- 1State Key Laboratory of Food Nutrition and Safety, College of Food Science and Engineering, Tianjin University of Science and Technology, Tianjin, China
- 2Department of Rehabilitation Medicine, Shandong Provincial Third Hospital, Shandong University, Jinan, Shandong, China
- 3Tianjin International Joint Academy of Biomedicine, Tianjin, China
- 4China-Russia Agricultural Processing Joint Laboratory, Tianjin Agricultural University, Tianjin, China
Introduction: Ulcerative colitis (UC), a chronic non-specific colorectal inflammatory disease with unclear etiology, has long plagued human health. Gut microbiota dysbiosis destroy homeostasis of the colon, which is closely related to ulcerative colitis progress. Apigenin, a flavonoid widely present in celery, has been found to improve ulcerative colitis. However, the potential molecular mechanism of apigenin ameliorating ulcerative colitis through protecting intestinal barrier and regulating gut microbiota remains undefined.
Methods: Dextran sodium sulfate (DSS)-induced colitis mouse model was conducted to evaluate the effect of apigenin on UC. Disease activity index score of mice, colon tissue pathological, cytokines analysis, intestinal tight junction proteins expression, and colonic content short-chain fatty acids (SCFAs) and 16S rRNA gene sequencing were conducted to reflect the protection of apigenin on UC.
Results: The results indicated that apigenin significantly relieved the intestinal pathological injury, increased goblet cells quantity and mucin secretion, promoted anti-inflammatory cytokines IL-10 expression, and inhibited the expression of proinflammatory cytokines, TNF-α, IL-1β, IL-6 and MPO activity of colon tissue. Apigenin increased ZO-1, claudin-1 and occludin expressions to restore the integrity of the intestinal barrier. Moreover, apigenin remodeled the disordered gut microbiota by regulating the abundance of Akkermansia, Turicibacter, Klebsiella, Romboutsia, etc., and its metabolites (SCFAs), attenuating DSS-induced colon injury. We also investigated the effect of apigenin supplementation on potential metabolic pathways of gut microbiota.
Conclusion: Apigenin effectively ameliorated DSS-induced UC via balancing gut microbiome to inhibit inflammation and protect gut barrier. With low toxicity and high efficiency, apigenin might serve as a potential therapeutic strategy for the treatment of UC via regulating the interaction and mechanism between host and microorganism.
1 Introduction
Inflammatory bowel diseases (IBDs) are complex, chronic gastrointestinal inflammatory diseases such as Crohn’s disease (CD) and ulcerative colitis (UC) (1). Hereditary, immune response, and environmental factors are the potential pathogenesis for IBD (2). UC is a chronic non-specific colorectal inflammatory disease, which has a high global incidence (3). The clinical symptoms of UC are abdominal pain, diarrhea, and hematuria. Furthermore, the permeability of the intestinal barrier and the levels of inflammatory cytokines are significantly increased (4). Therefore, it was made one of the most challenging gastrointestinal diseases.
As a sophisticated microbial ecosystem, the gut microbiome has a great influence on the health of the host intestine through dietary fiber fermentation and necessary metabolites. In patients with UC, biodiversity in the gut microbial composition decreased, which manifested as a reduction of beneficial bacteria, for instance, Faecalibacterium and other butyrate producers (5). Short-chain fatty acids (SCFAs), the small molecule metabolic end-product of gut microbiota, are produced in the intestine through the fermentation of proteins and complex carbohydrates. It is confirmed that SCFAs can inhibit intestinal inflammation (6). Mucins, largely secreted by goblet cells, contain many immunomodulatory molecules, thereby protecting the host from invading pathogens (7). Lactobacillus spp. and Akkermansia muciniphila are important bacteria maintaining intestinal mucin homeostasis (8). Therefore, modulating gut microbiota to relieve intestinal inflammation is of clinical importance in UC studies.
Apigenin, a natural plant bioactive compound, is widely found in many fruits, vegetables, and herbs, such as celery. Increasing evidence has proved that apigenin has many biological and pharmacological activities, which can modulate cell proliferation and apoptosis (9), inflammation (10), and cancer development (11). Previous studies revealed that apigenin affects the growth of gut microbiota in mice, and it might be degraded by gut microbiota (12–14). However, the exact underlying mechanism of apigenin in the treatment of UC by modulating gut microbiota has not been clarified.
In this study, to evaluate the effect of apigenin administration on UC, we employed a 2% dextran sulfate sodium (DSS)-induced UC mouse model combined with apigenin intervention. Furthermore, we explored whether apigenin supplementation could alleviate colitis by inhibiting the inflammatory response, protecting the gut barrier, and balancing the gut microbiome structure in colitis mice.
2 Materials and methods
2.1 Animals and induction UC
C57BL/6 mice (6–8 weeks of age, weighing 16–18 g) were acquired from the SPF Biotechnology Co., Ltd. (Beijing, China). Before the experiment, the mice were given 3 days to adapt to the laboratory environment. Throughout the adaptation and study periods, all animals were maintained on a 12-h light-dark cycle (23 ± 1°C with a relatively constant humidity of 50 ± 5%) under specific pathogen-free (SPF) conditions. The animal study was carried out according to the guidelines of the Animal Experimentation Ethics Committee, Tianjin University of Science and Technology.
All the mice were randomly divided into five groups, namely, the control group, model group, positive medicine group (SASP), low-dose apigenin (AP-L) group, and high-dose apigenin (AP-H) group (n = 6 per group). The mice in the control group drank water normally, and experimental mice were administered 2.0% (w/v) dextran sodium sulfate (DSS, MW, 36–50 kDa; Meilunbio, Dalian, China) in drinking water for 23 days to induce experimental colitis. After 2 days, mice in the model groups received 0.5% of CMC-Na, mice in the positive medicine group received 200 mg/kg of SASP, and mice in AP-L and AP-H groups received 150 and 250 mg/kg of apigenin, respectively, which was given by intragastric administration once a day for 21 days.
2.2 Sample collection
During the experiment, mice in each group were weighed every 2 days, and feces were collected to observe their traits. Fecal consistency, fecal occult blood, and weight loss were used for Disease Activity Index (DAI) scores. Mice were sacrificed under 0.5% pentobarbital sodium (50 mg/kg, I.P.) on day 26. Blood was centrifuged at 4,000 r/min at 4°C for 20 min, and serum was stored at −80°C for further analysis. The general morphology of colon samples was photographed. The length of the colon was measured. Colonic contents were collected and stored at −80°C. Colon tissues were fixed in 10% formalin for further analysis.
2.3 H&E and AB-PAS staining
The colon samples were fixed, dehydrated, and embedded in paraffin. Tissues embedded in paraffin were cut into 3 μm sections. Before staining, the sections were deparaffinized and rehydrated. Hematoxylin-eosin (H&E) staining used standard techniques. Eosin (ZSGB-BIO, Beijing, China) was used to stain cytoplasm, and hematoxylin (ZSGB-BIO) was used to stain the nucleus. Alcian blue periodic acid-Schiff (AB-PAS) (LEAGENE, Beijing, China) staining was performed to measure the goblet cell quantity of colon tissue. The sections were stained with alcian blue for 15 min and oxidized with periodic acid for 5 min. Then, the sections were stained with Schiff dye for 15 min. All tissue sections were observed using an optical microscope (Olympus, Tokyo, Japan). Mucosal damage and inflammatory cell infiltration were used as study parameters to evaluate the histological scores.
2.4 ELISA
Colon tissues were ground with physiological saline and centrifuged (3,000 rpm, 20 min) at 4°C. The supernatant was collected for further analysis. Cytokines including TNF-α, IL-6, IL-1β, and IL-10 in colonic tissues were measured by ELISA Kits (mlbio, Shanghai, China) according to the manufacturer’s instructions. Absorbance values were detected with a microplate reader. The cytokine concentration was obtained according to the standard curve.
2.5 Myeloperoxidase
The level of myeloperoxidase (MPO) in colonic tissues was measured by an MPO activity assay kit (mlbio, Shanghai, China). The detection method was carried out according to the manufacturer’s instructions, and the absorbance value was measured at 460 nm.
