- 1Univ-Lyon, CarMeN Laboratory, Inserm U1060, INRAE UMR1397, Université Claude Bernard Lyon 1, Pierre Bénite, France
- 2Service de Biochimie et de Biologie Moléculaire, Centre de Biologie et de Pathologie Est, Hospices Civils de Lyon, Bron, France
Sphingolipids are structural components of cell membranes and lipoproteins but also act as signaling molecules in many pathophysiological processes. Although sphingolipids comprise a small part of the plasma lipidome, some plasma sphingolipids are recognized as implicated in the development of metabolic diseases and cardiovascular diseases. Plasma sphingolipids are mostly carried out into lipoproteins and may modulate their functional properties. Lipids ingested from the diet contribute to the plasma lipid pool besides lipids produced by the liver and released from the adipose tissue. Depending on their source, quality and quantity, dietary lipids may modulate sphingolipids both in plasma and lipoproteins. A few human dietary intervention studies investigated the impact of dietary lipids on circulating sphingolipids and lipid-related cardiovascular risk markers. On the one hand, dietary saturated fatty acids, mainly palmitic acid, may increase ceramide concentrations in plasma, triglyceride-rich lipoproteins and HDL. On the other hand, milk polar lipids may decrease some molecular species of sphingomyelins and ceramides in plasma and intestine-derived chylomicrons. Altogether, different dietary fatty acids and lipid species can modulate circulating sphingolipids vehicled by postprandial lipoproteins, which should be part of future nutritional strategies for prevention of cardiovascular diseases.
1. Introduction
There is a large consensus for the implication of dietary fats on cardiometabolic health and on nutrition-driven diseases. Beneficial or harmful effects of dietary fats (e.g., butter, margarine, oils, fat spread…) on cardiovascular events partly depend on the specific sources of dietary lipids, their structures and their amounts (1). Triglycerides, representing the major dietary lipids, modulate fasting and postprandial lipemia and modify the lipid composition of triglyceride-rich lipoproteins (TGRL), contributing to the incidence of various cardiovascular events (2). Advances in lipidomic analyses by mass spectrometry have helped to identify minor lipid species as surrogate markers of cardiovascular risk. Among them, sphingolipids constitute a very diverse category of lipids with different classes and more than a thousand individual molecular species (3, 4). Sphingolipids are composed of a long-chain amino-alcohol backbone as a sphingoid base (mostly d18:1 sphingosine in humans) to which a fatty acid is attached by an amide bond. Each sphingolipid class presents numerous molecular species varying in chain length (from C14 to C26), degree of saturation (mostly saturated and monounsaturated) and degree of hydroxylation. In plasma, sphingolipids account for 5% of total lipids. Among them, sphingomyelins (SM) represent 10% of total plasma phospholipids and account for 96% of total plasma sphingolipids while ceramides (Cer) represent 3% of plasma sphingolipids (3). Other plasma sphingolipids are glycosphingolipids including glucosylceramides (glucosylCer) and gangliosides as well as the bioactive sphingosine and sphingosine-1-phosphate molecules. Lipoproteins are the main carriers of plasma sphingolipids. Very few clinical studies have focused on the modifications of surface sphingolipids in lipoproteins, which might potentially alter the functional properties of lipoproteins, especially in cardiometabolic diseases. Although sphingolipids represent a small part of the plasma and lipoprotein lipidome, some of them are important structural and bioactive signaling molecules in mammals, and some have significant roles in the development of metabolic disorders (5). Indeed, specific Cer molecular species in plasma are known as potent predictive biomarkers of cardiovascular disease occurrence (6). Pharmacological treatments and nutritional interventions can modulate circulating sphingolipids in cancer and metabolic diseases respectively, and drugs targeting sphingolipid metabolism have indeed shown some promising results in anti-cancer therapeutics (7). The source, quality and quantity of dietary lipids may also impact circulating sphingolipids and possibly prevent nutrition-driven metabolic diseases. This mini-review will examine the effects of dietary fatty acids and SM on circulating sphingolipids, and will be mainly focused on human studies.
2. Dietary fatty acids and circulating sphingolipids
2.1. Effects of dietary fatty acids on plasma sphingolipids
Observational studies drew attention to the positive associations between plasma Cer concentrations and incident clinical events of cardiovascular diseases, and to the modulation of plasma sphingolipids by healthy diets. In the prospective case-cohort PREDIMED study (8), plasma concentrations of four Cer molecular species, Cer(d18:1/16:0), Cer(d18:1/22:0), Cer(d18:1/24:0) and Cer(d18:1/24:1) were positively associated with the incidence of cardiovascular events (nonfatal acute myocardial infarction, nonfatal stroke, or cardiovascular death). Moreover, a Mediterranean diet enriched with extra virgin olive oil or nuts might mitigate the potential deleterious effects of elevated plasma Cer concentrations on cardiovascular disease risk in participants initially free from cardiovascular diseases. Similarly, adherence of 93 overweight participants for 12 weeks to an healthy Nordic diet (consisting of whole grains, fruits, vegetables, berries, vegetable oils and margarines, fish, low-fat milk products, and low-fat meat) resulted in decreased plasma concentrations of Cer and especially Cer(d18:1/22:0), Cer(d18:1/23:0) and Cer(d18:1/24:0) when compared to participants assigned to a control Nordic diet (including low-fiber cereal products, dairy fat-based spreads, regular-fat milk products, and a limited amount of fruits, vegetables, and berries) (9). Fasting plasma Cer concentrations correlated positively with total cholesterol and LDL cholesterol concentrations, which is in favor of beneficial effects on cardiovascular risk by modulating Cer levels with healthy dietary habits.
