- 1Key Laboratory of Plant Resource Conservation and Germplasm Innovation in Mountainous Region (Ministry of Education), College of Life Sciences/Institute of Agro-Bioengineering, Guizhou University, Guiyang, Guizhou, China
- 2School of Liquor and Food Engineering, Guizhou University, Guiyang, Guizhou, China
Regular consumption of low-glycemic index (GI) foods is a common strategy for type 2 diabetes patients. To evaluate the potential application of adzuki beans in low-GI foods, the phenolic profile and alpha-amylase inhibitor (α-AI) activity of four varieties of adzuki beans (G24, Te Xiao Li No. 1, Gui Nong No. 1, and Qian Xiao Hei) were determined. The starch digestibility properties and in vitro glycemic index (IVGI) of these beans were also evaluated using the in vitro digestion model coupled with 3,5-dinitrosalicylic acid colorimetry. The results indicated that these adzuki beans, containing numerous phenolics, showed inhibitory activities to alpha-amylase with the α-AI activities between 1.760 ± 0.044 and 3.411 ± 0.186 U/g. The resistant starch (RS) contributed predominantly to the total starch with proportions between (69.78 ± 2.45%) and (81.03 ± 0.06%); Te Xiao Li No. 1 was the highest compared with the other varieties. The adzuki beans were categorized into low- or medium-GI foods, and the IVGI ranged from (39.00 ± 0.36) to (56.76 ± 4.21). These results suggested that adzuki beans can be used as a component of low-GI foods.
1 Introduction
Adzuki beans (Vigna angularis) are common legumes appearing in red, white, black, and green colors (1, 2). China is the largest cultivator and distributes these beans to Japan, Korea, the Philippines, and Malaysia (3). Owing to their delicious taste, these beans are widely used as ingredients in desserts and served at various celebrations, and processed adzuki beans are used more excessively compared with their raw counterpart (4). Adzuki beans contain an abundance of resistant starch (RS), which provides health benefits to type 2 diabetes patients (5, 6), and the RS contributes approximately 50% to the total starch (TS) in beans (7). RS presents resistance to gut digestive enzymes, and is fermented by anaerobic intestinal microbiota in the colon, producing short-chain fatty acids, which offer beneficial effects on type 2 diabetes (8). In addition, adzuki beans are an abundant source of phenolics, and gallic acid, p-hydroxybenzoic acid, ferulic acid, and catechin have been identified in beans (9, 10). These may inhibit the activities of α-amylase and α-glucosidase, frustrating the degradation of polysaccharides into oligosaccharides and monosaccharides (11–13). The α-AI is another dominant component which also inhibits the activities of alpha-amylase (14). Previous studies showed that beans containing functional components presented a lower glycemic index (GI), of which the bean containing total phenolics (61.50 ± 2.12 g/100 g) presented a GI of 55.23 (15), and the bean with RS proportion at 55.60% presented the GI at 60.0 (16), another study indicated that kidney beans classifying in low GI item contained abundant phenolics and RS, which also presented inhibitory activities to alpha-amylase with the specific activity range at 1.65 ± 0.05–4.16 ± 0.04 U/g (17).
Type 2 diabetes is one of the common chronic diseases, which is caused by a disorder of the human pancreas (β-cells). In normal human pancreatic β-cells, pancreatic insulin is stimulated by incretins, such as glucagon-like peptide-1 (GLP-1) and glucose-dependent insulinotropic peptide (GIP), which are responsible for glucose homeostasis (18). Previous studies have shown that adzuki beans are low-GI foods, which positively affects insulin sensitivity and resistance (19–21). In addition, an epidemiological study revealed that regular consumption of common beans can reduce the risk of type 2 diabetes (22). GI is defined as the response of blood sugar after consumption of foods that contain a definite quantity of carbohydrates compared with a reference food (white bread or glucose) (23, 24). Diets with elevated GIs are associated with a high risk of cancer (25), type 2 diabetes (26), and heart disease (27, 28).
Adzuki bean is a staple crop in Bijie city of Guizhou province, where the typical geography conditions are hilly topographic and changeable climate which induce the production of endogenous phytochemicals (29), and the adzuki beans were adopted as food supplements for hyperglycemic patients in the local area, the excellent varieties with a higher yield were selected for the functional evaluation, in which the phenolic profiles were determined using high-performance liquid chromatography (HPLC), the starch digestibility and inhibitory activities to the α-amylase were also determined, the potential GI was evaluated using in vitro digestion model, and the contribution of endogenous components to low-GI was also discussed. All of these results provide the fundamentals for the mechanism revealing that adzuki beans regulate the blood glucose content.
2 Materials and methods
2.1 Sample preparation
The four varieties of adzuki beans, namely G24, Te Xiao Li No. 1, Gui Nong No. 1, and Qian Xiao Hei (Figure 1), planted at the breeding base (27.32°N, 105.29°E, 1,640 m altitude), were supplied by He You Xun, Bijie Institute of Agricultural Science, Guizhou Province, China. In the growth cycle, the average temperature was 19°C, the precipitation was approximately 780.6 mm, and the sunshine duration was 667 h. The adzuki beans were ground into flour using a universal high-speed smashing machine (Dongguan Xinmeihua Electromechanical Equipment Co., Ltd., Dongguan City, P. R. China), and then screened using a 40-mesh sieve; the flours were stored at –20°C for further analysis.
2.2 Chemicals
Folin-Ciocalteu’s phenol reagent, pepsin (source: gastric mucosa of a pig), and gallic acid were purchased from Sigma-Aldrich Ltd. (St. Louis, MO, USA). 3, 5-Dinitrosalicylic acid (DNS) was purchased from Sinopharm Chemical Reagent Co., Ltd. Acetone and hydrochloric acid (36–38%) were purchased from Chongqing Chuandong Chemical Group Co., Ltd. (Chongqing, P. R. China). Sodium hydroxide was purchased from Chengdu Jinshan Chemical Reagent Co., Ltd. (Chengdu, P. R. China). Deionized water was purchased from Watsons Water Co., Ltd. (Guangzhou, P. R. China). HPLC grade acetonitrile and acetate were purchased from Aladdin company (Shanghai, P. R. China). Sodium carbonate was purchased from Yongda Chemical Reagent Co., Ltd. (Tianjin, P. R. China). Aluminum chloride hexahydrate was purchased from Kemio Chemical Co., Ltd. (Tianjin, P. R. China). Rutin, 2, 3, 4-trihydroxybenzoic acid, catechin, chlorogenic acid, p-hydroxybenzoic acid, and ferulic acid were purchased from Macklin Bioch. Co., Ltd (Shanghai, P. R. China). Alpha-amylase enzyme (type: pancreatic, source: Bacillus) was purchased from Solarbio Science & Tech. Co., Ltd. (Beijing, P. R. China). Hexane, ethyl acetate, acetic acid, petroleum ether, and methanol were purchased from Tianjin Fuyu Fine Chemical Co., Ltd. (Tianjin, P. R. China).
2.3 Extraction of phenolics
Phenolic extracts were obtained using the method described by Adom and Liu (30) and De La Parra et al. (31). In brief, 4 g of whole adzuki beans powder was homogenized in 15 ml of chilled acetone (80%, v/v) for 15 min. These mixtures were centrifuged (CenLee16R, Hunan Cenlee Scientific Instrument Co., Ltd., Hunan, China) at 8,000 × g for 5 min to collect the supernatants. The residues were again subjected to phenolic extraction twice following the procedures mentioned above, and the collected supernatants were evaporated at 45°C using a rotary evaporator (Shanghai Yarong Biochemical Instrument Factory) and eventually reconstituted to 10 ml with deionized water. The phenolic extracts were stored at –20°C for further analysis, and the phenolic extraction was performed in triplicate.
2.4 Determination of total phenolics
Total phenolics (TP) were determined using the method described by De La Parra et al. (31). A volume of 100 μl of gallic acid standard and adzuki bean extracts were mixed with 400 μl deionized water and 100 μl of Folin-Ciocalteu phenol reagent. The mixtures were kept at room temperature for 6 min, and subsequently mixed with 1 ml of 7% Na2CO3 solution and 0.8 ml of deionized water. After 90 min of reaction in the dark, 200 μl of the reaction mixtures were pipetted into a 96-well microplate reader (SPECTRAMAX 190, Molecular Devices, Silicon Valley, USA) and determined at 760 nm, and the standard curves were plotted from the absorbances and concentrations of the gallic acid standards. The TP was calculated from the standard curve and expressed as mg gallic acid equivalents (GAE)/100 g DW.
