- 1School of Marine and Bioengineering, Yancheng Institute of Technology, Yancheng, China
- 2Jiangsu Key Laboratory of Marine Bioresources and Environment, Jiangsu Ocean University, Lianyungang, China
Herein, we present a method for producing water-soluble polysaccharides (WSPs) by co-culture fermentation of straw and shrimp shells. The chitin-degrading strain was isolated and genotypically identified as the non-pathogen Photobacterium sp. LYM-1 in this study. Photobacterium sp. LYM-1 and Aureobasidium pullulans 2012 could coexist without antagonism. WSPs concentrations were higher in co-culture fermentations of Photobacterium sp. LYM-1 and A. pullulans 2012 (PsL/AP-WSPs) compared to monocultures (PsL-WSPs and AP-WSPs). FTIR was used to examine the polysaccharide properties of three WSP fractions. The monosaccharide compositions of three WSPs fractions were primarily composed of mannose, ribose, glucosamine, glucose, galactose, and arabinose with varying molecular weights and molar ratios according to HPLC analysis. PsL/AP-WSPs showed better scavenging effects on DPPH, ABTS, and OH free radicals, demonstrating the application potential of PsL/AP-WSPs from straw and shrimp shells. The maximum yield obtained under optimum conditions (fermentation time of 6 days, temperature of 31°C, inoculum concentration of 10% (w/v), and inoculum composition of 2:1) was 5.88 ± 0.40 mg/mL, based on the PsL/AP-WSPs production optimization by orthogonal design. The results suggest that an environmentally friendly approach for WSPs production from agro-food wastes straw and shrimp shells was developed.
Introduction
Polysaccharides play multiple roles in the life process with extensive biological activities and a huge potential in the fields of pharmaceutical, food, and cosmetics industries due to their efficacy and relatively low toxicity. Natural polysaccharides exhibited diverse bioactivities, such as antioxidant (1), anti-inflammatory (2, 3), antitumor (4), antiobesity (5), immunomodulatory (6), hypoglycemic (7), and prebiotics activities (8). Natural polysaccharides are typically extracted using various techniques or fermented by microbes. Hot-water extraction (HW), acid/alkali extraction (9), ultrasonic-assisted extraction (UA), microwave-assisted extraction (ME) (10), enzyme-assisted extraction (EA), and enzyme-ultrasonic assistance extraction (EUA) (11) are the most commonly used extraction techniques today. While each technique has advantages and disadvantages, the efficiency of polysaccharides extraction, time consumption, cost, and environmental impact must all be considered. During the preparation, the physiochemical and biological function of polysaccharides changed (12). Microbial polysaccharides produced by a wide range of microorganisms are generally water-soluble polysaccharides (WSPs) and the final products are more quality controlled (13). Microbial fermentation is a relatively green and environmentally friendly method of polysaccharide preparation when compared to other methods. They are gaining more recognition in the fields of food and Pharmaceuticals. Pullulan is a microbial polysaccharide produced by the yeast-like polymorphic fungus Aureobasidium pullulans (14).
Microbial exopolysaccharides (EPSs) include both homopolysaccharides and heteropolysaccharides. Pullulan is a type of homopolysaccharide, whereas xanthan and gellan are typical heteropolysaccharides. Microbial EPS are widely used in the development of functional foods (15). Submerged fermentation (SmF) and solid-state fermentation (SSF) are widely used two methods for the production of EPS. Many strategies have been implemented to improve the yield and titer of microbial EPS production during batch fermentation (16–18). However, the key factor restricting the wider application of EPS is the high cost of mass production. Therefore, cheap substrates for reducing the cost to facilitate applications of EPS are a practical approach (19). Many agro-food wastes, such as potato waste, de-oiled rice bran, jackfruit seeds, the hull hydrolysate, and bran of rice and wheat, have been used as materials for the production of EPSs by A. pullulans (20, 21). Straw is one of the richest agro-food wastes in the world, which is a potential candidate for the production of EPS by A. pullulans (22).
The disposal of a vast quantity of shrimp shell waste has become a major ecological and environmental issue. Even efforts to extract chitin and chitosan from shrimp shell waste via chemical processes or fermentation can result in environmental pollution (23). Acid and alkali treatments have to be used during the fermentation process for water-insoluble chitin or chitosan preparation from shrimp shells (24). In addition to chitin and chitosan, studies on the production of various bioactive metabolites by the fermentation of shrimp shells with different strains have been reported (24, 25). To the best of our knowledge, the exopolysaccharides preparation by the fermentation of chitin-degradation strain Photobacterium sp. has not been reported.
This work aims to obtain WSPs by co-fermenting straw and shrimp shell wastes. In this study, shrimp shell degradation strain was isolated and identified first using chitin as the index. To economically utilize these agro-food wastes, the compatibility between the straw-degradation strain and the shrimp shell degradation strain was further investigated. The molecular weight, monosaccharide compositions, chemical properties, and antioxidant activity of WSPs were analyzed. Finally, the fermentation conditions to produce WSPs were optimized. Our findings could be useful in the development of novel exopolysaccharides from low-cost substrates.
Materials and methods
Bacterial strains, chemicals, and media
Rice straw and shrimp shells were purchased from Yancheng Farmers Market, Jiangsu Province, China. Photobacterium sp. LYM-1 was isolated from mudflat collected in Yancheng coastal mudflat areas, Jiangsu, China. Aureobasidium pullulans 2012 was obtained from the College of Food Science and Technology, Nanjing Agricultural University, China, originally isolated from the food environment. All reagents and chemicals used in this study were of the highest grade available from the suppliers, except for the HPLC solvents (LCMS grade).
Liquid enrichment medium (g/L): chitin powder 8.0, (NH4)2SO4 2.0, natural pH, sterilized at 121°C for 20 min.
Colloidal chitin agar (g/L): colloidal chitin 4.0, (NH4)2SO4 2.0, agar 15.0, sterilized at 121°C for 20 min.
Seed medium: Luria–Bertani (LB) medium (g/L): yeast extract 5, tryptone 10, sodium chloride 10, natural pH, sterilized at 121°C for 20 min.
Yeast extract peptone dextrose (YPD) culture medium (g/L): tryptone 20, yeast extract 10, glucose 20, natural pH, sterilized at 121°C for 20 min.
Fermentation medium (g/L): straw 15, shrimp shell 30, natural pH, sterilized at 121°C for 20 min.
Screening, identification, and compatibility experiment of strains
Isolation of chitin-degrading bacteria
The samples were collected from Yancheng coastal mudflat areas of Jiangsu province, China. Nine soil samples (5 g) were diluted with 25 mL of sterile water in 50 mL centrifuge tubes and vortexed. Five milliliters of diluents were inoculated into an enrichment medium and incubated at 30°C for 48 h. Then, 500 μL of enriched culture was smeared onto the colloidal chitin agar petri dish with 10–4, 10–5, and 10–6 times dilution, respectively, and incubated at 28°C for 72 h. The target isolate was identified by the chitin hydrolysis zone.
Identification of chitin-degrading bacteria by 16S rDNA amplification
The genomic DNA of the best isolate was prepared using the Vazyme bacteria DNA isolation mini kit (DC112-01). Polymerase chain reaction (PCR) amplification of the 16S rRNA gene sequence was performed for species-level detection. The oligonucleotide primers for amplifying 16S rRNA were as follows: forward 27F: (5′ AGAGTTTGATCCTGGCTCAG 3′) and reverse primers 1492R: (5′ GGTTACCTTGTTACGACTT 3′). Purified PCR fragment was sequenced by DNA sequencing (Sangon Biotech, China) and compared with available 16S rRNA in the National Center for Biotechnology Information (NCBI) GenBank database.1 The information for the similarity rate and GenBank accession number was obtained. The MEGA7.0 software was used to construct the phylogenetic tree by the neighbor-joining technique.
Compatibility analysis of Photobacterium sp. LYM-1 and Aureobasidium pullulans 2012
The compatibility of the yeast strain and bacteria strain was tested using the previous method (26) with some modifications. In one group, yeast (A. pullulans 2012) was smeared on the surface of the YPD petri dish and bacteria (Photobacterium sp. LYM-1) were inoculated in the center. For another group, Photobacterium sp. LYM-1 was smeared on the surface of the LB petri dish and A. pullulans 2012 was inoculated in the center. The plates were incubated at 28°C for 48 h and the zone of inhibition was observed and recorded.