Recently, data from the Epidemiological Study on the Insulin Resistance Syndrome (D.E.S.I.R.) cohort highlighted the relationships between the consumption of dairy products and plasma sphingolipidomic profile. In 105 women from this prospective French cohort, higher consumption of fresh dairy foods, except cheese, was associated with lower plasma total concentrations of dihydroceramides (dihydroCer), direct precursors of ceramides, and a trend toward lower plasma Cer concentrations (10).
Based on cross-sectional data, a large scale sphingolipidomic analysis of plasma from 2,860 ethnic Chinese Singaporeans showed positive associations between the intake of dietary saturated fatty acids (SFA) estimated from food frequency questionnaires and higher plasma concentrations of sphingolipids with a d16:1 sphingoid base backbone, including Cer, monohexosylceramides, dihexosylceramides, SM and sphingosine 1-phosphate (11). Higher intake of polyunsatured fatty acids (PUFA) was associated with lower plasma long-chain Cer and long-chain hexosylceramides concentrations, while monounsaturated fatty acid intake was not associated with plasma sphingolipid concentrations (11).
More targeted studies on the specific effects of fatty acids that constitute dietary lipids showed differential effects of fatty acids on circulating sphingolipids depending on their saturation or unsaturation and their fatty acid family, i.e. ω3 or ω6 or ω9. A high-fat diet, enriched in SFA, resulted in the accumulation of Cer in plasma, liver and muscles, and was associated with adverse cardiovascular events (12, 13). In 14 overweight subjects, a 1,000 kCal/day overfeeding during 3 weeks with a diet including SFA (30 g coconut oil, 40 g butter and 100 g blue cheese 40% fat per day) resulted in increased plasma total Cer concentrations and particularly long-chain Cer(d18:1/24:0), Cer(d18:1/24:1) and dihydroceramide Cer(d18:0/24:0) as well as hepatic TG concentrations (14). No change in plasma Cer concentrations was observed in 12 subjects consuming a hypercaloric diet comprised of unsaturated fatty acids (14). Similarly, another double-blind randomized nutritional intervention study performed in 61 middle-aged overweight subjects for 8 weeks showed differential effects of diets enriched with different oils on some serum sphingolipids (15) when relative changes from baseline to post-intervention were compared between the two diets. Overfeeding with muffins containing palm oil, rich in palmitic acid (C16:0), resulted in increased serum Cer, dihydroCer and glucosylCer concentrations, while overfeeding with muffins containing sunflower oil, rich in linoleic acid (C18:2 ω6), decreased serum dihydroCer, glucosylCer and lactosylceramides (15). It was hypothesized that an increased intake of palmitic acid and SFA may have stimulated the de novo synthesis of Cer in the liver via the condensation of palmitoyl-CoA and serine by serine-palmitoyltransferase. These results are in line with studies in rodents showing that the dietary fatty acid composition impacts the main pathways of Cer generation via SM hydrolysis and de novo synthesis, and degradation by regulating ceramidase activities (16).
Regarding the effects of long-chain ω3 PUFA, eicosapentaenoic acid (EPA, C20:5 ω3) and docosahexaenoic acid (DHA, C22:6 ω3), several randomized controlled trials demonstrated their health benefits for secondary prevention of cardiovascular diseases, although still controversial (17, 18). In particular, high doses (>2 g/day) of ω3 PUFA lowered plasma TG concentrations (19) resulting primarily from the decrease in hepatic VLDL-TG production, and secondarily from the increase in VLDL clearance (20). However, very few data in humans are available on the effects of ω3 PUFA on circulating polar lipids. Rodent studies reported that EPA and DHA may reduce Cer concentrations in muscles, liver and adipose tissue (21, 22). In 33 patients with recent acute myocardial infarction or unstable ischemic attack, an 8-week consumption of fatty fish at least 4 times a week led to decreased concentrations of plasma Cer, diacylglycerols and lysophosphatidylcholines (23), in addition to increased proportions of plasma EPA, docosapentaenoic acid (C22:5 ω3) and DHA as expected. It remains to be determined whether lower plasma Cer concentrations might be part of the cardioprotective effects of long-chain ω3 PUFA.
2.2. Effects of dietary fatty acids on sphingolipids in lipoproteins
Sphingolipids are mostly transported in the bloodstream within lipoproteins (5) and are present at different amounts in the lipoprotein classes: VLDL, LDL and HDL (4, 24–26). To get a deeper insight into the dietary lipid-induced modifications of plasma sphingolipids, it is of key importance to determine the concentrations of sphingolipid classes into intestine-derived lipoproteins (27, 28). The intestine produces triglyceride-rich lipoproteins (TGRL), including chylomicrons, apoB48-containing VLDL (29), but also apoA-I, the principal apolipoprotein of HDL (30, 31). A randomized parallel group study in 30 obese type 2 diabetic patients compared the effects of a single fat meal on postprandial TGRL collected 4 h after ingestion of 20 g lipids from a hazelnut-cocoa palm oil-rich spread or butter (28); among fatty acids in each fat source, palmitic acid represented 33 mol% and 42 mol% of total fatty acids respectively. Following the consumption of either meal rich in SFA, total Cer concentrations were higher in postprandial TGRL compared to fasting TGRL, regardless of the saturated fats consumed. Detailed analyses of the sphingolipid molecular species enabled to show specific increases of Cer(d18:1/16:0) and very long chain Cer(d18:1/24:0), Cer(d18:1/24:1). No modification of SM and ganglioside GM3 concentrations nor sphingoid base concentrations were observed between postprandial TGRL and fasting TGRL, nor between the two breakfasts. These results confirm in humans that the supply of dietary palmitic acid may increase de novo synthesis of Cer resulting in increased concentrations of Cer in postprandial TGRL. This clinical study also shows that the ingestion of a single fat-rich meal from a plant-based or dairy source by type 2 diabetic patients is sufficient to modify the sphingolipid profile of postprandial TGRL, and potentially their functional properties. Complementary results of the same study indeed showed that postprandial TGRL induced acute in vitro platelet activation, via increased agonist-induced platelet aggregation and synthesis of thromboxane A2 (32), which may contribute to the increased atherogenicity of postprandial TGRL in type 2 diabetes (2). Extending the study with a longer duration and higher amounts of lipids could have led to stronger modifications of the sphingolipidomic profile of lipoproteins. During the postprandial state and hydrolysis of TG from TGRL by lipoprotein lipase, a rapid remodeling of TGRL occurs. Surface lipids of TGRL, including phospholipids and SM, are transferred to HDL via the phospholipid transfer protein (5). The intestine also synthesizes some HDL particles. It is then conceivable that the SFA-induced increase of TGRL Cer in turn may have effects on the sphingolipidome of HDL. One study investigated the effect of a high-fat meal composed of heavy whipping cream on fasting and postprandial HDL in 16 healthy subjects (33). The consumption of this SFA-rich meal led to increases in Cer(d18:1/22:0), Cer(d18:1/24:0), Cer(d18:2/24:0), SM(d18:1/14:0), and lactosylceramide LacCer(d18:1/16:0), as well as some phosphatidylcholine molecular species. Since HDL phosphosphingolipidome may strongly affect the atheroprotective functionalities of HDL (34), it can be speculated that SFA-induced higher Cer in postprandial HDL might have led to altered functionalities of HDL.
