toxicity and limitation of the desired degree of polymerization (75).
Furthermore, probiotics in the gut system respond differently to alternate types of prebiotics. Olano-Martin et al. (84) reported that different types of pectins and POSs had significant selective effects on the growth of gut bacteria as shown Figure 2. The data were regenerated using the principal component analysis (PCA). From the figure, the first two dimensions of the PCA accounted for 91.66% across the PCA score plot (PC1; 64.57% and PC2; 27.09% of the variance). Overall, it was found that the Lactobacillus spp. gave mostly positive responses to the prebiotic pectins, while Bifidobacteria appeared opposingly. It was also apparent that the low methoxyl pectin (LMP) and its pectic oligosaccharide hydrolysate (POS II) were specifically responsive to Lactobacillus plantarum 0207. Meanwhile, high methoxyl type (HMP) and its hydrolysate (POS I) had a slight influence on the growth of the two strains of Lactobacillus casei and Lactobacillus acidophilus.
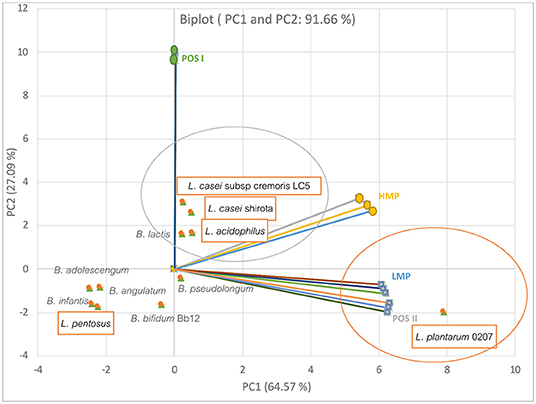
Figure 2. The relationship between prebiotic types and the specific growth rate of selected gut bacteria. The plot was regenerated from the data presented in (84).
Mango Pectic Oligosaccharides
Purification
Mango peel accounts for 20% of fruit biomass and is known as a potential source of dietary fiber consisting of a high pectin (5–10%) composition depending on fruit characteristics, the extractions, and varieties (16, 37–41). Previous studies have illustrated that peel from the Thai mango variety, Chok Anan, provided a substantially high amount of pectin (13%), mainly of low methoxyl content, illustrating gelation properties at low sugar content; thus, it has been widely used as a food additive (18). The MPP was used as a potential source for POS II with pectinases and the longer the hydrolysis time and pectinase concentrations, the lower the molecular weight (Mw) obtained (85). The MPOS was evaluated for prebiotic activity with L. reuteri and B. animalis, whose highest proliferation was 4% (w/v) of the MPOS supplemented condition at 72 h of the fermentation time (19). Pectinase and long hydrolysis cleaved pectin to be active molecules with high prebiotic efficiency (13). It is encouraged that purification processes are highly recommended to obtain food-grade final products. Generally, the purification of POS can be operated either by membrane-based technology, including those of ultrafiltration with the regenerated cellulose membrane of different pore sizes (83, 86), or chromatographic separation (87, 88) with a specific resin/matrix (74). The purification steps are chosen based on the selection of the components from the mixture. In the case of POS produced from orange peel wastes (OPWs), the POS was purified by a two-step membrane process (i.e., discontinuous diafiltration and concentration) to yield a refined product comprising up to 90% oligosaccharides (89). Likewise, Holck et al. (90) implemented a regenerated cellulose membrane for purification of sugar beet POS, while Iwasaki and Matsubara (91) purified the oligomers acquired from citrus pulp pectin from the membrane using two steps to maintain the high MW constituents and eliminate a small molecule of monosaccharides and saccharose.
Structural Characterization
The POS structure is complicated because of the complex chemical composition of pectin and the chemical alteration during POS production. The complex mixtures of POS can be analyzed using high sensibility and ability methods such as matrix-assisted laser desorption ionization-mass spectrometry (MALDI-MS) (92), electrospray ionization (ESI)-MS (93), capillary electrophoresis-MS (94), capillary electrophoresis with UV detection (95), and NMR and ESI-MS identification (96–98) with fluorescent labeling (99). Arabino-oligosaccharides degraded from sugar beet pulp pectin were able to be classified by MALDI-time-of-flight-MS and high-performance anion exchange chromatography with pulsed amperometric detection (HPAEC-PAD) (100). The Mw of POS can be evaluated using the size exclusion chromatography (SEC) (19, 101), HPAEC-PAD (64), as well as hydrophilic interaction liquid chromatography (HILIC) with online ESI ion trap-MS-evaporative light scattering detection (ELSD) (102). The presence of Mw of MPOS affected the probiotic growth because the utilization of prebiotics by lactic acid requires the presence of specific enzyme hydrolysis and transport systems for the particular prebiotic (103). The β-galactosidase activity in the tested strains was correspondent with low-molecular-weight substrate (104). For the quantity analysis of sugars in POS liquefaction, high-performance liquid chromatography (HPLC) (19), HPAEC-PAD (53), and HPAEC-fluorescence detection (105) are widely used to specify the monosaccharide contents in the recovered POS. The major sugar compositions of the oligosaccharide from mango peel were fructose (24.41%) and glucose (19.52%) (19).
In vivo and in vitro Performance
Pectic oligosaccharides have been proposed as a new class of prebiotics capable of exerting a number of health-promoting effects, including bifidogenic flora promotion, antioxidant activity (106), lowering the serum levels of total cholesterol and triglyceride (107), antiadhesive properties for food pathogen toxins (E. coli O157:H7), and apoptosis stimulation of colon cancer cells (108). For the simulation of prebiotic fermentation, B. animalis TISTR 2195 showed higher proliferation in 4% (w/v) of MPOS supplemented (8.92 log CFU/ml) than that of L. reuteri (8.53 CFU/ml) at 72 h of the fermentation time. This may be as a result of the intracellular enzymes of Bifidobacterium, which could hydrolyze the oligosaccharides into monosaccharides (i.e., glucose and fructose phosphates) and utilize them as a nutrient source (67). The main SCFAs derived from MPOS were acetic acid and propionic acid. Both acids are known as the main SCFAs derived from POS fermentation (109). The highest value of total SCFA was achieved from the 4% (w/v) MPOS supplementation for both B. animalis (68.57 mM) and L. reuteri (69.15 mM).