2.6 Western blotting
Colon tissues were washed with phosphate-buffered saline (PBS) and lysed fully in ice-cold lysis buffer with the Protease Inhibitor Cocktail (MCE, New Jersey, USA) on ice. Lysates were separated by electrophoresis and transferred onto polyvinylidene difluoride membranes (Millipore, Massachusetts, USA). The membranes were blocked with 5% skim milk powder and incubated with primary antibody against ZO-1 (1:1,000, Bioss), Occludin (1:500, Bioss, Beijing, China), Claudin1 (1:1,000, Bioss), and GAPDH (1:25,000, Proteintech, Chicago, USA) at 4°C overnight and then incubated with a horseradish peroxidase-conjugated goat anti-mouse/rabbit IgG secondary antibody (1:5,000, YEASEN, Shanghai, China) for 1 h at room temperature. The intensity of protein was assessed using a chemiluminescent imaging system (Image Quant LAS 4000, Atlanta, USA).
2.7 Immunohistochemistry staining and analysis
Immunohistochemistry (IHC) was conducted according to the previously former method (15). Colon tissue embedded in paraffin was sliced (3 μm) and then deparaffinized and rehydrated with dimethyl-benzene and alcohol. Next, samples were incubated with 3% H2O2 for 10 min to block endogenous peroxidase activity and then blocked with goat serum (Proteintech, Chicago, USA) for 1 h. After being blocked, the samples were incubated with the following primary antibodies overnight at 4°C: ZO-1 (1:250, Bioss, Beijing, China), Occludin (1:400, Bioss), and Claudin1 (1:200, Bioss). The tissues were washed three times with PBS for 5 min each time. After that, the primary antibody in the negative group was displaced by PBS. The secondary antibody was subsequently added with the horseradish peroxidase-polymer anti-mouse/rabbit IHC kit (Maixin Biotech, Fuzhou, China) for 1 h at room temperature. 3,3′-Diaminobenzidine-tetrahydrochloride (DAB) was used to stain the tissues and counterstained with hematoxylin. All the samples were observed with a microscope (Olympus, Tokyo, Japan). The immunohistochemistry score was calculated by multiplying the intensity (0 = negative, 1 = canary yellow, 2 = claybank, 3 = brown) and the positive cell percentage scores (1 = less than 25%, 2 = 25–50%, 3 = 51–75%, 4 = more than 75%).
2.8 Short-chain fatty acids
The short-chain fatty acids in the colonic contents were measured by GC-MS (SHIMADZU, GCMS-QP2010 Ultral, Kyoto, Japan). The method of SCFA determination is according to a previously described method (16). After the fecal samples were dissolved and homogenized, the mixture of sulfuric acid and ether was added to extract SCFAs and then centrifuged at 4°C, 12,000 rpm for 15 min to prepare the samples. Then, anhydrous sodium sulfate was added to the supernatant and centrifuged at 4°C (12,000 rpm, 15 min). The supernatant was collected and injected into GC-MS. The standard SCFAs are a mixture of acetic acid, propionic acid, butyric acid, isobutyric acid, valeric acid, and isovaleric acid (MACKLIN, Shanghai, China).
2.9 16S rRNA gene sequencing
Genomic DNA was extracted from colonic contents. DNA pyrosequencing of the 16S rRNA V3-V4 region was completed in Novogene Co., Ltd. (Beijing, China). The primers 341F and 806R (forward 5′-CCTAYGGGRBGCASCAG-3′ and reward 5′-GGACTACNNGGGTATCTAAT-3′) were used to amplify DNA. Bioinformatics analysis was performed on the Illumina MiSeq platform of Novogene.
2.10 Statistical analysis
All data are presented as means ± SD. ANOVA test (one-way or two-way, with post-hoc tests) was performed using GraphPad Prism 7.0 software (*P ≤ 0.05, **P ≤ 0.01).
3 Results
3.1 Apigenin protects against UC in mice
To investigate whether apigenin has a therapeutic effect on UC, mice were treated with 2.0% DSS in drinking water, while apigenin was administered by gavage from day 3 to day 24 (Figure 1A). As expected, DSS markedly reduced the body weight compared to normal mice. In contrast, SASP significantly increased body weights, and apigenin ameliorated weight loss in a dose-dependent manner in mice induced by DSS (Figure 1B) (P < 0.01). DAI score based on stool consistency, bloody stool, and weight loss assessments were also evaluated. DSS could significantly increase the DAI, whereas SASP and apigenin treatment could improve this phenomenon (Figure 1C) (P < 0.01). In addition, it was reported that DSS-induced colitis developed symptoms of spleen hypertrophy (17). The results showed that apigenin inhibited spleen hypertrophy in colitis mice compared to the mice in the DSS-induced colitis group (Figure 1D) (P < 0.01). Overall, these results indicated that apigenin administration treatment significantly ameliorated DSS-induced UC.
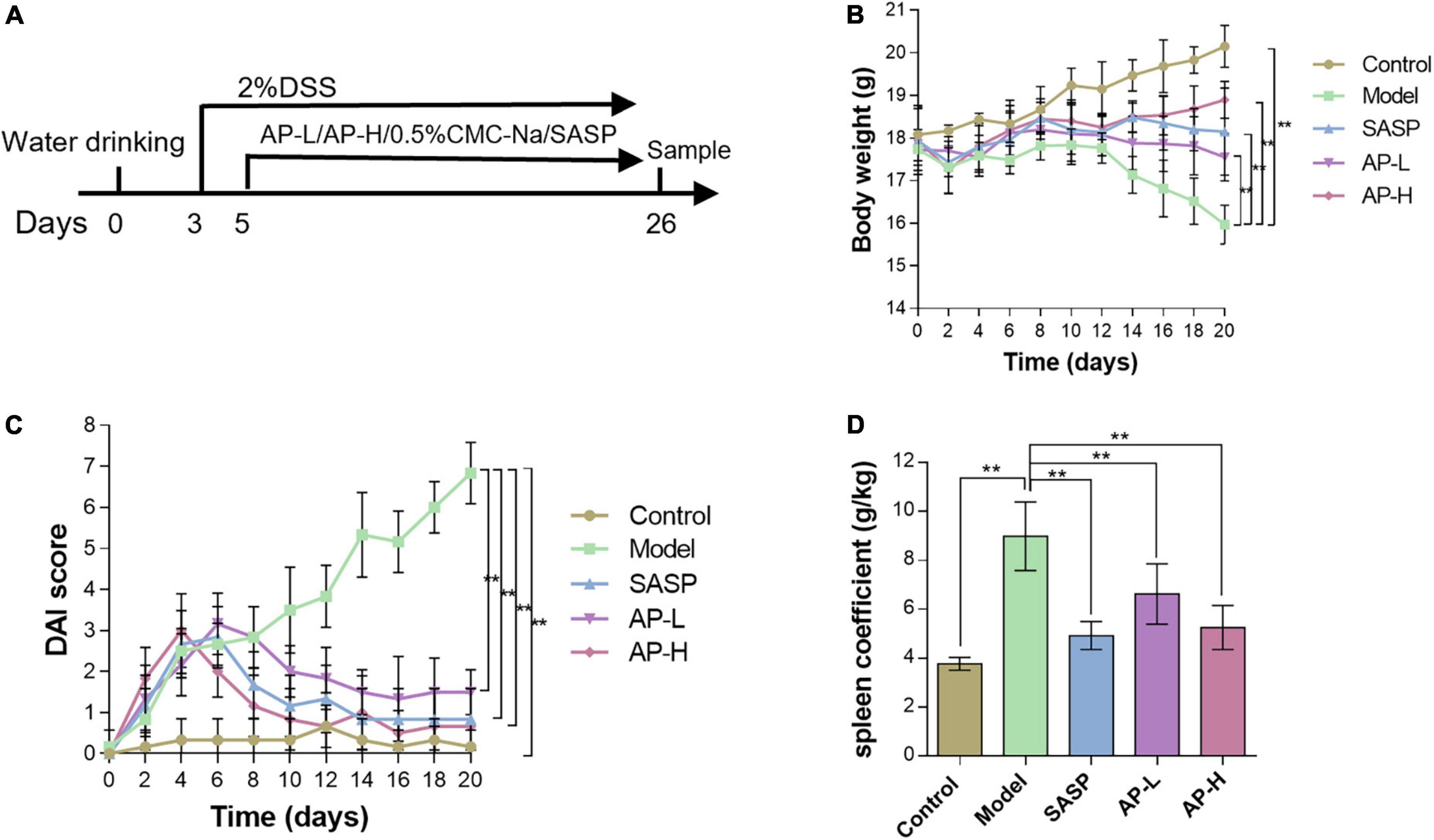
Figure 1. Apigenin protected experimental UC in mice. (A) A schematic of the UC and apigenin (AP) treatment design. (B) Changes in body weights (g) of mice. (C) Disease activity index (DAI) scores for weight loss and fecal status in each group. (D) Spleen coefficient of each treatment group. **P < 0.01.