2.5 Determination of total flavonoids
The total flavonoids (TF) were estimated using the methods described by Zhishen et al. (32) and Bakar et al. (33). Five hundred microliters of adzuki bean extracts or rutin standard was allowed to react with 2.25 ml of deionized water and 0.15 ml of 5% NaNO2 for 6 min; the mixtures were continuously reacted with 0.3 ml of 10% AlCl3.6H2O for 5 min. Afterward, 1 ml of 1 mol/L NaOH was vigorously mixed with the mixtures to terminate the reactions. After 15 min of reaction, 200 μl of the mixtures was pipetted into a 96-well microplate reader and determined at 510 nm. The standard curve was plotted from the absorbance and concentration of the rutin standards, and the TF was calculated from the standard curve and expressed as mg rutin equivalents (RE)/100 g DW.
2.6 Profile of phenolic compounds
The profile of the phenolic compounds of the adzuki beans was determined using HPLC (34, 35). The adzuki bean extracts were directly passed through a 0.45-μm filter film, and then the filtered extracts (20 μl) were injected into a 1260 HPLC device (1260 Infinity, Agilent Technologies, Santa Clara, CA, USA) equipped with a C-18 chromatography column (30°C) (Agilent ZORBBAX 250 mm × 4.6 mm, 5 μm). Acetonitrile (A) and 1% acetic acid (B) solutions were obtained using the gradient elution program: 0–5 min, 5–15% A; 5–35 min, 15–35% A; 35–40 min, 35–45% A; 40–50 min, 45–5% A, with a flow rate of 1 ml/min. These individual phenolic compounds were detected using an ultraviolet absorption detector at 280 nm. The individual phenolic compounds were identified and quantified according to the retention time and calibration curve of the corresponding standards. The individual phenolic compound contents were expressed as mg phenolics/100 g DW.
2.7 Determination of α-AI activity
Four grams of adzuki beans powder was adequately mixed with 20 ml of deionized water at 25°C for 1 h. Next, the mixtures were centrifuged (8,000 × g, 4°C) for 30 min to collect the water extracts, and then the volume was recomposed to 20 ml. The above process was performed in triplicate (23).
The α-AI specific activity was determined using the method described by Yang et al. (14), with minor modifications. First, the water extracts were appropriately diluted to keep the α-amylase inhibition rate (IR) under 50%. Second, 0.25 ml of diluted water extracts were adequately mixed with 0.25 ml of α-amylase solution (1 U/ml) and 0.5 ml of PBS (pH 6.9) in a water bath (37°C) for 10 min, and then a 5-min reaction was allowed after the addition of 0.5 ml of 1% (w/v) aqueous starch solution to the mixtures. Eventually, the mixtures were mixed with 1 ml DNS solution and subsequently heated in a boiling water bath for 10 min. After cooling to room temperature, the volume of the mixtures was reconstituted to 20 ml with deionized water and measured at 540 nm using a spectrophotometer instrument (L5S, Shanghai Yidian Analytical Instrument Co. Ltd., Shanghai, China).
The trial groups were estimated as (1) blank tube (no addition of water extracts), (2) blank control tube (addition of neither α-amylase solution nor water extracts), and (3) inhibition control tube (no addition of α-amylase solution). PBS was used to make up for the deficiency.
The inhibition rate of the α-AI in adzuki beans to α-amylase was calculated using formula (1):
Where A1, A2, A3, and A4 are the absorbances of the blank tube, blank control tube, inhibition tube, and inhibitory control tube at 540 nm wavelength, respectively. The amount of α-amylase that catalyzes the formation of 1 mol glucose/min at 37°C and pH 6.9 was defined as an activity unit (U). The amount of α-AI that catalyzes the inhibition of 1 mol glucose formation per min in an α-amylase catalytic starch hydrolysis reaction at 37°C and pH 6.9 was defined as an activity unit (U), while the α-AI specific activity (U/g) is defined as the α-AI activity unit in 1 g of adzuki bean, and was calculated using formula (2):
Where “1” represents the activity of α-amylase solution (U/ml), “n” is the water extract dilution times, “V” is the total volume of water extract (ml), and “m” is the mass of the adzuki beans powder (g).
2.8 Determination of total starch
The TS of adzuki beans was estimated by referring to the National Food Safety Standards of China GB 5009.9 (36). Adzuki bean powder was first processed in fat to remove sugar, and then it underwent continuous processes, such as gelatinization, saccharification, acid hydrolysis, and neutralization, to obtain the mixtures adopted for TS determination using the alkaline copper tartrate solution titration method.
2.9 In vitro digestion and glucose content determination
In brief, an aliquot of bean powder comprising 50 mg of starch was mixed with 2 ml of deionized water, and then the mixture was heated for 10 min in a boiling water bath. In addition, 10 ml of HCl-KCl buffer (0.1 mol/L, pH 1.5) and 0.2 ml pepsin solution (1 mg/ml, dissolved in 0.1 mol/L of HCl-KCl buffer) were sequentially added to the gelatinized mixture, which was then agitated for 60 min in a water bath at 40°C. Furthermore, 15 ml of PBS (pH 6.9) and 5 ml of α-amylase solution (2.6 U/ml) (pH 6.9) were sequentially added to the mixture, which was then agitated for 180 min in a water bath at 37°C, and aliquots of the digested mixture (1 ml) were collected at intervals of 30 min (0, 30, 60, 90, 120, 150, and 180 min) for GI determination. The white bread was subjected to the above processes except for the gelatinization process (37). The other aliquots of the digested mixture (1 ml) were collected for 0, 20, and 120 min to determine the digestive properties of the starch (38). The adzuki beans were processed in triplicate. These mixtures were instantly heated to denature the α-amylase activity; eventually, the collected digestion mixtures were sequentially cooled and centrifuged (4°C, 8,000 × g, 10 min) before glucose content determination. The DNS colorimetry method was adopted to determine glucose content. The aliquots of the digestion mixture were equivalently mixed with DNS (200 μl). In addition, the mixtures were sequentially processed by heating in boiled water for 6 min and cooling in running water to room temperature before recomposing the volume to 2 ml using deionized water. The absorbances of the reacted mixtures were measured using a spectrophotometer at 540 nm.
2.10 Calculation of starch digestibility properties
The slowly digestible starch (SDS), rapidly digestible starch (RDS), and RS contents were calculated using formula (3), formula (4), and formula (5) (38, 39).
Where C0 represents the initial glucose concentration (mg/ml) before the enzymatic hydrolysis reaction, “C20” and “C120” represent the glucose concentration (mg/ml) released within 20 and 120 min, respectively, “V” refers to the initial volume of the enzymatic hydrolysis, “0.9” is the conversion factor of glucose to starch, and “50” represents the starch content (mg) in the measured sample.
2.11 Calculation of the in vitro glycemic index
The IVGI of adzuki beans was computed as described in previous studies (23, 37). The starch hydrolysis curves were plotted, and the enzymatic hydrolysis time and starch hydrolysis rate were designated as the abscissa and ordinate, respectively.
The starch hydrolysis rate (SR%) was calculated using formula (6):
“Ct” represents the glucose concentration at a time “t” (mg/ml), “C0” represents the glucose concentration at a time “0” (mg/ml), “32.2” represents the initial volume of the enzymatic hydrolysis reaction system (ml), “0.9” is the conversion factor of glucose to starch, and “50” represents the amount of starch in adzuki bean powder.
Formula (7) was used to calculate the enzymatic hydrolysis kinetic constant (k) and the infinite glucose content (C∞) (mg/ml).
The area under the hydrolysis curve (AUC) was delineated using formula (8).
Where “tf” and “t0” represent the last time point (180 min) and the initial time point (0 min) of the enzymatic hydrolysis reaction, respectively.
The IVGI was computed using formula (9).
The calcHI-starch hydrolysis index indicates the AUC sample/AUC white bread standard.
2.12 Statistical analysis
The values were expressed as means ± standard deviation (n = 3) and tested using the IBM SPSS (one-way ANOVA plus post-hoc Tukey’s b test) software (IBM, NY, USA). P-values less than 0.05 indicated a significant difference.