Preparation, separation, and purification of water-soluble polysaccharides
Production of water-soluble polysaccharides by co-fermentation of straw and shrimp shell
Separate seed cultures of two strains were prepared by inoculating 10 μL of frozen glycerol stock into 20 mL of seed medium and incubated at 30°C, 200 rpm for 24 h. Batch fermentation was carried out by inoculating 5 mL of seed culture into 100 mL of fermentation medium and incubating at 28°C, 200 rpm for 72 h. Monocultures and co-culture fermentation groups of Photobacterium sp. LYM-1 and A. pullulans 2012 were set up (named PsL-WSPs, AP-WSPs, and PsL/AP-WSPs, respectively). The supernatant containing WSPs was collected by centrifugation at 10,000 g for 20 min.
Preliminary purification of water-soluble polysaccharides
After collection, the one-quarter volume of the primary crude WSPs solution was obtained by vacuum evaporation at 50°C. To precipitate WSPs, three times the volume of ethanol was added and kept at 4°C for 12 h. Subsequently, the precipitates were obtained by centrifugation at 10,000 g for 20 min and washing with absolute ethanol once more. The crude WSPs were obtained. The protein was then removed using the Sevage reagent (chloroform: normal butanol, 4:1, v/v) method (27). The resulting aqueous phase was dialyzed for 3 days using a dialysis tube (Solarbio, MWCO 8,000–12,000 Da), concentrated, and lyophilized, yielding the three WSPs fractions (PsL-WSPs, AP-WSPs, and PsL/AP-WSPs).
Determination of chemical composition of water-soluble polysaccharides
The polysaccharides content was determined by the phenol–sulfuric acid method with glucose (Glc) as the standard (28). The content of ash was determined as described in Shi et al. (29). The content of protein was determined with bovine serum albumin as the standard using the Bradford method (30). The content of total polyphenol was conducted by the previously reported method of Banik et al. with some modifications (31). The content of total flavonoids was determined following the method of Maina et al. with rutin as the standard (32), and the content of uronic acid was determined with galacturonic acid (GalA) as the standard as described by Blumenkrantz and Asboe-Hansen (33).
Molecular weight distribution determination of water-soluble polysaccharides
The molecular weight of WSPs was determined using an Agilent 1260 high-performance liquid chromatographic (HPLC) instrument (Agilent Technologies, Santa Clara, CA, USA) equipped with a column (7.8 mm × 300 mm, Tosoh Crop., Tokyo, Japan), at a constant flow rate of 0.7 mL/min and a refractive index detector (RID). The mobile phase was K2HPO4 (0.03 mM) and the sample injection volume was 18 μL. The temperature of the column and detector was 37°C. The molecular weights of WSPs were estimated by reference to the calibration curve made from Dextran standards of series known molecular weight (5, 12, 25, 50, 80, and 150 kDa).
Monosaccharide composition determination of water-soluble polysaccharides
The monosaccharide composition of WSPs was determined using HPLC following a previously reported method with minor modifications (34). A 20 mM mother monosaccharide standard was prepared which consists of galactose (Gal), mannose (Man), fucose (Fuc), glucosamine (GlcN), Glc, GalA, rhamnose (Rha), and arabinose (Ara). WSPs samples (3 mg) were added to 1 mL of 4 M trifluoroacetic acid (TFA) solution in a sealed flask filled with N2 at 100°C and hydrolyzed for 4 h. Excess TFA in the samples was removed by repeated evaporation with absolute ethanol at 50°C. Subsequently, 50 μL of prepared hydrolysate samples or monosaccharide standard were added to 50 μL of 1-phenyl-3-methyl-5-pyrazolone (PMP, 0.5 M) solution and 50 μL of NaOH solution (0.3 M) at 70°C for 50 min. The supernatant from centrifugation was mixed with 50 μL of HCl (0.3 M). Finally, 1 mL of chloroform was used to remove the excess PMP, and this step was repeated three times. The aqueous layer was filtered by a 0.22 μm membrane before HPLC analysis. The analytes were separated using the Agilent 1260 HPLC instrument (Agilent Technologies, Santa Clara, CA, USA) equipped with Eclipse Plus reverse C18 column (4.6 × 250 mm, 5 μm, Agilent), at a constant flow rate of 0.4 mL/min and monitored by UV-detection (245 nm). The column oven temperature was set to 35°C. A linear gradient of ammonium acetate in water (50 mM, pH 4.5, solvent A) and acetonitrile (solvent B) was applied, and the elution procedure is shown in Supplementary Table 1.
Fourier transform infrared spectrometer analysis
The chemical structure of three WSPs fractions from agro-food wastes was characterized by Fourier transform infrared (FTIR). FTIR was performed using IR Affinity-1S, SHIMADZU equipment (Kyoto, Japan) over a wave-number range of 400–4,000 cm–1. The target products were examined using the KBr pellet technique.
Determination of in vitro antioxidant activity of water-soluble polysaccharides
Determination of 1,1-diphenyl-2-picrylhydrazyl radical scavenging activity
The scavenging ability of three WSPs fractions on DPPH radical (1,1-diphenyl-2-picrylhydrazyl free radical) was measured in accordance with the previously described method (35). In brief, 1 mL of DPPH (0.1 mM) and 1 mL of WSPs (1–5 mg/mL) were mixed at 25°C for 0.5 h in dark. Ascorbic acid (Vc) was used as a positive control. The solutions lacking WSPs or DPPH were designated as the blank group and control group, respectively. The absorbance of the solution was then measured at 517 nm. DPPH scavenging activity was calculated using Eq. (1).
where A0 is the absorbance of the blank group, and A1 and A2 are the absorbance of the control and specimen groups, respectively.
Determination of 2,2-azinobis-(3-ethylbenzthiazoline-6-sulphonate) radical scavenging activity
The scavenging ability of three WSPs fractions on ABTS radical (2,2-azinobis-(3-ethylbenzthiazoline-6-sulphonate) was measured as described by Meng et al. (36) with minor modifications. Briefly, the ABTS radical solution was prepared by adding 2.6 mM of K2S2O8 to 7 mM ABTS solution and incubated in dark for 16 h. 0.1 mL of WSPs (1–5 mg/mL) were mixed with 3.9 mL of the diluted ABTS radical solution and incubated at room temperature in dark for 10 min. The absorbance at 734 nm was measured. The ABTS scavenging activity was calculated using Eq. (1) above.
Determination of OH radical scavenging activity
The scavenging ability of three WSPs fractions on OH radical was measured by the Fenton method (37). Hydroxyl radical solutions were prepared by mixing 1 mL of H2O2 (9 mM), 1 mL of FeSO4 (9 mM), and 0.5 mL of salicylic acid–ethanol solution (9 mM). One milliliter of WSPs (1–5 mg/mL) was mixed with prepared hydroxyl radical solutions and measured at 510 nm absorbance. The scavenging ability of WSPs was calculated using Eq. (1) above.
Determination of ferric-reducing power
Ferric-reducing power (FRAP) of three WSPs fractions was determined according to the previous report (38) with minor modifications. Various concentrations (1–5 mg/mL) of WSPs were prepared with 0.2 mM PBS (pH 6.6). The reaction solution containing 1 mL of WSPs solution, 1 mL of 1% K3Fe(CN)6, and 1 mL of 0.2 mM PBS (pH 6.6) buffer was incubated at 50°C for 20 min. One milliliter of 10% trichloroacetic acid was added to quench the reaction. One milliliter of the supernatant was added to 1 mL of 0.1% FeCl3 solution and incubated at room temperature for 30 min in dark. The absorbance at 700 nm was measured. The ferric-reducing power of WSPs was calculated using Eq. (2).
where A1 and A2 are the absorbance of the specimen and blank group, respectively.
Determination of superoxide anion scavenging activity
The scavenging ability of three WSPs fractions on superoxide anion radical (O2) was investigated as described by Cheng et al. (39) with a minor modification. Briefly, Tris–HCl buffer (50 mM, pH 8.2), pyrogallol (25 mM), and WSPs (1–5 mg/mL) were mixed at 25°C for 5 min. The absorbance at 299 nm was measured to determine the superoxide anion scavenging ability using Eq. (3).
where A1 and A2 are the absorbance of the blank and specimen group, respectively.
Orthogonal test design of co-culture fermentation conditions for water-soluble polysaccharides production
In order to optimize the co-culture fermentation conditions for WSPs production, an orthogonal test design was conducted. Fermentation time (3, 4, 5, 6, and 7 days), fermentation temperature (28°C, 31°C, 34°C, 37°C, and 40°C), strain proportion [Photobacterium sp. LYM-1 (PsL): A. pullulans 2012 (AP), 1:3, 1:2, 1:1, 2:1, and 3:1], and the inoculum concentration (4, 6, 8, 10, and 12%) were all tested in single-factor experiment. The orthogonal experiments were carried out with four factors and three levels on the basis of fundamental single-factor tests as shown in Supplementary Table 2. The yield (%) of WSPs was the dependent variable.