Besides, POS defends colonic cells against Shiga toxins (Stx) secreted from E. coli O157:H7 by neutralization of Stx activity from POS interaction with the galabiose receptor (110). The higher the molecular mass of POS, the greater the inhibitory activity obtained. This may be due to the increased access to the receptor-binding sites on the toxin. POS also prevents the adhesion of P-fimbriated E. coli to uroepithelial cells in vitro. Oligogalacturonic acid, disaccharide, and trisaccharide were the most active POS (111). In the case of cancer preventative ability, POS can stimulate the apoptosis process in human colonic adenocarcinoma cells (112). The incident is due predominantly to the growth enhancement of Bifidobacteria and further promotes their immunomodulatory capacity (113). In vivo synergistic empowerment of immunomodulation caused by GOSs and FOSs mixed with pectin-derived acidic oligosaccharides showed systemic Th1-dependent immune responses in a murine vaccination model. It can therefore be assumed that the application of these oligosaccharides in infant formulas is beneficial for the development of the infant's immune system (114). The potential for in vivo cardiovascular protection of POS is also reported by Li et al. (107), and it was found that haw POS (HPOS) significantly reduced the serum levels of total cholesterol and triglycerides and inhibited the accumulation of body fat. Therefore, HPOS can be applied as a drug therapy to combat cardiovascular diseases. Additionally, in vivo and in vitro studies confirmed that acidic POS was not cytotoxic or mutagenic in the Ames test, making it suitable for use in food products for children and babies (60).
Clinical Practice Guidelines
Prebiotics are substances that exist naturally in food or are fortified during manufacturing to improve the functional efficacy of probiotics. Food containing both prebiotics and probiotics is usually recognized as “symbiotic” (43). The fructan inulin is the common prebiotic that has been categorized as “Generally Accepted as Safe” by the American Food and Drug Administration (58). POSs of plant origins have been proposed as excellent candidates for new-generation prebiotics (115–117). It is believed that microorganisms are able to utilize the carbon sources from POS better than they are with the polysaccharides, and in fact, pectinolytic enzymes are only characterized by Bacteroides sp. and Clostridium butyricum-Clostridium beijerinckii group in the human gut (84). The prebiotic effect of POS depends upon the Mw of the fractions. Low-molecular-weight POS has better prebiotic potential than high-molecular-weight POS (118), even though the clinical trials and toxicity studies are limited. POSs have also been shown to possess in vitro anti-inflammatory and antioxidant activity (66, 119). It also illustrates a vasoprotective effect that increased the serum SOD activity and lowered the content of MDA in these mice that are fed a high-fat diet, which can be used as a supplement for protection against cardiovascular diseases (107). Moreover, POS improves the gut mucosal structure, which is a barrier for rotavirus infection that induces diarrhea caused by damaging the intestinal organs in children and young animals (120). It is no doubt that MPOS is a novel prebiotic dietary fiber with antipathogenic potential against Staphylococcus aureus, E. coli, Bacillus subtilis, and Salmonella typhimurium (121). The research study regarding these novel prebiotic resources is in a very early stage. Optimization of the extraction and purification steps together with the characterization of POS derived from the MPP still needs to be explored to a greater extent. Consequently, the clinical studies and safety evaluation of MPOS used in food should be performed. All in all, it is conclusively recommended from the information gathered herein that MPOS is a novel functional food ingredient that provides the opportunity for a sustainable development approach through biomass utilization (Figure 3).
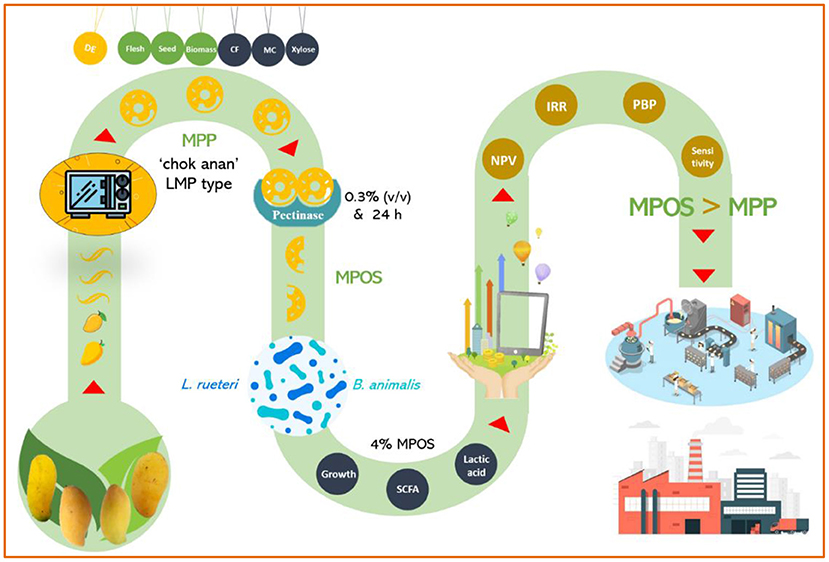
Figure 3. Mango peel pectin and mango pectic oligosaccharide recovery from different varieties of mango fruits.
Author Contributions
SS and MW conceptualized the topic, researched and analyzed the literature, wrote the manuscript, and including interpretations. PT analyzed the background literature and drafted portions of the manuscript. NL, KJ, PR, PS, YP, TC, WR, and PJ provided substantial scholarly guidance on the conception of the topic, manuscript draft, and interpretation and revised the manuscript critically for intellectual content. All authors contributed to the article and approved the submitted version.
Funding
This research was partially supported by the Chiang Mai University.
Conflict of Interest
The authors declare that the research was conducted in the absence of any commercial or financial relationships that could be construed as a potential conflict of interest.
