- 1Faculty of Life Science and Technology, Kunming University of Science and Technology, Kunming, Yunnan, China
- 2Yunnan Provincial Key Laboratory of Panax Notoginseng, Kunming, China
- 3China Academy of Chinese Medical Sciences, Beijing, China
- 4Resource Institute for Chinese and Ethnic Materia Medica, Guizhou University of Traditional Chinese Medicine, Guiyang, China
The sulfur dioxide gas (SO2) generated by sulfur burning can improve the appearance quality of food and enhance the storage time. However, excessive sulfur dioxide will pollute the environment and cause deterioration of food quality, and even the high residual levels can increase the risk of cancer. As Gastrodia elata Blume is prone to corruption during processing, sulfur fumigation is often used for preservation. In this study, spectral analysis and Texture Profile Analysis (TPA) were used to investigate the effects of traditional sulfur fumigation processing on the morphology quality, edible quality and structural characteristics of G. elata. The results showed that compared with direct drying, the pH decreased by 0.399 of the sulfur fumigated after steamed treatment G. elata, and the morphology quality, pasting ability and gel edible quality of the starch were significantly improved. In addition, it was suggested that sulfur fumigation after steaming could promote the release of molecular chains from starch granules and thus enhance the cross-linking between molecules, which explained the reason for the improve of starch edible quality. This study can provide technical and theoretical support for improving the quality of starch rich foods, replacing sulfur fumigation and reducing potential environmental hazards.
1. Introduction
Sulfur fumigation is often used for food mildew prevention and storage. However, SO2 and its derivatives generated in the process of sulfur fumigation are the main pollutants in the atmosphere that are harmful to the environment (1). At the same time, excessive SO2 residues in food have carcinogenic effects on human health. Therefore, to maintain the environment and consumer health, the residual amount of SO2 in food processing is limited strictly (1). The Chinese government stipulates that the SO2 content in food shall not exceed 100 mg/kg (2), and the sulfur-fumigated Chinese herbal medicine should be less than 400 mg/kg (3).
Gastrodia elata Blume (G. elata) is the rhizome of the genus Gastrodia belonging to family Orchidaceae. It has the function of relieving wind and spasm, calming liver Yang, dispelling wind and dredging collaterals. It is traditionally used as treatment for manage convulsions in children, epilepsy, tetanus, headache and dizziness, hand and foot failure, numbness of limbs, rheumatism, and arthralgia (4). G. elata is a kind of traditional medicine and food widely used in many Asian countries (5). In 2002, G. elata was included in the list of items potentially used as health food by the Chinese government, representing a “health food” in the legal sense. In 2019, G. elata was designated by the Chinese Health Commission as a pilot variety for the management of “substances that are both food and Chinese herbal medicines according to tradition” and represented a “medicine-food homology” in the legal sense. With G. elata becoming a food of legal significance, its edible range and usage are also expanding rapidly.
The common drying processes of G. elata are sun drying, hot air drying and vacuum freeze drying. Sun drying efficiency is low, but the drying cost is also low. Vacuum freeze-drying is the best method to maintain the appearance and active ingredients, but it requires expensive equipment and high drying costs. However, due to the conditions of the production base and the processing cost, hot air drying is the most commonly used processing technology. G. elata is rich in starch and sugar, with levels as high as 12.5 and 8%, respectively. Nonetheless, the water content of fresh G. elata is as high as 75%, with an average fresh weight of more than 100 g, which decreases the rate of water loss during the drying process and increases bacterial and fungal contamination and rotting because of the high levels of sugars and starch (6).
Sulfur fumigation can prevent food decay caused by bacterial and fungal pollution and can improve the appearance quality of food, such as copra and yam (7). In addition, the presteaming treatment can avoid the composition change of fresh food due to its own metabolic reaction. However, excessive sulfur fumigation will cause sulfur dioxide residue, which is harmful to human health. The sulfur fumigation process is a traditional Chinese method for processing G. elata, which can prevent enzymatic browning caused by the decomposition of gastrodin and phenolic substances by enzymes during the processing of G. elata, and the SO2 produced by sulfur combustion can increase the color while preventing mildew (4).
High-quality G. elata is characterized by strong waxiness and a high degree of sweetness. The hardness and viscoelasticity of foods with high starch content are mainly affected by starch composition and structure. For example, the amylose content and the molecular chain size are positively correlated with the hardness of rice, while the content of amylopectin and short-chain starch is positively correlated with the viscosity of rice (8). Long amylopectin chains (DP > 35) were positively correlated with the degree of swelling (9). In addition, the cooking characteristics of food are also related to the composition and structure of starch, such as the higher disintegration temperature of starch crystals, which prolongs the cooking time of rice (10). Therefore, dry heat treatment, acetic acid acetylation and hydrochloric acid hydrolysis are used to modify starch to increase its edible quality (11–13). Jyothi et al. (14) soaked cassava starch with 2% sodium metabisulfite and found that the starch viscosity first increased and then decreased with the extension of soaking time, which increased the storage time and solubility of cassava starch. Sodium metabisulfite will dissociate into sulfurous acid in aqueous solution, and the SO2 from sulfur combustion products combines with water to generate sulfurous acid, thus changing the structure of starch (15).
To preserve the nutritional ingredients in food, fresh food is usually presteamed and then dried for preservation. However, this high temperature condition will change the composition and structural characteristics of starch. For example, the amylose content of corn and potato increases after steaming, and the crystal structure of lily starch changes from type B to type A after heat treatment (16, 17). The previous research of this study shows that the taste of G. elata fumigated with sulfur in the traditional way was significantly increased in terms of chewiness, but the mechanism is still unclear. Zhuowen Chen et al. (15) have shown that the proportion of amylose in yam after sulfur fumigation is higher than that without sulfur fumigation. The starch content of G. elata is similar to that of yam and cassava, accounting for approximately 50% of its dry weight. We infer that this may be because sulfur fumigation leads to the breaking of amylopectin and the formation of short amylose, which increases the cross-linking degree between starch molecules, increases the hardness of starch gel, and thus increases the chewiness of G. elata. However, the inference and the mechanism of action need to be further studied.
To explain the scientific connotation of the influence of traditional processing technology of sulfur fumigation after steaming on the color, viscosity and chewiness quality of G. elata starch, this study explored the influence of sulfur fumigation after steaming on the crystallinity and viscoelasticity of starch during processing and revealed the relationship between the edible quality of starch and the change in starch structure. This study can provide data support for the scientific nature of traditional processing technology of G. elata and provide a theoretical basis for the rational use of sulfur fumigation in food and the control of potential environmental hazards.
2. Materials and methods
2.1. Materials
Fresh G. elata Bl. f. glauca S. Chow (Grade II, 2 years old, weighing 100–200 g per G. elata, with 75% water content) was collected in December 2021 from Xiaocaoba Town, Zhaotong, Yunnan, China (104° 15′E, 27° 46′ N), for use in this study. Sulfur was purchased from Sinopharm Chemical Reagent Co., Ltd., (Shanghai, China, No. 80120528). The steaming equipment is an electric cooker (ZG500, Medical Equipment Factory of Boxun Industrial Co., Ltd., Shanghai, China). All chemical reagents used in this study were of analytical grade.
2.2. Steaming and sulfur fumigation of G. elata
After the fresh G. elata was cleaned, it was divided into steam treatment (St) and nonsteam treatment (NSt). In steam treatment, G. elata is steamed in a steamer for 30 min. After drying the surface moisture, G. elata was removed for sulfur fumigation treatment (Sf) and nonsulfur fumigation treatment (NSf) from the St and NSt treatments. The Sf treatment according to the ratio of G. elata:sulfur (20:1) and placed in a fumigation box (60 cm × 45 cm × 35 cm). After the sulfur was ignited, the fumigation box was capped, and a small hole of approximately 1 cm2 was left for ventilation. After fumigation for 90 min, it was removed. All G. elata were placed into the electric constant temperature blast drying oven (DGG-9123AD, Senxin Experimental Instrument Co., Ltd., Shanghai, China) with 50°C hot air and dried for 5 days, and the final water content was approximately 5%. After drying, the G. elata was powdered and passed through the 200 mesh sieve. The starch was extracted following the method of Jiang et al. (18).
2.3. Determination of the pH and sulfur dioxide residue analysis of G. elata powder
The pH of G. elata powder was determined following the method of Liu et al. (19) with a slight modification. Take 0.8 g of G. elata powder, add 20 mL of distilled water for cold immersion for 12 h, then extract it by ultrasonic for 30 min, and measure it with a pH meter (STARTER-3100, Aohaosi Instrument Co., Ltd., Shanghai, China). The sulfur dioxide residue was determined following the method of Kang et al. (4).
2.4. Determination of chromaticity, solubility, swelling power, amylose content, and paste transparency of G. elata starch
The chromaticity is measured with a colorimeter (SC-10, Sanenchi Technology Co., Ltd., Shenzhen, China). Solubility and swelling power were determined following the method of Lu et al. (20) with a slight modification. Take 0.5 g sample powder, add 25 mL distilled water, mix well, shake and paste it in boiling water bath for 30 min, then cool it and centrifuge it at 4,000 r/min for 25 min, take the supernatant, dry it to constant weight and weigh it.
where M1 is the starch mass in the supernatant after drying, M2 is the sample mass, M3 is the sediment mass after centrifugation, S is the sample solubility.
Amylose content following the method of Uarrota et al. (21) with a slight modification. Take 50 mg of G. elata starch, mix it with 5 mL of 0.5 mol/L KOH solution, dilute it 10-fold, take 1 mL of solution, adjust the pH to 3.5 with 0.1 mol/L HCl, add 0.1 mL iodine reagent (20 mg KI–2 mg I/mL, AR), and measure the absorbance at 625 nm. Calculate the content according to the following standard curve:
Use the mixed solution with amylose content (0, 20, 40, 60, and 80%) to measure the absorbance at 625 nm to obtain the standard curve. Where Y is the amylose content (%) in the mixed standard solution, and X is the absorbance measured at 625 nm.
Paste transparency was determined following the method of Correia et al. (22). G. elata starch was stirred in a boiling water bath for 1 h to prepare a 2% starch suspension. After cooling at 25°C, the transmittance was measured at 620 nm.
2.5. Texture characteristics of G. elata starch gel (TPA)
Texture characteristics were determined following the method of Lu et al. (20) with a slight modification. Mix G. elata starch and water to prepare a 10% aqueous starch solution, shake and mix in a boiling water bath for 30 min. Then, the sample was refrigerated for 12 h at 4°C. After the formation of the gel, it was removed and restored to room temperature. Texture analyzer (TA. XT plus, London, UK) was used for measurement, and P/0.5 was used as the probe. The parameters were set as follows: the test speed was 1 mm/s, the test distance was 13 mm, and the trigger force was 2 g. Hardness, adhesiveness, springiness, cohesiveness, gumminess, chewiness, and resilience were recorded.
2.6. Fourier transform infrared spectroscopy
Following the method of Lu et al. (20), the FT-IR spectra of the samples were determined using FT-IR spectroscopy (Sensor 27, Bruker Inc., Saarbrucken, Germany). Mix and grind G. elata starch and dried KBr and scan them in the wavenumber range of 500–4,000 cm–1 at room temperature after tablet pressing.
2.7. X-ray diffraction
Following the method of Lu et al. (20) with a slight modification to determine the crystalline structure of starch by XRD (D8 Advance, Bruker Inc., Saarbrucken, Germany). The parameters were set as follows: the diffraction angle was 2 θ, with a range of 4°∼70°, and the scanning rate was 4°/min. The relative crystallinity was calculated using JADE 6.5 (Java Agent Development Framework) software.
2.8. Measurement of paste properties with a rapid viscosity analyzer
Following the method of Li et al. (17) with a slight modification to determine the adhesive properties of G. elata starch by rapid viscosity analyzer (RVA-Taskmaster, Perton Inc., Stockholm, Sweden). Dissolve 1.25 g of G. elata starch in 25 mL of water, stir for 30 min, mix well, and measure it according to the method of rice configuration file in RVA.
2.9. Thermogravimetric analysis
Following the method of Ma et al. (23), the thermogravimetric properties of G. elata starch were determined by STA (STA-2500, NETZSCH Inc., Selb, Germany). The test temperature was 30°C–600°C, nitrogen was the ambient gas, and the rising temperature was 10°C/min.