3.2 Apigenin attenuates DSS-induced colon injury
To further investigate the therapeutic effect of apigenin on UC intuitively, we compared the length, gross morphology, and tissue damage of colorectal sites in each group of mice. DSS typically causes colonic shortening while such change was also improved by 150 and 250 mg/kg of apigenin. Moreover, there was no significant difference in colorectal length between high-dose apigenin-treated mice and SASP-treated mice (Figure 2A) (P < 0.05). The colorectal tissue displayed obvious fold, good elasticity, and reflectance in the wild mice. Colon tissues from UC mice displayed indistinguishable upper colorectal folds, granular mucous membranes, multiple hyperemia and swelling, and surface ulcers. Apigenin ameliorated these colonic damages and reduced colon macroscopic damage scores (Figure 2B) (P < 0.01). Histological examination of the hematoxylin and eosin-stained sections of the colon revealed severe inflammatory lesions, crypt erosion, and inflammatory cell infiltration from UC mice. Strikingly, SASP and apigenin-treated mice exhibited less inflammatory cell infiltration and intact colonic architecture without mucosal damage, reducing microscopic damage scores (Figure 2C) (P < 0.05). AB-PAS staining results demonstrated that compared with the control group, there was a lower goblet cell quantity of colon in model mice, while apigenin administration restored the damage induced by DSS (Figure 2D) (P < 0.01). Overall, apigenin supplementation could effectively alleviate the severity of colon injury in mice with UC.
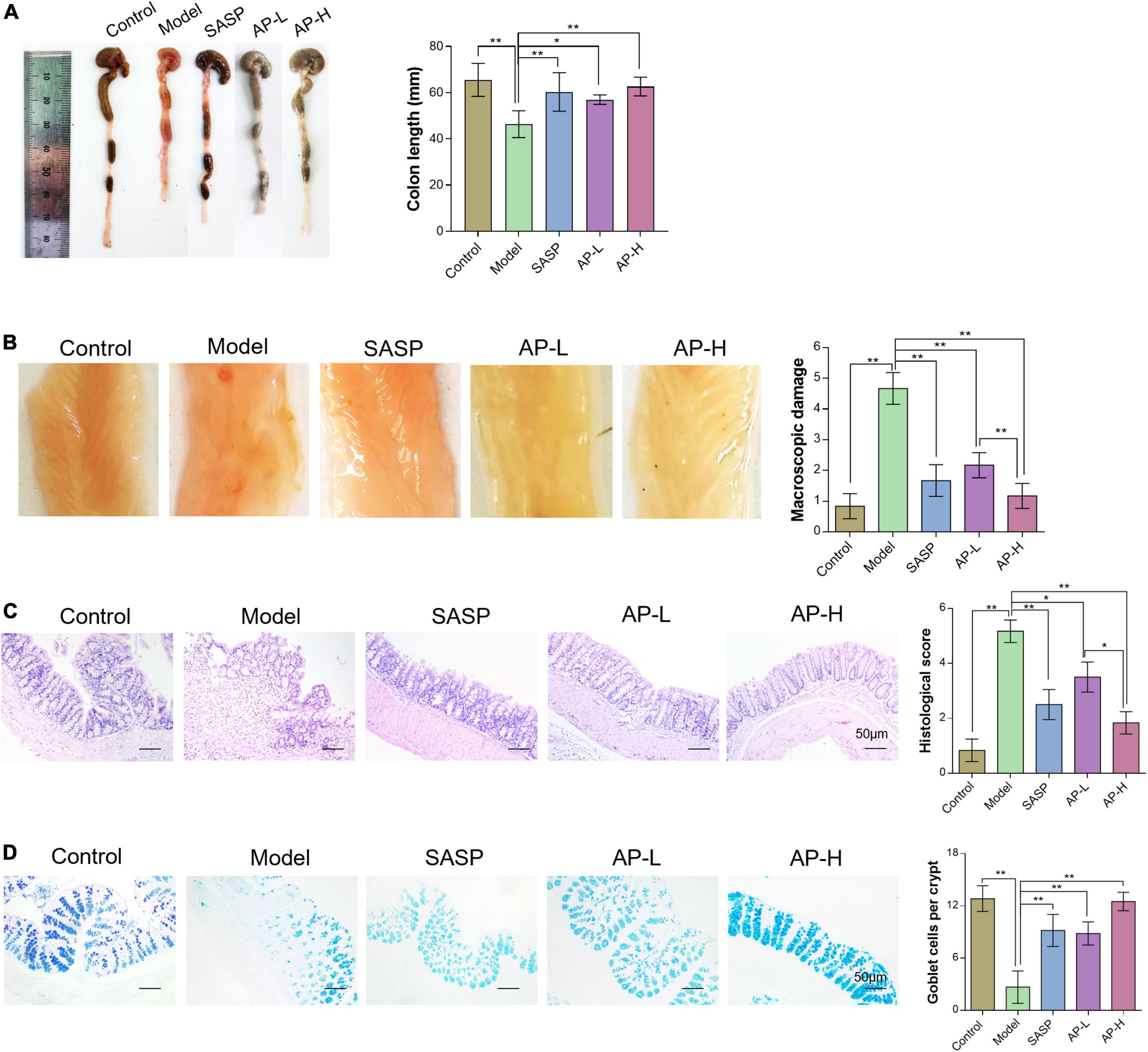
Figure 2. Apigenin attenuated DSS-induced colon injury. (A) Representative pictures of colon gross appearance and colon length. (B) Colonic morphology and associated macroscopic damage scores in mice. (C) H&E staining of the colon in mice and related microscopic lesion scores. (D) AB-PAS staining of colon tissues in mice among different groups. *P < 0.05, **P < 0.01.
3.3 Effect of apigenin on inflammatory cytokines in UC mice
To demonstrate the effect of apigenin on inflammation, inflammatory cytokines (TNF-α, IL-1β, IL-6, and IL-10) were determined. Increased proinflammatory cytokines TNF-α, IL-1β, and IL-6 expressions were observed in the model group. Compared with mice induced by DSS, SASP and apigenin treatment decreased TNF-α, IL-6, and IL-1β levels (Figure 3A) (P < 0.05). IL-10 produced by intestinal CD4+ T cells is responsible for maintaining immune homeostasis, thereby inhibiting colitis development (18). The results showed that apigenin increased the level of IL-10 in the colitis of DSS-induced colitis mice (Figure 3B) (P < 0.05). MPO, an enzyme present in neutrophils, is positively correlated with the concentration of neutrophils in the inflammatory region, and increased MPO activity is an indicator of neutrophil infiltration and inflammation (19). In the model group, MPO activity increased, and apigenin treatment inhibited neutrophil infiltration and inflammation in UC mice (Figure 3C) (P < 0.01). It is noteworthy that apigenin had an effect on TNF-α, IL-6, IL-1β, IL-10, and MPO in a dose-dependent manner. These results suggested that apigenin alleviated inflammation and kept intestinal homeostasis.