Publisher's Note
All claims expressed in this article are solely those of the authors and do not necessarily represent those of their affiliated organizations, or those of the publisher, the editors and the reviewers. Any product that may be evaluated in this article, or claim that may be made by its manufacturer, is not guaranteed or endorsed by the publisher.
References
1. Parracho H, McCartney AL, Gibson GR. Probiotics and prebiotics in infant nutrition. Proc Nutr Soc. (2007) 66:405–11. doi: 10.1017/S0029665107005678
2. Bharadia L, Agrawal N, Joshi N. Development and functions of the infant gut microflora: Western vs. Indian infants. Int J Pediatr. (2020) 2020:7586264. doi: 10.1155/2020/7586264
3. Rotimi V, Duerden B. The development of the bacterial flora in normal neonates. J Med Microbiol. (1981) 14:51–62. doi: 10.1099/00222615-14-1-51
4. Harmsen HJ, Wildeboer-Veloo AC, Grijpstra J, Knol J, Degener JE, Welling GW. Development of 16S rRNA-based probes for the coriobacterium group and the Atopobium cluster and their application for enumeration of coriobacteriaceae in human feces from volunteers of different age groups. Appl Environ Microbiol. (2000) 66:4523–7. doi: 10.1128/AEM.66.10.4523-4527.2000
5. Sierra C, Bernal M-J, Blasco J, Martínez R, Dalmau J, Ortuño I, et al. Prebiotic effect during the first year of life in healthy infants fed formula containing GOS as the only prebiotic: a multicentre, randomised, double-blind and placebo-controlled trial. Eur J Nutr. (2015) 54:89–99. doi: 10.1007/s00394-014-0689-9
6. Boehm Gn, Moro G. Structural and functional aspects of prebiotics used in infant nutrition. J Nutr. (2008) 138:1818S−28. doi: 10.1093/jn/138.9.1818S
7. Roberfroid M, Gibson GR, Hoyles L, McCartney AL, Rastall R, Rowland I, et al. Prebiotic effects: metabolic and health benefits. Br J Nutr. (2010) 104:S1–63. doi: 10.1017/S0007114510003363
8. A Gosling, Stevens GW, Barber AR, Kentish SE, Gras SL. Recent advances refining galactooligosaccharide production from lactose. Food Chem. (2010) 121:307–18. doi: 10.1016/j.foodchem.2009.12.063
9. Rycroft CE, Jones MR, Gibson GR, Rastall RA. A comparative in vitro evaluation of the fermentation properties of prebiotic oligosaccharides. J Appl Microbiol. (2001) 91:878–87. doi: 10.1046/j.1365-2672.2001.01446.x
10. Vandenplas Y, Zakharova I, Dmitrieva Y. Oligosaccharides in infant formula: more evidence to validate the role of prebiotics. Br J Nutr. (2015) 113:1339–44. doi: 10.1017/S0007114515000823
11. Kunz C, Rudloff S, Baier W, Klein N, Strobel S. Oligosaccharides in human milk: structural, functional, metabolic aspects. Annu Rev Nutr. (2000) 20:699–722. doi: 10.1146/annurev.nutr.20.1.699
12. Cummings JH. Short chain fatty acids in the human colon. Gut. (1981) 22:763. doi: 10.1136/gut.22.9.763
13. Gullón B, Gómez B, Martínez-Sabajanes M, Yáñez R, Parajó JC, Alonso JL. Pectic oligosaccharides: manufacture and functional properties. Trends Food Sci Technol. (2013) 30:153–61. doi: 10.1016/j.tifs.2013.01.006
14. Vos Av, Van Esch B, Stahl B, M'rabet L, Folkerts G, Nijkamp F, et al. Dietary supplementation with specific oligosaccharide mixtures decreases parameters of allergic asthma in mice. Int Immunopharmacol. (2007) 7:1582–7. doi: 10.1016/j.intimp.2007.07.024
15. Sommano S, Ounamornmas P, Nisoa M, Sriwattana S, Page P, Colelli G. Characterisation and physiochemical properties of mango peel pectin extracted by conventional and phase control microwave-assisted extractions. Int Food Res J. (2018) 25:2657–65. doi: 10.1016/j.ijbiomac.2016.06.011
16. Wongkaew M, Kittiwachana S, Phuangsaijai N, Tinpovong B, Tiyayon C, Pusadee T, et al. Fruit characteristics, peel nutritional compositions, and their relationships with mango peel pectin quality. Plants. (2021) 10:1148. doi: 10.3390/plants10061148
17. Chaiwarit T, Masavang S, Mahe J, Sommano S, Ruksiriwanich W, Brachais C-H, et al. Mango (cv. Nam Dokmai) peel as a source of pectin and its potential use as a film-forming polymer. Food Hydrocoll. (2020) 102:105611. doi: 10.1016/j.foodhyd.2019.105611
18. Wongkaew M, Sommano SR, Tangpao T, Rachtanapun P, Jantanasakulwong K. Mango peel pectin by microwave-assisted extraction and its use as fat replacement in dried Chinese sausage. Foods. (2020) 9:450. doi: 10.3390/foods9040450
19. Wongkaew M, Tinpovong B, Sringarm K, Leksawasdi N, Jantanasakulwong K, Rachtanapun P, et al. Crude pectic oligosaccharide recovery from thai chok anan mango peel using pectinolytic enzyme hydrolysis. Foods. (2021) 10:627. doi: 10.3390/foods10030627
20. FAO/WHO. Probiotics in food Health and nutritional properties and guidelines for evaluation. Report of a Joint FAO/WHO Expert Consultation on Evaluation of Health and Nutritional Properties of Probiotics in Food including Powder Milk with Live Lactic Acid Bacteria. Rome: FAO of The United Nation (2001).