2.10. Rheological measurement
The rheological properties of the samples were determined using a rheometer (DHR-2, TA Instruments Inc., New Castle, DE, USA). The test was conducted at 25°C using 40 mm parallel plates. Take 0.25 g of starch to prepare a 5% starch suspension (mix it evenly in boiling water for 10 min and cool it to room temperature), then transfer it to a rheological plate and balance it for 3 min. According to the method of Lu et al. (20), frequency scanning was carried out at 1% strain in the angular frequency range of 0.1–100 rad/s. The shear viscosity was measured between 0.1 and 100 s–1. Data were fitted by the power law equation:
where σ is the shear stress (Pa), K is the consistency coefficient (Pa⋅s–1), γ is the shear rate (s–1), and n is the non-Newtonian exponent.
2.11. Molecular weight analysis with high-performance gel permeation chromatography
Accurately weigh the starch sample and dextran standards (Sigma-Aldrich Co., Ltd., Darmstadt, Germany) to prepare 5 mg/mL in 0.2 M NaOH solution, hydrolyzed at 120°C for 1 h, centrifuged at 12,000 rpm for 10 min, and a 0.22 μm microporous filter membrane was used, and then the sample was transferred into a 1.8 mL injection vial. 0.05 M NaCl solution was used as the mobile phase for analysis at a flow rate of 0.6 mL/min. BRT105-104-102 series gel column (8 × 300 mm), column temperature: 40°C, injection volume: 20 μL, detector: differential detector RI-10A. A standard curve was drawn with the retention time (RT) of dextran standards on the X-axis and lgMw and lgMn on the Y-axis.
lgMw-RT of the correction curve equation:
lgMn-RT of the correction curve equation:
In (1), Y is lgMw, and X is the retention time (RT).
In (2), Y is lgMn, and X is the retention time (RT).
2.12. In vitro digestion characteristics of starch
Following the method of Zeng et al. (24). Add 7.5 mL of acetic acid buffer (0.2 mol/L, pH 5.2) to 100 mg of sample, keep stirring in boiling water bath, cool to room temperature, add 5 mL of enzyme solution (each mL contains 294 U of porcine pancreatic amylase and 15 U of glucoamylase), shake and mix at 37°C for enzymatic hydrolysis, take 0.5 mL of sample every 20 min, and immediately add 4 mL of absolute ethanol to terminate the reaction. Monitor continuously for 4 h. The glucose content of the sample to be tested was determined by the Ghose method. Different starch contents were calculated according to the following formula: rapidly digesting starch (RDS, digestion within 20 min), slowly digesting starch (SDS, digestion within 20–120 min), and resistant starch (RS, residual starch after digestion for 120 min).
G20 is the glucose content in the solution at 20 min of enzymatic hydrolysis, G120 is the glucose content in the solution at 120 min of enzymatic hydrolysis, FG is the free sugar content in the starch solution at 0 min, TS is the total mass of the starch sample used, Y is the glucose content (mg), and X is the absorbance measured at 540 nm.
2.13. Scanning electron microscopy
The microstructure of the starch powder was observed with SEM (S-3400 N, Hitachi Inc., Tokyo, Japan), and the sample powder was fixed on the sample rack and placed in the instrument for observation.
2.14. Atomic force microscopy
AFM (5500LS, Agilent Inc., Palo Alto, CA, USA) was used to observe the microscopic morphology of the starch paste. One hundred milligrams of the sample was added to 20 mL of distilled water, stirred and mixed in a boiling water bath, and the paste was continued for 10 min and diluted with hot water to 50 μg/mL. The sample was placed on the mica surface and observed after drying.
2.15. Confocal laser scanning microscopy
Following the method of Ma et al. with a slight modification (23). Samples of 0.5 g starch mixed with 9.5 mL deionized water were stirred for 20 min. Then, they were stirred and mixed in a boiling water bath for 10 min. Wet starch samples (30 μL) were then stained by fluorescein isothiocyanate (FITC, 0.2 mg/mL, 30 μL). Images were collected using CLSM (TI-E-A1R, NIKON Inc., Tokyo, Japan) with an excitation wavelength of 488 nm and an emission wavelength of 450–540 nm.
2.16. Statistical analysis
All experiments were repeated at least in triplicate. Data were analyzed using SPSS 26.0 (SPSS Inc., Chicago, IL, USA) for statistical significance (P < 0.05) under Tukey’s test. All values are expressed as the mean ± standard deviation. Drawing was performed with Prism 9.0 (GraphPad Inc., San Diego, CA, USA).
3. Results
3.1. Effect of steaming and sulfur fumigation on the sulfur dioxide residue content and pH of G. elata
No residual sulfur dioxide was detected in the G. elata with NSt-NSf and St-NSf treatment, but the residual sulfur dioxide in the G. elata with sulfur fumigation treatment (NSt-Sf and St-Sf) was 223.67 and 356.67 mg/kg, respectively, which indicate that the samples had been successfully pretreated by sulfur fumigation. The sulfur dioxide residue of G. elata with St Sf treatment was 1.59-fold that with NSt-Sf treatment, which indicates that the steamed G. elata more easily absorbed sulfur dioxide than the nonsteamed G. elata (Figure 1A).
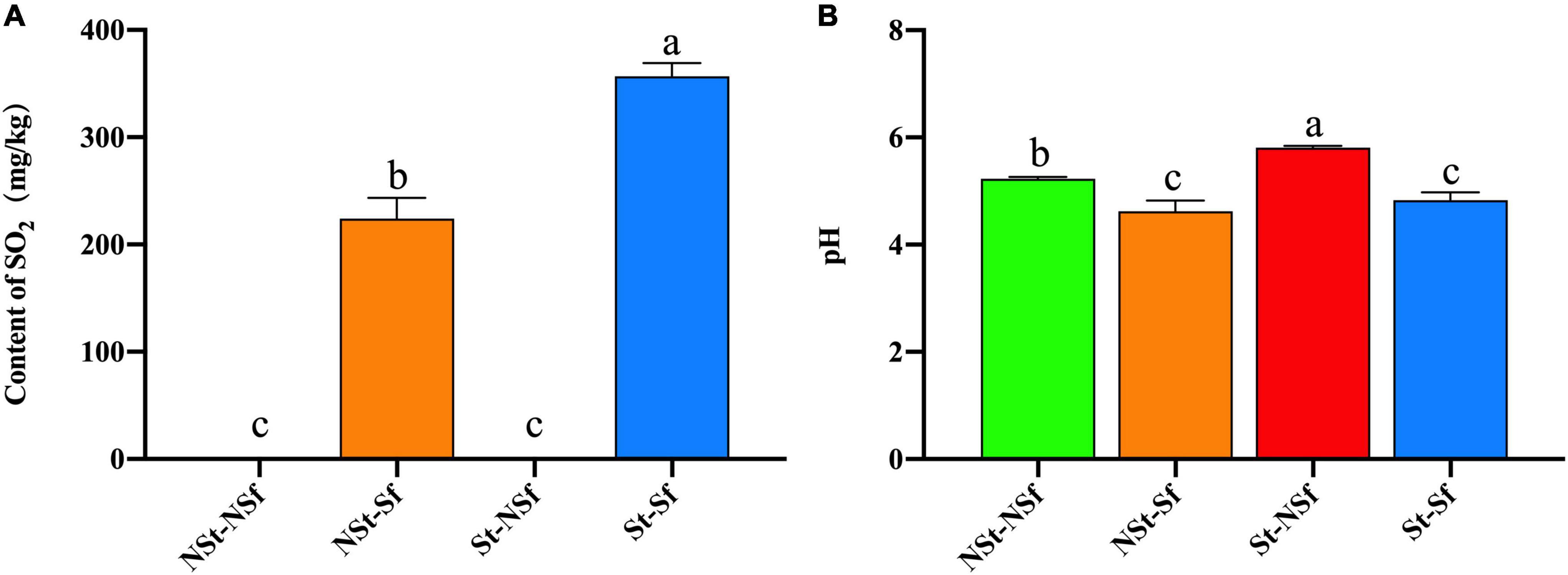
Figure 1. Residual sulfur dioxide content after processing of Gastrodia elata (A), Effect of steaming and sulfur fumigation on the pH of G. elata powder (B). NSt-NSf, nonsteam and nonsulfur fumigation treatment; NSt-Sf, nonsteam and sulfur fumigation treatment; St-NSf, steam and nonsulfur fumigation treatment; St-Sf, steam and sulfur fumigation treatment.
The G. elata powder pH of the St-NSf and St-Sf treatments was 0.58 and 0.21 higher than that of the NSt-NSf and NSt-Sf treatments, respectively. The SO2 produced by sulfur fumigation dissolved in water and formed H2SO3, resulting in the pH of G. elata reduced. NSt-Sf and St-Sf treatments reduced the pH of G. elata powder lower than NSt-NSf and St-NSf treatment by 0.61 and 0.98, respectively. However, sulfur fumigation had no significant effect on the pH of G. elata regardless of steaming treatment (Figure 1B).
3.2. Effects of steaming and sulfur fumigation on paste transparency and amylose content of G. elata starch
After St treatment, the transparency of G. elata starch paste was significantly higher than that of NSt. Compared with the NSt-NSf and NSt-Sf treatments, the paste transparency after the St-NSf and St-Sf treatments increased by 1.05 and 1.03-fold, respectively. When fresh G. elata was directly sulfur fumigation treated without steaming, the paste transparency of starch was also significantly increased. The effect of the NSt-Sf treatment was 1.01-fold higher than that of the NSt-NSf treatment. Sulfur fumigation had no significant effect on the starch paste transparency of steamed G. elata, and there was no significant difference in the starch paste transparency between the St-NSf and St-Sf treatments (Figure 2A).
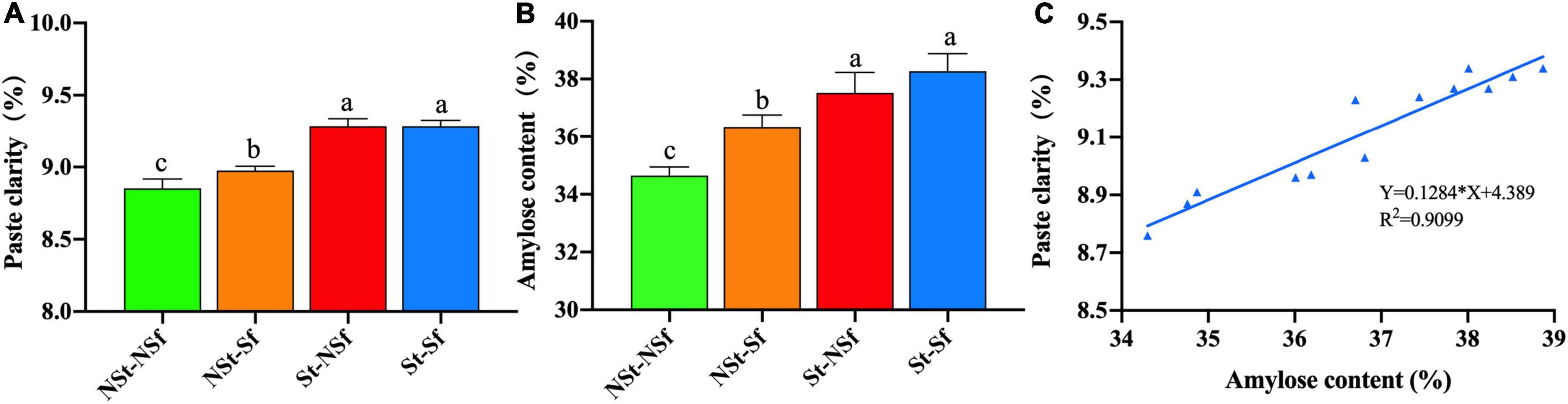
Figure 2. Effects of steaming and sulfur fumigation on paste transparency (A), amylose content (B), and the correlation between paste transparency and amylose content (C) of Gastrodia elata starch.
After St treatment, the amylose content of G. elata was significantly higher than that of NSt treatment. Compared with NSt-NSf and NSt-Sf treatments, the amylose content after St-NSf and St-Sf treatments was increased by 1.08 and 1.05-fold, respectively. The amylose content of G. elata subjected to NSt-Sf and St-Sf treatment was 1.05 and 1.02-fold higher than that of NSt-NSf and St-NSf treatment without sulfur fumigation, respectively. In the NSt treatment, Sf treatment significantly increased the amylose content. After St treatment, Sf treatment had no significant effect on the amylose content of G. elata (Figure 2B). The paste transparency of G. elata starch increased with increasing amylose content, resulting in a very significant positive correlation (Figure 2C, P < 0.0001).