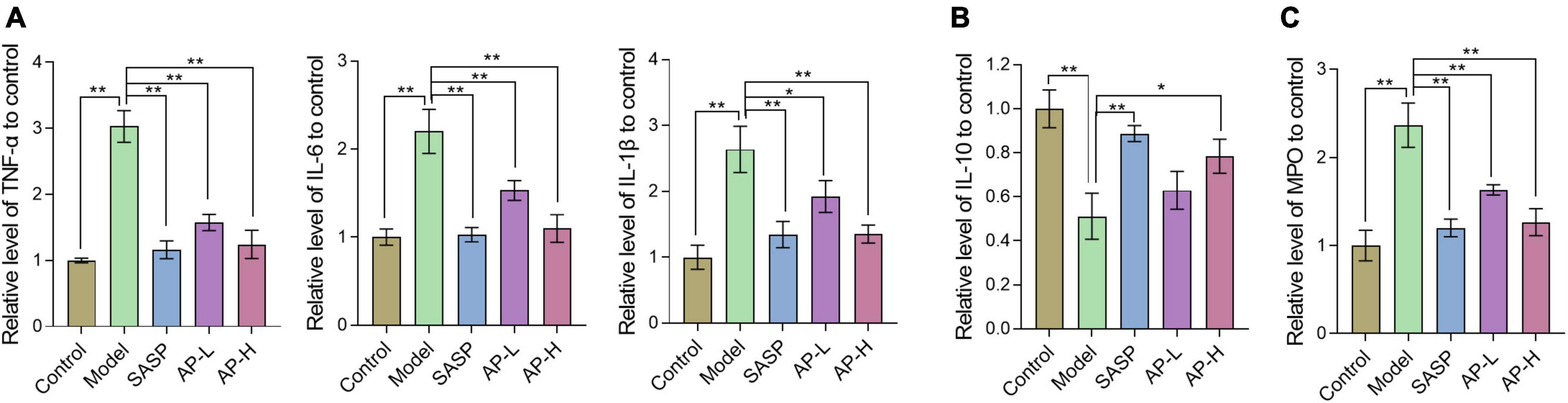
Figure 3. Apigenin treatment altered the cytokine expression in colitis mice. (A) Expression levels of inflammatory cytokines TNF-α, IL-6, and IL-1β in colon tissues. (B) Expression levels of inflammatory cytokines IL-10 in colon tissues. (C) Expression levels of MPO in colon tissues. *P < 0.05, **P < 0.01.
3.4 Apigenin increases gut barrier integrity of colitis mice
Gut barrier damage was the moment pathological characteristic of colitis (2), such as UC. The zonula occluden (ZO), occludin, and claudin family proteins are essential tight junction proteins of epithelial barrier (20). The levels of tight junction proteins were determined through Western blot analysis and IHC analysis. As shown in Figure 4A, the protein expressions of ZO-1, claudin-1, and occludin in the model group were reduced, while the supplement of SASP and apigenin could improve the expression of these proteins. Furthermore, the IHC images and staining scores indicated that substantial loss of staining intensity of ZO-1, claudin-1, and occludin in mice induced by DSS was observed compared with the control group (Figures 4B, C) (P < 0.01). However, SASP and apigenin might improve this change, and high-dose apigenin might have a better effect compared with SASP. The above results demonstrated that apigenin prevented gut barrier damage in DSS-induced colitis mice.
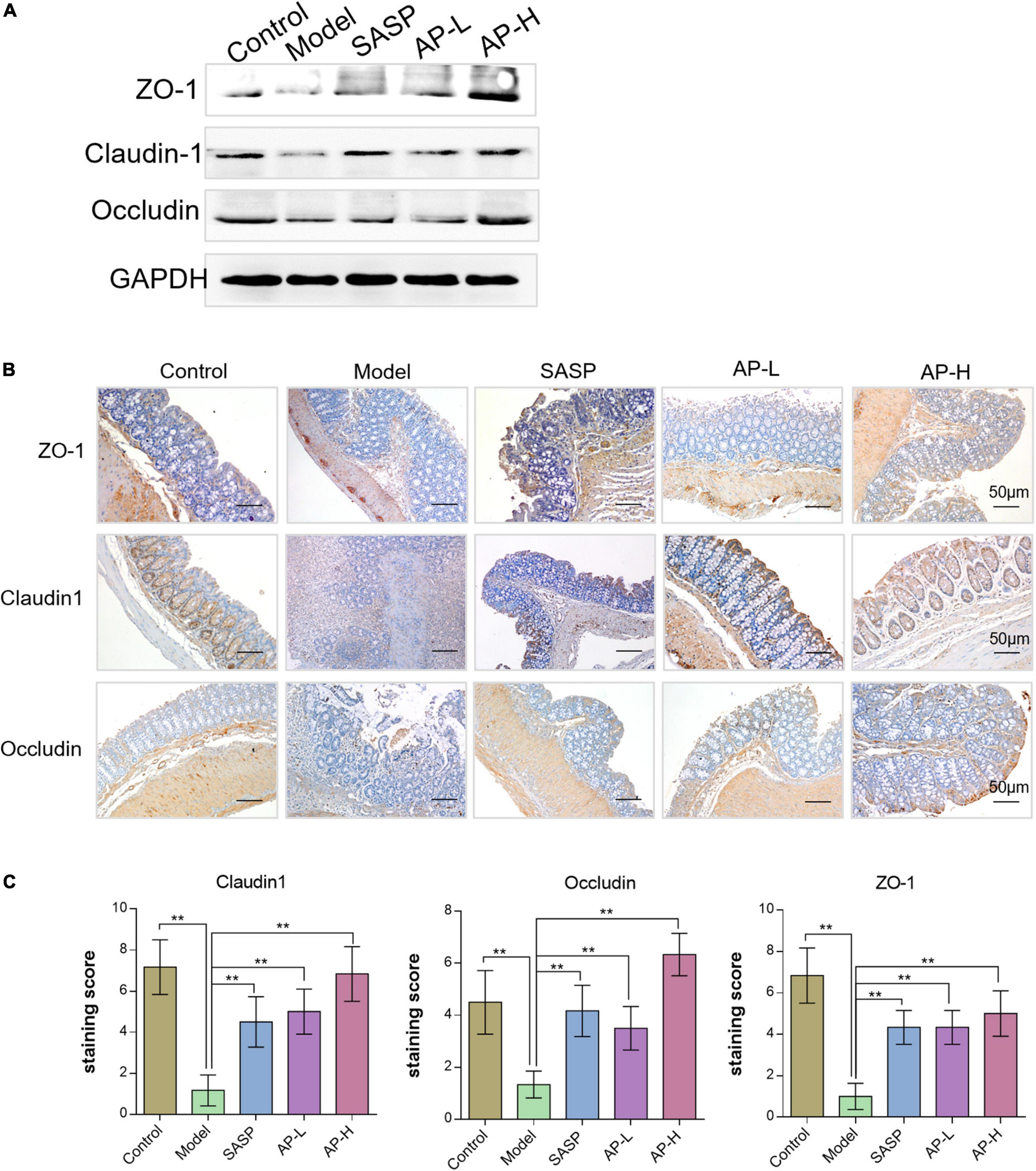
Figure 4. Effects of apigenin on gut barrier integrity in DSS-induced colitis mice. (A) Western blot analysis detecting ZO-1, claudin-1, and occludin expressions of colon tissues in each group. (B) ZO-1, claudin-1, and occludin expression levels in the colon tissues analyzed through IHC staining. (C) IHC staining index of ZO-1, claudin-1, and occludin in colon tissues. **P < 0.01.
3.5 Effect of apigenin on gut microbiota and its metabolites in UC mice
As metabolites of intestinal microflora, SCFAs maintain intestinal function by inhibiting inflammation and protecting the intestinal epithelial barrier (21). We detected the contents of SCFAs using GC-MS. Compared with the control group, the concentration of SCFAs in model groups was downregulated (P < 0.01). But apigenin and SASP administration significantly increased the level of SCFAs, and the SCFA content of AP groups was almost as effective as positive drugs (Figure 5) (P < 0.05).