Results and discussion
Screening and identification of strains
Strain ability to degrade chitin was determined by examining the size of the transparent circle on the colloidal chitin screening plate as shown in Figure 1. The one showing the largest transparent circle was selected as the experimental strain. The type of the strain was identified by 16S rRNA gene sequencing and further comparison in the NCBI database. In this study, the strain identified as Photobacterium sp. (named Photobacterium sp. LYM-1) was the best microorganism for utilizing shrimp shells (Figure 1A1). The phylogenetic tree in Figure 1B shows that the bacterium Photobacterium sp. LYM-1 is most closely related to Photobacterium sp. LAM9072. Photobacterium sp. LAM9072, a Gram-stain-negative, aerobic, motile, rod-shaped bacterium, was first identified to degrade sulfonylurea herbicides, a type of drug residue in soil (40).
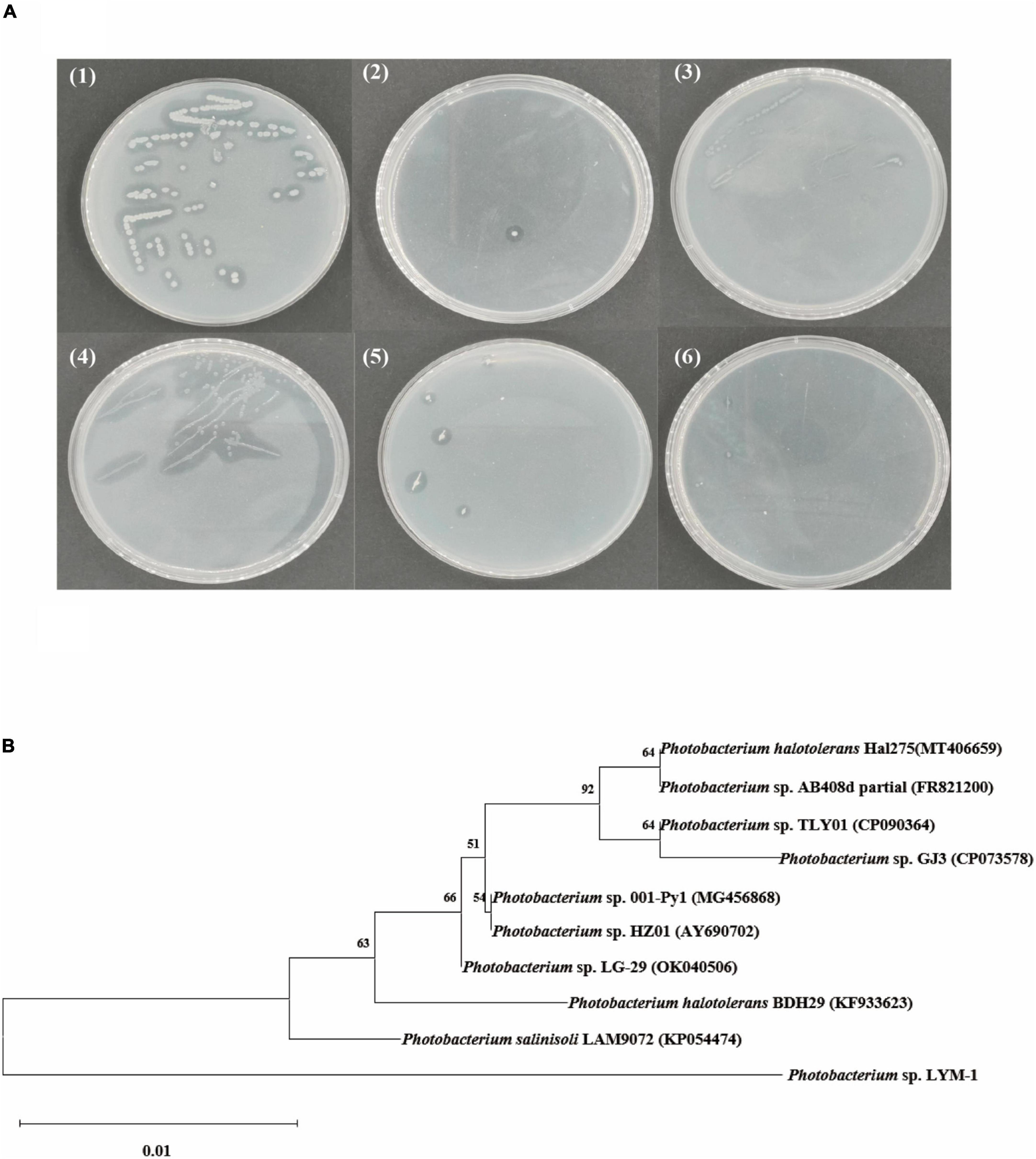
Figure 1. (A) Screening of strains: (1) Photobacterium sp. LYM-1, (2) Aeromonas sp. ZXY-3, (3) Vibrio sp. WM-1, (4) Rheinheimera sp. ZXY-2, (5) Shewanella sp. ZXY-1, and (6) Pseudomonas sp. LYM-2; (B) neighbor-joining tree based on 16S rRNA gene sequences showing the phylogenetic relationships between strain Photobacterium sp. LYM-1 and its related taxa.
Compatibility of Photobacterium sp. LYM-1 and Aureobasidium pullulans 2012
An antagonistic test between Photobacterium sp. LYM-1 and A. pullulans 2012 revealed that there was no antagonism between them (Figure 2). After 24 h, the two strains grew at a similar rate and no bacteriostatic zone was observed in either strain. After 48 h, these two strains could fuse with no discernible boundaries. This result demonstrated that these two strains could coexist and grow harmoniously. Co-culture fermentation of yeast and bacteria has been successfully applied to other products (41).
Chemical components of water-soluble polysaccharides
Both straw and shrimp shells are examples of agro-food wastes. Straw is rich in carbohydrates and shrimp shells contain carbohydrates, protein, and other nutrients. However, the carbohydrates (cellulose in straw and chitin in shrimp shells) are not water soluble, which limits their application. The WSPs production by the fermentation of these two materials could be viable, green, and high-value utilization strategy (22, 42). Monocultures and co-culture fermentations of Photobacterium sp. LYM-1 and A. pullulans 2012 for the production of WSPs were investigated in this study. Three types of WSPs (PsL-WSPs, AP-WSPs, and PsL/AP-WSPs) were obtained by fermentation broth collection, ethanol precipitation, deproteinization, and dialysis.
Table 1 summarizes the content of saccharides, uronic acid, protein, flavone, polyphenol, and ash of WSPs. Saccharides make up the majority of all three fractions, particularly the WSPs fraction from co-culture fermentation (64.88% of saccharides). A. pullulans is the type strain for the production of the polysaccharide, especially pullulan (20). Co-culture of Photobacterium sp. LYM-1 and A. pullulans 2012 could produce more WSPs, indicating that these two strains have a mutual promotion on the WSPs production, which is consistent with the compatibility test result. Cellulolytic enzyme production by co-cultured of Trichoderma reesei and Aspergillus niger in solid substrate fermentation using alkali-treated sugar cane bagasse as substrate was reported (43), demonstrating that co-culture of agro-food waste could be used for the preparation of high-value products. Co-culture fermentation of yeast and bacteria has also been used successfully in other applications (41). Uronic acid was found in all three WSPs fractions, with the highest concentration found in PsL/AP-WSPs, reaching 12.97%. Although proteins were detected in all three WSPs fractions, their content (1.31% in PsL/AP-WSPs, 0.82% in PsL-WSPs, and 0.50% in AP-WSPs) could be ignored in this study when compared to saccharides contents. AP-WSPs contained more flavonoids (14.81%) compared to the other two WSPs fractions. The polyphenol and ash contents in the three WSP fractions were very low and did not differ significantly.
Molecular weight of water-soluble polysaccharides
In general, the molecular weight of polysaccharides produced through fermentation is highly centralized. The molecular weight distributions of three WSPs fractions are shown in Figure 3. Only a distinct group peak was observed in PsL-WSPs and AP-WSPs fractions with a similar molecular weight of 257.6 and 227.6 kDa, respectively, quantified by molecular weight regression equation (log MW = 0.326t+9.202, R2 = 0.9947) showing in Supplementary Figure 1 (9). The characteristic peaks of PsL/AP-WSPs were adjacent and complex, with distinct differences from those of PsL-WSPs and AP-WSPs. The molecular weights of PsL/AP-WSPs were 414.9, 346.8, 144.6, and 50.9 kDa, which could be attributed to the synergistic effect of complex enzymes (e.g., cellulose, chitinase, and deacetylase) expressed by Photobacterium sp. LYM-1 and A. pullulans 2012. Similar phenomena were also reported in previous studies (26, 44).