3.3. Effects of steaming and sulfur fumigation treatment on the color of G. elata and starch
After St treatment of fresh G. elata, the whiteness of starch was significantly increased compared with that of NSt treatment (Figure 3B). The starch whiteness of St-NSf and St-Sf were significantly increased by 1.10 and 1.03-fold, respectively, compared with NSt-NSf and NSt-Sf. Sf treatment also significantly increased the whiteness of G. elata starch. Compared with the NSt-NSf and St-NSf treatments, the whiteness of G. elata starch after the NSt-NSf and St-NSf treatments increased by 1.11 and 1.04-fold, respectively (Figure 3, Table 1).
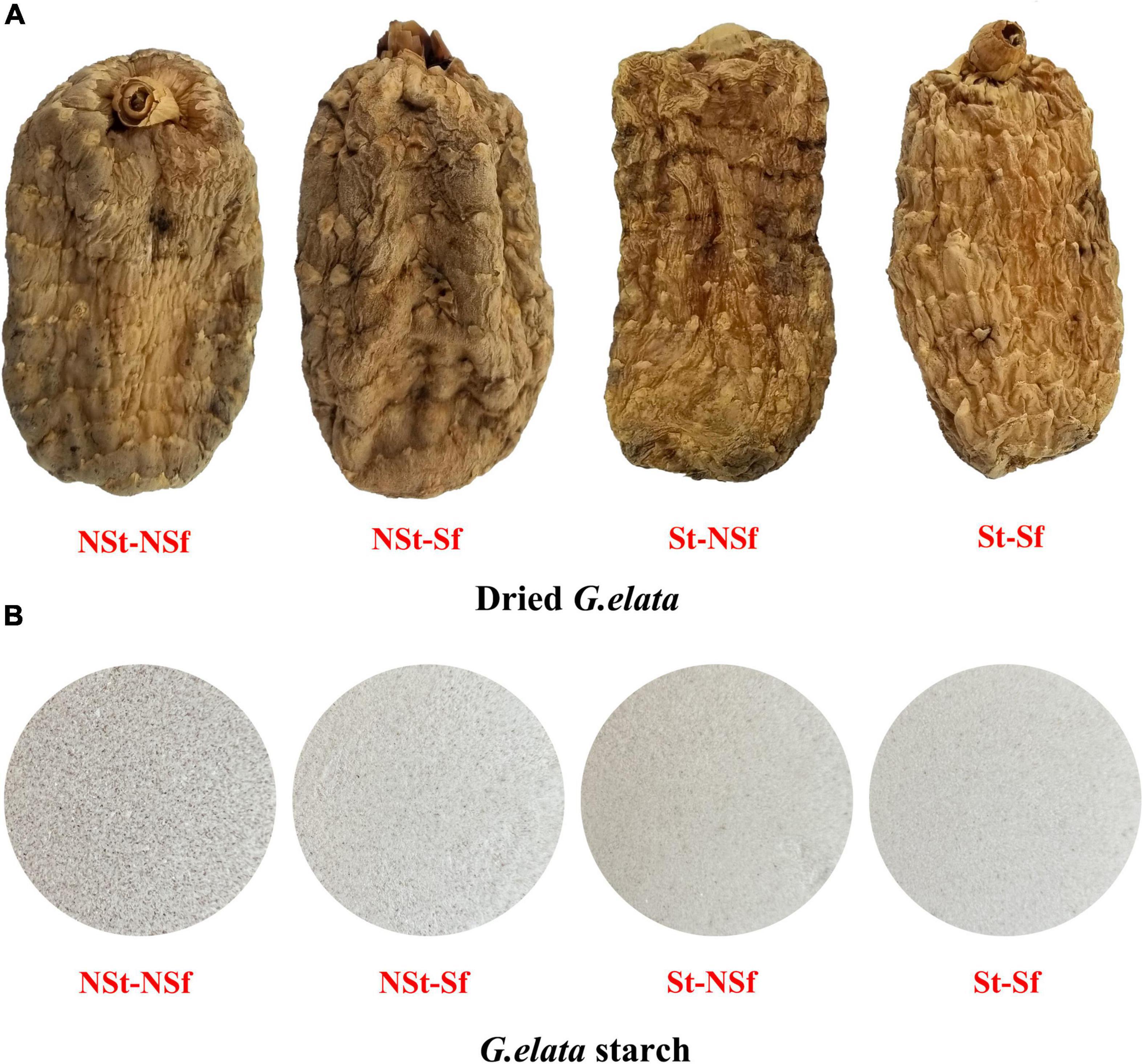
Figure 3. Effects of steaming and sulfur fumigation treatment on the morphology of Gastrodia elata and starch (A,B).
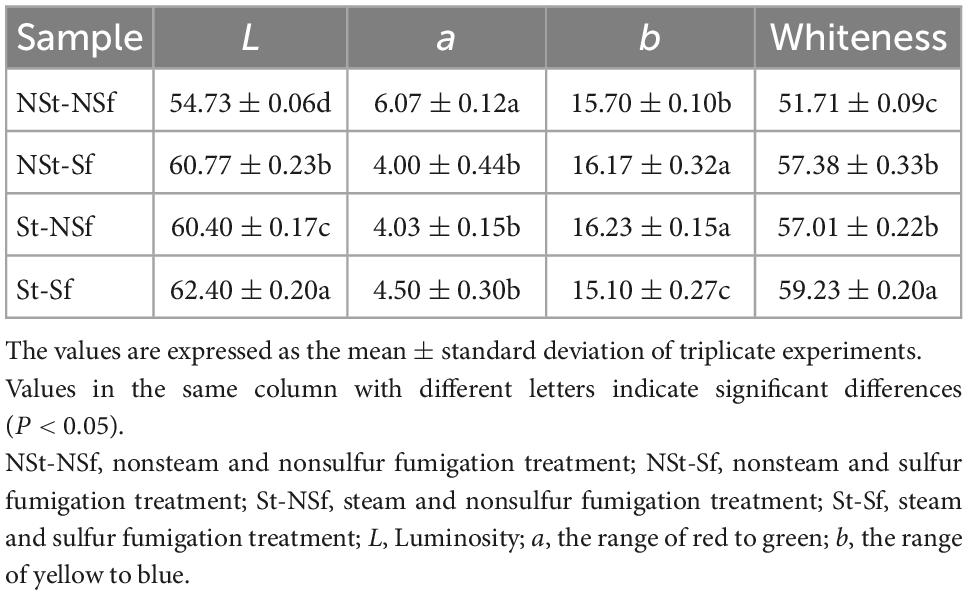
Table 1. Effect of steaming and sulfur fumigation treatment on the whiteness of Gastrodia elata starch.
3.4. Effect of steaming and sulfur fumigation on the solubility and swelling power of G. elata starch
After St treatment, the starch solubility of G. elata was significantly lower than that of NSt treatment starch. Compared with the NSt-NSf and NSt-Sf treatments, the solubility of starch after the St-NSf and St-Sf treatments were decreased to 63 and 36%, respectively. The Sf treatment also reduced the solubility of starch compared with the NSt-NSf and St-NSf treatments, and the solubility of starch after the NSt-Sf and St-Sf treatments decreased to 83 and 48%, respectively (Figure 4A). The St treatment significantly increased the starch swelling power, and compared with the NSt-NSf and NSt-Sf treatments, the swelling power of starch after the St-NSf and St-Sf treatments was significantly increased by 211 and 187%, respectively. Sf treatment had no significant effect on the swelling of NSt-treated G. elata starch. No significant difference was found between the NSt-NSf and NSt-Sf treatments. The St-Sf treatment significantly reduced the swelling of G. elata starch compared with the St-NSf treatment. Compared with St-NSf-treated starch, the swelling of St-Sf-treated starch was decreased to 79% (Figure 4B).
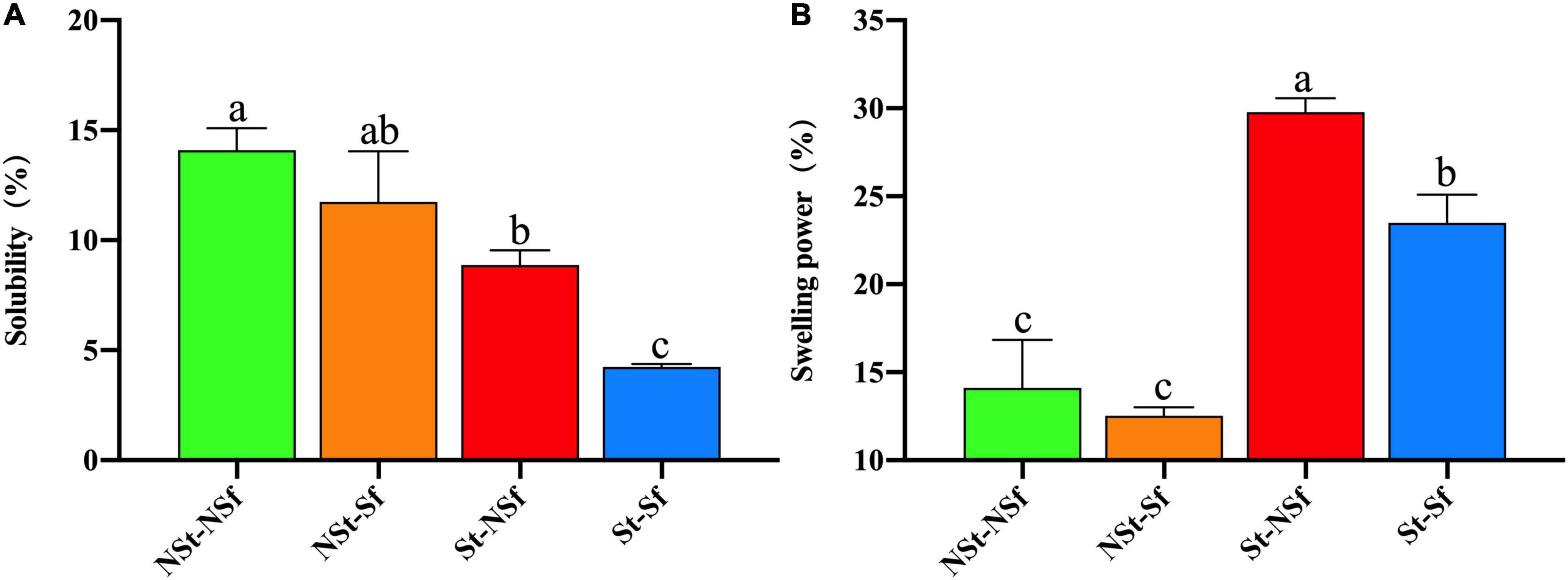
Figure 4. Effect of steaming and sulfur fumigation on solubility (A) and swelling power (B) of Gastrodia elata starch.
3.5. Effect of steaming and sulfur fumigation on the TPA of G. elata starch gel
The St treatment of fresh G. elata led to a significant increase in the hardness and adhesiveness of starch gel. The hardness of St-NSf and St-Sf was 1.18 and 1.78-fold higher than that of NSt-NSf and NSt-Sf, respectively. The adhesiveness of St-NSf and St-Sf was 3.48 and 2.91-fold higher than that of NSt-NSf and NSt-Sf, respectively. St treatment decreased the cohesiveness and resilience by increasing the viscosity of the G. elata starch gel. The cohesiveness of the St-NSf and St-Sf treatments was 79 and 85% lower than that of the NSt-NSf and NSt-Sf treatments, and the resilience of the St-NSf and St-Sf treatments was 83 and 62% lower than that of the NSt-NSf and NSt-Sf treatments, respectively. The St treatment had no significant effect on the springiness, gumminess and chewiness of G. elata starch gel (Table 2).
The Sf treatment of G. elata also significantly increased the hardness and adhesiveness of starch gel. The hardness of the NSt-Sf and St-Sf treatments was 1.16 and 1.76-fold higher than that of the NSt-NSf and St-NSf treatments, respectively. The adhesiveness of the NSt-Sf and St-Sf treatments was 1.35 and 1.12-fold higher than that of the NSt-NSf and St-NSf treatments, respectively. Sf treatment decreased the cohesiveness and resilience of G. elata starch. The cohesiveness of the NSt-Sf and St-Sf treatments was 23 and 18% lower than that of the NSt-NSf and St-NSf treatments, respectively, and the resilience of the NSt-Sf and St-Sf treatments was 28 and 47% lower than that of the NSt-NSf and St-NSf treatments, respectively. Compared with the NSt-NSf, NSt-Sf, and St-NSf treatments, the St-Sf treatment decreased the springiness but significantly increased the gumminess and chewiness. The springiness decreased by 14, 15, and 16%, while the gumminess increased by 1.36, 1.52, and 1.46-fold, and the chewiness increased by 1.17, 1.29, and 1.24-fold, respectively (Table 2).