The metabolism of lipoproteins is highly dynamic and thereby all lipoproteins classes, including liver-derived VLDL and LDL, can be modified by exogenous dietary lipids. In this regard, a pilot study in 6 hypertriglyceridemic men showed that the supplementation for 8 weeks with 3 g/d fish oil rich in EPA and DHA decreased fasting TG in plasma and large VLDL but also Cer concentrations in VLDL, without any modification of SM concentrations in VLDL (35). Regarding LDL, a dietary intervention study comparing the effects of different types of fats on plasma lipoproteins in 36 overweight middle-aged subjects showed that a 3-week consumption of extra daily 1,000 kCal coming from SFA resulted in modifications of LDL composition, independently of LDL cholesterol (36). Excess consumption of SFA (30 g coconut oil, 40 g butter, 100 g blue cheese 40% fat), but not unsaturated fats (36 g olive oil, 266 g pesto, 54 g pecan nuts, 20 g butter), increased concentrations of SM, Cer and saturated TG in LDL and increased their susceptibility to aggregate and consequently their atherogenicity. Altogether, these studies show that the type of fatty acids in the diet affects the sphingolipidomic composition of plasma lipoproteins and consequently their functional properties and lipid-related cardiovascular risk markers.
3. Dietary sphingolipids and circulating sphingolipids
3.1. Absorption and transport of sphingolipids
Dietary sphingolipids are a minor part of dietary lipids compared to triglycerides. Their intake has been estimated to be around 300–400 mg/day in the United States (37). In Japan, total amounts of sphingolipids, i.e., SM and glucosylCer, in a typical 3,000 kCal meal represent 128–292 mg (38). Dairy products are the primary sources of sphingolipids in the diet, followed by meats, eggs, fishes and some vegetables (37). Polar lipids are the main constituents of the milk fat globule membrane and mainly include 21–24% SM, 25–36% phosphatidylethanolamine, 27–29% phosphatidylcholine, 8–12% phosphatidylserine and 8% phosphatidylinositol (39, 40). Pioneering work from Nilsson group showed that SM are not absorbed intact (41). In the small intestine, SM are sequentially hydrolyzed by alkaline sphingomyelinase to phosphocholines and Cer, which are hydrolyzed to sphingosine and unesterified fatty acids by a neutral ceramidase. The mechanism of incorporation of sphingolipids derived from dietary SM into lipoproteins remains not precisely known. Studies in lymph duct cannulated rats fed with a triolein emulsion together with radiolabeled SM and Cer showed that traces of intact SM or Cer were incorporated into chyle lipids. Regarding absorption of free sphingosine, a metabolite of SM, <10% tritiated sphingosine was recovered into chyle lipids, mainly into TG and fatty acids and a small percentage into Cer and SM (42).
3.2. Effects of dietary sphingolipids on circulating lipids
Several initial studies in rodents showed benefits of milk polar lipids and SM supplementations on plasma cholesterol levels [reviewed by Norris et al. (43)] via a SM-induced inhibition of cholesterol absorption. A few studies in humans determined the effects of diets enriched in dairy products on plasma lipids. A 4-week supplementation of 48 healthy volunteers with a buttermilk-based formulation enriched with sphingolipids (700 mg SM, 180 mg glucosylCer and 95 mg gangliosides) had no effect on fasting plasma TG, cholesterol, LDL-cholesterol and HDL-cholesterol concentrations (44). By contrast, a 4-week supplementation with 45 g/day buttermilk composed of 187 mg total phospholipids and 24 mg SM reduced serum concentrations of TG (-11%) and total cholesterol (-3%) in 34 healthy volunteers with fasting LDL-cholesterol concentrations of 3.75 mM (1.45 g/L) (45). Besides fasting TG, postprandial TG are independently associated with the incidence of cardiovascular events (46). Therefore, the influence of dietary lipids on the postprandial plasma sphingolipidome needs to be determined. In 18 healthy volunteers, a high fat (40 g) standard breakfast including 975 mg sphingolipids had no effect on postprandial plasma lipid levels measured from 1 to 7 h after the meal consumption, compared to a placebo drink of skim milk powder (47). In 16 normolipemic obese men, the consumption for 4–6 weeks of a breakfast comprising 54 g lipids issued from dairy fat or soybean oil modified the postprandial plasma phospholipid concentrations in relation with the source of dietary fat (12). The concentrations of dihydroCer, SM, GM3 ganglioside, dihexosylceramide, plasmalogens, phosphatidylcholine and phosphatidylinositol increased 4 h after the dairy breakfast compared to fasting concentrations. Despite similar lipemic responses and increased TG concentrations compared to the dairy meal, the consumption of the soy meal did not result in any changes of plasma phospholipids but decreased SM and ganglioside GM3 concentrations 1 h after the soy meal.