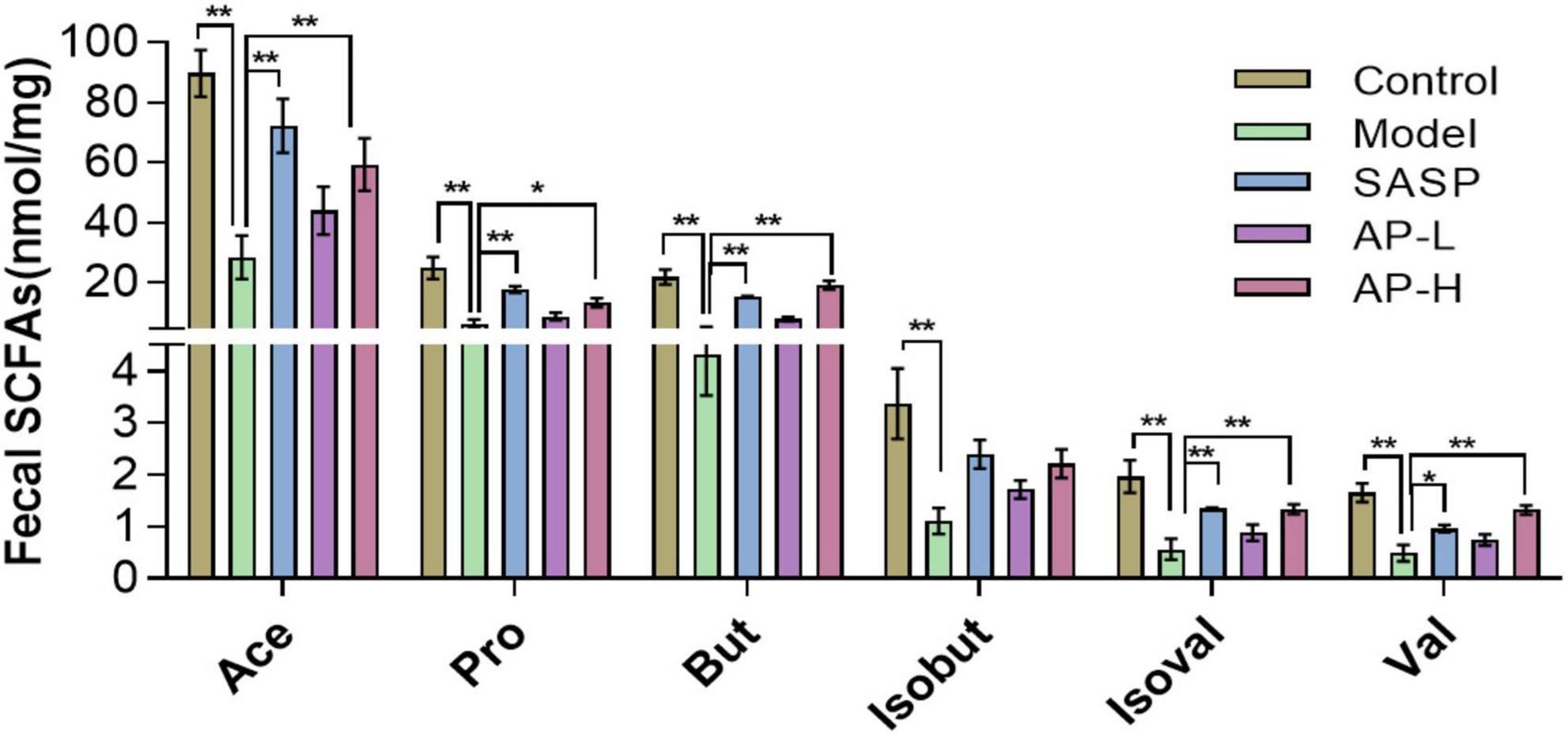
Figure 5. Apigenin treatment improved short-chain fatty acid (SCFA) contents. *P < 0.05, **P < 0.01.
To investigate whether apigenin has a moderating effect on the composition and diversity of gut microbiota, we performed 16S rRNA gene sequencing analysis of the colonic content of different groups of mice. Alpha diversity, such as Chao1 and ACE index, was recovered in apigenin and SASP groups (Figure 6A). To explore the specific variations in different groups, we evaluated the relative abundance of taxonomic groups. At the phylum level, we found that the dominant phylum was Firmicutes, Verrucomicrobia, and Proteobacteria. The results showed that DSS induction contributed to decreases in Firmicutes and Verrucomicrobia and increases in Proteobacteria. Apigenin and SASP intervention could significantly reverse the DSS-induced variation in the relative abundance of Verrucomicrobia and Proteobacteria (Figure 6B).
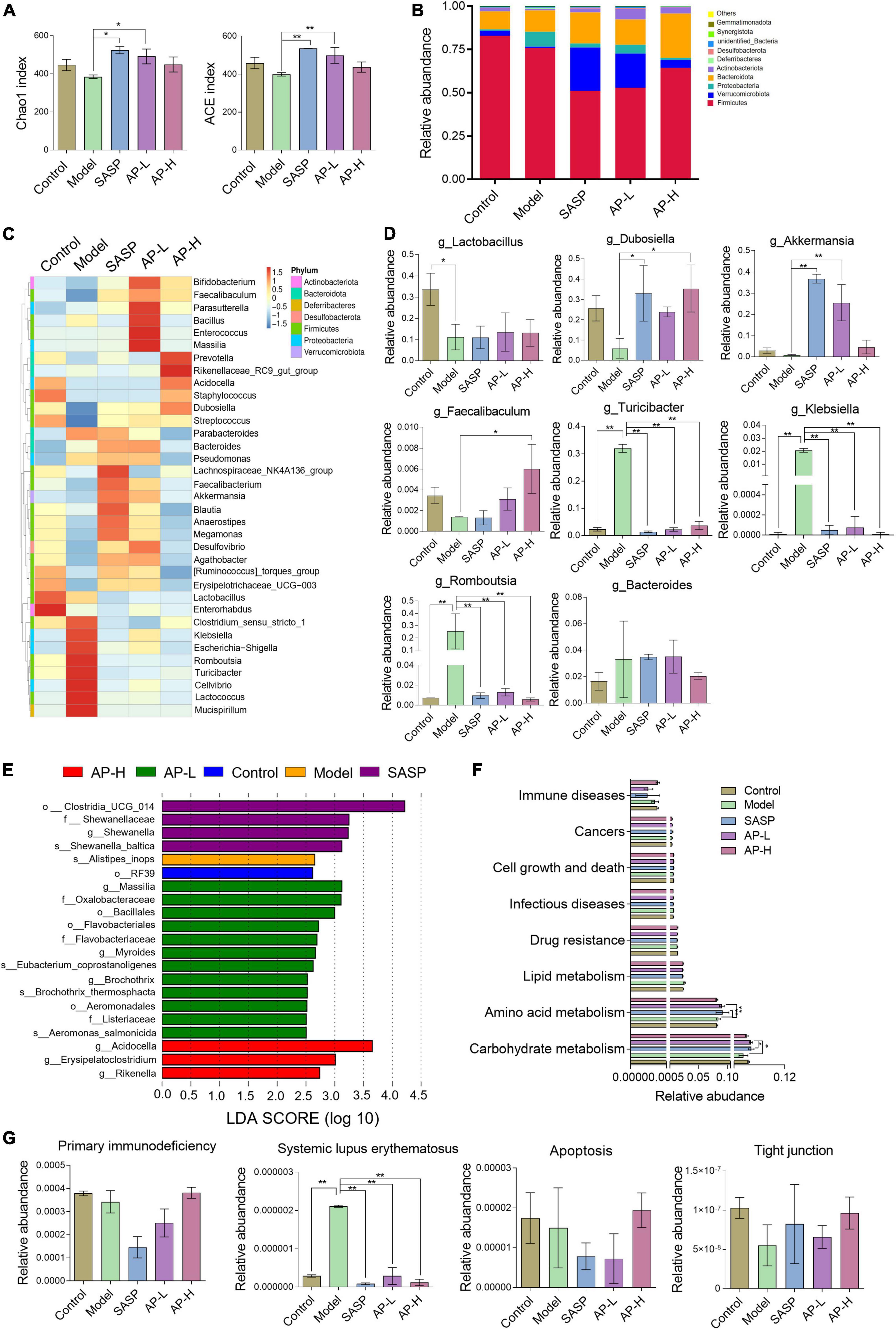
Figure 6. Effect of apigenin on gut microbiota in UC mice. (A) Chao1 and ACE index of samples. (B) Relative abundance of the top 10 microbial groups at the phylum level. (C) Community heatmap at the genus level. (D) Relative abundances of dominant bacteria at the genus level between different groups. (E) LDA discriminant histogram. (F) Microbial catabolism function of KEGG pathways among control, model, SASP, AP-L, and AP-H groups. (G) The effect of apigenin administration on primary immunodeficiency, systemic lupus erythematosus, apoptosis, and tight junction. *P < 0.05, **P < 0.01.