3 Results
3.1 Total phenolics, total flavonoids, and phenolic profile
The TP and TF of adzuki beans are shown in Table 1. The TP ranges from (137.11 ± 4.81) to (154.90 ± 4.45) mg GAE/100 g DW; Te Xiao Li No. 1 has the highest TP content, followed by Qian Xiao Hei, G24, and Gui Nong No. 1. The TF ranges from (279.78 ± 2.54) to (602.00 ± 10.23) mg RE/100 g DW; G24 has the highest TF content. In addition, gallic acid, 2, 3, 4-trihydroxybenzoic acid, chlorogenic acid, catechin, rutin, and p-hydroxybenzoic acid were identified in adzuki beans, as shown in Table 2 and Figure 2. The 2, 3, 4-trihydroxybenzoic acid, chlorogenic acid, and p-hydroxybenzoic acid contents range from (1.19 ± 0.13) to (7.46 ± 0.75) mg/100 g DW, (3.94 ± 0.27) to (7.15 ± 1.08) mg/100 g DW, and (5.96 ± 0.59) to (7.54 ± 0.16) mg/100 g DW, respectively. The catechin and rutin contents range from (12.63 ± 1.38) to (23.05 ± 1.60) mg/100 g DW and (2.41 ± 0.19) to (16.31 ± 2.09) mg/100 g DW, respectively. In Te Xiao Li No. 1, the rutin and catechin contents are dominant with 16.31 ± 2.09 and 23.05 ± 1.60 mg/100 g DW, respectively.
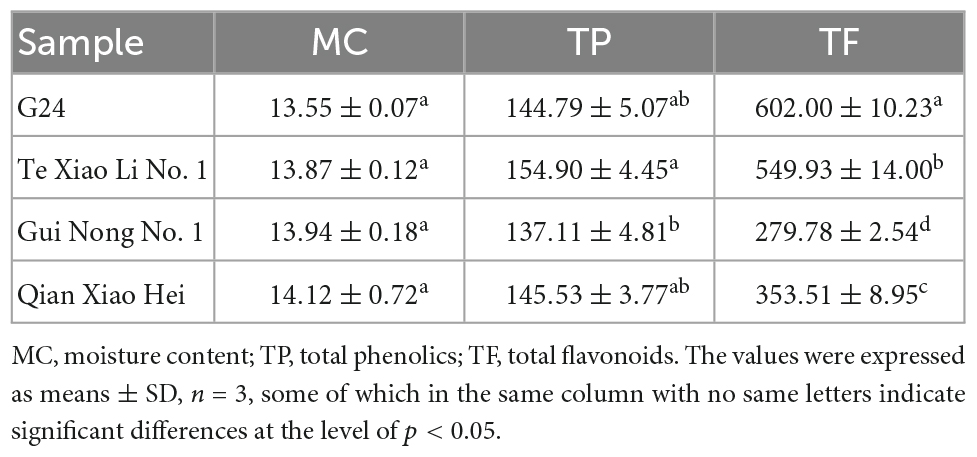
Table 1. Total phenolics (mg GAE/100 g DW), total flavonoids (mg RE/100 g DW), and moisture contents (%) of adzuki beans.
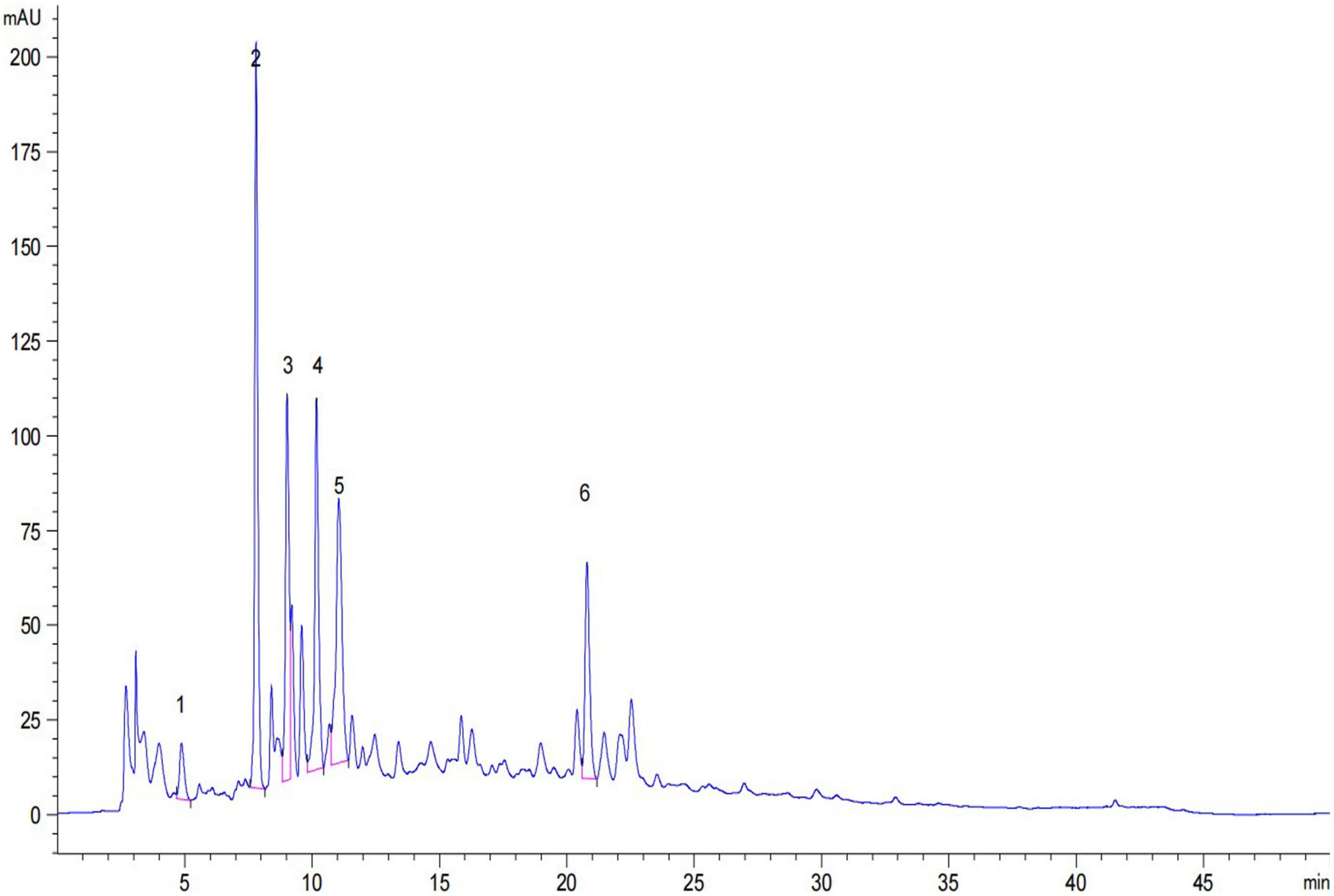
Figure 2. High-performance liquid chromatography (HPLC) chromatogram of individual phenolic compounds in adzuki beans. 1, gallic acid; 2, 2, 3, 4-trihydroxybenzoic acid; 3, chlorogenic acid; 4, catechin; 5, rutin and 6, p-hydroxybenzoic acid.
3.2 α-AI specific activity
The α-AI specific activity indicates the inhibitory efficiency of α-AI to α-amylase in the starch hydrolysis reaction. The α-AI specific activities of adzuki beans range from (1.76 ± 0.04) to (3.41 ± 0.18) U/g. G24 shows the highest activity and Te Xiao Li No. 1 and Gui Nong No. 1 show minor fluctuations; both are ranked intermediate, while Qian Xiao Hei shows the lowest α-AI specific activity (Figure 3).