Monosaccharide composition of water-soluble polysaccharides
The monosaccharide composition of three WSPs fractions was analyzed by HPLC. Monosaccharide analysis showed that they were heteropolysaccharides composed primarily of Man, Rib, GlcN, Glc, Gal, and Ara in different molar percentages (as shown in Supplementary Figure 2 and Table 2). Results revealed that PsL/AP-WSPs and PsL-WSPs comprised the same monosaccharides in different molar ratios. Gal (16.38%) was the major monosaccharide structure of PsL/AP-WSPs, while Glc (19.20%) and GlcN (14.52%) were the most common monosaccharides of PsL-WSPs, possibly due to chitin degradation by Photobacterium sp. LYM-1 to its monomer N-acetylglucosamine as the main carbon source instead of Glc. However, Rib was not detected in AP-WSPs, and the proportion of Rib in PsL/AP-WSPs was higher, owing to the benefit of co-culture fermentation (44).
Fourier transform infrared spectrometer analysis
Fourier transform infrared (FTIR) spectrometer result of AP-WSPs, PsL-WSPs, and PsL/AP-WSPs is shown in Figure 4. Spectra peaks at 3,430, 2,930, 1,650, 1,440, 1,050, and 610 cm–1 were the typical characteristic of polysaccharides (45). There is a strong and wide stretching peak around 3,430 cm–1, which is caused by the stretching vibration of hydroxyl groups, the basic FTIR peak of polysaccharides (35). The weak absorption peak around 2,930 cm–1 was assigned to the C-H stretching vibration of -CH3, -CH2, and -CH of polysaccharides. The band at close to 1,650 cm–1 was attributed to the asymmetric stretching vibration of the carbonyl group (46). C-H angular vibration caused the characteristic peak around 1,440 cm–1. The strong absorption peak at 1,000–1,200 cm–1 is dominated by stretching vibrations of ring structures, C-C, and deformation of -CH2 groups vibration characteristic of polysaccharide (47). The discussed characteristic FTIR spectra peaks demonstrated that AP-WSPs, PsL-WSPs, and PsL/AP-WSPs obtained from the monoculture and co-culture fermentation of straw and shrimp shells were polysaccharides.
Antioxidant activities of water-soluble polysaccharides
1,1-diphenyl-2-picrylhydrazyl radical scavenging ability
Excess free radicals in the human body can cause various adverse reactions, such as aging, obesity, and diabetes, whereas, polysaccharides can improve immune function, antioxidation, and exhibit low cellular toxicity (48). DPPH radical has been widely used as an indicator to estimate the free radical scavenging activities of antioxidants because of its high sensitivity, fast reaction, and stability (11). The three WSP fractions demonstrated remarkable DPPH radical scavenging activities, as shown in Figure 5A, which is consistent with previous research (49). PsL/AP-WSPs (∼94%) showed higher DPPH radicals scavenging activity than the other two fractions (∼90%) and the polysaccharides GPs1 (∼68%) from Glycyrrhiza uralensis at 4 mg/mL (50). The monosaccharide composition of the three WSPs fractions might cause differences in the glucosidic bonds, configurations, and sequence of monosaccharides, and have a major influence on their hydrogen-donating abilities (51). The PsL/AP-WSPs superior scavenging ability against the DPPH radical compared to the other two WSPs fractions might be attributed to its strong hydrogen-donating ability and uronic acid groups (52, 53).
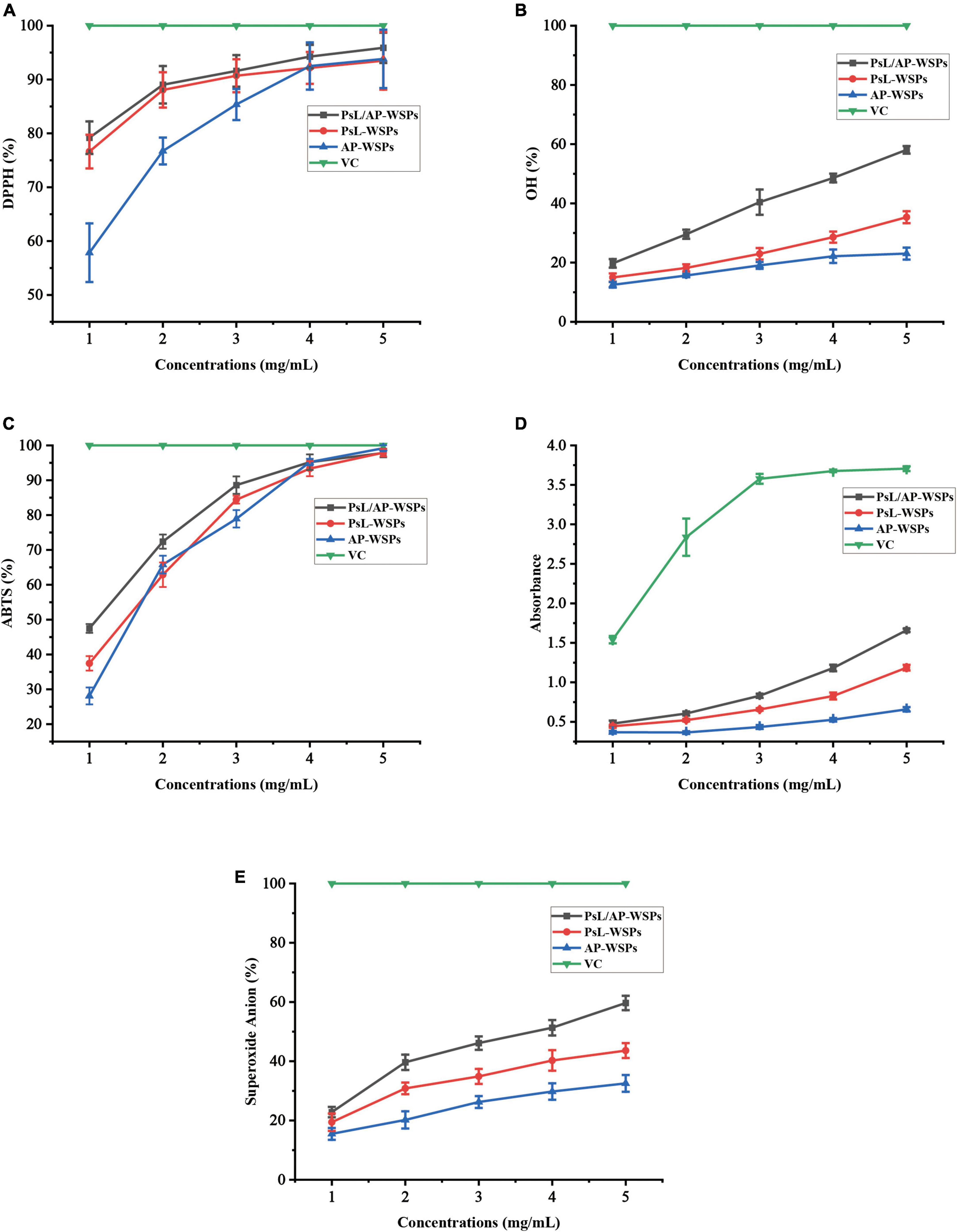
Figure 5. Evaluation of antioxidant activities of WSPs. (A) DPPH radical scavenging ability, (B) OH radical scavenging ability, (C) ABTS radical scavenging ability, (D) ferric-reducing antioxidant power, and (E) superoxide anion scavenging ability.
Hydroxyl radical scavenging ability
The ability of hydroxyl radical scavenging abilities of three WSPs fractions is shown in Figure 5B. Three WSPs fractions demonstrated a concentration-dependent scavenging ability against hydroxyl radicals within the determined dosage range. Furthermore, in each determination concentration, PsL/AP-WSPs demonstrated a greater ability to scavenge hydroxyl radicals than the other two WSP fractions. At the concentration of 2 mg/mL, the hydroxyl radical scavenging capacity of PsL/AP-WSPs (∼25%) was higher than that of GPN (24%) from G. glabra (54).
2,2-azinobis-(3-ethylbenzthiazoline-6-sulphonate) radical scavenging ability
2,2-azinobis-(3-ethylbenzthiazoline-6-sulphonate) scavenging experiment has been widely used to assess the antioxidant capacity of various natural products, including, polyphenols, flavonoids, and polysaccharides (55). As shown in Figure 5C, the ABTS radical scavenging activity of three WSPs fractions increased in a quadratic concentration-dependent pattern. At low concentrations (1–4 mg/mL), the ABTS radical scavenging abilities differed in the following order: PsL/AP-WSPs > PsL-WSPs > AP-WSPs. However, in each determination concentration, all three WSPs fractions exhibited higher ABTS radical ability than that of FWPS1-1 from Fritillaria unibracteata var. wabuensis (56). At a concentration of 5 mg/mL, the ABTS radical scavenging activities of the three WSPs showed a similar scavenging level of VC, indicating that WSPs possessed notable ABTS radical scavenging ability.