3.6. Effects of steaming and sulfur fumigation on FT-IR and XRD characteristics of G. elata starch
Gastrodia elata starch shows five absorption peaks under FT-IR: 3,411 cm–1 (O-H stretching), 2,933 cm–1 (C-H stretching), 1,643 cm–1 (C-O bending), 1,384 cm–1 (C-H bending), and 1,024 cm–1 (C-O stretching). A weak absorption peak was generated between 1,176 and 1,024 cm–1, which was caused by the tensile behavior of C-C and C-O. The weak absorption peak near 1,383 cm–1 was attributed to the bending vibration of -C-H, C-C-H, and C-O-H. Compared with CK (pure starch, V900508, Sigma-Aldrich Co., Ltd., Darmstadt, Germany), G. elata starch did not produce new absorption peaks after St treatment or Sf treatment, indicating that steaming or sulfur fumigation treatment did not alter the functional groups of G. elata starch (Figure 5A).
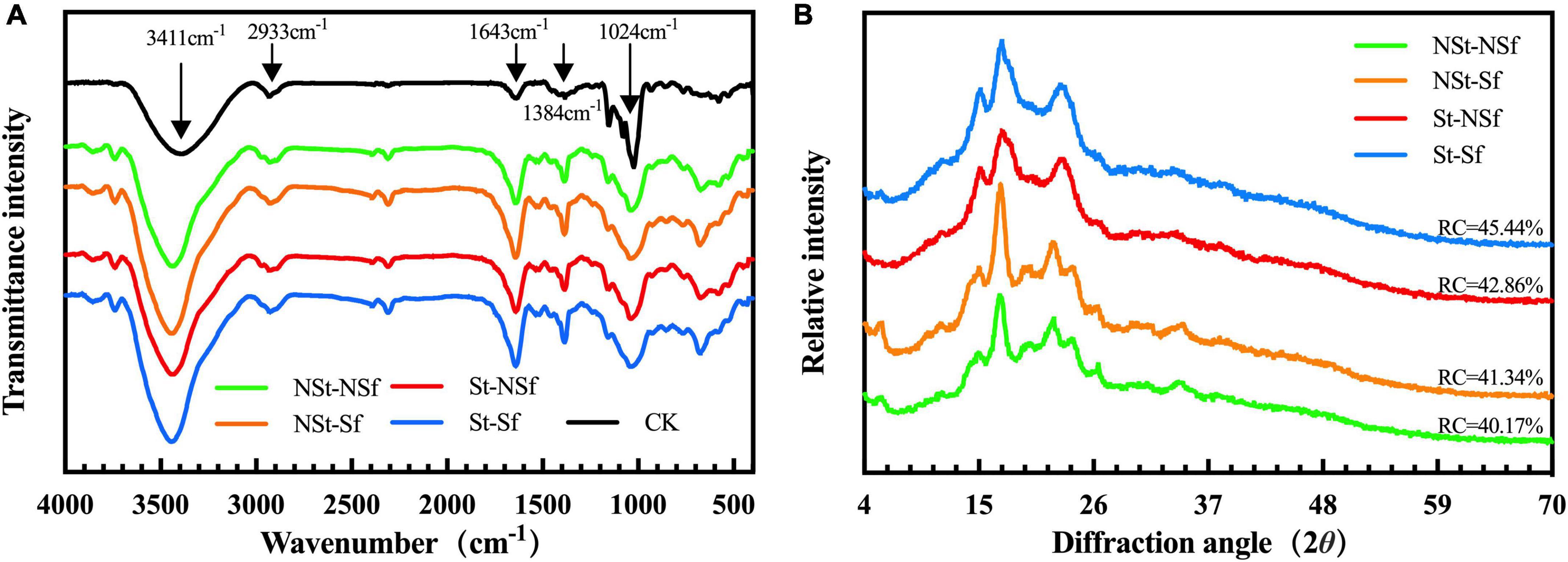
Figure 5. Fourier transform infrared spectroscopy (FT-IR) (A) and X-ray diffraction (XRD) (B) spectra of Gastrodia elata starch.
The XRD scanning results of G. elata starch showed diffraction peaks at 5.675°, 17.019°, 22.219°, and 24.031° after NSt-NSf and NSt-Sf treatments, indicating that the NSt treatment of G. elata starch was a typical B-type starch. Steamed G. elata lacked the diffraction peaks of St-NSf and St-Sf at 5.675°, and the double peaks of 22.219° and 24.031° merged into a single peak at 23.19°, indicating that the structure of the steamed G. elata starch changed to A-type (17). The relative crystallinity (RC) of starch treated with St-NSf and St-Sf increased by 1.17 and 2.58%, respectively, compared with NSt-NSf and NSt-Sf. Sulfur fumigation sharpened the characteristic peak shape of G. elata starch compared with that of nonfumigated starch, indicating enlarged grain size and increased RC. The RC of starch treated with NSt-Sf and St-Sf increased by 2.69 and 4.10%, respectively, compared with that of starch treated with NSt-NSf and St-NSf (Figure 5B).
3.7. Effect of steaming and sulfur fumigation on the RVA characteristics of G. elata starch
During RVA, starches treated with St-NSf and St-Sf showed obvious peak viscosity (PV) and final viscosity (FV), but neither NSt-NSf nor NSt-Sf-treated starches reached PV and FV. Therefore, the PV and FV of St G. elata starch were further studied. After St treatment, the pasting temperature (Pt) of G. elata starch decreased, while PV and FV increased. Compared with G. elata starch treated with NSt-NSf and NSt-Sf, the Pt of the starch treated with St-NSf and St-Sf decreased by 11.7 and 15.47°C, respectively. The Sf treatment also reduced Pt and promoted the increase in PV and FV. Compared with starch treated with NSt-NSf and NSt-Sf, the Pt of starch treated with St-NSf and St-Sf decreased by 0.48 and 4.24°C, respectively, and the PV and FV of St-Sf increased by 1.95 and 1.33-fold, respectively, compared with St-NSf (Figure 6 and Supplementary Table 1).
3.8. Effect of steaming and sulfur fumigation on TG characteristics of G. elata starch
Gastrodia elata starch exhibits two rapid weight loss stages at 50–100°C and 250–350°C. The weight loss in the first stage is caused by the evaporation of crystal water in starch, while the second stage is attributed to the decomposition of starch. The presence of only two weight loss stages indicates that starch is a single component except water (Figures 7A, B). The St treatment decreased the degradation temperature (Td) of G. elata starch. The Td values of the St-NSf and St-Sf treatments were 8.6 and 8.5°C lower than those of the NSt-NSf and NSt-Sf treatments, respectively. The Sf treatment also decreased the Td value of G. elata starch. The NSt-Sf and St-Sf treatments decreased the Td values by 4.3 and 4.2°C, respectively, compared with the NSt-NSf and St-NSf treatments. St treatment reduced the loss of G. elata starch weight. The St-NSf and St-Sf treatments reduced the loss by 4.67 and 3.44%, respectively, compared with the NSt-NSf and NSt-Sf treatments. The Sf treatment also reduced the loss of G. elata starch weight. Compared with the NSt-NSf and St-NSf treatments, the weight loss of the NSt-Sf and St-Sf treatments decreased by 6.49 and 5.26%, respectively (Supplementary Table 1).
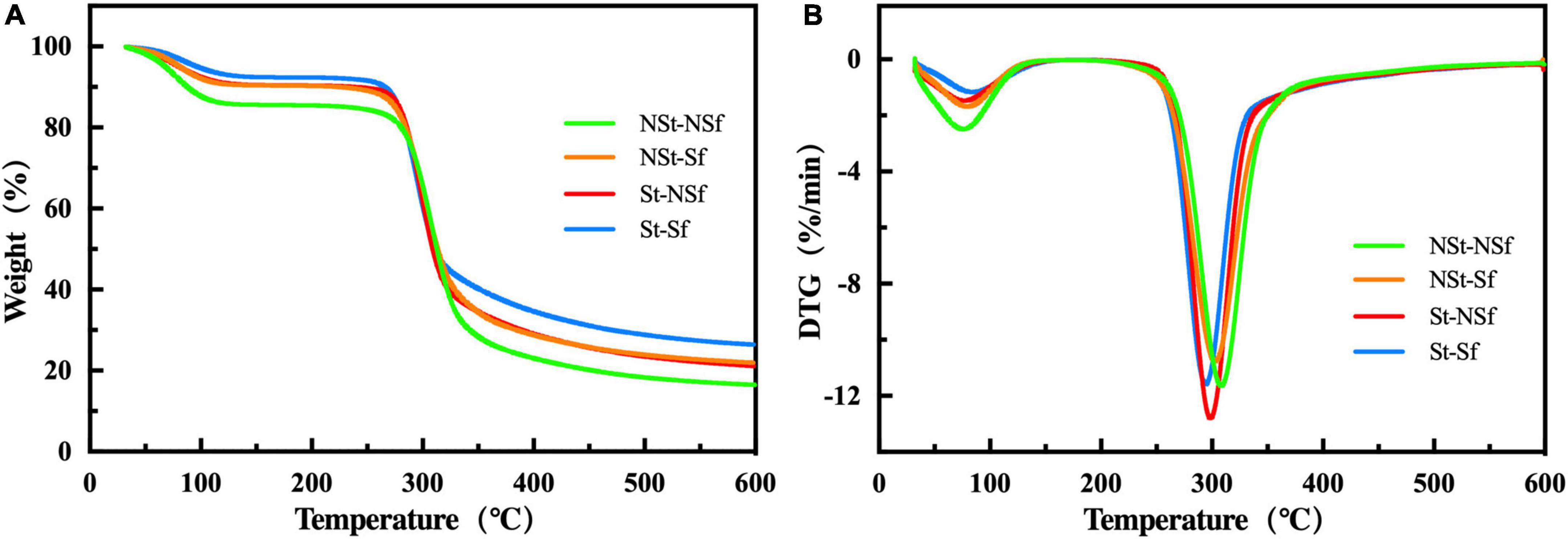
Figure 7. Thermogravimetric curves of weight changes with temperature (A) and derivative thermogravimetry (DTG) changes with temperature (B) of Gastrodia elata starch treated with nonsteam (NSt), steam (St) and nonsulfur fumigation (NSf), sulfur fumigation (Sf).
3.9. Effects of steaming and sulfur fumigation on rheological characteristics of G. elata starch
The apparent viscosity of G. elata starch decreased with increasing shear rate, showing the characteristics of pseudoplastic fluid (Figure 8A). St-NSf and St-Sf treatment led to a significant increase in the apparent viscosity of starch compared with that of NSt-NSf and NSt-Sf treatment. In addition, the NSt-Sf and St-Sf treatments also increased the apparent viscosity of starch compared with the NSt-NSf and St-NSf treatments. The rheological behavior of starch shown in Figure 8B was fitted with a power law equation, and the results showed that all non-Newtonian indices (n) were less than 1, indicating that the samples were all non-Newtonian fluids. The n values of the St-NSf and St-Sf treatments were significantly higher than those of the NSt-NSf and NSt-Sf treatments, indicating that steaming enhanced the pseudoplasticity of the starch gel, and sulfur fumigation also enhanced the pseudoplasticity of the starch gel (Supplementary Table 2). In addition, the increased value of the consistency coefficient K in the fitted equation also indicates that both steaming and sulfur fumigation can increase the viscosity of G. elata starch.