In the top 35 genus level, 16 genera were downregulated and 12 genera were upregulated in the DSS-induced mice, while SASP and apigenin supplementation selectivity reversed this change (Figure 6C). Among them, the relative abundances of Lactobacillus, Dubosiella, Akkermansia, and Faecalibaculum were decreased and Turicibacter, Klebsiella, Romboutsia, and Bacteroides were increased at genus level in DSS-induced mice. Apigenin and SASPs administration selectively reversed the relative abundance (Figure 6D). Previous studies proved that Lactobacillus (22, 23), Dubosiella (24), Akkermansia (25, 26), and Faecalibaculum (27) are probiotics protecting the intestinal immune barrier from damage. Turicibacter (28, 29), Klebsiella (30), Romboutsia (31), and Bacteroides, pernicious bacteria in IBD, might have a positive correlation with the malignant development of UC. In addition, linear discriminant analysis (LDA) showed the main feature of the dominant flora among five different groups (Figure 6E). Our results clarified that DSS treatment altered species diversity of intestinal microbial homeostasis, while apigenin restored that to the normal level.
To investigate the effect of apigenin supplementation on the potential metabolic pathways of gut microbiota in DSS-induced mice, Tax4Fun analysis was executed. As shown in Figure 6F, apigenin notably reversed the changes in many basic metabolic pathways such as carbohydrate metabolism and amino acid metabolism. Furthermore, we analyzed the metabolic changes of gut microbiota related to immune diseases, cell growth, and cell death. The results indicated that apigenin possibly had a close correlation in ameliorating the primary immunodeficiency, systemic lupus erythematosus, apoptosis, and tight junction motivated by DSS (Figure 6G). These findings demonstrated that treatment of apigenin may be effective in restoring dysregulated gut microbiota, which might play its role in the metabolite of bacteria. The protective mechanism of apigenin against UC is shown in Figure 7.
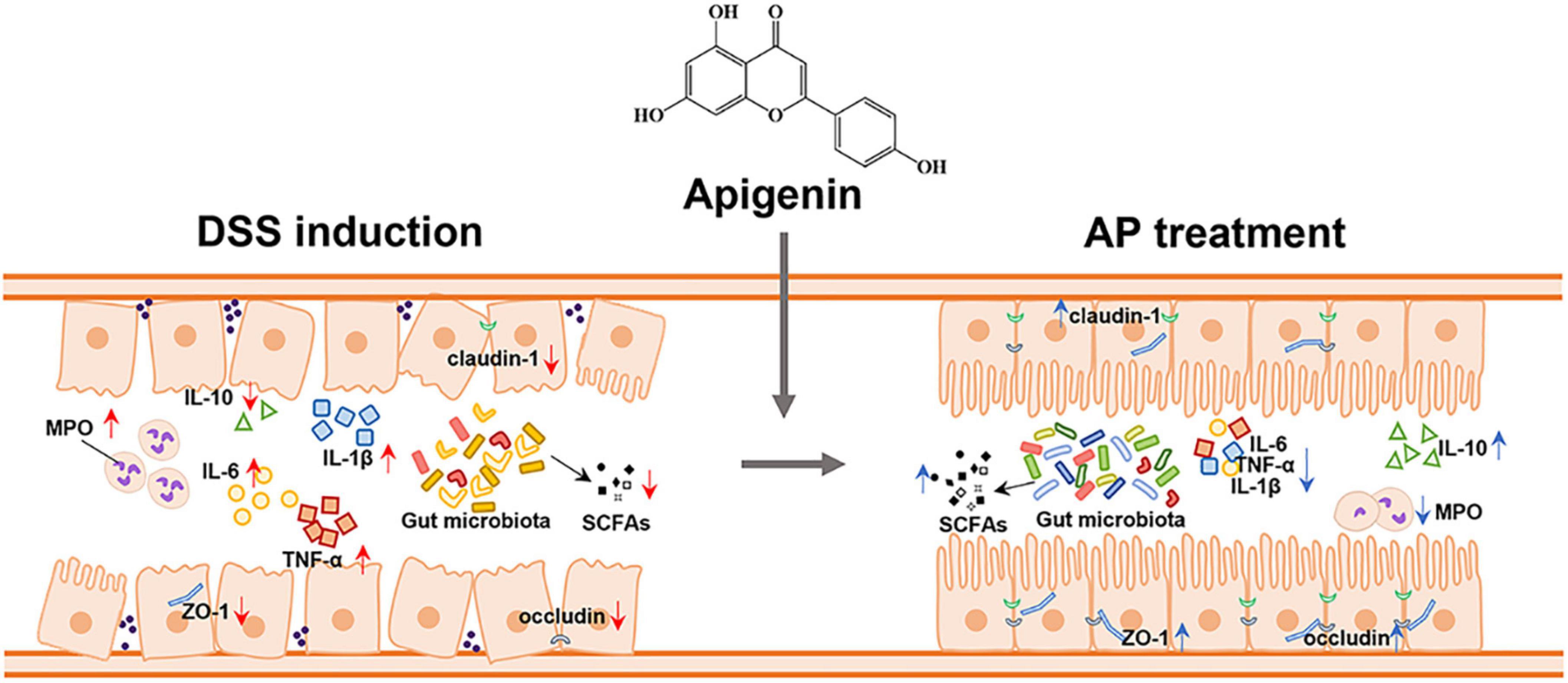
Figure 7. Apigenin effectively ameliorated DSS-induced ulcerative colitis by balancing the gut microbiome structure and its metabolites (SCFAs) to inhibit the inflammatory response and protect the gut barrier.
4 Discussion
Ulcerative colitis is an inflammatory condition that affects the alimentary canal, and the lesion location mainly includes the colon, rectum, or entire colorectal region (32). Owning to the high gradual incidence and therapeutic drugs with limited side effects on the treatment of patients with UC, the optimal treatment approaches for UC are needed to be explored. In this study, we constructed a UC model in mice treated with 2% DSS for 21 days. The apigenin treatment might suppress inflammatory responses and improve gut barrier damage by regulating the gut microbiome structure.
DSS-induced mice have poor mental states and suffer from weight loss, diarrhea, hematochezia, shorten colon, and splenomegaly (33). In this study, we found that apigenin significantly inhibited the decrease of body weight and colon shortening in mice. Apigenin significantly ameliorated hyperemia, swelling, surface ulcers, and splenomegaly. Moreover, goblet cells located in the intestinal epithelium generate mucin, protecting the intestinal barrier (34). Our results suggested that apigenin and SASP increased the goblet cell quantity and mucin content of colon tissues.
Overexpression of proinflammatory cytokines contributes to intestinal and mucosal inflammation, which can promote neutrophil migration and increase the phagocytic capacity of macrophages (35, 36). Our in vivo studies showed that apigenin inhibited TNF-α, IL-6, and IL-1β secretions in colitis of DSS-induced mice. IL-10 inhibits the secretion of proinflammatory cytokines and blocks the differentiation and proliferation of macrophages, thereby preventing excessive immune reaction and tissue damage (19). We found that apigenin increased the IL-10 level, improving the intestine’s immune barrier function. In addition, the results showed that apigenin decreased the MPO level, which inhibited neutrophil infiltration and inflammation in UC mice. Therefore, apigenin restored intestinal barrier function by regulating inflammatory factors and inhibiting neutrophil infiltration in UC.
In the development of colitis, the expression of tight junction proteins, such as ZO-1, occludin, and claudin-1, is negatively correlated with increased intestinal barrier permeability (37). The damage of this barrier is related to the pathogenesis of UC and its clinical symptoms, such as diarrhea and hematochezia. Our data showed that apigenin might have a better therapeutic effect on mechanical barrier integrity by maintaining the expression of ZO-1, claudin-1, and occludin to relieve the UC.