Ferric-reducing antioxidant power
Ferric-deducing power (FRAP) assay is widely used to estimate the antioxidant capacity of polysaccharides (57). In this study, all three WSPs showed apparent FRAP within the elevated concentration of WSPs, indicating that WSPs were electron donors and could quench the radical chain reactions (Figure 5D). In the determination concentration, the FRAP of three WSPs followed the order of PsL/AP-WSPs > PsL-WSPs > AP-WSPs.
Superoxide anion scavenging ability
Superoxide anion radicals have a lower reactivity and a longer lifetime than other free radicals (58). As a result, the scavenging effect of superoxide anion radicals is an essential experimental index for the determination of antioxidant activity (59). As shown in Figure 5E, all three WSPs fractions have superoxide anion radical scavenging activity, with increasing capacity proportional to WSPs concentrations. The capacity of the three WSPs fractions was as follows: PsL/AP-WSPs > PsL-WSPs > AP-WSPs, which showed a free radical scavenging trend similar to the other free radical reagents.
Optimization of water-soluble polysaccharides production by orthogonal design
Effect of fermentation time on polysaccharides yield
When the chemical components, molecular weights, monosaccharide composition, and antioxidant capacity of three WSP fractions were combined, PsL/AP-WSPs demonstrated the best characteristics. Co-cultured microorganisms could be beneficial because of the synergistic expression of the microorganisms’ metabolic pathways. The strategy for improving the production of PsL/AP-WSPs was conducted by the orthogonal experimental design. The single-factor analysis results are shown in Figure 6. The concentration of WSPs increased slowly and then fast from 1 to 5 days of the fermentation period and the highest concentration of WSPs was observed at 5 days (Figure 6A). Fewer nutrients (e.g., yeast extract and glucose) in the fermentation medium resulted in low WSPs production by these two organisms at an early period. As straw and shrimp shells were hydrolyzed by secreted enzymes (such as cellulases, chitinases, and proteases) for nutrients, they grew rapidly and produced extra polysaccharides, leading to the high polysaccharides concentration in the fermentation broth (20). According to the effect of fermentation time on the yield of WSPs, the factor levels of feeding time were chosen for 4, 5, and 6 days.
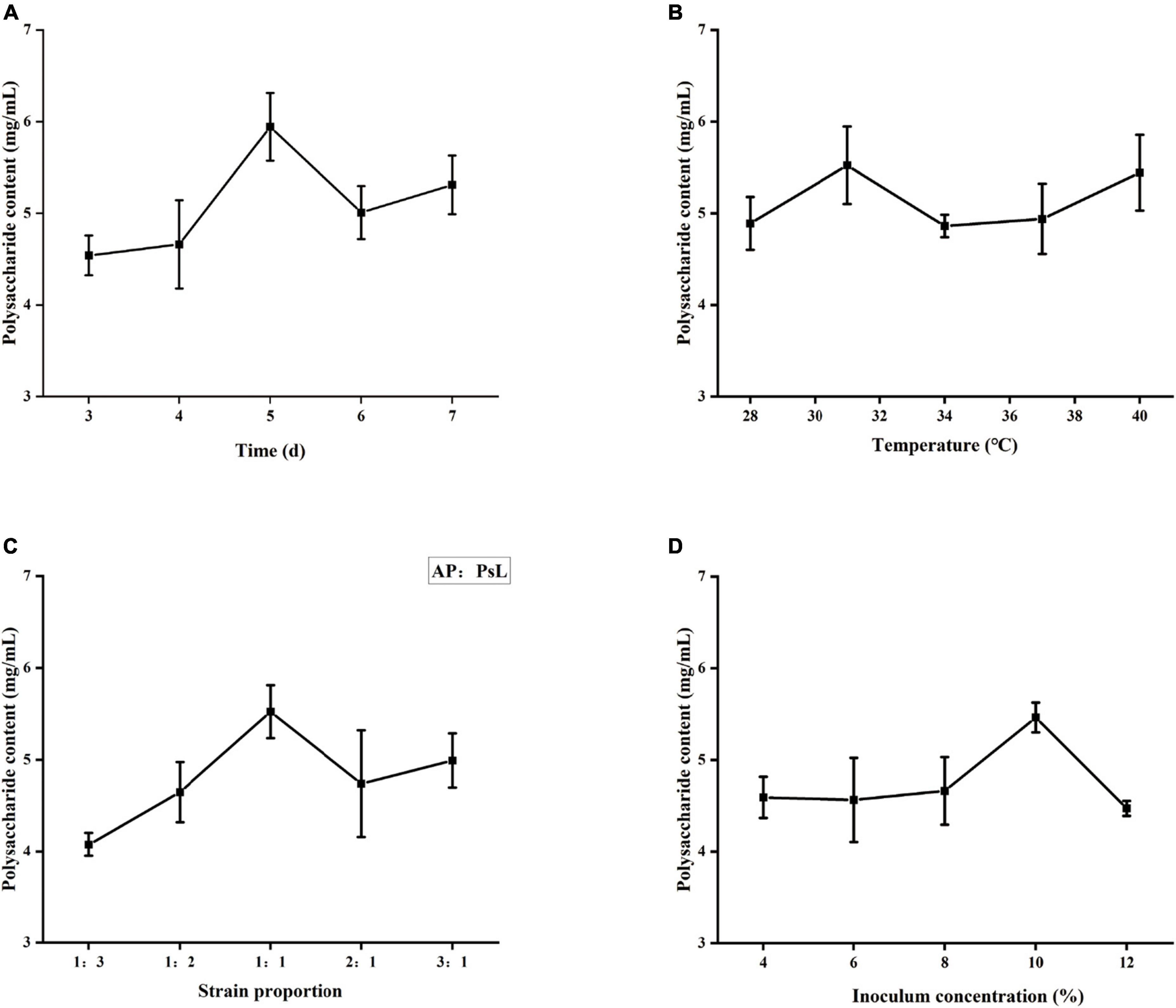
Figure 6. Effects of different factors on polysaccharide yield. (A) Fermentation time, (B) fermentation temperature, (C) strain proportion, and (D) inoculum concentration.
Effect of fermentation temperature on polysaccharide yield
Each organism requires a specific optimum temperature for reproduction. Extreme temperatures will affect enzyme activities and metabolism and eventually lead to death. As shown in Figure 6B, the concentration of WSPs in fermentation broth increased with temperature from 28 to 31°C and then decreased when the temperature was increased to 31°C. The optimum fermentation temperature for the production of PsL/AP-WSPs was 31°C. Our findings are consistent with the previous studies relating to the growth temperatures of Aureobasidium sp. and Photobacterium sp. (60, 61). According to the effect of temperature on the yield of WSPs, the factor levels for choosing the fermentation temperature were 28, 31, and 34°C.
Effect of strain proportion on polysaccharide yield
On account of several microbial interactions, the growth and the metabolite of monoculture differ from that of mixed culture (62). As shown in Figure 6C, the highest PsL/AP-WSPs concentration in the fermentation broth was observed when the equivalent strain proportion (1:1) of A. pullulans 2012 (AP) with Photobacterium sp. LYM-1 (PsL) was used. It can be explained as the phenomenon of mutual competition and interdependence among different strains (41). The microbial interactions can be antagonistic or synergistic, resulting in inhibited or enhanced proliferation, respectively. In this study, A. pullulans 2012 showed that synergistic interaction to Photobacterium sp. LYM-1 results in more extracellular polysaccharides in their equivalent proportion. Therefore, the factor levels for choosing the strain proportion were 1:2, 1:1, and 2:1.
Effect of inoculation amount on polysaccharide yield
As shown in Figure 6D, the PsL/AP-WSPs concentration increased in the elevated inoculation concentration of 4–10%, and then slightly decreased over 10% of the inoculation concentration. The hypothesis is that the increased concentration of cultures consumed more nutrients and resulted in insufficient dissolved oxygen, inhibiting culture growth and metabolism and resulting in less metabolites, including WSPs (63). According to the influence of inoculation amount on WSPs yield, the factor levels for choosing the inoculation amount were 8, 10, and 12%.