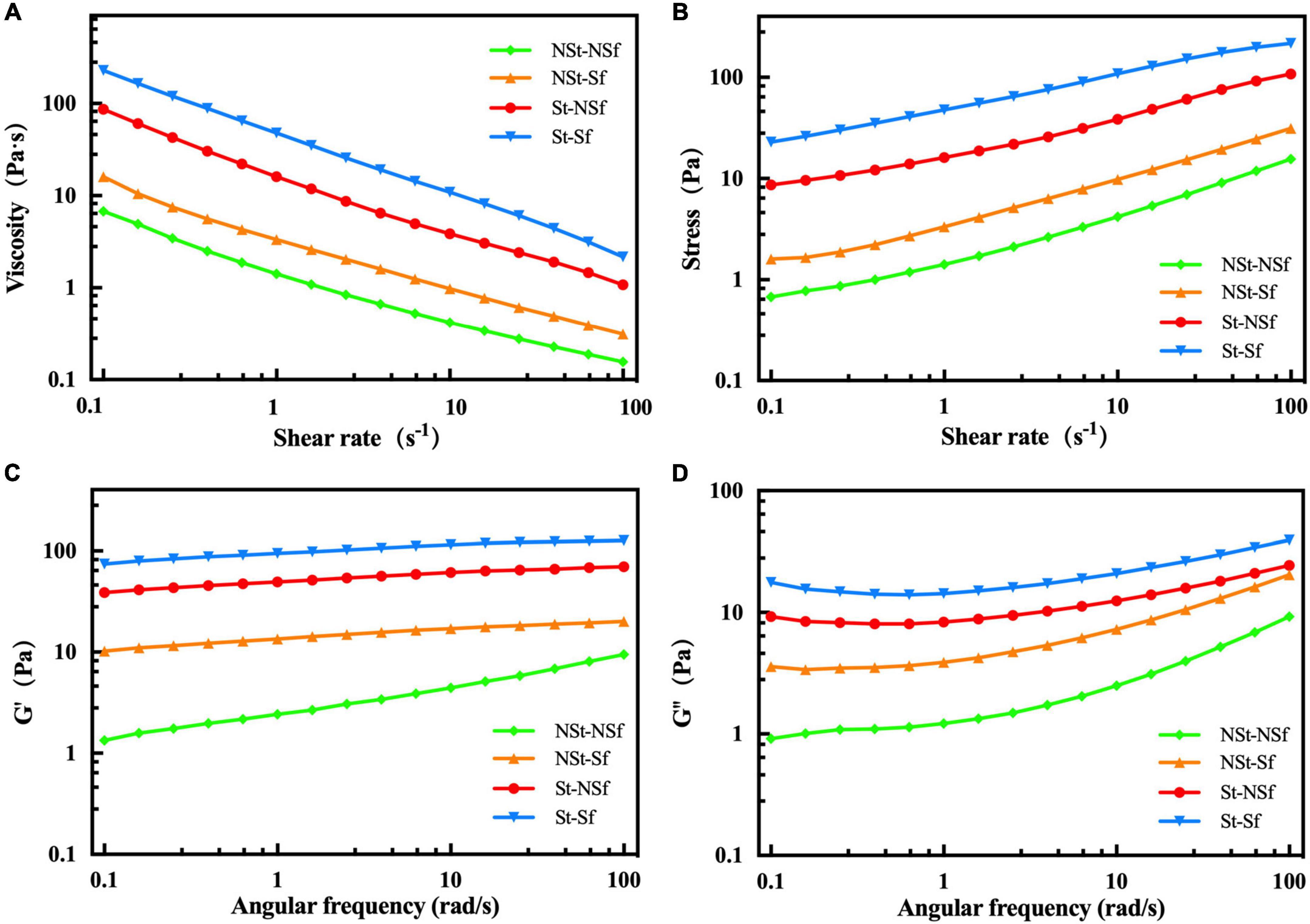
Figure 8. Stable shear flow curves of viscosity changes with shear rate (A) and stress changes with shear rate (B). The changes in storage modulus (G′) (C) and loss modulus (G′′) (D) of Gastrodia elata starch at 25°C and 1% strain.
The storage modulus (G′) and loss modulus (G′′) gradually increase with increasing angular frequency, and G′ > G′′, indicating that G. elata starch is a weak gel (Figures 8C, D). The G′ and G′′ of steaming treatments (St-NSf and St-Sf) were significantly higher than those of nonsteaming treatments (NSt-NSf and NSt-Sf), indicating that the degree of molecular crosslinking of gelatinized G. elata starch solution was significantly enhanced by the St treatment. The G′ and G′′ of the sulfur fumigation treatment (NSt-Sf and St-Sf) were significantly higher than those of the nonfumigation treatment (NSt-NSf and St-NSf), indicating that the degree of molecular crosslinking of gelatinized G. elata starch solution was also significantly enhanced following sulfur fumigation.
3.10. Effect of steaming and sulfur fumigation on the molecular weight distribution of G. elata starch
The high-molecular-weight amylopectin in G. elata starch eluted at 22–34 min, and the low-molecular-weight amylose eluted at 34–45 min (Figure 9). After normalizing the intensity, the peak time of G. elata amylopectin elution after steaming was earlier than that of nonsteamed amylopectin. St-NSf and St-Sf were 0.76 and 0.76 min earlier than NSt-NSf and NSt-Sf; the number-average molecular mass (Mn) was 1.27 and 1.49-fold higher; the weight-average molecular mass (Mw) was 1.30 and 1.53-fold higher; and the polydispersity index (PDI) was 1.03 and 1.02-fold higher, respectively. After Sf treatment, the peak time of G. elata amylopectin elution was earlier than that of NSf-treated amylopectin. Compared with NSt-NSf and St-NSf, NSt-Sf and St-Sf increased by 0.34 and 0.33 min; Mn decreased by 17.55 and 2.94%; Mw decreased by 18.34 and 4.14%; and PDI decreased by 1.09 and 1.60%, respectively (Supplementary Table 3).
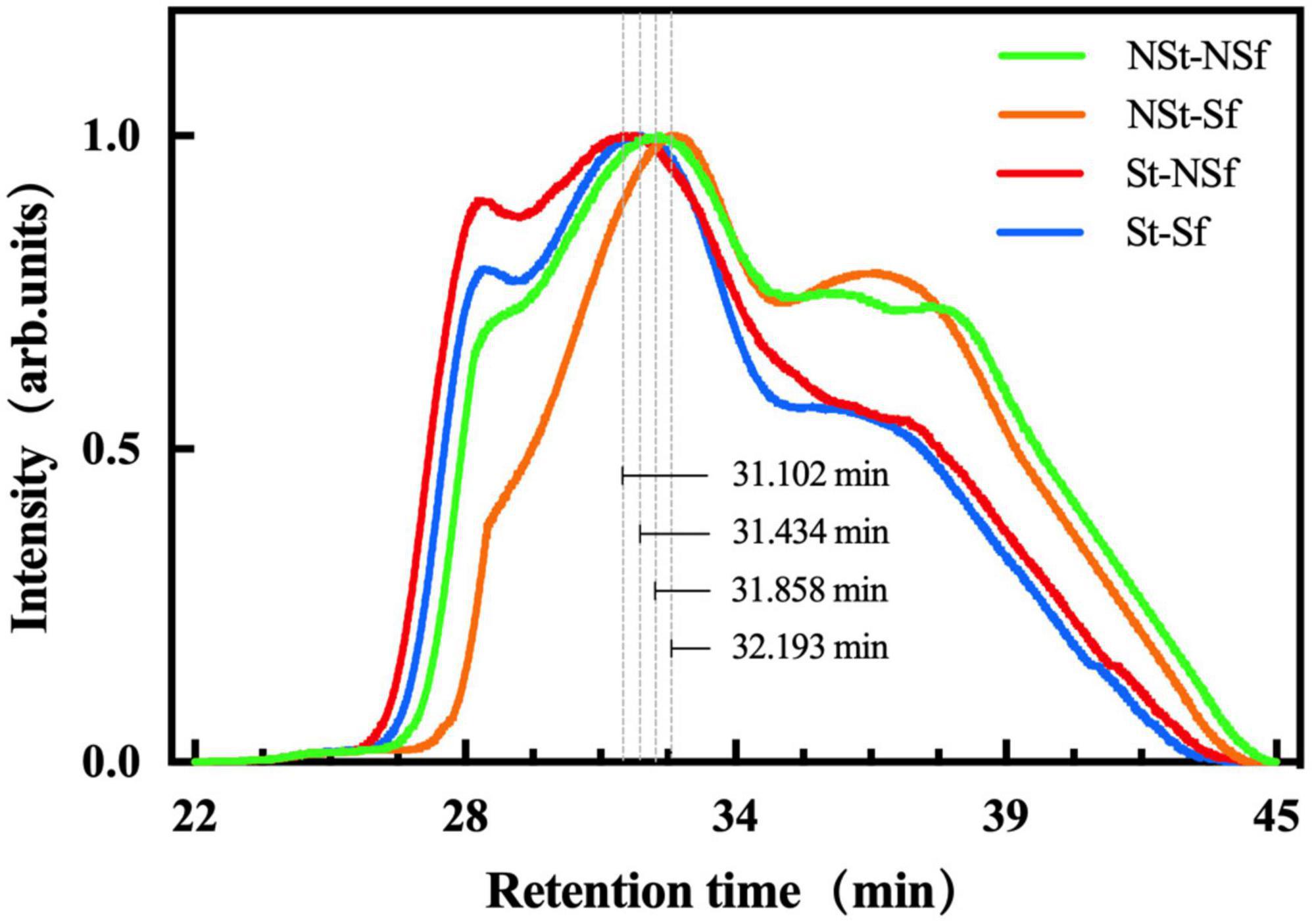
Figure 9. Molecular weight and size distribution of Gastrodia elata starch (with normalized intensity).
3.11. Effect of steaming and sulfur fumigation on the in vitro digestibility of G. elata starch
The RSD content of G. elata was significantly increased after steaming. Compared with NSt-NSf and NSt-Sf, it was 1.05 and 1.04-fold higher under St-NSf and St-Sf treatments, respectively. Sulfur fumigation also promoted an increase in the RSD content of G. elata. Compared with the NSt-NSf and St-NSf treatments, the RSD content under NSt-Sf and St-Sf increased by 1.04 and 1.04-fold, respectively. However, the effect of steaming and sulfur fumigation on the levels of SDS and RS showed no obvious change (Table 3). The linear fitting results indicated that the starch digestion rate from 20 to 240 min under the four treatments was significantly higher under steaming than under nonsteaming conditions, indicating the enhanced stability of the starch hydrolysis rate after steaming treatment (Figure 10).
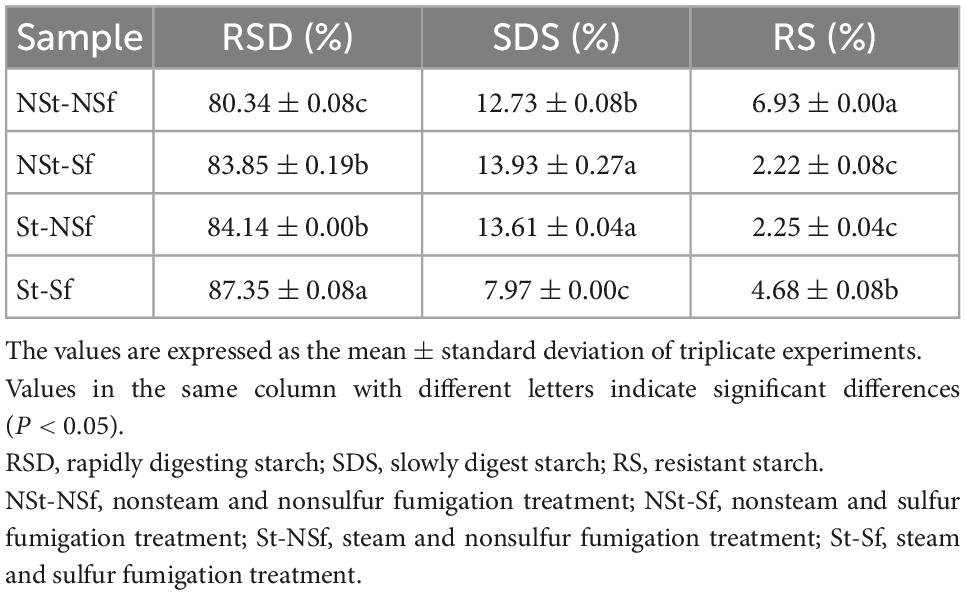
Table 3. Effects of steaming and sulfur fumigation treatment on different starch contents in Gastrodia elata.
3.12. Effect of steaming and sulfur fumigation on the morphology of G. elata starch granules
The starch granuless of nonsteamed G. elata (NSt-NSf and NSt-Sf) was finer and irregular (Figures 11A, B, E, F). Steaming resulted in fusion of the small granules on the surface of the starch granules, resulting in a flat and smooth texture (Figures 11C, D, G, H). Compared with the St-NSf treatment, sulfur fumigation (St-Sf) roughens the surface of the G. elata starch granules, and the structures swell and are even destroyed (Figures 11C, D). Sulfur fumigation (NSt-Sf) decreased the diameter of the starch granules from 0.5 to 0.1–0.3 μm and changed the irregular shape to a near circular shape (Figures 11E, F). Sulfur fumigation (St-Sf) also increased the surface roughness of the steamed starch granules (Figures 11G, H).