To go further on the effects of milk polar lipids on markers of cardiometabolic risk, the next step is to determine their effects in patients at risk for metabolic and cardiovascular diseases and to consider the molecular species of sphingolipid classes. Only one comprehensive dietary intervention study in overweight post-menopausal women at risk of cardiovascular diseases explored the impact of milk polar lipids on some lipid cardiovascular risk markers and also on the circulating concentrations of SM and Cer in plasma and chylomicrons both at fasting and during the postprandial state (48). Milk polar lipids were provided by a cream cheese enriched with 5 g polar lipids via a butterserum concentrate. The 4-week daily supplementation with milk polar lipids led to decreased serum TG (-15%), LDL-cholesterol (-9%), and increased HDL-cholesterol (+5%) concentrations as well as to decreased postprandial concentrations of TG and cholesterol in intestine-derived chylomicrons in volunteers compared to volunteers who daily consumed cream cheese devoid of polar lipids (containing only milk TG). In addition, circulating concentrations of sphingolipids were modified by the consumption of milk polar lipids (27). In fasting serum, the second most abundant Cer species, Cer(d18:1/24:1) as well as SM(d18:1/16:1), SM(d18:1/18:1), SM(d18:1/20:1) decreased in the group who consumed 5 g/day of milk polar lipids while serum concentrations of total SM, Cer and phospholipids did not differ significantly between groups. In intestine-derived chylomicrons from the milk polar lipid group, total SM concentrations decreased and total Cer concentrations tended to decrease after the 4-week intervention vs. before intervention. Regarding the molecular species within each class of SM and Cer in chylomicrons, several molecular species of SM relative to CM TG decreased after milk polar lipid intervention, i.e., SM(d18:1/16:0), SM(d18:1/18:0), SM(d18:1/16:1), SM(d18:1/18:1), SM(d18:1/20:1), SM(d18:1/24:0), SM(d18:1/24:1), as well as very long chain Cer species Cer(d18:1/22:0), Cer(d18:1/24:0) and Cer(d18:1/24:1). These observed decreases of SM and Cer molecular species within chylomicrons after milk polar lipid intervention might be due to the endogenous metabolism of sphingolipids inside the enterocytes following the supply of exogenous dietary SM and the supply of one of its degradation products such as sphingosine and/or Cer (41).
4. Conclusion and perspectives