Orthogonal experimental results
The influences of the fermentation factors on the indicator were determined using range (R) analysis. According to the orthogonal experiment in Table 3, the influence of each factor on the polysaccharide content was in the following order: B > D > C > A. The fermentation temperature had the greatest impact on the polysaccharide yield, followed by inoculation amount, strain ratio, and time. According to the analysis, the combination A1B2C3D2 was optimal, which indicated that the optimum conditions for the production of PsL/AP-WSPs were 5 days, at 31°C, inoculation concentration of 10%, and 2:1 (AP: PsL) strains ratio. As the combination of A1B2C3D2 did not appear in the orthogonal test design, a verification test with three parallel experiments was carried out. The yield of polysaccharides was 5.88 ± 0.40 mg/mL, which was higher than other conditions. Therefore, it was confirmed that A1B2C3D2 provided the best fermentation conditions.
EPSs and their production from agro-industrial waste have been extensively researched (20, 64). Sugarcane bagasse hydrolysate and jackfruit seed powder were used for the production of pullulan by A. pullulans in shake-flask fermentations at 28°C for 4 days, with a yield of 25.19 and 18.76 g/L, respectively, which was higher than that from straw and shrimp shell (5.88 g/L) (65, 66). However, in the reported studies, almost all the fermentation media contained nutrients, such as yeast extract and peptone, except for agro-food wastes. Whereas, the fermentation media for mono- and co-cultures of Photobacterium sp. LYM-1 and A. pullulans 2012 only contained straw and shrimp shells for nutrients, which reduced the costs.
Conclusion
In this study, the strain Photobacterium sp. LYM-1, isolated from the mudflat containing shrimp shell, was identified to degrade chitin for the first time. Photobacterium sp. LYM-1 and A. pullulans 2012 are compatible and could be co-cultured using shrimp shells and straw as a substrate for the production of WSPs. Co-culture and monoculture fermentations of Photobacterium sp. LYM-1 and A. pullulans 2012 yielded three types of WSP fractions (PsL/AP-WSPs, PsL-WSPs, and AP-WSPs). All three WSPs fractions were heteropolysaccharides, primarily composed of mannose, ribose, glucose, glucosamine, galactose, and arabinose in varying molar ratios and exhibited significant antioxidant activity. However, PsL/AP-WSPs outperformed the other two WSPs fractions in terms of characteristics and antioxidant activity. The high antioxidant activity of PsL/AP-WSPs could be attributed to their compositional diversity and higher uronic acid content. The production conditions of PsL/AP-WSPs were optimized by orthogonal design and the optimum fermentation conditions were determined to be 5 days fermentation time, at 31°C, in a 2:1 AP to PsL ratio, and inoculation amount of 10%. The highest yield of PsL/AP-WSPs was 5.88 mg/mL under optimum fermentation conditions. In conclusion, this research demonstrated that preparing PsL/AP-WSPs from agro-food wastes using the co-culture fermentation of Photobacterium sp. LYM-1 and A. pullulans 2012 were feasible, economical, and environmentally friendly.
Data availability statement
The data presented in the study are deposited in: https://figshare.com/articles/dataset/Raw_Data_of_the_manuscript_1047932_zip/21563475. The 16S rRNA information is deposited in: https://www.ncbi.nlm.nih.gov/. GenBank accession numbers are: OP824621; OP824622; OP824623; OP824624; OP824625; and OP824626. Further inquiries can be directed to the corresponding author.
Author contributions
YL: conceptualization, resources, supervision, project administration, writing—review and editing, and funding acquisition. MWa: data curation, writing—original draft, and funding acquisition. YZ and XZ: data curation and software. XL: software and methodology. FL and DW: methodology and writing—original draft. MWe: methodology. XY: investigation, data curation, project administration, writing—review and editing, and funding acquisition. All authors contributed to the article and approved the submitted version.
Funding
This work was supported in parts by the Natural Science Research of Jiangsu Higher Education Institutions of China (Grant no. 19KJA480001), Postgraduate Research and Practice Innovation Program of Yancheng Institute of Technology (Grant no. KYCX21_XZ003), the Funding for School-level Research Projects of Yancheng Institute of Technology (Grant no. xjr2021027), and Open-end Funds of Jiangsu Key Laboratory of Marine Bioresources and Environment (Grant no. SH20211211).
Conflict of interest
The authors declare that the research was conducted in the absence of any commercial or financial relationships that could be construed as a potential conflict of interest.
Publisher’s note
All claims expressed in this article are solely those of the authors and do not necessarily represent those of their affiliated organizations, or those of the publisher, the editors and the reviewers. Any product that may be evaluated in this article, or claim that may be made by its manufacturer, is not guaranteed or endorsed by the publisher.
Supplementary material
The Supplementary Material for this article can be found online at: https://www.frontiersin.org/articles/10.3389/fnut.2022.1047932/full#supplementary-material
Abbreviations
WSPs, water-soluble polysaccharides; PsL/AP-WSPs, WSPs from co-culture fermentation of Photobacterium sp. LYM-1 and A. pullulans 2012; PsL-WSPs, WSPs from monoculture fermentation of Photobacterium sp. LYM-1; AP-WSPs, WSPs from monoculture fermentation of A. pullulans 2012; FTIR, Fourier transform infrared spectra; EPS, microbial exopolysaccharides; RID, refractive index detector; NCBI, National Center for Biotechnology Information; Gal, galactose; Man, mannose; Fuc, fucose; GlcN, glucosamine; Glc, glucose; GalA, galacturonic acid; Rha, rhamnose; Ara, arabinose; FRAP, ferric-reducing antioxidant power; TFA, trifluoroacetic acid; PMP, 1-phenyl-3-methyl-5-pyrazolone; DPPH, 1,1-diphenyl-2-picrylhydrazyl; ABTS, 2,2-azinobis-(3-ethylbenzthiazoline-6-sulphonate); SmF, submerged fermentation; SSF, solid-state fermentation; HW, hot-water extraction; UA, ultrasonic-assisted extraction; ME, microwave-assisted extraction; EA, enzyme-assisted extraction; EUA, enzyme-ultrasonic assistance extraction; HPLC, high-performance liquid chromatography.
Footnotes
References
1. Zhang R, Gao J, Shi Y, Lan Y, Liu H, Zhu W, et al. Characterization of structure and antioxidant activity of polysaccharides from sesame seed hull. Front Nutr. (2022) 9:928972. doi: 10.3389/fnut.2022.928972
2. Mzoughi Z, Abdelhamid A, Rihouey C, Le Cerf D, Bouraoui A, Majdoub H. Optimized extraction of pectin-like polysaccharide from Suaeda fruticosa leaves: characterization, antioxidant, anti-inflammatory and analgesic activities. Carbohydr Polym. (2018) 185:127–37. doi: 10.1016/j.carbpol.2018.01.022
3. Yuan D, Li C, Huang Q, Fu X, Dong H. Current advances in the anti-inflammatory effects and mechanisms of natural polysaccharides. Crit Rev Food Sci Nutr. (2022) 1–21. doi: 10.1080/10408398.2022.2025535
4. Wang K, Cai M, Sun S, Cheng W, Zhai D, Ni Z, et al. Therapeutic prospects of polysaccharides for ovarian cancer. Front Nutr. (2022) 9:879111. doi: 10.3389/fnut.2022.879111
5. Friedman M. Mushroom polysaccharides: chemistry and antiobesity, antidiabetes, anticancer, and antibiotic properties in cells, rodents, and humans. Foods. (2016) 5:80. doi: 10.3390/foods5040080