21. Masco L, Ventura M, Zink R, Huys G, Swings J. Polyphasic taxonomic analysis of Bifidobacterium animalis and Bifidobacterium lactis reveals relatedness at the subspecies level: reclassification of Bifidobacterium animalis as Bifidobacterium animalis subsp. animalis subsp nov and Bifidobacterium lactis as Bifidobacterium animalis subsp lactis subsp nov. Int J Syst Evol Microbiol. (2004) 54:1137–43. doi: 10.1099/ijs.0.03011-0
22. Holzapfel WH, Haberer P, Geisen R, Björkroth J, Schillinger U. Taxonomy and important features of probiotic microorganisms in food and nutrition. Am J Clin Nutr. (2001) 73:365–73s. doi: 10.1093/ajcn/73.2.365s
23. Kechagia M, Basoulis D, Konstantopoulou S, Dimitriadi D, Gyftopoulou K, Skarmoutsou N, et al. Health benefits of probiotics: a review. ISRN Nutr. (2013) 2013:481651. doi: 10.5402/2013/481651
24. Islam SU. Clinical uses of probiotics. Medicine. (2016) 95:e2658. doi: 10.1097/MD.0000000000002658
25. Hill C, Guarner F, Reid G, Gibson GR, Merenstein DJ, Pot B, et al. Expert consensus document. The international scientific association for probiotics and prebiotics consensus statement on the scope and appropriate use of the term probiotic. Nat Rev Gastroenterol Hepatol. (2014) 11:506–14. doi: 10.1038/nrgastro.2014.66
26. Plaza-Diaz J, Ruiz-Ojeda FJ, Gil-Campos M, Gil A. Mechanisms of action of probiotics. Adv Nutr. (2019) 10:S49–66. doi: 10.1093/advances/nmy063
27. Kandler O, Stetter K-O, Köhl R. Lactobacillus reuteri sp. nov, a new species of Heterofermentative Lactobacilli. Zentralblatt für Bakteriologie: I Abt Originale C: Allgemeine, angewandte und ökologische Mikrobiologie. (1980) 1:264–9. doi: 10.1016/S0172-5564(80)80007-8
28. Jacobsen CN, Rosenfeldt Nielsen V, Hayford AE, Møller PL, Michaelsen KF, Paerregaard A, et al. Screening of probiotic activities of forty-seven strains of Lactobacillus spp. by in vitro techniques and evaluation of the colonization ability of five selected strains in humans. Appl Environ Microbiol. (1999) 65:4949–56. doi: 10.1128/AEM.65.11.4949-4956.1999
29. Valeur N, Engel P, Carbajal N, Connolly E, Ladefoged K. Colonization and immunomodulation by Lactobacillus reuteri ATCC 55730 in the human gastrointestinal tract. Appl Environ Microbiol. (2004) 70:1176–81. doi: 10.1128/AEM.70.2.1176-1181.2004
30. Mu Q, Tavella VJ, Luo XM. Role of lactobacillus reuteri in human health and diseases. Front Microbiol. (2018) 9:757. doi: 10.3389/fmicb.2018.00757
31. Turroni F, Foroni E, Pizzetti P, Giubellini V, Ribbera A, Merusi P, et al. Exploring the diversity of the bifidobacterial population in the human intestinal tract. Appl Environ Microbiol. (2009) 75:1534–45. doi: 10.1128/AEM.02216-08
32. I Mainville, Arcand Y, Farnworth ER. A dynamic model that simulates the human upper gastrointestinal tract for the study of probiotics. Int J Food Microbiol. (2005) 99:287–96. doi: 10.1016/j.ijfoodmicro.2004.08.020
33. Jayamanne VS, Adams MR. Determination of survival, identity and stress resistance of probiotic bifidobacteria in bio-yoghurts. Lett Appl Microbiol. (2006) 42:189–94. doi: 10.1111/j.1472-765X.2006.01843.x
34. Quigley EMM, Chapter 13 - Bifidobacterium animalis spp. lactis. In: Floch MH, Ringel Y, Allan Walker W, editors. The Microbiota in Gastrointestinal Pathophysiology. Boston; Academic Press (2017).
35. Larsen N, Cahú TB, Isay Saad SM, Blennow A, Jespersen L. The effect of pectins on survival of probiotic Lactobacillus spp. in gastrointestinal juices is related to their structure and physical properties. Food microbiol. (2018) 74:11–20. doi: 10.1016/j.fm.2018.02.015
36. Corcoran BM, Stanton C, Fitzgerald GF, Ross RP. Survival of Probiotic Lactobacilli in Acidic Environments Is Enhanced in the Presence of Metabolizable Sugars. Appl Environ Microbiol. (2005) 71:3060–7. doi: 10.1128/AEM.71.6.3060-3067.2005
37. Hernandez-Hernandez O, Muthaiyan A, Moreno FJ, Montilla A, Sanz ML, Ricke SC. Effect of prebiotic carbohydrates on the growth and tolerance of Lactobacillus. Food Microbiol. (2012) 30:355–61. doi: 10.1016/j.fm.2011.12.022
38. Quigley ME, Hudson GJ, Englyst HN. Determination of resistant short-chain carbohydrates (non-digestible oligosaccharides) using gas–liquid chromatography. Food Chem. (1999) 65:381–90. doi: 10.1016/S0308-8146(98)00178-2