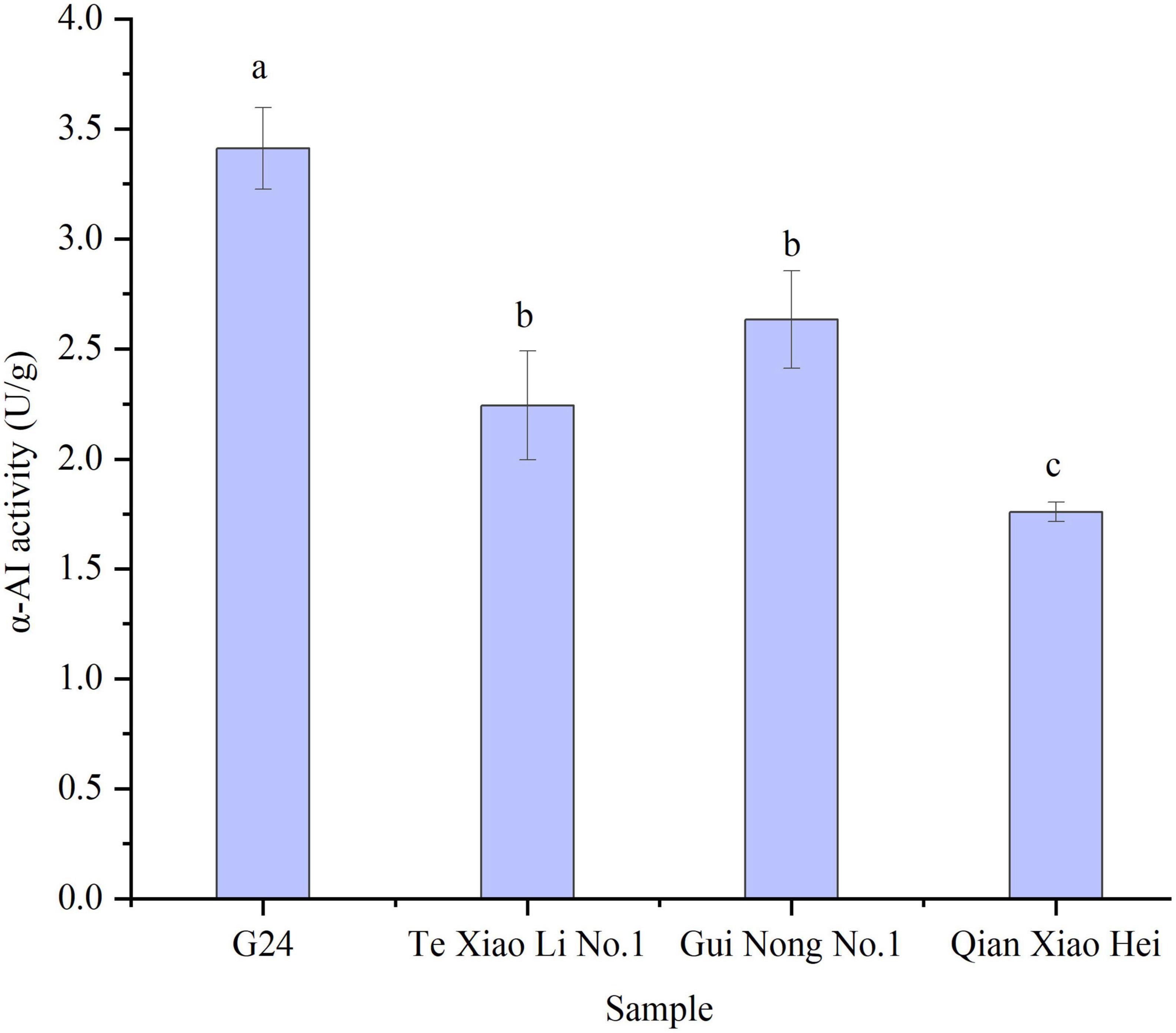
Figure 3. The α-AI specific activities of adzuki beans. Bars with no same letters indicate significant differences at the level of p < 0.05.
3.3 Starch digestibility properties
The starch digestibility properties can be categorized using the digestive process in which the RDS can be digested in 0–20 min, the SDS can be digested in 20–120 min, and the RS cannot be digested after 120 min (39). In adzuki beans, the proportions of RS to TS range from (69.78 ± 2.45%) to (81.03 ± 0.06%); Te Xiao Li No. 1 has the highest proportion of RS to TS, followed by Gui Nong No. 1, Qian Xiao Hei, and G24. The proportions of SDS to TS are between (11.02 ± 1.58%) and (15.57 ± 0.17%), and the proportions of RDS to TS are between (3.38 ± 0.12%) and (18.62 ± 1.81%) (Table 3).
3.4 In vitro glycemic index
The SR was determined using the in vitro digestion model coupled with DNS colorimetry, which was used to plot the starch hydrolysis curves (Figures 4A–D). Using the curves, the enzymatic hydrolysis kinetic constant (k) and the infinite glucose content (C∞) were obtained, and both were eventually used to calculate the GI; the parameters and IVGI are presented in Table 4. The SR of adzuki beans at 180 min ranges from (17.02 ± 0.33%) to (26.60 ± 1.81%). Qian Xiao Hei, Gui Nong No. 1, and Te Xiao Li No. 1 present a low SR in the whole hydrolysis period. The IVGI of adzuki beans ranges from (39.00 ± 0.36) to (56.76 ± 4.21), and Te Xiao Li No. 1 presents the lowest IVGI.
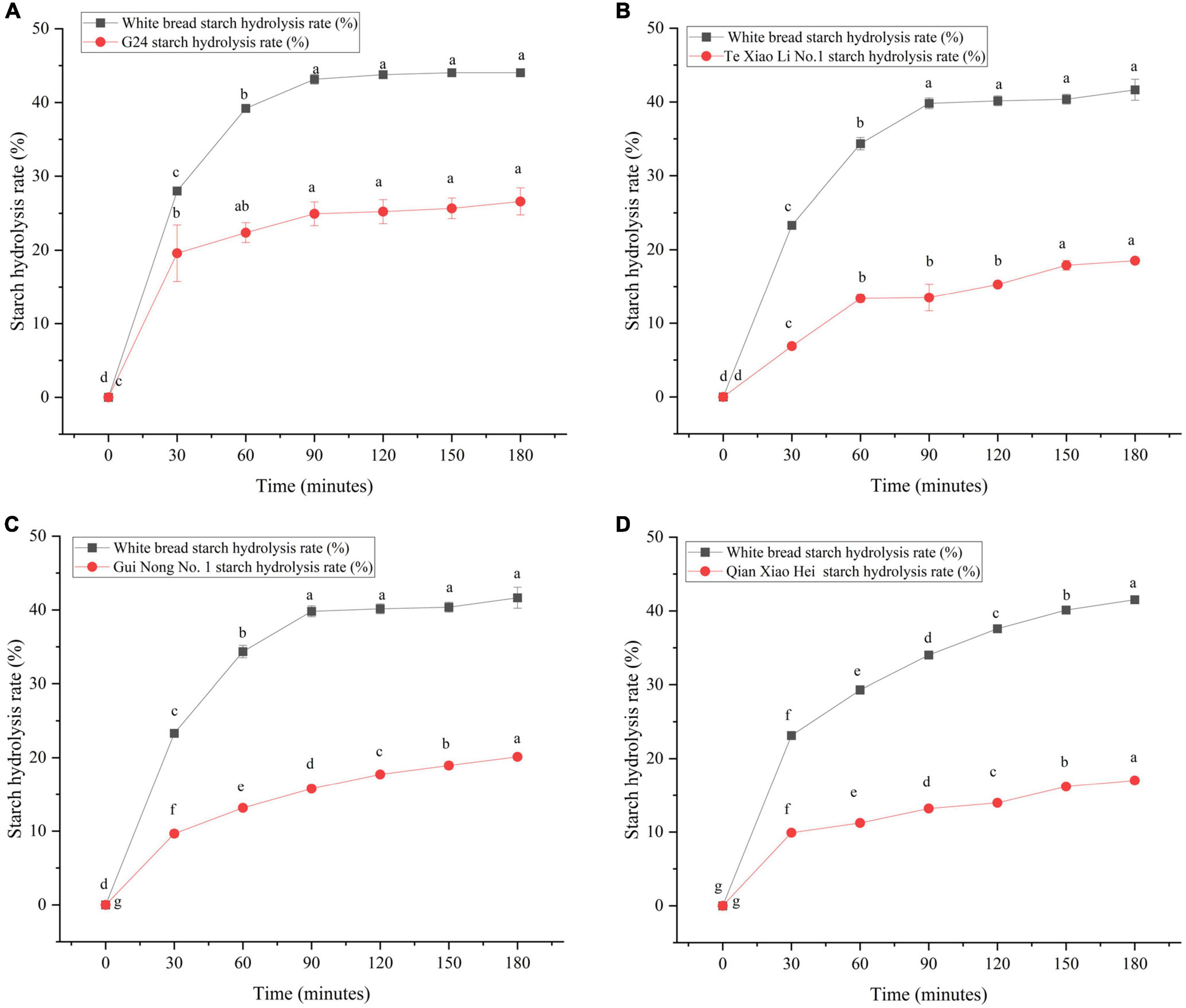
Figure 4. Dynamic changes of starch hydrolysis rate of adzuki beans. Panel (A) exhibits the starch hydrolysis curves of G24 and white bread, panel (B) exhibits the starch hydrolysis curves of Te Xiao Li No. 1 and white bread, panel (C) exhibits the starch hydrolysis curves of Gui Nong No. 1 and white bread, and panel (D) exhibits the starch hydrolysis curves of Qian Xiao Hei and white bread. Bars in the same curves with no same letters indicate significant differences at the level of p < 0.05.