6. Wang B, Niu J, Mai B, Shi F, Li M, Chen L, et al. Effects of extraction methods on antioxidant and immunomodulatory activities of polysaccharides from superfine powder Gynostemma pentaphyllum Makino. Glycoconj J. (2020) 37:777–89. doi: 10.1007/s10719-020-09949-5
7. Mutailifu P, Nuerxiati R, Lu C, Huojiaaihemaiti H, Abuduwaili A, Yili A. Extraction, purification, and characterization of polysaccharides from Alhagi pseudoalhagi with antioxidant and hypoglycemic activities. Process Biochem. (2022) 121:339–48. doi: 10.1016/j.procbio.2022.06.026
8. Chen D, Chen G, Chen C, Zeng X, Ye H. Prebiotics effects in vitro of polysaccharides from tea flowers on gut microbiota of healthy persons and patients with inflammatory bowel disease. Int J Biol Macromol. (2020) 158:968–76. doi: 10.1016/j.ijbiomac.2020.04.248
9. Zhang X, Kong X, Hao Y, Zhang X, Zhu Z. Chemical structure and inhibition on α-glucosidase of polysaccharide with alkaline-extracted from Glycyrrhiza inflata residue. Int J Biol Macromol. (2020) 147:1125–35. doi: 10.1016/j.ijbiomac.2019.10.081
10. Cheng Z, Song H, Yang Y, Liu Y, Liu Z, Hu H, et al. Optimization of microwave-assisted enzymatic extraction of polysaccharides from the fruit of Schisandra chinensis Baill. Int J Biol Macromol. (2015) 76:161–8. doi: 10.1016/j.ijbiomac.2015.01.048
11. Chen S, Shang H, Yang J, Li R, Wu H. Effects of different extraction techniques on physicochemical properties and activities of polysaccharides from comfrey (Symphytum officinale L.) root. Ind Crops Prod. (2018) 121:18–25. doi: 10.1016/j.indcrop.2018.04.063
12. Yi Y, Xu W, Wang H, Huang F, Wang L. Natural polysaccharides experience physiochemical and functional changes during preparation: a review. Carbohydr Polym. (2020) 234:115896. doi: 10.1016/j.carbpol.2020.115896
13. NithyaBalaSundari S, Nivedita V, Chakravarthy M, Srisowmeya G, Antony U, Nandhini Dev G. Characterization of microbial polysaccharides and prebiotic enrichment of wheat bread with pullulan. LWT. (2020) 122:109002. doi: 10.1016/j.lwt.2019.109002
14. Singh RS, Kaur N, Kennedy JF. Pullulan production from agro-industrial waste and its applications in food industry: a review. Carbohydr Polym. (2019) 217:46–57. doi: 10.1016/j.carbpol.2019.04.050
15. Muninathan C, Guruchandran S, Viswanath Kalyan AJ, Ganesan ND. Microbial exopolysaccharides: role in functional food engineering and gut-health management. Int J Food Sci Technol. (2022) 57:27–34. doi: 10.1111/ijfs.15334
16. Hamidi M, Kennedy JF, Khodaiyan F, Mousavi Z, Hosseini SS. Production optimization, characterization and gene expression of pullulan from a new strain of Aureobasidium pullulans. Int J Biol Macromol. (2019) 138:725–35. doi: 10.1016/j.ijbiomac.2019.07.123
17. Haghighatpanah N, Mirzaee H, Khodaiyan F, Kennedy JF, Aghakhani A, Hosseini SS, et al. Optimization and characterization of pullulan produced by a newly identified strain of Aureobasidium pullulans. Int J Biol Macromol. (2020) 152:305–13. doi: 10.1016/j.ijbiomac.2020.02.226
18. Schmid J. Recent insights in microbial exopolysaccharide biosynthesis and engineering strategies. Curr Opin Biotechnol. (2018) 53:130–6. doi: 10.1016/j.copbio.2018.01.005
19. He C, Zhang Z, Zhang Y, Wang G, Wang C, Wang D, et al. Efficient pullulan production by Aureobasidium pullulans using cost-effective substrates. Int J Biol Macromol. (2021) 186:544–53. doi: 10.1016/j.ijbiomac.2021.07.068
20. Singh RS, Kaur N. Understanding response surface optimization of medium composition for pullulan production from de-oiled rice bran by Aureobasidium pullulans. Food Sci Biotechnol. (2019) 28:1507–20. doi: 10.1007/s10068-019-00585-w
21. Wang D, Ju X, Zhou D, Wei G. Efficient production of pullulan using rice hull hydrolysate by adaptive laboratory evolution of Aureobasidium pullulans. Bioresour Technol. (2014) 164:12–9. doi: 10.1016/j.biortech.2014.04.036
22. Han YW, Cheeke PR, Anderson AW, Lekprayoon C. Growth of Aureobasidium pullulans on straw hydrolysate. Appl Environ Microbiol. (1976) 32:799–802. doi: 10.1128/aem.32.6.799-802.1976
23. Sini TK, Santhosh S, Mathew PT. Study on the production of chitin and chitosan from shrimp shell by using Bacillus subtilis fermentation. Carbohydr Res. (2007) 342:2423–9. doi: 10.1016/j.carres.2007.06.028
24. Taser B, Ozkan H, Adiguzel A, Orak T, Baltaci MO, Taskin M. Preparation of chitosan from waste shrimp shells fermented with Paenibacillus jamilae BAT1. Int J Biol Macromol. (2021) 183:1191–9. doi: 10.1016/j.ijbiomac.2021.05.062
25. Setiawan A, Widyastuti W, Irawan A, Wijaya OS, Laila A, Setiawan WA, et al. Solid state fermentation of shrimp shell waste using Pseudonocardia carboxydivorans 18A13O1 to produce bioactive metabolites. Fermentation. (2021) 7:247. doi: 10.3390/fermentation7040247
26. Prasad AA, Babu S. Compatibility of Azospirillum brasilense and Pseudomonas fluorescens in growth promotion of groundnut (Arachis hypogea L.). An Acad Bras Ciênc. (2017) 89:1027–40. doi: 10.1590/0001-3765201720160617
27. Xu J, Yuan T, Xiao L, Sun R. Effect of ultrasonic time on the structural and physico-chemical properties of hemicelluloses from Eucalyptus grandis. Carbohydr Polym. (2018) 195:114–9. doi: 10.1016/j.carbpol.2018.04.067
28. DuBois M, Gilles KA, Hamilton JK, Rebers PA, Smith F. Colorimetric method for determination of sugars and related substances. Anal Chem. (1956) 28:350–6. doi: 10.1021/ac60111a017
29. Shi X, Huang J, Wang S, Yin J, Zhang F. Polysaccharides from Pachyrhizus erosus roots: extraction optimization and functional properties. Food Chem. (2022) 382:132413. doi: 10.1016/j.foodchem.2022.132413
30. Bradford MM. A rapid and sensitive method for the quantitation of microgram quantities of protein utilizing the principle of protein-dye binding. Anal Biochem. (1976) 72:248–54. doi: 10.1016/0003-2697(76)90527-3
31. Banik R, Santhiagu A, Upadhyay S. Optimization of nutrients for gellan gum production by Sphingomonas paucimobilis ATCC-31461 in molasses based medium using response surface methodology. Bioresour Technol. (2007) 98:792–7. doi: 10.1016/j.biortech.2006.03.012
32. Maina S, Ryu DH, Bakari G, Misinzo G, Nho CW, Kim H. Variation in phenolic compounds and antioxidant activity of various organs of african cabbage (Cleome gynandra L.) accessions at different growth stages. Antioxidants. (2021) 10:1952. doi: 10.3390/antiox10121952
33. Blumenkrantz N, Asboe-Hansen G. New method for quantitative determination of uronic acids. Anal Biochem. (1973) 54:484–9. doi: 10.1016/0003-2697(73)90377-1
34. Ye Z, Wang W, Yuan Q, Ye H, Sun Y, Zhang H, et al. Box–Behnken design for extraction optimization, characterization and in vitro antioxidant activity of Cicer arietinum L. hull polysaccharides. Carbohydr Polym. (2016) 147:354–64. doi: 10.1016/j.carbpol.2016.03.092
35. Wang F, Ye S, Ding Y, Ma Z, Zhao Q, Zang M, et al. Research on structure and antioxidant activity of polysaccharides from Ginkgo biloba leaves. J Mol Struct. (2022) 1252:132185. doi: 10.1016/j.molstruc.2021.132185
36. Meng Q, Chen Z, Chen F, Zhang Z, Gao W. Optimization of ultrasonic−assisted extraction of polysaccharides from Hemerocallis citrina and the antioxidant activity study. J Food Sci. (2021) 86:3082–96. doi: 10.1111/1750-3841.15806
37. Li Q, Yu N, Wang Y, Sun Y, Lu K, Guan W. Extraction optimization of Bruguiera gymnorrhiza polysaccharides with radical scavenging activities. Carbohydr Polym. (2013) 96:148–55. doi: 10.1016/j.carbpol.2013.03.054
38. Kan Y, Chen T, Wu Y, Wu J, Wu J. Antioxidant activity of polysaccharide extracted from Ganoderma lucidum using response surface methodology. Int J Biol Macromol. (2015) 72:151–7. doi: 10.1016/j.ijbiomac.2014.07.056