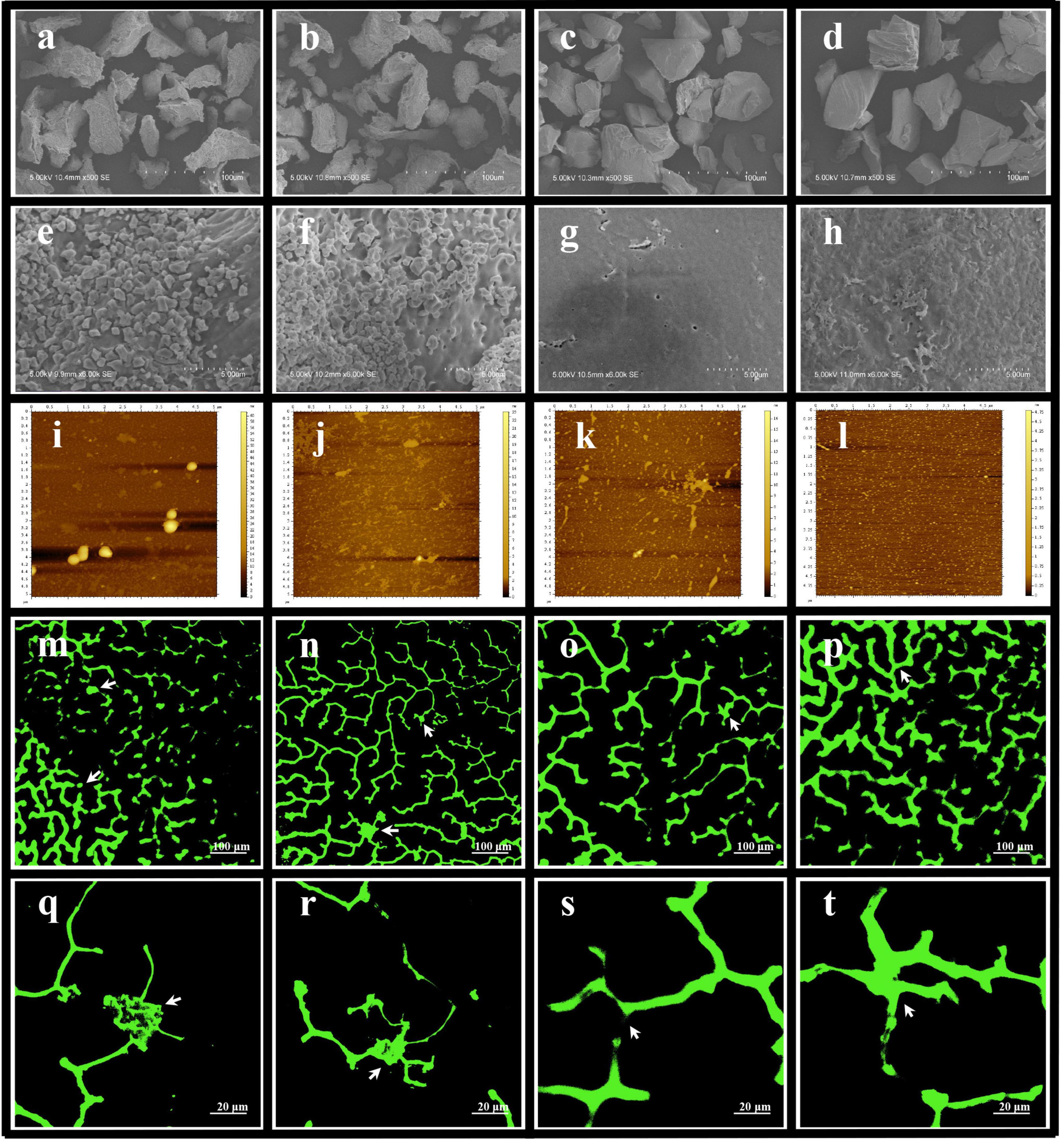
Figure 11. Effect of steaming and sulfur fumigation on the morphology of Gastrodia elata starch granules. Scanning electron microscopy (SEM) images of G. elata starch granules [(a,e) nonsteam and nonsulfur fumigation treatment (NSt-NSf); (b,f) nonsteam and sulfur fumigation treatment (NSt-Sf); (c,g) steam and nonsulfur fumigation treatment (St-NSf); (d,h) steam and sulfur fumigation treatment (St-Sf)]. AFM images of G. elata starch granules after gelatinization for 10 min of NSt-NSf (i), NSt-Sf (j), St-NSf (k), St-Sf (l). Confocal experimental images of G. elata starch granules after gelatinization for 10 min [(m,q) NSt-NSf; (n,r) NSt-Sf; (o,s) St-NSf; (p,t) St-Sf].
Compared with NSt treatments (Figures 11I, J), the gelatinized starch diluent of G. elata was reduced in height and decreased in size in the St treatments (Figures 11K, L), indicating increased leaching of starch chains from the starch granules. Compared with the NSf treatment (Figures 11I, K), the Sf treatment (Figures 11J, L) decreased the granule height and significantly reduced the large starch granules, indicating that sulfur fumigation also promotes the leaching of starch chains from the granules. Among the four treatment conditions, the G. elata starch granules treated with St-Sf showed the least height and exhibited uniform granule shape and size, which indicates that sulfur fumigation after steaming was the most appropriate strategy to promote the leach out of starch chains from starch granules.
Figures 11M–T green fluorescence shows the reticular structure formed by cross-linking of leached out starch chains from starch granules after starch gelatinization. In Figure 11M, the light and dark distribution of green fluorescence without steaming and sulfur fumigation (NSt-NSf) is uneven, and there is an obvious granular structure (as indicated by the white arrow in Figure 11M), which shows that starch granules without steaming and sulfur fumigation treatment are not completely leached out during gelatinization. Compared with the treatment of NSt-NSf and St-NSf (Figures 11M, O, Q, S), the reticular structure (Figures 11N, P, R, T) after treatment of NSt-NSf and St-Sf is clearer and more evenly distributed, which indicates that sulfur fumigation can promote the leaching of starch chains from starch granules. Compared with the nonsteaming treatment (NSt-NSf and NSt-Sf, Figures 11M, N), the steaming treatment (St-NSf and St-Sf) has a more complicated degree of interlacing and winding of the reticular structure formed by the starch chains leached out from the starch granules (Figures 11O, P), which is due to the traction effect of forming hydrogen bonds between short amylose chains produced by steaming with long starch chains. In addition, compared with that without steaming (Figures 11Q, R), no obvious starch granules are found after the starch granules treated by steaming are gelatinized (Figures 11S, T), which indicates that starch chains are almost completely leached out from starch granules.
4. Discussion
After washing the fresh G. elata, it was steamed under normal pressure for 30 min until no white core was observed in the cross section. Sulfur and G. elata were placed in an enclosed fumigation chamber based on specific proportions and ignited the sulfur, which is the traditional processing technology of G. elata (25). The processing technology of G. elata has an 1,800-year history. Although it has been increased several times, the basic operating process of steaming and sulfur fumigation has not changed, suggesting that these two methods play a decisive role in the quality of G. elata. However, their effects on the taste, perception and quality of G. elata and the mechanism of action still lack scientific research. To validate the effect of steaming and sulfur fumigation on G. elata taste, sensation and quality during manufacture, this study focused on the changes in starch properties that are most closely related to the quality of G. elata and show the highest content.
4.1. Steaming and sulfur fumigation changed the morphology of G. elata starch
The morphological quality of starch is mainly measured in terms of color, which is usually described qualitatively based on whiteness, brightness and paste transparency. Light, bright, and transparent starch is more popular with consumers. In this study, both steaming and sulfur fumigation significantly increased the whiteness, brightness and paste transparency of G. elata starch, indicating that the traditional processing technology of G. elata can increase the starch morphology.
Polyphenols in fresh fruits and vegetables will enzymatically brown under the action of polyphenol oxidase to produce brown substances, which will reduce their morphology quality. In the food industry, the color quality of fruits and vegetables is often increased by inhibiting the activity of browning enzymes (26). For example, Hemachandran et al. (27) used cyanidin-3-sophoroside in mangosteen peel to reduce the activity of polyphenol oxidase in a noncompetitive manner, thereby inhibiting the browning of apple and increasing its antioxidant ability. In this study, the whiteness of steamed G. elata starch was significantly higher than that of nonsteamed starch because high-temperature steaming inactivated the browning enzyme in fresh G. elata and reduce enzymatic browning. Sulfur fumigation generates SO2 after combustion, and the gas combines with water in the fumigated product to form sulfite (15). Sulfite is a weak acid that reduces the pH of fumigated substances. Liu et al. (19) reported that after fumigating ginseng with sulfur, the pH of ginseng decoction decreased from 5.32 to 5.09. In this study, the pH of the extract of sulfur-fumigated G. elata was significantly lower than that of the nonfumigated starch, which indicates that the sulfur-fumigated G. elata exhibited the effects of fumigation in this study. Sulfur fumigation is based on the principle of sulfite generation and reaction with organic pigments to form colorless compounds, which inhibit nonenzymatic browning to achieve the bleaching effect (28). In addition, sulfites also form salts to induce antiseptic and antioxidant effects. In this study, the significant increase in the whiteness of G. elata after sulfur fumigation was attributed to the bleaching effect of sulfurous acid generated after fumigation on organic pigments and the inhibition of enzymes with a browning effect, as well as the reduction in the accumulation of pigments produced during microbial reproduction. The combined action increases the whiteness (28, 29).
Nonetheless, we also observed a very interesting phenomenon. Without sulfur fumigation, the pH of G. elata after St treatment was significantly higher than the pH of NSt treatment. Jorge et al. (30) and Jorge et al. (31) reported that cooking and steaming resulted in damage to the plasma membrane of cabbage and increased permeability. Organic acids such as oxalic acid, citric acid, and ascorbic acid spill out of the substances during high-temperature cooking, and acidic substances are lost. Compared with fresh cabbage, the pH increased by approximately 0.15. Therefore, we speculate that the increase in the pH of G. elata after steaming may be related to the loss of organic acids. However, irrespective of G. elata steaming, no significant difference in pH was observed after sulfur fumigation. This is because the pH decrease (0.977) induced by sulfur fumigation is significantly higher than the pH increase (0.578) due to steaming, resulting in a relatively small effect of steaming on pH.
Paste transparency is affected by the color of starch and the size of granules in the solution. The lighter the color and smaller the starch granules are, the higher the paste transparency (32). To increase the quality of starch, starch modification is often used to increase its paste transparency. For example, Lee et al. (11) reported that the paste transparency of pregelatinized rice starch after dry heat treatment at 130°C for 3 h was 1.02-fold higher than that in the absence of dry heat treatment. In addition, Ali et al. (12) reported that acetylation of starch with acetic acid was also effective in increasing the transparency of starch paste. In this study, the increase in the transparency of starch paste was attributed to the increased whiteness of G. elata starch promoted by steaming and sulfur fumigation. However, steaming and sulfur fumigation decreased the granule size of gelatinized starch. Compared with sulfur fumigation, steaming has a more significant effect on increasing the transparency of starch paste. However, sulfur fumigation can further consolidate and enhance the effect of steaming on the transparency of starch paste. It can be seen that the combination of steaming and sulfur fumigation can enhance the whiteness and paste transparency of G. elata starch.
4.2. Steaming and sulfur fumigation increase the pasting properties and chewiness of G. elata starch
The taste index of starch usually refers to the hardness, chewiness and viscosity of the gel. An appropriate gel hardness and viscosity will soften the food but will not be loose and soft or sticky when eating. If the index is very low, it will be loose and tasteless. If it is excessively high, it will be hard to chew. In this study, the branched chains of G. elata starch were broken and polymerized after sulfur fumigation, resulting in an increase in final viscosity (FV) and chewability. This is consistent with the research conclusion of Xie et al. (33).