Circulating sphingolipids can be modulated by exogenous dietary lipids (Figure 1). Observational studies and nutritional intervention trials showed that dietary palmitic acid as well as milk polar lipids and dietary SM modified the concentrations of some sphingolipids in plasma but also in lipoprotein classes (summarized in Table 1). In particular, some specific molecular species of Cer and SM, rather than total Cer and SM, were modified in postprandial TGRL and HDL, which could modify the functionalities of lipoproteins, especially in the context of nutrition-driven chronic diseases. Detailed analyses of sphingolipid classes and molecular species in intestine-derived lipoproteins and also in liver-derived ones could unravel the mechanisms that link sphingolipids species modified by dietary lipids and cardiovascular risk outcomes. It will help to identify novel sphingolipid molecular species in lipoproteins as biomarkers of cardiovascular disease risk, independently of LDL-cholesterol and TG (49). Most clinical data indicate that circulating Cer are modified by dietary SFA and SM. Considering that Cer are at the center of sphingolipid metabolism, it is likely that dietary lipids may also modify the concentrations of other sphingolipid derivatives including sphingoid bases. Therefore, the endogenous metabolism of sphingolipids, especially Cer and sphingosine, inside enterocytes has to be fully elucidated.
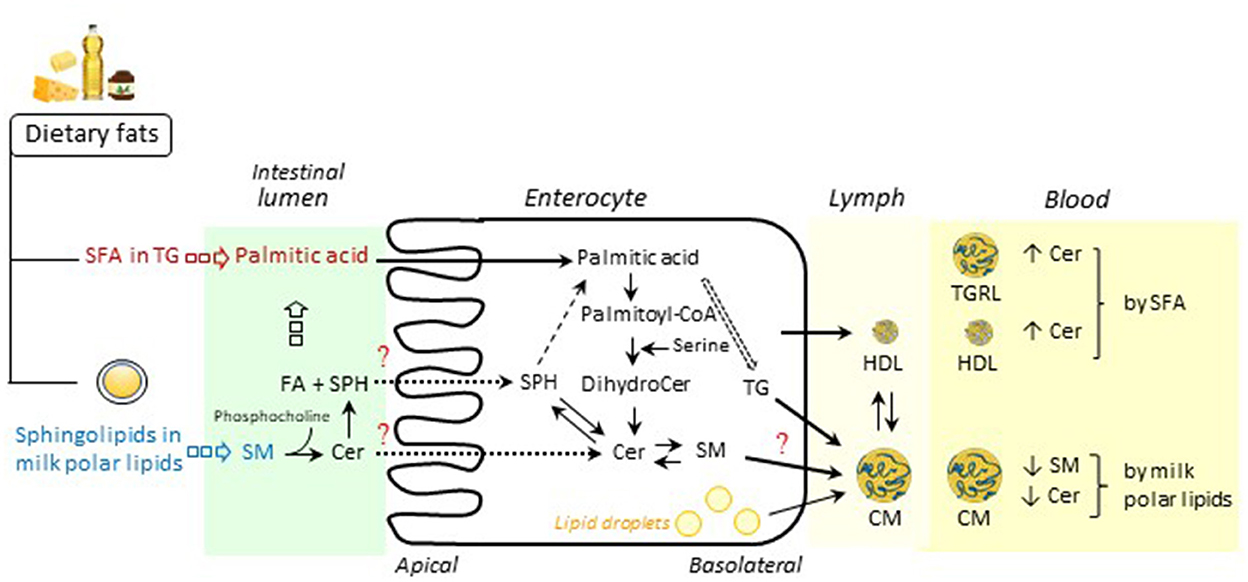
Figure 1. Schematic overview of postprandial intestinal metabolism of dietary lipids, especially sphingolipids. Following ingestion of dietary fats, triglycerides (TG) including saturated fatty acids (SFA) are digested in the intestinal lumen by pancreatic lipases into 2-monoacylglycerols and unesterified fatty acids (FA) (e.g., palmitic acid), which are then absorbed in the enterocytes, resynthesized as TG and packaged into chylomicrons (CM) for secretion into lymph. In the enterocyte, condensation of palmitoyl-CoA and serine by serine palmitoyltransferase (SPT) produces dihydroceramide (dihydroCer) and Cer. Among milk polar lipids including sphingolipids, sphingomyelin (SM) is hydrolyzed by an alkaline sphingomyelinase to ceramide (Cer) and phosphocholine. Cer is further hydrolyzed by a neutral ceramidase into sphingosine (SPH) and FA. In the enterocyte, SPH is mainly converted to palmitic acid, which is incorporated into TG but may be reincorporated into Cer and complex sphingolipids. Intestine-derived lipoproteins, i.e., CM, the major triglyceride-rich lipoproteins (TGRL) and HDL, are released into the bloodstream via the lymph. As described in the present review, SFA consumption may lead to increased concentrations of Cer in TGRL (28) and HDL (33). Supplementation with milk polar lipids may result in decreased concentrations of SM and Cer in CM (27). Future research should study the endogenous metabolism of sphingolipid species in enterocytes. Some figure elements were obtained from Servier Medical Art (https://smart.servier.com/).
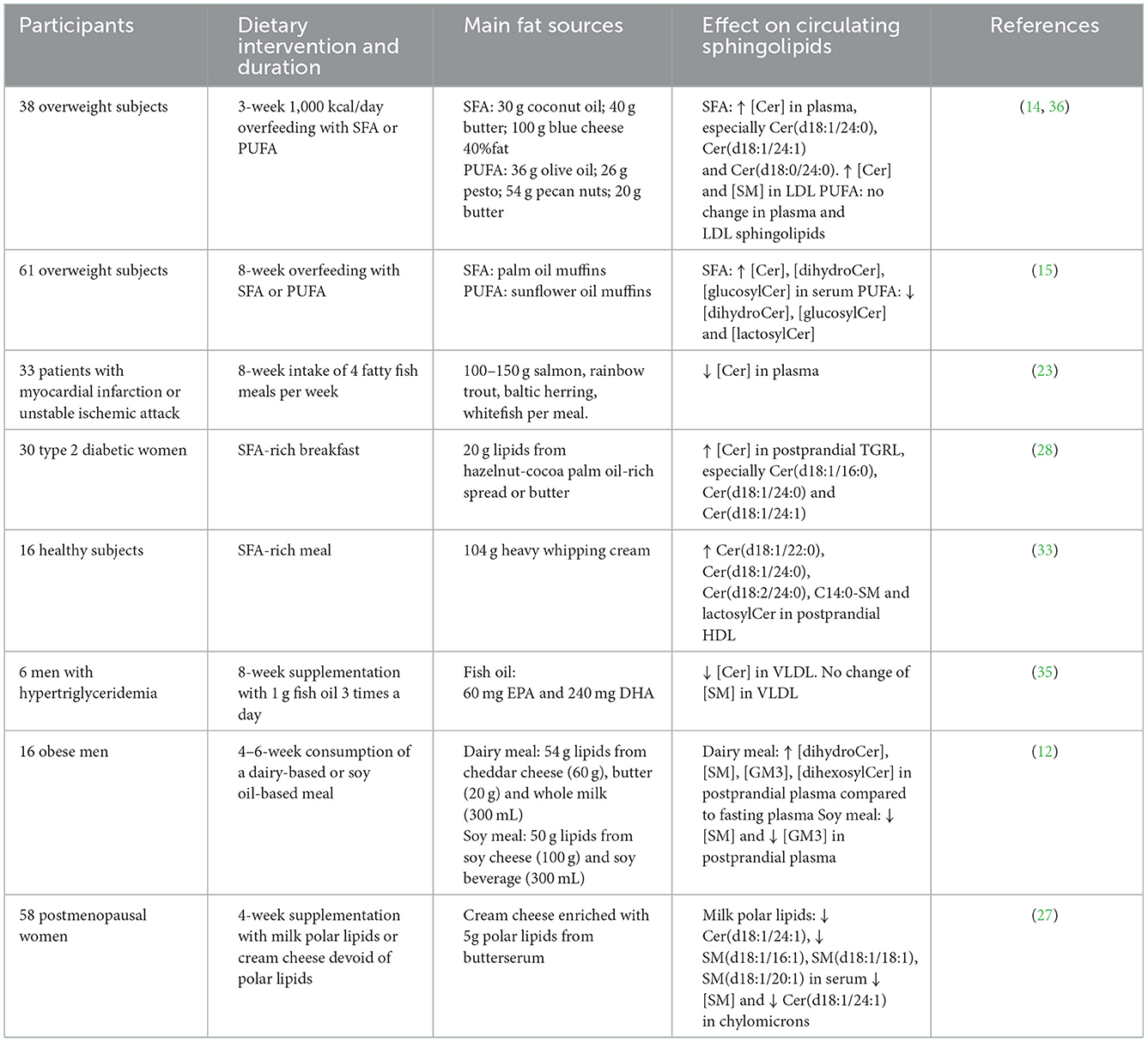
Table 1. Impact of dietary fatty acids and sphingomyelin on circulating sphingolipids in the randomized controlled trials mentioned in the text.