More evidence indicates that the gut microbiome plays a vital role in the development of UC (5). Recent studies showed that severe dysbacteriosis is observed in UC, which exhibits an abnormal abundance of Firmicutes, Bacteroidota, and Proteobacteria (29). Through 16S rRNA gene sequencing, we found that the reduction of Proteobacteria was observed by the apigenin and SASP intervention, which indicated that apigenin supplementation may regulate intestinal microbial composition and richness to rebalance gut microbiome. It has been proved that Lactobacillus could be profitable in UC treatment (38, 39). Our study showed that DSS induced Lactobacillus reduction in UC mice. However, apigenin treatment did not significantly affect the abundance of Lactobacillus. Therefore, the combination therapy of supplementing Lactobacillus with apigenin may be a potential treatment strategy for UC. Moreover, apigenin supplementation significantly enhanced the abundance of Akkermansia and Faecalibaculum. Intestinal flora produces kinds of metabolites that interact with intestinal epithelial cells, which are closely related to human disease (40). SCFAs, mainly including acetic acid, propionic acid, and butyric acid, were produced by some beneficial bacteria. Microbiota-derived SCFAs and SCFA supplementation upregulated IL-22 production, which protects intestines from inflammation to maintain intestinal homeostasis (41). Besides, amino acid metabolisms such as tryptophan deficiency and its decreased metabolic derivatives could contribute to the development of IBD (42). UC may be associated with the development of colorectal cancer (43). Function prediction data suggested that DSS-induced UC may result in disequilibrium of immune homeostasis and metabolic changes, such as primary immunodeficiency and apoptosis. Overall, apigenin administration remodeled the intestinal bacterial community to relieve UC. The precise mechanism of apigenin affecting the interaction between gut microbiota and the metabolic products in the UC should be further determined.
In conclusion, our studies indicated that apigenin, a natural functional food component, could effectively ameliorate DSS-induced UC by improving the intestine’s immune barrier function, mechanical barrier integrity, and biological barrier function in UC mice. With the advantages of low toxicity and side effects, apigenin is expected to be a potential therapeutic strategy for patients with UC.
Data availability statement
The original contributions presented in this study are included in the article/supplementary material, further inquiries can be directed to the corresponding authors.
Ethics statement
This animal study was reviewed and approved by Animal Experimentation Ethics Committee, Tianjin University of Science and Technology.
Author contributions
RF, LW, and YM contributed to experimental operation and data analysis. WX and JL contributed to data analysis and wrote sections of the manuscript. ZP conducted sections of experimental operation. JM and MZ arranged subject ideas and provided theoretical, technical guidance, and financial support. All authors contributed to the article and approved the submitted version.
Funding
This work was supported by grants from the National Natural Science Foundation of China (Grant Nos. 81902441 and 82072709), the China Postdoctoral Science Foundation (Grant No. 2022M712374), Tianjin “131” Innovative Talent Team Project (Grant No. 201926), and Open Project Program of State Key Laboratory of Food Nutrition and Safety (Grant No. SKLFNS-KF-202002).
Conflict of interest
The authors declare that the research was conducted in the absence of any commercial or financial relationships that could be construed as a potential conflict of interest.
Publisher’s note
All claims expressed in this article are solely those of the authors and do not necessarily represent those of their affiliated organizations, or those of the publisher, the editors and the reviewers. Any product that may be evaluated in this article, or claim that may be made by its manufacturer, is not guaranteed or endorsed by the publisher.
References
1. Ganjare A, Nirmal S, Patil A. Use of apigenin from Cordia dichotoma in the treatment of colitis. Fitoterapia. (2011) 82:1052–6. doi: 10.1016/j.fitote.2011.06.008
2. Martel J, Chang S, Ko Y, Hwang T, Young JD, Ojcius DM. Gut barrier disruption and chronic disease. Trends Endocrinol Metab. (2022) 33:247–65. doi: 10.1016/j.tem.2022.01.002
3. Cui H, Cai Y, Wang L, Jia B, Li J, Zhao S, et al. Berberine regulates Treg/Th17 balance to treat ulcerative colitis through modulating the gut microbiota in the colon. Front Pharmacol. (2018) 9:571. doi: 10.3389/fphar.2018.00571
4. Ma X, Hu Y, Li X, Zheng X, Wang Y, Zhang J, et al. Periplaneta americana ameliorates dextran sulfate sodium-induced ulcerative colitis in rats by Keap1/Nrf-2 activation, intestinal barrier function, and gut microbiota regulation. Front Pharmacol. (2018) 9:944. doi: 10.3389/fphar.2018.00944
5. Imhann F, Vila AV, Bonder MJ, Fu J, Gevers D, Visschedijk MC, et al. Interplay of host genetics and gut microbiota underlying the onset and clinical presentation of inflammatory bowel disease. Gut. (2018) 67:108–19. doi: 10.1136/gutjnl-2016-312135
6. Behary J, Amorim N, Jiang X, Raposo A, Gong L, McGovern E, et al. Gut microbiota impact on the peripheral immune response in non-alcoholic fatty liver disease related hepatocellular carcinoma. Nat Commun. (2021) 12:187. doi: 10.1038/s41467-020-20422-7
7. Johansson M, Hansson G. Immunological aspects of intestinal mucus and mucins. Nat Rev Immunol. (2016) 16:639–49. doi: 10.1038/nri.2016.88
8. Ouwerkerk J, de Vos W, Belzer C. Glycobiome: bacteria and mucus at the epithelial interface. Best Pract Res Clin Gastroenterol. (2013) 27:25–38. doi: 10.1016/j.bpg.2013.03.001
9. Duan H, Zhang Q, Liu J, Li R, Wang D, Peng W, et al. Suppression of apoptosis in vascular endothelial cell, the promising way for natural medicines to treat atherosclerosis. Pharmacol Res. (2021) 168:105599. doi: 10.1016/j.phrs.2021.105599
10. Jantan I, Haque MA, Arshad L, Harikrishnan H, Septama AW, Mohamed-Hussein Z. Dietary polyphenols suppress chronic inflammation by modulation of multiple inflammation-associated cell signaling pathways. J Nutr Biochem. (2021) 93:108634. doi: 10.1016/j.jnutbio.2021.108634
11. Mascaraque C, González R, Suárez MD, Zarzuelo A, Medina FS, Martínez-Augustin O. Intestinal anti-inflammatory activity of apigenin K in two rat colitis models induced by trinitrobenzenesulfonic acid and dextran sulphate sodium. Br J Nutr. (2015) 113:618–26. doi: 10.1017/S0007114514004292
12. Wang M, Firrman J, Zhang L, Arango-Argoty G, Tomasula P, Liu L, et al. Apigenin impacts the growth of the gut microbiota and alters the gene expression of Enterococcus. Molecules. (2017) 22:1292. doi: 10.3390/molecules22081292
13. Bian S, Wan H, Liao X, Wang W. Inhibitory effects of apigenin on tumor carcinogenesis by altering the gut microbiota. Mediators Inflamm. (2020) 2020:7141970. doi: 10.1155/2020/7141970
14. Qiao Y, Zhang Z, Zhai Y, Yan X, Zhou W, Liu H, et al. Apigenin alleviates obesity-associated metabolic syndrome by regulating the composition of the gut microbiome. Front Microbiol. (2021) 12:805827. doi: 10.3389/fmicb.2021.805827
15. Meng J, Chen S, Lei Y, Han J, Zhong W, Wang X, et al. Hsp90beta promotes aggressive vasculogenic mimicry via epithelial-mesenchymal transition in hepatocellular carcinoma. Oncogene. (2019) 38:228–43. doi: 10.1038/s41388-018-0428-4