39. Gibson GR, Roberfroid MB. Dietary modulation of the human colonic microbiota: introducing the concept of prebiotics. J Nutr. (1995) 125:1401–12. doi: 10.1093/jn/125.6.1401
40. Al-Sheraji SH, Ismail A, Manap MY, Mustafa S, Yusof RM, Hassan FA. Prebiotics as functional foods: a review. J Funct Foods. (2013) 5:1542–53. doi: 10.1016/j.jff.2013.08.009
42. Roberfroid MB. Introducing inulin-type fructans. Br J Nutr. (2005) 93(Suppl. 1) S13–25. doi: 10.1079/BJN20041350
43. Ozcan O, Ozcan T, Yilmaz-Ersan L, Akpinar-Bayizit A, Delikanli B. The use of prebiotics of plant origin in functional milk products. Food Sci Technol. (2016) 4:15–22. doi: 10.13189/fst.2016.040201
44. Padma Ishwarya S, Prabhasankar P. Prebiotics: application in bakery and pasta products. Crit Rev Food Sci Nutr. (2014) 54:511–22. doi: 10.1080/10408398.2011.590244
45. Eeckhaut V, Van Immerseel F, Dewulf J, Pasmans F, Haesebrouck F, Ducatelle R, et al. Arabinoxylooligosaccharides from wheat bran inhibit Salmonella colonization in broiler chickens. Poult Sci. (2008) 87:2329–34. doi: 10.3382/ps.2008-00193
46. Singh M, Sharma R, Banerjee UC. Biotechnological applications of cyclodextrins. Biotechnol Adv. (2002) 20:341–59. doi: 10.1016/S0734-9750(02)00020-4
47. Barczynska R, Slizewska K, Jochym K, Kapusniak J, Libudzisz Z. The tartaric acid-modified enzyme-resistant dextrin from potato starch as potential prebiotic. J Funct Foods. (2012) 4:954–62. doi: 10.1016/j.jff.2012.07.003
48. Sangeetha PT, Ramesh MN, Prapulla SG. Recent trends in the microbial production, analysis and application of Fructooligosaccharides. Trends Food Sci Technol. (2005) 16:442–57. doi: 10.1016/j.tifs.2005.05.003
49. Alander M, Mättö J, Kneifel W, Johansson M, Kögler B, Crittenden R, et al. Effect of galacto-oligosaccharide supplementation on human faecal microflora and on survival and persistence of Bifidobacterium lactis Bb-12 in the gastrointestinal tract. Int Dairy J. (2001) 11:17–825. doi: 10.1016/S0958-6946(01)00100-5
50. Lina BAR, Jonker D, Kozianowski G. Isomaltulose (Palatinose®): a review of biological and toxicological studies. Food Chem Toxicol. (2002) 40:1375–81. doi: 10.1016/S0278-6915(02)00105-9
51. Kaneko T, Kohmoto T, Kikuchi H, Shiota M, Iino H, Mitsuoka T. Effects of isomaltooligosaccharides with different degrees of polymerization on human fecal bifidobactcria. Biosci Biotechnol Biochem. (1994) 58:2288–90. doi: 10.1271/bbb.58.2288
52. Villamiel M, Corzo N, Foda MI, Montes F, Olano AN. Lactulose formation catalysed by alkaline-substituted sepiolites in milk permeate. Food Chem. (2002) 76:7–11. doi: 10.1016/S0308-8146(01)00239-4
53. Babbar N, Dejonghe W, Sforza S, Elst K. Enzymatic pectic oligosaccharides (POS) production from sugar beet pulp using response surface methodology. J Food Sci Technol. (2017) 54:3707–15. doi: 10.1007/s13197-017-2835-x
54. Zhang S, Hu H, Wang L, Liu F, Pan S. Preparation and prebiotic potential of pectin oligosaccharides obtained from citrus peel pectin. Food Chem. (2018) 244:232–7. doi: 10.1016/j.foodchem.2017.10.071
55. Johansen HN, Glitsø V, Bach Knudsen KE. Influence of extraction solvent and temperature on the quantitative determination of oligosaccharides from plant materials by high-performance liquid chromatography. J Agric Food Chem. (1996) 44:470–1474. doi: 10.1021/jf950482b
56. Mussatto SI, Mancilha IM. Non-digestible oligosaccharides: a review. Carbohydr Polym. (2007) 68:587–97. doi: 10.1016/j.carbpol.2006.12.011
57. Vázquez MJ, Alonso JL, Dominguez H, Parajó JC. Xylooligosaccharides: manufacture and applications. Trends Food Sci Technol. (2000) 11:387–93. doi: 10.1016/S0924-2244(01)00031-0
58. González-Herrera SM, Herrera RR, López MG, Rutiaga OM, Aguilar CN, Esquivel JCC, et al. Inulin in food products: prebiotic and functional ingredient. Br Food J. (2015) 117:371–8. doi: 10.1108/BFJ-09-2013-0238
59. Míguez B, Gómez B, Gullón P, Gullón B, Alonso JL. Pectic oligosaccharides and other emerging prebiotics. Probiotics and Prebiotics in Human Nutrition and Health. London: IntechOpen Limited (2016).