As cooking indicators, solubility and swelling power refer to the mass loss of dry matter and the change in water absorption of food after cooking. Hormdok et al. (34) reported that low solubility and limited swelling can stabilize the structure of starch gel, which can be described as low solubility and limited swelling and water absorption to enhance the food taste by cooking. Li et al. (35) showed that the cooking quality of noodles with high solubility is worse, and a high degree of swelling results in a soft texture of the noodles, while an extremely low degree of swelling results in a hard texture. Lu et al. (20) also showed that the increased degree of swelling in a solution with a specific water content promotes cross-linking between starch chains and thus enhances the gel strength. The amylose content alters the degree of dissolution and swelling. The results of Yang et al. (36) show that starch with high amylose content is more difficult to dissolve. In addition, Singh et al. (37) reported that the swelling degree of wheat starch was negatively correlated with the amylose content (r = −0.444, P = 0.06). Amylopectin α-1,6 glycosidic bonds form amylose after hydrolysis and debranching, and the unbranched chains become short amylose chains (38). The results of Yang et al. (39) show that the corn starch α- 1,4 glycoside bond is more stable than the α- 1,6 glycoside bond, which indicates that under acidic conditions, α- 1,6 glycosidic bonds are preferentially hydrolyzed. Gunaratne et al. (13) treated wheat, potato, and corn starch with 0.1 mol/L dilute hydrochloric acid for 1.5 h, which increased the amylose content by 1.07, 1.04, and 1.07-fold, respectively. Chen et al. (15) also reported that the contents of amylose and total starch in yam after sulfur fumigation increased by 1.05 and 1.04-fold compared with those of the nonsulfur fumigation treatment, respectively. In this study, the amylose content after St-Sf treatment was 1.10-fold higher than that of NSt-NSf treatment. This is because, on the one hand, the high-temperature treatment induces depolymerization and molecular degradation of starch granules and increases the amylose content (16). On the other hand, the weak acidic conditions formed by sulfite during sulfur fumigation of G. elata also promote the preferential hydrolysis of more unstable α- 1,6 glycosidic bonds of G. elata amylopectin to form short amylose chains and increase their content (15, 39). In this study, the elution peak time of amylopectin after sulfur fumigation is longer than in the absence of sulfur fumigation. This indicates that amylopectin is degraded, which proves that the sulfite we mentioned earlier can hydrolyze α- 1,6 glycosidic bonds, speculation of increasing amylose content. However, the elution peak time of amylopectin after steaming treatment is advanced, and Mn and Mw are significantly increased, which may be caused by the aggregation of hydrogen bonds between starch chains, the molecules formed by this aggregation are not easy to separate in the chromatographic column, thus showing higher molecular weight (Figure 12; 40). Interestingly, the Mn and Mw of starch molecules after steaming are not significantly affected by sulfur fumigation, which may be attributed to the breakage of most molecular chains caused by steaming, so that the sulfites formed by sulfur fumigation cannot break additional molecular chains. To summarize, it can be seen that steaming and sulfur fumigation in this study not only reduce the solubility and increase the swelling degree of starch by changing the molecular crosslinking degree of starch but also further reduce the solubility of starch and limit the excessive increase in swelling degree by increasing the content of amylose, ultimately promoting the increase in starch gel strength.
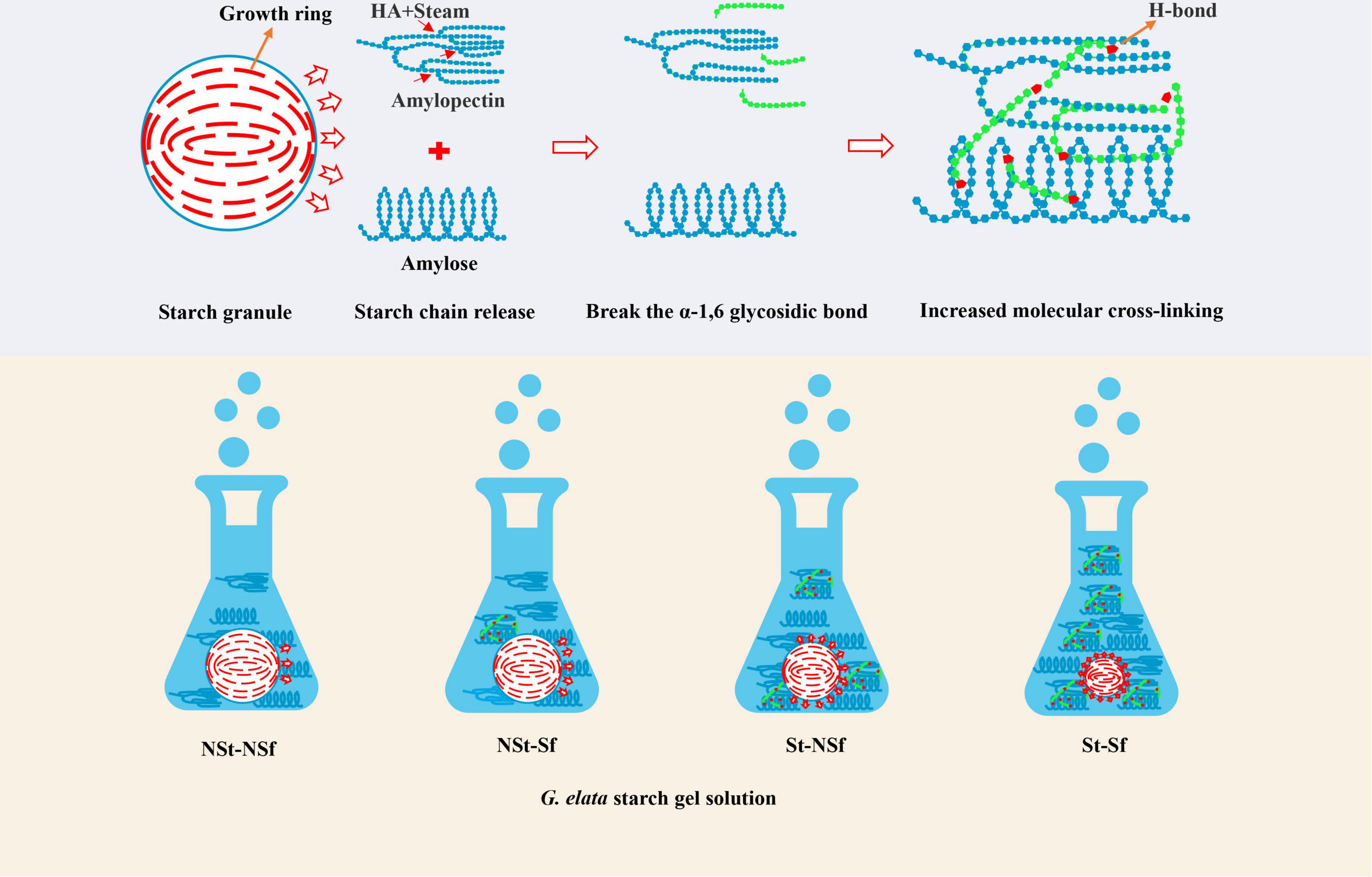
Figure 12. Schematic diagram of starch cross-linking degree change of Gastrodia elata after steaming and sulfur fumigation process.
During cooking, with the gelatinization of starch and the destruction of crystal structure, amylose, and amylopectin chains are leached from starch granules, and the branched structure of the chain is connected by hydrogen bonds, resulting in stronger viscosity (9). Although the structure of starch chains cannot be observed at the granule level, it can be inferred from the height of starch granules. The lower the height of starch granules, the higher the degree of starch chains leached out from starch granules (41). In this study, the AFM results showed that sulfur fumigation and steaming reduced the height of starch granules in starch gelatinization solution, which proved that the leaching out of starch chains was significantly increased. With the gradual increase in the leach out of starch chains from the granules, the crosslinking of the starch chains was enhanced, thus strengthening the gel structure of starch. This is the reason for the increase in starch gel hardness and viscosity, PV and FV during RVA, G′ and G′′ modulus in rheology. Finally, the chewability and sticky taste of G. elata starch gel was increased.
4.3. Steaming and sulfur fumigation change the structure and digestibility of G. elata starch
Starch is the main energy source of human beings. The digestible RSD content is an important index of food quality. The high temperature destroys the double helical structure of starch molecules, reduces the crystal stability and generates further short-chained molecules, resulting in increased RSD content (42). In addition, the release of starch chains caused by high temperature is completely exposed to the sulfurous acid environment produced by sulfur fumigation, which aggravates the breaking of α- 1,6 glycosidic bonds (15, 39, 41). In this study, the heat loss rate of starch after steaming treatment and sulfur fumigation treatment was reduced, and the relative crystallinity was increased. Because it promotes the leaching out and exposure of starch chains from granules, it not only forms more short starch chains but also reduces the size of starch granules, increasing their contact area with hydrolytic enzymes and leading to a significant increase in starch RSD content.
Fourier transform infrared spectroscopy (FT-IR) and XRD can reflect the molecular structure and crystal structure of starch. In this study, there was no significant difference between the functional groups of standard starch (CK) and G. elata starch under the four processing methods (Figure 5A), which indicates that the extracted G. elata starch does not contain protein. TG test results reveal no other loss except for the loss of significant mass in the two stages caused by crystal water and starch molecule loss. Thus, the presence of no other substances except starch in the extracted G. elata starch indicates the absence of impurities affecting the structure and pasting performance of starch. The XRD results showed that steaming induced the transformation of G. elata starch crystals from a B-type structure to an A-type structure and increased the relative crystallinity of starch. This can be attributed to the opening of the B-type structure of starch caused by steaming, and the water molecules enter the double helix channel. Then, loss of water leads to rearrangement of the crystal structure into a more stable A-type crystal (17). Moreover, the movement of water molecules inside starch induced by high-temperature treatment of starch alters the direction of the starch double helix in the crystal region, resulting in a highly ordered crystal configuration and thereby increasing the relative crystallinity of starch (17). Sulfur fumigation has no significant effect on the crystal structure of G. elata starch, but it increases the relative crystallinity, which may be due to the possible hydrolysis of the noncrystalline region of starch under the weakly acidic environment induced by sulfur fumigation, followed by the crystalline region (17). In addition, the digestibility of starch is also related to its crystal structure. According to Xie et al. (43), type A starch is easier to digest than type B starch. In this study, steaming changed the crystalline form of G. elata from type B to type A, accompanied by an increase in RSD content. Therefore, we speculate that the altered crystal structure of G. elata starch is also one of the factors contributing to the increased RSD content.
5. Conclusion
This study showed that sulfur fumigation after steaming could not only increase the morphological quality of G. elata starch by increasing the whiteness and paste transparency through the bleachability of sulfite but also cause the degradation of amylopectin to produce amylose and promote an increase in RSD content to increase its edible quality. In addition, the release degree of starch chains from starch granules was increased by sulfur fumigation after steaming, thus enhancing the pasting characteristics of gel and cooking qualities such as hardness, chewiness, and stickiness, which meant that the taste quality of starch was increased. This work explained the improvement mechanism of steaming and sulfur fumigation treatment on G. elata edible quality and provided a scientific basis for the rational use of sulfur fumigation technology in food processing. This modified starch method may be used to improve the gel taste of low viscosity food and the digestion rate of starch products.
Data availability statement
The original contributions presented in this study are included in the article/Supplementary material, further inquiries can be directed to the corresponding authors.
Author contributions
JG, ZC, and YY wrote and reviewed the manuscript. TZ, JG, TX, and FW collected the samples. JG, ZC, LG, CW, and YY provided analysis ideas and reviewed the manuscript. JG and ZC reviewed the manuscript and drew the picture. LG and XC supervised the work and read the manuscript. All authors contributed to the article and approved the submitted version.
Funding
We are grateful for support from Yunnan Major Scientific and Technological Projects (ID No. 202202AG050008), the Key Natural Science Foundation of Yunnan Province (ID No. 202102AA310045), and the Innovation Team and Talents Cultivation Program of the National Administration of Traditional Chinese Medicine (ID No. ZYYCXTD-D-202005).
Acknowledgments
The authors thank EditorBar Language Editing Services (www.editorbar.com) for English language editing and review services.
Conflict of interest
The authors declare that the research was conducted in the absence of any commercial or financial relationships that could be construed as a potential conflict of interest.
Publisher’s note
All claims expressed in this article are solely those of the authors and do not necessarily represent those of their affiliated organizations, or those of the publisher, the editors and the reviewers. Any product that may be evaluated in this article, or claim that may be made by its manufacturer, is not guaranteed or endorsed by the publisher.
Supplementary material
The Supplementary Material for this article can be found online at: https://www.frontiersin.org/articles/10.3389/fnut.2022.1087453/full#supplementary-material
References
1. Chen YP, Li ZB, Ettoumi F, Li D, Wang L, Zhang XC, et al. The detoxification of cellular sulfite in table grape under SO2 exposure: Quantitative evidence of sulfur absorption and assimilation patterns. J Hazard Mater. (2022) 439:129685. doi: 10.1016/j.jhazmat.2022.129685
2. NHFPC National Health and Family Planning Commission of the People’s Republic of China. Chinese National Standard for the Use of Food Additives (GB2076–2014). Beijing: NHFPC (2014).