Finally, there is a need for additional randomized controlled trials in healthy subjects and patients at increased cardiovascular risk to support the beneficial impact of dairy products including polar lipids on circulating lipids, including sphingolipids, lipid metabolism and cardiometabolic health.
Author contributions
CC wrote the original draft of the document. CV, AP, DC, and M-CM critically revised the document. All authors have made a substantial and intellectual contribution to the work and approved the final version of the manuscript.
Funding
This work was funded by Inserm.
Conflict of interest
The authors declare that the research was conducted in the absence of any commercial or financial relationships that could be construed as a potential conflict of interest.
Publisher's note
All claims expressed in this article are solely those of the authors and do not necessarily represent those of their affiliated organizations, or those of the publisher, the editors and the reviewers. Any product that may be evaluated in this article, or claim that may be made by its manufacturer, is not guaranteed or endorsed by the publisher.
Abbreviations
Cer, ceramide; DHA, docosahexaenoic acid; dihydroCer, dihydroceramide; EPA, eicosapentaenoic acid; glucosylCer, glucosylceramides; HDL, high-density lipoproteins; LDL, low-density lipoproteins; PUFA, polyunsaturated fatty acids; SFA, saturated fatty acids; SM, sphingomyelins; TG, triglycerides; TGRL, triglyceride-rich lipoproteins; VLDL, very-low density lipoproteins.
References
1. Wu JHY, Micha R, Mozaffarian D. Dietary fats and cardiometabolic disease: mechanisms and effects on risk factors and outcomes. Nat Rev Cardiol. (2019) 16:581–601. doi: 10.1038/s41569-019-0206-1
2. Bansal S, Buring JE, Rifai N, Mora S, Sacks FM, Ridker PM. Fasting compared with nonfasting triglycerides and risk of cardiovascular events in women. JAMA. (2007) 298:309–16. doi: 10.1001/jama.298.3.309
3. Quehenberger O, Armando AM, Brown AH, Milne SB, Myers DS, Merrill AH, et al. Lipidomics reveals a remarkable diversity of lipids in human plasma. J Lipid Res. (2010) 51:3299–305. doi: 10.1194/jlr.M009449
4. Hammad SM, Pierce JS, Soodavar F, Smith KJ, Al Gadban MM, Rembiesa B, et al. Blood sphingolipidomics in healthy humans: impact of sample collection methodology. J Lipid Res. (2010) 51:3074–87. doi: 10.1194/jlr.D008532
5. Iqbal J, Walsh MT, Hammad SM, Hussain MM. Sphingolipids and Lipoproteins in Health and Metabolic Disorders. Trends Endocrinol Metab. (2017) 28:506–18. doi: 10.1016/j.tem.2017.03.005
6. Choi RH, Tatum SM, Symons JD, Summers SA, Holland WL. Ceramides and other sphingolipids as drivers of cardiovascular disease. Nat Rev Cardiol. (2021) 28:701–11. doi: 10.1038/s41569-021-00536-1
7. Truman JP, García-Barros M, Obeid LM. Evolving concepts in cancer therapy through targeting sphingolipid metabolism. Biochim Biophys Acta. (2014) 184:1174–88. doi: 10.1016/j.bbalip.2013.12.013
8. Wang DD, Toledo E, Hruby A, Willett WC, Sun Q, Razquin C, et al. Plasma ceramides, Mediterranean diet, and incident cardiovascular disease in the PREDIMED Trial (Prevención con Dieta Mediterránea). Circulation. (2017) 135:2028–40. doi: 10.1161/circ.135.suppl_1.p194
9. Lankinen M, Schwab U, Kolehmainen M, Paananen J, Nygren H, Seppänen-Laakso T, et al. A healthy Nordic diet alters the plasma lipidomic profile in adults with features of metabolic syndrome in a multicenter randomized dietary intervention. J Nutr. (2016) 146:662–72. doi: 10.3945/jn.115.220459
10. Fumeron F, Nicolas A, Bastard JP, Fellahi S, Wigger L, Ibberson M, et al. Dairy consumption is associated with lower plasma dihydroceramides in women from the DESIR cohort. Diabetes Metab. (2020) 46:144–9. doi: 10.1016/j.diabet.2019.06.002
11. Seah JYH, Chew WS, Torta F, Khoo CM, Wenk MR, Herr DR, et al. Dietary fat and protein intake in relation to plasma sphingolipids as determined by a large-scale lipidomic analysis. Metabolites. (2021) 11:93. doi: 10.3390/metabo11020093
12. Meikle PJ, Barlow CK, Mellett NA, Mundra PA, Bonham MP, Larsen A, et al. Postprandial plasma phospholipids in men are influenced by the source of dietary fat. J Nutr. (2015) 145:2012–8. doi: 10.3945/jn.115.210104
13. Meeusen JW, Donato LJ, Bryant SC, Baudhuin LM, Berger PB, Jaffe AS. Plasma ceramides. Arterioscler Thromb Vasc Biol. (2018) 38:1933–9. doi: 10.1161/ATVBAHA.118.311199
14. Luukkonen PK, Sädevirta S, Zhou Y, Kayser B, Ali A, Ahonen L, et al. Saturated fat is more metabolically harmful for the human liver than unsaturated fat or simple sugars. Diabetes Care. (2018) 41:1732–9. doi: 10.2337/dc18-0071