4 Discussion
Abundant phenolics were identified in the seed coats of beans, and intrinsic anthocyanins exist in colored beans (40, 41). Phenolic acids and flavonoids are the dominant subclasses of phenolic compounds; phenolic acids can be divided into two major categories, namely hydroxybenzoic acids and hydroxycinnamic acids (42). Flavonoids are polyphenolic-secondary metabolites containing a 15-carbon atom structure in which phenyl rings (A and B) are coupled with a heterocyclic ring (C) (43). Phenolic acids and flavonoids are the dominant phenolics in plant-based foods, and flavonoids existing in free and glycosidic forms are the most common type in plants (40). In the present study, the TP of adzuki beans was higher than that of red and black beans in a previous study (44). In the present study, the TP of adzuki beans was lower than their counterpart reported by previous studies (45, 46), while the TP of common beans (0.72–1.04 mg GAE/g) reported by a previous study (47) was lower than that reported by the present study. The TP and TF of various adzuki beans ranged from (2.11 ± 0.01) to (2.75 ± 0.04) GAE/g of DW and (59.17 ± 2.06) to (70.41 ± 1.32) RE/g of DW, respectively (48), which were higher than those of the present study. In addition, the TF of G24 was higher than that of green beans (49). Previous studies have suggested that the consumption of beans can provide numerous health benefits to humans, such as lowering the risks of cardiovascular disease, obesity, type 2 diabetes, and different types of malignant growths (50, 51). Thus, beans also possesses inhibitory activities to α-amylase and α-glucosidase (52, 53), which are positively associated with type 2 diabetes.
The 2, 3, 4-trihydroxybenzoic acid and chlorogenic acid contents of Te Xiao Li No. 1 were higher than those of legumes as reported by a previous study, in which gallic acid was the dominant phenolic with the contents between (0.39 ± 0.00) and (479.26 ± 10.40) μg/g (45), whereas the gallic acid content of Te Xiao Li No. 1 and Qian Xiao Hei was 0.71 ± 0.03 and 0.73 ± 0.04 mg/100 g DW, respectively. Moreover, gallic acid was not detected in a previous study (46). The variations may be attributed to the differences in varieties, genomics, geographical, and environmental conditions (54, 55). Previous studies have suggested that gallic acid, chlorogenic acid, p-hydroxybenzoic acid, and caffeic acid in beans have positive influences on type 2 diabetes (56, 57). In the present study, Te Xiao Li No. 1 presented the highest catechin and rutin contents; a previous study suggested that catechin in dietary beans presented an inhibitory response toward fungal amylase (58). Furthermore, catechin showed inhibitory activities to α-amylase, lipase, and α-glucosidase, which is directly linked with blood sugar content (10, 59, 60).
Alpha-amylase is the key enzyme that hydrolyzes carbohydrates to disaccharides and oligosaccharides in the gastrointestinal tract, both of which are further degraded into monosaccharides or simple sugars by α-glucosidase, resulting in an increased level of postprandial glucose (61). α-AI (α-AI-1, α-AI-2, α-AI-3, and α-AI-L) is a functional component of beans, which presents anti-hyperglycemic activities. The most common isoforms of α-AIs isolated from beans are α-AI-1, α-AI-2, and α-AI-L (62–66). α-AI is a glycoprotein containing active inhibitory subunits, which are named α- and β-chains; the α-chain lacks tryptophan and the β-chain lacks methionine, while cysteine is absent in both of them (67, 68). Previous studies have suggested that phenolics also show inhibitory activity to α-amylase (15, 69), which indicates that the α-AI specific activity of adzuki beans may be attributed to glycoprotein and phenolics. A previous study indicated that the water extract of adzuki beans presents an α-AI activity to α-amylases and α-glucosidases, providing health benefits to type 2 diabetes patients (70). Alpha-amylase contains an active site for digesting starch, which is completely blocked by α-AI (62); the quantity of the inhibitor that colonizes the active site should be defined. A certain amount of sample is required to maintain the inhibition rate of enzymes (71).
Alpha-D-glucopyranosyl linked with α-D- (1–4) or α-D- (1–6) linkages are the basic units of starches, which are composed of amylose and amylopectin (72, 73). Previous studies have shown that the abundant RS in legumes is associated with postprandial blood glucose content, contributing to a positive effect on type 2 diabetes (74, 75). In the present study, the proportions of RS to TS in adzuki beans ranged from (69.78 ± 2.45%) to (81.03 ± 0.06%), which was higher than the results reported by previous studies (38, 76, 77). RS can hardly be digested in the stomach and small intestine (8), and it is eventually fermented by gut microbiota (78), producing short-chain fatty acids in the colon, which impose positive effects on type 2 diabetes (21, 79).
GI indicates the sugar level in the blood after the consumption of certain foods, and can be classified into three categories, namely low GI (<55), medium GI (56–69), and high GI (70–100) (80). In the present study, adzuki beans can be classified as low- or medium-GI foods and the IVGI ranges from (39.00 ± 0.36) to (56.76 ± 4.21); Te Xiao Li No. 1 presented the lowest IVGI, and the IVGI of adzuki beans in ascending order is Qian Xiao Hei < Gui Nong No. 1 < G24. The GIs of these adzuki beans were lower than those of whole beans (81), mung beans (82), and adzuki beans (83). Previous studies have suggested that phenolics impose positive effects on postprandial blood glucose levels in aspects of inhibiting amylase activities, decreasing glucose absorption, and stimulating insulin secretion. Furthermore, phenolics may also reduce the glucose content by suppressing glucose release in the liver, which is regulated by the intracellular signal pathway (84). The IVGIs of these adzuki beans may be due to the whole effects of endogenous functional components. Phenolics, α-amylase inhibitors, and RS are the dominant contributors to the delays in blood sugar responses after the consumption of adzuki beans. In addition, the IVGI of Qian Xiao Hei (black-colored) was comparable with Te Xiao Li No. 1 (red-colored); however, the phenolic profile, α-AI specific activity, and RS proportion of Qian Xiao Hei were not close to those of Te Xiao Li No. 1. This finding indicates that Qian Xiao Hei may contain some unidentified endogenous components, which may contribute to low GI.
5 Conclusion
In the present study, adzuki beans contain numerous phenolics, including gallic acid, 2, 3, 4-trihydroxybenzoic acid, chlorogenic acid, catechin, rutin, and p-hydroxybenzoic acid; of which catechin is the dominant phenolic compound in Te Xiao Li No. 1 and G24 varieties. The TP of Te Xiao Li No. 1 is higher than that of the other varieties, and the TF of G24 is higher than that of the other varieties. In addition, α-amylase inhibitors and RS are also present in adzuki beans. These adzuki beans are classified as low- or medium-GI foods, which may be caused by the whole effects of endogenous functional components. Phenolics, α-amylase inhibitors, and RS are the dominant contributors to the delays in starch digestion. These results indicate that these adzuki beans can be used as components of low-GI foods.
Data availability statement
The original contributions presented in this study are included in the article/supplementary material, further inquiries can be directed to the corresponding author.
Author contributions
MM: data curation, validation, writing—original draft, software, formal analysis, and investigation. LQ: investigation, resources, methodology, supervision, conceptualization, project administration, and funding acquisition. YZ: resources, writing—review and editing, and funding acquisition. AW and NL: formal analysis. All authors contributed to the article and approved the submitted version.
Funding
This research was supported by Guizhou Science and Technology Program Qian Ke He Ping Tai Ren Cai (Grant Nos. [2018] 5254 and [2018] 5781), Qian Ke He Zhicheng (Grant No. [2022] Zhongdian007), Qian Ke Zhong Yin Di (Grant No. [2022] 4003), Qian Ke He Zhicheng (Grant No. [2021] yiban175), Qian Ke He Jichu (Grant No. [2019] 1071), Qian Ke He Zhicheng (Grant No. [2020] 1Y171), Establishment of Guizhou Modern Agriculture Industry Technical System (Characteristic Coarse Cereals) (Grant No. Qian Cai Nong [2018] 81), and Key Agricultural Technology Extension Project of Guizhou Province (Grant No. [2017] 106).