39. Cheng Z, Zhang Y, Song H, Zhou H, Zhong F, Hu H, et al. Extraction optimization, characterization and antioxidant activity of polysaccharide from Gentiana scabra bge. Int J Biol Macromol. (2016) 93:369–80. doi: 10.1016/j.ijbiomac.2016.08.059
40. Li M, Kong D, Wang Y, Ma Q, Han X, Zhou Y, et al. Photobacterium salinisoli sp. nov., isolated from a sulfonylurea herbicide-degrading consortium enriched with saline soil. Int J Syst Evol Microbiol. (2019) 69:3910–6. doi: 10.1099/ijsem.0.003705
41. Karim A, Islam MA, Mishra P, Muzahid AJM, Yousuf A, Khan MDMR, et al. Yeast and bacteria co-culture-based lipid production through bioremediation of palm oil mill effluent: a statistical optimization. Biomass Convers Biorefinery. (2021) 1–12. doi: 10.1007/s13399-021-01275-6
42. Wang S, Chang T, Liang T. Conversion and degradation of shellfish wastes by Serratia sp. TKU016 fermentation for the production of enzymes and bioactive materials. Biodegradation. (2010) 21:321–33. doi: 10.1007/s10532-009-9303-x
43. Gutierrez-Correa M, Portal L, Moreno P, Tengerdy RP. Mixed culture solid substrate fermentation of Trichoderma reesei with Aspergillus niger on sugar cane bagasse. Bioresour Technol. (1999) 68:173–8. doi: 10.1016/S0960-8524(98)00139-4
44. Prabhu G, Bhat D, Bhat RM, Selvaraj S. A critical look at bioproducts co-cultured under solid state fermentation and their challenges and industrial applications. Waste Biomass Valorization. (2022) 13:3095–111. doi: 10.1007/s12649-022-01721-0
45. Qu Y, Li C, Zhang C, Zeng R, Fu C. Optimization of infrared-assisted extraction of Bletilla striata polysaccharides based on response surface methodology and their antioxidant activities. Carbohydr Polym. (2016) 148:345–53. doi: 10.1016/j.carbpol.2016.04.081
46. Wang X, Zhang Z, Yao Q, Zhao M, Qi H. Phosphorylation of low-molecular-weight polysaccharide from Enteromorpha linza with antioxidant activity. Carbohydr Polym. (2013) 96:371–5. doi: 10.1016/j.carbpol.2013.04.029
47. Zhao Y, Wang J, Wu Z, Yang J, Li W, Shen L. Extraction, purification and anti-proliferative activities of polysaccharides from Lentinus edodes. Int J Biol Macromol. (2016) 93:136–44. doi: 10.1016/j.ijbiomac.2016.05.100
48. Wassie T, Niu K, Xie C, Wang H, Xin W. Extraction techniques, biological activities and health benefits of marine algae Enteromorpha prolifera polysaccharide. Front Nutr. (2021) 8:747928. doi: 10.3389/fnut.2021.747928
49. Jana S, Mukherjee S, Ali I, Ray B, Ray S. Isolation, structural features, in vitro antioxidant activity and assessment of complexation ability with β-lactoglobulin of a polysaccharide from Borassus flabellifer fruit. Heliyon. (2020) 6:e05499. doi: 10.1016/j.heliyon.2020.e05499
50. Wang Y, Li Y, Ma X, Ren H, Fan W, Leng F, et al. Extraction, purification, and bioactivities analyses of polysaccharides from Glycyrrhiza uralensis. Ind Crops Prod. (2018) 122:596–608. doi: 10.1016/j.indcrop.2018.06.011
51. Shen S, Cheng H, Li X, Li T, Yuan M, Zhou Y, et al. Effects of extraction methods on antioxidant activities of polysaccharides from camellia seed cake. Eur Food Res Technol. (2014) 238:1015–21. doi: 10.1007/s00217-014-2183-2
52. Shang X, Liu C, Dong H, Peng H, Zhu Z. Extraction, purification, structural characterization, and antioxidant activity of polysaccharides from wheat bran. J Mol Struct. (2021) 1233:130096. doi: 10.1016/j.molstruc.2021.130096
53. Wang J, Guo H, Zhang J, Wang X, Zhao B, Yao J, et al. Sulfated modification, characterization and structure–antioxidant relationships of Artemisia sphaerocephala polysaccharides. Carbohydr Polym. (2010) 81:897–905. doi: 10.1016/j.carbpol.2010.04.002
54. Mutaillifu P, Bobakulov K, Abuduwaili A, Huojiaaihemaiti H, Nuerxiati R, Aisa HA, et al. Structural characterization and antioxidant activities of a water soluble polysaccharide isolated from Glycyrrhiza glabra. Int J Biol Macromol. (2020) 144:751–9. doi: 10.1016/j.ijbiomac.2019.11.245
55. Zheng Q, Li X, Liu T, Zhang Y, Liu J, Zhang H, et al. Effects of air-impingement jet drying on drying kinetics, color, polyphenol compounds, and antioxidant activities of Boletus aereus slices. J Food Sci. (2021) 86:2131–44. doi: 10.1111/1750-3841.15702
56. Pan F, Su T, Liu Y, Hou K, Chen C, Wu W. Extraction, purification and antioxidation of a polysaccharide from Fritillaria unibracteata var. wabuensis. Int J Biol Macromol. (2018) 112:1073–83. doi: 10.1016/j.ijbiomac.2018.02.070
57. Xu W, Zhang F, Luo Y, Ma L, Kou X, Huang K. Antioxidant activity of a water-soluble polysaccharide purified from Pteridium aquilinum. Carbohydr Res. (2009) 344:217–22. doi: 10.1016/j.carres.2008.10.021
58. Wu Y, Huo Y, Xu L, Xu Y, Wang X, Zhou T. Purification, characterization and antioxidant activity of polysaccharides from Porphyra haitanensis. Int J Biol Macromol. (2020) 165:2116–25. doi: 10.1016/j.ijbiomac.2020.10.053
59. Chen Y, Xue Y. Purification, chemical characterization and antioxidant activities of a novel polysaccharide from Auricularia polytricha. Int J Biol Macromol. (2018) 120:1087–92. doi: 10.1016/j.ijbiomac.2018.08.160
60. Seo HP, Son CW, Chung CH, Jung DI, Kim SK, Gross RA, et al. Production of high molecular weight pullulan by Aureobasidium pullulans HP-2001 with soybean pomace as a nitrogen source. Bioresour Technol. (2004) 95:293–9. doi: 10.1016/j.biortech.2003.02.001
61. Rivas R, García-Fraile P, Mateos PF, Martínez-Molina E, Velázquez E. Photobacterium halotolerans sp. nov., isolated from Lake Martel in Spain. Int J Syst Evol Microbiol. (2006) 56:1067–71. doi: 10.1099/ijs.0.64099-0
62. Pin C, Baranyi J. Predictive models as means to quantify the interactions of spoilage organisms. Int J Food Microbiol. (1998) 41:59–72. doi: 10.1016/S0168-1605(98)00035-X
63. Pereira ALF, Maciel TC, Rodrigues S. Probiotic beverage from cashew apple juice fermented with Lactobacillus casei. Food Res Int. (2011) 44:1276–83. doi: 10.1016/j.foodres.2010.11.035
64. Sugumaran KR, Ponnusami V. Conventional optimization of aqueous extraction of pullulan in solid-state fermentation of cassava bagasse and Asian palm kernel. Biocatal Agric Biotechnol. (2017) 10:204–8. doi: 10.1016/j.bcab.2017.03.010
65. Terán Hilares R, Resende J, Orsi CA, Ahmed MA, Lacerda TM, Da Silva SS, et al. Exopolysaccharide (pullulan) production from sugarcane bagasse hydrolysate aiming to favor the development of biorefineries. Int J Biol Macromol. (2019) 127:169–77. doi: 10.1016/j.ijbiomac.2019.01.038
Keywords: polysaccharide, straw, shrimp shell, co-culture fermentation, antioxidation
Citation: Lyu Y, Wang M, Zhang Y, Zhang X, Liu X, Li F, Wang D, Wei M and Yu X (2022) Antioxidant properties of water-soluble polysaccharides prepared by co-culture fermentation of straw and shrimp shell. Front. Nutr. 9:1047932. doi: 10.3389/fnut.2022.1047932
Received: 19 September 2022; Accepted: 17 October 2022;
Published: 21 November 2022.
Edited by:
Faiza Rasheed, Quaid-i-Azam University, PakistanReviewed by:
Yongshuai Jing, Hebei University of Science and Technology, ChinaWang Xuede, Henan University of Technology, China
Copyright © 2022 Lyu, Wang, Zhang, Zhang, Liu, Li, Wang, Wei and Yu. This is an open-access article distributed under the terms of the Creative Commons Attribution License (CC BY). The use, distribution or reproduction in other forums is permitted, provided the original author(s) and the copyright owner(s) are credited and that the original publication in this journal is cited, in accordance with accepted academic practice. No use, distribution or reproduction is permitted which does not comply with these terms.
*Correspondence: Xiaohong Yu, eXhoMTEyN0B5Y2l0LmVkdS5jbg==
†These authors have contributed equally to this work