3. Chinese Pharmacopoeia Commission. Pharmacopoeia of the People’s Republic of China. Beijing: Chinese Pharmacopoeia Commission (2020).
4. Kang CZ, Lai CJS, Zhao D, Zhou T, Liu DH, Lv CG, et al. A practical protocol for comprehensive evaluation of sulfur fumigation of gastrodia rhizoma using metabolome and health risk assessment analysis - sciencedirect. J Hazard Mater. (2017) 340:221–30. doi: 10.1016/j.jhazmat.2017.07.003
5. Li Y, Shabani KI, Liu HB, Guo Q, Liu X. Structural, physicochemical and rheological properties of a novel native starch obtained from Rhizoma Gastrodiae. Food Struct. (2020) 25:100148. doi: 10.1016/j.foostr.2020.100148
6. Cao S, Ba LJ, Pan C, Ma C, Xie XL, Wang R. Effect of different darvest time on the storage of gastrodia elata. Food Ind. (2019) 40:192–6.
7. Panjaitan L, Achrom M, Suherman B, Nursusilawati, Fauziaty MR, Kurniasih KT, et al. Quality comparison of indirect sun-drying and sulphur fumigation methods on copra production and storage. IOP Conf Ser Earth and Environ Sci. (2022):012120. doi: 10.1088/1755-1315/974/1/012120
8. Li H, Prakash S, Nicholson TM, Fitzgerald MA, Gilbert RG. The importance of amylose and amylopectin fine structure for textural properties of cooked rice grains. Food Chem. (2016) 196:702–11. doi: 10.1016/j.foodchem.2015.09.112
9. Li Q, Li C, Li E, Gilbert RG, Xu B. A molecular explanation of wheat starch physicochemical properties related to noodle eating quality. Food Hydrocoll. (2020) 108:106035. doi: 10.1016/j.foodhyd.2020.106035
10. Fitzgerald MA, Mccouch SR, Hall RD. Not just a grain of rice: the quest for quality. Trends Plant Sci. (2009) 14:133–9. doi: 10.1016/j.tplants.2008.12.004
11. Lee SJ, Yang YJ, Chung HJ, Lim ST. Effect of dry heating on physicochemical properties of pregelatinized rice starch. Cereal Chem. (2017) 94:928–33. doi: 10.1094/cchem-03-17-0045-r
12. Ali TM, Hasnain A. Physicochemical, morphological, thermal, pasting, and textural properties of starch acetates. Food Rev Int. (2016) 32:150609042312001. doi: 10.1080/87559129.2015.1057842
13. Gunaratne A, Corke H. Influence of prior acid treatment on acetylation of wheat, potato and maize starches. Food Chem. (2007) 105:917–25. doi: 10.1016/j.foodchem.2007.04.042
14. Jyothi AN, Kiran KS, Wilson B, Moorthy SN, Nambisan B. Wet storage of cassava starch: Use of sodium metabisulphite and acetic acid and the effect on starch properties. Starch. (2007) 59:141–8. doi: 10.1002/star.200600583
15. Chen X, Li X, Mao X, Huang H, Wang T, Qu Z, et al. Effects of drying processes on starch-related physicochemical properties, bioactive components and antioxidant properties of yam flours. Food Chem. (2017) 224:224–32. doi: 10.1016/j.foodchem.2016.12.028
16. Kankate D, Panpalia SG, Kumar KJ, Kennedy JF. Studies to predict the effect of pregelatinization on excipient property of maize and potato starch blends. Int J Biol Macromol. (2020) 164:1206–14. doi: 10.1016/j.ijbiomac.2020.07.170
17. Li H, Wang RR, Liu J, Zhang Q, Li GY, Shan Y, et al. Effects of heat-moisture and acid treatments on the structural, physicochemical, and in vitro digestibility properties of lily starch. Int J Biol Macromol. (2020) 148:956–68. doi: 10.1016/j.ijbiomac.2020.01.181
18. Jiang QQ, Gao WY, Shi YP, Li X, Wang HY, Huang LQ, et al. Physicochemical properties and in vitro digestion of starches from different dioscorea plants. Food Hydrocoll. (2013) 32:432–9. doi: 10.1016/j.foodhyd.2013.02.001
19. Liu H, Wang SY, Zhu JH, Xu JD, Zhou SS, Kong M, et al. Effects of sulfur-fumigated ginseng on the global quality of Si-Jun-Zi decoction, a traditional ginseng-containing multi-herb prescription, evaluated by metabolomics and glycomics strategies. J Pharm Biomed Anal. (2022) 219:114927. doi: 10.1016/j.jpba.2022.114927
20. Lu Y, Shen MY, Li YP, Xiao YH, Wen HL, Ren YM, et al. Effect of Mesona chinensis polysaccharide on pasting, rheological and structural properties of corn starches varying in amylose contents. Carbohydr Polym. (2019) 230:115713. doi: 10.1016/j.carbpol.2019.115713
21. Uarrota VG, Amante ER, Demiate IM, Vieira F, Delgadillo I, Maraschin M. Physicochemical, thermal, and pasting properties of flours and starches of eight Brazilian maize landraces (Zea mays L.). Food Hydrocoll. (2013) 30:614–24. doi: 10.1016/j.foodhyd.2012.08.005
22. Correia PR, Nunes MC, Beirão-da-Costa ML. The effect of starch isolation method on physical and functional properties of Portuguese nuts starches. I. Chestnuts (Castanea sativa Mill. var. Martainha and Longal) fruits. Food Hydrocoll. (2012) 27:256–63. doi: 10.1016/j.foodhyd.2011.05.010
23. Ma YS, Zhang H, Jin YM, Xu D, Xu XM. Impact of superheated steam on the moisture transfer, structural characteristics and rheological properties of wheat starch. Food Hydrocoll. (2021) 2021:107089. doi: 10.1016/j.foodhyd.2021.107089
24. Zeng F, Li T, Gao QY, Liu B, Yu SJ. Physicochemical properties and in vitro digestibility of high hydrostatic pressure treated waxy rice starch. Int J Biol Macromol. (2018) 120:1030–8. doi: 10.1016/j.ijbiomac.2018.08.121
25. Yang F, Wang X, Ma CJ, Chen YH, Sun AP, Jia J, et al. Research progress of Gastrodiae Rhizoma machining processing, composition analysis and in vivo metabolism. China J Chin Materia Med. (2018) 43:2207–15. doi: 10.19540/j.cnki.cjcmm.20180322.008
26. Bangar SP, Trif M, Ozogul F, Kumar M, Chaudhary V, Vukic M, et al. Recent developments in cold plasma-based enzyme activity (browning, cell wall degradation, and antioxidant) in fruits and vegetables. Compr Rev Food Sci Food Saf. (2022) 21:1958–78. doi: 10.1111/1541-4337.12895
27. Hemachandran H, Anantharaman A, Mohan S, Mohan G, Kumar DT, Dey D, et al. Unraveling the inhibition mechanism of cyanidin-3-sophoroside on polyphenol oxidase and its effect on enzymatic browning of apples. Food Chem. (2017) 227:102–10. doi: 10.1016/j.foodchem.2017.01.041
28. Sun JX, Luo HX, Li XH, Li XS, Lu YY, Bai WB. Effects of low power ultrasonic treatment on the transformation of cyanidin-3-O-glucoside to methylpyranocyanidin-3-O-glucoside and its stability evaluation. Food Chem. (2019) 276:240–6. doi: 10.1016/j.foodchem.2018.10.038
29. Ahmadi F, Lee YH, Lee WH, Oh YK, Kwak AK. Preservation of fruit and vegetable discards with sodium metabisulfite. J Environ Manag. (2018) 224:113–21. doi: 10.1016/j.jenvman.2018.07.044
30. Jorge A, Gómez-Limia L, Carballo J, Martínez S. Effects of different cooking methods on some chemical and sensory properties of Galega kale. Int J Food Sci Technol. (2016) 51:2071–80. doi: 10.1111/ijfs.13181
31. Jorge A, Gómez-Limia L, Carballo J, Martínez S. Effects of different cooking methods on the antioxidant capacity and flavonoid, organic acid and mineral contents of Galega Kale (Brassica oleracea var. acephala cv. Galega). Int J Food Sci Nutr. (2018) 70:136–49. doi: 10.1080/09637486.2018.1482530
32. Tu ZC, Yin YB, Wang H, Liu GX, Chen LL, Zhang P, et al. Effect of dynamic high-pressure microfluidization on the morphology characteristics and physicochemical properties of maize amylose. Starch. (2012) 65:390–7. doi: 10.1002/star.201200120
33. Xie YK, Li XY, Zhang Y, Zheng ZA, Liu YH. Effects of high-humidity hot air impingement steaming on gastrodia elata: steaming degree, weight loss, texture, drying kinetics, microstructure and active components. Food Bioprod Process. (2021) 127:255–65. doi: 10.1016/j.fbp.2021.03.005
34. Hormdok R, Noomhorm A. Hydrothermal treatments of rice starch for improvement of rice noodle quality. LWT Food Sci Technol. (2006) 40:1723–31. doi: 10.1016/j.lwt.2006.12.017
35. Li QQ, Liu SY, Obadi MH, Jiang YY, Zhao FF, Jiang S, et al. The impact of starch degradation induced by pre-gelatinization treatment on the quality of noodles. Food Chem. (2020) 302:125267. doi: 10.1016/j.foodchem.2019.125267
36. Yang SY, Li C, Wang LG. Dissolution of starch and its role in the flotation separation of quartz from hematite. Powder Technol. (2017) 320:346–57. doi: 10.1016/j.powtec.2017.07.061
37. Singh S, Singh N, Isono N, Noda T. Relationship of granule size distribution and amylopectin structure with pasting, thermal, and retrogradation properties in wheat starch. J Agric Food Chem. (2010) 58:1180–8. doi: 10.1021/jf902753f
38. Qi X, Tester RF. Is starch or maltodextrin “Glucose?”. Starch. (2018) 70:1700304. doi: 10.1002/star.201700304
39. Yang QY, Lu XX, Chen YZ, Luo ZG, Xiao ZG. Fine structure, crystalline and physicochemical properties of waxy corn starch treated by ultrasound irradiation. Ultrason Sonochem. (2018) 51:350–8. doi: 10.1016/j.ultsonch.2018.09.001
40. Wu WJ, Huang NW, Huang JP, Wang LL, Wu LL, Wang Q, et al. Effects of the steaming process on the structural properties and immunological activities of polysaccharides from Polygonatum cyrtonema. J Funct Foods. (2022) 88:104866. doi: 10.1016/j.jff.2021.104866
41. An HJ, Liang HB, Liu ZD, Yang HS, Liu QD, Wang HB. Nano-Structures of debranched potato starch obtained by isoamylolysis. J Food Sci. (2011) 76:N11–4. doi: 10.1111/j.1750-3841.2010.01881.x
42. Liu H, Liang R, Antoniou J, Liu F, Shoemaker CF, Li Y, et al. The effect of high moisture heat-acid treatment on the structure and digestion property of normal maize starch. Food Chem. (2014) 159:222–9. doi: 10.1016/j.foodchem.2014.02.162
Keywords: Gastrodia elata starch, steaming, sulfur fumigation, edible quality, sulfur dioxide gas
Citation: Guan J, Chen Z, Guo L, Cui X, Xu T, Wan F, Zhou T, Wang C and Yang Y (2023) Evaluate how steaming and sulfur fumigation change the microstructure, physicochemical properties and in vitro digestibility of Gastrodia elata Bl. starch. Front. Nutr. 9:1087453. doi: 10.3389/fnut.2022.1087453
Received: 02 November 2022; Accepted: 09 December 2022;
Published: 05 January 2023.
Edited by:
A. M. Abd El-Aty, Cairo University, EgyptReviewed by:
Zhen Wu, Chongqing Academy of Chinese Materia Medica, ChinaBaoqing Dum, Institute of Crop Sciences (CAAS), China
Copyright © 2023 Guan, Chen, Guo, Cui, Xu, Wan, Zhou, Wang and Yang. This is an open-access article distributed under the terms of the Creative Commons Attribution License (CC BY). The use, distribution or reproduction in other forums is permitted, provided the original author(s) and the copyright owner(s) are credited and that the original publication in this journal is cited, in accordance with accepted academic practice. No use, distribution or reproduction is permitted which does not comply with these terms.
*Correspondence: Ye Yang, ✉ yangyekm@163.com; Chengxiao Wang, ✉ wangcx@kust.edu.cn
†These authors share first authorship