16. Wang K, Liao M, Zhou N, Bao L, Ma K, Zheng Z, et al. Parabacteroides distasonis alleviates obesity and metabolic dysfunctions via production of succinate and secondary bile acids. Cell Rep. (2019) 26:222.e–35.e. doi: 10.1016/j.celrep.2018.12.028
17. Zhang M, Zhao Y, Wu N, Yao Y, Xu M, Du H, et al. The anti-inflammatory activity of peptides from simulated gastrointestinal digestion of preserved egg white in DSS-induced mouse colitis. Food Funct. (2018) 9:6444–54. doi: 10.1039/c8fo01939h
18. Yang W, Liu H, Xu L, Yu T, Zhao X, Yao S, et al. GPR120 Inhibits colitis through regulation of CD4(+) T cell interleukin 10 production. Gastroenterology. (2022) 162:150–65. doi: 10.1053/j.gastro.2021.09.018
19. Li Q, Cui Y, Xu B, Wang Y, Lv F, Li Z, et al. Main active components of Jiawei Gegen Qinlian decoction protects against ulcerative colitis under different dietary environments in a gut microbiota-dependent manner. Pharmacol Res. (2021) 170:105694. doi: 10.1016/j.phrs.2021.105694
20. Zhao B, Xia B, Li X, Zhang L, Liu X, Shi R, et al. Sesamol supplementation attenuates DSS-induced colitis via mediating gut barrier integrity, inflammatory responses, and reshaping gut microbiome. J Agric Food Chem. (2020) 68:10697–708. doi: 10.1021/acs.jafc.0c04370
21. Lavelle A, Sokol H. Gut microbiota-derived metabolites as key actors in inflammatory bowel disease. Nat Rev Gastroenterol Hepatol. (2020) 17:223–37. doi: 10.1038/s41575-019-0258-z
22. Liu Z, Liu F, Wang W, Sun C, Gao D, Ma J, et al. Study of the alleviation effects of a combination of Lactobacillus rhamnosus and inulin on mice with colitis. Food Funct. (2020) 11:3823–37. doi: 10.1039/c9fo02992c
23. Cervantes-Barragan L, Chai JN, Tianero MD, Luccia BD, Ahern PP, Merriman J, et al. Lactobacillus reuteri induces gut intraepithelial CD4(+)CD8alphaalpha(+) T cells. Science. (2017) 357:806–10. doi: 10.1126/science.aah5825
24. Du C, Wang K, Zhao Y, Nan X, Chen R, Quan S, et al. Supplementation with milk-derived extracellular vesicles shapes the gut microbiota and regulates the transcriptomic landscape in experimental colitis. Nutrients. (2022) 14:1808. doi: 10.3390/nu14091808
25. Wang L, Tang L, Feng Y, Zhao S, Han M, Zhang C, et al. A purified membrane protein from Akkermansia muciniphila or the pasteurised bacterium blunts colitis associated tumourigenesis by modulation of CD8(+) T cells in mice. Gut. (2020) 69:1988–97. doi: 10.1136/gutjnl-2019-320105
26. Cani P, Depommier C, Derrien M, Everard A, de Vos W. Akkermansia muciniphila: paradigm for next-generation beneficial microorganisms. Nat Rev Gastroenterol Hepatol. (2022) 19:625–37. doi: 10.1038/s41575-022-00631-9
27. Zagato E, Pozzi C, Bertocchi A, Schioppa T, Saccheri F, Guglietta S, et al. Endogenous murine microbiota member Faecalibaculum rodentium and its human homologue protect from intestinal tumour growth. Nat Microbiol. (2020) 5:511–24. doi: 10.1038/s41564-019-0649-5
28. Li H, Cao W, Xie J, Che H, Liu L, Dong X, et al. alpha-D-1,6-glucan from Castanea mollissima Blume alleviates dextran sulfate sodium-induced colitis in vivo. Carbohydr Polym. (2022) 289:119410. doi: 10.1016/j.carbpol.2022.119410
29. Tong L, Hao H, Zhang Z, Lv Y, Liang X, Liu Q, et al. Milk-derived extracellular vesicles alleviate ulcerative colitis by regulating the gut immunity and reshaping the gut microbiota. Theranostics. (2021) 11:8570–86. doi: 10.7150/thno.62046
30. Chiang M, Hsiao P, Liu Y, Tang H, Chiou C, Lu M, et al. Two ST11 Klebsiella pneumoniae strains exacerbate colorectal tumorigenesis in a colitis-associated mouse model. Gut Microbes. (2021) 13:1980348. doi: 10.1080/19490976.2021.1980348
31. Wu Z, Huang S, Li T, Li N, Han D, Zhang B, et al. Gut microbiota from green tea polyphenol-dosed mice improves intestinal epithelial homeostasis and ameliorates experimental colitis. Microbiome. (2021) 9:184. doi: 10.1186/s40168-021-01115-9
32. Luo X, Yue B, Yu Z, Ren Y, Zhang J, Ren J, et al. Obacunone protects against ulcerative colitis in mice by modulating gut microbiota, attenuating TLR4/NF-kappaB signaling cascades, and improving disrupted epithelial barriers. Front Microbiol. (2020) 11:497. doi: 10.3389/fmicb.2020.00497
33. Ge H, Cai Z, Chai J, Liu J, Liu B, Yu Y, et al. Egg white peptides ameliorate dextran sulfate sodium-induced acute colitis symptoms by inhibiting the production of pro-inflammatory cytokines and modulation of gut microbiota composition. Food Chem. (2021) 360:129981. doi: 10.1016/j.foodchem.2021.129981
34. Gonzalez-Perez V, Martinez-Espinosa P, Sala-Rabanal M, Bharadwaj N, Xia X, Chen A, et al. Goblet cell LRRC26 regulates BK channel activation and protects against colitis in mice. Proc Natl Acad Sci USA. (2021) 118:e2019149118. doi: 10.1073/pnas.2019149118
35. Bian X, Wu W, Yang L, Lv L, Wang Q, Li Y, et al. Administration of Akkermansia muciniphila ameliorates dextran sulfate sodium-induced ulcerative colitis in mice. Front Microbiol. (2019) 10:2259. doi: 10.3389/fmicb.2019.02259
36. Zhang Z, Li S, Cao H, Shen P, Liu J, Fu Y, et al. The protective role of phloretin against dextran sulfate sodium-induced ulcerative colitis in mice. Food Funct. (2019) 10:422–31. doi: 10.1039/c8fo01699b
37. Martens E, Neumann M, Desai M. Interactions of commensal and pathogenic microorganisms with the intestinal mucosal barrier. Nat Rev Microbiol. (2018) 16:457–70. doi: 10.1038/s41579-018-0036-x
38. Wu J, Wei Z, Cheng P, Qian C, Xu F, Yang Y, et al. Rhein modulates host purine metabolism in intestine through gut microbiota and ameliorates experimental colitis. Theranostics. (2020) 10:10665–79. doi: 10.7150/thno.43528
39. Yu P, Ke C, Guo J, Zhang X, Li B. Lactobacillus plantarum L15 alleviates colitis by inhibiting LPS-mediated NF-kappaB activation and ameliorates DSS-induced gut microbiota dysbiosis. Front Immunol. (2020) 11:575173. doi: 10.3389/fimmu.2020.575173
40. Sidebottom A, Chang E. IBD microbial metabolome: the good, bad, and unknown. Trends Endocrinol Metab. (2020) 31:807–9. doi: 10.1016/j.tem.2020.05.001
41. Yang W, Yu T, Huang X, Bilotta AJ, Xu L, Lu Y, et al. Intestinal microbiota-derived short-chain fatty acids regulation of immune cell IL-22 production and gut immunity. Nat Commun. (2020) 11:4457. doi: 10.1038/s41467-020-18262-6
42. Nikolaus S, Schulte B, Al-Massad N, Thieme F, Schulte D, Bethge J, et al. Increased tryptophan metabolism is associated with activity of inflammatory bowel diseases. Gastroenterology. (2017) 153:1504.e–16.e. doi: 10.1053/j.gastro.2017.08.028
Keywords: apigenin, ulcerative colitis, anti-inflammatory, gut microbiota, gut barrier integrity
Citation: Fu R, Wang L, Meng Y, Xue W, Liang J, Peng Z, Meng J and Zhang M (2022) Apigenin remodels the gut microbiota to ameliorate ulcerative colitis. Front. Nutr. 9:1062961. doi: 10.3389/fnut.2022.1062961
Received: 06 October 2022; Accepted: 28 November 2022;
Published: 16 December 2022.
Edited by:
Yang Xu, Zhejiang University, ChinaReviewed by:
Jin-Long Tian, Shenyang Agricultural University, ChinaJiaojiao Zhang, Zhejiang Agriculture and Forestry University, China
Copyright © 2022 Fu, Wang, Meng, Xue, Liang, Peng, Meng and Zhang. This is an open-access article distributed under the terms of the Creative Commons Attribution License (CC BY). The use, distribution or reproduction in other forums is permitted, provided the original author(s) and the copyright owner(s) are credited and that the original publication in this journal is cited, in accordance with accepted academic practice. No use, distribution or reproduction is permitted which does not comply with these terms.
*Correspondence: Jing Meng, ✉ amluZy5tZW5nQHR1c3QuZWR1LmNu; Min Zhang, ✉ em0wMTAyQHR1c3QuZWR1LmNu
†These authors have contributed equally to this work