15. Rosqvist F, Kullberg J, Ståhlman M, Cedernaes J, Heurling K, Johansson HE, et al. Overeating saturated fat promotes fatty liver and ceramides compared with polyunsaturated fat: a randomized trial. J Clin Endocrinol Metab. (2019) 104:6207–19. doi: 10.1210/jc.2019-00160
16. Blachnio-Zabielska A, Baranowski M, Zabielski P, Gorski J. Effect of high fat diet enriched with unsaturated and diet rich in saturated fatty acids on sphingolipid metabolism in rat skeletal muscle. J Cell Physiol. (2010) 225:786–91. doi: 10.1002/jcp.22283
17. Hu Y, Hu FB, Manson JE. Marine omega-3 supplementation and cardiovascular disease: an updated meta-analysis of 13 randomized controlled trials involving 127 477 participants. J Am Heart Assoc. (2019) 8:e013543. doi: 10.1161/JAHA.119.013543
18. Siscovick DS, Barringer TA, Fretts AM, Wu JH, Lichtenstein AH, Costello RB, et al. Omega-3 polyunsaturated fatty acid (fish oil) supplementation and the prevention of clinical cardiovascular disease: a science advisory from the American heart association. Circulation. (2017) 135:e867–84. doi: 10.1161/CIR.0000000000000482
19. Park Y, Harris WS. Dose-response of n-3 polyunsaturated fatty acids on lipid profile and tolerability in mildly hypertriglyceridemic subjects. J Med Food. (2009) 12:803–8. doi: 10.1089/jmf.2008.1250
20. Shearer GC, Savinova OV, Harris WS. Fish oil - how does it reduce plasma triglycerides? Biochim Biophys Acta. (2012) 1821:843–51. doi: 10.1016/j.bbalip.2011.10.011
21. Walchuk C, Wang Y, Suh M. The impact of EPA and DHA on ceramide lipotoxicity in the metabolic syndrome. Br J Nutr. (2021) 125:863–75. doi: 10.1017/S0007114520003177
22. Kasbi-Chadli F, Ferchaud-Roucher V, Krempf M, Ouguerram K. Direct and maternal n-3 long-chain polyunsaturated fatty acid supplementation improved triglyceridemia and glycemia through the regulation of hepatic and muscle sphingolipid synthesis in offspring hamsters fed a high-fat diet. Eur J Nutr. (2016) 55:589–99. doi: 10.1007/s00394-015-0879-0
23. Lankinen M, Schwab U, Erkkilä A, Seppänen-Laakso T, Hannila ML, Mussalo H, et al. Fatty fish intake decreases lipids related to inflammation and insulin signaling - a lipidomics approach. PLoS ONE. (2009) 4:e5258. doi: 10.1371/journal.pone.0005258
24. Wiesner P, Leidl K, Boettcher A, Schmitz G, Liebisch G. Lipid profiling of FPLC-separated lipoprotein fractions by electrospray ionization tandem mass spectrometry. J Lipid Res. (2009) 50:574–85. doi: 10.1194/jlr.D800028-JLR200
25. Scherer M, Bottcher A, Schmitz G, Liebisch G. Sphingolipid profiling of human plasma and FPLC-separated lipoprotein fractions by hydrophilic interaction chromatography tandem mass spectrometry. Biochim Biophys Acta. (2011) 1811:68–75. doi: 10.1016/j.bbalip.2010.11.003
26. Le Barz M, Boulet MM, Calzada C, Cheillan D, Michalski MC. Alterations of endogenous sphingolipid metabolism in cardiometabolic diseases: Towards novel therapeutic approaches. Biochimie. (2020) 169:133–43. doi: 10.1016/j.biochi.2019.10.003
27. Le Barz M, Vors C, Combe E, Joumard-Cubizolles L, Lecomte M, Joffre F, et al. Milk polar lipids favorably alter circulating and intestinal ceramide and sphingomyelin species in postmenopausal women. JCI Insight. (2021) 6:e146161. doi: 10.1172/jci.insight.146161
28. Boulet MM, Calzada C, Pettazzoni M, Lelekov-Boissard T, Buisson C, Di Filippo M, et al. A meal rich in palm oil or butter modifies the sphingolipid profile of postprandial triglyceride-rich lipoproteins from type 2 diabetic women. Biochimie. (2022) 203:11–9. doi: 10.1016/j.biochi.2022.07.003
29. Björnson E, Packard CJ, Adiels M, Andersson L, Matikainen N, Söderlund S, et al. Apolipoprotein B48 metabolism in chylomicrons and very low-density lipoproteins and its role in triglyceride transport in normo- and hypertriglyceridemic human subjects. J Intern Med. (2020) 288:422–38. doi: 10.1111/joim.13017
30. Brunham LR, Kruit JK, Iqbal J, Fievet C, Timmins JM, Pape TD, et al. Intestinal ABCA1 directly contributes to HDL biogenesis in vivo. J Clin Invest. (2006) 116:1052–62. doi: 10.1172/JCI27352
31. Chétiveaux M, Croyal M, Ouguerram K, Fall F, Flet L, Zair Y et al. Effect of fasting and feeding on apolipoprotein A-I kinetics in preβ1- HDL, α-HDL, and triglyceride-rich lipoproteins. Sci Rep. (2020) 10:15585. doi: 10.1038/s41598-020-72323-w
32. Boulet MM, Cheillan D, Di Filippo M, Lelekov-Boissard T, Buisson C, Lambert-Porcheron S et al. Postprandial triglyceride-rich lipoproteins from type 2 diabetic women stimulate platelet activation regardless of the fat source in the meal. Mol Nutr Food Res. (2020) 64:e2000694. doi: 10.1002/mnfr.202000694
33. Averill M, Rubinow KB, Cain K, Wimberger J, Babenko I, Becker JO, et al. Postprandial remodeling of high-density lipoprotein following high saturated fat and high carbohydrate meals. J Clin Lipidol. (2020) 14:66–76.e11. doi: 10.1016/j.jacl.2019.11.002