Acknowledgments
We thank the “Guizhou Bijie Institute of Agricultural Sciences of China” for providing experimental material.
Conflict of interest
The authors declare that the research was conducted in the absence of any commercial or financial relationships that could be construed as a potential conflict of interest.
Publisher’s note
All claims expressed in this article are solely those of the authors and do not necessarily represent those of their affiliated organizations, or those of the publisher, the editors and the reviewers. Any product that may be evaluated in this article, or claim that may be made by its manufacturer, is not guaranteed or endorsed by the publisher.
Abbreviations
α -AI, alpha-amylase inhibitor; ELISA, enzyme-linked immunosorbent assay; Na2CO3, sodium carbonate; NaNO2, sodium nitrite; AlCl3.6H2O, aluminum chloride hexahydrate; DNS, 3,5-dinitrosalicylic acid; PBS, phosphate buffered saline; HPLC, high-performance liquid chromatography; AUC, area under the curve; TP, total phenolics; TF, total flavonoids; DW, dry weight; IR, inhibition rate; IVGI, in vitro glycemic index; HI, hydrolysis index; SPSS, statistical product service solutions; ANOVA, analysis of variance.
References
1. Li H, Zou L, Li X, Wu D, Liu H, Li H, et al. Adzuki bean (Vigna angularis): chemical compositions, physicochemical properties, health benefits, and food applications. Compr Rev Food Sci Food Saf. (2022). 21:2335–62.
2. Zhao P, Chu L, Wang K, Zhao B, Li Y, Yang K, et al. Analyses on the pigment composition of different seed coat colors in adzuki bean. Food Sci Nutr. (2022) 10:2611–9. doi: 10.1002/fsn3.2866
3. Pandiyan M, Sivakumar P, Krishnaveni A, Sivakumar C, Radhakrishnan V, Vaithiyalingam M, et al. Adzuki bean. In: Pratap A, Sanjeev Gupta F editors. The beans and the peas. Amsterdam, NL: Elsevier (2021). p. 89–103. doi: 10.1016/B978-0-12-821450-3.00006-8
4. Yousif A, Kato J, Deeth H. Effect of storage on the biochemical structure and processing quality of adzuki bean (Vigna angularis). Food Rev Int. (2007) 23:1–33. doi: 10.1080/87559120600865172
5. Capriles V, Coelho K, Guerra-Matias A, Arêas J. Effects of processing methods on amaranth starch digestibility and predicted glycemic index. J Food Sci. (2008) 73:H160–4. doi: 10.1111/j.1750-3841.2008.00869.x
6. Eleazu C, Eleazu K, Iroaganachi M, Kalu W. Starch digestibility and predicted glycemic indices of raw and processed forms of hausa potato (Solenostemon rotundifolius poir). J Food Biochem. (2017) 41:e12355. doi: 10.1111/jfbc.12355
7. Bednar G, Patil A, Murray S, Grieshop C, Merchen N, Fahey G Jr. Starch and fiber fractions in selected food and feed ingredients affect their small intestinal digestibility and fermentability and their large bowel fermentability in vitro in a canine mode. J Nutr. (2001) 131:276–86. doi: 10.1093/jn/131.2.276
8. Tan F, Beltranena E, Zijlstra R. Resistant starch: implications of dietary inclusion on gut health and growth in pigs: a review. J Anim Sci Biotechnol. (2021) 12:124. doi: 10.1186/s40104-021-00644-5
9. Damián-Medina K, Salinas-Moreno Y, Milenkovic D, Figueroa-Yáñez L, Marino-Marmolejo E, Higuera-Ciapara I, et al. In silico analysis of antidiabetic potential of phenolic compounds from blue corn (Zea mays L.) and black bean (Phaseolus vulgaris L.). Heliyon. (2020) 6:e03632. doi: 10.1016/j.heliyon.2020.e03632
10. Zhang B, Deng Z, Ramdath D, Tang Y, Chen P, Liu R, et al. Phenolic profiles of 20 Canadian lentil cultivars and their contribution to antioxidant activity and inhibitory effects on α-glucosidase and pancreatic lipase. Food Chem. (2015) 172:862–72. doi: 10.1016/j.foodchem.2014.09.144
11. Beninger C, Hosfield G. Antioxidant activity of extracts, condensed tannin fractions, and pure flavonoids from Phaseolus vulgaris L. seed coat color genotypes. J Agric Food Chem. (2003) 51:7879–83. doi: 10.1021/jf0304324
12. Aparicio-Fernández X, Manzo-Bonilla L, Loarca-Piña GF. Comparison of antimutagenic activity of phenolic compounds in newly harvested and stored common beans Phaseolus vulgaris against aflatoxin B1. J Food Sci. (2005) 70:S73–8. doi: 10.1111/j.1365-2621.2005.tb09068.x
13. Roopchand D, Kuhn P, Rojo L, Lila M, Raskin I. Blueberry polyphenol-enriched soybean flour reduces hyperglycemia, body weight gain and serum cholesterol in mice. Pharmacol Res. (2013) 68:59–67. doi: 10.1016/j.phrs.2012.11.008
14. Yang M, Zhang X, Ma Y, Shen J, Song J, Zhu H. Purification and partial characterization of a glycoprotein alpha-amylase inhibitor from white kidney bean (phaseolus vulgaris. L). J Food Biochem. (2008) 32:72–84. doi: 10.1111/j.1745-4514.2007.00147.x
15. Chinedum E, Sanni S, Theressa N, Ebere A. Effect of domestic cooking on the starch digestibility, predicted glycemic indices, polyphenol contents and alpha amylase inhibitory properties of beans (Phaseolis vulgaris) and breadfruit (Treculia africana). Int J Biol Macromol. (2018) 106:200–6. doi: 10.1016/j.ijbiomac.2017.08.005
16. García-Alonso A, Goni I, Saura-Calixto F. Resistant starch and potential glycaemic index of raw and cooked legumes (lentils, chickpeas and beans). Zeitschrift für Lebensmitteluntersuchung und-Forschung A. (1998) 206:284–7. doi: 10.1007/s002170050258
17. Xu S, Qin L, Mazhar M, Zhu Y. Functional components profile and glycemic index of kidney beans. Front Nutr. (2022) 9:1044427. doi: 10.3389/fnut.2022.1044427
18. Sterrett J, Bragg S, Weart C. Type 2 diabetes medication review. Am J Med Sci. (2016) 351:342–55. doi: 10.1016/j.amjms.2016.01.019
19. Hartman T, Albert P, Zhang Z, Bagshaw D, Kris-Etherton P, Ulbrecht J, et al. Consumption of a legume-enriched, low-glycemic index diet is associated with biomarkers of insulin resistance and inflammation among men at risk for colorectal cancer. J Nutr. (2010) 140:60–7. doi: 10.3945/jn.109.114249
20. Grundy S. Pre-diabetes, metabolic syndrome, and cardiovascular risk. J Am Coll Cardiol. (2012) 59:635–43. doi: 10.1016/j.jacc.2011.08.080
21. Wong T, Louie J. The relationship between resistant starch and glycemic control: a review on current evidence and possible mechanisms. Starch-Stärke. (2017) 68:1600205. doi: 10.1002/star.201600205
22. Villegas R, Gao Y, Yang G, Li H, Elasy T, Zheng W, et al. Legume and soy food intake and the incidence of type 2 diabetes in the shanghai women’s health study. Am J Clin Nutr. (2008) 87:162–7. doi: 10.1093/ajcn/87.1.162
23. Ma Y, Rang Y, Yang R, Zhao W. Effect of white kidney bean extracts on estimated glycemic index of different kinds of porridge. LWT Food Sci Technol. (2018) 96:576–82. doi: 10.1016/j.lwt.2018.06.018
24. Udani J, Singh B, Barrett M, Preuss H. Lowering the glycemic index of white bread using a white bean extract. Nutr J. (2009) 8:1–5. doi: 10.1186/1475-2891-8-52
25. Liu S, Willett W, Stampfer M, Hu F, Franz M, Sampson L, et al. A prospective study of dietary glycemic load, carbohydrate intake, and risk of coronary heart disease in US women. Am J Clin Nutr. (2000) 71:1455–61. doi: 10.1093/ajcn/71.6.1455