60. Garthoff JA, Heemskerk S, Hempenius RA, Lina BA, Krul CA, Koeman JH, et al. Safety evaluation of pectin-derived acidic oligosaccharides (pAOS): genotoxicity and sub-chronic studies. Regul Toxicol Pharmacol. (2010) 57:31–42. doi: 10.1016/j.yrtph.2009.12.004
61. Baldan B, Bertoldo A, Navazio L, Mariani P. Oligogalacturonide-induced changes in the developmental pattern of Daucus carota L. somatic embryos. Plant Sci. (2003) 165:337–48. doi: 10.1016/S0168-9452(03)00193-6
62. Manderson K, Pinart M, Tuohy KM, Grace WE, Hotchkiss AT, Widmer W, et al. In vitro determination of prebiotic properties of oligosaccharides derived from an orange juice manufacturing by-product stream. Appl Environ Microbiol. (2005) 71:8383–9. doi: 10.1128/AEM.71.12.8383-8389.2005
63. Chen J, Liang RH, Liu W, Li T, Liu CM, Wu SS, et al. Pectic-oligosaccharides prepared by dynamic high-pressure microfluidization and their in vitro fermentation properties. Carbohydr Polym. (2013) 91:175–82. doi: 10.1016/j.carbpol.2012.08.021
64. Combo AMM, Aguedo M, Goffin D, Wathelet B, Paquot M. Enzymatic production of pectic oligosaccharides from polygalacturonic acid with commercial pectinase preparations. Food Bioprod Process. (2012) 90:588–96. doi: 10.1016/j.fbp.2011.09.003
65. A Wilkowska, Nowak A, Antczak-Chrobot A, Motyl I, Czyzowska A, Paliwoda A. Structurally different pectic oligosaccharides produced from apple pomace and their biological activity in vitro. Foods. (2019) 8:365. doi: 10.3390/foods8090365
66. Sabater A, Blanco-Doval A, Montilla A, Corzo N. Optimisation of an enzymatic method to obtain modified artichoke pectin and pectic oligosaccharides using artificial neural network tools. In silico and in vitro assessment of the antioxidant activity. Food Hydrocoll. (2021) 110:106161. doi: 10.1016/j.foodhyd.2020.106161
67. Ho Y-Y, Lin C-M, Wu M-C. Evaluation of the prebiotic effects of citrus pectin hydrolysate. J Food Drug Anal. (2017) 25:550–8. doi: 10.1016/j.jfda.2016.11.014
68. A Gómez, Gullón B, Yáñez R, Schols H, Alonso JL. Prebiotic potential of pectins and pectic oligosaccharides derived from lemon peel wastes and sugar beet pulp: a comparative evaluation. J Funct Foods. (2016) 20:108–21. doi: 10.1016/j.jff.2015.10.029
69. Li P-J, Xia J-l, Nie Z-Y, Shan Y. Pectic oligosaccharides hydrolyzed from orange peel by fungal multi-enzyme complexes and their prebiotic and antibacterial potentials. LWT Food Sci Technol. (2016) 69:203–10. doi: 10.1016/j.lwt.2016.01.042
70. Ferreira-Lazarte A, Kachrimanidou V, Villamiel M, Rastall RA, Moreno FJ. In vitro fermentation properties of pectins and enzymatic-modified pectins obtained from different renewable bioresources. Carbohydr Polym. (2018) 199:482–91. doi: 10.1016/j.carbpol.2018.07.041
71. Gómez B, Gullón B, Yáñez R, Parajó JC, Alonso JL. Pectic oligosacharides from lemon peel wastes: production, purification, chemical characterization. J Agric Food Chem. (2013) 61:10043–53. doi: 10.1021/jf402559p
72. Zhu R, Wang C, Zhang L, Wang Y, Chen G, Fan J, et al. Pectin oligosaccharides from fruit of actinidia arguta: structure-activity relationship of prebiotic and antiglycation potentials. Carbohydr Polym. (2019) 217:90–7. doi: 10.1016/j.carbpol.2019.04.032
73. Zhu R, Zhang X, Wang Y, Zhang L, Wang C, Hu F, et al. Pectin oligosaccharides from hawthorn (Crataegus pinnatifida Bunge. Var major): molecular characterization and potential antiglycation activities. Food Chem. (2019) 286:129–35. doi: 10.1016/j.foodchem.2019.01.215
74. Babbar N, Dejonghe W, Gatti M, Sforza S, Elst K. Pectic oligosaccharides from agricultural by-products: production, characterization and health benefits. Crit Rev Biotechnol. (2016) 36:594–606. doi: 10.3109/07388551.2014.996732
75. Kim S-K, Rajapakse N. Enzymatic production and biological activities of chitosan oligosaccharides (COS): a review. Carbohydr Polym. (2005) 62:357–68. doi: 10.1016/j.carbpol.2005.08.012
76. Concha Olmos J, Zúñiga Hansen ME. Enzymatic depolymerization of sugar beet pulp: Production and characterization of pectin and pectic-oligosaccharides as a potential source for functional carbohydrates. Chem Eng J. (2012) 192:29–36. doi: 10.1016/j.cej.2012.03.085
77. Pedrolli D, Monteiro A, Gomes E, Carmona E. Pectin and pectinases: production, characterization and industrial application of microbial pectinolytic enzymes. Open Biotechnol J. (2009) 3:9–18. doi: 10.2174/1874070700903010009
78. Shevchik VE, Hugouvieux-Cotte-Pattat N. Identification of a bacterial pectin acetyl esterase in Erwinia chrysanthemi 3937. Mol Microbiol. (1997) 24:1285–301. doi: 10.1046/j.1365-2958.1997.4331800.x
79. Cameron R, Luzio G, Savary B, Goodner K. Digestion patterns of two commercial endopolygalacturonases on polygalacturonate oligomers with a degree of polymerization of 7 to 21. Proc Fla State Hortic Soc. (2009) 122:295–302.