34. Camont L, Lhomme M, Rached F, Le Goff W, Nègre-Salvayre A, Salvayre R, et al. Small, dense high-density lipoprotein-3 particles are enriched in negatively charged phospholipids: relevance to cellular cholesterol efflux, antioxidative, antithrombotic, anti-inflammatory, and antiapoptotic functionalities. Arterioscler Thromb Vasc Biol. (2013) 33:2715–23. doi: 10.1161/ATVBAHA.113.301468
35. Ferchaud-Roucher V, Zair Y, Aguesse A, Krempf M, Ouguerram K. Omega 3 improves both apoB100-containing lipoprotein turnover and their sphingolipid profile in hypertriglyceridemia. J Clin Endocrinol Metab. (2020) 105:dgaa459. doi: 10.1210/clinem/dgaa459
36. Ruuth M, Lahelma M, Luukkonen PK, Lorey MB, Qadri S, Sädevirta S, et al. Overfeeding saturated fat increases LDL (Low-Density Lipoprotein) aggregation susceptibility while overfeeding unsaturated fat decreases proteoglycan-binding of lipoproteins. Arterioscler Thromb Vasc Biol. (2021) 41:2823–36. doi: 10.1161/ATVBAHA.120.315766
37. Vesper H, Schmelz EM, Nikolova-Karakashian MN, Dillehay DL, Lynch DV, Merrill AH Jr. Sphingolipids in food and the emerging importance of sphingolipids to nutrition. J Nutr. (1999) 129:1239–50. doi: 10.1093/jn/129.7.1239
38. Yunoki K, Ogawa T, Ono J, Miyashita R, Aida K, Oda Y, et al. Analysis of sphingolipid classes and their contents in meals. Biosci Biotechnol Biochem. (2008) 72:222–5. doi: 10.1271/bbb.70463
39. Contarini G, Povolo M. Phospholipids in milk fat: composition, biological and technological significance, and analytical strategies. Int J Mol Sci. (2013) 14:2808–31. doi: 10.3390/ijms14022808
40. Burling H, Graverholt G. Milk - a new source for bioactive phospholipids for use in food formulations. Lipid Technol. (2008) 20:229–31. doi: 10.1002/lite.200800058
41. Nilsson A. Metabolism of sphingomyelin in the intestinal tract of the rat. Biochim Biophys Acta. (1968) 164:575–84. doi: 10.1016/0005-2760(68)90187-2
42. Nilsson A, Duan RD. Absorption and lipoprotein transport of sphingomyelin. J Lipid Res. (2006) 47:154–71. doi: 10.1194/jlr.M500357-JLR200
43. Norris GH, Milard M, Michalski MC, Blesso CN. Protective properties of milk sphingomyelin against dysfunctional lipid metabolism, gut dysbiosis, and inflammation. J Nutr Biochem. (2019) 73:108224. doi: 10.1016/j.jnutbio.2019.108224
44. Ohlsson L, Burling H, Nilsson A. Long term effects on human plasma lipoproteins of a formulation enriched in butter milk polar lipid. Lipids Health Dis. (2009) 8:44. doi: 10.1186/1476-511X-8-44
45. Conway V, Couture P, Richard C, Gauthier SF, Pouliot Y, Lamarche B. Impact of buttermilk consumption on plasma lipids and surrogate markers of cholesterol homeostasis in men and women. Nutr Metab Cardiovasc Dis. (2013) 23:1255–62. doi: 10.1016/j.numecd.2013.03.003
46. Nordestgaard BG, Benn M, Schnohr P, Tybjaerg-Hansen A. Nonfasting triglycerides and risk of myocardial infarction, ischemic heart disease, and death in men and women. JAMA. (2007) 298:299–308. doi: 10.1001/jama.298.3.299
47. Ohlsson L, Burling H, Duan RD, Nilsson A. Effects of a sphingolipid-enriched dairy formulation on postprandial lipid concentrations. Eur J Clin Nutr. (2010) 64:1344–9. doi: 10.1038/ejcn.2010.164
48. Vors C, Joumard-Cubizolles L, Lecomte M, Combe E, Ouchchane L, Drai J, et al. Milk polar lipids reduce lipid cardiovascular risk factors in overweight postmenopausal women: towards a gut sphingomyelin-cholesterol interplay. Gut. (2020) 69:487–501. doi: 10.1136/gutjnl-2018-318155
Keywords: sphingolipids, ceramide, sphingomyelin, dietary lipids, nutrition, postprandial metabolism
Citation: Calzada C, Vors C, Penhoat A, Cheillan D and Michalski M-C (2023) Role of circulating sphingolipids in lipid metabolism: Why dietary lipids matter. Front. Nutr. 9:1108098. doi: 10.3389/fnut.2022.1108098
Received: 25 November 2022; Accepted: 21 December 2022;
Published: 11 January 2023.
Edited by:
Christopher Blesso, University of Connecticut, United StatesReviewed by:
Guoxun Chen, The University of Tennessee, United StatesCopyright © 2023 Calzada, Vors, Penhoat, Cheillan and Michalski. This is an open-access article distributed under the terms of the Creative Commons Attribution License (CC BY). The use, distribution or reproduction in other forums is permitted, provided the original author(s) and the copyright owner(s) are credited and that the original publication in this journal is cited, in accordance with accepted academic practice. No use, distribution or reproduction is permitted which does not comply with these terms.
*Correspondence: Catherine Calzada, Y2F0aGVyaW5lLmNhbHphZGEmI3gwMDA0MDt1bml2LWx5b24xLmZy