26. Schulze M, Liu S, Rimm E, Manson J, Willett W, Hu F. Glycemic index, glycemic load, and dietary fiber intake and incidence of type 2 diabetes in younger and middle-aged women. Am J Clin Nutr. (2004) 80:348–56. doi: 10.1093/ajcn/80.2.348
27. Augustin L, Dal Maso L, La Vecchia C, Parpinel M, Negri E, Vaccarella S, et al. Dietary glycemic index and glycemic load, and breast cancer risk: a case-control study. Ann Oncol. (2001) 12:1533–8. doi: 10.1023/A:1013176129380
28. Franceschi S, Dal Maso L, Augustin L, Negri E, Parpinel M, Boyle P, et al. Dietary glycemic load and colorectal cancer risk. Ann Oncol. (2001) 12:173–8. doi: 10.1023/A:1008304128577
29. Li H, Tsao R, Deng Z. Factors affecting the antioxidant potential and health benefits of plant foods. Can J Plant Sci. (2012) 92:1101–11. doi: 10.4141/cjps2011-239
30. Adom K, Liu R. Antioxidant activity of grains. J Agric Food Chem. (2002) 50:6182–7. doi: 10.1021/jf0205099
31. De La Parra C, Serna Saldivar S, Liu R. Effect of processing on the phytochemical profiles and antioxidant activity of corn for production of masa, tortillas, and tortilla chips. J Agric Food Chem. (2007) 55:4177–83. doi: 10.1021/jf063487p
32. Zhishen J, Mengcheng T, Jianming W. The determination of flavonoid contents in mulberry and their scavenging effects on superoxide radicals. Food Chem. (1999) 64:555–9. doi: 10.1016/S0308-8146(98)00102-2
33. Bakar M, Mohamed M, Rahmat A, Fry J. Phytochemicals and antioxidant activity of different parts of bambangan (Mangifera pajang) and tarap (Artocarpus odoratissimus). Food Chem. (2009) 113:479–83. doi: 10.1016/j.foodchem.2008.07.081
34. Zhu Y, Li T, Fu X, Abbasi A, Zheng B, Liu R. Phenolics content, antioxidant and antiproliferative activities of dehulled highland barley (Hordeum vulgare L.). J Funct Foods. (2015) 19:439–50. doi: 10.1016/j.jff.2015.09.053
35. Chen Y, Qin L, Wen A, Mazhar M, Wang H, Zhu Y. Three-solvent extracting method comprehensively evaluates phenolics profile and antioxidant activities of Tartary buckwheat. J Food Process Preserv. (2021) 45:e15020. doi: 10.1111/jfpp.15020
36. National Health and Family Planning Commission of the People’s Republic of China, State Food and Drug Administration [SFDA]. National standards of the people’s republic of china method for the determination of starch in food in: GB/5009.9- 2016 GB/5009.9-2016. Beijing: China Standard Press (2016).
37. Ferrer-Mairal A, Penalva-Lapuente C, Iglesia I, Urtasun L, Miguel-Etayo D, Remón S, et al. In vitro and in vivo assessment of the glycemic index of bakery products: influence of the reformulation of ingredients. Eur J Nutr. (2012) 51:947–54. doi: 10.1007/s00394-011-0272-6
38. Wu X, Yu H, Bao G, Luan M, Wang C. Preparation of adzuki bean starch-lipid complexes and their anti-digestion mechanism. J Food Meas Charact. (2022) 16:945–56. doi: 10.1007/s11694-021-01222-z
39. Li Y, Xiao J, Tu J, Yu L, Niu L. Matcha-fortified rice noodles: characteristics of in vitro starch digestibility, antioxidant and eating quality. LWT Food Sci Technol. (2021) 149:111852. doi: 10.1016/j.lwt.2021.111852
40. Ganesan K, Xu B. Polyphenol-rich dry common beans (Phaseolus vulgaris L.) and their health benefits. Int J Mol Sci. (2017) 18:2331. doi: 10.3390/ijms18112331
41. Sahasakul Y, Aursalung A, Thangsiri S, Wongchang P, Sangkasa-Ad P, Wongpia A, et al. Nutritional compositions, phenolic contents, and antioxidant potentials of ten original lineage beans in Thailand. Foods. (2022) 11:2062. doi: 10.3390/foods11142062
42. Heleno S, Martins A, Queiroz M, Ferreira I. Bioactivity of phenolic acids: metabolites versus parent compounds: a review. Food Chem. (2015) 173:501–13. doi: 10.1016/j.foodchem.2014.10.057
43. De Souza Farias S, Da Costa K, Martins J. Analysis of conformational, structural, magnetic, and electronic properties related to antioxidant activity: revisiting flavan, anthocyanidin, flavanone, flavonol, isoflavone, flavone, and flavan-3-ol. ACS Omega. (2021) 6:8908–18. doi: 10.1021/acsomega.0c06156
44. Ombra M, D’acierno A, Nazzaro F, Riccardi R, Spigno P, Zaccardelli M, et al. Phenolic composition and antioxidant and antiproliferative activities of the extracts of twelve common bean (Phaseolus vulgaris L.) endemic ecotypes of Southern Italy before and after cooking. Oxid Med Cell Longev. (2016) 2016:1398298. doi: 10.1155/2016/1398298
45. Zhang Y, Meenu M, Yu H, Xu B. An investigation on phenolic and antioxidant capacity of under-utilized food legumes consumed in China. Foods. (2020) 9:438. doi: 10.3390/foods9040438
46. Khang D, Dung T, Elzaawely A, Xuan T. Phenolic profiles and antioxidant activity of germinated legumes. Foods. (2016) 5:27. doi: 10.3390/foods5020027
47. Wodajo D, Emire S. Haricot beans (Phaseolus vulgaris L.) flour: effect of varieties and processing methods to favor the utilization of underconsumed common beans. Int J Food Prop. (2022) 25:1186–202. doi: 10.1080/10942912.2022.2074029
48. Shi Z, Yao Y, Zhu Y, Ren G. Nutritional composition and biological activities of 17 Chinese adzuki bean (Vigna angularis) varieties. Food Agric Immunol. (2017) 28:78–89. doi: 10.1080/09540105.2016.1208152
49. Zhang X, Shang P, Qin F, Zhou Q, Gao B, Huang H, et al. Chemical composition and antioxidative and anti-inflammatory properties of ten commercial mung bean samples. LWT Food Sci Technol. (2013) 54:171–8. doi: 10.1016/j.lwt.2013.05.034
50. Liu R. Whole grain phytochemicals and health. J Cereal Sci. (2007) 46:207–19. doi: 10.1016/j.jcs.2007.06.010
51. Chung H, Liu Q, Pauls K, Fan M, Yada R. In vitro starch digestibility, expected glycemic index and some physicochemical properties of starch and flour from common bean (Phaseolus vulgaris L.) varieties grown in Canada. Food Res Int. (2008) 41:869–75. doi: 10.1016/j.foodres.2008.03.013
52. Mojica L, Meyer A, Berhow M, De Mejía E. Bean cultivars (Phaseolus vulgaris L.) have similar high antioxidant capacity, in vitro inhibition of α-amylase and α-glucosidase while diverse phenolic composition and concentration. Food Res Int. (2015) 69:38–48. doi: 10.1016/j.foodres.2014.12.007
53. Mojica L, Berhow M, De Mejia E. Black bean anthocyanin-rich extracts as food colorants: physicochemical stability and antidiabetes potential. Food Chem. (2017) 229:628–39. doi: 10.1016/j.foodchem.2017.02.124
54. Dinelli G, Bonetti A, Minelli M, Marotti I, Catizone P, Mazzanti A. Content of flavonols in Italian bean (Phaseolus vulgaris L.) ecotypes. Food Chem. (2006) 99:105–14. doi: 10.1016/j.foodchem.2005.07.028
55. Gan R, Li H, Gunaratne A, Sui Z, Corke H. Effects of fermented edible seeds and their products on human health: bioactive components and bioactivities. Compr Rev Food Sci Food Saf. (2017) 16:489–531. doi: 10.1111/1541-4337.12257
56. Ranilla L, Kwon Y, Genovese M, Lajolo F, Shetty K. Effect of thermal treatment on phenolic compounds and functionality linked to type 2 diabetes and hypertension management of Peruvian and Brazilian bean cultivars (Phaseolus vulgaris L.) using in vitro methods. J Food Biochem. (2010) 34:329–55. doi: 10.1111/j.1745-4514.2009.00281.x