80. Parenicová L, Benen JA, Kester HC, Visser J. pgaA and pgaB encode two constitutively expressed endopolygalacturonases of Aspergillus niger. Biochem J. (2000) 345:637–44. doi: 10.1042/bj3450637
81. Pedrolli DB, Carmona EC. Purification and characterization of a unique pectin lyase from Aspergillus giganteus able to release unsaturated monogalacturonate during pectin degradation. Enzyme Res. (2014) 2014:353915. doi: 10.1155/2014/353915
82. Martínez M, Gullón B, Schols HA, Alonso JL, Parajó JC. Assessment of the production of oligomeric compounds from sugarbeet pulp. Ind Eng Chem Res. (2009) 48:4681. doi: 10.1021/ie8017753
83. Lama-Muñoz A, Rodríguez-Gutiérrez G, Rubio-Senent F, Fernández-Bolaños J. Production, characterization and isolation of neutral and pectic oligosaccharides with low molecular weights from olive by-products thermally treated. Food Hydrocoll. (2012) 28:92–104. doi: 10.1016/j.foodhyd.2011.11.008
84. Olano-Martin A, Gibson GR, Rastell RA. Comparison of the in vitro bifidogenic properties of pectins and pectic-oligosaccharides. J Appl Microbiol. (2002) 93:505–11. doi: 10.1046/j.1365-2672.2002.01719.x
85. Ignatova T, Iliev I, Kirilov N, Vassileva T, Dalgalarrondo M, Haertle T, et al. Effect of oligosaccharides on the growth of Lactobacillus delbrueckii subsp. bulgaricus strains isolated from dairy products. J Agric Food Chem. (2009) 57:9496–502. doi: 10.1021/jf901684z
86. Holck J, Lorentzen A, Vigsnæs LK, Licht TR, Mikkelsen JD, Meyer AS. Feruloylated and nonferuloylated arabino-oligosaccharides from sugar beet pectin selectively stimulate the growth of Bifidobacterium spp. in human fecal in vitro fermentations. J Agric Food Chem. (2011) 59:6511–9. doi: 10.1021/jf200996h
87. Garna H, Mabon N, Nott K, Wathelet B, Paquot M. Kinetic of the hydrolysis of pectin galacturonic acid chains and quantification by ionic chromatography. Food Chem. (2006) 96:477–84. doi: 10.1016/j.foodchem.2005.03.002
88. Leijdekkers A, Bink J, Geutjes S, Schols HA, Gruppen H. Enzymatic saccharification of sugar beet pulp for the production of galacturonic acid and arabinose, a study on the impact of the formation of recalcitrant oligosaccharides. Bioresour Technol. (2012) 128C:518–25. doi: 10.1016/j.biortech.2012.10.126
89. Gómez A, Gullón B, Remoroza C, Schols HA, Parajó JC, Alonso JL. Purification, characterization, and prebiotic properties of pectic oligosaccharides from orange peel wastes. J Agric Food Chem. (2014) 62:9769–82. doi: 10.1021/jf503475b
90. Holck J, Hjernø K, Lorentzen A, Vigsnæs LK, Hemmingsen L, Licht TR, et al. Tailored enzymatic production of oligosaccharides from sugar beet pectin and evidence of differential effects of a single DP chain length difference on human faecal microbiota composition after in vitro fermentation. Process Biochem. (2011) 46:1039–49. doi: 10.1016/j.procbio.2011.01.013
91. Iwasaki K-I, Matsubara Y. Purification of pectate oligosaccharides showing root-growth-promoting activity in lettuce using ultrafiltration and nanofiltration membranes. J Biosci Bioeng. (2000) 89:495–7. doi: 10.1016/S1389-1723(00)89104-5
92. Guillaumie A, Justesen SF, Mutenda KE, Roepstorff P, Jensen KJ, Thomas OR. Fractionation, solid-phase immobilization and chemical degradation of long pectin oligogalacturonides. Initial steps towards sequencing of oligosaccharides. Carbohydr Res. (2006) 341:118–29. doi: 10.1016/j.carres.2005.10.011
93. Ralet M-C, Cabrera JC, Bonnin E, Quéméner B, Hellìn P, Thibault J-F. Mapping sugar beet pectin acetylation pattern. Phytochemistry. (2005) 66:1832–43. doi: 10.1016/j.phytochem.2005.06.003
94. Coenen GJ, Kabel MA, Schols HA, Voragen AG. CE-MSn of complex pectin-derived oligomers. Electrophoresis. (2008) 29:2101–11. doi: 10.1002/elps.200700465
95. Ström A, Williams MA. On the separation, detection and quantification of pectin derived oligosaccharides by capillary electrophoresis. Carbohydr Res. (2004) 339:1711–6. doi: 10.1016/j.carres.2004.05.010
96. Ralet MC, Lerouge P, Quéméner B. Mass spectrometry for pectin structure analysis. Carbohydr Res. (2009) 34:1798–807. doi: 10.1016/j.carres.2008.08.036
97. Colquhoun IJ, de Ruiter GA, Schols HA, Voragen AG. Identification by nmr spectroscopy of oligosaccharides obtained by treatment of the hairy regions of apple pectin with rhamnogalacturonase. Carbohydr Res. (1990) 206:131–44. doi: 10.1016/0008-6215(90)84012-J
98. Renard CM, Lahaye M, Mutter M, Voragen FG, Thibault JF. Isolation and structural characterisation of rhamnogalacturonan oligomers generated by controlled acid hydrolysis of sugar-beet pulp. Carbohydr Res. (1997) 305:271–80. doi: 10.1016/S0008-6215(97)10028-3
99. Ishii T, Ichita J, Matsue H, Ono H, Maeda I. Fluorescent labeling of pectic oligosaccharides with 2-aminobenzamide and enzyme assay for pectin. Carbohydr Res. (2002) 337:1023–32. doi: 10.1016/S0008-6215(02)00087-3
100. Westphal Y, Kühnel S, Schols HA, Voragen AG, Gruppen H. LC/CE-MS tools for the analysis of complex arabino-oligosaccharides. Carbohydr Res. (2010) 345:2239–51. doi: 10.1016/j.carres.2010.07.011
101. Combo AMM, Aguedo M, Quiévy N, Danthine S, Goffin D, Jacquet N, et al. Characterization of sugar beet pectic-derived oligosaccharides obtained by enzymatic hydrolysis. Int J Biol Macromol. (2013) 52:148–56. doi: 10.1016/j.ijbiomac.2012.09.006
102. Remoroza C, Buchholt HC, Gruppen H, Schols HA. Descriptive parameters for revealing substitution patterns of sugar beet pectins using pectolytic enzymes. Carbohydr Polym. (2014) 101:1205–15. doi: 10.1016/j.carbpol.2013.10.034
103. Barrangou R, Altermann E, Hutkins R, Cano R, Klaenhammer T. Functional and comparative genomic analyses of an operon involved in fructooligosaccharide utilization by Lactobacillus acidophilus. Proc Natl Acad Sci U S A. (2003) 100:8957–62. doi: 10.1073/pnas.1332765100
104. Mueller I, Kiedorf G, Runne E, Seidel-Morgenstern A, Hamel Synthesis C. Kinetic analysis and modelling of galacto-oligosaccharides formation. Chem Eng Res Des. (2017) 130:154–66. doi: 10.1016/j.cherd.2017.11.038
105. Burana-Osot J, Soonthornchareonnon N, Chaidedgumjorn A, Hosoyama S, Toida T. Determination of galacturonic acid from pomelo pectin in term of galactose by HPAEC with fluorescence detection. Carbohydr Polym. (2010) 81:461. doi: 10.1016/j.carbpol.2010.03.001
106. Kang HJ, Jo C, Kwon JH, Son JH, An BJ, Byun MW. Antioxidant and cancer cell proliferation inhibition effect of citrus pectin-oligosaccharide prepared by irradiation. J Med Food. (2006) 9:313–20. doi: 10.1089/jmf.2006.9.313
107. Li T, Li S, Du L, Wang N, Guo M, Zhang J, et al. Effects of haw pectic oligosaccharide on lipid metabolism and oxidative stress in experimental hyperlipidemia mice induced by high-fat diet. Food Chem. (2010) 121:1010–3. doi: 10.1016/j.foodchem.2010.01.039
108. Hotchkiss A, Olano-Martin E, Grace W, Gibson G, Rastall R. Pectic Oligosaccharides as Prebiotics. Cambridge: Society for Applied Microbiology Wiley (2003).