57. Pérez-Ramírez I, Becerril-Ocampo L, Reynoso-Camacho R, Herrera M, Guzmán-Maldonado S, Cruz-Bravo R. Cookies elaborated with oat and common bean flours improved serum markers in diabetic rats. J Sci Food Agric. (2018) 98:998–1007. doi: 10.1002/jsfa.8548
58. Telles A, Kupski L, Furlong E. Phenolic compound in beans as protection against mycotoxins. Food Chem. (2017) 214:293–9. doi: 10.1016/j.foodchem.2016.07.079
59. Yue J, Xu J, Cao J, Zhang X, Zhao Y. Cucurbitane triterpenoids from Momordica charantia L. and their inhibitory activity against α-glucosidase, α-amylase and protein tyrosine phosphatase 1B (PTP1B). J Funct Foods. (2017) 37:624–31. doi: 10.1016/j.jff.2017.07.041
60. Kuriya K, Nishio M, Ono N, Masuda Y, Katsuzaki H, Kondo S, et al. Isolation and characterization of antihyperglycemic compounds from Vigna angularis extracts. J Food Sci. (2019) 84:3172–8. doi: 10.1111/1750-3841.14840
61. Ortiz-Andrade R, Garcia-Jimenez S, Castillo-Espana P, Ramirez-Avila G, Villalobos-Molina R, Estrada-Soto S. α-Glucosidase inhibitory activity of the methanolic extract from Tournefortia hartwegiana: an anti-hyperglycemic agent. J Ethnopharmacol. (2007) 109:48–53. doi: 10.1016/j.jep.2006.07.002
62. Obiro W, Zhang T, Jiang B. The nutraceutical role of the Phaseolus vulgaris α-amylase inhibitor. Br J Nutr. (2008) 100:1–12. doi: 10.1017/S0007114508879135
63. Oliveira R, De Oliveira V, Deconte S, Calábria L, Da Silva Moraes A, Espindola F. Phaseolamin treatment prevents oxidative stress and collagen deposition in the hearts of streptozotocin-induced diabetic rats. Diab Vasc Dis Res. (2014) 11:110–7. doi: 10.1177/1479164114521643
64. Uddin N, Hasan M, Hossain M, Sarker A, Hasan A, Islam A, et al. In vitro α-amylase inhibitory activity and in vivo hypoglycemic effect of methanol extract of Citrus macroptera Montr. fruit. Asian Pac J Trop Biomed. (2014) 4:473–9. doi: 10.12980/APJTB.4.2014C1173
65. Brain-Isasi S, Álvarez-Lueje A, Higgins T. Heterologous expression of an α-amylase inhibitor from common bean (Phaseolus vulgaris) in Kluyveromyces lactis and Saccharomyces cerevisiae. Microb Cell Fact. (2017) 16:110. doi: 10.1186/s12934-017-0719-4
66. Habib H, Theuri S, Kheadr E, Mohamed FE. DNA and BSA damage inhibitory activities, and anti-acetylcholinesterase, anti-porcine α-amylase and antioxidant properties of Dolichos lablab beans. Food Funct. (2017) 8:881–7. doi: 10.1039/C6FO01164K
67. Yamaguchi H. Isolation and characterization of the subunits of a heat-labile α-amylase inhibitor from Phaseolus vulgaris white kidney bean. Biosci Biotechnol Biochem. (1993) 57:297–302. doi: 10.1271/bbb.57.297
68. Le Berre-Anton V, Bompard-Gilles C, Payan F, Rougé P. Characterization and functional properties of the α-amylase inhibitor (α-AI) from kidney bean (Phaseolus vulgaris) seeds. Biochim Biophys Acta Prot Struct Mol Enzym. (1997) 1343:31–40. doi: 10.1016/S0167-4838(97)00100-3
69. Wang Y, Yao X, Shen H, Zhao R, Li Z, Shen X, et al. Nutritional composition, efficacy, and processing of Vigna angularis (Adzuki bean) for the human diet: an overview. Mol. (2022) 27:6079. doi: 10.3390/molecules27186079
70. Itoh T, Umekawa H, Furuichi Y. Potential ability of hot water adzuki (Vigna angularis) extracts to inhibit the adhesion, invasion, and metastasis of murine B16 melanoma cells. Biosci Biotechnol Biochem. (2005) 69:448–54. doi: 10.1271/bbb.69.448
71. Sabeghi Khosroshahi Z, Abbasipour H, Rezazadeh A. Inhibitory effect of aqueous bean extract, Phaseolus vulgaris (fabaceae), on α-amylase of the cabbage aphid, Brevicoryne brassicae. Arch Agron Soil Sci. (2021) 67:1425–33. doi: 10.1080/03650340.2020.1796982
72. Haralampu S. Resistant starch—a review of the physical properties and biological impact of RS3. Carbohydr Polym. (2000) 41:285–92. doi: 10.1016/S0144-8617(99)00147-2
73. Sharma A, Yadav B, Ritika. Resistant starch: physiological roles and food applications. Food Rev Int. (2008) 24:193–234. doi: 10.1080/87559120801926237
74. Higgins J, Higbee D, Donahoo W, Brown I, Bell M, Bessesen D. Resistant starch consumption promotes lipid oxidation. Nutr Metab. (2004) 1:8. doi: 10.1186/1743-7075-1-8
75. Punia S, Dhull S, Sandhu K, Kaur M, Purewal S. Kidney bean (Phaseolus vulgaris) starch: a review. Legum Sci. (2020) 2:e52. doi: 10.1002/leg3.52
76. Hooper S, Bassett A, Sadohara R, Cichy K. Elucidation of the low resistant starch phenotype in Phaseolus vulgaris exhibited in the yellow bean cebo cela. J Food Sci. (2021) 86:3975–86. doi: 10.1111/1750-3841.15883
77. Sharma V, Kaur M, Sandhu K, Godara S. Effect of cross-linking on physico-chemical, thermal, pasting, in vitro digestibility and film forming properties of faba bean (Vicia faba L.) starch. Int J Biol Macromol. (2020) 159:243–9. doi: 10.1016/j.ijbiomac.2020.05.014
78. Tharanathan R. Food-derived carbohydrates-structural complexity and functional diversity. Crit Rev Biotechnol. (2002) 22:65–84. doi: 10.1080/07388550290789469
79. Den Besten G, Van Eunen K, Groen A, Venema K, Reijngoud D, Bakker B. The role of short-chain fatty acids in the interplay between diet, gut microbiota, and host energy metabolism. J Lipid Res. (2013) 54:2325–40. doi: 10.1194/jlr.R036012
80. Atkinson F, Foster-Powell K, Brand-Miller J. International tables of glycemic index and glycemic load values. Diabet Care. (2008) 31:2281–3. doi: 10.2337/dc08-1239
81. Singh M, Manickavasagan A, Shobana S, Mohan V. Glycemic index of pulses and pulse-based products: a review. Crit Rev Food Sci Nutr. (2021) 61:1567–88. doi: 10.1080/10408398.2020.1762162
82. Nair R, Yang R, Easdown W, Thavarajah D, Thavarajah P, Hughes J, et al. Biofortification of mungbean (Vigna radiata) as a whole food to enhance human health. J Sci Food Agric. (2013) 93:1805–13. doi: 10.1002/jsfa.6110
83. Ren F, Yang X, Wang L, Zhou S. Effects of different processing methods and internal components on physicochemical properties and glycemic index of adzuki bean powder. Foods. (2021) 10:1685. doi: 10.3390/foods10081685
Keywords: adzuki beans, phenolic compounds, alpha-amylase inhibitory activity, resistant starch, in vitro glycemic index
Citation: Muhammad M, Zhu Y, Wen A, Liu N and Qin L (2022) Phenolic profile, alpha-amylase inhibitory activity, and in vitro glycemic index of adzuki beans. Front. Nutr. 9:1063602. doi: 10.3389/fnut.2022.1063602
Received: 07 October 2022; Accepted: 05 December 2022;
Published: 22 December 2022.
Edited by:
Er Sheng Gong, Gannan Medical University, ChinaReviewed by:
Jinle Xiang, Henan University of Science and Technology, ChinaJiangping Ye, Nanchang University, China
Copyright © 2022 Muhammad, Zhu, Wen, Liu and Qin. This is an open-access article distributed under the terms of the Creative Commons Attribution License (CC BY). The use, distribution or reproduction in other forums is permitted, provided the original author(s) and the copyright owner(s) are credited and that the original publication in this journal is cited, in accordance with accepted academic practice. No use, distribution or reproduction is permitted which does not comply with these terms.
*Correspondence: Likang Qin, ✉ lkqin@gzu.edu.cn