109. Dongowski A, Lorenz A. Unsaturated oligogalacturonic acids are generated by in vitro treatment of pectin with human faecal flora. Carbohydr Res. (1998) 314:237–44. doi: 10.1016/S0008-6215(98)00304-8
110. Olano-Martin E, Williams MR, Gibson GR, Rastall RA. Pectins and pectic-oligosaccharides inhibit Escherichia coli O157:H7 Shiga toxin as directed towards the human colonic cell line HT29. FEMS Microbiol Lett. (2003) 218:101–5. doi: 10.1111/j.1574-6968.2003.tb11504.x
111. Rhoades J, Manderson K, Wells A, Hotchkiss A, Gibson G, Formentin K, et al. Oligosaccharide-mediated inhibition of the adhesion of pathogenic Escherichia coli strains to human gut epithelial cells in vitro. J Food Prot. (2008) 71:2272–7. doi: 10.4315/0362-028X-71.11.2272
112. Zhang A, Zhang M, Tao Y, Wang G, Xia B. Madecassic acid inhibits the mouse colon cancer growth by inducing apoptosis and immunomodulation. J BUON. (2014) 19:372–6.
113. Vulevic J, Drakoularakou A, Yaqoob P, Tzortzis G, Gibson GR. Modulation of the fecal microflora profile and immune function by a novel trans-galactooligosaccharide mixture (B-GOS) in healthy elderly volunteers. Am J Clin Nutr. (2008) 88:1438–46. doi: 10.3945/ajcn.2008.26242
114. Vos AP, Haarman M, van Ginkel JW, Knol J, Garssen J, Stahl B, et al. Dietary supplementation of neutral and acidic oligosaccharides enhances Th1-dependent vaccination responses in mice. Pediatr Allergy Immunol. (2007) 18:304–12. doi: 10.1111/j.1399-3038.2007.00515.x
115. Gullón B, Gullón P, Sanz Y, Alonso JL, Parajó JC. Prebiotic potential of a refined product containing pectic oligosaccharides. LWT Food Sci Technol. (2011) 44:1687–96. doi: 10.1016/j.lwt.2011.03.006
116. Chung WSF, Meijerink M, Zeuner B, Holck J, Louis P, Meyer AS, et al. Prebiotic potential of pectin and pectic oligosaccharides to promote anti-inflammatory commensal bacteria in the human colon. FEMS Microbiol Ecol. (2017) 93:fix127. doi: 10.1093/femsec/fix127
117. Hotchkiss AT, Olano-Martin E, Grace WE, Gibson GR, Rastall RA. Pectic Oligosaccharides as Prebiotics, Oligosaccharides in Food and Agriculture, American Chemical Society. Washington, DC: American Chemical Society (2003).
118. Al-Tamimi AHM, Palframan RJ, Cooper JM, Gibson GR, Rastall RA. In vitro fermentation of sugar beet arabinan and arabino-oligosaccharides by the human gut microflora. J Appl Microbiol. (2006) 100:407–14. doi: 10.1111/j.1365-2672.2005.02780.x
119. Yeung YK, Kang Y-R, So BR, Jung SK, Chang YH. Structural, antioxidant, prebiotic and anti-inflammatory properties of pectic oligosaccharides hydrolyzed from okra pectin by Fenton reaction. Food Hydrocoll. (2021) 118:106779. doi: 10.1016/j.foodhyd.2021.106779
120. Mao X, Xiao X, Chen D, Yu B, He J, Chen H, et al. Dietary apple pectic oligosaccharide improves gut barrier function of rotavirus-challenged weaned pigs by increasing antioxidant capacity of enterocytes. Oncotarget. (2017) 8:92420–30. doi: 10.18632/oncotarget.21367
Keywords: fruit biomass, intestinal microflora, lactic acid bacteria, short-chain fatty acids, probiotic
Citation: Wongkaew M, Tangjaidee P, Leksawasdi N, Jantanasakulwong K, Rachtanapun P, Seesuriyachan P, Phimolsiripol Y, Chaiyaso T, Ruksiriwanich W, Jantrawut P and Sommano SR (2022) Mango Pectic Oligosaccharides: A Novel Prebiotic for Functional Food. Front. Nutr. 9:798543. doi: 10.3389/fnut.2022.798543
Received: 20 October 2021; Accepted: 22 February 2022;
Published: 24 March 2022.
Edited by:
Jinhu Tian, Zhejiang University, ChinaReviewed by:
Shashanka Prasad, JSS Academy of Higher Education and Research, IndiaCopyright © 2022 Wongkaew, Tangjaidee, Leksawasdi, Jantanasakulwong, Rachtanapun, Seesuriyachan, Phimolsiripol, Chaiyaso, Ruksiriwanich, Jantrawut and Sommano. This is an open-access article distributed under the terms of the Creative Commons Attribution License (CC BY). The use, distribution or reproduction in other forums is permitted, provided the original author(s) and the copyright owner(s) are credited and that the original publication in this journal is cited, in accordance with accepted academic practice. No use, distribution or reproduction is permitted which does not comply with these terms.
*Correspondence: Sarana Rose Sommano, c2FyYW5hLnNAY211LmFjLnRo